- 1Guangdong Provincial Laboratory of Marine Biotechnology, Institute of Marine Sciences, Shantou University, Shantou, China
- 2College of Chemical and Environmental Engineering, Hanshan Normal University, Chaozhou, China
- 3Department of Applied Chemistry, Northumbria University, Newcastle upon Tyne, United Kingdom
Introduction: B vitamins play a crucial role in shaping phytoplankton and zooplankton communities in marine ecosystems, yet their impact on community dynamics remains poorly understood.
Methods: We carried out in situ incubation experiments of B vitamins supplementation to explore the response pattern of phytoplankton and zooplankton community compositions.
Results: The results showed that vitamins B1, B2, B6 and B12 promoted the growth of phytoplankton, and the total Chl α in 87.5% of the supplemented B vitamin treatments showed a significant positive response (p < 0.05). Supplementation with these B vitamins significantly altered the community composition of phytoplankton, and 75% of the B vitamin-supplemented treatments showed an increase in the relative abundance of Minutocellus, Thalassiosirales, Odontella, Prymnesiales and Ditylum, considered mainly to be the result of B vitamin auxotrophy. In contrast, a significant decrease in Copepoda, including Calanoida and Cyclopoida, was observed in 87.5% of treatments. The observed shifts in community composition were attributed to the auxotrophy of certain diatoms and Prymnesiales for B vitamins. These shifts subsequently led to negative correlations (Spearman Rho < -0.8) between the abundance of these phytoplankton species and Copepoda populations.
Discussion: These findings advance our understanding of the complex interactions between micronutrient availability and plankton community dynamics.
1 Introduction
B vitamins are water-soluble micronutrients and act as key coenzyme factors in catalyzing many important biochemical reactions in biological central metabolism. These reactions include C-C bond rearrangement reduction and methyl transfer reactions, deoxyribose/fatty acid/carbohydrate/branched amino acid synthesis, electron transfer in redox reactions and the fixation of CO2 (Matthews et al., 2003; Frank et al., 2007; Dowling et al., 2012; Waldrop et al., 2012). For the past few decades, scientists have been studying the production, transfer, and circulation of nutrients in the ocean. However, previous studies have focused on the roles of C, N, P and Si in the regulation of marine ecological processes (Sañudo-Wilhelmy et al., 2014). The importance of vitamin B in aquatic products was first considered in the early 20th century (Helliwell, 2017). B vitamins in the ocean are mainly derived from bacteria and/or phytoplankton. Croft et al. (2006) showed that 76% of the 400 marine bacteria surveyed synthesized vitamin B1, and 50% of sequenced phytoplankton had B1 synthesis pathways. Vitamin B2 and B6 can be synthesized by plants and bacteria (Monteverde et al., 2017). 78% of marine bacteria and some of phytoplankton in Sañudo-Wilhelmy et al. (2014) had a pathway to synthesize vitamin B7 de novo. However, it was generally believed that eukaryotic couldn’t synthesize vitamin B12(Bertrand et al., 2011; Helliwell, 2017). It could only be synthesized by some bacteria and archaea (Rodionov et al., 2002). At the same time, B vitamins auxotrophy were also widespread in the ocean (Croft et al., 2006; Sañudo-Wilhelmy et al., 2014). Without an exogenous source of B vitamins, some effects can occur: impaired central metabolism of organisms deficient in B vitamins, blocked cell growth, and significant impacts on many biological processes in the ocean. These processes include primary productivity, phytoplankton community structure, and biocarbon pumps (Giovannoni, 2012; Sañudo-Wilhelmy et al., 2012; Monteverde et al., 2017).
Marine microbial loop supplements to the main food chain. As the main energy flow path in the sea, it converts dissolved organic matter difficult to transfer into granular organic matter, and then return it to the main food chain (Nichols, 2003; Pomeroy et al., 2007). Phytoplankton, which are primary producers in the ocean, play a key role in the microbial loop; however, their growth and reproduction are affected by certain B vitamins. Previous studies have shown that although vitamin B12 cannot be synthesized by phytoplankton de novo, more than half of phytoplankton species require it (Croft et al., 2005; Croft et al., 2006; Watanabe and Bito, 2018). While most phytoplankton species are deficient of vitamin B1, it is produced by most diatoms (Tang et al., 2010; Heal et al., 2017). Previous field and laboratory nutrient amendment studies showed that the availability of vitamins B1 and B12 strongly affected the abundance and community composition of phytoplankton and bacteria (Sañudo-Wilhelmy et al., 2006; Bertrand et al., 2011; King et al., 2011; Koch et al., 2011; Koch et al., 2012; Joglar et al., 2020; Joglar et al., 2021). Some phytoplankton rely on B7 during their growth process (Helliwell, 2017).
Zooplankton feed on phytoplankton, bacteria and debris, and also food for fish and other aquatic animal, thus act as an important link in the vertical circulation of marine nutrients (Chiba et al., 2018; Abo-Taleb, 2019). In addition, due to their small size and short life cycle, zooplankton are very sensitive to environmental stress, with their biomass and community structure altering significantly following a disturbance. These changes alter nutrient linkages in marine microbial food webs, affecting fish and other marine animal stocks (Chiba et al., 2018; Srichandan et al., 2021). Several fish species, such as bighead carp, herring, mackerel, various juvenile fishes and baleen whales rely on zooplankton, particularly Copepoda, as a food source. In fact, the yield of herring in Europe was shown to be closely related to the abundance and distribution of Copepoda (Bils et al., 2022; Randall et al., 2022).
However, most studies of phytoplankton and bacterial responses to B vitamins are only limited to vitamins B1 and B12. There is little coverage of the effect of other B vitamins. Moreover, most studies have focused on the factors influencing transfer dynamics of B vitamins from phytoplankton and bacteria to zooplankton (e.g., seasonal variations (Fridolfsson et al., 2019), species variations (Fridolfsson et al., 2020), and local environmental conditions (Majaneva et al., 2020). Knowledge on the effects of B vitamins on zooplankton abundance and community compositions, especially for Copepoda, remains scarce. In this context, we conducted a series of in situ experiments to evaluate the response of prokaryote, phytoplankton and Copepoda biomasses/relative abundance to the addition of B1, B2, B6 and B12. The aims of our study were to explore the effects of different B vitamins on phytoplankton biomass and community composition and, more importantly, to further analyze the roles of B vitamins on the growth limitation of Copepoda.
2 Materials and methods
2.1 Study site
Fieldwork and sampling were conducted at the coastal experimental station of Shantou University, located on Nan’ao Island (23.48°N, 117.11°E, Figure 1), Guangdong, China: Shantou. Nan’ao Island features multiple bays with typical tropical and subtropical characteristics. Its surrounding waters exhibit complex hydrological conditions (Cai et al., 2011; Shu et al., 2018) due to the influence of surface runoff from the Hanjiang and Huanggang Rivers and upwelling from the Taiwan Shoal, which usually occurs in summer (June to September) (Jiang & Wang, 2018; Huang et al., 2021). These unique current conditions promote rich marine life. Enrichment experiments were conducted in autumn (November 2020) and spring (April 2021) to capture diverse initial ecological conditions.
2.2 Experimental design
Surface water samples (1 m depth) for enrichment experiments were collected using Niskin metal-free bottles. Chlorophyll α (Chl α), B vitamins, and microbial plankton community samples were obtained at the beginning (day 0). Portable hand-held water quality meters (HACH, USA) measured physicochemical indices such as pH, DO, temperature, and salinity. Subsequently, 300 mL of seawater was filtered through a 200 μm mesh to remove larger zooplankton and debris and added to photosynthetically active radiation (PAR) and ultraviolet radiation (UVR) transparent, sterile, and nontoxic (Whirl-Pak) bags along with nutrients or B vitamins, as shown in Table 1. The bags and nutrient concentrations were consistent with those used in previous enrichment experiments (Martínez-García et al., 2010; Joglar et al., 2020). The added amounts of B1, B2, B6, and B12 were approximated to the maximum concentrations observed in earlier studies (Sañudo-Wilhelmy et al., 2006; Sañudo-Wilhelmy et al., 2012; Heal et al., 2014). Each treatment had five replicates. Experimental bags were incubated in situ in coastal floating cages at a depth of 1 m for 5 days. Untreated groups served as control treatments (C) alongside B vitamin addition treatments, while inorganic nutrient addition was set as another control treatment (I) with inorganic nutrients and B vitamin addition treatments.
