Differential analysis of fish meal substitution with two soybean meals on juvenile pearl gentian grouper
- 1Laboratory of Aquatic Animal Nutrition and Feed, College of Fisheries, Guangdong Ocean University, Zhanjiang, Guangdong, China
- 2Aquatic Animals Precision Nutrition and High Efficiency Feed Engineering Research Center of Guangdong Province, Zhanjiang, Guangdong, China
- 3Key Laboratory of Aquatic, Livestock and Poultry Feed Science and Technology in South China, Ministry of Agriculture, Zhanjiang, Guangdong, China
- 4Guangdong Evergreen Feed Industry Co., Ltd, Zhangjiang, Guangdong, China
Fermented soybean meal and soybean protein concentrate are products of soybean that have been processed physically or biologically, and their use as an alternative to fish meal results in a significant reduction in the effects of anti-nutritional factors (ANFs) in soybean on aquatic species. Replacing fish meal with soybean protein concentrate and fermented soybean meal can meet the high protein requirements of carnivorous fish while effectively reducing aquaculture costs; however, excessive substitution can also cause economic losses. In this study, we used transcriptome sequencing to investigate the impacts of fermented soybean meal and soybean protein concentrate on the growth and physiology of pearl gentian grouper (Epinephelus fuscoguttatus ♀ × Epinephelus lanceolatus ♂) juveniles and to examine the mechanisms by which fermented soybean meal and soybean protein concentrate impair the intestinal condition of fish. Originally weighed 12.55 ± 0.06 g, the selected pearl gentian groupers were categorised into three treatment groups: one group was fed fish meal-based diets (FM, control group), one group was fed fish meal- and soybean protein concentrate-based diets (SPC40) and one group was fed fish meal- and fermented soybean meal-based diets (FSBM40), with the same crude protein and crude fat content in all three diets. The experiment was conducted for 10 weeks. The growth results showed that both the fermented soybean meal and soybean protein concentrate diets significantly inhibited the growth of the fish. Based on the results of enzyme activity, substance content and gene expression levels associated with intestinal damage and intestinal inflammation, it is highly likely that the fermented soybean meal and soybean protein concentrate diets affected the intestinal health of the fish and triggered intestinal inflammation. This study provides a theoretical basis to further explore the mechanism of soybean-initiated intestinal problems in fish.
1 Introduction
Many aquaculture species are carnivorous, and their traditional feeds require high levels of fish meal and fish oil. The booming aquaculture fuelled the market demand for fish meal, thus causing unbalanced growth between fishing for feed and food, decreasing the productive potential of fisheries and accelerating the depletion of traditional fisheries. Therefore, it is detrimental to the future growth of aquaculture (Zhang et al., 2020). Replacing fish meal with plant-based ingredients in aquaculture is feasible. Soybean meal is a common plant-based feed ingredient in aquatic feeds. However, it contains saponins, lectins, soybean antigenic proteins, trypsin inhibitors and other anti-nutritional factors (ANFs) that have been shown to adversely affect the digestion and absorption of some aquatic species (Sørensen et al., 2011). Soybean protein concentrate extraction mainly adopts the alcohol process, and its protein content is further increased and ANFs are substantially reduced (Yang et al., 2014). The level of ANFs in fermented soybean meal produced by microbial fermentation of soybean meal is also significantly reduced compared to soybean meal. During the fermentation process of soybean meal, soybean proteins can be broken down into smaller peptide proteins that are more easily digested and absorbed (Mugwanya et al., 2022). These products obtained by processing soybean meal through different processes have been used as alternative protein sources to fish meal in the culture of a variety of aquatic fish species.
Replacing fish meal with soybean meal and its processing products can essentially meet the nutritional requirements of most aquatic species’ diets (Król et al., 2016; Uczay et al., 2019). However, fish farmed with high levels of soybean meal instead of fish meal often suffer from reduced growth performance, weakened immunity, disruption of body metabolism, and other adverse reactions during the breeding process. The intestinal mucosal barrier of fish is found to be damaged and intestinal health is affected, leading to intestinal inflammation (Rumsey et al., 1994; Urán et al., 2008). Studies have shown that replacing fish meal with soybean meal often causes soybean meal-induced enteritis (SBMIE) in carnivorous fish. SBMIE is a non-infectious subacute enteritis that triggers shortening of fish distal intestinal mucosal folds, widening of the lamina propria, reduction of absorptive cell vacuoles and inflammatory cell infiltration. This will greatly affect the growth and survival of fish and reduce production efficiency (van den Ingh et al., 1991). Numerous studies have shown that replacing fish meal with excessive amounts of soybean protein concentrate or fermented soybean meal can also affect the growth performance of fish by disrupting their intestinal structure and promoting an inflammatory response (Kissil et al., 2000; Deng et al., 2006; Shiu et al., 2015; He et al., 2020). The intestinal inflammation caused by soybean processing products following this substantial reduction in ANFs requires further study.
The intestine is the main digestive and absorption organ of fish, and the intestinal mucosa struggles in the front line against pathogens. An intact intestinal mucosal epithelial structure and sound intestinal function are particularly important for the intestinal health of fish (Brandl et al., 2017). Feeding excessive plant-based ingredients, such as soybean meal, can damage the fish’s intestinal tract and also affect its intestinal permeability, allowing pathogens to invade (Hu et al., 2016). Moreover, the importance of the vast microbial communities in the intestines to maintain the integrity of the intestinal structure and function cannot be ignored, and harmful microbial infections resulting from an imbalance in the intestinal flora trigger the host to perform an inflammatory response (Nayak, 2010). The causes and specific mechanisms of intestinal inflammation in fish caused by the replacement of fish meal with plant-based raw materials, such as soybean meal, are still unclear, and past studies have tended to focus on the effects of ANFs in soybean meal on fish intestinal (Krogdahl et al., 2010). Fermented soybean meal and soybean protein concentrate contain fewer ANFs, and relatively little research has been done on their effects on fish intestinal health.
Pearl gentian grouper (Epinephelus fuscoguttatus ♀ × E. lanceolatus ♂) is an excellent carnivorous marine hybrid fish that combines the excellent quality of both parents. With fast growth and strong disease resistance, the pearl gentian grouper has gradually become the largest grouper species cultured in China (Zhang et al., 2021). Research concerning the epistasis of pearl gentian grouper at the transcriptional level is relatively scarce and needs to be further improved. Since the advancement of the high-throughput sequencing technique, transcriptome sequencing has been commonly used in gene expression regulation. Comparative transcriptomics is now common in studying various aquatic species, which includes various aspects such as growth and development, toxicology and immune response (Chen et al., 2020). In this study, we fed pearl gentian grouper with soybean protein concentrate or fermented soybean meal in place of 40% fish meal, aiming to compare the differences in the distal intestine of fishes eating these two soybean processing products at the transcriptome level, analyse the mechanisms that induce enteritis and determine the impact of the highly replaced fish meal with these two soybean processing products on the growth and physiology of pearl gentian grouper.
2 Materials and methods
2.1 Experimental diets
There were three experimental diets, among which the control feed (FM) was mainly made of fish meal, while soybean protein concentrate (SPC40) or fermented soybean meal (FSBM40) was employed to substitute 40% fish meal protein in the remaining two groups, respectively. The contents of crude protein and crude fat in the three diets were the same. SPC40 and FSBM40 groups were supplemented with appropriate amounts of lysine and methionine to maintain equal lysine and methionine contents in the three diets. Table 1 shows the details of the diet formula and the approximate ingredients. Accurate weighing of raw materials that have been ground into fine powder according to the formulation and then mixed uniformly using a stepwise expansion method. Then, the weighed fine powder, fish oil, soybean oil, soy lecithin and an appropriate amount of water were mixed sequentially according to the formulation before being granulated by the twin-screw extruder. The granules with diameters of 2.0 mm and 3.0 mm were put at −20°C for later experiments after being air-dried to about 10% moisture.
2.2 Feeding management
The pearl gentian grouper juveniles used in the study were purchased from Zhanjiang, Guangdong, China, and the culture experiments were conducted in an indoor culture system at the Zhanjiang Marine Biological Research Base, China. Seven hundred and twenty fish weighing 12.55 ± 0.06 g were equally and randomly divided into 12 tanks (three treatment groups, four biological replicates per treatment group). The tank has a capacity of one cubic meter (stocking density of 60 fish per cubic meter of water) and is equipped with oxygenation equipment to prevent dissolved oxygen levels from falling below 7 mg L−1. The fish were fed commercial feeds containing ≥520 g/kg of crude protein and ≥130 g/kg of crude fat content for one week before the culture experiment. During the culture process, satiety feeding was provided daily at 8:00 and 16:00 with a water temperature of 29 ± 1°C.
