- 1Fundación Internacional para la Naturaleza y la Sustentabilidad (FINS), Chetumal, Quintana Roo, Mexico
- 2Department of Biology, University of Vermont, Burlington, VT, United States
- 3National Marine Mammal Foundation, San Diego, CA, United States
- 4Department of Biology, State University of New York at Cortland, Cortland, NY, United States
- 5Department of Psychology, Hunter College, City University of New York, New York, NY, United States
- 6Department of Ecology, Universidade do Estado do Rio de Janeiro (UERJ), Rio de Janeiro, Rio de Janeiro, Brazil
- 7Research Group on Biology and Conservation of Amazonian Aquatic Mammals (BioMA), Universidade Federal Rural da Amazônia (UFRA), Belém, Pará, Brazil
- 8Consejo Nacional de Ciencia y Tecnología, Universidad Autónoma del Estado de Quintana Roo, Chetumal, Quintana Roo, Mexico
- 9Dolphin Discovery Group Veterinary Group, Isla Mujeres, Quintana Roo, Mexico
- 10Louisiana Universities Marine Consortium, Chauvin, LA, United States
Acoustic signals play a crucial role in communication among animals, particularly in dolphins. Signature whistles, one of their most extensively studied vocalizations, enable dolphins to convey their identity to conspecifics through individually distinct whistle contours. However, it remains unclear whether rough-toothed dolphins (Steno bredanensis) also produce signature whistles with individually identifying contours and, if so, whether they are associated with stress and poor health, such as in bottlenose dolphins. To bridge this knowledge gap, we recorded sounds emitted by a live-stranded rough-toothed dolphin during its rehabilitation in May 2017 at Isla Mujeres, Quintana Roo, Mexico. We assessed if the dolphin produced a signature whistle and whether whistle rate, inter-whistle interval, mean low and high frequencies, and blood chemistry measures, changed significantly over time. While isolated from conspecifics during rehabilitation, the dolphin generated a single, repeated, and stereotyped whistle contour that met the previously established SIGnature IDentification criteria for signature whistle emissions for bottlenose dolphins. Whistle characteristics varied over the 11 recording days: whistle rate and inter-whistle interval significantly decreased over time; the number of whistles with preceding echolocation click trains decreased over time; and mean low and high frequencies changed over recording days. We conclude that this rough-toothed dolphin possessed what resembles a signature whistle contour, and the emission of this contour underwent significant changes throughout the rehabilitation process. While our study presents evidence of a single rough-toothed dolphin producing a signature whistle, further research is necessary to determine whether this vocal behavior is prevalent across the species.
1 Introduction
Toothed whales produce a diverse range of sounds employed in prey detection, navigation, and communication with conspecifics (Caldwell et al., 1990; Janik and Slater, 1998). Many dolphin species emit narrowband, frequency-modulated tonal sounds known as whistles, which serve in maintaining contact and social communication (Caldwell et al., 1990). The structure and functions of these calls differ within and between dolphin species, influenced by factors such as group size, behavioral activity, body size, and phylogenetic relatedness (Janik and Slater, 1998; Lammers et al., 2003; Cook et al., 2004; May-Collado and Wartzok, 2008). Signature whistles, the most commonly produced type by bottlenose dolphins (Tursiops truncatus), are individually distinct in their frequency modulation pattern and convey information about individual identity (Caldwell and Caldwell, 1965; Caldwell et al., 1990). They have been found to be the predominant whistle type emitted when bottlenose dolphins are isolated (Caldwell and Caldwell, 1965), serve as contact calls during intraspecific communication (King and Janik, 2013), and underwater contact maintenance when dispersed (Janik and Slater, 1998). Notably, signature whistles have been shown to carry both identity and context-related information to conspecifics (Sayigh et al., 1999; Sayigh et al., 2007; Sayigh et al., 2023).
The role of signature whistles in dolphin social communication has been extensively studied in both wild dolphins and professionally managed bottlenose dolphin populations (Caldwell et al., 1990; Sayigh et al., 2007; Janik and Sayigh, 2013). They have also been documented in wild populations of other toothed whale species including Atlantic spotted dolphins (Stenella frontalis) (Caldwell et al., 1973), Indo-Pacific bottlenose dolphins (T. aduncus) (King and Janik, 2013; Gridley et al., 2014), Indo-Pacific humpback dolphins (Sousa chinensis) (Van Parijs and Corkeron, 2001; Cheng et al., 2017), and narwhals (Monodon monoceros) (Shapiro, 2006). However, due to the challenges of recording isolated individuals and limited access to various offshore delphinid species, few studies have investigated the presence of signature whistles in other dolphin species.
Signature whistle production has been linked to changes in behavioral states (Quick and Janik, 2008) and stress levels (Esch et al., 2009), often produced at high rates when dolphins were separated from conspecifics (Watwood et al., 2005; Esch et al., 2009). Esch et al. (2009) showed that whistle rate, number of loops, and loop durations varied in bottlenose dolphins recorded during brief capture-release events compared to undisturbed dolphins. Additionally, Caldwell and Caldwell (1965) reported that distressed dolphins produced signature whistle contours at a higher rate and intensity. Ill bottlenose dolphins, both in professional care (Ridgway, 1983) and in the wild (Kuczaj et al., 2015), produced their signature whistle at a relatively high rate. We hypothesize this high rate of whistle production could potentially help to elicit help from conspecifics by repeating calls until receiving sufficient aid (Lilly, 1963; Kuczaj et al., 2015), as for example, is observed in dolphin mother-calf reunions in some species (Herzing, 1996). Although these findings collectively suggest that whistle production characteristics can provide valuable information about stress levels and health conditions in dolphins, further studies combining physiological measures with acoustic communication are needed to enhance our understanding of this relationship.
Rough-toothed dolphins (Steno bredanensis) inhabit tropical, subtropical, and temperate deep-sea ecosystems across the Atlantic, Indian, and Pacific Oceans (Jefferson, 2009). Research on their acoustic signaling reveals that they produce tonal whistles and click sounds typical of most delphinids (Busnell and Dziedzic, 1966; Rankin et al., 2015) and live in socially complex societies (Lodi, 1992; Kuczaj and Yeater, 2007; Jefferson, 2009). Comparisons of call parameters from different populations have unveiled a diverse and intricate sound repertoire, often used to devise methods for species detection and identification through passive acoustic monitoring (e.g., Rankin et al., 2008; Lima et al., 2012; Rankin et al., 2015; Lima et al., 2016). To the best of our knowledge, signature whistle production has not yet been documented in this species, but previous studies have shown repeated whistle contours in acoustic recordings of wild rough-toothed dolphins suggestive of the existence of signature whistles (Caruso et al., 2019). Additionally, this species regularly strands in large groups (e.g., Struntz et al., 2014; Karns et al., 2019; Ewing et al., 2020) and associating the sound production of stranded and captive dolphins can provide more avenues for assessing their behavior and welfare, for example, as in other captive animals (Wemelsfelder and Mullan, 2014) including marine mammals like bottlenose dolphins (Jones et al., 2021).
In this study, we analyzed the acoustic signals of a stranded adult rough-toothed dolphin and the associated health changes during its rehabilitation before its release into the wild. This unique opportunity allowed us to examine the characteristics of its sound production during 8 days of the first 11 days of its rehabilitation, testing the following two hypotheses: 1) the repeated whistle contour produced by the rough-toothed dolphin was a signature whistle contour; and 2) over the course of a dolphin’s successful rehabilitation, whistle production rate would decrease as health condition improved. Additionally, other characteristics of whistle production and whistle features (e.g., number of loops, presence of preceding click trains, inter-whistle interval) and features of whistles were examined over time to determine their associations with dolphin whistle use and health status.
2 Methods
2.1 Study subject and location
The acoustic behavior of an adult male rough-toothed dolphin (“Paki”) was recorded following its live-stranding and rescue on 02 May 2017 at 1700 h on the eastern coast of Isla Mujeres in Quintana Roo, Mexico (21°13’31” N; 86°43’24” W). The rescue was a collaborative effort between Dolphin Discovery, la Zona Federal Marítimo Terrestre (ZOFEMAT), la Secretaría de Gestión Integral de Riesgos y Protección Civil, and la Secretaría de Ecología y Medio Ambiente of Isla Mujeres. The animal was a full-grown adult. Upon stranding, it exhibited signs of illness and fresh wounds, likely from direct contact with rocks and nearby coral reefs. A team of marine mammal specialists and veterinarians from Dolphin Discovery (Mexico) assessed the animal’s condition upon arrival as dehydrated and afflicted with chronic infections and parasites.
The dolphin was housed in a sea-pen enclosure (~100 m2) during its recovery at the Tortugranja turtle farm alongside several species of sea turtle and rays. He was fed a mixture of herring, capelin, and squid during his rehabilitation, including forced feeding for 6 days from 04 May 2017 to 11 May 2017.
2.2 Health assessments
Regular health assessments, treatments, and monitoring were conducted by members of the veterinary team until its condition improved sufficiently for release. Paki was released into the wild on 22 June 2017, equipped with a satellite radio tag to track its movements and monitor its reintroduction to the wild. Paki was treated with various medications including multivitamins from the day of his rescue (02 May 2017) during his rehabilitation to ameliorate his condition until he improved sufficiently to go without medicines.
2.3 Acoustic recordings
Recordings were made opportunistically once or twice each day on 8 days in 2017 (May 6–11, 14, and 16) using a single hydrophone during veterinary observations at the seaside pen. An Aquarian Audio H2a-XLR hydrophone (effective sensitivity: -180 dB re 1V/μPa; frequency response: 0.02–4.5 kHz ± 4 dB; useable frequency range: 0.01–100 kHz) sampled sounds at 48-kHz in 24-bit resolution to a Tascam DR-60D digital recorder in WAV format. The transducer was placed 1 m below the water’s surface (depth: ~2.5 m). All recorded signals were attributed to the focal dolphin that was not housed near any other marine mammals and was always within 10 m of the hydrophone.
