Seasonal variation and nutrient jointly drive the community structure of macrophytes in lakes with different trophic states
- 1State Key Laboratory of Freshwater Ecology and Biotechnology, Institute of Hydrobiology, Chinese Academy of Sciences, Wuhan, China
- 2University of Chinese Academy of Sciences, Beijing, China
- 3The Guangxi Key Laboratory of Theory and Technology for Environmental Pollution Control, Guilin University of Technology, Guilin, China
Introduction: Macrophytes are essential for maintaining the health of shallow lake ecosystems, however, the driving and responsive relationship between ecological factors (such as seasonal changes and nutrition, etc.) and plant communities is not yet clear.
Methods: In this study, we conducted seasonal surveys of macrophyte community composition in lakes with different nutrient states, aiming to understand the incidence relation between macrophyte community diversity, seasonal changes and environmental factors.
Results: According to the classification criteria of comprehensive nutritional index, there were significant differences in the trophic status of the three lakes. Among them, the Xihu Lake has reached mild eutrophication with a TLI value of 56.33, both Cibi Lake and Haixihai Lake are mesotrophic with TLI value of 36.03 and 33.48, respectively. The results of diversity analysis showed a significant negative correlation between α-diversity (include Species richness, Shannon-Wiener index, Simpson index and Pielou index) and lake nutrient status. Among them, Xihu Lake showed the lowest α-diversity in all seasons, Haixihai Lake exhibited the middle α-diversity, Cibi Lake indicated the highest α-diversity. Non-metric multidimensional ordination showed that there were obvious spatial structures differences among the macrophyte communities in the three lakes. Macrophyte community composition in the three lakes was more similar in summer and autumn, but there was a wider gap in spring and winter. The redundancy analysis indicated distinct differences between diversity index and ecological factors, the eigenvalues of Axis 1 and Axis 2 being, respectively, 36.13% and 8.15%. Environmental factors could explain 44.8% of the total variation in macrophyte communities structure. Among these, nitrogen, phosphorus, water transparency and water temperature contributed 50.2%, 3.5%, 3.8% and 27.5%, respectively.
Conclusions: In summary, the community structure of macrophytes in plateau shallow lakes is co-regulated by seasons and nutrients.
1 Introduction
The composition of macrophyte communities has a great impact on lake ecosystems, especially in shallow lakes (Carpenter and Lodge, 1986; Blindow et al., 1998; Sondergaard et al., 2013), as they not only limit sediment resuspension (Zuccarini et al., 2011), and also play a crucial role in sediment flow, accumulation and stability (Carpenter, 1980). They are responsible for an important part of the primary productivity of the lakeshore zone, providing complex habitats and food for aquatic organisms, ranging from macroinvertebrates to mammals (Schneider, 2007; Liu et al., 2014). In shallow lakes, they are particularly important as they can contribute to transparency (Mjelde and Faafeng, 1997; Zuccarini et al., 2011). Macrophyte communities can also contribute to a clear lake state through various self-reinforcing feedback mechanisms (Scheffer and Carpenter, 2003), as they can control phytoplankton growth (Scheffer, 1998). Its occurrence and structure depend on environmental factors such as light conditions and nutrient availability (Mjelde and Faafeng, 1997; Nagengast and Kuczynska-Kippen, 2015) and the economic development of lakes and their use of catchments (Kissoon et al., 2013). Degradation of macrophytes due to water quality decline is a typical result of eutrophication and degradation of shallow lakes in all climatic regions, usually from a clear-water state dominated by macrophytes to a stable turbid water state (Moss, 1990; Jin et al., 2006; Klimaszyk et al., 2015). In particular, the change in water nutrition has been proven to significantly alter the species richness and diversity of plants and animals (Thiebaut and Muller, 1998). This means that lake eutrophication will lead to changes in the structure of macrophyte communities.
The reality is that the degradation of macrophyte communities in shallow lakes has been accelerating globally in response to an increasing anthropogenically-driven eutrophication in recent decades (Jeppesen et al., 1998; Scheffer, 1998; Zhang et al., 2017). In China, the majority of lakes have been affected by eutrophication and water quality degradation as a result of social development and other human activities (Cheng and Li, 2006; Qin et al., 2013). Consequently, the diversity of macrophyte communities in lakes has been negatively affected, leading to a disruption in the ecological balance of the lake (Sand-Jensen et al., 2000; Hilt and Gross, 2008; Bornette and Puijalon, 2011; Alahuhta et al., 2013; Qin et al., 2013). In order to restore the health of lakes and promote their beneficial effects on human survival, the restoration of aquatic ecosystems, especially the restoration of macrophytes, has become a top priority (Zhang et al., 2012; Wang et al., 2016). However, the changes in macrophyte communities are affected by both biological and abiotic factors in nature, such as nutritional constraints, seasonal changes, light availability, and the relationship with fish and algae (Irfanullah and Moss, 2004; Bornette and Puijalon, 2011; Alahuhta et al., 2013; Li et al., 2015; Wang et al., 2016). Therefore, it is essential to adopt sustainable management and effective recovery for macrophytes (Peretyatko et al., 2009).
Substantial studies have investigated the differences in macrophyte communities with varying nutrient levels, such as long term monitoring in Europe has found that species richness in moderately eutrophic lakes is higher than that in low or high eutrophic waters, eutrophic lakes support more species than malnourished or nutrient poor waters (Rorslett, 1991). However, it has also been reported that the submerged flora in eutrophic lakes decreased (Rorslett, 1991). In Finnish naturally oligotrophic lakes, eutrophication typically increases the species richness of aquatic flora (Leka et al., 2008). Among the environmental driving factors, nutrient enrichment is the main factor affecting the composition of macrophyte communities in lakes (Wang et al., 2014; Phillips et al., 2016; Gerdol et al., 2018; Salgado et al., 2018a; Wang et al., 2020). Coupled with water transparency and competition from phytoplankton, eutrophication not only filters out species that are not well adapted to stress, but may also exclude functionally similar species (Arthaud et al., 2012; Fu et al., 2017; Salgado et al., 2018b). At the same time, it has been found that indirect facilitation can prevent the decline of aquatic plant diversity in freshwater ecosystems threatened by eutrophication (Le Bagousse-Pinguet et al., 2012). In eutrophic lakes in China, nitrogen content and water transparency are important factors affecting aquatic plants. Restoring submerged vegetation is a feasible method to improve water environment and enhance ecological function (Ye et al., 2018). However, few studies have tested the macrophyte communities of shallow lakes on the plateau, especially those with different nutrients in the same basin. An enhanced understanding of these response mechanisms of macrophytes under eutrophication will provide useful information for the management of macrophytes.
To examine the diversity and driving factors of macrophyte communities in plateau water bodies of different trophic states, we conducted field surveys on three lakes located in Yunnan Province, China. We main concern: (1)What is the nutritional status of the three lakes? And what impact will it have on the diversity and community structure of macrophyte? (2)Which ecological factors play an important role in regulating the structure of macrophyte community?