2.3 Sample collection
After the culture was carried out for 10 weeks and feeding was stopped for one day, the researchers counted and weighed the surviving fish in each of the 12 tanks to calculate the weight gain rate(WGR), specific growth rate (SGR), feed conversion ratio (FCR), and survival rate (SR). After weighing the fish, we anaesthetised it with eugenol (1:10,000), dissected and removed the distal intestine and immediately stored it in liquid nitrogen. The distal intestine was stored at −80°C for subsequent experiments.
The WGR, SGR, FCR and SR were calculated by the following formulas:
2.4 Biochemical analysis
To determine the activities of trypsin, total superoxide dismutase (T-SOD), glutathione peroxidase (GSH-Px) and the concentration of complement 3 (C3), complement 4 (C4) and immunoglobulin M (IgM) in the distal intestine of fish, the researchers used the fish ELISA kit manufactured by Shanghai Jiang Lai Biotechnology Co., Ltd (Shanghai, China). The experiment was in strict accordance with the instructions. The protein content of the distal intestine was measured using the BCA method (Beyotime Biotechnology Co., Ltd., Shanghai, China) to calculate the relevant indicators.
2.5 Transcriptome sequencing and analysis
Oligo (dT) magnetic beads were employed to enrich the mRNA was enriched, and SMARTer PCR cDNA Synthesis Kit (Clontech, Japan) was used to synthesise the cDNA via reverse transcription amplification. cDNA fragments ≥4 kb were enriched by BluePippin screening. The full-length cDNA was ligated by SMRT dumbbell-shaped connector repair. The complete SMRT bell library was checked and sequenced using the Pacific Biosciences Sequel platform. Seven databases were used to annotate the full-length transcript: Gene Ontology, Kyoto Encyclopaedia of Genes and Genomes Orthology database, Swiss-Prot, Clusters of Orthologous Groups of proteins, Protein family, NCBI non-redundant nucleotide sequences and NCBI non-redundant protein sequences. In addition, four software were employed, including Diamond BLASTX, BLAST, Hmmscan and Blast2GO. Based on the thresholds of |Log2FC| > 1 and P< 0.05, the researchers carried out the screening of differentially expressed genes (DEGs).
2.6 RNA extraction and real-time quantitative PCR
Trizol (Invitrogen, Carlsbad, CA, USA) was employed to extract RNA from the distal intestinal tissues. The researchers then used NanoDrop 2000 (Thermo Fisher Scientific, USA) to measure RNA concentrations and RNA integrity on a 1% agarose gel. To prepare cDNA, RNA was processed using the Evo M-MLV Reverse Transcription Kit (Takara, Japan).
Real-time quantitative PCR was carried out on a PCR Mastercycler (Mastercycler® ep realplex, Eppendorf, Germany) using the SYBR® Premix ExTaq™ II Kit (Takara, Japan). The thermal profile included a 95°C cycle, 2 minutes for each and a compound cycle twice, which is 95°C for 15 seconds, 60°C for 10 seconds and 72°C for 20 seconds. Primer synthesis template sequences were referenced to the PacBio SMART pearl gentian grouper distal intestine tissue full-length transcriptome sequencing database obtained from past studies, as detailed in Table 2. Primers were synthesised by Bioengineering Co., Ltd. (Shanghai, China). The 2−ΔΔCT method (Livak and Schmittgen, 2001) was employed to carry out the target gene expressions.
2.7 Statistical analysis
In this study, the mean and standard deviation ( ± SD) was used to present the data, and SPSS version 22.0 (SPSS Inc., Chicago, IL, USA) was selected for a one-way analysis of variance (ANOVA). The Duncan multiple comparison test was performed to determine the significant differences between the groups. Statistically significant differences were described as P< 0.05.
3 Results
3.1 Growth performance
The WGR, SGR and FCR of pearl gentian grouper were significantly affected by high contents of soybean protein concentrate or fermented soybean meal in the diets (P< 0.05), as illustrated in Figure 1. The two comparative groups did not show a significant difference in WGR, SGR and FCR (P > 0.05), but the WGR and SGR were considerably lower, and the FCR was substantially higher in the FSBM40 group. Survival rates were not significantly different and were similar between the three groups (P > 0.05).
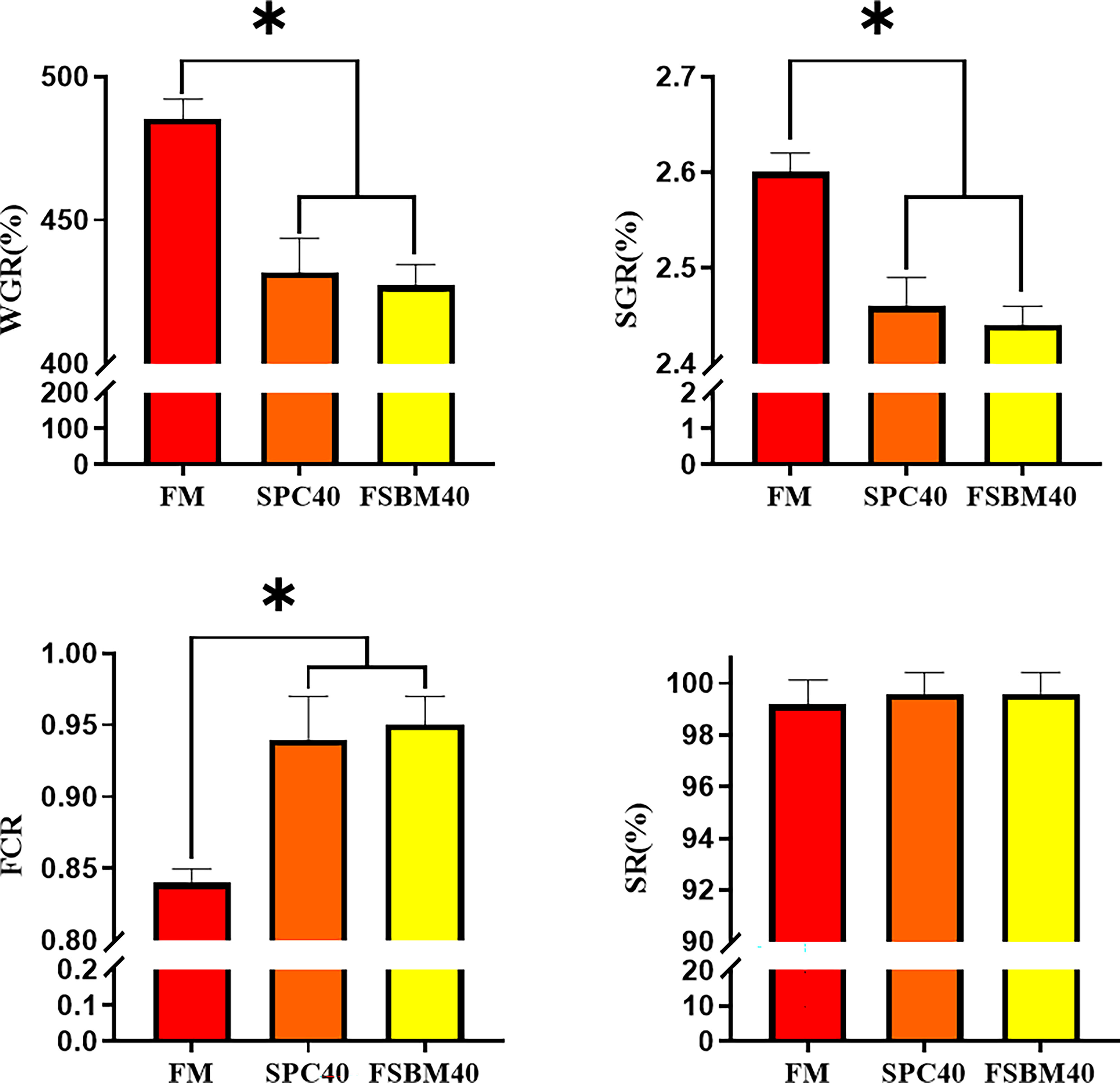
Figure 1 Effect of soybean protein concentrate or fermented soybean meal substitution of 40% fish meal protein on the growth of pearl gentian grouper (n=4). WGR, weight gain rate; SGR, specific growth rate; FCR, feed conversion ratio; SR, survival rates; *P< 0.05.