2.4 Acoustic measurements
Two experienced acoustic analysts (EAR and KAC) examined the spectrograms of recordings in Raven 1.5 (Bioacoustics Research Program, 2019) and manually selected vocalizations (DFT: 512 samples; Hann window; 90% overlap; frequency resolution: 11.7 kHz; temporal resolution: 21.3 ms). Signals were boxed off with a selection tool and categorized as: echolocation clicks, burst-pulses (clicks train with a pulse rate > 300 Hz, following Lammers et al. (2003), or frequency-modulated whistles (Caldwell et al., 1990). Whistles were defined as narrowband, tonal and harmonically stacked sounds. An advanced research intern with an inter-observer reliability for identifying and counting whistles in acoustic recordings of > 80% manually identified and counted all whistles present in the acoustic recordings. Whistle rate was calculated by dividing the total number of whistles by the number of minutes recorded for each day (whistles/min). The individual repetitive elements in whistles were defined as loops (Caldwell et al., 1990; Sayigh et al., 1990). A repeated whistle contour was considered looped when separated by less than 250 ms from another contour of the same type (Esch et al., 2009). Whistles were measured by drawing a selection box in the spectrogram around the visible boundary of the fundamental contour of each loop (i.e., the harmonic with the most energy) and around all loops in the whistle (Figures 1A–C).
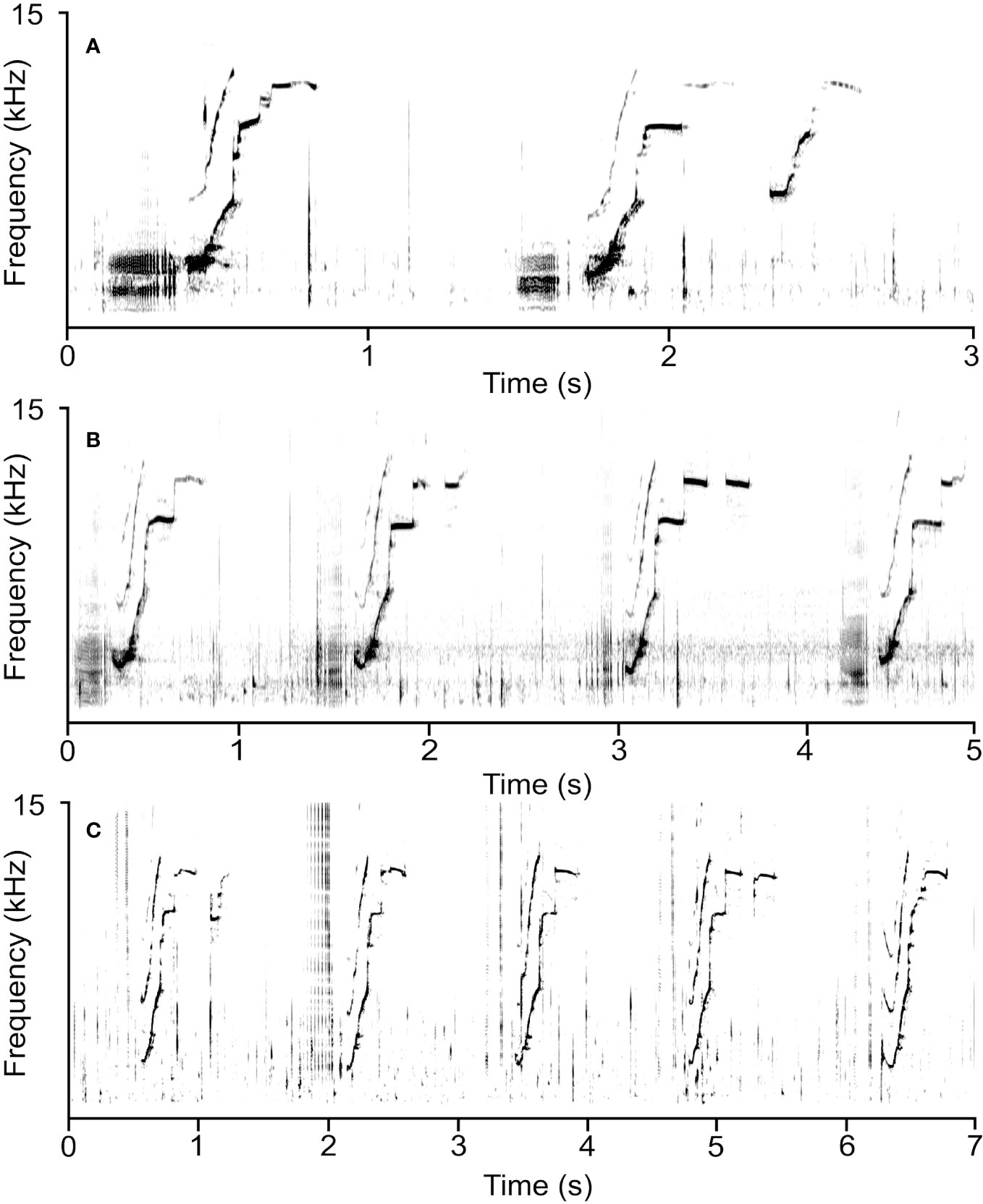
Figure 1 Spectrograms of rough-toothed dolphin acoustic signals recorded during its rehabilitation in captivity. (A) An example of the highly stereotyped rising whistle contour produced by the dolphin, its confirmed signature whistle, primarily with one loop but sometimes multi-looped. (B) Preceding click trains were present immediately prior to the production of the first loop in most whistles. (C) Whistles were typically emitted in continuous, repetitive sequences. Spectrogram parameters: 512 points; 90% overlap; Hann window; 31.3 ms frame length; 21.3 ms time step.
The dolphin produced a high volume of whistles for a minimum of an hour in each recording day, thus, we randomly selected a 10 min sample of sound recordings from each of the eight recording days with clear sequences of whistles to measure changes in the parameters of the dolphin’s individual acoustic signals and characteristics over time. Only signals with a clear and strongly defined fundamental contour were further analyzed. The following parameters were measured for each whistle: low frequency (i.e., the lowest frequency with energy in Hz), high frequency (i.e., the highest frequency with energy in Hz), peak frequency (i.e., the frequency with the most energy in Hz), and delta frequency (i.e., the difference between high and low frequency in Hz). Whistle production characteristics were measured for each whistle including: the number of loops per whistle; inter-whistle interval (IWI: the end time of one whistle subtracted from the begin time of the following next whistle); the presence/absence of a preceding echolocation click train (PCT) (Figure 1A) in the 100 ms prior to whistle onset; and the time from the end of the PCT to the beginning of the whistle. These final two parameters were selected to distinguish echolocation click trains not clearly temporally associated with whistles.
2.5 Signature whistle analysis
To explore the hypothesis that the repeated whistle type produced by the rough-toothed dolphin represented its signature whistle, two definitions were used to classify signature whistles. First, the whistle type was the most common type produced by a single individual in isolation (Caldwell et al., 1990) and second, whistles met the criteria of the SIGnature IDentification (SIGID) method (Janik et al., 2013) that classifies signature whistles according to the temporal patterns of whistles in bout analysis. Whistles were classified as signature whistles if 75% were produced in a bout of whistles of the same type within 1–10 s of each other. IWI values were plotted on a log-survivorship curve in R (R Core Team, 2022) to determine if whistle bout structure was in accordance with SIGID (Janik et al., 2013).
To identify if the dolphin produced a dominant stereotyped whistle contour like those of signature whistles, selected whistles underwent contour analysis comparing contours across whistles produced across all recordings. Selected whistles had a clear and distinct contour (dark in our spectrographic analysis) from start to end with a signal-to-noise ratio (SNR) 4 dB above background noise. To maximize the number of whistles for analysis, all whistles with a strong SNR were included for analysis. Whistles were considered part of the same loop sequence if they were separated by less than 250 ms. Selections were made with a border of 0.5 seconds in order to avoid cutoffs of the contour. Whistle selections underwent semi-automated contour extraction in Beluga (http://biology.st-andrews.ac.uk/SoundAnalysis/), a MATLAB based program. In Beluga, the user draws a box around the signal of interest and the program automatically detects the peak frequency to extract. The noise filter, which calculates the average noise spectrum of the spectrogram (excluding harmonics) and subtracts it, was used prior to extraction. Extractions were performed using the following settings: Extraction method = peak frequency; FFT length = 2048; frame length = 512; frame overlap = 87%; temporal resolution = 1.333 ms; and frequency resolution = 11.719 Hz.
After extraction, all resulting contours were categorized using ARTwarp (http://biology.st-andrews.ac.uk/SoundAnalysis/). ARTwarp is an accompanying MATLAB software to Beluga that uses Dynamic Time Warping (DTW) to compare whistle contours and an Artificial Resonance Theory (ART2) neural network to automatically categorize them based on similarity. The similarity threshold was set to 96%, which has been determined to successfully categorize signature whistles in bottlenose dolphins (Deecke and Janik, 2006). Whistles are compared one at a time to a “reference” contour for each category; if a whistle does not meet the similarity threshold of an existing category, a new one is created. The reference contour is continuously updated with each addition to a category to fully capture potential diversity of signals within a category. The program randomly shuffles the contours and runs through multiple iterations to avoid primacy bias in categorization; it runs through iterations until no contours are recategorized. The following settings were used: vigilance = 96.00; bias = 0.00000100; learning rate = 0.100; maximum number of categories = 50; maximum number of iterations = 100; resample contours = 10.00 ms. Whistles categorized with single-loop and multi-loop contours were stitched together in MATLAB afterwards to enable comparisons between subunits. Distribution of categories and plotting of reference contours was done via a custom MATLAB script. Discrete-time Markov chain analysis of subunit ordering was done with the Econometrics Toolbox (MathWorks, Version 6.0). All analyses were conducted with MATLAB 2022a (MathWorks Inc., 2022).
Finally, to demonstrate that the signature whistle contour of this dolphin was distinct from other whistles produced by other members of its species, we provide examples of whistle contours of rough-toothed dolphins from acoustic recordings gathered in the Eastern Tropical Pacific Ocean (ETP). These examples were acquired from the Macaulay Library at the Cornell Lab of Ornithology from the following files: ML122031, ML120528, and ML120709.
2.6 Temporal trends in sound production
A linear regression was performed in IBM SPSS v24 to assess the relationship between whistle rate and day of rehabilitation. Generalized Additive Models (GAMs) were used to evaluate whistle parameters over the course of the dolphin’s rehabilitation. Mean minimum and maximum frequencies were used as response variables in a multivariate normal additive model so that the modeling process would compensate for correlations among high and low frequencies, with day of rehabilitation included as the predictor variable. Inter-whistle interval, number of multi-looped whistles, and presence/absence of PCT were analyzed in separate models after determining that the response variables were not correlated. The PCT response variable was modeled using a binomial distribution. Each of the GAMs used individual whistles as independent samples (N = 1355). The whistle rate (whistles/min) was calculated for each day of observation and analyzed using a GAM with day as the predictor variable (n = 8). GAMS were conducted in R (R Core Team, 2022) using the gam function from the mgcv package (Wood and Wood, 2015).
