- 1Department of Science and Technology, National Oceanography Centre (NOC), Southampton, United Kingdom
- 2Consiglio Nazionale delle Ricerche (CNR) - Istituto per lo Studio degli Impatti Antropici e Sostenibilità in Ambiente Marino, Genoa, Italy
- 3Oceanic Platform of the Canary Islands (PLOCAN), Telde, Spain
- 4European Multidisciplinary Seafloor and Water-Column Observatory (EMSO ERIC), Rome, Italy
- 5Istituto Nazionale di Geofisica e Vulcanologia (INGV), Rome, Italy
- 6Aix Marseille Université, Université de Toulon, CNRS, IRD, MIO, UM 110, Marseille, France
- 7Institute of Oceanography, Hellenic Centre for Marine Research (HCMR), Attiki, Greece
- 8Sezione di Oceanografia, Istituto Nazionale di Oceanografia e di Geofisica Sperimentale (OGS), Sgonico, TS, Italy
- 9Consejo Superior de Investigaciones Científicas (CSIC-UTM), Barcelona, Spain
- 10Ifremer, Plouzané, France
- 11Service Hydrographique et Océanographique de la Marine (SHOM), Brest, France
- 12Research Infrastructure, Finnish Environment Institute, Helsinki, Finland
- 13Consiglio Nazionale delle Ricerche - Istituto di Scienze Marine (CNR-ISMAR), Venice, Italy
- 14Institut de Ciències del Mar (ICM-CSIC), Barcelona, Spain
Accurate and traceable measurements are required to understand ocean processes, to address pressing societal challenges, such as climate change and to sustainably manage marine resources. Although scientific and engineering research has resulted in advanced methods to measure Essential Ocean Variables (EOVs) there is a need for cross comparison of the techniques and traceability to recognized standards. Metrological laboratories are experienced in accredited methods and assessment of methodology. An EU INFRAIA-02-2020: Integrating Activities for Starting Communities project MINKE (Metrology for Integrated marine maNagement and Knowledge-transfer nEtwork https://minke.eu) brings European marine science and metrology Research Infrastructures together to identify synergies and create an innovative approach to Quality Assurance of oceanographic data. Quality depends both on the accuracy (that can be provided through the metrology component) and the completeness of the data sets. The collaboration between different Marine Research Infrastructures (RIs) places a fundamental role on assuring the completeness of the datasets, particularly at global scales. The MINKE project encourages enhancement through collaboration of national metrology laboratories and the oceanographic community. Metrological assessment of the accuracy and uncertainties within multidisciplinary ocean observations will provide data that are key to delivering policy information. Objectives across all the RIs are to facilitate ocean observation and build wider synergies. MINKE will investigate these synergies, then introduce metrology to the core of various EOV measurements. Currently the marine RIs cover laboratory and field operations, from the surface seafloor, coastal waters to deep sea, fixed ocean stations to ship and autonomous vehicle operations to ships of opportunity, and flux stations focusing on carbonate system variables. The nexus of these operations is the focal point for coordinated improvement of ocean observing methods. Measurement intercomparisons, traceability and uncertainty assessments should be at the core of the scientific observations. Specifically, MINKE will work with RIs and Metrology Institutes to improve the quality of dissolved oxygen, carbonate system, chlorophyll-fluorescence, ocean sound and current meter measurements, through access to metrology laboratories, Transnational Access and intercomparison studies across existing marine consortia and RIs. MINKE will also promote the development of absolute salinity observation, and improvements in marine litter measurements.
1 Introduction
European Research Infrastructure Consortia (ERICs) encourage interaction between research communities addressing large-scale questions and challenges (Arvanitidis et al., 2018). Marine Research Infrastructures (RIs) share many synergies, including shared locations, measurement types, and infrastructure (Dañobeitia et al., 2020). They largely measure GOOS (Global Ocean Observing System) EOVs (Miloslavich et al., 2018) and Essential Climate Variables (ECVs, Bojinski et al., 2014), following best practices (Pearlman et al., 2019) often endorsed by GOOS (eg: Lopez-Garcia et al., 2022).
Although joint research activities have different scientific foci, RIs aim to improve data quality and service provision at the European level. In Europe, statutory environmental monitoring programs such as the EU Marine Strategy Framework Directive (MSFD) are drivers for high quality measurements of good environmental status as is the United Nations (UN) Development Program’s Sustainable Development Goal 14 (Life Below Water).
Measurements from various sensors and samplers (some without traceable standards), hosted on different platforms within various RIs (Huber et al., 2021) can lead to challenges in applying best practices. Metrology laboratories associate quantification of uncertainties to in-situ measurements assuring the missing link between operational observations of EOVs and making comparable data. Metrology approaches such as accreditation (e.g., ISO/IEC 17025) provide a QA management framework when used alongside in-laboratory or field validation. The EURopean Association of national METrology institutes (EURAMET) aims to develop and disseminate a cost effective and internationally competitive measurement infrastructure for Europe. Its services support members to meet their national requirements and to establish a balanced European measurement infrastructure. It is important however to note that national metrology institutes handle only a limited number of marine variables with most of them being limited to temperature, pressure, density and conductivity.
