- 1The Key Laboratory of Aquatic Biodiversity and Conservation of the Chinese Academy of Sciences, Institute of Hydrobiology, Chinese Academy of Sciences, Wuhan, Hubei, China
- 2University of Chinese Academy of Sciences, Beijing, China
- 3College of Fisheries, Huazhong Agricultural University, Wuhan, Hubei, China
- 4Wuhan Pindu Technology Co., Wuhan, Hubei, China
- 5College of Science and Technology, Ningbo University, Cixi, Zhejiang, China
- 6Wuhan Baiji Conservation Foundation, Wuhan, Hubei, China
- 7Administration Bureau of Chinese Guangdong White Dolphin National Nature Reserve, Zhuhai, Guangdong, China
- 8School of Marine Science, Ningbo University, Ningbo, Zhejiang, China
Underwater noise pollution from the world’s longest cross-sea bridge—the Hong Kong-Zhuhai-Macao Bridge (HZMB)—which stretches across the Chinese White Dolphin National Nature Reserve (of the People’s Republic of China, PRC) in the Pearl River Estuary may affect the distribution of local humpback dolphins. In this study, static passive acoustic monitoring was applied to monitor biosonar activity of humpback dolphins and underwater noise adjacent to the tunnel section of the HZMB for more than one year. During the monitoring period, dolphin biosonar signals were detected on 88.5% of days. A significant temporal pattern occurred in dolphin biosonar activity and in anthropogenic noise. Biosonar activity was significantly higher at night than during the day, whereas underwater noise occurred more in the day than at night. Significantly more echolocation signals were detected in winter-spring than in summer-autumn, and highest acoustic activity occurred significantly more during high tide than at other tidal periods. In addition, the negative correlation between elevated underwater noise and dolphin sonar activity in winter suggests that dolphins may avoid noisy waters for short periods, perhaps due to auditory stress, but fish prey movement cannot be ruled out. These findings facilitate understanding activity patterns of humpback dolphins in the Pearl River Estuary and may contribute to conservation efforts.
1 Introduction
Human-derived underwater noise in the ocean is ubiquitous and has posed health risks to cetaceans. For example, underwater noise can interfere with the reception of natural signals by cetaceans. This reduces communication space (Richardson et al., 1995; Erbe et al., 2019), leading to changes in cetacean habitat use and distribution patterns (Morton and Symonds, 2002; Merchant et al., 2014). Moreover, high-intensity noise can even cause auditory system damage to animals (Wang et al., 2014b; Finneran et al., 2015; Leunissen et al., 2019; Wang et al., 2020). Cetaceans serve as sentinel and indicator species for assessing marine ecosystems, and they rely on their sophisticated biosonar system for pivotal life activities, including communication, navigation, localization, foraging, and predator avoidance (Richardson et al., 1995; Au et al., 2008). Therefore, monitoring cetacean acoustic signals can clarify the distribution of these species and their behaviors (Gregorietti et al., 2021). Investigating the potential effects of anthropogenic noise pollution on local cetaceans is crucial to direct appropriate conservation management measures.
Nearshore estuaries, as transitional zones linking land and sea at the interface of saltwater and freshwater, often have high primary productivity (Mallin et al., 1991), and these areas also tend to be important habitats for most marine mammals (Jefferson and Hung, 2004; Hornby et al., 2016; Monteiro-Filho et al., 2018). However, human activities in nearshore estuaries, including shipping traffic, bridge construction, and shoreline development, crowd out the habitats of marine mammals, raise ambient native noise levels and increase the risk of their extinction (Davidson et al., 2012). The Pearl River Estuary (PRE) in southern China has typical nearshore estuarine features and is home to the world’s largest population of Indo-Pacific humpback dolphins (IPHD, Sousa chinensis), comprised of approximately 2500 individuals (Chen et al., 2010). However, recent demographic analyses indicate that the IPHD population in the PRE is decreasing at a rate of 2.5% per year (Huang et al., 2012).
IPHD are distributed in shallow coastal waters of the eastern Indian Ocean and western Pacific Ocean, with their range overlapping closely with areas of human activity (Jefferson and Curry, 2015; Jefferson and Smith, 2016). This dolphin species is classified as “Vulnerable (VU)” by the International Union for Conservation of Nature (IUCN) Red List of Threatened Species and categorized as a Grade One National Key Protected Animal by the Chinese Wild Animal Protection Law. However, in the various areas of China inhabited by IPHD, noise pollution from boat traffic, water construction projects, and other heavily anthropogenic activities has impacted the local cetaceans (Yamagiwa and Karczmarski, 2014). For example, the communication and echolocation signals of IPHD might be masked by various shipping noises (such us small high-speed boats and commercial ships) up to 1000 m from the source (Li et al., 2015; Liu et al., 2017). Furthermore, increases in noise pollution may force IPHD to change their vocalization frequency to avoid sound masking (Yuan et al., 2021).
Among the numerous water infrastructure projects in the PRE, the world’s longest sea-crossing bridge—of the Hong Kong-Zhuhai-Macao Bridge (HZMB), which began operating in 2018, has attracted the more concerned. The HZMB connects Guangdong, Hong Kong, and Macao, promoting economic and trade growth in the Greater Bay Area and the wider world. The structure comprises the bridge, islands, and tunnel schemes, and has a total length of 55 km of which the section passing through the Pearl River Estuary Chinese White Dolphin National Nature Reserve is approximately 20 km (Xiao, 2020). Additionally, the east and west artificial islands are located in the core area of the reserve. The stretch of water between these two artificial islands is an essential channel for ships to enter and exit the port of Guangzhou. Piling noise during HZMB construction and its impact on local IPHD was assessed (Wang et al., 2014b); however, noise pollution during bridge operation and its potential negative impact on local dolphins has yet to be investigated.
Visual expeditions and acoustic monitoring are mainly used for marine mammal surveys. Passive acoustic monitoring (PAM) allows continuous tracking of marine mammals and habitats over longer time scales compared with traditional visual surveys (Zimmer, 2011; Todd et al., 2020), as well as at night and under extreme weather conditions (Thompson et al., 2015). In this study, PAM was employed to investigate IPHD sonar activity and the varying temporal patterns of underwater noise in the waters near the HZMB tunnel, aiming to inform underwater noise mitigation and conservation management strategies for humpback dolphins.
2 Materials and methods
2.1 Study area
A PAM station (22°16′36′′N, 113°48′18′′E) was deployed in a fairway beacon located in the core area of the Pearl River Estuary Chinese White Dolphin National Nature Reserve at approximately 700 m from the HZMB, 4 km from the east artificial island, and 2 km from the west artificial island (Figure 1). This beacon was located on the channel boundary, approximately 150 m from the center of the waterway, and provides an essential indication of the entry and departure of ships from the Lingding waterway.
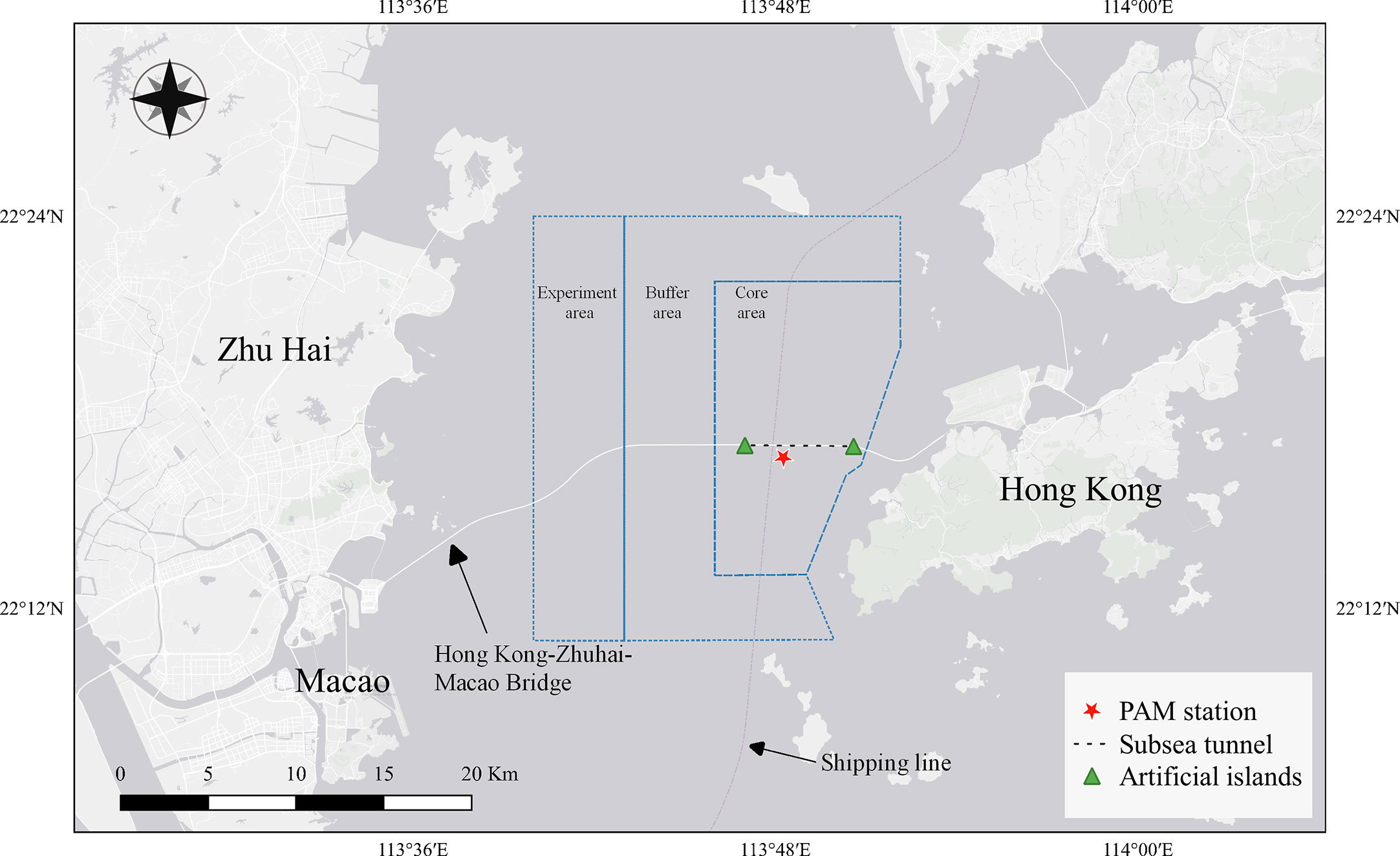
Figure 1 Locations of passive acoustic monitoring stations. Two triangles show the east and west artificial islands. The black dashed line indicates the subsea tunnel. The blue area is the Pearl River Estuary Chinese White Dolphin National Nature Reserve, and from left to right are the experimental, buffer, and core areas.