2.7 Blood chemistry and hematology values
A suite of hematological and blood chemistry tests was assessed to evaluate Paki’s recovery over 21 days from May 02 to June 20, 2017. Values were sampled more in the first several weeks of the dolphin’s rehabilitation and sparsely right before its release. During each blood sample collection, approximately 10 mL of blood was collected by venipuncture via the ventral tail vein of the fluke while the animal was restrained. Standard manual cell counts of blood cells and hematologic parameters were acquired within a day of blood sampling with standard protocols. Parameters of serum and plasma were analyzed with an IDEXX VetTest Chemistry Analyzer and electronic cell counts with an IDEXX LaserCyte Dx Hematology Analyzer (IDEXX Laboratories, Inc., Westbrook, ME, USA).
Measures of white blood cells included counts of white blood cells (WBC), neutrophils, monocytes, lymphocytes, and eosinophils. Red cell blood cell parameters included erythrocyte sedimentation rate (ESR), total red blood cell count (RBC), hemoglobin, mean corpuscular hemoglobin (MCH), mean corpuscular hemoglobin concentration (MCHC), and mean corpuscular volume (MCV). Liver parameters measures included aspartate aminotransferase (AST), alanine aminotransferase (ALT), alkaline phosphatase (AP), total bilirubin, and protein parameters total protein and albumin. The values for globulin, blood urea nitrogen (BUN) and lactate dehydrogenase (LDH) were calculated. The electrolytes calcium was measured.
2.8 Health status at rescue and release
To identify the health status of the dolphin upon its rescue compared to its release time, and throughout its rehabilitation, we compared biochemistry and hematology values with a previous study on rough-tooth dolphin health and survival (Manire et al., 2018). Manire et al. (2018) demonstrated that these measures are critical in the assessment of rough-toothed dolphin health following stranding and during rehabilitation in anticipation of release. The health of the dolphin was inferred using a subset of hematological measures (i.e., WBC, neutrophils, lymphocytes, eosinophils, ESR, and RBC) collected at three time points (i.e., post stranding, throughout rehabilitation, and prior to release) and compared them to values of fatally-ill, stranded rough-toothed dolphins and other dolphins that were rehabilitated and deemed healthy enough for release (Manire et al., 2018).
2.9 Trends in health condition and association with sound production
To identify if the dolphin’s sound production was associated with differences in its health condition, physiological data gathered on dolphin blood chemistry were compared to sound production characteristics over time. The relationship between three health biomarkers (WBC, neutrophils, eosinophils) were compared to the dolphin’s whistle rate using Pearson’s correlation using IBM SPSS v24.
3 Results
3.1 Recordings and signal characteristics
A total of 16.1 hours of acoustic recordings were assessed for rough-toothed dolphin sounds recorded over 8 different days. Sound recordings ranged in duration from 5 to 85 min [mean ± standard deviation (SD); 13.7 min ± 27.2]. The dolphin primarily produced a single repeated stereotyped whistle with an ascending contour shape and numerous steps and breaks (Figures 1A–C). Table 1 provides details on dolphin sound production characteristics for the measured whistles (n = 1,200). A total of 9,272 whistles were counted over the course of the 16.1 recorded hours. Whistles were produced at a mean rate of 10.1 whistles/min and ranged from 16.6 whistles/min on the first recording day (Day 5 of rehabilitation) to 6.39 whistles/min on the last recording date (Day 15 of rehabilitation) (Table 1). PCT were detected within 100 ms of whistles in 85.5% of all analyzed whistles and had a mean duration of 623 ms ± 99 (min–max); (49–10,383 ms) and began on average 65.7 ms ± 106.2 (0–490 ms) prior to the begin time of the first loop.
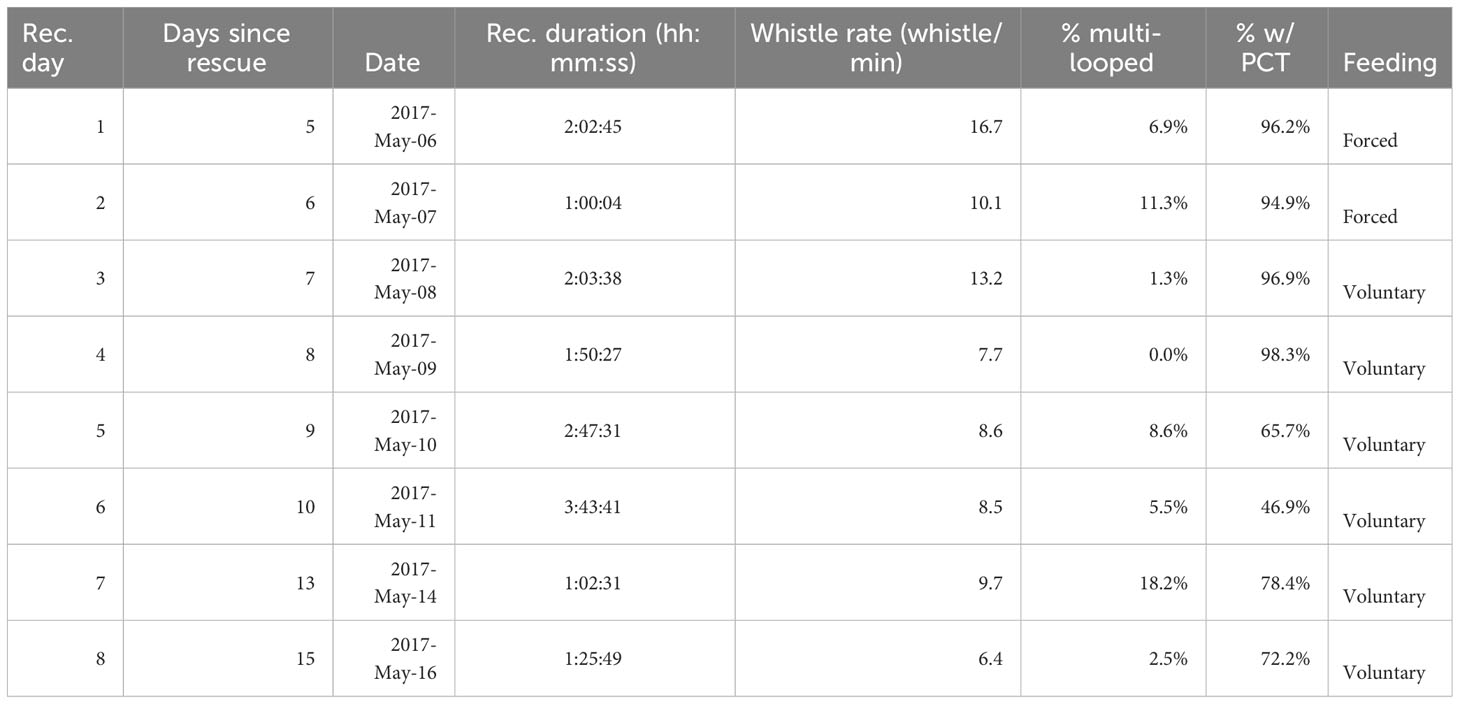
Table 1 Details on acoustic analysis of the vocalizations of the live-stranded rough-toothed dolphin.
3.2 Signature whistle analysis
SIGID analysis confirmed this whistle meets the criteria to be considered a signature whistle. The log-survivorship plot of IWI revealed the most common interval between whistles of the same type (n = 650) was below 10 s (Figure 2), conforming to the bout interval criterion predicted for signature whistles according to SIGID.
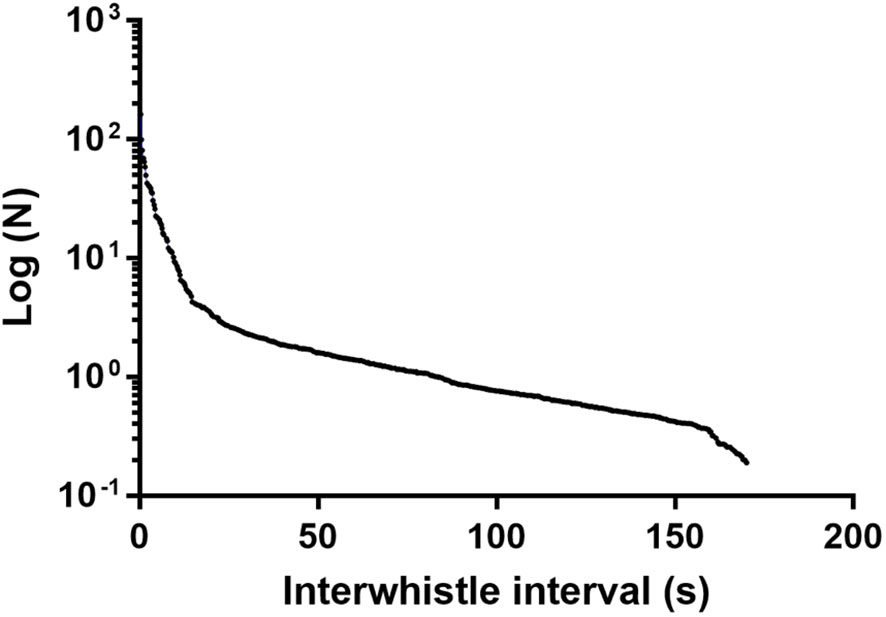
Figure 2 The log-survivorship plot of inter-whistle interval (IWI) in seconds as a function of the number of analyzed whistles (n = 650). The curve illustrates that most whistles occurred in bouts with an IWI of <10 s.
A total of 1,930 whistle contours were extracted for contour analysis. Of these, 76.4% were single looped whistles, 21.9% were double looped whistles, and 1.7% were triple looped whistles. The majority (99.7%) of the whistles were confirmed to contain “Whistle 1,” Paki’s proposed signature whistle (Figure 3A). This whistle category was the result of a 96% similarity threshold, which has been proven to successfully categorize signature whistles in bottlenose dolphins (Deecke and Janik, 2006). The remaining 5 whistles (0.3%) visually appear to be a connected multiloop whistle that starts with the same contour structure as Whistle 1 (Figure 3B). All of the separated loop whistles started with Whistle 1 (Figure 3C). Many of the embellishments were stereotyped with high repetition rates, particularly Whistle 3, which appears to be a truncated version of Whistle 1, and Whistle 4, an upsweep (i.e., predominantly increasing in frequency) (Table 2). Whistle 3 only ever occurred as a pair following Whistle 1 (Figure 3D), whereas the other whistles were found as part of three-looped whistles (Figure 3E).