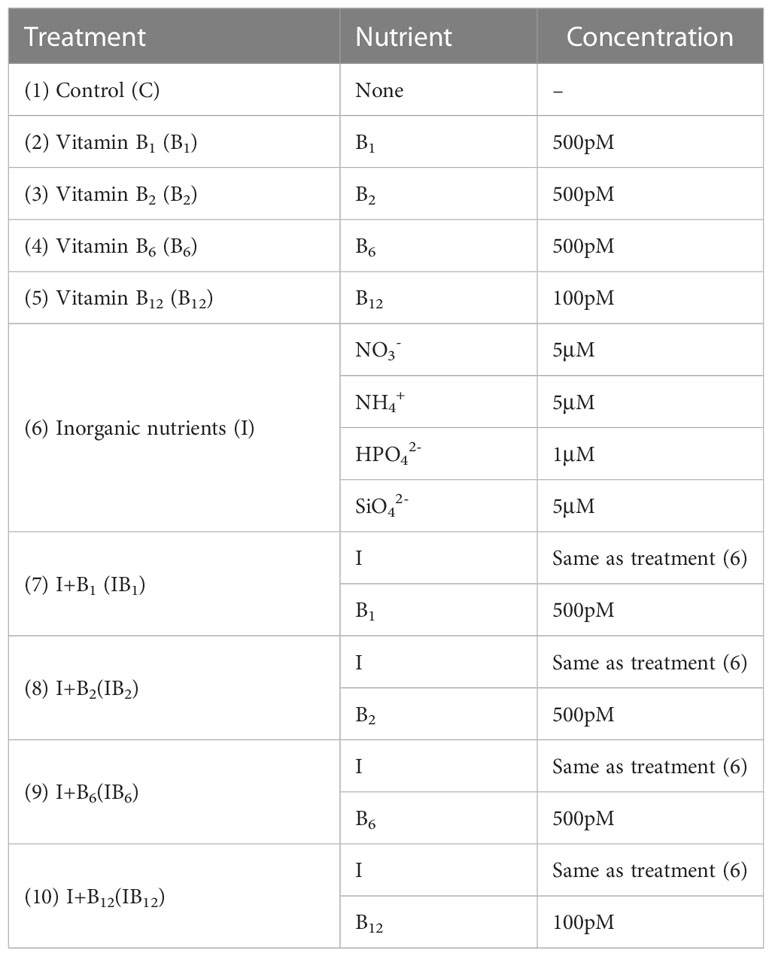
Table 1 List of amendment treatments: (1) control treatment (C), no nutrients added; (2) vitamin B1 treatment (B1), 500 pM; (3) vitamin B2 treatment (B2), 500 pM; (4) vitamin B6 treatment (B6), 500 pM; (5) vitamin B12 treatment (B12), 100 pM; (6) inorganic nutrients treatment (I), 5 μM nitrate, 5 μM ammonium, 1 μM phosphate, 5 μM silicate; (7) inorganic nutrients and vitamin B1 treatment (IB1); (8) inorganic nutrients and vitamin B2 treatment (IB2); (9) inorganic nutrients and vitamin B6 treatment (IB6); (10) inorganic nutrients and vitamin B12 treatment (IB12).
2.3 Size-fractionated chlorophyll α (Chl α)
The Chl α concentration was measured initially and after a 5-day incubation. Seawater samples (300 mL) were sequentially filtered through 3 and 0.2 μm Millipore polycarbonate (PC) filters, which were then frozen at -20 °C until analysis. Chl α extraction involved 90% acetone and 5 minutes of vortexing, followed by overnight storage in darkness at 4 °C. Finally, samples were analyzed using a spectrophotometer (General Administration of Quality Supervision et al., 2007, China).
2.4 B vitamins
2.4.1 Dissolved B vitamin preconcentration
Dissolved B vitamin samples were collected on day 0 and day 5 of the enrichment experiments, filtered through 0.2 μm Millipore polycarbonate (PC) filters, and stored at -20 °C until further analysis. Dissolved B vitamins were determined following the methods described by Sañudo-Wilhelmy et al. (Sañudo-Wilhelmy et al., 2012) and Heal et al. (Heal et al., 2014), with minor modifications. Samples (300 mL) were adjusted to pH 5.5-6.5 using 1 M HCl and preconcentrated with HLB solid-phase extraction columns (500 mg, 6 mL, Shimadzu) at a rate of 1 mL min-1 using a peristaltic pump with tygon tubing. Columns were rinsed with 20 mL of Milli-Q water to remove salts and eluted with 20 mL HPLC grade methanol. Eluents were dried under clean N2 gas without heat and reconstituted in 500 μL of Milli-Q water. Samples were stored at −20°C until UHPLC/MS/MS analysis within 24 h. Preconcentration was conducted in the dark to minimize photodegradation.
2.4.2 Particulate B vitamin extraction
Particulate B vitamin extraction followed the method described by Suffridge et al. (Suffridge et al., 2017). Frozen filters were placed in 15 mL thick-walled polypropylene centrifuge tubes containing 5 mL of lysis solution (5% methanol solution, pH adjusted to 3.5) and 2 mL of 0.5 mm zirconia beads. Samples were vortexed for 5 min and placed in an ice bath for 1 min to maintain a temperature below 30°C, and this process was repeated six times. After cell lysis, samples were incubated in a dark water bath at 30 °C for 30 min to fully extract target analytes. Liquid phase extraction (LPE) removed hydrophobic components from cell lysates using chloroform (5 mL), vigorous shaking for 3 min, and centrifugation at 5000 rpm for 10 min. The aqueous phase was transferred to a new centrifuge tube, and the extraction process was repeated. Sample pH was adjusted to 6.5 after liquid extraction, filtered into a brown bottle with a 0.22 μm filter, and stored at -20 °C until UHPLC−MS/MS analysis.
2.4.3 HPLC−MS/MS analysis
B1, B2, B6, and B12 were quantitatively detected using a UHPLC−MS/MS system. Standards for B1 (thiamine hydrochloride), B2 (riboflavin), B6 (pyridoxine), and B12 (cyanocobalamin) were purchased from Sigma Aldrich. A Thermo Scientific Ultimate 3000 UHPLC system with an Agilent Zorbax Eclipse Plus C18 column (2.1×100 mm, 3.5-micron) at 30 °C separated B vitamins. A 17-min gradient flow was employed with mobile phases of acetonitrile (solvent A) and 0.1% formic acid solution (solvent B). The flow rate was set at 0.3 mL min-1, and the sample injection volume was 100 μL. A Thermo Scientific TSQ Endura triple quadrupole mass spectrometer operating in selective response monitoring (SRM) mode with positive polarity was used for mass spectrometry. The H-ESI spray voltage was set at 3000 V, with sheath gas and aux gas velocities at 35 Arb and 10 Arb, respectively. The ion transfer tube and vaporizer temperatures were maintained at 325 °C and 300°C, respectively. SRM specifications are detailed in Table 2. Average B vitamin recovery percentages after preconcentration and extraction of B-vitamin-spiked samples, as well as their limits of detection (LOD) and limits of quantitation (LOQ), are presented in Table 3.
2.5 Phytoplankton and zooplankton communities
Samples were filtered through 0.22 μm polycarbonate filters, immediately frozen in liquid nitrogen, and stored at -80 °C until analysis. Phytoplankton and zooplankton communities were assessed by sequencing the entire 18S rRNA gene (18S rDNA). DNA extraction was performed using the PowerSoil DNA Isolation Kit (MoBio Laboratories Inc., CA, USA) following the kit’s instructions. Eukaryotes were amplified using the primers “Euk-A: AACCTGGTTGATCCTGCCAGT and Euk-B: GATCCTTCTGCAGGTTCACCTAC”(Countway et al., 2005). The KOD One PCR Master Mix (TOYOBOLife Science) was used to perform 25 cycles of PCR amplification, with initial denaturation at 95°C for 5 min, followed by 25 cycles of denaturation at 95°C for 30 s, annealing at 50°C for 30 s, and extension at 72°C for 1 min, and a final step at 72°C for 7 min. Amplified regions were sequenced with the PacBio platform at Biomarker Technologies (Beijing, China). CCS sequences were derived from the original data, and chimeras were removed to obtain effective CCS. Effective CCS sequences were clustered/denoised, OTUs/ASVs (features) were classified, and species classification was obtained according to feature sequences. The coverages of the samples ranged from 0.9936 to 0.9998. Finally, the SILVA reference database was used for taxonomic assignment of 18S ASVs through the Naive Bayes classifier combination comparison method.
2.6 Statistical analysis
Response ratios (RRs) were calculated to intuitively illustrate the responses of phytoplankton and bacteria to B vitamin supplementation (Barber-Lluch et al., 2019; Joglar et al., 2020). Phytoplankton and bacterial biomass of B vitamin amendment treatments were divided by control treatment values, and when combined with inorganic nutrients and B vitamins, treatments were divided by inorganic nutrient (I) treatment values. RR values >1 indicate a positive response, RR values = 1 imply no response, and RR values <1 signify a negative response; the further RR values deviate from 1, the more pronounced the response. One-way ANOVA and t-tests were employed to test significant differences between treatments. Principal coordinates analysis (PCoA) and non-metric multidimensional scaling (NMDS) were used to display community composition differences across seasons at the OTU level. Pearson/Spearman correlation analysis and redundancy analysis (RDA) were employed to assess correlations between specific copepods and phytoplankton. Detrended correspondence analysis (DCA) for biological data was applied to determine whether to use linear or unimodal ordination methods. Correlation networks were constructed using OmicStudio tools at https://www.omicstudio.cn/tool. All statistical analyses were considered significant at the 0.05 significance level.
3 Results
3.1 Initial conditions at the experimental site
The initial environmental conditions in November and April were compared to assess changes in hydrographic and biochemical conditions during the in situ amendment experiments (Table 4). Differences in pH, temperature, dissolved oxygen, and salinity were observed between the two months, with November having slightly lower temperature salinity. Phytoplankton biomass was 1.16 times higher in April, particularly for smaller phytoplankton, which were 2.44 times higher in April. The B vitamin content in the experimental field exhibited seasonal variation, with B1 and B2 concentrations 37.08% and 80.14% lower in November than in April, while B6 and B12 concentrations were 3.03 times and 5.92 times higher in November, respectively.