Thus, the European landscape on metrology is characterized by significant variability in terms of stakeholders and metrology performing actors and is modulated by the increasing needs of the marine community. MINKE lays the foundations towards a pan-European calibration framework, to support the activities of Europe’s marine observatories. A framework of this kind will be vital to optimize the utility of the data supplied by these observatories to policymakers, research and industry at the transnational level. The major hurdles to integration include the vast variety of parameters, measuring techniques and instrumentation involved, the multiplicity of calibration methodologies in use, the lack of (and often discordance regarding) reference material, the dearth of validation procedures for many variables (particularly biological and ecological ones), the want of metrological rigor, and the scarcity of available expertise.
Introducing metrology at the core of EOV measurements has been identified, to improve reproducibility between RIs, and the EU INFRAIA-02-2020: Integrating Activities for Starting Communities project MINKE (Metrology for Integrated marine maNagement and Knowledge) was set up. It brings key European marine science and metrology RIs together to create an innovative approach to QA of oceanographic data. MINKE encourages closer interaction between metrology and oceanographic communities, assessing accuracy and uncertainty in multidisciplinary ocean observations providing data to deliver policy information.
Here are our perspectives on the importance of building synergies between European RIs and consortiums to enhance outcomes through interaction and cooperation. We describe initial steps of the MINKE project, outlining how metrology can be applied to measurements of: absolute salinity, dissolved oxygen, pH, CO2 gas, chlorophyll fluorescence and emerging EOVs like underwater sound, current direction measurements and marine litter (Figure 1; Table 1). MINKE is a step towards coordinated ocean observing method improvement through harmonization and intercomparison, with common approaches to verification.
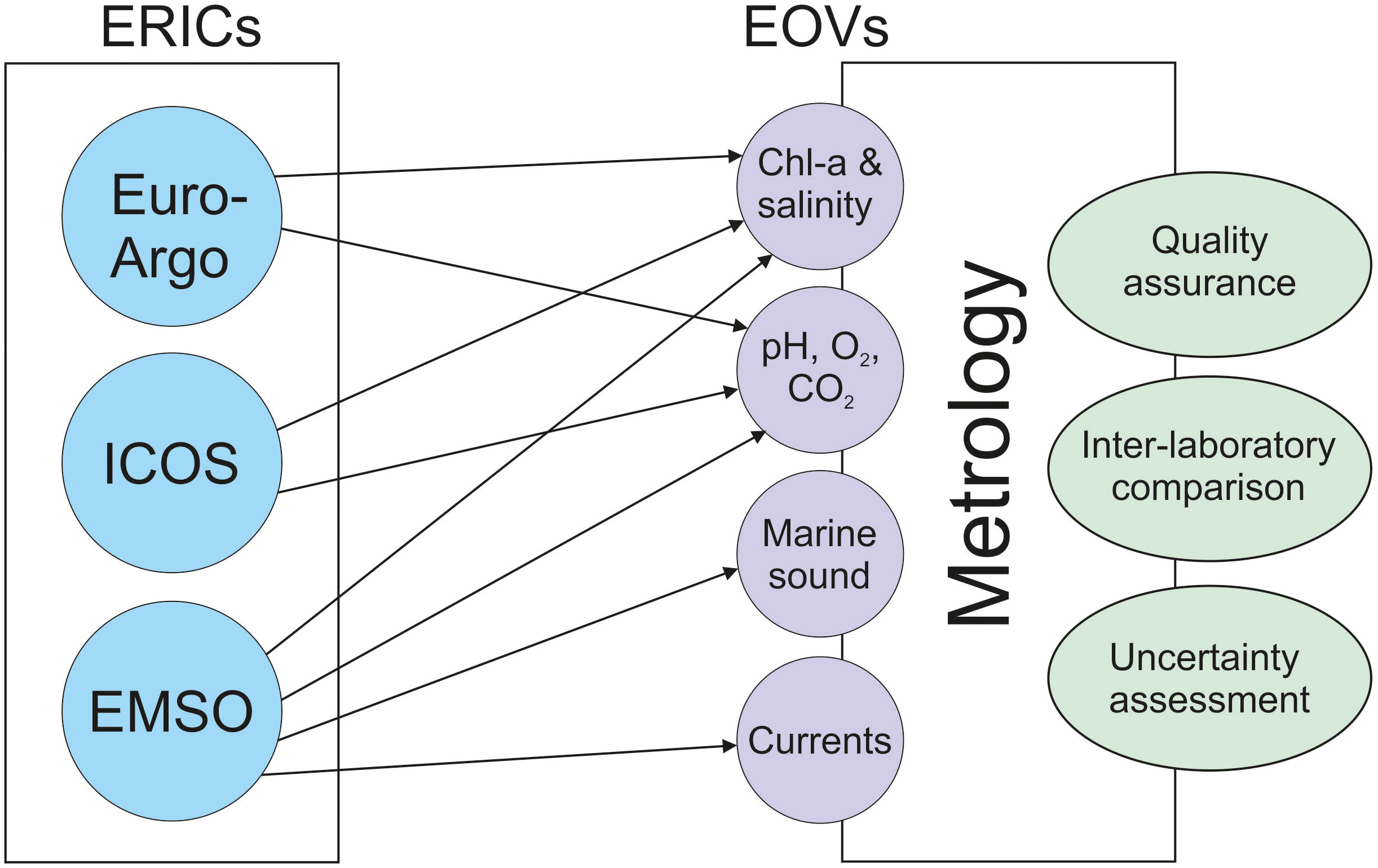
Figure 1 Potential overlaps between examples of the marine European Research Infrastructure Consortia (RIs) and metrology methods, to improve measurements of various Essential Ocean Variables (EOVs).