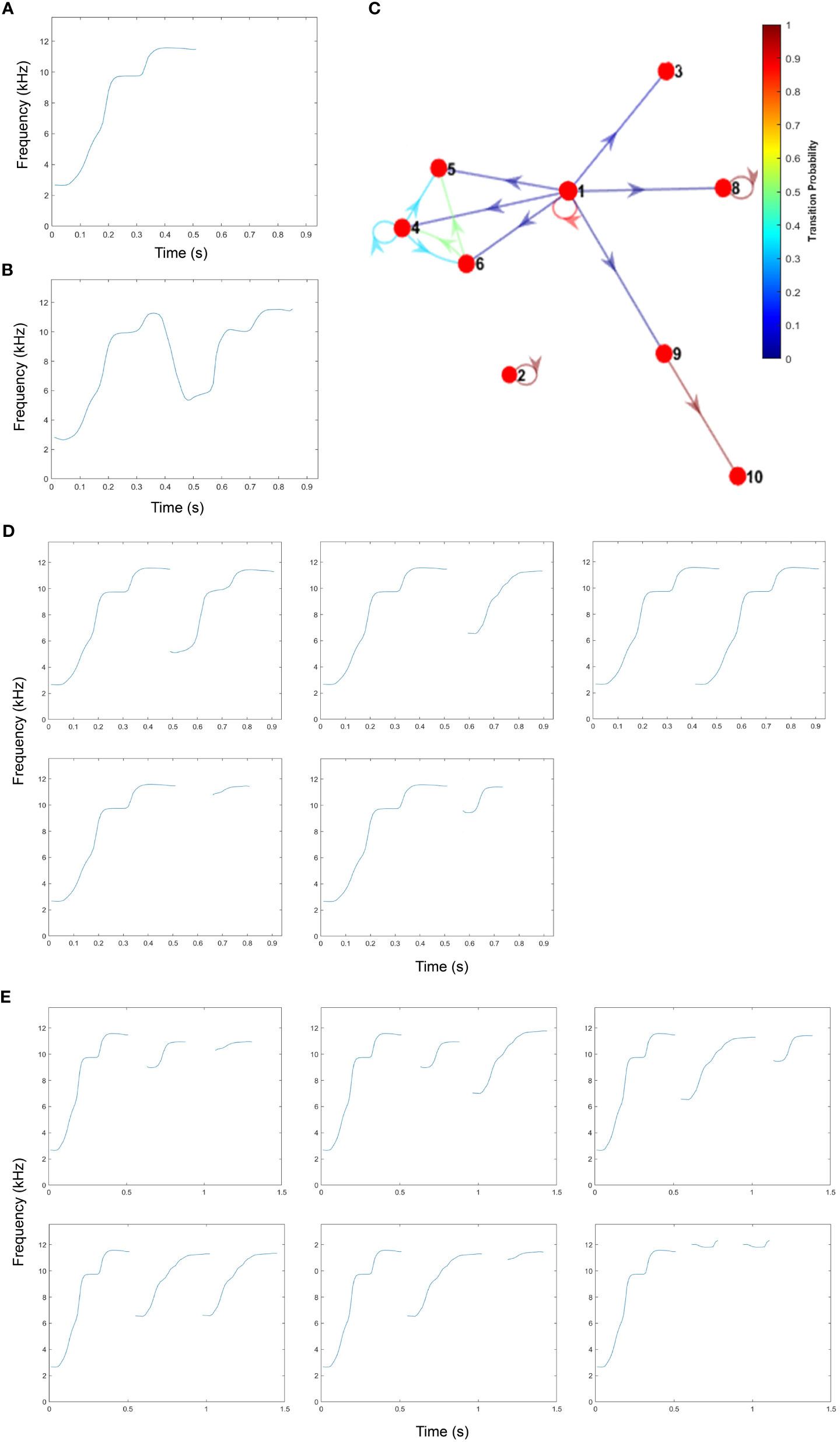
Figure 3 Whistle contours representing ARTwarp categorizations of the single rough-toothed dolphin. (A) Whistle 1, the proposed Signature Whistle of the individual dolphin. (B) Whistle 2, proposed multiloop embellishment of Whistle 1. (C) Discrete-Time Markov Chain derived from ARTwarp categorizations and position in loop sequence. (D) Stereotyped two-loop whistle; (clockwise) Whistles 1 and 3; Whistles 1 and 4; Whistle 1 repeat; Whistles 1 and 6; Whistles 1 and 5. (E) Three-looped whistles; (clockwise) Whistles 1, 6, and 5; Whistles 1, 6, and 4; Whistles 1, 4, and 6; Whistles 1, 8, and 8; Whistles 1, 4, and 5; Whistles 1, 5, and 5. All contours were generated from ARTwarp output and plotted with a custom MatLab Script.
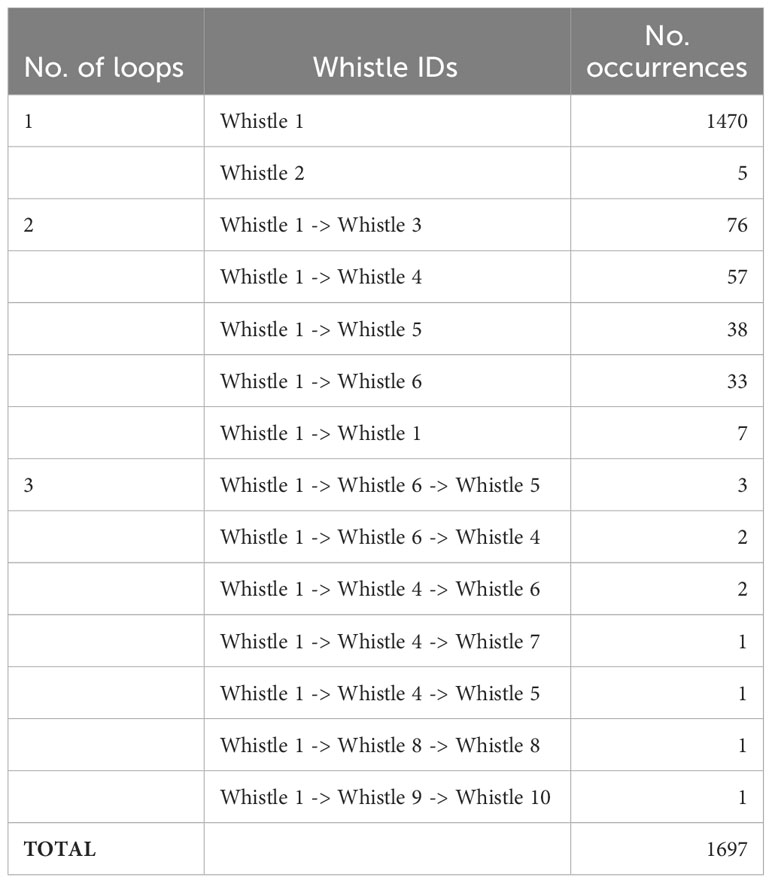
Table 2 Occurrences of whistle contour categories across whistles with different numbers of loops and loop sequences.
To demonstrate the whistle type produced by Paki was not potentially a species-typical whistle, we provided spectrograms showing different whistle contours from recordings of wild rough-toothed dolphins accessed from the Macaulay Library at the Cornell Lab of Ornithology (Figures 4A–F). The contours of rough-toothed dolphin whistles from the ETP varied in shape, with numerous examples of downsweeps (i.e., predominantly decreasing frequencies) (Figures 4A, C), upsweeps (Figure 4B), and other contours (Figures 4D–F). This included repeated stereotyped whistle contours from the same recordings (Figures 4D, E).