2 Materials and methods
2.1 Study area
The three studied lakes (Xihu Lake, Cibi Lake and Haixihai Lake; 25°25′-26°16′N, 99°32′-100°27′E) are located in Dali Bai Autonomous Prefecture, the northwest of Yunnan Province, China (Figure 1, Table 1). They are located in the Lancang River Basin, which is located in mid-subtropical southwest monsoon climate zone. (Chen and Wu, 2020; Li et al., 2020). These three lakes are all connected to Erhai Lake through rivers, which making them an important water source for the Erhai Lake. As the upstream lake, Xihu Lake flows into the Erhai Lake through the Luoshi River, while Cibi Lake and Haixihai Lake flow into the Erhai Lake through the Miju River. It is affected by the Indian Ocean climate. Rainfall is unevenly distributed throughout the year, with approximately 80% occurring from May to October, and the annual average rainfall is 994.2 mm. The multiyear average temperature is 14.6 °C (Zheng et al., 2021).
2.2 Macrophyte community
Macrophytes were sampled in three lakes, with 5 (Xihu Lake), 4 (Cibi Lake) and 3 (Haixihai Lake) sections. For each section we start at a depth of 1 m with a depth gradient of 0.5m, until no plants were present by using a rotatable reaping hook with a diameter 0.5m and area of 0.2m2. Each Sampling, three times at per water depth site (Figure 1). All collected samples were washed before identified. Then the plants were dried, and weighed to determine the biomass. Every three months of sampling were conducted from June 2020 to March 2022, corresponding to spring, summer, autumn, and winter, respectively. In order to ensure that as many plants as possible were present in our survey, the cross section of each lake was evenly distributed along the shoreline. A total of 33 macrophyte species were recorded during the survey period (Appendix 1).
2.3 Environmental factor
We collected water samples at each sampling section. They were collected from a mixture of subsamples taken from the surface (below 0.2m of water surface), middle (the middle of the water depth at that site), and bottom layers (0.5 m above sediment surface) of the water column. The water transparency (SD) was used by Secchi disk to determine onsite. At the same time, water temperature (T), dissolved oxygen (DO), Salinity (SAL), and pH were measured at a water depth of 0.5 m using a YSI Professional Plus Water Quality Monitor (YSI Inc., Yellow Springs, Ohio, USA). Water samples were placed in a cooler and sent to a laboratory for testing as soon as possible. Analysis indicators included total nitrogen (TN), total phosphorus (TP), ammonium (NH4-N), and phytoplankton chlorophyll a (Chl.a) according to relevant detection methods (Huang et al., 1999). A total of 9 environmental driver variables were included.
2.4 Data analysis
The comprehensive nutrient index method was used to evaluate lake eutrophication (Jin, 1995). The formula for calculating the comprehensive nutritional status index is as follows:
In the formula, W (Chl.a), W (TP), W (TN), W (SD) are 0.3260, 0.2300, 0.2192, 0.2246, respectively. Lake nutrient status is graded from 0 to 100: TLI (Σ)≤30, poor nutrition; 30≤TLI (Σ)≤50, medium nutrition; 50≤TLI (Σ)≤60, mild eutrophication; 60≤TLI (Σ)≤70, moderate eutrophication; 70≤TLI (Σ)≤100, highly eutrophication.
The α-diversity index was used to measure the taxonomic diversity attributed to each lake, while the diversity was measured by the Species richness (S), Shannon-Wiener index (H’), Simpson index (D) and Pielou index (J’). To examine the α-diversity index of the macrophyte community in different lakes, the one-way ANOVA was used to test the differences of macrophyte community diversity between the three lakes.
To test the similarity of macrophyte communities in different lakes, the non-metric multidimensional scaling (NMDS) was used to visualize the overall differences using the “vegan” package.
The relationships between the diversity indices and the environmental parameters were defined using direct gradient analysis, with the CANOCO 5 program. And the redundancy analysis (RDA) was chosen to further test the potential effects of the environmental parameters on the diversity indices. Forward selection of variables was used to provide a ranking of the relative importance of specific variables and to avoid colinearity.
All data were tested for normality and homogeneity before analysis, and log (x+1) or log (1/x) conversion was performed on data that did not meet the above conditions. The significance levels were all set to p<0.05. All the statistical analyses were completed by R statistical package (vegan), SPSS 22.0 software and Origin 2021.
3 Results
3.1 Water environmental factors and nutrient levels of different lakes
The one-way ANOVA showed that except for NH4-N and T indicators, there was strong heterogeneity among the physicochemical factors of water in different lakes (Table 2). The mean values have little difference. The average concentrations of TN and TP in the three lakes were between 0.25-1.10 mg/L and 0.02-0.09 mg/L, respectively. One-way ANOVA showed that there were significant differences in TN and TP between the Xihu Lake and other lakes. The Chl.a concentration was significantly different among the three lakes, with Cibi Lakes and Haixihai Lake had 4.23 ug/L and 4.36 ug/L, respectively, while Xihu Lake had a much higher concentration of 34.83 ug/L. Regarding SD, there were also significant differences between the Xihu Lake and the other two lakes, with Xihu Lake having a much lower SD of only 1.21 m compared to the other two lakes with SD values of 3.72 m and 3.59 m, respectively. In addition to the above indicators, all three lakes had higher DO and the mean values ranged form 7.78-8.64 mg/L. And there were significant differences between the Xihu Lake and the Cibi Lake. In terms of SAL, the highest of 0.22 belongs to the Xihu Lake, and the Cibi Lake had 0.18. The Haixihai Lake had the lowest of 0.11. The three studied lakes were all alkaline with a mean pH range of 8.34-8.73.
The comprehensive nutrient index method showed that the three lakes had different nutrition level (Table 3). Among them, the Xihu Lake of eutrophic index is between 50-60, of the mean value of the nutrient index was 56.33. As a result, it has reached the standard of a mildly entrophication lake. The eutrophication index of the other two lakes ranges from 30 to 40, of which the mean value of the nutrient index of the Cibi lake and the Haixihai lake was 36.03 and 33.48, respectively. These two lakes are classified as medium nutrition lakes.
3.2 Change of α-diversity index of different lakes
This study showed that the α-diversity index of different lakes had hump-shape relationships with seasons (Figure 2), and there were significant differences among lakes (p<0.05). For the three lakes, the α-diversity index of Xihu Lake was the lowest and that of Cibi Lake was the highest in each of the four seasons. Both showed a lower trend in spring and winter, and a higher trend in summer and autumn.