3.2 Changes in phytoplankton biomass and community composition
The total chlorophyll α (Chl α) was measured to assess phytoplankton biomass (Figure 2A). After the end of the experiments, the phytoplankton biomass of all treatments increased significantly in November compared with the initial biomass (T0), and the total Chl α ranged from 12.10-29.52 μg L-1, while that in April was only 0.64-4.58 μg L-1. In 87.5% of the eight treatments supplemented with B vitamins, total Chl α showed significant positive responses compared to the control treatment (ANOVA, p < 0.05). In terms of response rate (RR) (Figure 2B), the range of positive RR of treatments in November was 1.22-2.08, while in April, the highest was as high as 7.12, and the average positive RR was 3.75. The positive response ratios in April were 1.54-8.96 times higher than those in November (Figure 2B). Only 50% of treatments comprising a mixture of B vitamins and inorganic nutrients had a slight secondary response, and there were IB1 and IB6 treatments with RRs slightly greater than 1. The response rates of IB2 and IB12 were less than 1, indicating that there was no secondary response of phytoplankton to vitamin B in these two treatments.
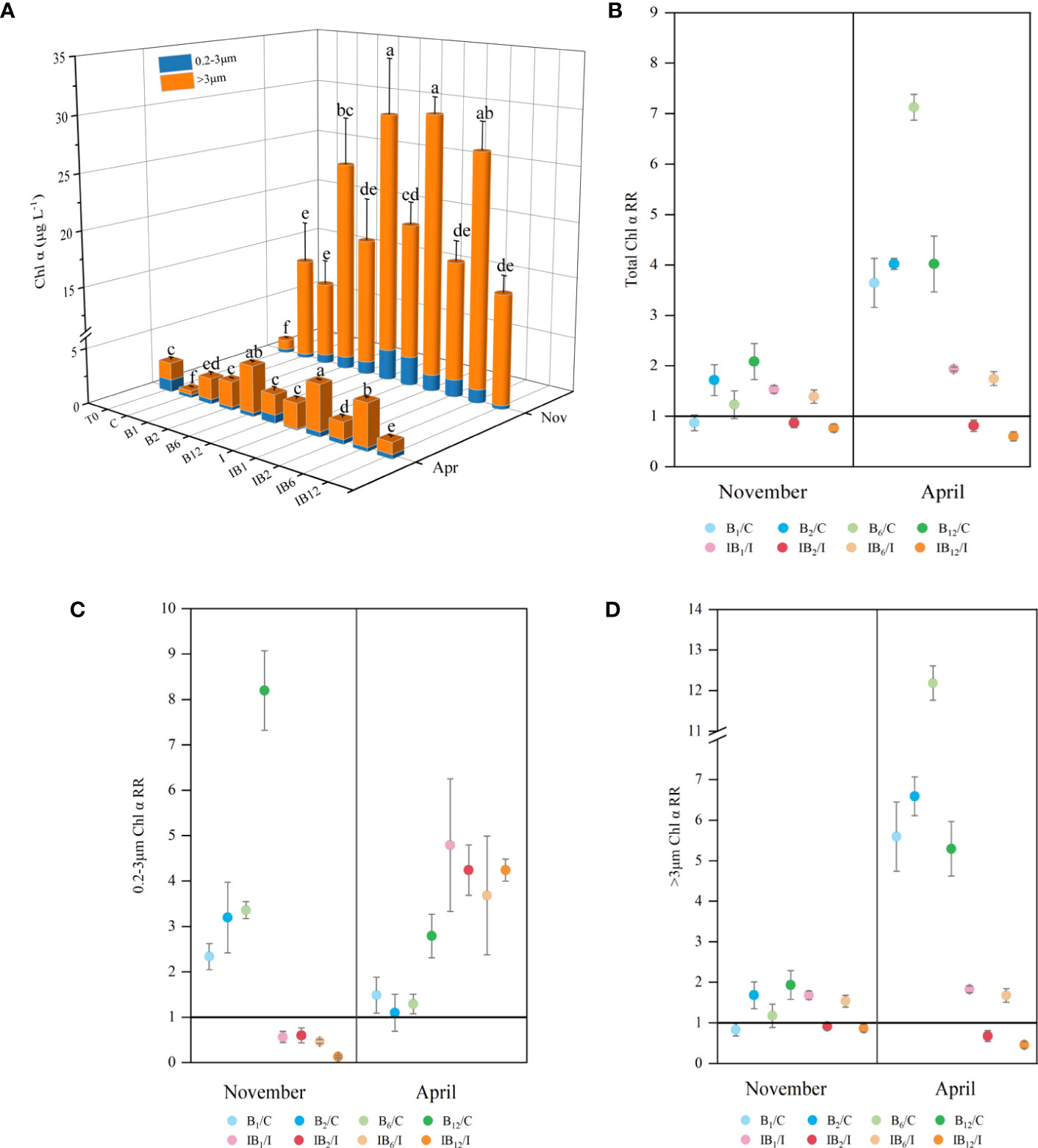
Figure 2 Chlorophyll α concentration (μg L-1) and response ratio (RR) of Chl α for each treatment. (A) Chlorophyll α concentration; (B) Response ratios of total Chl α; (C, D): Response ratios of size-fractionated Chl α. The value of RR >1 means a positive response, and the value of RR = 1 implies no response, while RR <1 shows a negative response, and the farther the RR is from 1, the more obvious the response is. Among them, the treatments of adding B vitamins alone were compared with the control group, and the treatments of adding inorganic nutrients and B vitamins were compared with the treatment of inorganic nutrients. Different alphabets above columns in (A) denote significant differences (p < 0.05) between treatments. The bars in the (B–D) indicates standard error of mean.
Analysis of different particle sizes of phytoplankton revealed that smaller phytoplankton (0.22-3 μm) exhibited strong positive responses to B vitamins in November treatments and mixed inorganic nutrients and B vitamins treatments in April, regardless of inorganic nutrient levels. The average Chl α (0.22-3 μm) RR of the treatments supplemented with B vitamins reached 4.27 in November, and that of the mixed inorganic nutrients and B vitamins treatments in April was 3.83 (Figure 2C). Conversely, larger phytoplankton (> 3 μm) showed stronger positive responses in the April than in the November experiments, with a maximum Chl α (> 3 μm) RR of 12.18 in April (Figure 2D). These findings suggest that B1, B2, B6, and B12 vitamins may ultimately limit phytoplankton growth in the marine environment.
Community composition analysis revealed the impact of vitamin B supplementation on phytoplankton communities. The initial community compositions differed between November and April, with diatoms dominating in November and Picochlorum dominating in April. In both seasons, B vitamin supplemented treatments showed increases in the relative abundance of several phytoplankton compared to the control treatments, such as Thalassiosirales, Odontella in B6 and B12 treatments, Prymnesiales, Thalassiosirales and Ditylum in B1 treatment, Minutocellus in B2 treatment in November (Figure 3A), and then Picochlorum in both of the B vitamin supplemented treatments in April (Figure 3B). Principal coordinate analysis (PCoA) and nonmetric multidimensional scaling (NMDS) based on Bray−Curtis distance at the endpoint of each experiment confirmed that the community composition of phytoplankton in each treatment was obviously different from that in the control treatment after B vitamin supplementation (Figures 4A-1, 2). The points of each B vitamin treatment in PCoA and NMDS were far from the points represented by the control treatment. These results demonstrate that B vitamins have a substantial impact on phytoplankton community composition.
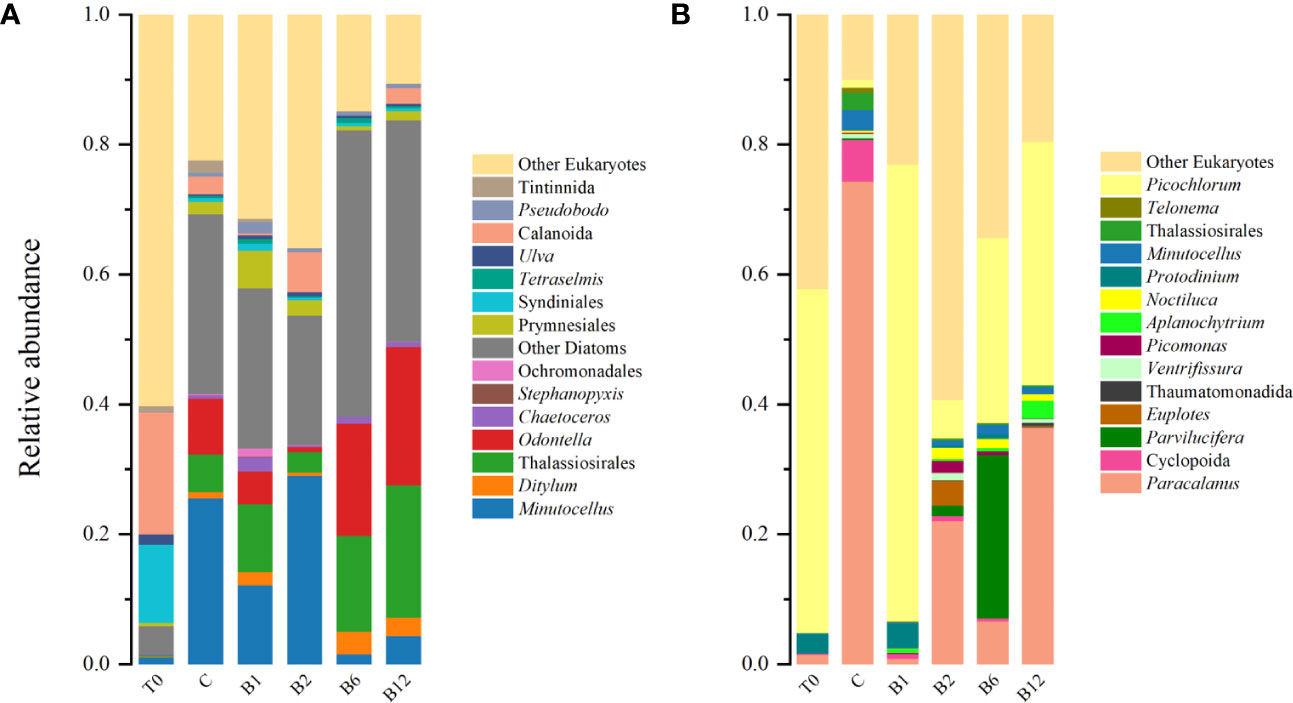
Figure 3 Relative abundance of sequence reads assigned to the major taxonomic groups of eukaryotes at the initial (T0) and endpoint of control treatment (C) and each B vitamins supplementation experiment conducted (A) in November and (B) in April.