3.2 Biochemical indices
The outcomes suggested that compared to the FM group, both the SPC40 and FSBM40 groups increased fish intestinal trypsin, T-SOD and GSH-Px activity and decreased IgM, C3 and C4 protein content (P< 0.05) significantly. The SPC40 group had the highest trypsin, GSH-Px and T-SOD activity and the lowest IgM, C3 and C4 protein content (Table 3).
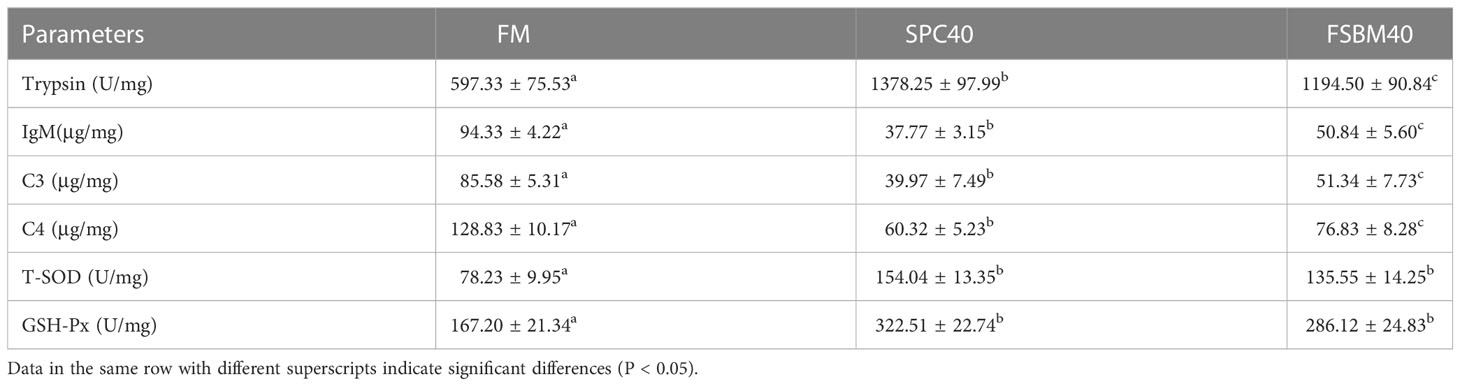
Table 3 Effect of different soybean processing products substitute for fish meal protein on the enzyme activities of pearl gentian grouper (n = 3).
3.3 Immune-related gene expression
Table 4 shows the expression levels of anti-inflammatory and pro-inflammatory cytokines in the distal intestine of pearl gentian groupers. The mRNA in pro-inflammatory cytokines, including TNFα, IL1β, IL8, IL12, IL17 and IL32, were higher in the FSBM40 and SPC40 groups (P< 0.05). Furthermore, compared with those in the FSBM40 group, the expressions of IL32 and TNFα in the SPC40 group were considerably lower (P< 0.05). Among the anti-inflammatory cytokines, compared to the FM group, the mRNA in TGFβ1, IL4, IL5 and IL10 exhibited lower levels in the distal intestine of the SPC40 and FSBM40 groups (P< 0.05). In addition, compared to the FSBM40 group, the expression level of IL5 was significantly higher in the SPC40 group (P< 0.05).
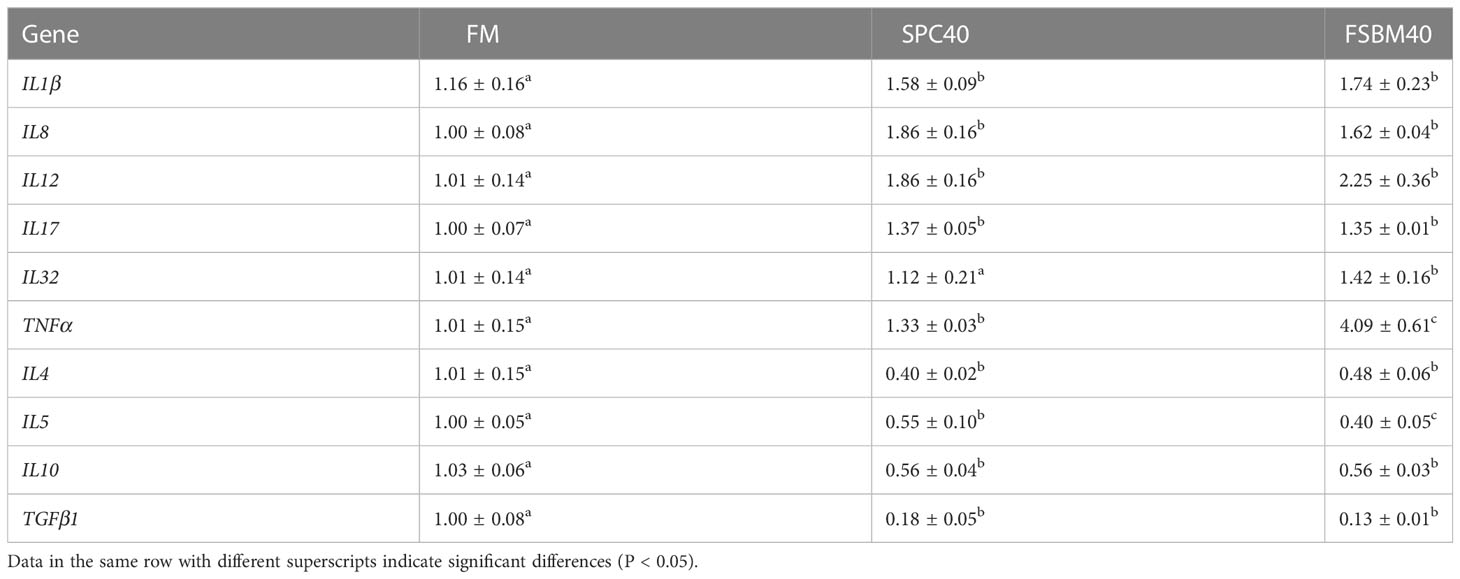
Table 4 Expression of immune-related genes in the distal intestine of pearl gentian grouper fed different soybean processing products diets (n=3).
3.4 Analysis of differentially expressed transcripts
3.4.1 Statistics of DEGs
The statistical results of the DEGs screened based on differential analysis are shown in Table 5. It is indicated that by comparison with the FM group, there are 2,328 up-regulated genes and 1,748 down-regulated genes in the SPC40 group (P< 0.05), with a total of 4076 significantly differential genes. In addition, compared with the FM group, there are 1,457 genes significantly down-regulated and 2,005 genes significantly up-regulated in the FSBM40 group (P< 0.05), for a total of 3,462 significantly different genes. As shown in Figure 2, there were 1,440 overlapping DEGs between the SPC40 and FSBM40 groups as compared to the FM group (Profile A), 2,636 DEGs unique to the SPC40 group (Profile B) and 2,022 DEGs unique to the FSBM40 group (Profile C).
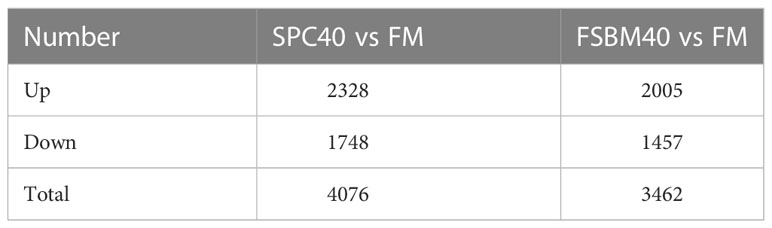
Table 5 Different soybean processing products substituted for fishmeal in the distal intestine of the pearl gentian grouper for comparison of significantly different genes (n = 4).
3.4.2 Functional enrichment analysis
GO and KEGG were used to perform the functional enrichment and classification of the DEGs. The annotation outcomes for the three broad functional categories of GO (cellular component, molecular function and biological process) show that Profile A has 47 subclasses. In addition, in the biological process, the metabolic process (342) was the most enriched. Profile B comprises 53 subclasses, and the single-organism process (535) was the most enriched in the biological process. Profile C has 51 subclasses, of which the metabolic process (342) was the most enriched in the biological process category. Binding was the most enriched sub-categories in both Profile A (418), Profile B (670) and Profile C (554) among the molecular functional categories. Among the cellular component categories, the membrane was the most enriched sub-category in both Profile A (301), Profile B (430) and Profile C (276) (Figure 3).
