- 1Cooperative Institute for Marine and Atmospheric Studies, University of Miami, Miami, FL, United States
- 2Southeast Fisheries Science Center, National Oceanic and Atmospheric Administration (NOAA)-National Marine Fisheries Service, Miami, FL, United States
- 3Rosenstiel School of Marine, Atmospheric, and Earth Science, University of Miami, Miami, FL, United States
- 4Coral Restoration Foundation, Tavernier, FL, United States
Here, we provide the first reports of spawning activity by Acropora palmata colonies outplanted to reefs in Florida, USA. In 2020, we observed light spawning from A. palmata colonies five years after they had been outplanted on two Florida reefs. In 2021 and 2022, we observed outplanted A. palmata colonies spawning synchronously with other nearby (<3 m) outplants and wild colonies more than 100 m away. During the 2022 spawning event, some colonies spawned in as few as four years after they had been outplanted. Among all spawning seasons, gametes collected from the outplanted colonies yielded high fertilization rates and viable larvae. These observations are promising for A. palmata restoration as they indicate fragments of A. palmata can spawn four years after outplanting and that efforts to restore A. palmata may be close to achieving the first step towards self-sustaining populations that can produce viable larvae, resulting in an increase in the population’s genotypic diversity upon successful recruitment to the reef.
1 Introduction
Acropora palmata (elkhorn coral) is a branching species of coral that, historically, built large monotypic thickets on Caribbean/Atlantic reefs. This species reproduces asexually through fragmentation and sexually by broadcasting eggs and sperm synchronously allowing fertilization between neighboring genotypes to occur at the surface. Populations of this once ubiquitous coral have declined rapidly over the past 40 years leading to its listing as Threatened under the U.S. Endangered Species Act (NMFS, 2006). The decline of A. palmata began as human impacts on coral reef ecosystems increased in the 1950s (Cramer et al., 2021) and accelerated dramatically in the 1980s due to an Acropora-specific disease outbreak that spread throughout the Atlantic basin (Porter and Meier, 1992; Green and Bruckner, 2000). These dramatic losses both contributed to and were further exacerbated by the general collapse of Caribbean/Atlantic reef ecosystems (Jackson et al., 2001). The declining population has become increasingly vulnerable to depensatory factors, which are compromising this species’ ability to reproduce both asexually and sexually. As A. palmata thickets become less dense, storm-generated fragments are not retained on the reef, and the increasingly sparse colonies are too far apart to allow for gametes to fertilize during spawning (Williams et al., 2014; Miller et al., 2016). Alongside the decreasing abundance, the genotypic diversity of the present-day wild A. palmata population on Florida reefs has dramatically declined in the past 15 years (Williams et al., 2020) greatly reducing the chances of successful fertilization during spawning events. Compounding the loss of abundance and genotypic diversity, the timing of spawning can vary among A. palmata genotypes, resulting in even fewer genotypes that spawn synchronously (Miller et al., 2016). Additionally, genotypes that do spawn synchronously may have incompatible gametes (Miller et al., 2018), further hampering the potential for reproductive success.
Outplanting nursery-propagated A. palmata is increasingly implemented (Bostrom-Einarsson et al., 2020) as a tool to recover diminished populations on Atlantic/Caribbean reefs. Ultimately, the goal of these efforts is to restore genotypically diverse coral populations containing reproductively mature colonies that contribute to self-sustaining populations via the production of offspring that successfully recruit into the population. While outplanting coral colonies helps to restore live coral abundance on degraded reefs, outplanted colonies in Florida are mostly produced via asexual fragmentation, which does not increase the genotypic diversity of a population. Augmenting genotypic diversity is particularly important for species like A. palmata, with an estimated <150 unique genotypes remaining in the entire Florida population (D. Williams unpubl data). Consequently, successful recruitment of offspring from reproductive events is paramount for threatened species like A. palmata.
To date, published observations of spawning in outplanted Atlantic acroporid species have only been reported in the Caribbean (Carne et al., 2016; Chamberland et al., 2016; Calle-Triviño et al., 2018). However, there have been no reports in Florida of spawning activity by outplanted A. palmata. Here, we report spawning observations of A. palmata colonies outplanted as part of coral restoration and research efforts at two reef sites in the Upper Florida Keys, USA. The corals were outplanted in 2015, 2016, and 2018, and monitored for spawning activity during the predicted spawning windows from 2019 to 2022. In 2020, 2021, and 2022, we observed spawning activity among the outplanted colonies of A. palmata. These are the first reported observations of spawning activity by A. palmata outplanted to reefs in Florida, an important milestone for efforts to restore populations of this ESA-listed coral species.