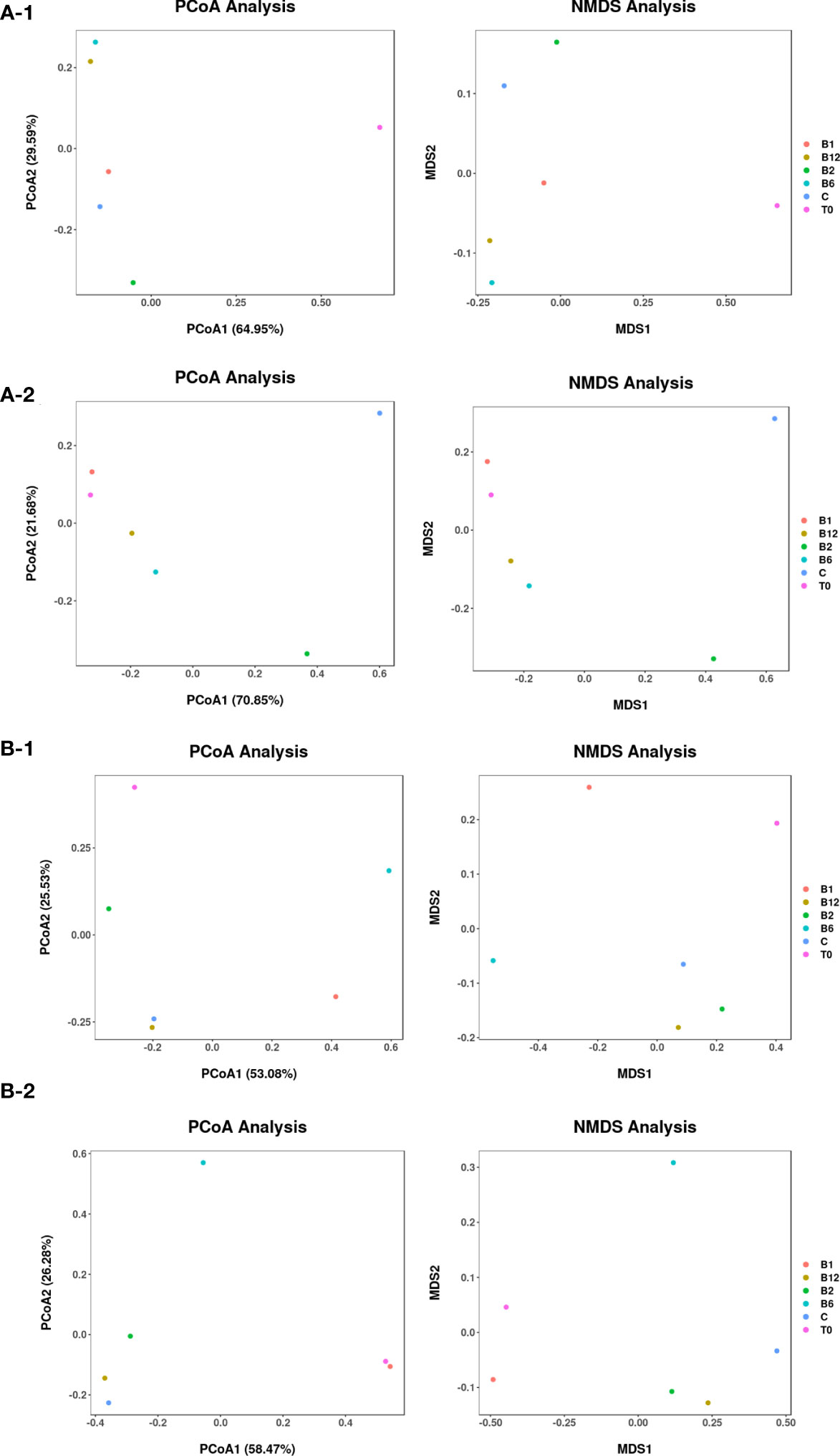
Figure 4 Principal coordinate analysis (PCoA) and nonmetric multidimensional scaling (NMDS) based on Bray−Curtis distance at the operational taxonomic unit (OTU) level of phytoplankton (A) and zooplankton (B) at the initial (T0) and endpoint of experiments, which were conducted in November (A-1, B-1) and April (A-2, B-2).
3.3 Changes in zooplankton community composition
The initial community compositions of the identified zooplankton were similar in the two experimental seasons, with Calanoida (Copepoda) predominating, which was not the case with phytoplankton. In addition, their relative abundances were at low levels, at 0.2 in November and less than 0.02 in April. However, compared with the C treatment, the relative abundance of identified zooplankton in 87.5% of the treatments supplemented with B vitamins had negative responses (Figure 3). Calanoida showed a decrease in the B1 and B6 treatments, which decreased to almost undetectable levels in November. The decline also occurred in April, when the relative abundance of Cyclopoida and Paracalanus sharply decreased in all B vitamin treatments, and the maximum reduction was in the B1 treatment, decreasing to less than 0.02, while that of the C treatment was greater than 0.8.
We also used PCoA and NMDS analysis to show the differences in identified zooplankton communities between experiments (Figures 4B-1, 2). The first principal coordinate explained 53.08% and 58.47% of the total variation in November and April, respectively, and the second principal coordinate explained 25.53% and 26.28% of the total variation in November and April, respectively. Both stress values of the NMDS analysis were less than 0.01. Accordingly, significant differences between B vitamin treatments and C treatments were observed. Besides, we used RDA analysis to show the relationship between specific phytoplankton and zooplankton as the first axis lengths of gradient in the DCA analysis is less than 3.0. The RDA results showed that different patterns of response in phytoplankton and zooplankton to B-vitamin amendments appeared to be mostly explained by the certain phytoplankton and zooplankton (Figure 5). In details, Calanoida dominated the plankton community changes in the vitamin B2 treatments, while Thalassiosirales, Ditylum, Odontella, Chaetoceros dominated the plankton community changes in the vitamin B1, B6, and B12 treatments in November. The changes of plankton community composition in the B vitamins treatments in April were mostly dominated by Paracalanus, Protodinium and Picochlorum. At the same time different correlations were observed between different species of zooplankton and phytoplankton, most phytoplankton species showed negative correlation with zooplankton, such as Calanoida with Thalassiosirales, Ditylum, Odontella, Prymnesiales and Chaetoceros in November (Figure 5A), Paracalanus with Protodinium and Picochlorum in April (Figure 5B).
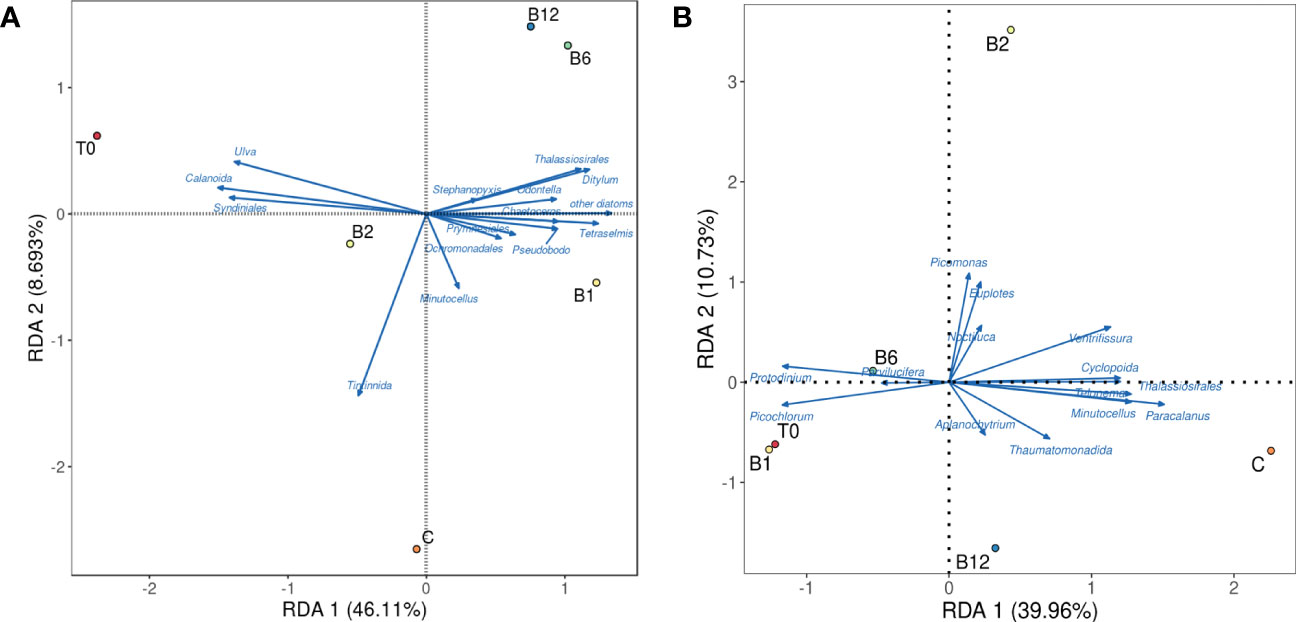
Figure 5 Redundancy analysis (RDA) of B vitamin responses by specific species phytoplankton and zooplankton. Filled symbols represent B vitamins supplementation samples in (A) November and (B) April.