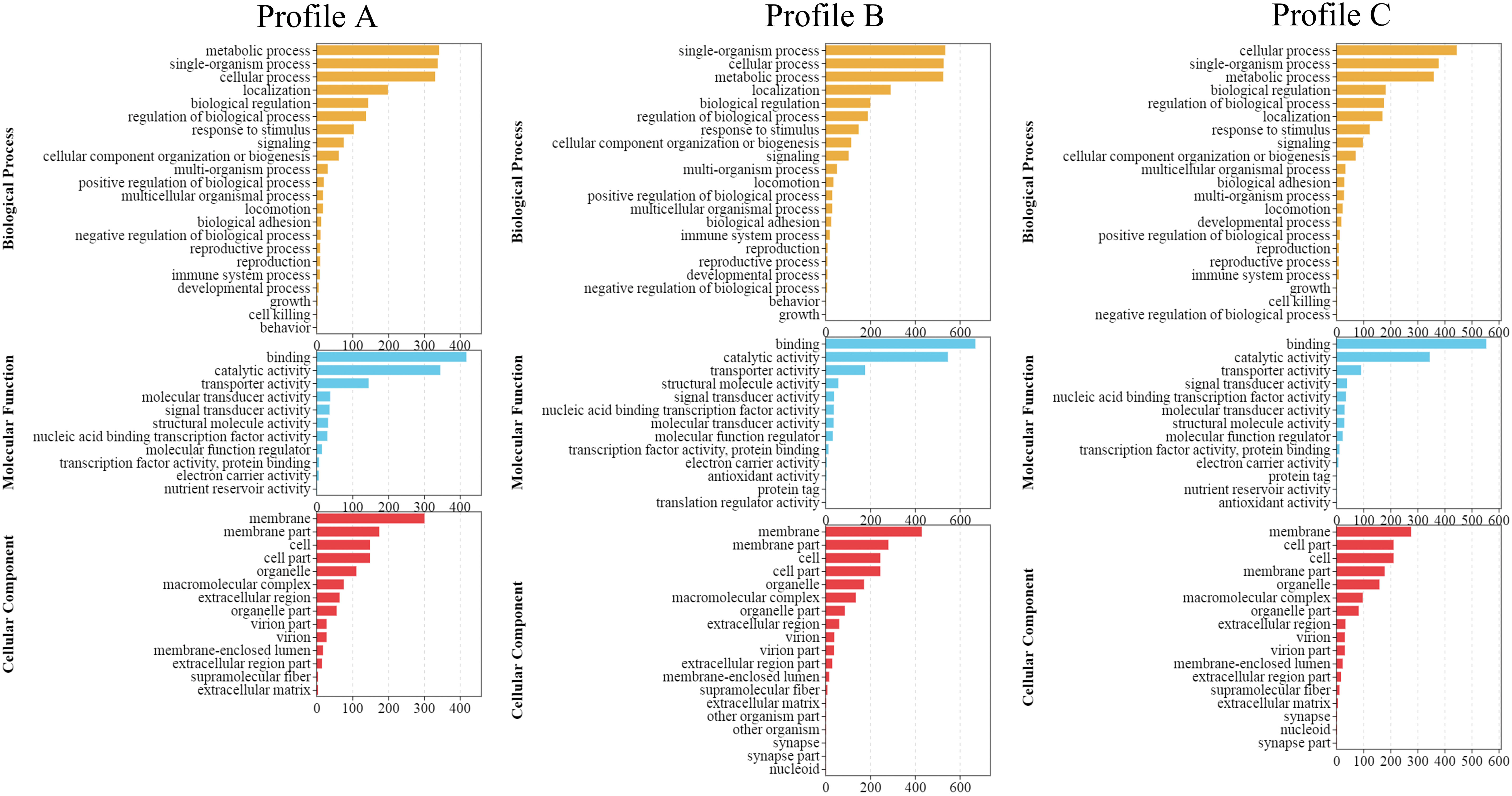
Figure 3 Results of GO enrichment analysis of DEGs enrichment under three broad functional categories (n = 4).
According to the significant results of the KEGG enrichment analysis, in Profile A, 287 signalling pathways were enriched in Profile A, with 38 signalling pathways showing substantial change (P< 0.05). The largest proportion of these significantly changed pathways involved the digestive system-related pathways (5/38), lipid metabolism-related pathways (5/38) and those related to the metabolism of cofactors and vitamins (5/38), including the digestion and absorption of vitamin, fat and protein, mineral absorption, linoleic and alpha-linolenic acid metabolism, nicotinate and nicotinamide metabolism, arachidonic acid metabolism, vitamin B6 metabolism, thiamine metabolism, glycerophospholipid metabolism, ether lipid metabolism, retinol metabolism, folate biosynthesis and pancreatic secretion (Figure 4A). In Profile B, 326 signalling pathways were enriched, with 34 signalling pathways exhibiting a significant change (P< 0.05). Most of these significantly changed pathways were lipid metabolism-related (14/34) and digestive system-related (7/34), including the digestion and absorption of vitamin, protein, fat, steroid biosynthesis, synthesis and degradation of ketone bodies, alpha-Linolenic acid metabolism, sphingolipid metabolism, glycerophospholipid metabolism, mineral absorption, primary bile acid biosynthesis, bile secretion, arachidonic acid metabolism, linoleic acid metabolism, steroid hormone biosynthesis, fatty acid degradation, biosynthesis of unsaturated fatty acids, ether lipid metabolism, glycerolipid metabolism, carbohydrate digestion and absorption, pancreatic secretion and fatty acid biosynthesis (Figure 4B). In Profile C, 314 signalling pathways were enriched, with 56 signalling pathways showing significant change (P< 0.05), and most were related to infectious diseases (11/56) and the immune system (8/56), including Epstein-Barr virus infection, the intestinal immune network for IgA production, staphylococcus aureus infection, leishmaniasis, measles, B cell receptor signalling pathway, amoebiasis, natural killer cell-mediated cytotoxicity, Fc gamma R-mediated phagocytosis, tuberculosis, Th17 cell differentiation, legionellosis, hepatitis C, complement and coagulation cascades, hematopoietic cell lineage, African trypanosomiasis, Fc epsilon RI signalling pathway, toxoplasmosis and influenza A (Figure 4C).
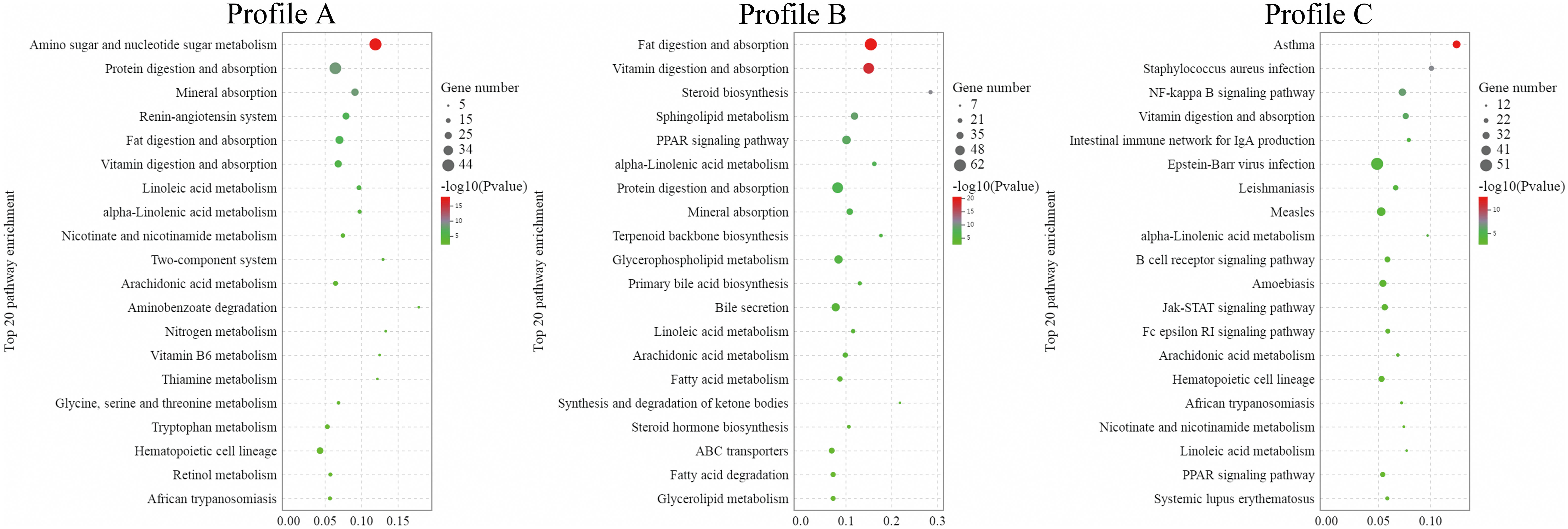
Figure 4 The top 20 differentially significant enrichment pathways in KEGG enrichment analysis of DEGs (n = 4).