2.2 Data collection
An acoustic recorder (SoundTrap 300 HF, Ocean Instruments Ltd, New Zealand) was fixed to the anchor chain of the beacon 5 m underwater by a diving support service. The effective operating frequency range of this hydrophone was 20 Hz to 150 kHz, with a 3 dB margin of error. The hydrophone was equipped with a 16-bit analog-to-digital converter. Acoustic sound was continuously recorded for 24 h at a sampling rate of 288 kHz, and the recorded audio files (.wav) were imported into a computer. Data were collected seasonally between July 2021 and March 2022 (Table 1).
2.3 Data analysis
Dolphin sonar signals were identified using a custom-written analysis program in MATLAB R2021b (The Math Works, Natick, MA, USA). The WAV files were uploaded into the program, which first divided the waveform data into several tone frames in seconds, and filtered out low-frequency (20 kHz) noise through a high-pass filter. Filtered signals with a sound pressure level (SPL) greater than 120 dB were marked as specific frames and Fourier transformed to calculate the power spectral densities of each frame (Window size = 0.01 s, 90% frame overlap; Time resolution = 0.001 s, Frequency resolution = 100 Hz). The peak frequency, center frequency, −3 dB bandwidth and the rms bandwidth were calculated. These were then compared to the pulse parameters of IPHD (Fang et al., 2015), marking and extracting the frames that matched the statistical range of the four parameters simultaneously.
Spectrograms of each file (.wav) were examined with Raven Pro 1.6 (The Cornell Lab of Ornithology, Ithaca, NY, USA) to manually verify the dolphin sonar activity. MATLAB R2021b sequentially cut and analyzed acoustic data each second and calculated the root mean square sound pressure level (SPLrms) for each clip of underwater noise (Au et al., 2008).
The diel phase was divided into daytime (sunrise time to sunset time) and nighttime (sunset time to sunrise time) (Deconto and Monteiro-Filho, 2015; Guan et al., 2015). The seasons were defined as spring (March, April, and May), summer (June, July, and August), autumn (September, October, and November), and winter (December, January, and February). The tidal phase was divided into four phases: high, ebb, low, and flood. The high and low phases were the highest water level (Th), and the lowest water level (Tl) of the tidal phase pushed forward and backward by 1.5 h, respectively. Ebb and flood phases were the periods between the high-to-low and low-to-high phases, respectively (Wang et al., 2015b). The times of Tl and Th were obtained from the website of China Shipping Service (https://www.cnss.com.cn/tide/).
Biosonar and noise data were partitioned into 10-min bins for convenient analysis and statistical evaluation (Todd et al., 2009; Wang et al., 2015b). Each 10-min bin was assigned to different diel, seasonal, and tidal phases to investigate the pattern of IPHD sonar activity and underwater noise under different diel, tidal, and seasonal patterns. A collection of click trains with spacing of 10 min or less was considered an echolocation encounter (Carlstrom, 2005; Todd et al., 2009; Wang et al., 2015b). The seasonal acoustic signal detection rate was calculated by dividing the number of detections each season by the total number of hours recorded per season.
2.4 Statistical analysis
Descriptive parameters were calculated, including the mean and standard deviation of the number of click trains per 10-min and buzzes per 10 min (Wang et al., 2015a). The Kolmogorov–Smirnov test was used to test for normality. Owing to the discrete non-normally distribution of most of the acoustic data, the variables of the median, interquartile range (IQR), 5th percentile (P5), and 95th percentile (P95) were adopted to describe the SPLrms data. A Mann-Whitney U test (Mann and Whitney, 1947) was used to compare the biosonar activity between daytime and nighttime. For comparison of multiple data groups, Kruskal–Wallis analysis of variance (Kruskal and Wallis, 1952) tested the overall variability, and Dunn’s post hoc test (Zar, 1984) compared the variability of each group. Spearman correlation analysis was employed to investigate the relationship between underwater noise and the sonar activity of IPHD. All statistical analyses were completed using IBM SPSS Statistic 26.0 (IBM, Armonk, NY, USA).
3 Results
Acoustic monitoring data were collected for 52 days, consisting of 1209 hours of data that could be divided into 7254 10-min bins. Dolphin sonar was detected on 46 days, accounting for 88.5% of the total monitoring period. Overall, 96 IPHD echolocation encounters were monitored, 40 of which contained buzzes (Table 1). Among the 10-min bins, 260 bins contained click trains, representing 3.6% of the total dataset, and 8992 echolocation signals were monitored (Figure 2).
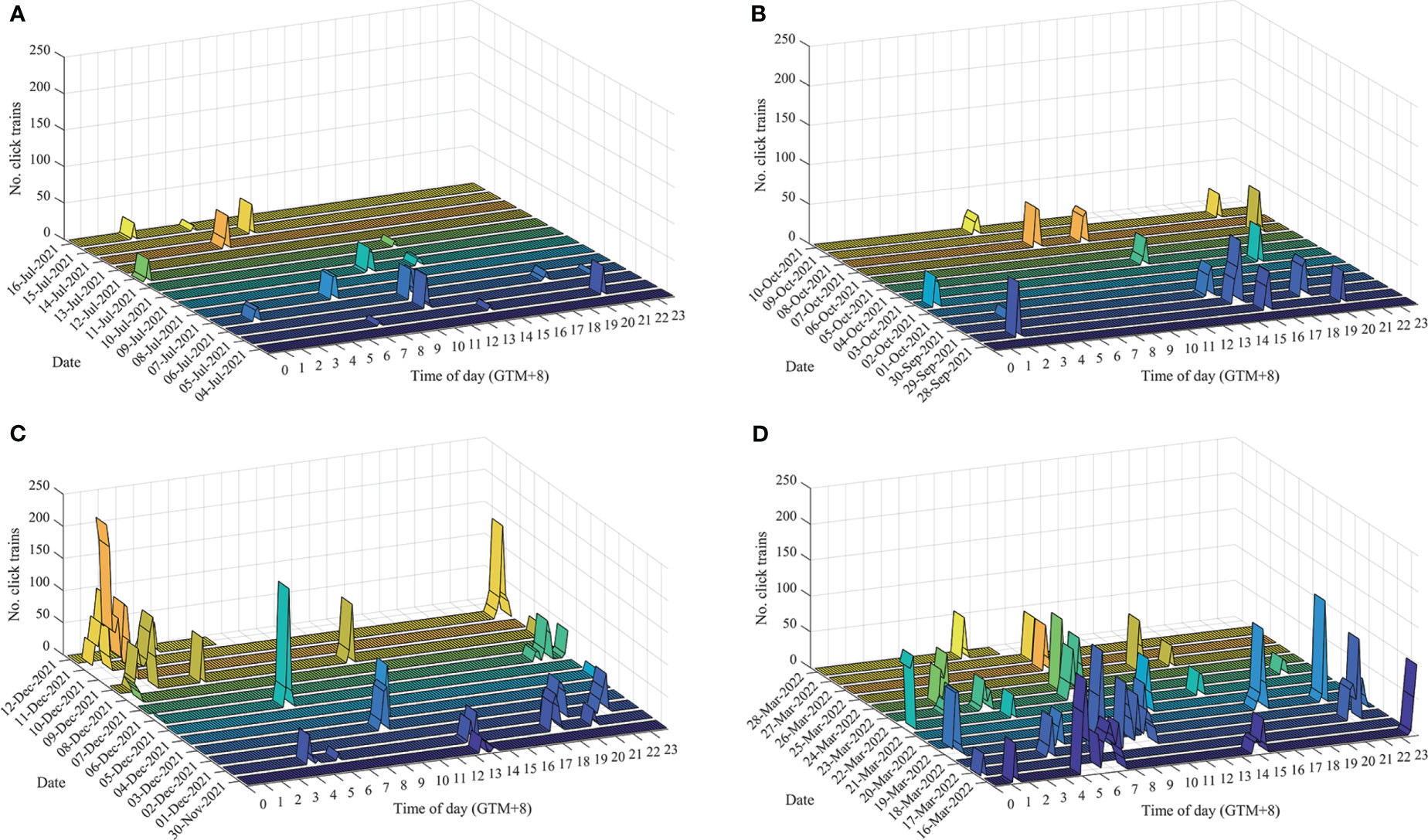
Figure 2 Daily occurrence of dolphin click trains per 10 min by the time of day in (A) summer 2021, (B) autumn 2021, (C) winter 2021, and (D) spring 2022.