4 Discussion
4.1 B vitamin auxotrophy may dominate the changes in phytoplankton communities
Our study highlights the importance of B vitamins, including B2 and B6, in addition to B1 and B12, in stimulating phytoplankton growth. These findings challenge the conventional belief that only B1 and B12 are significant to phytoplankton (Barber-Lluch et al., 2019; Joglar et al., 2020). Additionally, our results demonstrate distinct response patterns among phytoplankton of different sizes in relation to B vitamins. For example, since the inorganic nutrient content was higher in spring and lowest in autumn in the experimental area, the probability of nitrogen restriction in autumn is higher than that in spring (Ke et al., 2019; Chen et al., 2020). Therefore, smaller phytoplankton (0.22-3 μm) exhibited strong positive responses to B vitamins in the autumn when inorganic nutrition was relatively limited, suggesting that they may have an advantage in the absorption and assimilation of B1, B2, B6, and B12 under nutrient-limited conditions (Koch et al., 2012; Fridolfsson et al., 2019). In contrast, larger phytoplankton (> 3 μm) were mainly limited by inorganic nutrients under nutrient-limited conditions, exhibiting less pronounced responses to B vitamin supplementation. However, when inorganic nutrients were abundant, larger phytoplankton outcompeted smaller species, and vitamin B limitation emerged as the primary constraint (Gobler, 2007). The observed limitation of single B vitamins in spring implies that organic micronutrient reserves may be insufficient during more productive seasons (Barber-Lluch et al., 2019).
Previous studies have found that vitamin B1 (Barber-Lluch et al., 2019; Joglar et al., 2020) and B12 (Sañudo-Wilhelmy et al., 2006; Koch et al., 2011; Barber-Lluch et al., 2019) supplementation increased phytoplankton biomass. There is widespread auxotrophy for B vitamins among phytoplankton (Giovannoni, 2012; Sañudo-Wilhelmy et al., 2014). In fact, most phytoplankton (80%) are auxotrophic for B1 (Croft et al., 2006; Paerl et al., 2015), and 50% of sequenced phytoplankton, including most diatoms, possess a pathway for B1 synthesis (Sañudo-Wilhelmy et al., 2014). Our study observed an increased relative abundance of diatom species (Thalassiosirales, Chaetoceros, and Ditylum) and Prymnesiales following B1 supplementation. However, eukaryotic phytoplankton are widely believed to be incapable of de novo B12 synthesis (Bertrand and Allen, 2012; Helliwell, 2017), and more than half of marine phytoplankton require B12, indicating widespread B12 auxotrophy in the ocean (Tang et al., 2010). Chaetoceros, Ditylum, and Thalassiosirales have also been reported as B12 auxotrophs (Croft et al., 2006), which accounts for the increase in the relative abundance of these species following B12 supplementation observed in this study and another (Koch et al., 2011).
Limited information exists about B2 and B6 auxotrophy, but our study demonstrated changes in phytoplankton community composition following their supplementation. We hypothesize that Chaetoceros, Ditylum, and Thalassiosirales may also be B6 auxotrophs, given the similarity of their relative abundance trends in the B1 and B12 treatments. In contrast, the relative abundance of Minutocellus decreased in most treatments compared to the control, which suggests that they might not have significant B vitamin dependence. The increase in Picochlorum abundance in April suggests an obvious stimulation by B vitamins, particularly B1, B6, and B12. Picochlorum, a member of the Chlorophyta phylum, is a group of small, fast-growing nanoplanktonic algae known for high oil-producing potential and applications in wastewater remediation, biomass production, and aquaculture feedstock (Henley et al., 2004; De la Vega et al., 2011; Chen et al., 2012b; Zhu and Dunford, 2013; Wang et al., 2016; Watanabe and Fujii, 2016; Kumar et al., 2017). However, the B vitamin auxotrophy of Picochlorum remains unclear. Our findings highlight the need for further research on the relationship of Picochlorum with B vitamins to better understand their ecological role and potential applications.
4.2 Phytoplankton effects on Copepoda and potential underlying mechanisms
Our study identified a decrease in the relative abundance of Copepoda, including Calanoida, Paracalanus, and Cyclopoida, following B vitamin amendments. A network diagram based on Spearman’s rank correlation analysis (Figure 6) and redundancy analysis (RDA) (Figure 5) revealed negative correlations between Copepoda and several phytoplankton species, such as Thalassiosirales, Chaetoceros, Ditylum, Picochlorum, and Protodinium.
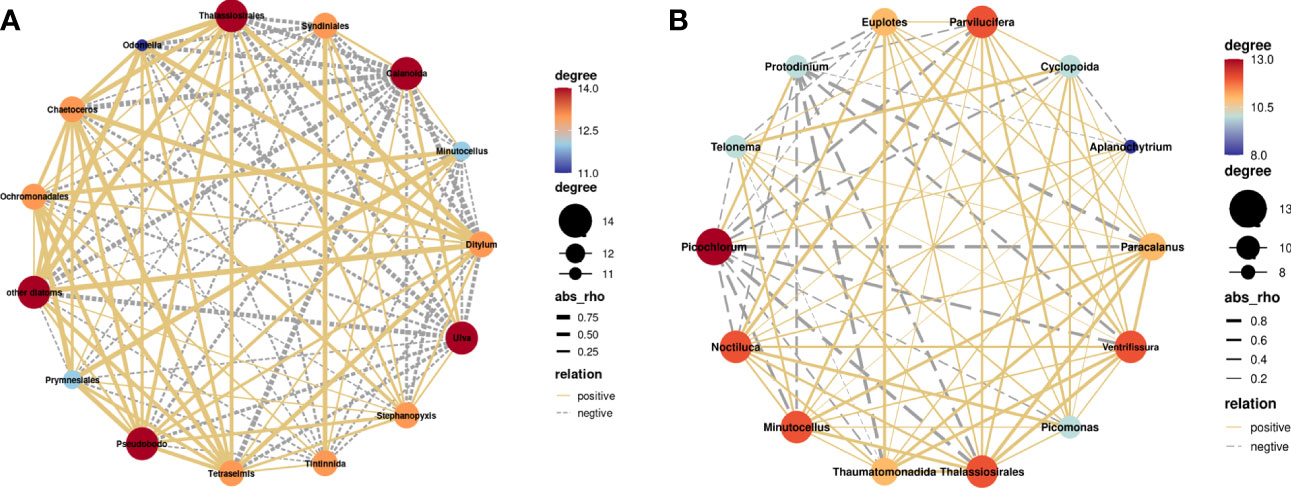
Figure 6 Correlation networks between the top 15 taxonomic groups of phytoplankton or zooplankton with relative abundance at the endpoint of each B vitamin addition experiment conducted (A) in November and (B) in April. The solid yellow lines indicate positive relationships, and the dashed gray lines indicate negative relationships. The thicker the line is, the larger the value of Rho.
The addition of B vitamins stimulated the abundance of specific diatoms (Odontella, Thalassiosirales, Ditylum, and Chaetoceros), which may be auxotrophic for B vitamins. These diatoms displayed significant negative correlations with Copepoda, with Spearman Rho values greater than 0.8 (p < 0.05 or 0.01) (Figure 7). Copepoda were known to feed on phytoplankton. Fridolfsson et al. (2018) found in their study on marine vitamin B1 that the presence of filamentous cyanobacteria could negatively affect copepods reproduction through phytoplankton and copepods vitamin B1 content. Previous research has demonstrated that high concentrations of certain diatoms can inhibit Copepoda reproduction (Carotenuto et al., 2002; Miralto et al., 2003; Pierson et al., 2005), with two prevailing hypotheses: the “nutritional deficiency hypothesis”, suggesting that diatom nutrient composition is insufficient for Copepoda growth and reproduction (Irigoien et al., 2002), and the “toxicity hypothesis”, proposing that diatoms produce toxic secondary metabolites that directly affect Copepoda (Ianora et al., 2003). Thalassiosirales have been shown to produce mitogenic aldehydes at peak levels, which then affect copepods hatching and survival (Halsband-Lenk et al., 2005; Ask et al., 2006). At the same time, laboratory studies have reported negative effects of specific diatoms on Copepoda spawning and/or hatching (Ceballos and Ianora, 2003; Poulet et al., 2007; Dutz et al., 2008). Based on the above discussion, we believed that vitamin B induced changes in phytoplankton community composition may negatively affect Copepoda through food inhibition.