3.5 Validation of the transcriptome data by qRT-PCR
To confirm the reliability of the full-length transcriptome sequencing method, the real-time quantitative PCR findings of nine immune-related genes, IL4, IL5, IL10, TGFβ1, P65, MyD88, IκBα, IgA and pIgR, were compared with the results of the transcriptome sequencing method used in this experiment (Figure 5).
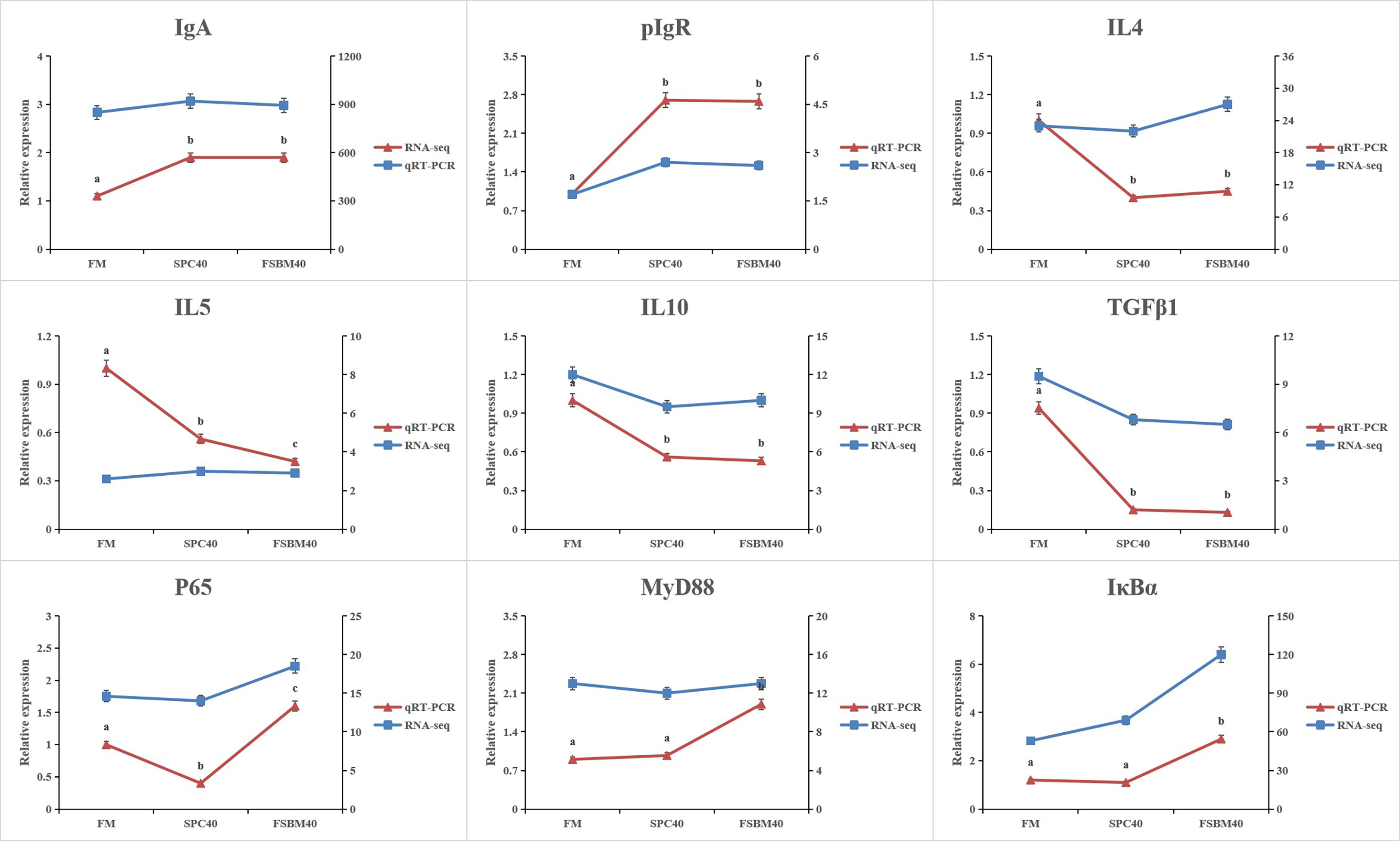
Figure 5 Comparison of RNA Seq and qRT-PCR results (n = 4). The mRNA expression level of qRT-PCR was normalized by β-actin. The relative expression level in RNA-seq analysis was calculated by FPKM value. Different letters assigned to the lines represented significant differences between the groups at P< 0.05.
4 Discussion
Although the use of plant protein instead of fish meal can effectively reduce the production cost of aquaculture, excessive use is not conducive to the digestion and absorption of certain aquatic species, which is more intuitive in terms of its growth performance (Barros et al., 2002; Yun et al., 2017). Compared to the FM group, the growth performance of both the soybean protein concentrate and fermented soybean meal groups was inhibited, which was reflected by the significantly changed values of WGR, SGR and FCR. Wang et al. (2020) concluded that the optimal percentage of fish meal substitution with soybean protein concentrate for juvenile pearl gentian grouper was 37.32% and that excessive substitution was detrimental to the results of growth. In studies on both Sparus aurata juveniles and Paralichthys olivaceus juveniles, it was found that their growth capacity decreased continuously with increasing soybean protein concentrate content (Kissil et al., 2000; Deng et al., 2006). However, Zhao et al. (2010) showed that completely replacing fish meal with soybean protein concentrate did not significantly affect the SGR of Nile tilapia (Oreochromis niloticus) fry. In addition, replacing fish meal completely with soybean protein concentrate did not affect the growth of Litopenaeus vannamei in a 72-day culture (Sá et al., 2013). This may be related to differences in species and feeding habits. Studies on the impacts of fermented soybean meal on aquatic animals are less frequent than those on soybean protein concentrate, and the growth performance of different species is affected by fermented soybean meal, showing different results. Acanthopagrus schlegeli ingestion of diets with fermented soybean meal replacing 32% and below of pollack fish meal had no significant effect on its SGR (Azarm and Lee, 2014). He et al. (2020) observed that replacing 30% fish meal with fermented soybean meal had no significant effect on the growth of Micropterus salmoides juveniles, however, increasing the replacement amount to 60% resulted in an increase in FCR and a decrease in WGR. In this study, compared to the FM and SPC40 groups, where fish meal was replaced with 40% soybean protein concentrate, WGR and SGR were found to be lower when fish meal was replaced with 40% fermented soybean meal. The study of Epinephelus coioides by Shiu et al. (2015) also concluded that growth performance was significantly diminished when fish meal was replaced with 40% fermented soybean meal. Soybean protein concentrate and fermented soybean meal have substantially eliminated the ANFs they contain, but there are still residuals that can cause growth problems in some fish and seem more pronounced in carnivorous fish.