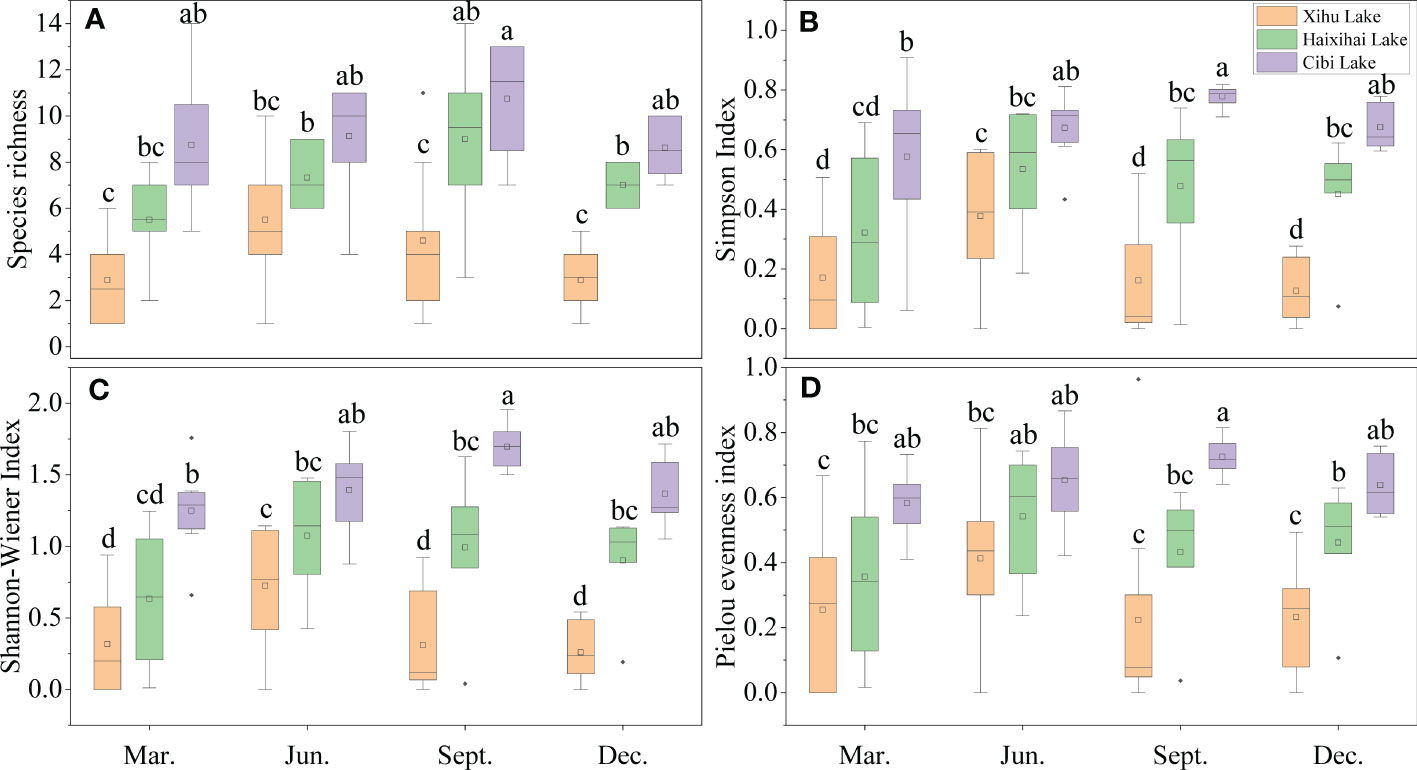
Figure 2 Seasonal variation of macrophytes aquatic plant a-diversity index in the three lakes. Different lowercase letters indicate a significant difference (p<0.05). (A) (Species richness), (B) (Simpson index), (C) (Shannon-Wiener index), (D) (Pielou index). Spring (March), Summer (June), Autumn (September), Winter (December), the same in Figure 3.
For the mean species richness, the minimum value of Xihu Lake was 3 in winter and the maximum value was 6 in summer. The minimum value of Haixihai Lake appeared in spring, which was 6, and the maximum value appeared in autumn, which was 9. The minimum value of Cibi Lake was 9 in spring and autumn, and the maximum value was 11 in autumn (Figure 2A). The maximum value of Cibi Lake was about 2 times higher than that of Xihu Lake.
Simpson index mean resulted that the lowest value of Xihu Lake was 0.13 in winter and the highest value was 0.38 in summer. The lowest value of Haixihai Lake was 0.32 in spring, and the highest value was 0.53 in summer. The lowest value of Cibi Lake was 0.58 in spring, and the highest value was 0.78 in autumn (Figure 2B).
The research on the Shannon-Wiener index mean showed that Xihu Lake was the minimum of the three lakes. Its lowest value was 0.26 in winter, and its highest value was 0.72 in summer. The lowest value of Haixihai Lake was 0.63 in spring and the highest value was 1.07 in summer. And Cibi Lake was the largest of the three lakes, the lowest value was 1.25 in spring, and the highest value was 1.70 in autumn (Figure 2C).
The Pielou index indicated that the minimum value of Xihu Lake was 0.22, which appeared in autumn, and the maximum value was 0.41, which appeared in summer. The minimum value of Haixihai Lake was 0.36, which appeared in spring, and the maximum value was 0.54, which appeared in summer. The minimum value of Cibi Lake was 0.58, which appeared in spring, and the maximum value was 0.73, which appeared in autumn (Figure 2D).
The results of diversity analysis showed a significant negative correlation between α-diversity and lake nutrient status (Appendix 2). The difference of the three lakes in that although the change trend was the same, the seasonal differences of the maximum value of different diversity indexes were different. In terms of the mean species richness, the Xihu Lake reached its maximum in summer, while Haixihai Lake and Cibi Lake reached their maximum in autumn. For Simpson index, Shannon-Wiener index and Pielou index, the Xihu Lake and Haixihai Lake reached the maximum in summer, while the Cibi Lake reached the maximum in autumn.
3.3 Macrophyte communities in functional space of different lakes
Non-metric multidimensional ordination (NMDS) showed that there were obvious spatial structures differences among the macrophyte communities in the three lakes (Figure 3).
In terms of seasons, the community samples of the three lakes in spring and winter were far away from each other, indicating that the macrophyte communities structure were quite different in these two seasons. The stress coefficients of the spatial structures of macrophyte communities in the two seasons were 0.1299 and 0.1398, respectively. The results of NMDS analysis were basically reliable. In summer and autumn, the spatial structures of macrophyte communities in the three lakes were more similar, especially in Haixihai Lake and Cibi Lake, indicating that there was little difference in the structure of macrophyte communities between the two seasons. The stress coefficients of the spatial structures of macrophyte communities in the two seasons were 0.1633 and 0.1491, respectively. The results of NMDS analysis were also reliable.