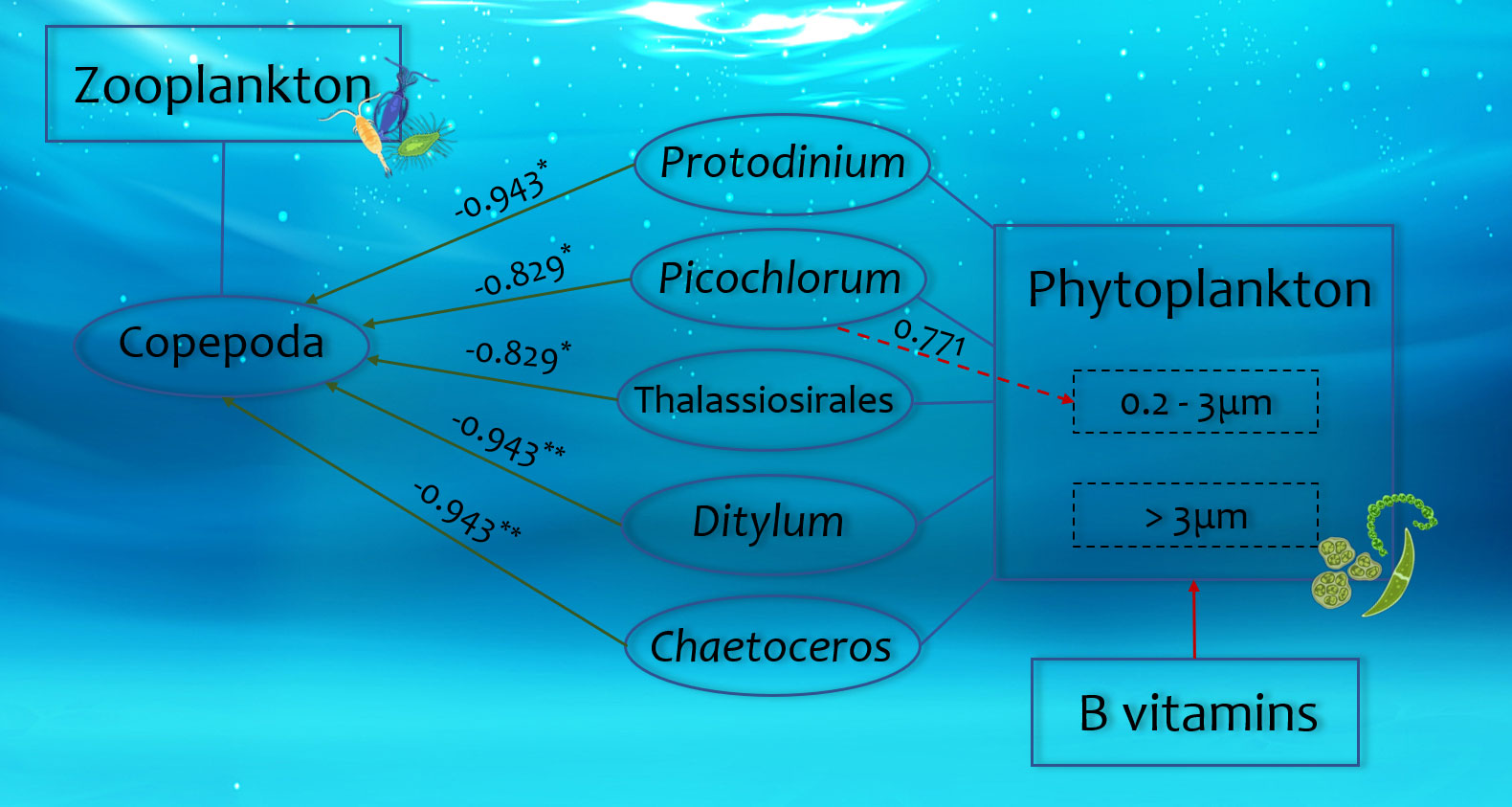
Figure 7 The correlation of certain species of phytoplankton and zooplankton after B vitamin amendments. Red arrows represent positive correlations, and green arrows represent negative correlations. The numbers represent the values of the correlation coefficients (Rho), “*” symbols represent p < 0.05 and “**” symbols represent p < 0.01.
In addition to some certain diatoms, Protodinium and Picochlorum also showed significant negative correlations with Copepoda, with Spearman Rho values greater than 0.8 (p < 0.05) (Figure 7). Previous studies have reported that Copepoda biomass did not increase with phytoplankton biomass in some coastal waters (Vadstein et al., 2004; Hong et al., 2013). Copepoda were known to exhibit adaptive food selection to meet their growth and reproduction requirements (Chen et al., 2012a), and their ingestion rate was linearly related to food concentration (Frost, 1972). Copepods increase their ingestion rate when food concentration is low, but when it exceeds a certain threshold, their feeding rate decreases. Copepoda typically preferred phytoplankton that were nutritious and within a suitable size range (Liu et al., 2010; Lee et al., 2012; Fridolfsson et al., 2019). Picochlorum has been reported to be too small for Copepoda to graze effectively, making top-down control of their bloom less likely (Ma et al., 2021). Thus, our study suggests that B vitamin-induced changes in phytoplankton community composition may also negatively affect Copepoda through feeding inhibition.
5 Conclusion
This study used in situ amendments to demonstrate the effects of vitamins B1, B2, B6 and B12 on the growth of phytoplankton. We found that B vitamin supplementation, particularly B1, B12, and to a lesser extent B2 and B6, led to significant shifts in phytoplankton community composition. Certain phytoplankton species, including diatoms and Prymnesiales, were found to be potentially auxotrophic for B vitamins and exhibited increased relative abundance in response to B vitamin supplementation. And the resulting changes in phytoplankton communities had a negative impact on Copepoda populations, leading to a decrease in their relative abundance. In conclusion, our findings augmented our knowledge on the effect of B vitamins other than B1 and B12 on phytoplankton and had important implications for our understanding of the dynamics of zooplankton community composition under the influence of different B vitamins.
Data availability statement
The datasets presented in this study can be found in online repositories. The names of the repository/repositories and accession number(s) can be found below: BioProject, PRJNA956500.
Author contributions
LW: Methodology, Formal analysis, Investigation, Writing – original draft. HZ: Investigation, Formal analysis, Writing – review & editing. ES: Formal analysis, Writing – review & editing. BL: Methodology, Formal analysis, Resources. XC: Methodology, Formal analysis, Resources. QM: Investigation, Writing – review & editing. JL: Methodology, Writing – review & editing. WL: Conceptualization, Funding acquisition, Project administration, Writing – review & editing. All authors contributed to the article and approved the submitted version.
Funding
This study was supported by the National Natural Science Foundation of China (42230413) and the Key Special Project for Introduced Talents Team of Southern Marine Science and Engineering Guangdong Laboratory (Grant No. GML2019ZD0606).
Conflict of interest
The authors declare that the research was conducted in the absence of any commercial or financial relationships that could be construed as a potential conflict of interest.
Publisher’s note
All claims expressed in this article are solely those of the authors and do not necessarily represent those of their affiliated organizations, or those of the publisher, the editors and the reviewers. Any product that may be evaluated in this article, or claim that may be made by its manufacturer, is not guaranteed or endorsed by the publisher.
References
Abo-Taleb H. A. (2019). Key to the Red Sea Labidocera (Crustacea; Calanoida: Pontellidae) copepods, the distribution of the species in various habitats, with special reference to two new records, and a historical correction. Egyptian J. Aquat. Res. 45, 367–374. doi: 10.1016/j.ejar.2019.11.004
Ask J., Reinikainen M., Bamstedt U. (2006). Variation in hatching success and egg production of Eurytemora affinis (Calanoida, Copepoda) from the Gulf of Bothnia, Baltic Sea, in relation to abundance and clonal differences of diatoms. J. Plankton Res. 28 (7), 683–694. doi: 10.1093/plankt/fbl005
General Administration of Quality Supervision, Inspection and Quarantine of the People's Republic of China & Standardization Administration of the People's Republic of China. (2007). Sepcifications for oceanographic survey – Part 6: Marine biological survey (Beijing, China: Standards Press of China). GB/T12763.6-2007.