The values for noise SPLrms were 136.98 ± 9.70 dB (median ± IQR) with a range of 125.81–149.80 dB for P5–P95 (Figure 3).
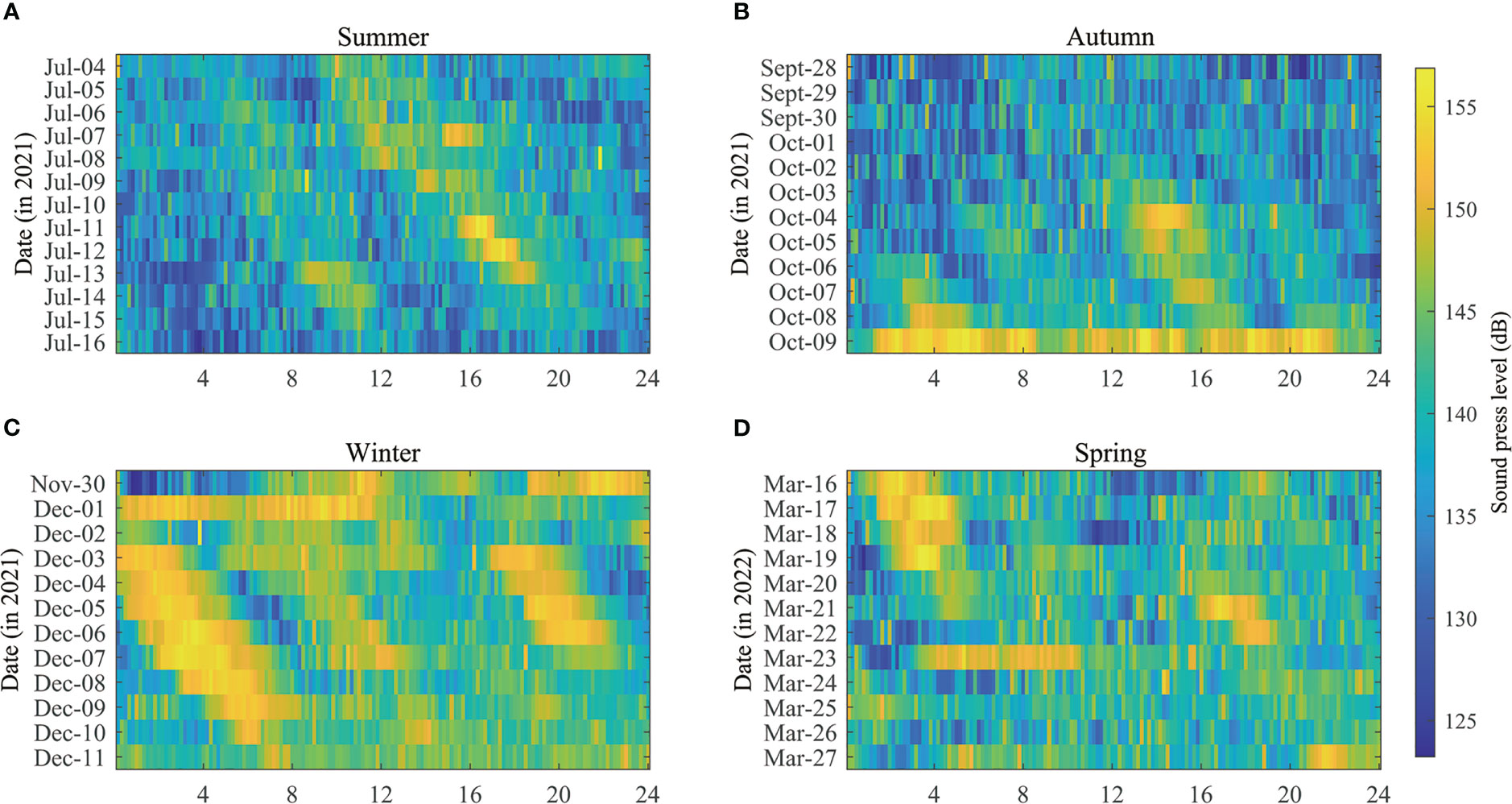
Figure 3 Sound pressure levels (SPLrms) of the water environment in different seasons as a function of time of day (X-axis) and date (Y-axis), (A) summer 2021, (B) autumn 2021, (C) winter 2021, and (D) spring 2022.
3.1 Sonar activity
The detection rates of humpback dolphin acoustic signals at the monitoring station were 16% and 12% in winter and spring, respectively, which were higher than those in summer (6%) and autumn (5%) (Figure 4). Kruskal-Wallis tests showed significant differences in IPHD acoustic activity between seasons (number of click trains per 10 min: χ2 = 74.326, df = 3, P < 0.05; the number of buzzes per 10 min: χ2 = 53.993, df = 3, P < 0.05). Dunn’s post hoc multiple comparisons revealed that the number of click trains per 10 min and the number of buzzes per 10 min were significantly higher in winter and spring than in summer and autumn (P < 0.05) (Figure 5A).
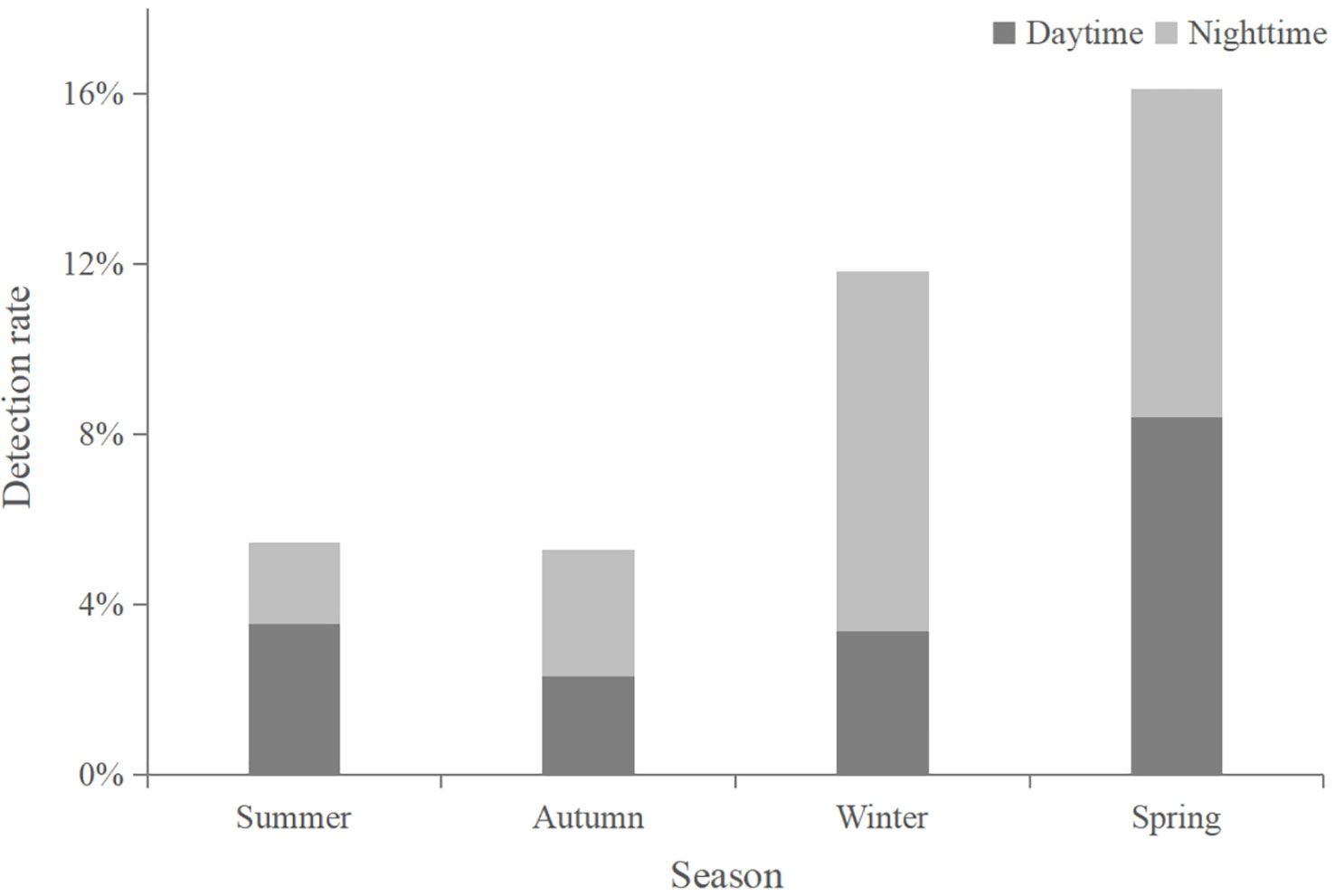
Figure 4 Echolocation detection rates of Indo-Pacific humpback dolphins in different seasons and during day and night.