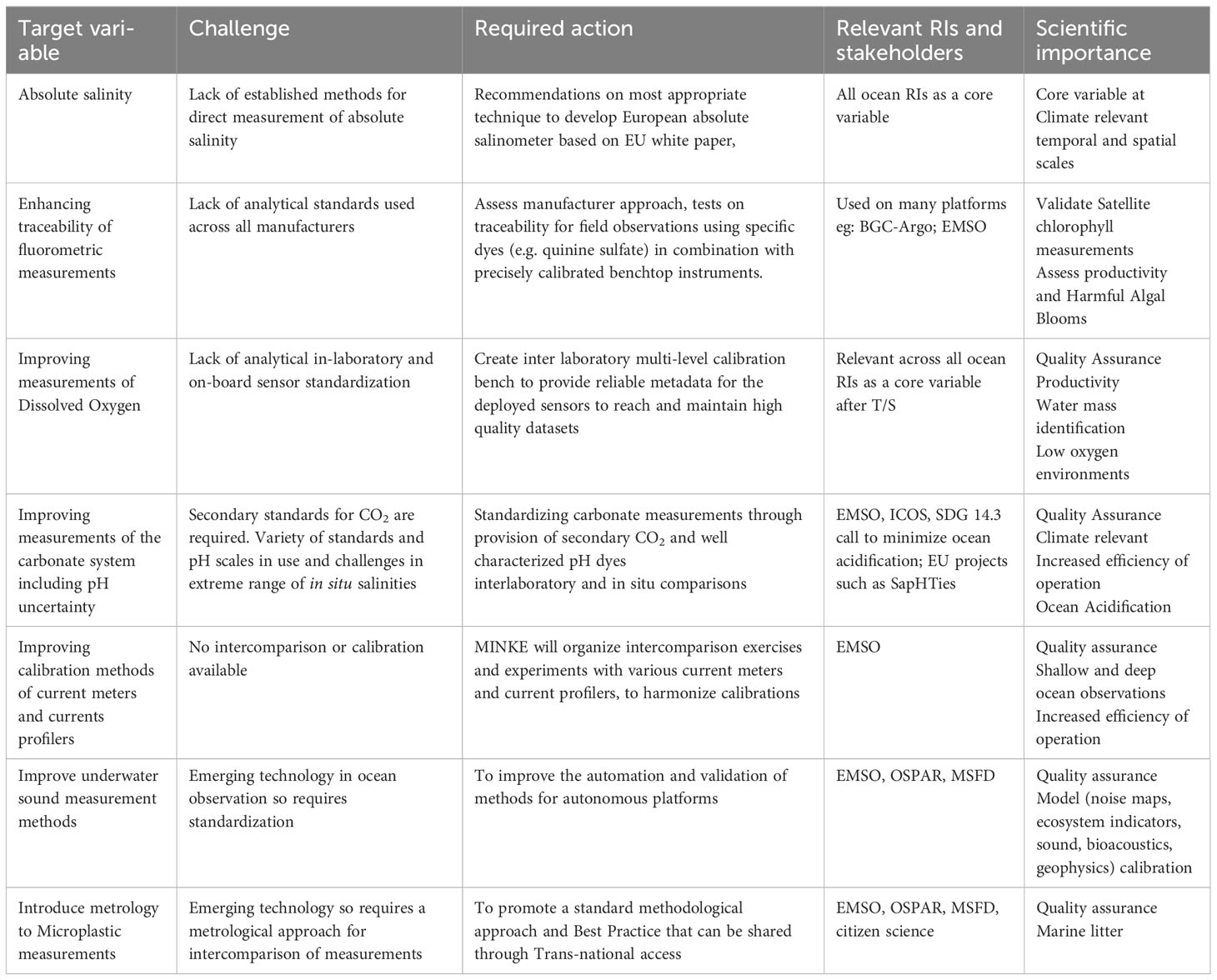
Table 1 Target areas for method improvement, including the challenges, required actions and potential scientific interest and relevance to Research Infrastructures (RIs).
2 The European ocean observation landscape
Marine RIs include EMSO (European Multidisciplinary Seafloor and water column Observatory), providing multidisciplinary data and services from surface to seabed from Regional Facilities in the open ocean around Europe. Also, ICOS (International Carbon Observation system), which focuses on the carbonate system and cross domain (ocean, atmospheric and ecosystem) measurements of greenhouse gases. Euro-Argo and GROOM use autonomous profilers and gliders to make measurements in the open ocean, JERICO has a coastal focus, while fluvial regions are the focus for DANUBIUS. RIs encourage sustained observations, aligning with the UN Decade of Ocean Science for Sustainable Development (von Schuckmann et al., 2020).
All RIs need to ensure high quality and traceable measurements with FAIR (Findable, Accessible, Interoperable and Reusable) data (Wilkinson et al., 2016), to achieve broad impact and ensure longevity with multiple uses for data. RIs aim to meet multiscale (national, regional to global) ocean and climate challenges through integrated and sustained observations (Dañobeitia et al., 2020). Collaboration among European marine RIs aligns with and has contributed to the OceanObs’19 Conference statement (Speich et al, 2019) and objectives of the UN Decade of Ocean Science for Sustainable Development (2021–2030).