The replacement of fish meal with soybean meal as a protein source ensures dietary protein content and its amino acid composition is appropriate. However, previous reports have often suggested that soybean meal dietary manipulation affects multiple physiological responses in a variety of fish that can affect the digestive tract. Among these, the most widely studied is SBMIE, a non-infectious subacute enteritis (Baeverfjord and Krogdahl, 1996; Urán et al., 2008; Hedrera et al., 2013). ANFs are widely considered one of the causes of this type of enteritis, and although two soybean processing products, soybean protein concentrate and fermented soybean meal, have been effective in eliminating most of the ANFs, the physiological status of pearl gentian grouper fed diets containing soybean processing products was distinctive from that of the FM group (Krogdahl et al., 2010). The soybean protein concentrate and fermented soybean meal in the diet resulted in a substantial increase in trypsin activity in the distal intestine of pearl gentian grouper, similar to the findings of Lilleeng et al. (2007) in SBMIE-affected Atlantic salmon (Salmo salar). It has also been found in humans and other animals with intestinal inflammation, which may be related to a decrease in the degradation and reabsorption capacity of trypsin due to intestinal damage (Motta et al., 2011). In the study, the changes in the levels of the immune-related components such as C3, C4 and IgM in the distal intestinal tissues indicate that excessive addition of the two soybean processing products mentioned above during culture impairs the immune function of pearl gentian grouper. Adaptive and innate immunity are two acknowledged components of the immune system of teleost fish (Uribe et al., 2011). The complement pathway belongs to innate immunity and is crucial in defending against pathogens. The functions of C3 and C4, important bioactive proteins in this pathway, have been described in various teleost fish, such as Oreochromis niloticus (Bai et al., 2022), Ctenopharyngodon idella (Meng et al., 2019), Paralichthys olivaceus (Wu et al., 2022) and Oncorhynchus mykiss (Løvoll et al., 2006). IgM is the main immunoglobulin in teleost fish and the immune response it is involved in belongs to the acquired immunity (Hikima et al., 2011). After adding soybean protein concentrate and fermented soybean meal to the feed, the levels of these three immune-related components in the intestinal tissue of pearl gentian grouper decreased significantly, which may be related to their impaired intestinal immune function. In terms of anti-oxidants, compared with the FM group, the GSH-Px and T-SOD activity in the experimental group that substituted fish meal with soybean processing products showed a significant difference, and the plant material in the diet may cause oxidative stress in the intestinal tissues of pearl gentian grouper. Similarly, Wang et al. (2020) showed that feeding pearl gentian grouper with soybean protein concentrate substitution for fish meal significantly affects its antioxidant enzyme activity. Many reports of protein source substitution in aquatic animal feeds have explored antioxidant capacity, and numerous studies of fish meal substitution with other protein sources fed to aquatic species have shown that excessive substitution is detrimental to the oxidative and antioxidant balance of the aquatic species organism (Uczay et al., 2019; Pervin et al., 2020; Shen et al., 2020; Wang et al., 2021). Severe oxidative and antioxidant imbalances damage the gut and other tissues of aquatic species, endangering their health and survival.
The analysis of the molecular responses of fish to different diets can provide a reference value for the nutritional needs of aquaculture species (Król et al., 2016). We employed the RNA-seq technique to analyse the impact of the soybean protein concentrate diet and fermented soybean meal diet on pearl gentian grouper. The third-generation sequencing technology, single-molecule real-time (SMRT), developed by Pacific Biosciences, was employed in our research to address the shortcoming of second-generation sequencing, which can only analyse small fragment sequences. There were more DEGs between the SPC40 and FM groups than between the FSBM40 and FM groups in terms of number, and about 35% of the DEGs between the SPC40 and FM groups were also found in the DEGs between the FSBM40 and FM groups. This indicates that there are large differences in the effects of these two soybean meals in the diets on fish at the transcriptional level. Functional enrichment analysis allows for the classification of differential genes and the description of gene functions. Three profiles were investigated using GO enrichment analysis and KEGG enrichment analysis, from which the similarities and differences of the impact of soybean protein concentrate or fermented soybean meal substitutes on pearl gentian grouper were inferred. The results of KEGG enrichment in Profile A revealed significant differences in multiple digestion and absorption pathways in fish, including the digestion and absorption of protein, fat and vitamin and mineral absorption, which corroborated the findings that both soybean protein concentrate and fermented soybean meal diets significantly affected fish growth performance. The activity of digestive enzymes in the fish intestine is influenced by the nutrients in the diet. The significant effect of feeding soybean protein concentrate and fermented soybean meal diets on the digestion and absorption of pearl gentian grouper may be due to changes in digestive enzyme activity, and the residual ANFs in soybean protein concentrate and fermented soybean meal may be one of the main reasons for changing the intestinal environment of fish and thus affecting digestive enzyme activity (Li et al., 2014; Liu et al., 2017). The nutrition of the soy processed product diet is not as nutritionally balanced as that of the fish meal diet, and fish growth receives an impact and responds through compensatory behaviour. This includes a series of means to promote biosynthesis, in which the secretion of many other substances related to digestive enzymes, in addition to digestive enzymes, is also improved (Kemski et al., 2020). In this research, the transcriptomic outcomes revealed that the soybean protein concentrate diet significantly impacted the fat digestion and absorption pathway in fish and that the bile secretion pathway was also greatly affected. Among the membrane protein, the second largest family is the solute carrier (SLC) superfamily. The structure of the various proteins in the SLC superfamily varies considerably, but their functions are united, and SLC transporter proteins are crucial in the absorption of nutrients (César-Razquin et al., 2015). In addition, the disruption of intestinal homeostasis in human Crohn’s disease patients is associated with SLC22A4 and SLC22A5 mutations affecting L-carnitine absorption (Fortin et al., 2009). Another study found that SBMIE decreases the expression level of the SLC22A5 gene associated with fatty acid metabolism (Sahlmann et al., 2013). The differential genes under the soybean processed product diet in the enrichment analysis results also included various SLC transporter protein genes, such as SLC22A5, SLC26A3, SLC23A1, and SLC4A4.
Diets that have an excessively detrimental effect on the intestinal environment can promote intestinal tissue damage and inflammation. More research is needed to determine the cause of SBMIE in carnivorous fish caused by plant protein sources such as soybean. In the present study, according to the outcomes of inflammation-related gene expression levels, the soybean protein concentrate diet and fermented soybean meal diet significantly increased the expression levels of multiple pro-inflammatory genes while reducing the expression levels of multiple anti-inflammatory genes in fish intestinal tissues compared to the FM group. It has been shown that a similar phenomenon occurs in fish with SBMIE (Hedrera et al., 2013; Sahlmann et al., 2013; Wu et al., 2018). In this study, the amount of soybean protein concentrate and fermented soybean meal used to substitute fish meal may have exceeded the tolerance level of pearl gentian grouper, causing intestinal inflammation. Urán et al. (2008) found that the expression levels of inflammatory genes in the distal intestine of carp (Cyprinus carpio) were also affected by soybean meal in the diet. In the early stages of feeding the soybean meal diet, the expression of pro-inflammatory cytokines TNFα and IL1β was significantly up-regulated, but there was no downregulation of anti-inflammatory cytokine IL10 expression in the intestinal tissues of carp. The other difference is that carp will gradually adapt to the soybean meal diet and normalise their intestinal inflammatory gene expression levels. The digestive system of fish differs depending on their dietary habits, and the protein requirements of carnivorous aquaculture fish are high. There is no effective treatment for intestinal inflammation in carnivorous fish induced by replacing fish meal with plant material to reduce costs. Undoubtedly, the reduction of ANFs in plants is effective, but the use of soybean processing products after a substantial reduction in ANFs did not produce better results in this experiment. Some studies have shown that the soybean meal component of the diet is detrimental to the stabilisation of the intestinal flora of teleost fish, which may be one of the causes of SBMIE (Nayak, 2010; Miao et al., 2018; Liu et al., 2019). In addition, whether adding dietary additives to improve nutrition and intestinal flora can effectively relieve intestinal inflammation needs further study.
To sum up, soybean protein concentrate or fermented soybean meal components in feed can lead to a significant reduction in the growth performance of juvenile pearl gentian grouper. Although the differences in the SR of the fish were not significant, the intestinal health of the SPC40 and FSBM40 groups had been severely challenged. The DEGs obtained from the transcriptomic analysis of the distal intestine in this study can help further explore the mechanisms of food-borne intestinal inflammation caused by soybean components of this species, providing a theoretical basis for solving SBMIE and thus promoting green and sustainable aquaculture.
Data availability statement
The data presented in the study are deposited in the NCBI Sequential Read Archive (SRA) repository, accession number PRJNA664623 and PRJNA66441.
Ethics statement
The animal study was reviewed and approved by the ethics review board of Guangdong Ocean University.
Author contributions
AP designed and took part in the whole process of the experiment, and wrote the draft of this manuscript. BT and ZW co-conceived the experiment, revised the draft critically for important intellectual content. YX and RX participated in the experiments and WZ revised the first draft. All authors contributed to the article and approved the submitted version.
Funding
This research was funded by the National Key R&D Program of China (2019YFD0900200), the National Natural Science Foundation of China (NSFC 31772864), the China Agriculture Research System of MOF and MARA (CARS-47), and the Guangdong Ocean University Doctoral Research Initiation Project (060302022008).