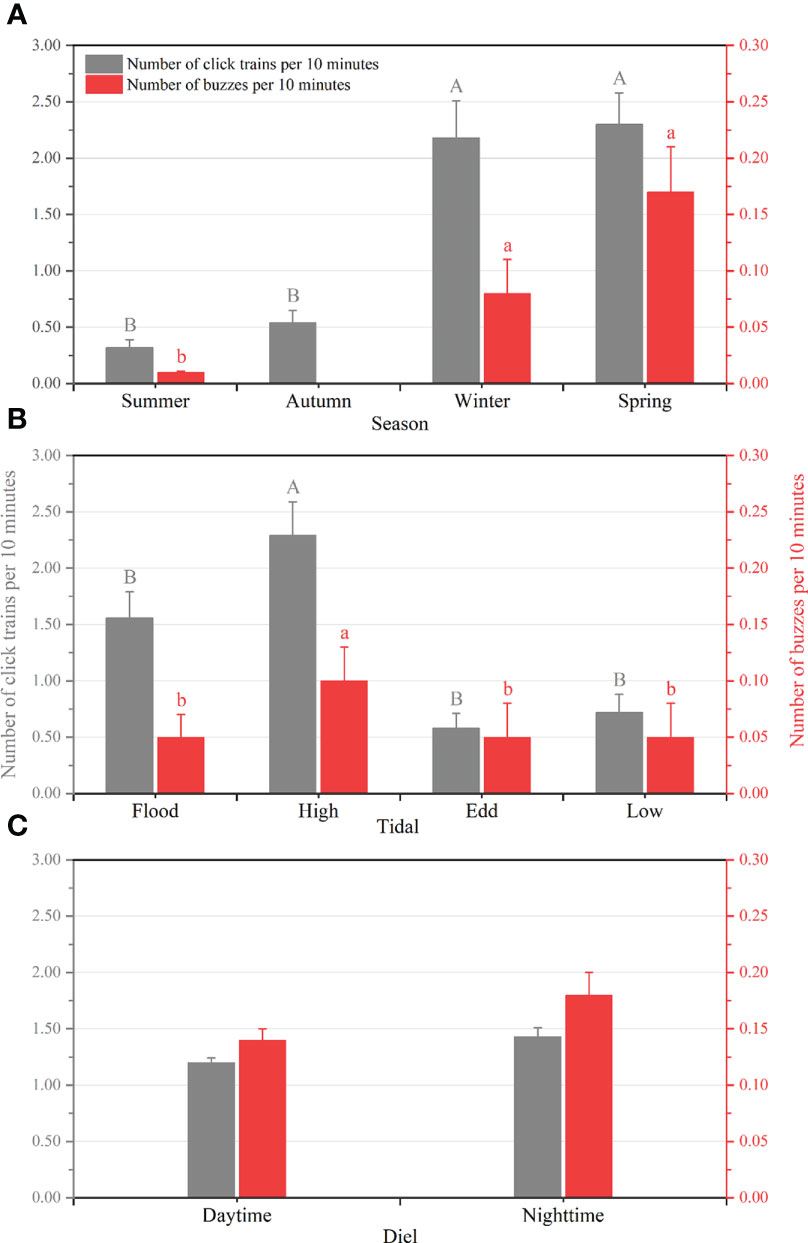
Figure 5 Number of click trains and number of buzzes per 10 min as a function of (A) seasonal, (B) tidal, and (C) diel phases. Error bars with different uppercase and lowercase letters refer to post-hoc Dunn’s multiple comparison tests with click trains and buzzes, respectively.
At high tide, the number of click trains per 10 min and the number of buzzes per 10 min was 2.29 ± 0.30 and 0.10 ± 0.03 (mean ± SD), respectively, which were significantly higher than those of other phases at this station (χ2 = 41.867, df = 3, P < 0.05, number of click trains per 10 min; χ2 = 21.402, df = 3, P < 0.05, number of buzzes per 10 min). No significant differences in the dolphin biosonar activities were found during flood, ebb, and low tide (Figure 5B).
The number of click trains and the number of buzzes per 10 min at the monitoring site did not show significant differences during the day or night (Figure 5C). However, dolphin echolocation detection rates during daytime and nighttime were 4% and 2% in summer, 2% and 3% in autumn, 3% and 8% in winter, and both 8% in spring, respectively (Figure 4). In winter, the number of click trains and buzzes per 10 min was significantly higher at night than during the daytime (P < 0.05), while no differences were found in other seasons (Table 2).
3.2 Underwater noise
Underwater noise SPLrms of the monitoring station exhibited significant differences between seasons (χ2 = 2487.139, df = 3, P < 0.05), with significantly higher SPLrms in winter (median ± IQR: 144.45 ± 6.94 dB) than in summer (133.26 ± 6.96 dB), autumn (136.21 ± 7.85 dB) and spring (136.15 ± 8.19 dB). Furthermore, SPLrms in autumn and spring was significantly higher than in summer (Dunn’s post hoc multiple comparisons, P < 0.05) (Figure 6). Throughout the acoustic monitoring period, SPLrms was significantly higher during the day than at night (Mann-Whitney U test, P < 0.05).
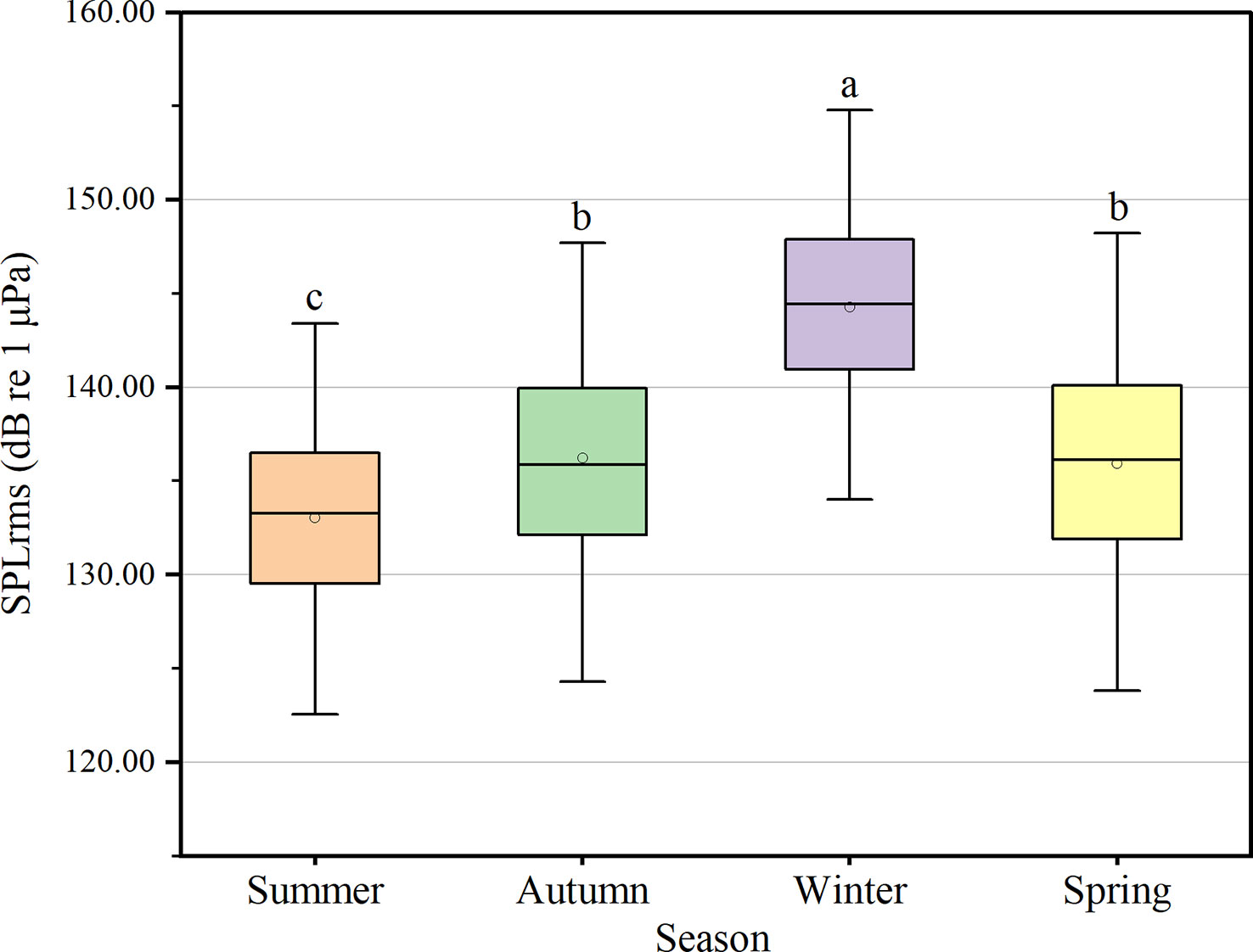
Figure 6 Comparison of broadband sound pressure levels (SPLrms) among different seasons. Different lowercase letters refer to post-hoc Dunn’s multiple comparison tests that yielded significant results (P < 0.05).
There was a significant negative correlation between SPLrms and the number of click trains per 10 min, only in winter (Spearman’s rho = −0.073, P < 0.01, n = 1776).
4 Discussion
PAM has previously been used to monitor IPHD biosonar activity in the PRE (Wang et al., 2015b; Pine et al., 2017; Wang et al., 2019; Liang et al., 2020). The subsea tunnel of HZMB is located within the core area of the Pearl River Estuary Chinese White Dolphin National Nature Reserve. Sonar signals of dolphins were detected near the HZMB subsea tunnel on 88.5% of monitoring days in this study, indicating the frequent occurrence of dolphins in the vicinity.
4.1 Sonar activity
Monitored biosonar activity revealed significant seasonal, tidal, and diurnal rhythms of IPHD. The echolocation detection rate and biosonar activity of dolphins in winter and spring were higher than those in summer and autumn. These findings are in contrast to those of the IPHD populations in the adjacent Hong Kong waters, in which a higher dolphin abundance was observed in summer than in winter (Chan and Karczmarski, 2017). The possible explanation for the difference between this study and the Hong Kong waters was that seasonal flux of dolphins may exist between mainland and Hong Kong waters. In western Taiwan, the spatial distribution of humpback dolphins in an estuary habitat varied seasonally, with the dolphins inhabiting waters close to the estuary during the dry period and migrating outside the estuary during the abundant period (Lin et al., 2014). The seasonal variation pattern observed in this study is consistent with that of the eastern waters of the PRE, Xiamen waters, and the western waters of Taiwan (Chen et al., 2008; Chen et al., 2010; Lin et al., 2014; Wang et al., 2015b). The seasonal variations of IPHD in various places reveal their multiple patterns of habitat use in different seasons.