Identification of synergies between marine RIs and Regional Metrology Organizations includes the introduction of metrology and traceable standards to promote data quality and reduce measurement uncertainties. Continuous exchanges between marine RIs through national and international projects will strengthen the role of RIs providing homogeneity of procedures for reliable intercomparisons (Figure 1).
3 Improvements in methodology and data assessment
MINKE will address metrological needs in the marine environment, progressing methodological thoroughness and measurement quality through harmonization of procedures. Challenges to effective integration between RIs include the variety of parameters, measuring techniques and instrumentation involved, alongside the multiplicity of calibration and validation methodologies often lacking accepted reference materials. Extensive effort is being devoted to fostering cooperation between groups directly involved in calibrating marine instruments and sensors, nurturing consensus on calibration reference material, methodologies and procedures, harmonizing operating practices then promoting knowledge exchange with a rational coordination of resources across the marine observing community.
MINKE will provide recommendations and demonstrations to improve data quality for a broad variety of biogeochemical variables (see Figure 1), many obtained from techniques that currently lack clear calibration and quality assessment protocols. Core tasks in MINKE are: addressing measurand definitions and reference material, methodology and interoperability, uncertainty estimation, common terminology and Best Practice, and Transnational Access.
3.1 Measurand definitions and reference material
Creating a permanent framework of cooperation between Europe’s marine research community and its system of national metrological institutions is ongoing. MINKE is working on the issues of measurand definitions and reference material in the context of calibrating instruments for measuring ocean variables. This synergy ensures more metrologically consistent observations, allowing better compliance of measurements with relevant international norms and greater comparability of resulting data. Absolute salinity, ocean currents, dissolved oxygen, pH and pCO2 have been targeted for investigation. These variables are widely measured and significant in the context of climate change, ocean circulation, oxygen deficiency/production, and ocean acidification, and they encompass many common types of calibration-validation issues affecting marine measurements.
3.2 Methodology and interoperability
MINKE will map the breadth and quality of collaborations, services, and training currently available for the marine instrumentation management in Europe. This will establish existing levels of harmonization for calibration, validation, intercomparison and proficiency testing at different levels (from in-house to internationally) to identify commonalities and gaps. Applicability of interoperability standards, such as the OGC (Open Geospatial Consortium) Sensor Model Language (SensorML), for handling instrument calibration information within, between and across marine observing networks and data management platforms is also being explored.
3.3 Uncertainty estimation
Measurement uncertainty is a principal concept in metrology and laboratory analyses but estimating it for marine measurands is challenging. This is multiplied when dealing with measurements from long-term autonomous instrumentation. However, standalone instruments working remotely, and often networked in real time, represent the modern face of marine environmental monitoring, and there is a lack of clearly defined practices for determining measurement uncertainty in similar operational settings. MINKE will review existing Best Practices in determination of uncertainty for field EOV measurements.
3.4 Common terminology and best practice
Definition and adoption of common terminology (ontologies) is a crucial step forward if critical technical and methodological issues, and reporting and metadata conventions, are to be addressed. MINKE aims to build on a specific vocabulary for measuring the marine environment using existing Guides to the expression of uncertainty in measurement (GUM series) and the international vocabulary of metrology - Basic and general concepts and associated terms (VIM) of the Joint Committee for Guides in Metrology (JCGM). MINKE will investigate Best Practice in calibration methodologies with a view to standardize approaches and procedures.
3.5 Transnational access
TNA will enhance cooperation between metrology laboratories, RIs and other stakeholders such as sensor manufacturing industries. It will provide access to ISO (International Organization for Standardization) accredited laboratories and promote Best Practice, and enable trials of novel low-cost methodology at RI facilities. Best Practices will be shared with the community at meetings, conferences and through peer-reviewed publication (e.g., OceanSITES and OOI Best Practices).
4 Advanced methods for improving data quality and services
Interlaboratory comparisons (ILC) and uncertainty assessment should be at the core of all measurements made. All scheduled intercomparison exercises within MINKE will involve specialists from metrological institutions and RI observatory personnel to define common agreed procedures for regular sensor data assessment and provision of long-term data products with known uncertainties. A diverse community of scientists and early career researchers external to the project, will improve data assessment through metrology training events and educational workshops. MINKE progress to date, on each target EOVs, and emerging EOVs, is described in the following subsections (and Table 1).
4.1 Improving measurements of the carbonate system
To understand the carbonate system requires measurement of at least two of: pCO2, Total Alkalinity (TA), pH and Dissolved Inorganic Carbon (DIC). In situ observations typically focus on sensor-based pH and pCO2 because Alkalinity and DIC require lab-based chemical reference methods. Products such as CO2 and other greenhouse gases flux can then be calculated (Heiskanen et al., 2022). MINKE will enhance data quality through secondary pCO2 gas standards production, building on the ICOS CO2 intercomparison (Steinhoff et al., in prep). Temperature and salinity need to be traceable to SI (International System of Units) standards, as these essential ocean variables are key within equations used to calculate all aspects of the carbonate system (Dickson et al., 2007).