2 Materials and methods
Outplanting of Acropora palmata fragments was conducted during three different years as part of three unrelated projects at two reefs in the Upper Florida Keys, USA: North Dry Rocks Reef (NDR; 25.13°, -80.29°) and Elbow Reef (EL; 25.14°, -80.26°). Both EL and NDR reefs are characterized as high-relief spur-and-groove habitats with extant colonies of A. palmata (henceforth “wild” colonies) present at the time of coral outplanting. All outplants monitored for spawning were generated from fragments of A. palmata propagated by the Coral Restoration Foundation in their off-shore nursery in Tavernier, Florida. In 2015 and 2016, corals were outplanted jointly by the Coral Research and Assessment Lab at NOAA’s Southeast Fisheries Science Center and the Coral Restoration Foundation, and in March 2018, additional corals were outplanted by the Coral Restoration Foundation. For all three sets of outplants, the fragments were 7–10 cm in diameter at the time of outplanting and were attached to the reef using two-part marine epoxy.
In May 2015 at NDR, we outplanted 120 A. palmata fragments of four genotypes. Fragments were outplanted into 30 clusters of four fragments approximately 50 cm apart, with one fragment of each genotype present in each cluster. In June 2016 at EL, we outplanted fragments in twelve 3 m x 2 m plots, with 24 fragments outplanted in each plot. Fragments within the plots were spaced 30 cm apart in various arrangements of two or eight genotypes (Figure 1). In 2018 at NDR, 50 genotypes of A. palmata were outplanted in 150 clusters of seven fragments within a plot ~50 cm diameter in size along a single reef spur. All fragments within a cluster were the same genotype. Fragments for outplanting were propagated as putative genotypes (Tables 1, 2) that were later identified using SNP genotyping (Kitchen et al., 2020). SNP genotyping was also used to genotype the wild A. palmata colonies found within ~100m of the outplanted colonies at both sites (Tables 1, 2).
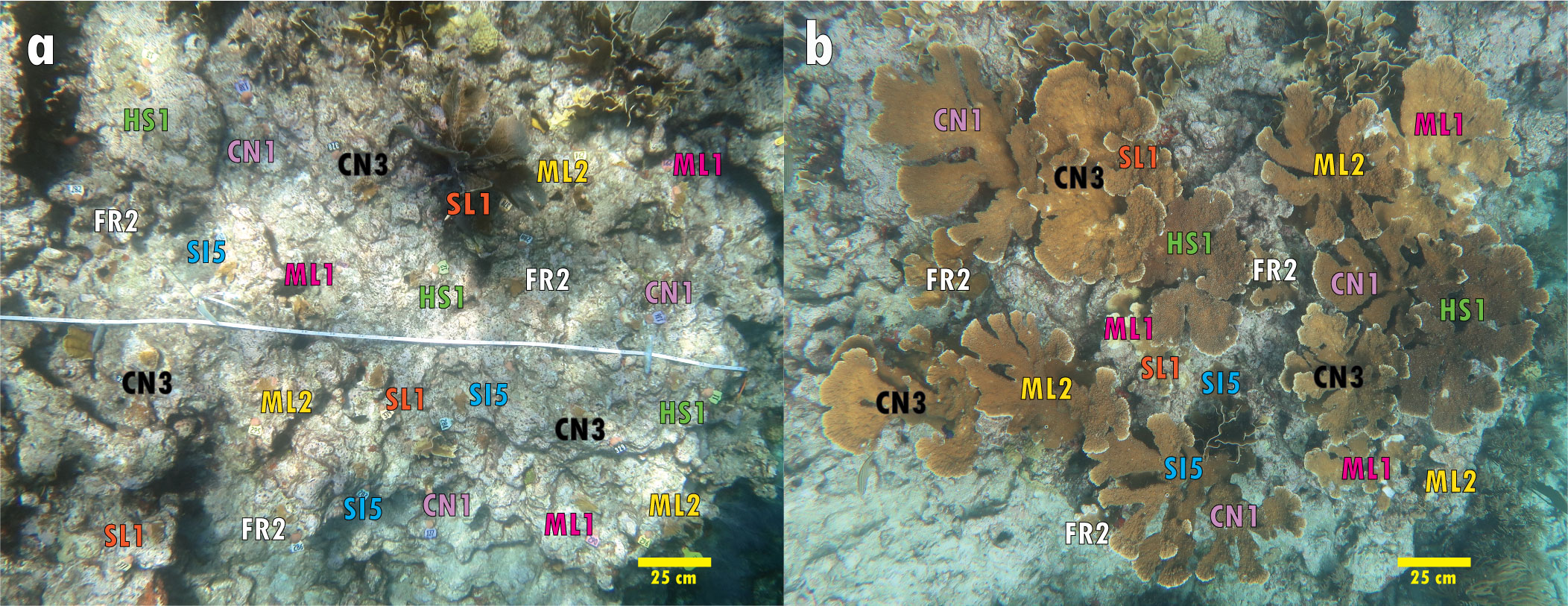
Figure 1 Outplant plot (3 m x 2 m) at Elbow Reef in the Florida Keys at the time of outplanting in (A) May 2016 and 5 years later in (B) July 2021. This plot includes eight interspersed nursery-raised genotypes, five of which were observed to spawn in July 2021.