Seasonal changes in cetacean distribution are often related to the availability of their prey (Forcada, 2009). Owing to seasonal variations in the amount of freshwater entering the ocean, which causes fish migration, dolphins are more likely to hunt in areas where their fish prey are more prevalent (Hung and Jefferson, 2004; Wang and Lin, 2006). The higher dolphin predation signals in the subsea tunnel water during the dry season may be explained by higher feeding activity in that period. Research on fish resources in the Chinese White Dolphin National Nature Reserve showed that the average biomass and abundance of the main prey of IPHD in the PRE—including the spiny head croaker (Collichthys lucidus), taper tail anchovy (Coilia mystus)—were higher in autumn compared to spring (Huang et al., 2018). Moreover, after the overfishing period (July to February of the following year), dolphin food resources might decrease in winter and spring. In the vicinity of the subsea tunnel of the HZMB, fishing intensity is relatively weak owing to the restrictions of channel administrations. Therefore, when food availability is relatively limited in spring, the increased food rewards in the central fairway may motivate dolphins to select these riskier waters and make more frequent vocalizations to catch prey.
In this study, the acoustic activity of IPHD shows a significant diurnal difference in winter, with higher detection at night than during the day. The same diurnal patterns of dolphins were widely observed in other regions of the PRE and the southwest of Hainan Island (Wang et al., 2015b; Munger et al., 2016; Dong et al., 2017; Pine et al., 2017). However, in the other three seasons, there were no diurnal differences in biosonar activity, which was consistent with observations in western Taiwan (Lin et al., 2013). This finding may indicate that there are no marked differences between the daytime and nighttime activities of IPHD near the monitoring station of this study. Greater dolphin vocalization rates at night than during the day were widely observed in previous studies conducted in the PRE (Wang et al., 2015b; Munger et al., 2016; Pine et al., 2017). However, this might be ascribed to a higher vocalizations rate per animal at night and is not necessarily indicative of increased dolphin abundance at night.
Tides are an environmental factor that can significantly influence nearshore cetacean activity, and various cetaceans display varied activity patterns during different tidal phases. Acoustic detections of bottlenose dolphins (Tursiops aduncus) in Baja California Sur, Mexico, peaked in floods and high tides (Gauger et al., 2022), while in the Shannon Estuary, Ireland, more detections occurred during ebb tide (Berrow et al., 1996; Philpott et al., 2007). Different subpopulations of the same species exhibit distinct variations in habitat usage patterns across different tidal cycles (Paitach et al., 2017). No significant tidal cycles for the biosonar activity of humpback dolphins were observed in the Modaomen estuary or the Guishan windfarms in the PRE (Wang et al., 2015b; Liang et al., 2020). Furthermore, tidal phases did not affect IPHD group size in Zhanjiang waters (Liu et al., 2021). In the nearshore area of Taiwan’s west coast, the acoustic monitoring rate was lower in the ebb tide phase than the other phases. However, the four tidal phases in the offshore area were not significantly different (Lin et al., 2013). The acoustic activity of IPHD in this study was highest at high tide and there were no significant differences between the ebb, low, and flood tide phases. This suggests that IPHD in the tunnel waters of the HZMB may be unaffected by tidal rhythm. The interaction between freshwater and seawater might have an impact on the tidal-driven behavior of estuarine dolphins and their prey (Mendes et al., 2002; Lin et al., 2013). Consequently, the wandering of prey into the intertidal zone during the tidal phase may account for the varying activity patterns of humpback dolphins during different tidal cycles.
4.2 Underwater noise
Underwater acoustic investigations of habitats are essential to determine the relationship between marine mammals and their habitat, along with analyzing the effects of various biological and anthropogenic activities on marine mammals (Sueur and Farina, 2015).
The underwater acoustic environments of Guishan windfarm in the PRE, southwest of Hainan Island and the west coast of Taiwan have been quantitatively described. This description indicates that there are various spatial and temporal patterns (including geospatial, seasonal, diurnal, and tidal) of underwater noise in different areas and that the primary sources of underwater noise include, but are not limited to, shipping traffic, pile-driving pulses, and fishing activities (Guan et al., 2015; Wang et al., 2019; Caruso et al., 2020; Xu et al., 2020; Dong et al., 2021).
The underwater noise created by pile driving with vibratory hammers during the construction period of the HZMB and subsea tunnel could mask dolphin whistles and have physiological impact (Wang et al., 2014b). The broadband SPLrms during the acoustic monitoring in this study was significantly lower than in the construction period (Wang et al., 2014b), and thus the impact on humpback dolphins during operation of the HZMB and subsea tunnel might be less than that during construction of the structure. However, compared with recordings prior to bridge construction, the ambient SPLrms increased after operation. This may be attributed to the fact that various noises generated by vehicles driving on the bridge and in the tunnel are transmitted through bridge the deck to the piers and then into the water, and this is coupled with numerous vessels congregating through the few navigable holes in the bridge. The underwater acoustic environment of the Monitor–Merrimac Memorial Bridge–Tunnel exhibited diurnally variable characteristics that were closely correlated with the temporal distribution of vehicular traffic in the underwater tunnel (Reeder et al., 2020).
Furthermore, the ambient underwater noise near the HZMB subsea tunnel exhibited a significant pattern of temporal variation in this study. Compared with the other three seasons, winter had a significantly higher SPLrms (median ± IQR: 144.45 ± 6.94 dB), which might be related to the increased waterway cargo flux during that season (http://gwj.gz.gov.cn/). In a previous study, there was no relationship between IPHD activities and underwater noise SPLrms of Qi’Ao and Sanjiao islands in PRE, but had a positive correlation with fish activity (Pine et al., 2017). In the Yangtze River, the attractiveness of fish resources for Yangtze finless porpoise (Neophocaena asiaeorientalis asiaeorientalis) also exceeded the repelling effect of shipping (Wang et al., 2014a; Wang et al., 2015a), which signified that finless porpoises were forced to be exposure to ship noise when hunting. The high biosonar detection rate of the increased underwater noise in winter in the current study may indicate that IPHD were forced by survival pressures to select the main channel, with higher predation risk. Meanwhile, the negative correlation between noise and sonar monitoring rate may indicate that with the temporary increase in SPLrms of underwater noise in winter, the fishery resources in this water area were insufficient to attract IPHD to overcome the auditory pressure forcing animals to avoid noisy areas of water in the short term.
Daytime traffic volumes on HZMB and in the channel waters of the subsea tunnel are larger than those at night, which may explain why SPLrms at this monitoring site is significantly higher during the day than at night. One third octave sound pressure level provided information to assess the frequency components of underwater noise audible to dolphins (Blackwell et al., 2004). The dominant frequency of the underwater tunnel was primarily concentrated in the frequency band below 200 Hz (Hou et al., 2020); thus, the SPL in low-frequency bands (25–400 Hz) may be ascribed by underwater tunnels (Figure 7). The optimal hearing range of fish is mostly concentrated in the range 100–400 Hz (Popper et al., 2003). Consequently, the low-frequency noise generated by the subsea tunnel may drive some fish away from the tunnel waters, thereby affecting the distribution of dolphins in the vicinity.
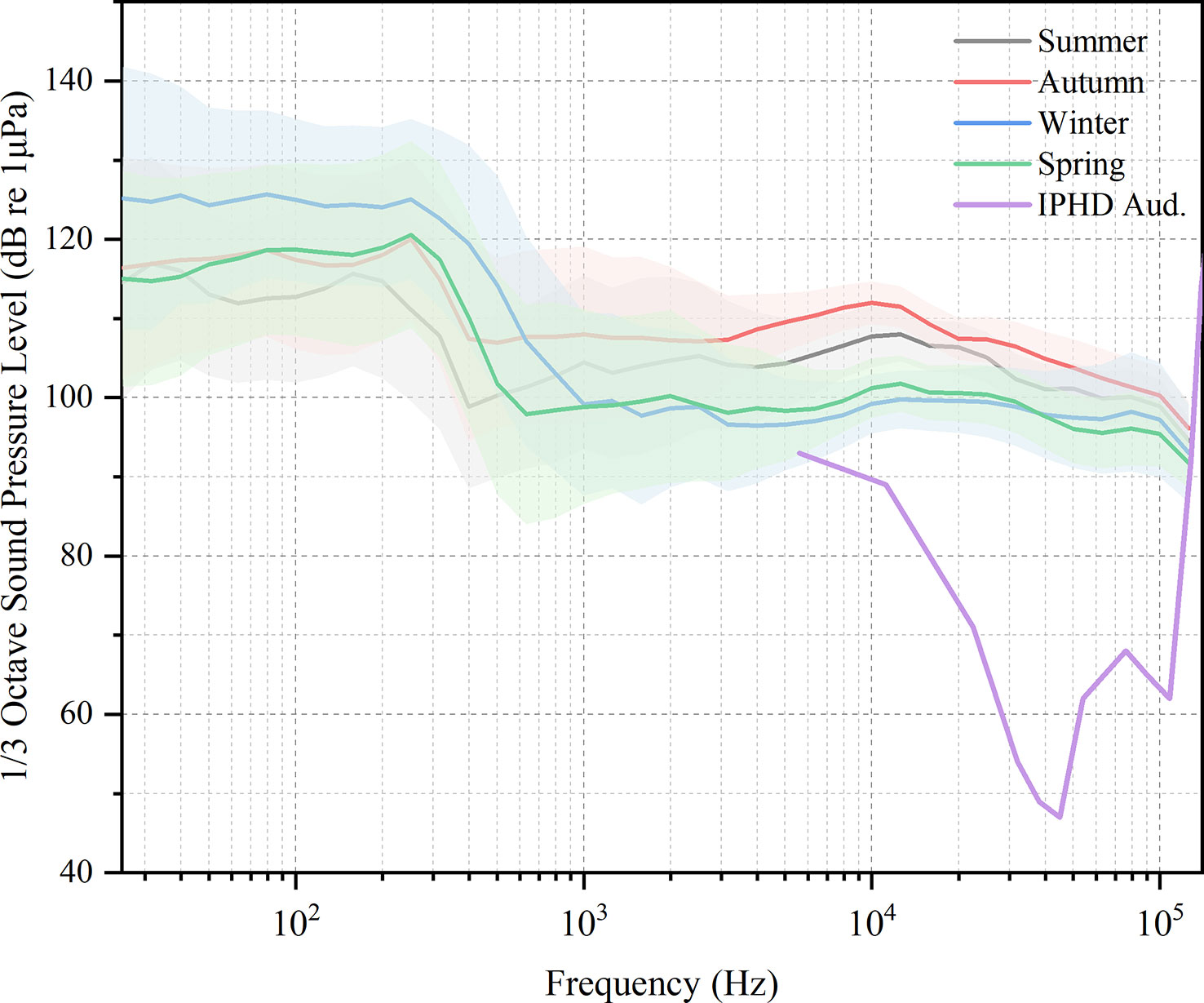
Figure 7 One third octave sound pressure level in different seasons and the average audiogram of the Indo-Pacific humpback dolphin (IPHD). Shaded areas denote the range from P25–P75, with the lower and upper boundaries representing P25 and P75, respectively. The purple line represents the average audiogram of young IPHD, which was adopted from Li et al., 2012.