Ocean Acidification (OA) assessments using pH requires comparable, multi decadal time scale in situ observations. In-situ solid-state field-effect transistor (ISFET) based sensors are commonly used (Martz et al., 2010) but uncertainty is high. GOA-ON (Global Ocean Acidification Observing Network) goals are already close to what can be achieved by primary standards in metrology laboratories. Other challenges include the variety of standards and pH scales in use, and salinity ranges from coastal water to the open Atlantic Ocean and enclosed European seas (e.g., Mediterranean Sea, Baltic Sea, Black Sea). Rigorous metrological methods are required to streamline individual methods.
Carbonates and dissolved oxygen sensors are frequently deployed jointly at RIs to survey ocean thermodynamics so MINKE will combine organization of in-situ carbonate and dissolved oxygen intercomparisons under a controlled environment. Further interlaboratory comparisons using measurements of a purified, fully characterized dye pH standard distributed to laboratories will create a standard approach for measuring total pH for climate assessments.
4.2 Improving dissolved oxygen measurement
Dissolved oxygen is the third-most measured EOV after temperature and salinity, providing a sensitive early warning system for subtle ongoing ocean deoxygenation (Keeling et al., 2010; Bosch et al., 2010), which requires an expanded scale of observation in space and time (Bittig et al., 2018). In situ sensors are essential, but data must be high quality and intercomparable. Within RIs there are approaches to standardization, e.g., from gliders (Lopez-Garcia et al., 2022), that should reach across platforms. To improve dissolved oxygen measurement comparison MINKE has designed a multi-platform laboratory calibration bench facility, with thermostated bath and two mass flow controllers for oxygen and nitrogen gases, to host stand-alone and integrated dissolved oxygen sensors providing multipoint calibration with temperature and oxygen ranges matching coastal to open ocean waters.
4.3 Enhancing traceability of fluorometric measurements
In situ fluorometry measures variables from algae pigments (chlorophyll, phycobilins) to carbon compounds (colored dissolved organic matter, PAH). Chlorophyll measurement can address Phytoplankton Biomass and Diversity EOVs, spanning methodologies from in situ small solid state fluorometric sensors, to laboratory based high performance liquid chromatography (HPLC), to satellite observations. In-situ use specific wavelengths and operate on multiple platforms. Pre and post deployment chlorophyll-a fluorometer validation is required but there is currently no physical standard for calibration.
Fluorometer manufacturers want to improve calibration traceability and method standardization and MINKE will facilitate this through fluorometry measurements intercomparison, building on fluorometer calibration workshops for the Baltic Sea FerryBox community (Seppälä et al., 2021) and a series of new workshops, including mesocosm experiments in ultra-oligotrophic conditions to compare sensitivity and resolution in real-world marine conditions (involving JERICO-S3 and AQUACOSM-plus RI communities).
Specific actions include checking fluorescence temperature dependence for some calibrants, evaluating sensor-specific wavelength settings on sensor-to-sensor variability, and testing if traceable calibration may be improved using calibrated bench-top reference spectrofluorometer and a calibrant with relatively constant fluorescence quantum yield, like quinine sulfate. Test results will provide recommendations for future actions.
4.4 Improving absolute salinity measurements
Chemical and biological EOVs require co-located, validated physical measurements, (salinity, temperature, and pressure) to create science quality measurements. Common procedures for temperature and salinity validation include CTD casts and post-deployment calibration baths on board research vessels (Cowley, 2022), plus comparisons with climatological data. Salinity is measured as Practical Salinity (a measure of seawater conductivity), but in 2010 a new standard, Thermodynamic Equation of Seawater (TEOS-10), using Absolute Salinity (mass fraction of salt in seawater), was adopted by the Intergovernmental Oceanographic Commission (IOC) of UNESCO, the International Association for Physical Sciences of the Oceans (IAPSO) and the Scientific Committee on Oceanic Research (SCOR) for calculation of seawater thermodynamic properties (Valladares et al., 2011). TEOS-10 is endorsed by the International Union of Geodesy and Geophysics (IUGG) and replaces the old International Equation of State of Seawater (EOS-80) standard which has been in place for 30 years.
Technology development for Absolute Salinity measurements is still at an early stage. (Le Menn and Naïr, 2022). A commercial sensor for measuring Absolute Salinity from refractive index determinations has been developed, and is being marketed by NKE Instrumentation. Europe has an opportunity to build a strong foothold in this space with potential for growth to a global leadership role. It is uniquely positioned to develop operating frameworks and facilities required to mainstream environmental Absolute Salinity measurements from local to global marine monitoring and modeling networks, using existing and planned regional marine observing infrastructure aggregations and observatories (Le Menn and Naïr, 2022; Le Menn et al., 2023).
Improving Absolute Salinity use in the oceanographic community requires effort to develop: 1) traceable and validated methods to measure seawater Absolute Salinity, including measurement protocols with uncertainties within oceanographic requirements (typically <0.002 g/kg), 2) validated methods, including traceable reference materials, for both laboratory and in situ, that are independent measuring instrument type, 3) new Absolute Salinity sensors design and validation using sound metrological characterizations especially at high pressures, 4) Best Practice Guides and recommendations for in-house instrument calibration and testing for Absolute Salinity measurements, 5) promoting technology uptake, supporting infrastructure developed for Absolute Salinity measurement internationally.