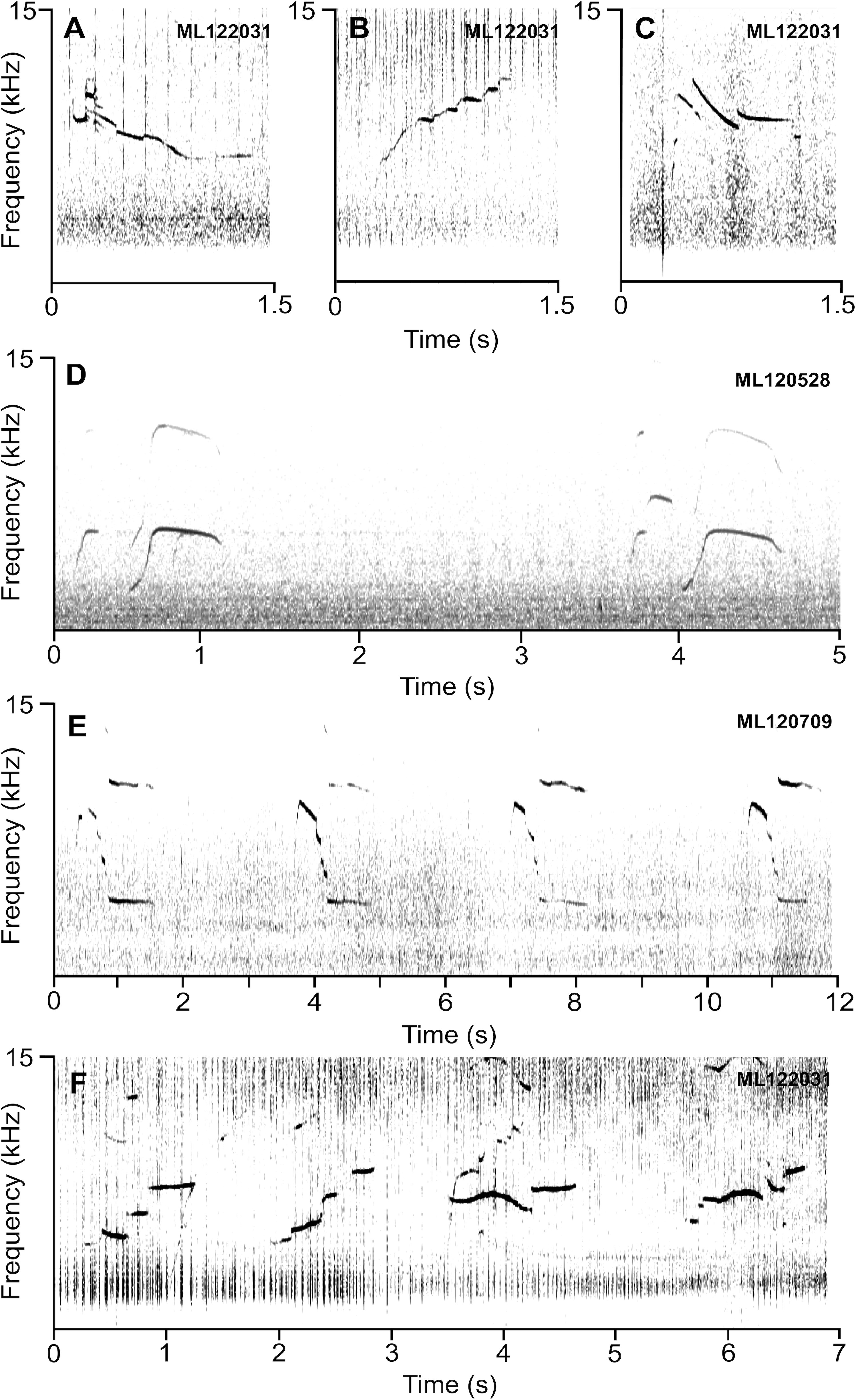
Figure 4 Example spectrograms of rough-toothed dolphin whistles recorded in the Eastern Tropical Pacific Ocean. (A) A whistle with a downsweep contour. (B) A whistle with an upsweep contour. (C) A downsweep whistle contour. (D) A sequence of two double-looped whistles of the same stereotyped contour. (E) A sequence of four single-looped whistles of the same stereotyped contour. (F) A sequence of whistles of different contours and overlapping echolocation click trains. These sounds were acquired from the Macaulay Library at the Cornell Laboratory of Ornithology. The following recordings were used: ML122031, ML120528, and ML120709. Spectrograms were produced in Raven 1.6 (Cornell Lab of Ornithology) with the following parameters: DFT: 1024 points; Hamming window; 10.3 ms temporal resolution.
3.3 Temporal analysis of sounds
Whistle rate significantly decreased over the course of Paki’s rehabilitation according to a Linear regression: y = 15.57-0.67x; p = 0.027, one-tailed; R2 = 0.486 (Figure 5A). The multivariate GAM that modeled mean minimum and maximum frequencies accounted for 3.5% of variation among whistle frequencies. Day was a statistically significant predictor for mean high (edf = 5.149, p < 0.001) and low frequencies (edf = 5.914, p < 0.001), with the frequencies oscillating over the course of rehabilitation (Figures 5B, C). Given the low percentage of variation accounted for by the model, variation in frequencies was likely explained by variables unrelated to the progression of time and rehabilitation.
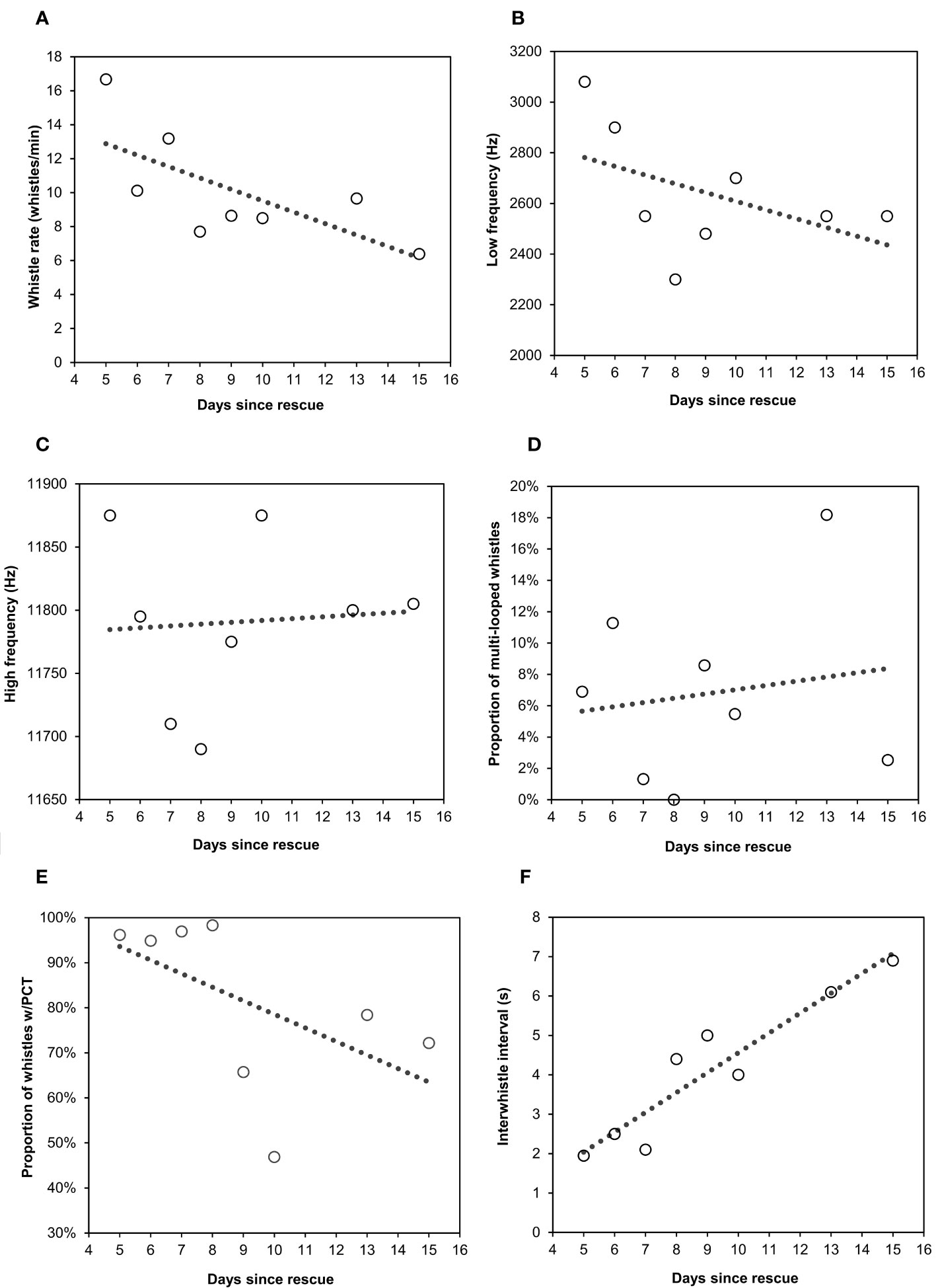
Figure 5 Scatterplots illustrating whistle production characteristics of the single rough-toothed dolphin plotted over 8 days of acoustic recordings acquired during the first 15 days of its captive rehabilitation. (A) Whistle rate (whistles/minute) decreased over time and was significantly related to recovery date according to a linear regression. (B) Mean low frequency and (C) high frequency were significantly predicted by day but oscillated throughout the assessed period according to Generalized Additive Models. (D) The proportion of multi-looped whistles varied over time with no clear increasing or decreasing trends over time. (E) The proportion of whistles with preceding click trains (PCT) decreased over the 8 recording days, as illustrated by the linear trend lines, while (F) inter-whistle interval (s) increased.
Day was a statistically significant predictor for the inter-whistle interval (edf = 6.715, p < 0.001), number of loops per whistle (edf = 5.58, p < 0.001) (Figure 5D), and the occurrence of PCT in whistles (edf = 6.461, p < 0.001). The proportion of whistles with PCT decreased significantly over the 11 days in rehabilitation with acoustic recordings (Figure 5E) with day accounting for 22.1% of the variation. The inter-whistle interval showed an oscillating increase in duration (Figure 5F) with day accounting for 24.7% of the variance.
3.4 Temporal trends in health status
One day following its rescue, values of WHC, neutrophils, eosinophiles, and ESR were more similar to that of fatally sick rough-toothed dolphins than healthy ones as reported by Manire et al. (2018) (Table 3). On the day prior to its release, these values were more similar to that of healthy dolphins than fatally ill individuals (Figure 6) suggesting rehabilitation was successful as returning Paki to an improved health status like previously released rough-toothed dolphins following rehabilitation.