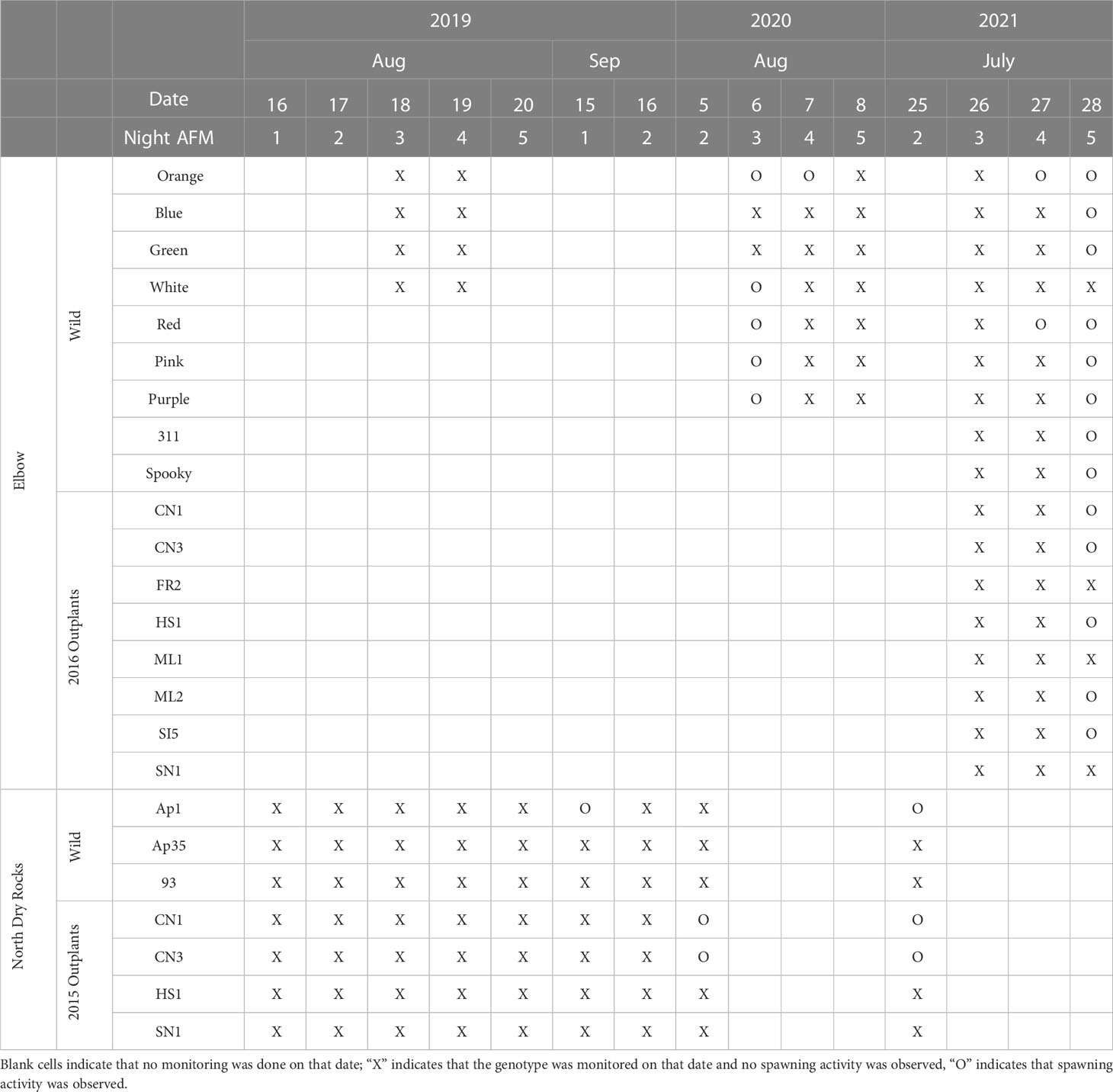
Table 1 Acropora palmata spawning observations for known genotypes during the predicted spawning windows (Nights 1-5 after the July and August full moon [AFM]) from 2019 to 2021 at Elbow and North Dry Rocks reefs.