4.5 Improving calibration methods of current compass and tilt meters
Acoustic Doppler Current Profilers (ADCP) are used to measure oceanic circulation, allowing water velocity profiles quantification. Velocity is obtained by measuring Doppler shifts of echoes backscattered by suspended particles in water and direction related to an electronic compass and tilt sensors. ADCPs are equipped with a magnetic compass and tilt sensors for converting measurements to a terrestrial reference frame. A standardized method is lacking that considers compass sensitivity to the magnetic environment. Calibration in the configuration of use will reduce measurement errors.
ADCPs and Doppler current-meters measure surface and subsurface currents (EOVs and ECVs). Typically, they are deployed on a mooring, or in a cage. Mooring configuration and battery pack location can affect compass measurements, degaussing battery packs for example may improve results (Hamilton, 2001). There is a requirement for intercomparison exercises and experiments assessing current-meters and current profilers from different RIs, to evaluate effects of caged equipment on current direction measurement accuracy. Within MINKE, experiments have been conducted on the SHOM (Service hydrographique et océanographique de la marine) platform for current meter and current profilers allowing compass calibration and studies on measurement error origins (Le Menn and Le Goff, 2007; Le Menn et al., 2014) and measurements to validate the calibration bench to detect anomalies in Doppler shifts measurements made by current meter transducers and current profilers (Le Menn and Morvan, 2020). These experiments will determine Best Practices in the mooring cage and line conception, increasing confidence levels in sea currents direction measurements (a Global Climate Observing System ECV).
4.6 Improving underwater sound measurement methods
Underwater sound can be exploited to study the sea, its boundaries, and its contents (Howe et al., 2019). Passive acoustics monitoring (PAM) is used to characterize ocean soundscapes by monitoring sound levels and frequency over time and space, detecting and identifying sources (biological, natural, or anthropogenic) contributing to the sound field. Data are often acquired for a single purpose so to enable intercomparison and ground truthing may require metrological method standardization.
Methods are still in development for robust ocean sound (emerging EOV) field measurement on mobile and fixed platforms. Processing methods need to be improved and compared to increase reliability and reduce uncertainty to produce data products like ocean noise maps, habitat distribution and species density estimations.
MINKE will review underwater ambient background sound features and their dependence on the marine environment and weather conditions; make an inventory of off-shelf devices for collecting ocean sound measurements including available software and algorithms for data processing; and establish Best Practices for underwater ambient noise measurement and estimation: defining robust metrics and their efficient estimation, while preserving initial signal properties.
In passive acoustics sound source characteristics are generally unknown, and received signals can contain propagation effects depending on the environment and distance from source to receiver. Automatic classification algorithms should deal with these propagation effects and the frequency content of the received sound whether from physical, biological, or anthropogenic origin. Trucco et al. (2023) considered temporal correlation in wind and rainfall prediction from underwater acoustic noise measurements, showing that modern machine learning techniques are superior compared to empirical equations. MINKE will share and evaluate algorithms for effective on-board processing to reduce acoustic data dimension and/or select which signals or features should be stored.
MINKE will perform an intercomparison exercise, deploying autonomous devices at a test site evaluating relative performance, assessing the capability to collect data useful for various applications such as underwater ambient noise modeling, noise pollution monitoring and MSFD indicators (Descriptor 11). MINKE will find a common approach for comparison between coarse resolution modeling and temporal high-resolution field measurement in local areas. MINKE will ensure that underwater sound measurements are reliable and comparable across different RIs and monitoring programs. Metrology use in ocean sound measurement is crucial to advance our understanding of the marine environment to develop effective conservation and management strategies.
4.7 Marine litter
Monitoring marine litter is of international importance for good environmental status (MSFD, OSPAR) and a research focus by RIs such as EMSO. MINKE will focus on shore-line macro litter for data availability and engagement with citizen scientists. MINKE will harmonize datasets from the Latin American Network of Litter Scientists (www.reciba.org), the East Pacific network “Plastic Pollution: Science to Solutions’’ (https://www.pacificplasticssciencetosolutions.com/), and the GESAMP (Group of Experts on the Scientific Aspects of Marine Environmental Protection) “Sources, Fate and Effects of plastics and microplastics in the marine environment”.
MINKE TNA makes available a Fourier Transform Infrared (FTIR) Imaging facility (NOC), an infrared imaging spectroscopic tool to detect, visualize and characterize natural and anthropogenic materials in complex environmental sample types. The facility currently specializes in detection, identification, and chemical characterization (polymer types) and plastic contaminant morphological properties from several centimeters (macroplastics) down to 3 microns (microplastics) extracted from environmental samples or plastic debris collected individually. Approaches to marine litter analysis will be shared with the international community.
5 Conclusions
Increased synergies between oceanographic and metrological communities will improve oceanographic measurement quality. Involving the metrology community and their established approaches improve standardization, methodology and Best Practice in essential ocean variables measurements. MINKE will promote improvements through interaction between research infrastructures, enhancing cooperation with national metrology laboratories, developing calibration, measurement capabilities and reference materials, uncertainty models and validation methods. Four main tasks in MINKE include definitions and reference material; methodology and interoperability; uncertainty estimation; common terminology and Best Practice.