Acknowledgments
We are grateful to the Key Laboratory of Aquatic, Livestock and Poultry Feed Science and Technology in South China, Ministry of Agriculture, for providing technical assistances.
Conflict of interest
Authors ZW and RX are employed by Guangdong Evergreen Feed industry Co., Ltd.
The remaining authors declare that the research was conducted in the absence of any commercial or financial relationships that could be construed as a potential conflict.
Publisher’s note
All claims expressed in this article are solely those of the authors and do not necessarily represent those of their affiliated organizations, or those of the publisher, the editors and the reviewers. Any product that may be evaluated in this article, or claim that may be made by its manufacturer, is not guaranteed or endorsed by the publisher.
References
Azarm H. M., Lee S. M. (2014). Effects of partial substitution of dietary fish meal by fermented soybean meal on growth performance, amino acid and biochemical parameters of juvenile black sea bream Acanthopagrus schlegeli. Aquac. Res. 45, 994–1003. doi: 10.1111/are.12040
Baeverfjord G., Krogdahl Å. (1996). Development and regression of soybean meal induced enteritis in Atlantic salmon, Salmo salar l., distal intestine: A comparison with the intestines of fasted fish. J. Fish Dis. 19, 375–387. doi: 10.1111/j.1365-2761.1996.tb00376.x
Bai H., Mu L., Qiu L., Chen N., Li J., Zeng Q., et al. (2022). Complement C3 regulates inflammatory response and Monocyte/Macrophage phagocytosis of Streptococcus agalactiae in a teleost fish. Int. J. Mol. Sci. 23, 15586. doi: 10.3390/ijms232415586
Barros M. M., Lim C., Klesius P. H. (2002). Effect of soybean meal replacement by cottonseed meal and iron supplementation on growth, immune response and resistance of channel catfish (Ictalurus puctatus) to edwardsiella ictaluri challenge. Aquaculture 207, 263–279. doi: 10.1016/S0044-8486(01)00740-2
Brandl K., Kumar V., Eckmann L. (2017). Gut-liver axis at the frontier of host-microbial interactions. Am. J. Physiol. - Gastrointest. Liver Physiol. 312, G413–G419. doi: 10.1152/ajpgi.00361.2016
César-Razquin A., Snijder B., Frappier-Brinton T., Isserlin R., Gyimesi G., Bai X., et al. (2015). A call for systematic research on solute carriers. Cell 162, 478–487. doi: 10.1016/j.cell.2015.07.022
Chen Y., Liu W., Ma J., Wang Y., Huang H. (2020). Comprehensive physiological and transcriptomic analysis revealing the responses of hybrid grouper (Epinephelus fuscoguttatus♀ × e. lanceolatus♂) to the replacement of fish meal with soy protein concentrate. Fish Physiol. Biochem. 46, 2037–2053. doi: 10.1007/s10695-020-00851-3
Deng J., Mai K., Ai Q., Zhang W., Wang X., Xu W., et al. (2006). Effects of replacing fish meal with soy protein concentrate on feed intake and growth of juvenile Japanese flounder, Paralichthys olivaceus. Aquaculture 258, 503–513. doi: 10.1016/j.aquaculture.2006.04.004
Fortin G., Yurchenko K., Collette C., Rubio M., Villani A. C., Bitton A., et al. (2009). L-carnitine, a diet component and organic cation transporter OCTN ligand, displays immunosuppressive properties and abrogates intestinal inflammation. Clin. Exp. Immunol. 156, 161–171. doi: 10.1111/j.1365-2249.2009.03879.x
He M., Li X., Poolsawat L., Guo Z., Yao W., Zhang C., et al. (2020). Effects of fish meal replaced by fermented soybean meal on growth performance, intestinal histology and microbiota of largemouth bass (Micropterus salmoides). Aquac. Nutr. 26, 1058–1071. doi: 10.1111/anu.13064
Hedrera M. I., Galdames J. A., Jimenez-Reyes M. F., Reyes A. E., Avendaño-Herrera R., Romero J., et al. (2013). Soybean meal induces intestinal inflammation in zebrafish larvae. PLoS One 8, e69983. doi: 10.1371/journal.pone.0069983
Hikima J. I., Jung T. S., Aoki T. (2011). Immunoglobulin genes and their transcriptional control in teleosts. Dev. Comp. Immunol. 35, 924–936. doi: 10.1016/j.dci.2010.10.011
Hu H., Kortner T. M., Gajardo K., Chikwati E., Tinsley J., Krogdahl A. (2016). Intestinal fluid permeability in Atlantic salmon (Salmo salar l.) is affected by dietary protein source. PLoS One 11, 1–18. doi: 10.1371/journal.pone.0167515
Kemski M. M., Rappleye C. A., Dabrowski K., Bruno R. S., Wick M. (2020). Transcriptomic response to soybean meal-based diets as the first formulated feed in juvenile yellow perch (Perca flavescens). Sci. Rep. 10, 1–12. doi: 10.1038/s41598-020-59691-z
Kissil G. W., Lupatsch I., Higgs D. A., Hardy R. W. (2000). Dietary substitution of soy and rapeseed protein concentrates for fish meal, and their effects on growth and nutrient utilization in gilthead seabream Sparus aurata l. Aquac. Res. 31, 595–601. doi: 10.1046/j.1365-2109.2000.00477.x
Krogdahl Å., Penn M., Thorsen J., Refstie S., Bakke A. M. (2010). Important antinutrients in plant feedstuffs for aquaculture: An update on recent findings regarding responses in salmonids. Aquac. Res. 41, 333–344. doi: 10.1111/j.1365-2109.2009.02426.x
Król E., Douglas A., Tocher D. R., Crampton V. O., Speakman J. R., Secombes C. J., et al. (2016). Differential responses of the gut transcriptome to plant protein diets in farmed Atlantic salmon. BMC Genomics 17, 1–16. doi: 10.1186/s12864-016-2473-0
Løvoll M., Kilvik T., Boshra H., Bøgwald J., Sunyer J. O., Dalmo R. A. (2006). Maternal transfer of complement components C3-1, C3-3, C3-4, C4, C5, C7, bf, and df to offspring in rainbow trout (Oncorhynchus mykiss). Immunogenetics 58, 168–179. doi: 10.1007/s00251-006-0096-3
Li Y., Ai Q., Mai K., Xu W., Deng J., Cheng Z. (2014). Comparison of high-protein soybean meal and commercial soybean meal partly replacing fish meal on the activities of digestive enzymes and aminotransferases in juvenile Japanese seabass, Lateolabrax japonicus (Cuvier 1828). Aquac. Res. 45, 1051–1060. doi: 10.1111/are.12042
Lilleeng E., Froystad M. K., Ostby G. C., Valen E. C., Krogdahl A. (2007). Effects of diets containing soybean meal on trypsin mRNA expression and activity in Atlantic salmon (Salmo salar l). Comp. Biochem. Physiol. - A Mol. Integr. Physiol. 147, 25–36. doi: 10.1016/j.cbpa.2006.10.043
Liu Y., Chen Z., Dai J., Yang P., Xu W., Ai Q., et al. (2019). Sodium butyrate supplementation in high-soybean meal diets for turbot (Scophthalmus maximus l.): Effects on inflammatory status, mucosal barriers and microbiota in the intestine. Fish Shellfish Immunol. 88, 65–75. doi: 10.1016/j.fsi.2019.02.064
Liu H., Jin J., Zhu X., Han D., Yang Y., Xie S. (2017). Effect of substitution of dietary fish meal by soybean meal on different sizes of gibel carp (Carassius auratus gibelio): digestive enzyme gene expressions and activities, and intestinal and hepatic histology. Aquac. Nutr. 23, 129–147. doi: 10.1111/anu.12375
Livak K. J., Schmittgen T. D. (2001). Analysis of relative gene expression data using real-time quantitative PCR and the 2-ΔΔCT method. Methods 25, 402–408. doi: 10.1006/meth.2001.1262
Meng X., Shen Y., Wang S., Xu X., Dang Y., Zhang M., et al. (2019). Complement component 3 (C3): An important role in grass carp (Ctenopharyngodon idella) experimentally exposed to aeromonas hydrophila. Fish Shellfish Immunol. 88, 189–197. doi: 10.1016/j.fsi.2019.02.061