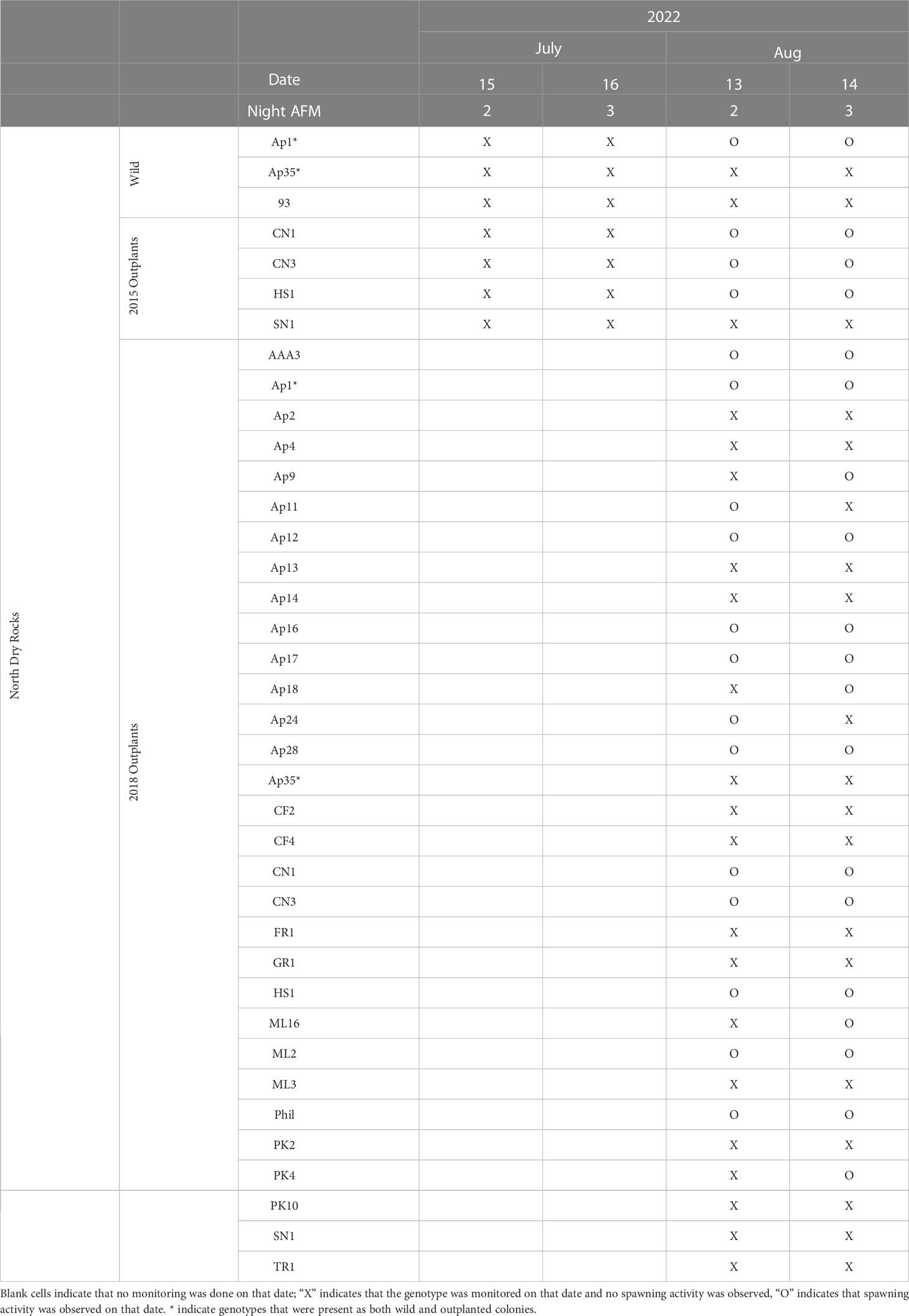
Table 2 Acropora palmata spawning observations for known genotypes at North Dry Rocks reef during the predicted spawning windows (Nights 2-3 after the July and August full moon [AFM]) in 2022.
The expected A. palmata spawning window is two to five nights after the July or August full moon based on more than ten years of observations on the upper Florida Keys Reef Tract (Miller et al., 2016). Using methods described by Miller et al. (2016), four to six divers monitored for spawning activity between 21:45 and 23:00 local time during the expected windows from 2019 to 2022 as shown in Tables 1, 2.