TNA will be a user-friendly tool to access ISO accredited metrology laboratories and enhance cooperation between metrology laboratories and stakeholders. Citizen science components of MINKE will contribute Best Practices globally and introduce metrology to emerging EOVs such as marine litter. Interlaboratory comparisons are key to assessing measurement uncertainty for metrology laboratories and MINKE has already organized several with participation encouraged across all marine focused networks and RIs.
Author contributions
SH, RB and SP wrote the first draft with contributions from PF. SH led the writing throughout, with whole sections contributed by RN, AG, PL-G, DL, FS, MM and JS. In later drafts there were additional contributions from AG, KS, SN, ED, JD and LC. The initial concept of MINKE was from GP and JP. All authors contributed to the article and approved the submitted version.
Funding
The authors declare financial support was received for the research, authorship, and/or publication of this article. This paper was a milestone within the MINKE project, which has received funding from the European Union’s Horizon 2020 research and innovation program under grant agreement 101008724 and under the grant agreement no. 731031(EMSO-link, https://cordis.europa.eu/project/id/731036). SH’s time was also covered by the UK Natural Environment Research Council Climate. Linked Atlantic Section Science (CLASS) project (NE/R015953/1) and iFADO project (Innovation in the Framework of the Atlantic Deep Ocean), which was supported with ERDF funds from the INTERREG Atlantic Area Programme under contract EAPA 165/2016 and grant agreement no. 862923 (AtlantECO, Atlantic Ecosystems Assessment, Forecasting & Sustainability). ICM-CSIC acknowledges the institutional support of the ‘Severo Ochoa Centre of Excellence’ accreditation (CEX2019-000928-S). PLG was supported by TechOceanS project, which received funding from the European Union’s Horizon 2020 research and innovation programme under grant agreement No 101000858. This output reflects only the author’s view, and the Research Executive Agency cannot be held responsible for any use that may be made of the information contained therein.
Acknowledgments
With thanks to the two reviewers and their comments on the text.
Conflict of interest
The authors declare that the research was conducted in the absence of any commercial or financial relationships that could be construed as a potential conflict of interest.
Publisher’s note
All claims expressed in this article are solely those of the authors and do not necessarily represent those of their affiliated organizations, or those of the publisher, the editors and the reviewers. Any product that may be evaluated in this article, or claim that may be made by its manufacturer, is not guaranteed or endorsed by the publisher.
References
Arvanitidis C. D., Warwick R. M., Somerfield P. J., Pavloudi C., Pafilis E., Oulas A., et al. (2018). Research Infrastructures offer capacity to address scientific questions never attempted before: Are all taxa equal? PeerJ Preprints 6, e26819v26812. doi: 10.7287/peerj.preprints.26819v2
Bittig H. C., Körtzinger A., Neill C., van Ooijen E., Plant J. N., Hahn J., et al. (2018). Oxygen optode sensors: principle, characterization, calibration, and application in the ocean. Front. Mar. Sci. 4. doi: 10.3389/fmars.2017.00429
Bojinski S., Verstraete M., Peterson T. C., Richter C., Simmons A., Zemp M. (2014). The concept of essential climate variables in support of climate research, applications, and policy. Bull. Am. Meteorological Soc. 95, 1431–1443. doi: 10.1175/BAMS-D-13-00047.1
Bosch T., Colijn F., Ebinghaus R., Körtzinger A., Latif M., Matthiessen B., et al. (2010). World ocean review 2010: living with the oceans. editors. by Gelpke, N., Visbeck, M., Mare, H. 234 pp. Available at: https://oceanrep.geomar.de/id/eprint/22086.
Cowley R. (2022). Report on the Quality Control of the IMOS East Australian Current (EAC) Deep Water Moorings Array (Hobart, Australia: CSIRO Oceans and Atmosphere). doi: 10.26198/rgns-v363
Dañobeitia J. J., Pouliquen S., Johannessen T., Basset A., Cannat M., Pfeil B. G., et al. (2020). Toward a comprehensive and integrated strategy of the european marine research infrastructures for ocean observations. Front. Mar. Sci. 7. doi: 10.3389/fmars.2020.00180
Dickson A. G., Sabine C. L., Christian J. R. (2007). Guide to Best Practices for Ocean CO2 Measurements. PICES Special Publication 3, 191.
Hamilton J. M. (2001). “AccurateOcean current direction measurements near the magnetic poles,” Paper presented at the The Eleventh International Offshore and Polar Engineering Conference, (Stavanger, Norway: ISOPE).