3.4 Macrophyte communities and environmental drivers
RDA results showed that the environmental driving factors of the three lakes vary significantly across seasons, as well as between lakes (Figure 4). Throughout the year, the RDA indicated distinct differences between diversity index and ecological factors, the eigenvalues of Axis 1 and Axis 2 being, respectively, 36.13% and 8.15%. Environmental factors could explain 44.8% of the total variation in macrophyte communities structure. Among these, nitrogen, phosphorus, water transparency and water temperature contributed 50.2%, 3.5%, 3.8% and 27.5%, respectively.
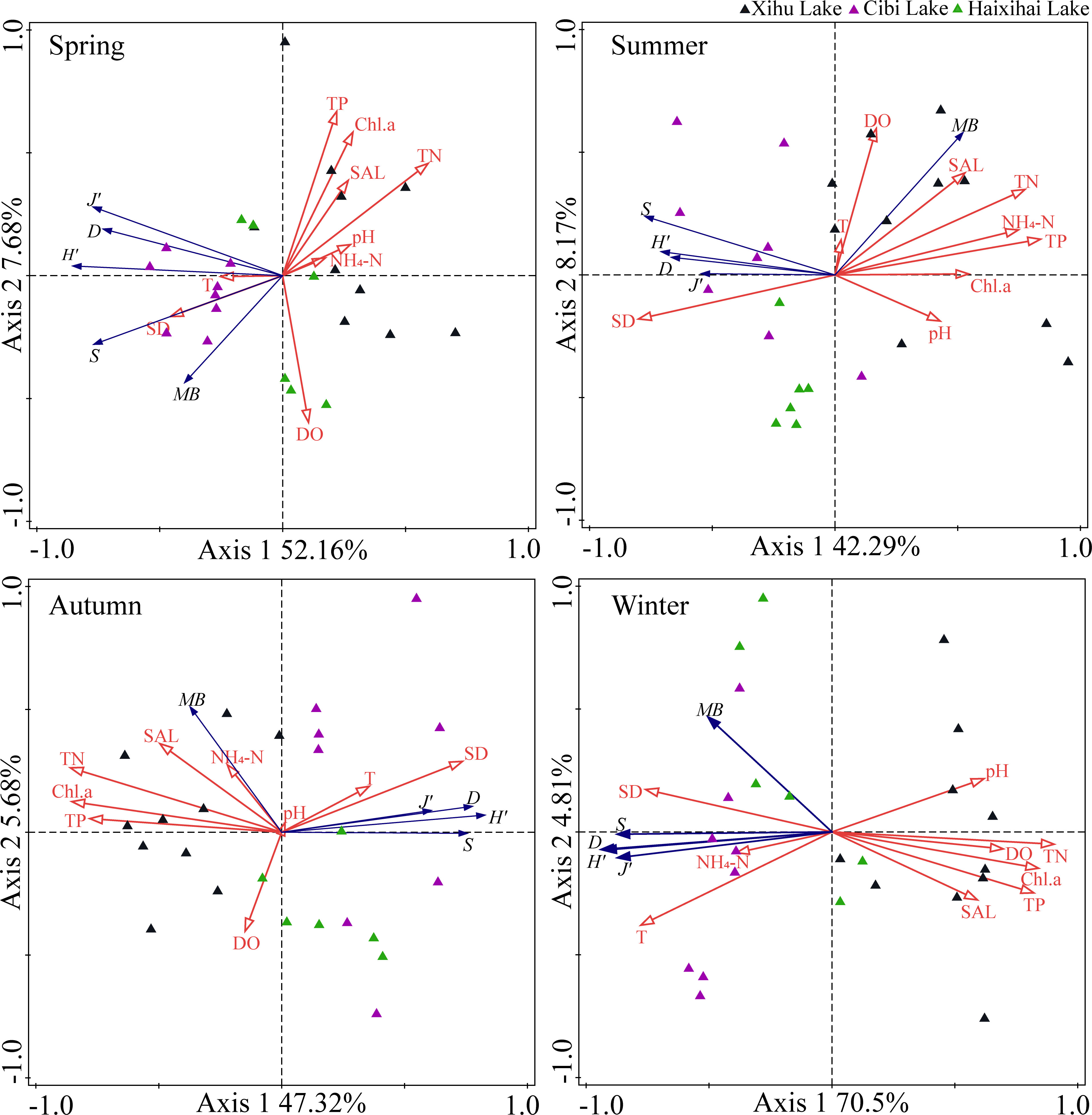
Figure 4 Redundancy analysis (RDA) for macrophyte communities and environmental factors in different seasons. S (Species richness), H’ (Shannon-Wiener index), D (Simpson index), J’ (Pielou index), MB (Mean biomass), SD (water transparency), pH (hydrogen ion concentration), T (water temperature), DO (dissolved oxygen), SAL (Salinity), TN (total nitrogen), TP (total phosphorus), NH4-N (ammonium), Chl.a (phytoplankton chlorophyll a).
The RDA analysis results in spring showed that the eigenvalues of the first two axis were 52.16% and 7.68%, respectively. Environmental factors could explain 60.7% of the total variation in macrophyte communities structure. Among these, nitrogen, phosphorus, water transparency and water temperature contributed 32.7%, 3.4%, 0.2% and 13.3%, respectively.
The eigenvalues of the first two axis of RDA analysis in summer were 42.29% and 8.17%, respectively. Environmental factors could explain 54.6% of the total variation in macrophyte communities structure. Among these, nitrogen, phosphorus, water transparency and water temperature contributed 6.3%, 54.6%, 0.4% and 4.0%, respectively.
The eigenvalues of the first two axis of RDA analysis in autumn were 47.32% and 5.68%, respectively. Environmental factors could explain 55.0% of the total variation in macrophyte communities structure. Among these, nitrogen, phosphorus, water transparency and water temperature contributed 64.4%, 1.1%, 7.3% and 4.2%, respectively.
The eigenvalues of the first two axis of RDA analysis in winter were 70.5% and 4.81%, respectively. Environmental factors could explain 77.2% of the total variation in macrophyte community structure. Among these, nitrogen, phosphorus, water transparency and water temperature contributed 74.6%, 2.6%, 5.4% and 9.3%, respectively.
These results indicated that the ranking could better reflect the relationship between environmental factors and macrophyte communities indicators. Based on the RDA analysis of macrophyte communities and environmental factors in the three lakes during the four seasons, our research showed that for the three lakes, TN and TP had a positive correlation effect on the macrophyte communities in the Xihu Lake, while a negative correlation effect on the Haixihai Lake and Cibi lake. In different seasons, SD and T were not the key factors affecting community biomass in summer and autumn (June and September), but the key factors affecting community biomass were various nutrients. In winter and spring (December and March), SD and T were the key factors affecting community biomass, and the effect of nutrients on community biomass was not the main factor.