The noise level was above the threshold of the IPHD audiogram at frequency bands between 5.6 and 128 kHz (Figure 7), indicating that these sounds may be perceived by dolphins and therefore limited their sound detection in these frequency bands. IPHD habitat overlaps heavily with the main channel of Guangzhou port. The increased vessel density of coastal regions frequently causes noise levels to rise significantly above ambient levels (Duarte et al., 2021). The Port of Guangzhou, a key global port, has one of the greatest shipping throughputs in the world, with massive cargo ships, tankers, fishing vessels, dredging or underwater operating vessels, and small speedboats passing through the subsea tunnel waters of HZMB. The busy vessel traffic in the Lingding waterway may further result in potential impacts on marine mammals including hearing masking and physiological damage to the auditory system (temporary threshold shift: TTS; permanent threshold shift: PTS), thereby shortening the communication distance of marine mammals (Merchant et al., 2014; Liu et al., 2017; Marley et al., 2017). When ships were presented, dolphins reduced vocalization behavior and emitted shorter calls in shorter frequency patterns, including whistles and echolocation signals (Hu et al., 2022). Hectic vessel traffic in the waters west of Hong Kong also interfered with the behavior and hearing of humpback dolphins (Sims et al., 2012).
Altering ship routes to limit the distance between vessels and dolphins, and reducing ship speed are essential measures to mitigate ambient noise levels in the habitats of marine mammals (Li et al., 2018; Schoeman et al., 2020). However, navigational safety restrictions, especially in coastal areas, mean it is not always possible to reroute a vessel (Conn and Silber, 2013). Vessel speed restrictions reduce engine noise and increase the chances of the crew spotting marine mammals, thereby reducing the probability of a collision between the vessel and animal (Vanderlaan and Taggart, 2007; Gende et al., 2011). Humpback whale-vessel collisions are much more likely to occur at speeds of more than 12 knots (6.2 m/s), independent of the size of the vessel (Gende et al., 2011; Currie et al., 2017). A forecast model suggested that the best compromise between noise exposure time and noise level is achieved at a cruising speed of 8 knots/h (McKenna et al., 2013).
This study provides baseline data of the relationships between underwater noise and dolphins in the HZMB tunnel waters of the PRE. However, the data are limited by the single monitoring site close to the tunnel and the two-week monitoring period for each season. Future research could improve information on IPHD acoustic activity and habitat use by increasing the number of monitoring sites and extending the monitoring duration to several years. In addition, the noise source is not solely correlated with traffic flux but also with the distance between the boat and the monitoring site, the boat type, the vessel traffic on the bridge, weather conditions, and the noise of buoy chains. Consequently, an attempt should be made to establish a noise calculation model to thoroughly assess the impact of various underwater acoustic sources on IPHD, including fish chorus, ship noise, and tunnel operation noise.
Data availability statement
The raw data supporting the conclusions of this article will be made available by the authors, without undue reservation.
Ethics statement
Ethical review and approval was not required for the animal study because In this study, noninvasive passive acoustic monitoring methods were used, and data were acquired from ambience without causing harm to the area dolphins.
Author contributions
XA: conceptualization, methodology, data curation, validation, investigation, and writing-original draft preparation. PD: data curation, investigation, and reviewing and editing. WL: software and reviewing and editing. JY: investigation and reviewing and editing. YC: investigation and reviewing and editing. FF: reviewing and editing, XD: reviewing and editing, YX: investigation and reviewing. XC: investigation and reviewing. DW: conceptualization, supervision and reviewing and editing. ZW: conceptualization, methodology and reviewing and editing, project administration. KW: supervised, conceptualization and reviewing and editing. All authors contributed to the article and approved the submitted version.
Funding
This research was supported by the National Natural Science Foundation of China (No. 41806197), Southern Marine Science and Engineering Guangdong Laboratory (Zhuhai) (No. SML2021SP202), the One Planet Nature Foundation project, projects from the Administration Bureau of Chinese White Dolphin National Nature Reserve, Guangdong and Monitoring and Analysis of Underwater Noise and Impact on Chinese White Dolphins during the Construction of Deep Water Channel Widening Project in Guangzhou Port (No. GZGHDTKGC- HTYXJC)
Acknowledgments
We are very grateful to Huantong Wan, Chaoming Zhang, and Yongxin Su from the Administration Bureau of the Nature Reserve for their assistance with field work and navigation; and the captain Yingqiang Li and divers Changqing Luo and Yuhao Zhang for their patience and active assistance to help us set up the instruments. We thank Haoran Ji for his guidance on statistics and graphing. We thank Dr. Xiaoling Wan for her revisions and suggestions on this manuscript. Special thanks are also extended to the academic editor and two reviewers for their helpful critique of an earlier version of this manuscript.
Conflict of interest
Author WL is employed by the company Wuhan Pindu Technology Co.
The remaining authors declare that the research was conducted in the absence of any commercial or financial relationships that could be construed as a potential conflict of interest.
Publisher’s note
All claims expressed in this article are solely those of the authors and do not necessarily represent those of their affiliated organizations, or those of the publisher, the editors and the reviewers. Any product that may be evaluated in this article, or claim that may be made by its manufacturer, is not guaranteed or endorsed by the publisher.
References
Au W. W. L., Hastings M. C. (2008). Principles of marine bioacoustics. (New York: Springer Science), 679 p.
Berrow S. D., Holmes B., Kiely O. R. (1996). Distribution and abundance of bottle-nosed dolphins tursiops truncatus (Montagu) in the Shannon estuary. Biol. Environment-Proceedings R. Irish Acad. 96B (1), 1–9. Available at: https://www.researchgate.net/publication/258918401
Blackwell S. B., Lawson J. W., Williams M. T. (2004). Tolerance by ringed seals (Phoca hispida) to impact pipe-driving and construction sounds at an oil production island. J. Acoustical Soc. America 115 (5), 2346–2357. doi: 10.1121/1.1701899
Carlstrom J. (2005). Diel variation in echolocation behavior of wild harbor porpoises. Mar. Mammal Sci. 21 (1), 1–12. doi: 10.1111/j.1748-7692.2005.tb01204.x
Caruso F., Dong L., Lin M., Liu M., Xu W., Li S. (2020). Influence of acoustic habitat variation on indo-pacific humpback dolphin (Sousa chinensis) in shallow waters of hainan island, China. J. Acoust Soc. Am. 147 (6), 3871. doi: 10.1121/10.0001384
Chan S. C., Karczmarski L. (2017). Indo-pacific humpback dolphins (Sousa chinensis) in Hong Kong: modelling demographic parameters with mark-recapture techniques. PloS One 12 (3), e0174029. doi: 10.1371/journal.pone.0174029
Chen T., Hung S. K., Qiu Y., Jia X., Jefferson T. A. (2010). Distribution, abundance, and individual movements of indo-pacific humpback dolphins (Sousa chinensis) in the pearl river estuary, China. mamm 74 (2), 117–125. doi: 10.1515/mamm.2010.024
Chen B. Y., Zheng D. M., Zhai F. F., Xu X., Sun P., Wang Q., et al. (2008). Abundance, distribution and conservation of Chinese white dolphins (Sousa chinensis) in xiamen, China. Mamm. Biol. 73 (2), 156–164. doi: 10.1016/j.mambio.2006.12.002
Conn P. B., Silber G. K. (2013). Vessel speed restrictions reduce risk of collision-related mortality for north Atlantic right whales. Ecosphere 4 (4), 1–16. doi: 10.1890/es13-00004.1
Currie J. J., Stack S. H., Kaufman G. D. (2017). Modelling whale-vessel encounters: the role of speed in mitigating collisions with humpback whales (Megaptera novaeangliae). J. Cetacean Res. Manage. 17, 57–63. Available at: https://www.researchgate.net/publication/280446928.