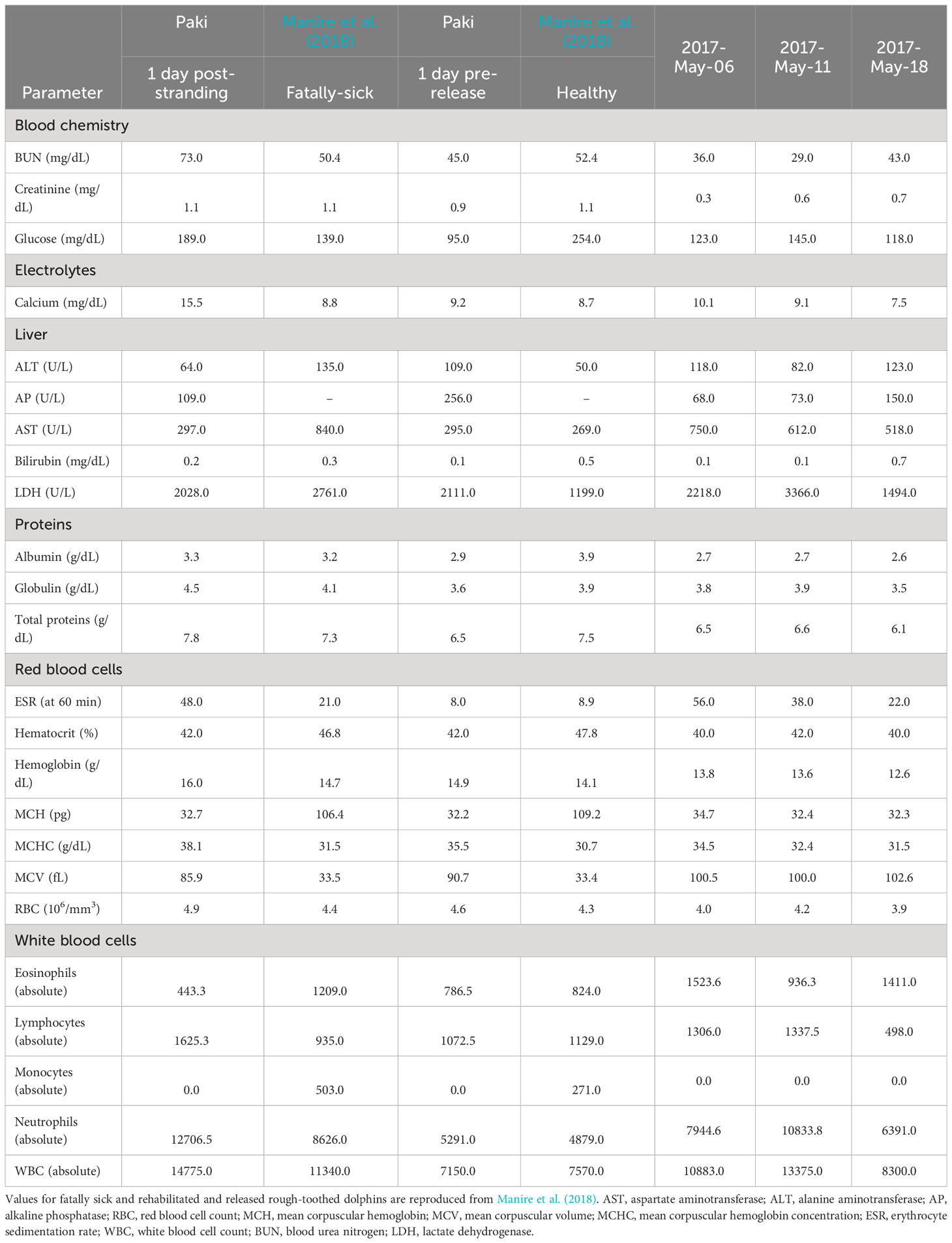
Table 3 Mean values for physiological and blood chemistry measures taken from “Paki,” the rehabilitated rough-toothed dolphin at Dolphin Discovery at Isla Mujeres, Quintana Roo, Mexico.
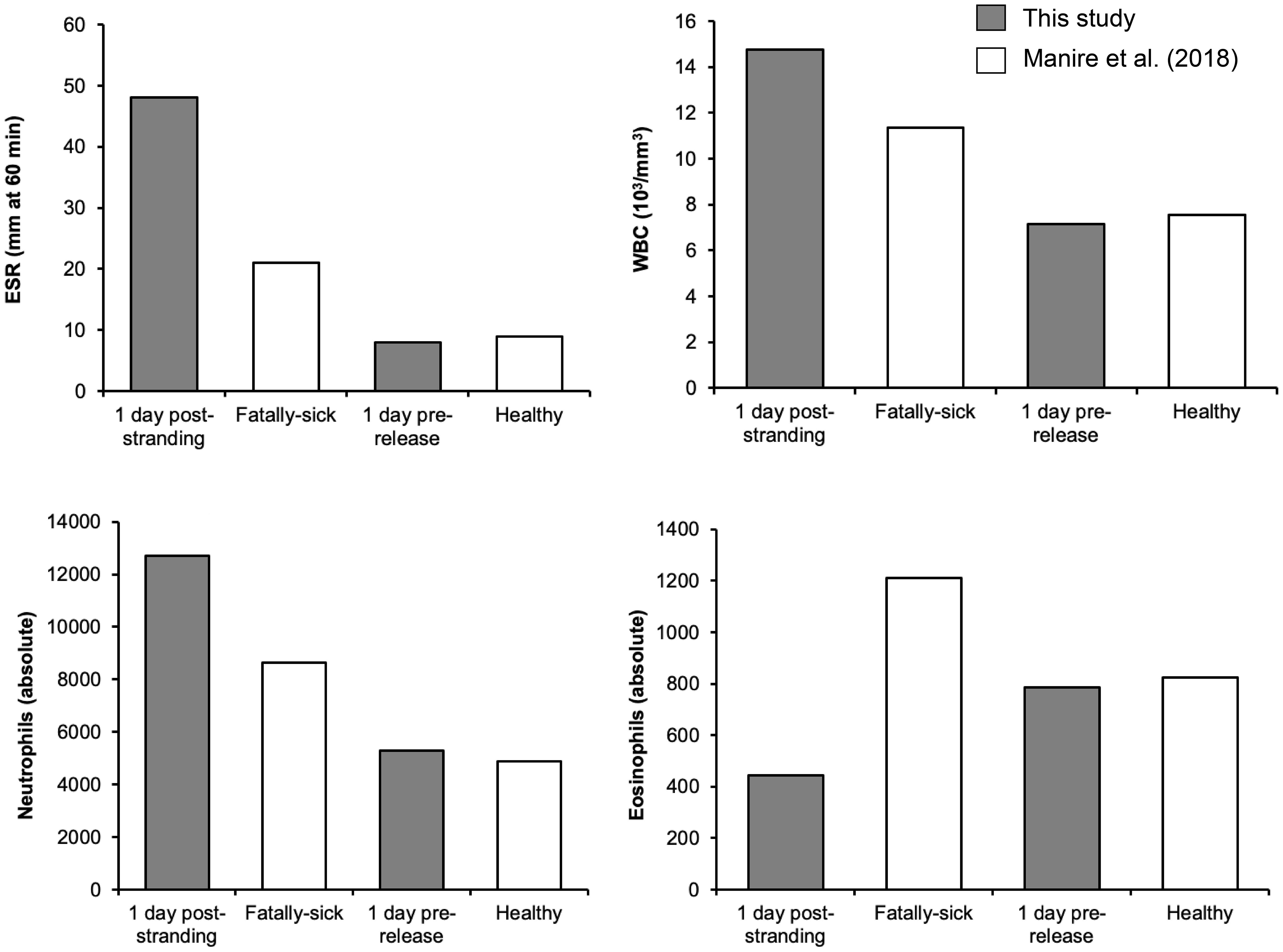
Figure 6 Comparisons of the measured hematology parameters for the rehabilitated rough-toothed dolphin recorded at Dolphin Discovery at Isla Mujeres in Quintana Roo, Mexico. Measures included absolute counts of neutrophils, eosinophils, white blood cells (WBC), and erythrocyte sedimentation rate (ESR). Parameters of Fatally-sick and Healthy dolphins were acquired from Manire et al. (2018) and were compared to hematology parameters for Paki recorded 1 day post-stranding and 1 day prior to his release.
3.5 Association between whistle production and health
Two example measures indicative of good health in rehabilitated rough-toothed dolphins (i.e., WBC, neutrophils) and its whistle rate were plotted over time on the same plot as characteristics of sound production (Figure 7). Significant decreases in whistle rate over time identified in the GAMs were associated with decreases in WBC and neutrophils but not with eosinophils.
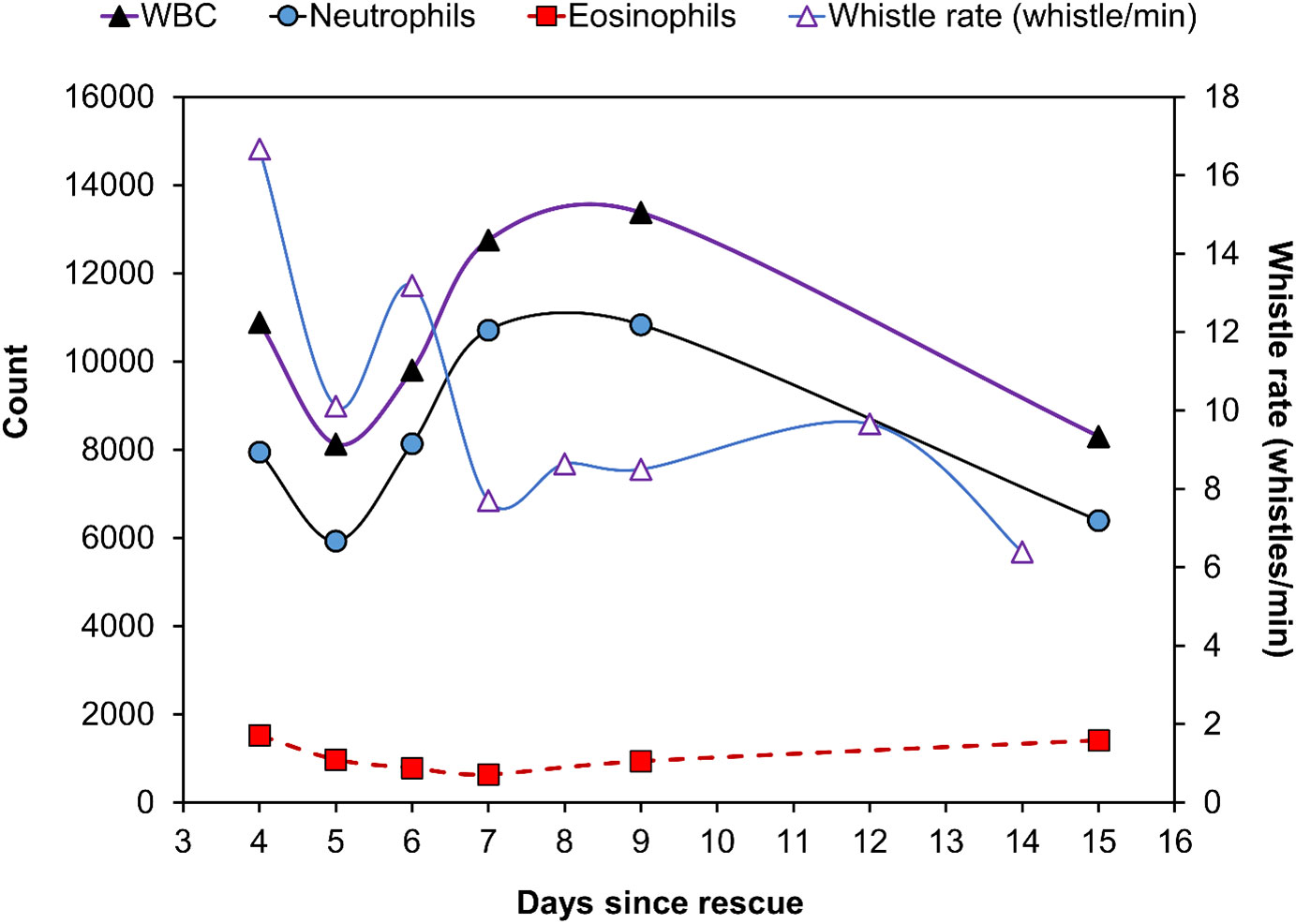
Figure 7 Scatterplot of dolphin whistle rate (whistles/min) and counts of white blood cells (WBC), neutrophils, and eosinophils of the single rough-toothed dolphin measured while in captive rehabilitation from May 5 to 19, 2017.