In 2019, we monitored wild and 2015 A. palmata outplants at NDR on nights 1–5 after the full moon (AFM) in August and nights 1–2 AFM in September, and wild colonies at EL on nights 3–4 AFM in August. In August 2020, we monitored wild and 2015 A. palmata outplants at NDR on night 2 AFM and wild colonies at EL on nights 3–5 AFM (Table 1). We did not monitor the 2016 A. palmata outplants at EL in 2020. In July 2021, we monitored wild and 2015 A. palmata outplants at NDR on night 2 AFM, and wild and 2016 A. palmata outplants at EL on nights 3–5 AFM. In July 2022, we monitored wild and 2015 A. palmata outplants at NDR on nights 3–4 AFM. In August 2022, we monitored the same wild and 2015 A. palmata outplants on nights 2-3 AFM along with 31 of the 50 genotypes of the 2018 A. palmata outplants.
During monitoring events, when bundle setting was observed (Miller et al., 2016), the divers placed collector nets (Miller et al., 2018) on corals and collected the gametes in 50 ml centrifuge tubes. Gametes were combined on the boat and transported to the lab in Miami, Florida, USA. Fertilization rates were determined by counting the number of embryos and unfertilized eggs (Miller et al., 2018) ~8 hours after the gametes were mixed.
3 Results
In 2019, no Acropora palmata spawning activity was observed at either NDR or EL in the monitoring window (Table 1). In July 2020, at NDR on night 2 AFM, we observed light spawning from two outplanted A. palmata colonies belonging to two genotypes (CN1 and CN3; Table 1). At EL, we observed spawning activity by five of the seven monitored wild genets on night 3 AFM, and we observed spawning by one genet on night 4 AFM. Gametes from the two NDR outplanted genotypes were combined and yielded 90% fertilization, and the resulting larvae survived and settled in the lab.
In July 2021, at NDR on night 2 AFM, spawning activity was observed in one wild genet and multiple colonies of the same two 2015 outplant genotypes that were observed in 2020 (CN1 and CN3; Table 1). Gametes from wild and outplanted genotypes (n=3) were combined. At EL on night 5 AFM, we observed synchronous spawning activity from five of eight A. palmata genotypes outplanted in 2016 (Figure 1) along with eight of the nine known wild genets. Gametes from the EL wild and outplanted genotypes (n=12) were combined. Batches of gametes from both sites yielded fertilization rates > 90%, and the resulting larvae survived and settled in the lab.
In July 2022, no spawning activity was observed. In August 2022, spawning activity was observed in 17 of the 31 genotypes monitored at NDR on night 2 and/or 3 AFM (Figure 2; Table 2). Two of the three wild genets monitored at NDR, Ap1 and Ap35, were also represented among the 31 outplanted genotypes that were monitored. Of those two genotypes, we observed both wild and outplanted colonies of only Ap1 spawn on nights 2 and 3 AFM. The timing of spawning by Ap1 colonies was synchronous between outplanted and wild colonies of this single genet and with colonies of other genotypes. No spawning was observed in the wild or outplanted colonies of Ap35 on either night (Table 2). Gametes collected from NDR 2018 outplants were combined in three separate batches that included 5–6 parent genotypes each. All three batches yielded fertilization rates > 90%, and the resulting larvae survived and settled in the lab.