4 Discussion
Previous studies have shown that transparency and lake nutrient status significantly affect the species diversity and biomass of submerged plants (Ye et al., 2018; Wang et al., 2022). It is well known that the relationship between water quality and macrophytes is mutually beneficial (Bornette and Puijalon, 2011). Macrophytes are strongly influenced by a variety of water quality parameters and sediment characteristics (Alahuhta et al., 2013). In addition, macrophytes can help improve water quality by absorbing nutrients from water and sediment and by preventing sediment suspension (Jeppesen et al., 1998). Owing to lake eutrophication, phytoplankton biomass will increase and water transparency will decrease. This also leads to the species richness of habitats from mesotrophic to eutrophic is higher, while the species richness of oligotrophic, malnourished and eutrophic ultra eutrophic water bodies is lower (Rorslett, 1991; Toivonen and Huttunen, 1995; Murphy, 2002). In fact, ultra eutrophic lakes that are heavily shaded by phytoplankton may lack submerged vegetation, even almost none in the coastal zone (Morris et al., 2003; RodrÃGuez-Gallego et al., 2010). The transparency of 13 eutrophic lakes in Denmark decreases with the increasing total phosphorus concentration. In the eutrophication process of Taihu lake, the richness and area of large plant species decreased (Qin et al., 2013). A study of 60 lakes in the United Kingdom and Poland found that macrophyte diversities with increasing nitrogen concentration, particularly nitrate nitrogen concentration in winter, followed by phosphorus concentration (James et al., 2005). These studies have demonstrated that eutrophication in lakes can lead to a series of changes in the physical and chemical properties of water bodies, which in turn affect the diversity of macrophytes. The evidence produced by this study suggested that the three lakes, Xihu Lake, Haixihai Lake and Cibi Lake, which belong to the same watershed, exhibit obvious heterogeneity in their nutrient composition, such as nitrogen, phosphorus contents and chlorophyll concentration, etc. In general, the contents of TN, TP and Chl.a in the Xihu Lake were higher than those in the other two lakes. And the SD was significantly lower than that of the other two lakes. As a mildly eutrophication lake, the α-diversity index of Xihu Lake were the lowest compared with the other two medium nutrition lakes. It also proved that eutrophication and water transparency had a significant effect on the diversity of aquatic plants. In this study, we found that the seasons in which the same α-diversity index reached its highest value were different in different lakes. This may be due to the different trophic states of different lakes. Chla, SD, etc. vary with the seasons. And due to the differences in nutrient status of lakes, the composition of macrophytes varies, the different growth requirements of different species, and the differences in competition among species. Therefore, in order to maintain the health of lake ecosystem, it is necessary to ensure the diversity of macrophyte. It is important to restore the health of lake and slow down or even eliminate the process of eutrophication in lakes.
NMDS analysis revealed that the characteristics of macrophyte communities in the three lakes were more similar in summer and autumn. Moreover, among the four seasons, the Haixihai Lake and the Cibi lake had closer similarities. Community structure is also affected by variations of ambient temperature (Lacoul and Freedman, 2006). In the plateau area of the northern hemisphere, there are obvious seasonal changes. For a long time, people have a clear understanding of the existence of their own living habits of macrophytes. The growth of macrophytes requires sufficient light and temperature conditions. Due to insufficient light and low water temperature in winter and spring, only a few macrophytes with low survival threshold survive in the lake, with low biomass and richness. Conversely, in summer and autumn, enhanced light and increased water temperature create favorable conditions for the rapid growth of large aquatic plants. Therefore, the species richness of the three lakes increases, and the community characteristics are similar. Based on the results of this study, the Haixihai Lake and the Cibi Lake were mesotrophic lakes with similar hydrological conditions and physical and chemical properties, resulting in similar in the community characteristics of macrophytes.
Studies have shown that the macrophyte communities in lakes are mainly affected by eutrophication parameters, and the species richness of lakes with low nutrient levels is higher than that of lakes with high nutrient levels, which is mainly related to TN, TP (Szoszkiewicz et al., 2014). Littorella uniflora is restricted to low phosphorus habitats, while Lemna minor, Spirodela polyrhiza and Zanichellia palustris require medium to high phosphorus concentrations (Heegaard et al., 2001; Trei and Pall, 2004). However, the distribution of Isoetes lacustris, Lemna minor, and Myriophyllum alterniflorum is influenced by integrated gradients of nitrogen, phosphorus, and sometimes other nutrients (Heegaard et al., 2001; Rubio et al., 2003; Paal and Trei, 2004). Studies have also revealed that N has a significantly adverse effect on the growth of submerged plants when TP concentration is not high (Olsen et al., 2015). Our research showed that increased nitrogen and phosphorus in mesotrophic lakes like the Haixihai Lake and the Cibi Lake decreases the abundance of macrophytes, resulting in changes in ecosystem structure. This is consistent with the results of previous studies. However, the relationship between N, P and macrophytes in the Xihu Lake was opposite to that in the other two lakes. This may be because Xihu Lake has an average water depth of only 2-3m, whereas the other two lakes have an average water depth of approximately 10m. Therefore, under the conditions of water eutrophication and high transparency, the large aquatic plants in the Xihu Lake allow different submerged plant species to replace each other along the environmental gradient of water temperature, light availability and wave exposure from shallow to deep water (Vestergaard and Sand-Jensen, 2000). Among lakes, macrophytes are not only limited by nutrients, but their biomass and distribution often change with the variations of water physical properties, such as transparency and temperature (Chambers and Kalff, 1985; Dale, 1986; Royle and King, 1991). The RDA results showed that in summer and autumn, SD and T were not the key factors affecting biomass, and nutrients played a decisive role in biomass. In winter and spring, on the contrary, SD and T become the key factors determining biomass, while nutrients are not the key factors. Some studies have found that in lakes after submerged vegetation restoration, the SD of water bodies with vegetation in summer is higher than that of water bodies without vegetation (Ye et al., 2018), and the water temperature in summer and autumn reaches the growth threshold suitable for large aquatic plants. This indicates that during summer and autumn, SD and T are not limiting factors. At this time, macrophytes grow rapidly and need ample nutrient support. Water nutrients have become a limiting factor. In winter and spring, due to seasonal fluctuations, changes in climatic and hydrological characteristics, the development of macrophytes basically stagnates in winter. In spring, the temperature and water temperature rise, the days grow longer, and when the light is sufficient, the biomass of macrophytes increases (Champion and Tanner, 2000; Riis et al., 2003). Therefore, the biomass is limited by SD and T during this time. Seasonal changes bring about changes in environmental factors (SD, T, etc.). In the process of change, macrophyte growth still needs to obtain sufficient nutrients. Therefore, throughout the year, although nutrients and seasons alternations play a major role, they also jointly drive the community structure of macrophytes.