Davidson A. D., Boyer A. G., Kim H., Pompa-Mansilla S., Hamilton M. J., Costa D. P., et al. (2012). Drivers and hotspots of extinction risk in marine mammals. Proc. Natl. Acad. Sci. United States America 109 (9), 3395–3400. doi: 10.1073/pnas.1121469109
Deconto L. S., Monteiro-Filho E. L. A. (2015). Day and night sounds of the Guiana dolphin, sotalia guianensis (Cetacea: delphinidae) in southeastern Brazil. Acta ethologica 19 (1), 61–68. doi: 10.1007/s10211-015-0223-y
Dong L., Dong J., Caruso F., Zhao L., Li S. (2021). Temporal variation of the underwater soundscape in jiaotou bay, an indo-pacific humpback dolphin (Sousa chinensis) habitat off hainan island, China. Integr. Zool 16 (4), 477–498. doi: 10.1111/1749-4877.12530
Dong L., Liu M., Dong J., Li S. (2017). Acoustic occurrence detection of a newly recorded indo-pacific humpback dolphin population in waters southwest of hainan island, China. J. Acoust Soc. Am. 142 (5), 3198. doi: 10.1121/1.5011170
Duarte C. M., Chapuis L., Collin S. P., Costa D. P., Devassy R. P., Eguiluz V. M., et al. (2021). The soundscape of the anthropocene ocean. Science 371 (6529), 583. doi: 10.1126/science.aba4658
Erbe C., Marley S. A., Schoeman R. P., Smith J. N., Trigg L. E., Embling C. B. (2019). The effects of ship noise on marine mammals - a review. Front. Mar. Sci. 6. doi: 10.3389/fmars.2019.00606
Fang L., Li S., Wang K., Wang Z., Shi W., Wang D. (2015). Echolocation signals of free-ranging indo-pacific humpback dolphins (Sousa chinensis) in sanniang bay, China. J. Acoust Soc. Am. 138 (3), 1346–1352. doi: 10.1121/1.4929492
Finneran J. J., Schlundt C. E., Branstetter B. K., Trickey J. S., Bowman V., Jenkins K. (2015). Effects of multiple impulses from a seismic air gun on bottlenose dolphin hearing and behavior. J. Acoustical Soc. America 137 (4), 1634–1646. doi: 10.1121/1.4916591
Gauger M. F. W., Romero-Vivas E., Peck M. A., Balart E. F., Caraveo-Patiño J. (2022). Seasonal and diel influences on bottlenose dolphin acoustic detection determined by whistles in a coastal lagoon in the southwestern gulf of California. PeerJ 10, e13246. doi: 10.7717/peerj.13246
Gende S. M., Hendrix A. N., Harris K. R., Eichenlaub B., Nielsen J., Pyare S. (2011). A Bayesian approach for understanding the role of ship speed in whale-ship encounters. Ecol. Appl. 21 (6), 2232–2240. doi: 10.1890/10-1965.1
Gregorietti M., Papale E., Ceraulo M., de Vita C., Pace D. S., Tranchida G., et al. (2021). Acoustic presence of dolphins through whistles detection in Mediterranean shallow waters. J. Mar. Sci. Eng. 9 (1), 12. doi: 10.3390/jmse9010078
Guan S., Lin T. H., Chou L. S., Vignola J., Judge J., Turo D. (2015). Dynamics of soundscape in a shallow water marine environment: a study of the habitat of the indo-pacific humpback dolphin. J. Acoust Soc. Am. 137 (5), 2939–2949. doi: 10.1121/1.4919312
Hornby C. A., Hoover C., Iacozza J., Barber D. G., Loseto L. L. (2016). Spring conditions and habitat use of beluga whales (Delphinapterus leucas) during arrival to the Mackenzie river estuary. Polar Biol. 39 (12), 2319–2334. doi: 10.1007/s00300-016-1899-9
Hou B., Zeng Q., Li J. (2020). Underwater Acoustic Characteristics of High-Speed Railway Subsea Tunnel. J. Coast. Res. 110 (sp1). doi: 10.2112/jcr-si110-010.1
Hu W.-C., Siddagangaiah S., Chen C.-F., Pieretti N. (2022). Impact of vessel transit on vocalizations of the Taiwanese humpback dolphin. Diversity 14 (6), 426. doi: 10.3390/d14060426
Huang S.-L., Karczmarski L., Chen J., Zhou R., Lin W., Zhang H., et al. (2012). Demography and population trends of the largest population of indo-pacific humpback dolphins. Biol. Conserv. 147 (1), 234–242. doi: 10.1016/j.biocon.2012.01.004
Huang J., Sun D., Liu Y., Liu S., Shan B., Yang C., et al. (2018). Diversity of fish community in Sousa chinensis nature reserve of Pearl River Estuary. Journal of Southern Agriculture. 49 (5), 1000–1007. doi: 10.3969/j.issn.2095-1191.2018.05.25. [in Chinese with English abstract]
Hung S. K., Jefferson T. A. (2004). Ranging patterns of indo-pacific humpback dolphins (Sousa chinensis) in the pearl river estuary, people's republic of China. Aquat. Mammals 30 (1), 159–174. doi: 10.1578/am.30.1.2004.159
Jefferson T. A., Curry B. E. (2015). “Humpback dolphins: a brief introduction to the genus Sousa,” in Humpback dolphins. Eds. Jefferson T. A., Curry B. E. (San Diego: Elsevier Academic Press Inc), 1–16.
Jefferson T. A., Hung S. K. (2004). A review of the status of the indo-pacific humpback dolphin (Sousa chinensis) in Chinese waters. Aquat. Mammals 30 (1), 149–158. doi: 10.1578/am.30.1.2004.149
Jefferson T. A., Smith B. D. (2016). “Re-assessment of the conservation status of the indo-pacific humpback dolphin (Sousa chinensis) using the IUCN red list criteria,” in Humpback dolphins. Eds. Jefferson T. A., Curry B. E. (San Diego: Elsevier Academic Press Inc), 1–26.
Kruskal W. H., Wallis W. A. (1952). Use of ranks in one-criterion variance analysis. J. Am. Stat. Assoc. 47 (260), 583–621. doi: 10.1080/01621459.1952.10483441
Leunissen E. M., Rayment W. J., Dawson S. M. (2019). Impact of pile-driving on hector's dolphin in lyttelton harbour, new Zealand. Mar. pollut. Bull. 142, 31–42. doi: 10.1016/j.marpolbul.2019.03.017
Li S., Wang D., Wang K., Taylor E.A., Cros E., Shi W., et al. (2012). Evoked-potential audiogram of an Indo-Pacific humpback dolphin (Sousa chinensis). J Exp Biol 215(Pt 17), 3055–3063. doi: 10.1242/jeb.070904
Li S., Liu M., Dong L., Dong J., Wang D. (2018). Potential impacts of shipping noise on indo-pacific humpback dolphins and implications for regulation and mitigation: a review. Integr. Zool 13 (5), 495–506. doi: 10.1111/1749-4877.12304
Li S., Wu H., Xu Y., Peng C., Fang L., Lin M., et al. (2015). Mid- to high-frequency noise from high-speed boats and its potential impacts on humpback dolphins. J. Acoust Soc. Am. 138 (2), 942–952. doi: 10.1121/1.4927416
Liang F., Lin W., Guo L., Cai H., Pine M., and Wu Y (2020). Monitoring indo-pacific humpback dolphin occurrences in a highly urbanized estuary for informing conservation and management. Aquat. Conservation: Mar. Freshw. Ecosystems. 31 (4), 685–695. doi: 10.1002/aqc.3475
Lin T.-H., Akamatsu T., Chou L.-S. (2013). Tidal influences on the habitat use of indo-pacific humpback dolphins in an estuary. Mar. Biol. 160 (6), 1353–1363. doi: 10.1007/s00227-013-2187-7
Lin T.-H., Akamatsu T., Chou L.-S. (2014). Seasonal distribution of indo-pacific humpback dolphins at an estuarine habitat: influences of upstream rainfall. Estuaries Coasts 38 (4), 1376–1384. doi: 10.1007/s12237-014-9886-2
Liu M., Dong L., Lin M., Li S. (2017). Broadband ship noise and its potential impacts on indo-pacific humpback dolphins: implications for conservation and management. J. Acoust Soc. Am. 142 (5), 2766. doi: 10.1121/1.5009444
Liu M., Lin M., Lusseau D., Li S. (2021). Intra-population variability in group size of indo-pacific humpback dolphins (Sousa chinensis). Front. Mar. Sci. 8. doi: 10.3389/fmars.2021.671568
Mallin M. A., Paerl H. W., Rudek J. (1991). Seasonal phytoplankton composition, productivity and biomass in the neuse river estuary, north-carolina. Estuar. Coast. Shelf Sci. 32 (6), 609–623. doi: 10.1016/0272-7714(91)90078-p
Mann H. B., Whitney D. R. (1947). On a Test of Whether one of Two Random Variables is Stochastically Larger than the Other. Annals of Mathematical Statistics 18, 50–60.