Barber-Lluch E., Hernandez-Ruiz M., Prieto A., Fernandez E., Teira E. (2019). Role of vitamin B12 in the microbial plankton response to nutrient enrichment. Mar. Ecol. Prog. Ser. 626, 29–42. doi: 10.3354/meps13077
Bertrand E. M., Allen A. E. (2012). Influence of vitamin B auxotrophy on nitrogen metabolism in eukaryotic phytoplankton. Front. Microbiol. 3, 375. doi: 10.3389/fmicb.2012.00375
Bertrand E. M., Saito M. A., Lee P. A., Dunbar R. B., Sedwick P. N., Ditullio G. R. (2011). Iron limitation of a springtime bacterial and phytoplankton community in the ross sea: implications for vitamin B12 nutrition. Front. Microbiol. 2, 160. doi: 10.3389/fmicb.2011.00160
Bils F., Aberle N., van Damme C. J. G., Peck M. A., Moyano M. (2022). Role of protozooplankton in the diet of North Sea autumn spawning herring (Clupea harengus) larvae. Mar. Biol. 169, 90. doi: 10.1007/s00227-022-04076-1
Cai S. Z., Wu R. S., Xu J. D. (2011). Characteristics of upwelling in eastern Guangdong and southern Fujian coastal waters during 2006 summer. Jouranl Oceanography Taiwan Strait 30, 4. doi: 10.3969/J.ISNN.1000-8160.2011.04.006
Carotenuto Y., Ianora A., Buttino I., ROmano G., Miralto A. (2002). Is postembryonic development in the copepod Temora stylifera negatively affected by diatom diets? J. Exp. Mar. Biol. Ecol. 276, 49–66. doi: 10.1016/S0022-0981(02)00237-X
Ceballos S., Ianora A. (2003). Different diatoms induce contrasting effects on the reproductive success of the copepod Temora stylifera. J. Exp. Mar. Biol. Ecol. 294, 189–202. doi: 10.1016/S0022-0981(03)00263-6
Chen D., Ke Z., Tan Y., Liu J. (2020). Seasonal distribution of nutrients concentrations and the potential limitation for phytoplankton growth in the coastal region of Nan'ao-Dongshan. Ecol. Sci. 39 (4), 41–50. doi: 10.14108/j.cnki.1008-8873.2020.04.006
Chen T. Y., Lin H. Y., Lin C. C., Lu C. K., Chen Y. M. (2012b). Picochlorum as an alternative to Nannochloropsis for grouper larval rearing. Aquaculture 338, 82–88. doi: 10.1016/j.aquaculture.2012.01.011
Chen M. R., Liu H. B., Chen B. Z. (2012a). Effects of dietary essential fatty acids on reproduction rates of a subtropical calanoid copepod, Acartia erythraea. Mar. Ecol. Prog. Ser. 455, 95–110. doi: 10.3354/meps09685
Chiba S., Batten S., Martin C. S., Ivory S., Miloslavich P., Weatherdon L. V. (2018). Zooplankton monitoring to contribute toward addressing global biodiversity conservation challenges. Jouranl Plankton Res. 40, 509–518. doi: 10.1093/plankt/fby030
Countway P. D., Gast R. J., Savai P., Caron D. A. (2005). Protistan diversity estimates based on 18S rDNA from seawater inCubations in the Western North Atlantic. J. Eukaryotic Microbiol. 52 (2), 95–106. doi: 10.1111/j.1550-7408.2005.05202006.x
Croft M. T., Lawrence A. D., Raux-Deery E., Warren M. J., Smith A. G. (2005). Algae acquire vitamin B12 through a symbiotic relationship with bacteria. Nature 438, 90–93. doi: 10.1038/nature04056
Croft M. T., Warren M. J., Smith A. G. (2006). Algae need their vitamins. Eukaryotic Cell 5, 1175–1183. doi: 10.1128/EC.00097-06
De la Vega M., Diaz E., Vila M., Leon R. (2011). Isolation of a new strain of Picochlorum sp and characterization of its potential biotechnological applications. Biotechnol. Prog. 27, 1535–1543. doi: 10.1002/btpr.686
Dowling D. P., Croft A. K., Drennan C. L. (2012). Radical use of Rossmann and TIM barrel architectures for controlling coenzyme B12 chemistry. Annu. Rev. Biophysics 41, 403–427. doi: 10.1146/annurev-biophys-050511-102225
Dutz J., Koski M., Jonasdottir S. H. (2008). Copepod reproduction is unaffected by diatom aldehydes or lipid composition. Limnology Oceanography 53, 225–235. doi: 10.4319/lo.2008.53.1.0225
Frank R. A. W., Leeper F. J., Luisi B. F. (2007). Structure, mechanism and catalytic duality of thiamine-dependent enzymes. Cell. Mol. Life Sci. 64, 892–905. doi: 10.1007/s00018-007-6423-5
Fridolfsson E., Lindehoff E., Legrand C., Hylander S. (2020). Species-specific content of thiamin (vitamin B1) in phytoplankton and the transfer to copepods. J. Plankton Res. 42, 274–285.
Fridolfsson E., Bunse C., Legrand C., Lindehoff E., Majaneva S., Hylander S. (2019). Seasonal variation and species-specific concentrations of the essential vitamin B1 (thiamin) in zooplankton and seston. Mar. Biol. 166, 70. doi: 10.1007/s00227-019-3520-6
Fridolfsson E., Lindehoff E., Legrand C., Hylander S. (2018). Thiamin (vitamin B1) content in phytoplankton and zooplankton in the presence of filamentous cyanobacteria. Limnology Oceanography 63, 2423–2435. doi: 10.1002/lno.10949
Frost B. W. (1972). Effects of size and concentration of food particles on the feeding behavior of the marine plankton copepod Calanus pacificus. Limnology Oceanography 17, 805–815. doi: 10.4319/lo.1972.17.6.0805
Giovannoni S. J. (2012). Vitamins in the sea. Proceedings of the National Academy of Sciences. 109, 13888–13889.
Gobler C. J. (2007). Effect of B-vitamins (B1, B12) and inorganic nutrients on algal bloom dynamics in a coastal ecosystem. Aquat. Microbial Ecol. 49, 181–194. doi: 10.3354/ame01132
Halsband-Lenk C., Pierson J. J., Leising A. W. (2005). Reproduction of Pseudocalanus newmani (Copepoda : Calanoida) is deleteriously affected by diatom blooms - A field study. Prog. Oceanography 67 (3-4), 332–348. doi: 10.1016/j.pocean.2005.09.003
Heal K. R., Carlson L. T., Devol A. H., Armbrust E. V., Moffett J. W., Stahl D. A., et al. (2014). Determination of four forms of vitamin B12 and other B vitamins in seawater by liquid chromatography/tandem mass spectrometry. Rapid Communcation Mass Spectrometry 28, 2398–2404. doi: 10.1002/rcm.7040
Heal K. R., Qin W., Ribalet F., Bertagnolli A. D., Coyote-Maestas W., Hmelo L. R., et al. (2017). Two distinct pools of B12 analogs reveal community interdependencies in the ocean. Proceedings of the National Academy of Sciences 114, 364–369.
Helliwell K. E. (2017). The roles of B vitamins in phytoplankton nutrition: New perspectives and prospects. New Phytol. 216, 62–68. doi: 10.1111/nph.14669
Henley W. J., Hironaka J. L., Guillou L., Buchheim M. A., Buchheim J. A., Fawley M. W., et al. (2004). Phylogenetic analysis of the 'Nannochloris-like' algae and diagnoses of Picochlorum oklahomensis gen. et sp nov (Trebouxiophyceae, Chlorophyta). Phycologia 43, 641–652. doi: 10.2216/i0031-8884-43-6-641.1
Hong Y., Burford M. A., Ralph P. J., Udy J. W., Doblin M. A. (2013). The cyanobacterium Cylindrospermopsis raciborskii is facilitated by copepod selective grazing. Harmful Algae 29, 14–21. doi: 10.1016/j.hal.2013.07.003
Huang Z., Hu J., Shi W. (2021). Mapping the coastal upwelling East of Taiwan using geostationary satellite data. Remote Sens 13, 170. doi: 10.3390/rs13020170
Ianora A., Poulet S. A., Miralto A. (2003). The effects of diatoms on copepod reproduction: a review. Phycologia 42, 351–363. doi: 10.2216/i0031-8884-42-4-351.1
Irigoien X., Harris R. P., Verheye H. M., Joly P., Runge J., Starr M., et al. (2002). Copepod hatching success in marine ecosystems with high diatom concentrations. Nature 419, 384–389. doi: 10.1038/nature01055
Jiang R., Wang Y. S. (2018). Modeling the ecosystem response to summer coastal upwelling in the northern South China Sea. Oceanologia 60, 32–51. doi: 10.1016/j.oceano.2017.05.004
Joglar V., Pontiller B., Martinez S., Fuentes-Lema A., Perez-Lorenzo M., Lundin D., et al. (2021). Microbial plankton community structure and function responses to vitamin B12 and B1 amendments in an upwelling system. Appl. Environ. Microbiol. 87, e01525–e01521. doi: 10.1128/AEM.01525-21
Joglar V., Prieto A., Barber-Lluch E., Hernández-Ruiz M., Fernández E., Teira E. (2020). Spatial and temporal variability in the response of phytoplankton and prokaryotes to B-vitamin amendments in an upwelling system. Biogeosciences 17, 2807–2823. doi: 10.5194/bg-17-2807-2020
Ke Z., Chen D., Tan Y., Liu H., Liu J. (2019). Temporal and spatial variations in primary production in the coastal region of Dongshan-Nan’ao. J. Fishery Sci. China 26 (1), 44–52. doi: 10.3724/SP.J.1118.2019.18208
King A. L., Sañudo-Wilhelmy S. A., Leblanc K., Hutchins D. A., Fu F. (2011). CO2 and vitamin B12 interactions determine bioactive trace metal requirements of a subarctic Pacific diatom. ISME J. 5, 1388–1396. doi: 10.1038/ismej.2010.211
Koch F., Hattenrath-Lehmann T. K., Goleski J. A., Sañudo-Wilhelmy S. A., Fisher N. S., Gobler C. J. (2012). Vitamin B1 and B12 uptake and cycling by plankton communities in coastal ecosystems. Front. Microbiol. 3, 363. doi: 10.3389/fmicb.2012.00363
Koch F., Marcoval M. A., Panzeca C., Bruland K. W., Sañudo-Wilhelmy S. A., Gobler C. J. (2011). The effect of vitamin B12 on phytoplankton growth and community structure in the Gulf of Alaska. Limnology Oceanography 56, 1023–1034. doi: 10.4319/lo.2011.56.3.1023
Kumar S. D., Santhanam P., Ananth S., Kaviyarasan M., Nithya P., Dhanalakshmi B., et al. (2017). Evaluation of suitability of wastewater-grown microalgae (Picochlorum maculatum) and copepod (Oithona rigida) as live feed for white leg shrimp Litopenaeus vannamei postlarvae. Aquaculture Int. 25, 393–411. doi: 10.1007/s10499-016-0037-6
Lee D. B., Song H. Y., Park C., Choi K. H. (2012). Copepod feeding in a coastal area of active tidal mixing: diel and monthly variations of grazing impacts on phytoplankton biomass. Mar. Ecol. 33, 88–105. doi: 10.1111/j.1439-0485.2011.00453.x
Liu H. B., Chen M. R., Suzuki K., Wong C. K., Chen B. Z. (2010). Mesozooplankton selective feeding in subtropical coastal waters as revealed by HPLC pigment analysis. Mar. Ecol. Prog. Ser. 407, 111–123. doi: 10.3354/meps08550
Ma X., Jacoby C. A., Johnson K. B. (2021). Grazing by the copepod Parvocalanus crassirostris on Picochlorum sp. at harmful bloom densities and the role of particle size. Front. Mar. Sci. 8, 1–8. doi: 10.3389/fmars.2021.664154
Majaneva S., Fridolfsson E., Casini M., Legrand C., Lindehoff E., Margonski P., et al (2020). Deficiency syndromes in top predators associated with large-scale changes in the Baltic Sea ecosystem. PLoS One 15, e0227714.