In addition to the above environmental factors, those not covered in this paper, such as lake area, lake shoreline length, shoreline morphology, water flow rate and physical characteristics of sediment, etc., may also have an impact on large plant community. Generally speaking, the longer length of lake shoreline, the more complex morphology of lake shoreline and the larger lake area, the higher diversity of macrophytes plants and the higher biomass (Kent et al., 1982). At the same time, the water flow rate may affect the growth of aquatic plant, thus affecting the reproduction and growth of macrophytes plant, leading to a decline in species diversity and biomass (Biggs, 1996). The physical characteristics of sediment usually affect the growth of macrophytes plant by influencing their rooting. These factors increase the heterogeneity of habitats, so they can provide more suitable habitats for macrophytes plant and affect large plant community.
5 Conclusion
In conclusion, we have conducted a study on the changes in diversity and environmental drivers of aquatic macrophyte communities in shallow eutrophic lakes on the plateau. It was found that the α-diversity of lakes with different nutrient types differs significantly, and the macrophyte communities of lakes with the changes of seasons makes more similar. Additionally, RDA analysis was employed to investigate the effects of environmental factors on macrophyte communities, which confirmed that nutrients were still the key factors affecting macrophytes in eutrophic plateau lakes, and SD and T also proved to play a key role in eutrophic plateau lakes. Seasons and nutrients jointly drive changes in the structure of macrophyte community. Therefore, more attention needs to be paid to the eutrophication of plateau lakes in the future, and adopt various measures to reduce pollution output and slow down the eutrophication rate of lakes. The research results can provide reference for the ecological restoration and lake health management of eutrophic plateau lakes in the future.
Data availability statement
The original contributions presented in the study are included in the article/supplementary material. Further inquiries can be directed to the corresponding author.
Author contributions
YT is the primary author who analyzed the datasets, generated results, and wrote the draft. CL is mainly responsible for data collection. LH and HS contributed to the data collection. WH provided critical technique support. ZW and CY helped interpret the results. QC contributed to the manuscript review and editing and provided comments for the data analyses. XZ and LN provided constructive suggestions in the manuscript. TC was responsible for the overall design of the work and provided constructive suggestions in the discussion section. All authors provided comments and suggestions for the manuscript revision. All authors contributed to the article and approved the submitted version.
Funding
This work was supported by the National Natural Science Foundation of China (32101319, 31930074, 32071574), and the Research funds of The Guangxi Key Laboratory of Theory and Technology for Environmental Pollution Control (Guikeneng2001K007).
Conflict of interest
The authors declare that the research was conducted in the absence of any commercial or financial relationships that could be construed as a potential conflict of interest.
Publisher’s note
All claims expressed in this article are solely those of the authors and do not necessarily represent those of their affiliated organizations, or those of the publisher, the editors and the reviewers. Any product that may be evaluated in this article, or claim that may be made by its manufacturer, is not guaranteed or endorsed by the publisher.
References
Alahuhta J., Kanninen A., Hellsten S., Vuori K.-M., Kuoppala M., Hamalainen H. (2013). Environmental and spatial correlates of community composition, richness and status of boreal lake macrophytes. Ecol. Indic. 32, 172–181. doi: 10.1016/j.ecolind.2013.03.031
Arthaud F., Vallod D., Robin J., Bornette G. (2012). Eutrophication and drought disturbance shape functional diversity and life-history traits of aquatic plants in shallow lakes. Aquat. Sci. 74, 471–481. doi: 10.1007/s00027-011-0241-4
Biggs B. (1996). Hydraulic habitat of plants in streams. Regulated. Rivers. Res. Manage. 12, 131–144. doi: 10.1002/(SICI)1099-1646(199603)12:2/3<131::AID-RRR385>3.0.CO;2-X
Blindow I., Hargeby A., Andersson G. (1998). Alternative stable states in shallow lakes: what causes a shift? (New York: Springer).
Bornette G., Puijalon S. (2011). Response of aquatic plants to abiotic factors: a review. Aquat. Sci. 73, 1–14. doi: 10.1007/s00027-010-0162-7
Carpenter S. R. (1980). The decline of Myriophyllum spicatum in a eutrophic Wisconsin lake. Can. J. Botany-Revue. Can. Botanique. 58, 527–535. doi: 10.1139/b80-064
Carpenter S. R., Lodge D. M. (1986). Effects of submersed macrophytes on ecosystem processes. Aquat. Bot. 26, 341–370. doi: 10.1016/0304-3770(86)90031-8
Chambers P. A., Kalff J. (1985). Depth distribution and biomass of submersed aquatic macrophyte communities in relation to secchi depth. Can. J. Fisheries. Aquat. Sci. 42, 701–709. doi: 10.1139/f85-090
Champion P. D., Tanner C. C. (2000). Seasonality of macrophytes and interaction with flow in a new Zealand lowland stream. Hydrobiologia 441, 1–12. doi: 10.1023/A:1017517303221
Chen J., Wu C. (2020). Evaluation of ecological sensitivity in erhai lake basin, southwest China. IOP. Conf. Series.: Earth Environ. Sci. 612 (1), 012072. doi: 10.1088/1755-1315/612/1/012072
Cheng X., Li S. (2006). An analysis on the evolvement processes of lake eutrophication and their characteristics of the typical lakes in the middle and lower reaches of Yangtze river. Chin. Sci. Bull. 51 (013), 1603–1613. doi: 10.1007/s11434-006-2005-4
Dale H. M. (1986). Temperature and light-the determining factors in maximum depth distribution of aquatic macrophytes in Ontario, Canada. Hydrobiologia 133, 73–77. doi: 10.1007/BF00010804
Fu H., Zhong J., Fang S., Hu J., Guo C., Lou Q., et al. (2017). Scale-dependent changes in the functional diversity of macrophytes in subtropical freshwater lakes in south China. Sci. Rep. 7 (1), 8294. doi: 10.1038/s41598-017-08844-8
Gerdol R., Brancaleoni L., Lastrucci L., Nobili G., Pellizzari M., Ravaglioli M., et al. (2018). Wetland plant diversity in a coastal nature reserve in Italy: relationships with salinization and eutrophication and implications for nature conservation. Estuaries. Coasts. 41, 2079–2091. doi: 10.1007/s12237-018-0396-5
Heegaard E., Birks H. H., Gibson C. E., Smith S. J., Wolfe-Murphy S. (2001). Species-environmental relationships of aquatic macrophytes in northern Ireland. Aquat. Bot. 70, 175–223. doi: 10.1016/S0304-3770(01)00161-9
Hilt S., Gross E. M. (2008). Can allelopathically active submerged macrophytes stabilise clear-water states in shallow lakes? Basic. Appl. Ecol. 9, 422–432. doi: 10.1016/j.baae.2007.04.003
Huang X. ,. F., Chen W. M., Cai Q. M. (1999). Survey, observation and analysis of lake ecology.Standard methods for observation and analysis in Chinese ecosystem research network, series V (Beijing: Standards Press of China).