Miao S., Zhao C., Zhu J., Hu J., Dong X., Sun L. (2018). Dietary soybean meal affects intestinal homoeostasis by altering the microbiota, morphology and inflammatory cytokine gene expression in northern snakehead. Sci. Rep. 8, 1–10. doi: 10.1038/s41598-017-18430-7
Motta J., Martin L., Vergnolle N. (2011). Proteases/Antiproteases in Inflammatory Bowel Diseases” in Proteases and Their Receptors in Inflammation. Springer (Basel) 173–215. doi: 10.1007/978-3-0348-0157-7
Mugwanya M., Dawood M. A. O., Kimera F., Sewilam H. (2022). Replacement of fish meal with fermented plant proteins in the aquafeed industry: A systematic review and meta-analysis. Rev. Aquac. 15, 62–88 doi: 10.1111/raq.12701
Nayak S. K. (2010). Role of gastrointestinal microbiota in fish. Aquac. Res. 41, 1553–1573. doi: 10.1111/j.1365-2109.2010.02546.x
Pervin M. A., Jahan H., Akter R., Omri A., Hossain Z. (2020). Appraisal of different levels of soybean meal in diets on growth, digestive enzyme activity, antioxidation, and gut histology of tilapia (Oreochromis niloticus). Fish Physiol. Biochem. 46, 1397–1407. doi: 10.1007/s10695-020-00798-5
Rumsey G. L., Siwicki A. K., Anderson D. P., Bowser P. R. (1994). Effect of soybean protein on serological response, non-specific defense mechanisms, growth, and protein utilization in rainbow trout. Vet. Immunol. Immunopathol. 41, 323–339. doi: 10.1016/0165-2427(94)90105-8
Sørensen M., Penn M., El-Mowafi A., Storebakken T., Chunfang C., Øverland M., et al. (2011). Effect of stachyose, raffinose and soya-saponins supplementation on nutrient digestibility, digestive enzymes, gut morphology and growth performance in Atlantic salmon (Salmo salar, l). Aquaculture 314, 145–152. doi: 10.1016/j.aquaculture.2011.02.013
Sá M. V. C., Sabry-Neto H., Cordeiro-Júnior E., Nunes A. J. P. (2013). Dietary concentration of marine oil affects replacement of fish meal by soy protein concentrate in practical diets for the white shrimp, Litopenaeus vannamei. Aquac. Nutr. 19, 199–210. doi: 10.1111/j.1365-2095.2012.00954.x
Sahlmann C., Sutherland B. J. G., Kortner T. M., Koop B. F., Krogdahl Å., Bakke A. M. (2013). Early response of gene expression in the distal intestine of Atlantic salmon (Salmo salar l.) during the development of soybean meal induced enteritis. Fish Shellfish Immunol. 34, 599–609. doi: 10.1016/j.fsi.2012.11.031
Shen J., Liu H., Tan B., Dong X., Yang Q., Chi S., et al. (2020). Effects of replacement of fishmeal with cottonseed protein concentrate on the growth, intestinal microflora, haematological and antioxidant indices of juvenile golden pompano (Trachinotus ovatus). Aquac. Nutr. 26, 1119–1130. doi: 10.1111/anu.13069
Shiu Y. L., Hsieh S. L., Guei W. C., Tsai Y. T., Chiu C. H., Liu C. H. (2015). Using Bacillus subtilis E20-fermented soybean meal as replacement for fish meal in the diet of orange-spotted grouper (Epinephelus coioides, Hamilton). Aquac. Res. 46, 1403–1416. doi: 10.1111/are.12294
Uczay J., Battisti E. K., Lazzari R., Pessatti M. L., Schneider T. L. S., Hermes L. B., et al. (2019). Fish meal replaced by hydrolysed soybean meal in diets increases growth and improves the antioxidant defense system of silver catfish (Rhamdia quelen). Aquac. Res. 50, 1438–1447. doi: 10.1111/are.14019
Urán P. A., Gonçalves A. A., Taverne-Thiele J. J., Schrama J. W., Verreth J. A. J., Rombout J. H. W. M. (2008). Soybean meal induces intestinal inflammation in common carp (Cyprinus carpio l.). Fish Shellfish Immunol. 25, 751–760. doi: 10.1016/j.fsi.2008.02.013
Uribe C., Folch H., Enriquez R., Moran G. (2011). Innate and adaptive immunity in teleost fish: A review. Vet. Med. (Praha). 56, 486–503. doi: 10.17221/3294-VETMED
van den Ingh T. S. G. A. M., Krogdahl Å., Olli J. J., Hendriks H. G. C. J. M., Koninkx J. G. J. F. (1991). Effects of soybean-containing diets on the proximal and distal intestine in Atlantic salmon (Salmo salar): a morphological study. Aquaculture 94, 297–305. doi: 10.1016/0044-8486(91)90174-6
Wang J., Liang D., Yang Q., Tan B., Dong X., Chi S., et al. (2020). The effect of partial replacement of fish meal by soy protein concentrate on growth performance, immune responses, gut morphology and intestinal inflammation for juvenile hybrid grouper (Epinephelus fuscoguttatus ♀ × epinephelus lanceolatus ♂). Fish Shellfish Immunol. 98, 619–631. doi: 10.1016/j.fsi.2019.10.025
Wang G., Peng K., Hu J., Mo W., Wei Z., Huang Y. (2021). Evaluation of defatted Hermetia illucens larvae meal for Litopenaeus vannamei: effects on growth performance, nutrition retention, antioxidant and immune response, digestive enzyme activity and hepatic morphology. Aquac. Nutr. 27, 986–997. doi: 10.1111/anu.13240
Wu M., Jia B. B., Li M. F. (2022). Complement C3 and activated fragment C3a are involved in complement activation and anti-bacterial immunity. Front. Immunol. 13. doi: 10.3389/fimmu.2022.813173
Wu N., Wang B., Cui Z. W., Zhang X. Y., Cheng Y. Y., Xu X., et al. (2018). Integrative transcriptomic and microRNAomic profiling reveals immune mechanism for the resilience to soybean meal stress in fish gut and liver. Front. Physiol. 9. doi: 10.3389/fphys.2018.01154
Yang J., Guo J., Yang X. Q., Wu N. N., Zhang J. B., Hou J. J., et al. (2014). A novel soy protein isolate prepared from soy protein concentrate using jet-cooking combined with enzyme-assisted ultra-filtration. J. Food Eng. 143, 25–32. doi: 10.1016/j.jfoodeng.2014.06.031
Yun H., Shahkar E., Hamidoghli A., Lee S., Won S., Bai S. C. (2017). Evaluation of dietary soybean meal as fish meal replacer for juvenile whiteleg shrimp, Litopenaeus vannamei reared in biofloc system. Int. Aquat. Res. 9, 11–24. doi: 10.1007/s40071-017-0152-7
Zhang W., Liu M., Sadovy de Mitcheson Y., Cao L., Leadbitter D., Newton R., et al. (2020). Fishing for feed in China: Facts, impacts and implications. Fish Fish. 21, 47–62. doi: 10.1111/faf.12414
Zhang W., Tan B., Deng J., Dong X., Yang Q., Chi S., et al. (2021). Mechanisms by which fermented soybean meal and soybean meal induced enteritis in marine fish juvenile pearl gentian grouper. Front. Physiol. 12. doi: 10.3389/fphys.2021.646853
Keywords: Epinephelus fuscoguttatus ♀ × Epinephelus lanceolatus ♂, soybean protein concentrate, fermented soybean meal, intestinal inflammation, transcriptome sequencing
Citation: Pang A, Xin Y, Xie R, Wang Z, Zhang W and Tan B (2023) Differential analysis of fish meal substitution with two soybean meals on juvenile pearl gentian grouper. Front. Mar. Sci. 10:1170033. doi: 10.3389/fmars.2023.1170033
Received: 20 February 2023; Accepted: 16 March 2023;
Published: 05 April 2023.
Edited by:
Marcel Martinez-Porchas, National Council of Science and Technology (CONACYT), MexicoReviewed by:
Mohamed Moaaz Refaey, Mansoura University, EgyptKai Zhang, Pearl River Fisheries Research Institute (CAFS), China
Copyright © 2023 Pang, Xin, Xie, Wang, Zhang and Tan. This is an open-access article distributed under the terms of the Creative Commons Attribution License (CC BY). The use, distribution or reproduction in other forums is permitted, provided the original author(s) and the copyright owner(s) are credited and that the original publication in this journal is cited, in accordance with accepted academic practice. No use, distribution or reproduction is permitted which does not comply with these terms.
*Correspondence: Wei Zhang, khzhangwei110@sina.com