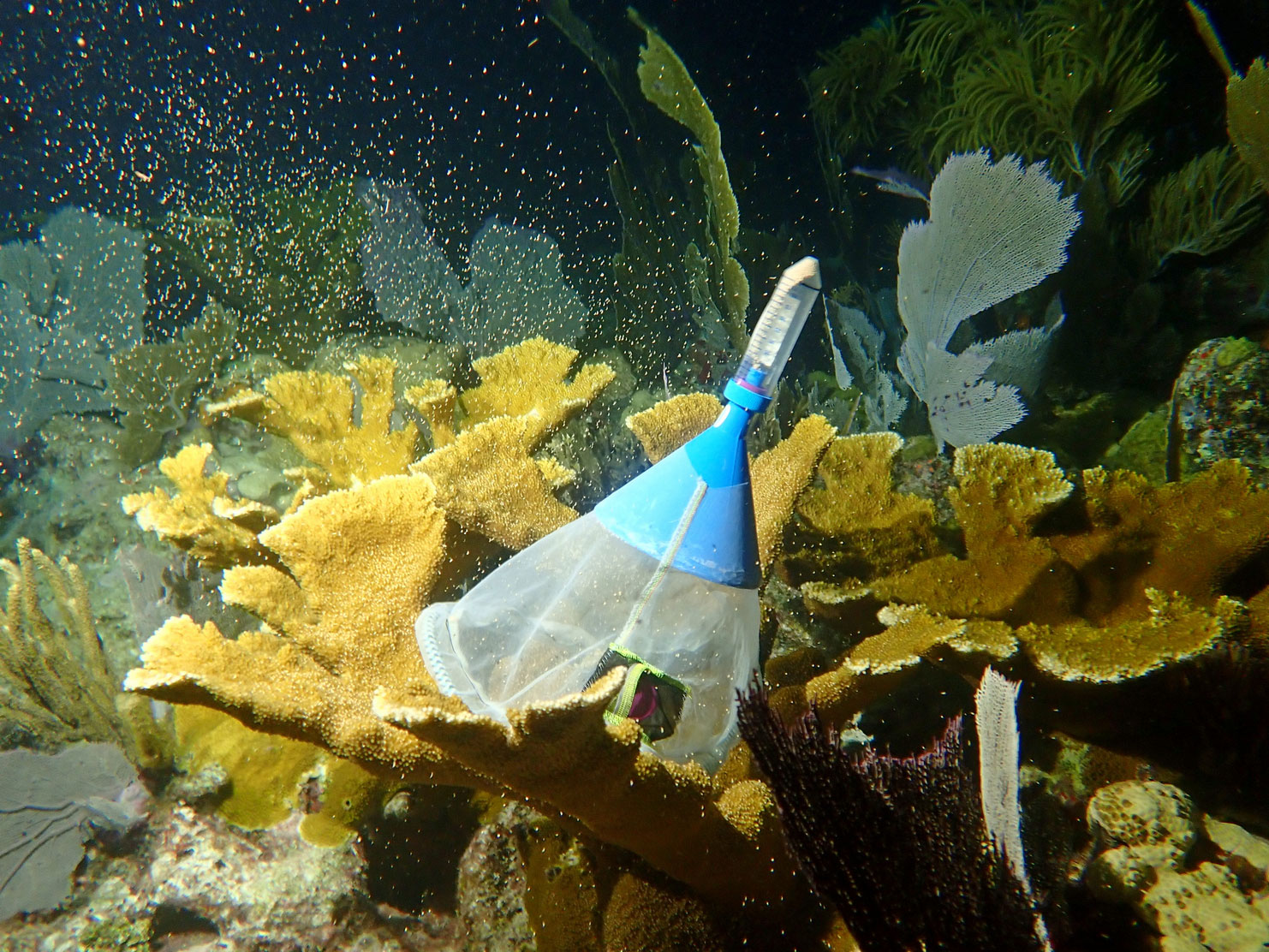
Figure 2 Acropora palmata outplanted in 2015 at North Dry Rocks in the Florida Keys releasing gamete bundles in August 2022. Small gamete collection nets are used to collect the bundles to create larval cultures on shore.
4 Discussion
Here, we provide the first reports of spawning by outplanted Acropora palmata colonies outplanted to reefs in Florida, USA. Spawning activity was first observed five years and two months (62 months) after the corals were outplanted, in the summer of 2020. In 2022, we observed spawning activity among A. palmata colonies outplanted in March 2018, indicating that nursery-raised fragments outplanted to Florida reefs can become reproductively mature in as little as four years and four months (52 months) after outplanting. This is consistent with the observation of A. palmata outplants spawning ~ 60 months post outplanting in Belize (Carne et al., 2016) and four-year-old (48 months) sexual recruits of A. palmata being reproductively mature in Curaçao (Chamberland et al., 2016) and other species of acroporids spawning at 4-5 years old (Calle-Triviño et al., 2018; Ligson and Cabaitan, 2021).
Gamete compatibility can vary widely among parent combinations of A. palmata, including combinations that are incompatible or have low fertilization rates (Baums et al., 2013; Miller et al., 2018). The successful development of larvae from batch cultures indicates reproductive compatibility among multiple A. palmata genets that spawned synchronously at each reef site. The spawning observed in 2021 included 12 genotypes sourced from five different Upper Florida Keys reefs, while the spawning observed in 2022 included 17 genotypes sourced from 12 reefs spanning 200 km of the Florida Reef Tract.