Heiskanen J., Brümmer C., Buchmann N., Calfapietra C., Chen H., Gielen B., et al. (2022). The integrated carbon observation system in Europe. Bull. Am. Meteorological Soc. 103 (3), E855–E872. doi: 10.1175/BAMS-D-19-0364.1
Howe B. M., Miksis-Olds J., Rehm E., Sagen H., Worcester P. F., Haralabus G. (2019). Observing the oceans acoustically. Front. Mar. Sci. 6, 426. doi: 10.3389/fmars.2019.00426
Huber R., D'Onofrio C., Devaraju A., Klump J., Loescher H. W., Kindermann S., et al. (2021). Integrating data and analysis technologies within leading environmental research infrastructures: Challenges and approaches. Ecol. Inf. 61, 101245. doi: 10.1016/j.ecoinf.2021.101245
Keeling R. F., Körtzinger A., Gruber N. (2010). Ocean deoxygenation in a warming world. Annu. Rev. Mar. Sci. 2, 199–229. doi: 10.1146/annurev.marine.010908.163855
Le Menn M., Le Goff M. (2007). A method for absolute calibration of compasses. Meas. Sci. Technol. 18, 1614–1621. doi: 10.1088/0957-0233/18/5/053
Le Menn M., Lusven A., Bongiovanni E., Le Dû P., Rouxel D., Lucas S., et al. (2014). Current profilers and current meters: compass and tilt sensors errors and calibration. Meas. Sci. Technol. 25, 85801. doi: 10.1088/0957-0233/25/8/085801
Le Menn M., Morvan S. (2020). Velocity calibration of doppler current profiler transducers. J. Mar. Sci. Eng. 8, 847. doi: 10.3390/jmse81108473
Le Menn M., Naïr R. (2022). Review of acoustical and optical techniques to measure absolute salinity of seawater. Front. Mar. Sci. 9. doi: 10.3389/fmars.2022.1031824
Le Menn M., Seitz S., Nair R., Ntoumas M. (2023). White paper on advances in absolute salinity measurements. Metrology for Integrated Marine Management and Knowledge-Transfer Network, MINKE. doi: 10.5281/zenodo.7599993
Lopez-Garcia P., Hull T., Thomsen S., Hahn J., Queste B. Y., Krahmann G., et al. (2022). OceanGliders oxygen SOP 59. doi: 10.25607/OBP-1756
Martz T. R., Connery J. G., Johnson K. S. (2010). Testing the Honey- well Durafet® for seawater pH applications. Limnol. Oceanogr.- Meth 8, 172–184. doi: 10.4319/lom.2010.8.172
Miloslavich P., Bax N. J., Simmons S. E., Klein E., Appeltans W., Aburto-Oropeza O., et al. (2018). Essential ocean variables for global sustained observations of biodiversity and ecosystem changes. Global Change Biol. 24, 2416–2433. doi: 10.1111/gcb.14108
Pearlman J., Bushnell M., Coppola L., Karstensen J., Buttigieg P. L., Pearlman F., et al. (2019). Evolving and sustaining ocean best practices and standards for the next decade. Front. Mar. Sci. 6. doi: 10.3389/fmars.2019.00277
Seppälä J., Maunula P., Haavisto N., Rehder G., Karlson B., Willstrand Wranne A., et al. (2021). “Transnational FerryBox monitoring in the Baltic Sea: common measures for quality assurance,” in IEEE International Workshop on Metrology for the Sea; Learning to Measure Sea Health Parameters (MetroSea). (IEEE), 121–125. Available at: https://doi.org/10.1109/MetroSea52177.2021.9611608
Speich S., Lee T., Muller-Karger F., Lorenzoni L., Pascual A., Jin D., et al. (2019). Editorial: oceanobs'19: an ocean of opportunity. Front. Mar. Sci. 6. doi: 10.3389/fmars.2019.00570
Trucco A., Barla A., Bozzano R., Pensieri S., Verri A., Solarna D. (2023). Introducing temporal correlation in rainfall and wind prediction from underwater noise. IEEE J. Oceanic Eng. 48 (2), 349–364. doi: 10.1109/JOE.2022.3223406
Valladares J., Fennel W., Morozov E. G. (2011). Announcement: replacement of EOS-80 with the international thermodynamic equation of seawater–2010 (TEOS-10). Deep-Sea Res. 58:978. doi: 10.1016/j.dsr.2011.07.005
von Schuckmann K., Holland E., Haugan P., Thomson P. (2020). Ocean science, data, and services for the UN 2030 Sustainable Development Goals. Mar. Policy 121, 104154. doi: 10.1016/j.marpol.2020.104154
Keywords: essential ocean variables (EOVs), metrology, ocean sound, dissolved oxygen, carbonate system, chlorophyll-fluorescence, current meters, absolute salinity
Citation: Hartman SE, Gates AR, Lopez-Garcia P, Bozzano R, Delory E, Favali P, Lefevre D, Chirurgien L, Pensieri S, Petihakis G, Nair R, Neves S, Dañobeitia JJ, Salvetat F, Le Menn M, Seppälä J, Schroeder K and Piera J (2023) Proposed synergies between oceanography and metrology. Front. Mar. Sci. 10:1192030. doi: 10.3389/fmars.2023.1192030
Received: 22 March 2023; Accepted: 06 October 2023;
Published: 24 October 2023.
Edited by:
Gonzalo S. Saldías, University of Bío-Bío, ChileReviewed by:
Fabio Nascimento, Federal University of Rio de Janeiro, BrazilPasquale Daponte, University of Sannio, Italy
Copyright © 2023 Hartman, Gates, Lopez-Garcia, Bozzano, Delory, Favali, Lefevre, Chirurgien, Pensieri, Petihakis, Nair, Neves, Dañobeitia, Salvetat, Le Menn, Seppälä, Schroeder and Piera. This is an open-access article distributed under the terms of the Creative Commons Attribution License (CC BY). The use, distribution or reproduction in other forums is permitted, provided the original author(s) and the copyright owner(s) are credited and that the original publication in this journal is cited, in accordance with accepted academic practice. No use, distribution or reproduction is permitted which does not comply with these terms.
*Correspondence: Susan Hartman, c3VoQG5vYy5hYy51aw==