Marley S. A., Salgado Kent C. P., Erbe C., Parnum I. M. (2017). Effects of vessel traffic and underwater noise on the movement, behaviour and vocalisations of bottlenose dolphins in an urbanised estuary. Sci. Rep. 7 (1), 13437. doi: 10.1038/s41598-017-13252-z
McKenna M. F., Wiggins S. M., Hildebrand J. A. (2013). Relationship between container ship underwater noise levels and ship design, operational and oceanographic conditions. Sci. Rep. 3 (1), 1760. doi: 10.1038/srep01760
Mendes S., Turrell W., Lutkebohle T., Thompson P. (2002). Influence of the tidal cycle and a tidal intrusion front on the spatio-temporal distribution of coastal bottlenose dolphins. Mar. Ecol. Prog. Ser. 239, 221–229. doi: 10.3354/meps239221
Merchant N. D., Pirotta E., Barton T. R., Thompson P. M. (2014). Monitoring ship noise to assess the impact of coastal developments on marine mammals. Mar. pollut. Bull. 78 (1-2), 85–95. doi: 10.1016/j.marpolbul.2013.10.058
Monteiro-Filho E. L. A., Deconto L. S., Louzada C. N., Wanderley R. P., Godoy D. F., Medeiros E. (2018). Long-term monitoring of dolphins in a Large estuarine system of southeastern Brazil. Coast. Res. Library 22, 15–40. doi: 10.1007/978-3-319-56985-7_2
Morton A. B., Symonds H. K. (2002). Displacement of orcinus orca (L.) by high amplitude sound in British Columbia, Canada. Ices J. Mar. Sci. 59 (1), 71–80. doi: 10.1006/jmsc.2001.1136
Munger L., Lammers M. O., Cifuentes M., Wursig B., Jefferson T. A., Hung S. K. (2016). Indo-pacific humpback dolphin occurrence north of lantau island, Hong Kong, based on year-round passive acoustic monitoring. J. Acoust Soc. Am. 140 (4), 2754. doi: 10.1121/1.4963874
Paitach R. L., Simoes-Lopes P. C., Cremer M. J. (2017). Tidal and seasonal influences in dolphin habitat use in a southern Brazilian estuary. Scientia Marina 81 (1), 49–56. doi: 10.3989/scimar.04495.25A
Philpott E., Englund A., Ingram S., Rogan E. (2007). Using T-PODs to investigate the echolocation of coastal bottlenose dolphins. J. Mar. Biol. Assoc. United Kingdom 87 (1), 11–17. doi: 10.1017/s002531540705494x
Pine M. K., Wang K., Wang D. (2017). Fine-scale habitat use in indo-pacific humpback dolphins,Sousa chinensis, may be more influenced by fish rather than vessels in the pearl river estuary, China. Mar. Mammal Sci. 33 (1), 291–312. doi: 10.1111/mms.12366
Popper A. N., Fay R. R., Platt C., Sand O. (2003). Sound detection mechanisms and capabilities of teleost fishes (New York: Springer-Verlag). 3–38
Reeder D. B., Joseph J. E., Rago T. A. (2020). Underwater sound generated by motor vehicle traffic in an underwater tunnel. J. Acoust Soc. Am. 148 (3), EL215. doi: 10.1121/10.0001805
Richardson W. J., Green C. R. Jr., Malme C. I., Thomson D. H., Richardson W. J., Green C. R. Jr., et al. (1995). Marine mammals and noise (San Diego: Academic Press). 576 p.
Schoeman R. P., Patterson-Abrolat C., Plön S. (2020). A global review of vessel collisions with marine animals. Front. Mar. Sci. 7. doi: 10.3389/fmars.2020.00292
Sims P. Q., Hung S. K., Würsig B. (2012). High-speed vessel noises in West Hong Kong waters and their contributions relative to indo-pacific humpback dolphins (Sousa chinensis). J. Mar. Biol. 2012, 1–11. doi: 10.1155/2012/169103
Sueur J., Farina A. (2015). Ecoacoustics: the ecological investigation and interpretation of environmental sound. Biosemiotics 8 (3), 493–502. doi: 10.1007/s12304-015-9248-x
Thompson P. M., Brookes K. L., Cordes L. S. (2015). Integrating passive acoustic and visual data to model spatial patterns of occurrence in coastal dolphins. Ices J. Mar. Sci. 72 (2), 651–660. doi: 10.1093/icesjms/fsu110
Todd N. R. E., Cronin M., Luck C., Bennison A., Jessopp M., Kavanagh A. S. (2020). Using passive acoustic monitoring to investigate the occurrence of cetaceans in a protected marine area in northwest Ireland. Estuar. Coast. Shelf Sci. 232, 8. doi: 10.1016/j.ecss.2019.106509
Todd V. L. G., Pearse W. D., Tregenza N. C., Lepper P. A., Todd I. B. (2009). Diel echolocation activity of harbour porpoises (Phocoena phocoena) around north Sea offshore gas installations. Ices J. Mar. Sci. 66 (4), 734–745. doi: 10.1093/icesjms/fsp035
Vanderlaan A. S. M., Taggart C. T. (2007). Vessel collisions with whales: the probability of lethal injury based on vessel speed. Mar. Mammal Sci. 23 (1), 144–156. doi: 10.1111/j.1748-7692.2006.00098.x
Wang Z. T., Akamatsu T., Duan P. X., Zhou L., Yuan J., Li J., et al. (2020). Underwater noise pollution in china's Yangtze river critically endangers Yangtze finless porpoises (Neophocaena asiaeorientalis asiaeorientalis). Environ. pollut. 262, 10. doi: 10.1016/j.envpol.2020.114310
Wang Z. T., Akamatsu T., Mei Z., Dong L., Imaizumi T., Wang K., et al. (2015a). Frequent and prolonged nocturnal occupation of port areas by Yangtze finless porpoises (Neophocaena asiaeorientalis): forced choice for feeding? Integr. Zool 10 (1), 122–132. doi: 10.1111/1749-4877.12102
Wang Z. T., Akamatsu T., Nowacek D. P., Yuan J., Zhou L., Lei P. Y., et al. (2019). Soundscape of an indo-pacific humpback dolphin (Sousa chinensis) hotspot before windfarm construction in the pearl river estuary, China: do dolphin engage in noise avoidance and passive eavesdropping behavior? Mar. pollut. Bull. 140, 509–522. doi: 10.1016/j.marpolbul.2019.02.013
Wang Z., Akamatsu T., Wang K., Wang D. (2014a). The diel rhythms of biosonar behavior in the Yangtze finless porpoise (Neophocaena asiaeorientalis asiaeorientalis) in the port of the Yangtze river: the correlation between prey availability and boat traffic. PloS One 9 (5), e97907. doi: 10.1371/journal.pone.0097907
Wang D., Lin Z. J. (2006). Spatial and temporal variations of fish community structure in the pearl river estuary waters. South China Fisheries Sci. 4, 37–45.
Wang Z. T., Nachtigall P. E., Akamatsu T., Wang K. X., Wu Y. P., Liu J. C., et al. (2015b). Passive acoustic monitoring the diel, lunar, seasonal and tidal patterns in the biosonar activity of the indo-pacific humpback dolphins (Sousa chinensis) in the pearl river estuary, China. PloS One 10 (11), e0141807. doi: 10.1371/journal.pone.0141807
Wang Z. T., Wu Y., Duan G., Cao H., Liu J., Wang K., et al. (2014b). Assessing the underwater acoustics of the world's largest vibration hammer (OCTA-KONG) and its potential effects on the indo-pacific humpbacked dolphin (Sousa chinensis). PloS One 9 (10), e110590. doi: 10.1371/journal.pone.0110590
Xiao Y. (2020). Protection of indo-pacific humpback dolphins during the construction and operation periods of the Hong Kong-Zhuhai-Macao bridge. China Fisheries 01, 57–59.
Xu W., Dong L., Caruso F., Gong Z., Li S. (2020). Long-term and large-scale spatiotemporal patterns of soundscape in a tropical habitat of the indo-pacific humpback dolphin (Sousa chinensis). PloS One 15 (8), e0236938. doi: 10.1371/journal.pone.0236938
Yamagiwa J., Karczmarski L. (2014). Primates and cetaceans: field research and conservation of complex mammalian societies – 2nd reprint of the original, 1st edition. (Tokyo: Springer). 249–272.
Yuan J., Wang Z., Duan P., Xiao Y., Zhang H., Huang Z., et al. (2021). Whistle signal variations among three indo-pacific humpback dolphin populations in the south China Sea: a combined effect of the qiongzhou strait's geographical barrier function and local ambient noise? Integr. Zoology 16 (4), 499–511. doi: 10.1111/1749-4877.12531
Keywords: Indo-Pacific humpback dolphin, passive acoustic monitoring (PAM), sonar activity, underwater noise, Hong Kong-Zhuhai-Macao Bridge
Citation: An X, Duan P, Li W, Yuan J, Chen Y, Fan F, Deng X, Xiao Y, Chen X, Wang D, Wang Z and Wang K (2023) Biosonar activity of the Indo-Pacific humpback dolphin (Sousa chinensis) near the tunnel section of the world’s longest cross-sea bridge—the Hong Kong-Zhuhai-Macao Bridge—is negatively correlated with underwater noise. Front. Mar. Sci. 10:1171709. doi: 10.3389/fmars.2023.1171709
Received: 22 February 2023; Accepted: 10 May 2023;
Published: 23 May 2023.
Edited by:
Roberto Carlucci, University of Bari Aldo Moro, ItalyReviewed by:
Xian Sun, Sun Yat-sen University, ChinaBernd Würsig, Texas A&M University Commerce, United States
Copyright © 2023 An, Duan, Li, Yuan, Chen, Fan, Deng, Xiao, Chen, Wang, Wang and Wang. This is an open-access article distributed under the terms of the Creative Commons Attribution License (CC BY). The use, distribution or reproduction in other forums is permitted, provided the original author(s) and the copyright owner(s) are credited and that the original publication in this journal is cited, in accordance with accepted academic practice. No use, distribution or reproduction is permitted which does not comply with these terms.
*Correspondence: Zhitao Wang, d2FuZ3poaXRhb0BuYnUuZWR1LmNu; Kexiong Wang, d2FuZ2tAaWhiLmFjLmNu