On many Florida reefs, Allee effects (Gascoigne and Lipcius, 2004) likely inhibit sexual reproduction because many stands consist of a single A. palmata genotype that is of reproductive size (Williams et al., 2020), and the distance between stands often makes it unlikely that fertilization will occur. The 2016 outplants at EL included eight genotypes, five of which spawned, effectively representing greater genotypic diversity within a single patch than can be found on present-day Florida reefs (Williams et al., 2020). Moreover, the presence of 13–15 spawning genotypes among the 2018 outplants is two- to three-fold higher than the genotypic diversity that was found on a single spur, even in 2006, which is the earliest analysis of genotypic diversity of A. palmata on Florida reefs (Williams et al., 2014). Thus, the spawning observed for A. palmata in two consecutive years and at two reef sites represents a substantial increase in the number of individual genotypes contributing to a reproductive event. These observations indicate that restoration via outplanting is a viable strategy to enhance reproductive events in ESA-listed coral populations on small (i.e. reef; 10–100m) scales.
4.1 Conclusions
These spawning observations coupled with successful fertilization, larval development, and settlement in the lab constitute an important milestone in efforts to restore populations of the ESA-listed coral species Acropora palmata. Synchronous spawning among colonies of A. palmata outplanted for restoration alongside wild colonies on reefs in Florida suggests that restoration efforts focused on this species are closer to the longer-term goal of restoring reproductively mature colonies capable of producing viable larvae and contributing to self-sustaining populations.
However, there has been no evidence of successful sexual recruitment of A. palmata to reefs in the Florida Keys in recent decades (Williams et al., 2008; Williams et al., 2014). This could result from poor recruitment success due to numerous factors (Ritson-Williams et al., 2009), including a hostile recruitment environment or insufficient reproductively mature colonies to produce the necessary threshold of viable larvae. With current trends of decreasing coral cover and increasing reef stressors, genotypically diverse populations are necessary to ensure the future success of a threatened population. Continued restoration efforts that result in reproductively mature individuals that contribute to the gene pool are an imperative first step to promote the likelihood of successful sexual recruitment to the reef.
Data availability statement
The original contributions presented in the study are included in the article. Further inquiries can be directed to the corresponding author.
Author contributions
DW, AB, OW, and ML contributed to the spawning observations. AM, DW, and AB contributed to the design and outplanting of the 2015 and 2016 outplanted corals. AM provided information on the putative genotype identification of outplanted corals and directed the outplanting of the 2018 corals. DW and ML wrote the first draft of the manuscript. All authors contributed to manuscript revision, read, and approved the submitted version.
Funding
Spawning observations and 2015 and 2016 outplanting activities were funded by NOAA’s Coral Reef Conservation Program and the Coral Restoration Foundation. The 2018 outplants were funded by NOAA Fisheries Restoration Center. Publication fees provided by the Cooperative Institute for Marine & Atmospheric Studies.
Acknowledgments
Spawning observations and collections were permitted by Florida Keys National Marine Sanctuary under permit FKNMS-2018-163. Outplanting activities were permitted under FKNMS-2011-159 (2015 and 2016) and FKNMS-2011-159-A4 (2018). We thank the numerous spawning field observers from the University of Miami Rosenstiel School of Marine, Atmospheric, and Earth Science, SECORE, Florida Aquarium, and Coral Restoration Foundation.
Conflict of interest
The authors declare that the research was conducted in the absence of any commercial or financial relationships that could be construed as a potential conflict of interest.
Publisher’s note
All claims expressed in this article are solely those of the authors and do not necessarily represent those of their affiliated organizations, or those of the publisher, the editors and the reviewers. Any product that may be evaluated in this article, or claim that may be made by its manufacturer, is not guaranteed or endorsed by the publisher.
References
Baums I. B., Devlin-Durante M. K., Polato N. R., Xu D. (2013). Genotypic variation influences reproductive success and thermal stress tolerance in the reef building coral, Acropora palmata. Coral Reefs. 32, 703–713. doi: 10.1007/s00338-013-1012-6
Bostrom-Einarsson L., Babcock R. C., Bayraktarov E., Ceccarelli D. (2020). Coral restoration - a systematic review of current methods, successes, failures and future directions. PloS One 15, e0226631. doi: 10.1371/journal.pone.0226631
Calle-Triviño J., Cortés-Useche C., Sellares-Blasco R. I., Arias-González J. E. (2018). Assisted fertilization of threatened staghorn coral to complement the restoration of nurseries in southeastern Dominican republic. Regional Stud. Mar. Sci. 18, 129–134. doi: 10.1016/j.rsma.2018.02.002
Carne L., Kaufman L., Scavo K. (2016). Measuring success for Caribbean acroporid restoration: key results from ten years of work in southern Belize. Proc. Int. Coral Reef Symp, 342–358. Available at: https://coralreefs.org/wp-content/uploads/2019/01/Session-42-B-2-Carne-ICRS-clean-and-final-ATB1.pdf.