Irfanullah H. M., Moss B. (2004). Factors influencing the return of submerged plants to a clear-water, shallow temperate lake. Aquat. Bot. 80, 177–191. doi: 10.1016/j.aquabot.2004.07.010
James C., Fisher J., Russell V., Collings S., Moss B. (2005). Nitrate availability and hydrophyte species richness in shallow lakes. Freshw. Biol. 50, 1049–1063. doi: 10.1111/j.1365-2427.2005.01375.x
Jeppesen E. S., Sondergaard M., Sondergaard M., Christoffersen K. (1998). “The structuring role of submerged macrophytes in lakes,” in Ecological studies (New York: Springer), 1–423.
Jin X., Xu Q., Yan C. (2006). Restoration scheme for macrophytes in a hypertrophic water body, wuli lake, China. Lakes. Reservoirs.: Res. Manage. 11, 21–27.
Kent C., Wong J.J.C.J.O.F., Sciences A. (1982). An index of littoral zone complexity and its measurement. Can J Fish Aquat Sci. 39, 847–853. doi: 10.1139/f82-115
Kissoon L. T. T., Jacob D. L., Hanson M. A., Herwig B. R., Bowe S. E., Otte M. L. (2013). Macrophytes in shallow lakes: relationships with water, sediment and watershed characteristics. Aquat. Bot. 109, 39–48. doi: 10.1016/j.aquabot.2013.04.001
Klimaszyk P., Piotrowicz R., Rzymski P. (2015). Changes in physico-chemical conditions and macrophyte abundance in a shallow soft-water lake mediated by a great cormorant roosting colony. J. Limnol. 74, 114–122. doi: 10.1111/j.1440-1770.2006.00291.x
Lacoul P., Freedman B. (2006). Environmental influences on aquatic plants in freshwater ecosystems. Environ. Rev. 14, 89–136. doi: 10.1139/a06-001
Le Bagousse-Pinguet Y., Liancourt P., Gross N., Straile D. (2012). Indirect facilitation promotes macrophyte survival and growth in freshwater ecosystems threatened by eutrophication. J. Ecol. 100, 530–538. doi: 10.1111/j.1365-2745.2011.01931.x
Leka J., Toivonen H., Leikola N., Hellsten S. (2008). Vesikasvit Suomen Järvien Tilan Ilmentäjinä Ekologisen Tilaluokittelun Kehittäminen (Macrophytes as Indicators of the Ecological Quality of Finnish Lakes. Development of Ecological Classifification) The Finnish Environment 18/2008, Finnish Environment Institute (SYKE). Available at: www.ymparisto.fifi/julkaisut (in Finnish with English summary)..
Li J., Bai Y., Alatalo J. M. (2020). Impacts of rural tourism-driven land use change on ecosystems services provision in erhai lake basin, China. Ecosyst. Serv. 42, 101081. doi: 10.1016/j.ecoser.2020.101081
Li H.-L., Xu Y.-S., Wang Y.-Y., Yu N.-Q., Zhang M.-X., Lei G.-C., et al. (2015). Does clonal fragmentation of the floating plant Eichhornia crassipes affect the growth of submerged macrophyte communities? Folia Geobot. 50, 283–291. doi: 10.1007/s12224-015-9226-8
Liu G., Liu Z., Gu B., Smoak J. M., Zhang Z. (2014). How important are trophic state, macrophyte and fish population effects on cladoceran community? a study in lake erhai. Hydrobiologia 736, 189–204. doi: 10.1007/s10750-014-1906-5
Mjelde M., Faafeng B. A. (1997). Ceratophyllum demersum hampers phytoplankton development in some small Norwegian lakes over a wide range of phosphorus concentrations and geographical latitude. Freshw. Biol. 37, 355–365. doi: 10.1046/j.1365-2427.1997.00159.x
Morris K., Bailey P. C., Boon P. I., Hughes L. (2003). Alternative stable states in the aquatic vegetation of shallow urban lakes. II. catastrophic loss of aquatic plants consequent to nutrient enrichment. Mar. Freshw. Res. 54, 201–215. doi: 10.1071/MF02003
Moss B. (1990). Engineering and biological approaches to the restoration from eutrophication of shallow lakes in which aquatic plant communities are important components. Hydrobiologia 200, 367–377. doi: 10.1007/BF02530354
Murphy K. J. (2002). Plant communities and plant diversity in softwater lakes of northern Europe. Aquat. Bot. 73, 287–324. doi: 10.1016/S0304-3770(02)00028-1
Nagengast B., Kuczynska-Kippen N. (2015). Macrophyte biometric features as an indicator of the trophic status of small water bodies. Oceanol. Hydrobiol. Stud. 44, 38–50. doi: 10.1515/ohs-2015-0005
Olsen S., Chan F., Li W., Zhao S., Sondergaard M., Jeppesen E. (2015). Strong impact of nitrogen loading on submerged macrophytes and algae: a long-term mesocosm experiment in a shallow Chinese lake. Freshw. Biol. 60, 1525–1536. doi: 10.1111/fwb.12585
Paal J., Trei T. (2004). Vegetation of Estonian watercourses; the drainage basin of the southern coast of the gulf of Finland. Annales. Bot. Fennici. 41, 157–177.