Although whistle rate was significantly related to recovery date (see linear regression in 4.3); it was not significantly related to any of the three health biomarkers we compared here (WBC; Pearson’s Correlation = -0.39, p = 0.258; Neutrophils; Pearson’s Correlation = -0.49; p = 0.201; Eosinophils; Pearson’s Correlation = 0.79; p = 0.057). This result is related to the above (see 4.4) where the health biomarkers were not linearly related to recovery day.
4 Discussion
Our study presents initial evidence of signature whistle production by a single male rough-toothed dolphin during its rehabilitation. This dolphin predominantly produced a single stereotyped whistle contour, which we identified as its signature whistle. The SIGID method results, contour analysis of the dolphin’s signature whistles, and analysis of whistle bouts bolster the hypothesis that the whistle type it produced can be classified as this individual’s signature whistle. These findings provide compelling evidence to further investigate the presence of signature whistles in this species. To the best of our knowledge, no studies have examined signature whistles in captive or wild rough-toothed dolphin populations. However, spectrograms found in other publications (Lima et al., 2016; Caruso et al., 2019) and available databases (Macaulay Library at the Cornell Lab of Ornithology) illustrate the production of various other whistle contours by rough-toothed dolphins in populations from the ETP (see Figure 4).
The signature whistles produced by this dolphin exhibited similar variation in features to those of bottlenose dolphins reported in previous studies. Most whistles contained a single stereotyped loop, but occasionally, the dolphin produced multi-looped whistles with disconnected loops that varied in structure, typically featuring the same introductory loop but different subsequent and terminal loops, sometimes appearing as segmented extensions of the first loop. Moreover, different whistles displayed features present in the largest database of bottlenose dolphin signature whistles from the dolphin population at Sarasota Bay, Florida (Sayigh et al., 2022). This includes deletions of certain sections of the whistle, amplitude modulations creating sidebands (Tyack and Recchia, 1991), and additions of other components to whistles, such as nonlinear phenomena (Tyson et al., 2007). Whistle contours sometimes exhibited abrupt changes in frequency (breaks) or steps (Oswald et al., 2007). Most whistles were preceded by short click trains or burst-pulse sounds and featured numerous steps and breaks (Lima et al., 2012; Lima et al., 2016). The regular occurrence of this click train immediately prior to its signature whistle suggests that it may be a component of the dolphin’s signature whistle (Sportelli et al., 2023). Future studies will need to ascertain whether this is a typical feature of rough-toothed dolphin signature whistles, more prominent in dolphins that are unwell, or if it is a feature individually unique to this animal. Finally, while day was a significantly significant predictor of mean low and high frequencies, these values oscillated throughout recordings and are unlikely to be directly linked to the dolphin’s progression in rehabilitation.
The dolphin exhibited high rates of whistle production, with statistically significant decreases in whistle production over time. The mean whistle rate of the rough-toothed dolphin we report here (16.67 whistles/min) was similar to the mean whistle rate (14.3 per/min) of bottlenose dolphins recorded during capture-release sessions in Sarasota Bay, Florida (Esch et al., 2009). The high whistle rate may have indicated distress, as the animal was unwell, stranded, and surrounded by humans for veterinary care. Animals experiencing stress may produce vocalizations with characteristics specific to stressful contexts (Lilly, 1963; Noirot and Pye, 1969; Sehrsweeney et al., 2018; Sehrsweeney et al., 2019). Similar high-repetition rate of signature whistles has previously been associated with both contact and distress signaling (e.g., Cook et al., 2004; Esch et al., 2009; Kuczaj et al., 2015), for example, 15.26 whistles per minute for a distressed dolphin reported by Kuczaj et al. (2015). Likewise, the decrease in the number whistles with PCT during the dolphin’s rehabilitation could correlate with decreased distress coinciding with improved health. Alternatively, some studies in odontocetes provide contradictory results with decreased vocal activity rates associated with stressful contexts (i.e., beluga whales [Delphinapterus leucas]; Castellote and Fossa, 2006). The proportion of multi-looped whistles was predicted by day but did not exhibit a clear trend over time and does not appear to be associated with health changes. In contrast, Esch et al. (2009) found that bottlenose dolphins restrained for health procedures in Sarasota Bay, Florida, produced signature whistles with more loops when stressed and fewer loops when undisturbed. Recordings captured following forced feedings in the early days of rehabilitation were likely influenced by this significant stressor, which required the dolphin’s physical restraint, handling by several people, and forced feeding. However, it is essential to consider that high vocal production rates may simply be characteristic of this species.
Concurrently, we conducted health assessments that involved measuring blood chemistry values which provided evidence of improvements in its health condition of the dolphin from stranding to release. Whistle rate significantly decreased over time at an average of -0.67 whistles per minute per day. That said, whistle rate remained high (6.39 whistles/min) even on the final recording day (i.e., 15 days following rescue). Although the whistle rate decreased as the dolphin recovered, it was not significantly correlated to the health biomarkers. This was largely a result of the health biomarkers during worsening before they recovered (Figure 7), and a small sample size (n = 5 blood sampling dates concurrent with recordings). The reduction in whistle rate over time could partly be related to habituation effects to the new environment and presence of human intervention but was also likely related to its recovery from fatally ill to releasable. Similarly, changes in vocal production could be the results of a habituation effect on the use of cohesion call in which the dolphin did not receive a response for several days. Finally, social deprivation for a social animal that typically lives in groups likely influenced the dolphin in ways we cannot readily account for.
Understanding the contextual use of sound is critical for passively identifying stressful circumstances in stranded and captive marine mammals, as well as for implementing strategies to improve their welfare conditions and facilitate optimal rehabilitation (Esch et al., 2009). We found that changes in the sound production characteristics of a rough-toothed dolphin during its rehabilitation could be associated with changes in sound production. Similar to the hematological parameters reported by Manire et al. (2018) for healthy rough-toothed dolphins after rehabilitation and release following a mass stranding in Florida, USA, the improvements in the dolphin’s health were reflected in changes in health parameters. More research is needed to test if acoustic monitoring and the assessment of whistle production rate can be employed as non-invasive indicators of health or stress in stranded rough-toothed dolphins and other cetaceans (Jones et al., 2021) and potentially as an indicator of their suitability for release. Other measures of body condition, body mass, and histopathology have been shown to be important to assessing rough-toothed dolphin health following mass strandings (Karns et al., 2019; Ewing et al., 2020).
Our findings should be considered preliminary given we only evaluated one individual, and the illness that caused Paki’s stranding may indicate it was not representative of healthy wild individuals. The dolphin demonstrated species-typical movement behavior in the two months (July to August) post-release until the battery of the radio tag failed, indicating its rehabilitation and release were successful and the dolphin had returned to normal behavior (R. Sanchez Okrucky, Unpublished data). The health of the dolphin during its rehabilitation improved, and similarly, we assumed that in its first days its stress levels were likely higher given the novel environment and daily manual restraint and forced feeding. However, we did not take direct measures of stress (i.e., cortisol levels).
5 Conclusions
Despite our study being limited to a single deep-water dolphin recorded in novel captive contexts in shallow water, it provides a unique and important foundation for future research to build upon. Our report of a possible signature whistle in a rough-toothed dolphin should encourage other scientists to search for the same in their own datasets, using the SIGID method and contour analysis. Furthermore, understanding signature whistles in this species can facilitate the detection and classification of signals from other delphinids detected in passive acoustic monitoring devices (Lima et al., 2012), track dolphins through mark-recapture studies (Longden et al., 2020), and inform decisions on recording strategies such as duty cycles using distributed arrays (Fearey et al., 2019). Finally, our study supports the value of exploring the use of non-invasive acoustic monitoring as a tool for evaluating dolphin health and welfare.
Data availability statement
The original contributions presented in the study are included in the article. Further inquiries can be directed to the corresponding author.
Ethics statement
The animal study was approved by Dolphin Discovery Veterinary Group. The study was conducted in accordance with the local legislation and institutional requirements.
Author contributions
ER: Conceptualization, Data curation, Formal Analysis, Methodology, Project administration, Visualization, Writing – original draft, Writing – review & editing. BJ: Formal Analysis, Investigation, Methodology, Validation, Visualization, Writing – review & editing. MA: Formal Analysis, Investigation, Methodology, Visualization, Writing – review & editing. KC: Writing – original draft, Formal Analysis, Investigation, Methodology, Visualization, Writing – review & editing. LE: Formal Analysis, Investigation, Methodology, Visualization, Writing – original draft, Writing – review & editing. GM-S: Formal Analysis, Investigation, Methodology, Writing – review & editing. NC-M: Conceptualization, Investigation, Methodology, Project administration, Writing – original draft, Writing – review & editing. MRA: Conceptualization, Data curation, Investigation, Methodology, Project administration, Supervision, Writing – original draft, Writing – review & editing. RSO: Data curation, Project administration, Writing – original draft, Formal Analysis, Investigation, Methodology, Supervision, Writing – review & editing, Conceptualization. GR: Formal Analysis, Investigation, Methodology, Supervision, Writing – review & editing.
Funding
The author(s) declare that no financial support was received for the research, authorship, and/or publication of this article.
Acknowledgments
Thank you to all of the personnel of Dolphin Discovery, la Zona Federal Marítimo Terrestre (ZOFEMAT), la Secretaría de Gestión Integral de Riesgos y Protección Civil, la Secretaría de Ecología y Medio Ambiente of Isla Mujeres, and la Procuraduría Federal de Protección al Ambiente (PROFEPA) in Mexico who helped coordinate rescue and rehabilitation effort. We thank all of the volunteers who assisted with the rescue efforts and the staff and the veterinary medicine team of Dolphin Discovery. Thanks to Tortugranja Isla Mujeres for generously housing the animal during recovery.
Conflict of interest
The authors declare that the research was conducted in the absence of any commercial or financial relationships that could be construed as a potential conflict of interest.