Chamberland V., Petersen D., Latijnhouwers K., Snowden S., Mueller B., Vermeij M. (2016). Four-year-old Caribbean Acropora colonies reared from field-collected gametes are sexually mature. Bull. Mar. Sci. 92, 263–264. doi: 10.5343/bms.2015.1074
Cramer K. L., Donovan M. K., Jackson J. B. C., Greenstein B. J. (2021). The transformation of Caribbean coral communities since humans. Ecol. Evol. 11, 10098–10118. doi: 10.1002/ece3.7808
Gascoigne J., Lipcius R. N. (2004). Allee effects in marine systems. Mar. Ecol. Prog. Ser. 269, 49–59. doi: 10.3354/meps269049
Green E. P., Bruckner A. W. (2000). The significance of coral disease epizootiology for coral reef conservation. Biol. Conserv. 96, 347–361. doi: 10.1016/S0006-3207(00)00073-2
Jackson J. B. C., Kirby M. X., Berger W. H., Bjorndal K. A. (2001). Historical overfishing and the recent collapse of coastal ecosystems. Science 293, 629–638. doi: 10.1126/science.1059199
Kitchen S. A., Von Kuster G., Kuntz K. L. V., Reich H. G. (2020). STAGdb: a 30K SNP genotyping array and science gateway for acropora corals and their dinoflagellate symbionts. Sci. Rep. 10, 12488. doi: 10.1038/s41598-020-69101-z
Ligson C. A., Cabaitan P. C. (2021). Survival and sexual maturity of sexually propagated Acropora verweyi corals 4 years after outplantation. Restor. Ecol. 29, e13363. doi: 10.1111/rec.13363
Miller M. W., Baums I. B., Pausch R. E., Bright A. J. (2018). Clonal structure and variable fertilization success in Florida keys broadcast-spawning corals. Coral Reefs 37, 239–249. doi: 10.1007/s00338-017-1651-0
Miller M. W., Williams D. E., Fisch J. (2016). Genet-Specific spawning patterns in Acropora palmata. Coral Reefs 35, 1393–1398. doi: 10.1007/s00338-016-1472-6
NMFS (2006). Endangered and threatened species: final listing determinations for elkhorn coral and staghorn coral. Fed. Reg. 71, 26852–26872.
Porter J. W., Meier O. W. (1992). Quantification of loss and change in Floridian reef coral populations. Am. Zool 32, 625–640. doi: 10.1093/icb/32.6.625
Ritson-Williams R., Arnold S. N., Fogarty N. D., Steneck R. S. (2009). New perspectives on ecological mechanisms affecting coral recruitment on reefs. Smithsonian Contributions to Mar. Sci. 38, 437–457. doi: 10.5479/si.01960768.38.437
Williams D. E., Miller M. W., Baums I. B. (2014). Cryptic changes in the genetic structure of a highly clonal coral population and the relationship with ecological performance. Coral Reefs 33, 595–606. doi: 10.1007/s00338-014-1157-y
Williams D. E., Miller M. W., Kramer K. L. (2008). Recruitment failure in Florida keys Acropora palmata, a threatened Caribbean coral. Coral Reefs 27, 697–705. doi: 10.1007/s00338-008-0386-3
Keywords: coral restoration, coral spawning, genotypic diversity, Acropora palmata, Florida (USA), Caribbean corals, outplanting
Citation: Williams DE, Bright AJ, Williamson OM, Moura A and Ladd MC (2023) Synchronous spawning of nursery-raised elkhorn coral (Acropora palmata) outplanted to reefs in the Florida Keys (United States). Front. Mar. Sci. 10:1180996. doi: 10.3389/fmars.2023.1180996
Received: 06 March 2023; Accepted: 08 June 2023;
Published: 06 July 2023.
Edited by:
Thierry Work, United States Department of the Interior, United StatesReviewed by:
Robert H. Richmond, University of Hawaii at Manoa, United StatesChe-Hung Lin, Academia Sinica, Taiwan
Copyright © 2023 Williams, Bright, Williamson, Moura and Ladd. This is an open-access article distributed under the terms of the Creative Commons Attribution License (CC BY). The use, distribution or reproduction in other forums is permitted, provided the original author(s) and the copyright owner(s) are credited and that the original publication in this journal is cited, in accordance with accepted academic practice. No use, distribution or reproduction is permitted which does not comply with these terms.
*Correspondence: Dana E. Williams, ZGFuYS53aWxsaWFtc0Bub2FhLmdvdg==