Martínez-García S., Fernandez E., Calvo-Diaz A., Maranon E., Moran X. A. G., Teira E. (2010). Response of heterotrophic and autotrophic microbial plankton to inorganic and organic inputs along a latitudinal transect in the Atlantic Ocean. Biogeosciences 7, 1701–1713. doi: 10.5194/bg-7-1701-2010
Matthews R. G., Smith A. E., Zhou Z. H. S., Taurog R. E., Bandarian V., Evans J. C., et al. (2003). Cobalamin-dependent and cobalamin-independent methionine synthases: Are there two solutions to the same chemical problem? Helv. Chimica Acta 86, 3939–3954. doi: 10.1002/hlca.200390329
Miralto A., Guglielmo L., Zagami G., Buttino I., Granata A., Ianora A. (2003). Inhibition of population growth in the copepods Acartia clausi and Calanus helgolandicus during diatom blooms. Mar. Ecol. Prog. Ser. 254, 253–268. doi: 10.3354/meps254253
Monteverde D. R., Gomez-Consarnau L., Suffridge C., Sañudo-Wilhelmy S. A. (2017). Life's utilization of B vitamins on early Earth. Geobiology 15, 3–18. doi: 10.1111/gbi.12202
Nichols D. S. (2003). Prokaryotes and the input of polyunsaturated fatty acids to the marine food web. FEMS Microbiol. Lett. 219, 1–7. doi: 10.1016/S0378-1097(02)01200-4
Paerl R. W., Bertrand E. M., Allen A. E., Palenik B., Azam F. (2015). Vitamin B1 ecophysiology of marine picoeukaryotic algae: Strain-specific differences and a new role for bacteria in vitamin cycling. Limnology Oceanography 60, 215–228. doi: 10.1002/lno.10009
Pierson J. J., Halsband-Lenk C., Leising A. W. (2005). Reproductive success of Calanus pacificus during diatom blooms in Dabob Bay, Washington. Prog. Oceanography 67, 314–331. doi: 10.1016/j.pocean.2005.09.002
Pomeroy L. R., Williams P. J. I., Azam F., Hobbie J. E. (2007). The microbial loop. Oceanography 20, 28–33. doi: 10.5670/oceanog.2007.45
Poulet S. A., Escribano R., Hidalgo P., Cueff A., Wichard T., Aguilera V., et al. (2007). Collapse of Calanus Chilensis reproduction in a marine environment with high diatom concentration. J. Exp. Mar. Biol. Ecol. 352, 187–199. doi: 10.1016/j.jembe.2007.07.019
Randall J. R., Murphy H. M., Robert D., Geoffroy M. (2022). Forage fish as a predator: summer and autumn diet of Atlantic herring in Trinity Bay, Newfoundland. Fisheries Res. 252, 106331. doi: 10.1016/j.fishres.2022.106331
Rodionov D. A., Vitreschak A. G., Mironov A. A., Gelfand M. S. (2002). Comparative genomics of thiamin biosynthesis in procaryotes: new genes and regulatory mechanisms. J. Biol. Chem. 277 (50), 48949–48959. doi: 10.1074/jbc.M208965200
Sañudo-Wilhelmy S. A., Cutter L. S., Durazo R., Smail E. A., Gomez-Consarnau L., Webb E. A., et al. (2012). Multiple B-vitamin depletion in large areas of the coastal ocean. Proceedings of the National Academy of Sciences 109, 14041–14045.
Sañudo-Wilhelmy S. A., G´omez-Consarnau L., Suffridge C., Webb E. A. (2014). The role of B vitamins in marine biogeochemistry. Annu. Reviwe Mar. Sci. 6, 339–367. doi: 10.1146/annurev-marine-120710-100912
Sañudo-Wilhelmy S. A., Gobler C. J., Okbamichael M., Taylor G. T. (2006). Regulation of phytoplankton dynamics by vitamin B-12. Geophysical Res. Lett. 33, L04604. doi: 10.1029/2005GL025046
Shu Y. Q., Wang Q., Zu T. T. (2018). Progress on shelf and slope circulation in the northern South China Sea. Sci. China Earth Sci. 48, 276–287. doi: 10.1007/s11430-017-9152-y
Srichandan S., Baliarsingh S. K., Lotliker A. A., Sahu B. K., Roy R., Nair T. M. B. (2021). Unraveling tidal effect on zooplankton community structure in a tropical estuary. Environ. Monit. Assess. 193, 362. doi: 10.1007/s10661-021-09112-z
Suffridge C., Cutter L., Sañudo-Wilhelmy S. A. (2017). A new analytical method for direct measurement of particulate and dissolved B-vitamins and their congeners in seawater. Front. Mar. Sci. 4, 1–11. doi: 10.3389/fmars.2017.00011
Tang Y. Z., Koch F., Gobler C. J. (2010). Most harmful algal bloom species are vitamin B1 and B12 auxotrophs. Proceedings of the National Academy of Sciences 107, 20756–20761.
Vadstein O., Stibor H., Lippert B., Loseth K., Roederer W., Sundt-Hansen L., et al. (2004). Moderate increase in the biomass of omnivorous copepods may ease grazing control of planktonic algae. Mar. Ecol. Prog. Ser. 270, 199–207. doi: 10.3354/meps270199
Waldrop G. L., Holden H. M., St Maurice M. (2012). The enzymes of biotin dependent CO2 metabolism: What structures reveal about their reaction mechanisms. Protein Sci. 21, 1597–1619. doi: 10.1002/pro.2156
Wang S. Y., Shi X. G., Palenik B. (2016). Characterization of Picochlorum sp use of wastewater generated from hydrothermal liquefaction as a nitrogen source. Algal Research-Biomass Biofuels Bioproducts 13, 311–317. doi: 10.1016/j.algal.2015.11.015
Watanabe F., Bito T. (2018). Vitamin B12 sources and microbial interaction. Exp. Biol. Med. 243, 148–158. doi: 10.1177/1535370217746612
Watanabe K., Fujii K. (2016). Isolation of high level CO2 preferring Picochlorum sp strains and their biotechnological potential. Algal Research-Biomass Biofuels Bioproducts 18, 135–143. doi: 10.1016/j.algal.2016.06.013
Keywords: B vitamins, phytoplankton communities, copepods, auxotrophy, plankton dynamics
Citation: Wang L, Zhao H, Sanganyado E, Liang B, Chen X, Ma Q, Lin J and Liu W (2023) B vitamins supplementation induced shifts in phytoplankton dynamics and copepod populations in a subtropical coastal area. Front. Mar. Sci. 10:1206332. doi: 10.3389/fmars.2023.1206332
Received: 15 April 2023; Accepted: 07 August 2023;
Published: 23 August 2023.
Edited by:
Dilip Kumar Jha, National Institute of Ocean Technology, IndiaReviewed by:
Gopalakrishnan Thilagam, Pachaiyappa’s College for Men, IndiaGajendra Joshi, National Institute of Ocean Technology, India
Naseera Kottangodan, National Institute of Ocean Technology, India
Copyright © 2023 Wang, Zhao, Sanganyado, Liang, Chen, Ma, Lin and Liu. This is an open-access article distributed under the terms of the Creative Commons Attribution License (CC BY). The use, distribution or reproduction in other forums is permitted, provided the original author(s) and the copyright owner(s) are credited and that the original publication in this journal is cited, in accordance with accepted academic practice. No use, distribution or reproduction is permitted which does not comply with these terms.
*Correspondence: Wenhua Liu, d2hsaXVAc3R1LmVkdS5jbg==