Peretyatko A., Teissier S., De Backer S., Triest L. (2009). Restoration potential of biomanipulation for eutrophic peri-urban ponds: the role of zooplankton size and submerged macrophyte cover. Hydrobiologia 634, 125–135. doi: 10.1007/s10750-009-9888-4
Phillips G., Willby N., Moss B. (2016). Submerged macrophyte decline in shallow lakes: what have we learnt in the last forty years? Aquat. Bot. 135, 37–45. doi: 10.1016/j.aquabot.2016.04.004
Qin B., Gao G., Zhu G., Zhang Y., Song Y., Tang X., et al. (2013). Lake eutrophication and its ecosystem response. Chin. Sci. Bull. 58, 961–970. doi: 10.1007/s11434-012-5560-x
Riis T., Biggs B. J. F., Flanagan M. (2003). Seasonal changes in macrophyte biomass in south island lowland streams, new Zealand. New Z. J. Mar. Freshw. Res. 37, 381–388. doi: 10.1080/00288330.2003.9517174
RodrÃGuez-Gallego L. R., Mazzeo N., Gorga J., Meerhoff M., Clemente J., Kruk C., et al. (2010). The effects of an artificial wetland dominated by free-floating plants on the restoration of a subtropical, hypertrophic lake. Lakes Reserv. Res. Manag. 9, 203–215. doi: 10.1111/j.1440-1770.2004.00245.x
Rorslett B. (1991). Principal determinants of aquatic macrophyte richness in northern European lakes. Aquat. Bot. 39, 173–193. doi: 10.1016/0304-3770(91)90031-Y
Royle R. N., King R. J. (1991). Aquatic macrophytes in lake Liddell, new south Wales biomass, nitrogen and phosphorus status, and changing distribution from 1981 to 1987. Aquat. Bot. 41, 281–298. doi: 10.1016/0304-3770(91)90048-A
Rubio G., Zhu J. M., Lynch J. P. (2003). A critical test of the two prevailing theories of plant response to nutrient availability. Am. J. Bot. 90, 143–152. doi: 10.3732/ajb.90.1.143
Salgado J., Sayer C. D., Brooks S. J., Davidson T. A., Goldsmith B., Patmore I. R., et al. (2018a). Eutrophication homogenizes shallow lake macrophyte assemblages over space and time. Ecosphere 9, e02406. doi: 10.1002/ecs2.2406
Salgado J., Sayer C. D., Brooks S. J., Davidson T. A., Okamura B. (2018b). Eutrophication erodes inter-basin variation in macrophytes and co-occurring invertebrates in a shallow lake: combining ecology and palaeoecology. J. Paleolimnol. 60, 311–328. doi: 10.1007/s10933-017-9950-6
Sand-Jensen K., Riis T., Vestergaard O., Larsen S. E. (2000). Macrophyte decline in Danish lakes and streams over the past 100 years. J. Ecol. 88, 1030–1040. doi: 10.1046/j.1365-2745.2000.00519.x
Scheffer M., Carpenter S. R. (2003). Catastrophic regime shifts in ecosystems: linking theory to observation. Trends Ecol. Evol. 18, 648–656. doi: 10.1016/j.tree.2003.09.002
Schneider S. (2007). Macrophyte trophic indicator values from a European perspective. Limnologica 37, 281–289. doi: 10.1016/j.limno.2007.05.001
Sondergaard M., Phillips G., Hellsten S., Kolada A., Ecke F., Maeemets H., et al. (2013). Maximum growing depth of submerged macrophytes in European lakes. Hydrobiologia 704, 165–177. doi: 10.1007/s10750-012-1389-1
Szoszkiewicz K., Ciecierska H., Kolada A., Schneider S. C., Szwabinska M., Ruszczynska J. (2014). Parameters structuring macrophyte communities in rivers and lakes - results from a case study in north-central Poland. Knowledge. Manage. Aquat. Ecosyst. 145 (415), 08. doi: 10.1051/kmae/2014034
Thiebaut G., Muller S. (1998). The impact of eutrophication on aquatic macrophyte diversity in weakly mineralized streams in the northern vosges mountains (NE France). Biodivers. Conserv. 7, 1051–1068. doi: 10.1023/A:1008809131487
Toivonen H., Huttunen P. (1995). Aquatic macrophytes and ecological gradients in 57 small lakes in southern Finland. Aquat. Bot. 51, 197–221. doi: 10.1016/0304-3770(95)00458-C
Trei T., Pall P. (2004). Macroflora in the watercourses of saaremaa island (Estonia). Boreal. Environ. Res. 9, 25–35.
Vestergaard O., Sand-Jensen K. (2000). Aquatic macrophyte richness in Danish lakes in relation to alkalinity, transparency, and lake area. Can. J. Fisheries. Aquat. Sci. 57, 2022–2031. doi: 10.1139/f00-156
Wang H.-J., Wang H.-Z., Liang X.-M., Wu S.-K. (2014). Total phosphorus thresholds for regime shifts are nearly equal in subtropical and temperate shallow lakes with moderate depths and areas. Freshw. Biol. 59, 1659–1671. doi: 10.1111/fwb.12372
Wang H., Wen Z., Ren W., Ni L., Zhang X., Li K., et al. (2022). What is the mechanism of submerged macrophyte biodiversity affecting biomass productivity along water depth: niche complementarity or selection effect? Ecol. Indic. 138, 108858. doi: 10.1016/j.ecolind.2022.108858
Wang H., Wen Z., Zhang Z., Zhang X., Fu H., Cao Y., et al. (2020). Environmental vs. spatial drivers of submerged macrophyte community assembly in different seasons and water depths in a mesotrophic bay of erhai lake, China. Ecol. Indic. 117, 106696. doi: 10.1016/j.ecolind.2020.106696
Wang P., Zhang Q., Xu Y.-S., Yu F.-H. (2016). Effects of water level fluctuation on the growth of submerged macrophyte communities. Flora 223, 83–89. doi: 10.1016/j.flora.2016.05.005
Ye C., Yao L., Deng A., Liu G., Liu W. (2018). Spatial and seasonal dynamics of water quality, sediment properties and submerged vegetation in a eutrophic lake after ten years of ecological restoration. Wetlands 38, 1147–1157. doi: 10.1007/s13157-018-1021-x
Zhang Q., Dong B.-C., Li H.-L., Liu R.-H., Luo F.-L., Zhang M.-X., et al. (2012). Does light heterogeneity affect structure and biomass of submerged macrophyte communities? Bot. Stud. 53, 377–385. doi: 10.1105/tpc.112.099994
Zhang Y., Jeppesen E., Liu X., Qin B., Shi K., Zhou Y., et al. (2017). Global loss of aquatic vegetation in lakes. Earth-Sci. Rev. 173, 259–265. doi: 10.1016/j.earscirev.2017.08.013
Zheng L., An Z., Chen X., Liu H. (2021). Changes in water environment in erhai lake and its influencing factors. Water 13 (10), 1362. doi: 10.3390/w13101362
Zuccarini P., Ciurli A., Alpi A. (2011). Implications for shallow lake manipulation: results of aquaria and enclosure experiments manipulating macrophytes, zooplankton and fish. Appl. Ecol. Environ. Res. 9, 123–140. doi: 10.15666/aeer/0902_123140
Appendix 1 List of the three lakes species.
Appendix 2 Correlation between nutrient status and α-diversity in lakes.
Keywords: macrophyte community, trophic state, environmental factors, plateau shallow lakes, α-diversity
Citation: Tian Y, Lv C, Huang L, Shan H, Wang H, Wen Z, Yin C, Chou Q, Zhang X, Ni L and Cao T (2023) Seasonal variation and nutrient jointly drive the community structure of macrophytes in lakes with different trophic states. Front. Mar. Sci. 10:1182823. doi: 10.3389/fmars.2023.1182823
Received: 09 March 2023; Accepted: 15 May 2023;
Published: 16 June 2023.
Edited by:
Alberto Basset, University of Salento, ItalyReviewed by:
Jiancai Deng, Chinese Academy of Sciences (CAS), ChinaArda Özen, Cankiri Karatekin University, Türkiye
Copyright © 2023 Tian, Lv, Huang, Shan, Wang, Wen, Yin, Chou, Zhang, Ni and Cao. This is an open-access article distributed under the terms of the Creative Commons Attribution License (CC BY). The use, distribution or reproduction in other forums is permitted, provided the original author(s) and the copyright owner(s) are credited and that the original publication in this journal is cited, in accordance with accepted academic practice. No use, distribution or reproduction is permitted which does not comply with these terms.
*Correspondence: Qingchuan Chou, chouqc@ihb.ac.cn