Publisher’s note
All claims expressed in this article are solely those of the authors and do not necessarily represent those of their affiliated organizations, or those of the publisher, the editors and the reviewers. Any product that may be evaluated in this article, or claim that may be made by its manufacturer, is not guaranteed or endorsed by the publisher.
References
Bioacoustics Research Program (2019). Raven Pro: Interactive sound analysis software (version 1.5) [computer program] (Ithaca, NY: The Cornell Lab of Ornithology). Available at: http://www.birds.cornell.edu/raven.
Busnell R. G., Dziedzic A. (1966). Caracteristiques physiques de certains signaux acoustiques du delphidide Steno bredanensis, Lesson (Physical characteristics of certain acoustic signals of the delphinid Steno bredanensis, Lesson). Comptes rendus l'Académie Des. Sciences Ser. D 268, 143–146.
Caldwell M. C., Caldwell D. K. (1965). Individualized whistle contours in bottle-nosed dolphins (Tursiops truncatus). Nature 207 (4995), 434–435.
Caldwell M. C., Caldwell D. K., Miller F. J. (1973). Statistical evidence for individual signature whistles in the spotted dolphin, Stenella plagiodon. Cetology 3, 1–9.
Caldwell M. C., Caldwell D. K., Tyack P. L. (1990). “Review of the signature whistle hypothesis for the Atlantic bottlenose dolphin,” in The bottlenose dolphin. Eds. Leatherwood S., Reeves R. R. (San Diego, CA: Academic Press), 199–234.
Caruso F., Sciacca V., Parisi I., Viola S., de Vincenzi G., Bocconcelli A., et al. (2019). Acoustic recordings of rough-toothed dolphin (Steno bredanensis) offshore Eastern Sicily (Mediterranean Sea). J. Acoust. Soc. Am. 146 (3), EL286–EL292. doi: 10.1121/1.5126118
Castellote M., Fossa F. (2006). Measuring acoustic activity as a method to evaluate welfare in captive beluga whales (Delphinapterus leucas). Aquat. Mammals 32 (3), 325–333. doi: 10.1578/AM.32.3.2006.325
Cheng Z., Wang D., Wu H., Huang S. L., Pine M. K., Peng C., et al. (2017). Stereotyped whistles may be first evidence to suggest the possibility of signature whistles in an injured Indo-Pacific humpback dolphin (Sousa chinensis). Aquat. Mammals 43, 185–192. doi: 10.1578/AM.43.2.2017.185
Cook M. L., Sayigh L. S., Blum J. E., Wells R. S. (2004). Signature–whistle production in undisturbed free–ranging bottlenose dolphins (Tursiops truncatus). Proc. R. Soc. London: B Ser. 271, 1043–1049. doi: 10.1098/rspb.2003.2610
Deecke V. B., Janik V. M. (2006). Automated categorization of bioacoustics signals: Avoiding perceptual pitfalls. J. Acoust. Soc. Am. 119, 645–653. doi: 10.1121/1.2139067
Esch H. C., Sayigh L. S., Blum J. E., Wells R. S. (2009). Whistles as potential indicators of stress in bottlenose dolphins (Tursiops truncatus). J. Mammalogy 90, 638–650. doi: 10.1644/08-MAMM-A-069R.1
Ewing R. Y., Rotstein D. S., McLellan W. A., Costidis A. M., Lovewell G., Schaefer A. M., et al. (2020). ). Macroscopic and histopathologic findings from a mass stranding of rough-toothed dolphins (Steno bredanensis) in 2005 on marathon key, florida, USA. Front. Veterinary Sci. 7. doi: 10.3389/fvets.2020.00572
Fearey J., Elwen S. H., James B. S., Gridley T. (2019). Identification of potential signature whistles from free-ranging common dolphins (Delphinus delphis) in South Africa. Anim. Cogn. 22, 777–789. doi: 10.1007/s10071-019-01274-1
Gridley T., Cockcroft V. G., Hawkins E. R., Blewitt M. L., Morisaka T., Janik V. M. (2014). Signature whistles in free-ranging populations of indo-pacific bottlenose dolphins, tursiops aduncus. Mar. Mammal Sci. 30, 512–527. doi: 10.1111/mms.12054
Herzing D. L. (1996). Vocalizations and associated underwater behavior of free-ranging Atlantic spotted dolphins, Stenella frontalis and bottlenose dolphins, Tursiops truncatus. Aquat. Mamm. 22, 61–80. doi: 10.12966/abc.02.02.2015
Janik V. M., King S. L., Sayigh L. S., Wells R. S. (2013). Identifying signature whistles from recordings of groups of unrestrained bottlenose dolphins (Tursiops truncatus). Mar. Mammal Sci. 29, 109–122. doi: 10.1111/j.1748-7692.2011.00549.x
Janik V. M., Sayigh L. S. (2013). Communication in bottlenose dolphins: 50 years of signature whistle research. J. Comp. Physiol. A 199.6, 479–489. doi: 10.1007/s00359-013-0817-7
Janik V. M., Slater P. J. B. (1998). Context-specific use suggest that bottlenose dolphin signature whistles are cohesion calls. Anim. Behav. 56, 829–838. doi: 10.1006/anbe.1998.0881
Jefferson T. A. (2009). “Rough-toothed dolphin–Steno bredanensis,” in Encyclopedia of Marine Mammals. Eds. Perrin W. F., Würsig B., Thewissen J. G. M. (Amsterdam: Academic), 990–992.
Jones B. L., Oswald M., Tufano S., Baird M., Mulsow J., Ridgway S. H. (2021). A system for monitoring acoustics to supplement an animal welfare plan for bottlenose dolphins. J. Zoological Botanical Gardens 2 (2), 222–233. doi: 10.3390/jzbg2020015
Karns B. L., Ewing R. Y., Schaefer A. M. (2019). Evaluation of body mass index as a prognostic indicator from two rough-toothed dolphin (Steno bredanensis) mass strandings in Florida. Ecol. Evol. 9 (18), 10544–10552. doi: 10.1002/ece3.5574
King S. L., Janik V. M. (2013). Bottlenose dolphins can use learned vocal labels to address each other. Proc. Natl. Acad. Sci. 110, 13216–13221. doi: 10.1073/pnas.1304459110
Kuczaj S. A., Frick E. E., Jones B. L., Lea J. S., Beecham D., Schnöller F. (2015). Underwater observations of dolphin reactions to a distressed conspecific. Learn. Behav. 43, 289–300. doi: 10.3758/s13420-015-0179-9
Kuczaj S. A. II, Yeater D. B. (2007). Observations of rough-toothed dolphins (Steno bredanensis) off the coast of Utila, Honduras. J. Mar. Biol. Assoc. United Kingdom 87, 141–148. doi: 10.1017/S0025315407054999
Lammers M. O., Au W. W. L., Herzing H. L. (2003). The broadband social acoustic signaling behavior of spinner and spotted dolphins. J. Acoustical Soc. America 114, 1629–1639. doi: 10.1121/1.1596173
Lilly J. C. (1963). Distress call of the bottlenose dolphin: stimuli and evoked behavioral responses. Science 139 (3550), 116–118.
Lima I. M., Andrade L. G., Bittencourt L., Bisi T. L., Flach L., Lailson-Brito J. Jr., et al. (2016). Whistle comparison of four delphinid species in Southeastern Brazil. J. Acoustical Soc. America 139, EL124–EL127. doi: 10.1121/1.4947310
Lima I. M. S., Guimarães de Andrade L., Ramos de Carvalho R., Lailson-Brito J. Jr., Azevedo A. (2012). Characteristics of whistles from rough-toothed dolphins (Steno bredanensis) in Rio de Janeiro coast, southeastern Brazil. J. Acoust. Soc. Am. 131, 4173–4181. doi: 10.1121/1.3701878
Lodi L. (1992). Epimeletic behavior of free-ranging rough-toothed dolphins, Steno bredanensis, from Brazil. Mar. Mamm. Sci. 8 (3), 284–287.
Longden E. G., Elwen S. H., McGovern B., James B. S., Embling C. B., Gridley T. (2020). Mark–recapture of individually distinctive calls—a case study with signature whistles of bottlenose dolphins (Tursiops truncatus). J. Mammalogy 101 (5), 1289–1301. doi: 10.1093/jmammal/gyaa081
Manire C. A., Reiber C. M., Gaspar C., Rhinehart H. L., Byrd L., Sweeney J., et al. (2018). Blood chemistry and hematology values in healthy and rehabilitated rough-toothed dolphins (Steno bredanensis). J. Wildlife Dis. 54 (1), 1–13. doi: 10.7589/2016-07-152
MathWorks Inc. (2022). MATLAB version: 9.13.0 (R2022b). (Natick, Massachusetts: The MathWorks Inc). Available at: https://www.mathworks.com.
May-Collado L. J., Wartzok D. (2008). A comparison of bottlenose dolphin whistles in the Atlantic Ocean: Factors promoting whistle variation. J. Mammalogy 89, 1229–1240. doi: 10.1644/07-MAMM-A-310.1
Noirot E., Pye D. (1969). Sound analysis of ultrasonic distress calls of mouse pups as a function of their age. Anim. Behav. 17, 340–349. doi: 10.1016/0003-3472(69)90020-7
Oswald J. N., Rankin S., Barlow J., Lammers M. O. (2007). A tool for real-time acoustic species identification of delphinid whistles. J. Acoust. Soc. Am. 122 (1), 587–595. doi: 10.1121/1.2743157
Quick N. J., Janik V. M. (2008). Whistle rates of wild bottlenose dolphins (Tursiops truncatus): Influences of group size and behavior. J. Comp. Psychol. 122 (3), 305–311. doi: 10.1037/0735-7036.122.3.305
Rankin S., Oswald J. N., Barlow J. (2008). Acoustic behavior of dolphins in the Pacific Ocean: Implications for using passive acoustic methods for population studies. Can. Acoustics 36, 88–92.
Rankin S., Oswald J. N., Simonis A., Barlow J. (2015). Vocalizations of the rough-toothed dolphin, Steno bredanensis, in the Pacific Ocean. Mar. Mammal Sci. 31, 1538–1548. doi: 10.1111/mms.12226
R Core Team (2022) R: A Language and Environment for Statistical Computing (Vienna, Austria: R Foundation for Statistical Computing) (Accessed 23 November 2022).
Ridgway S. H. (1983). "Dolphin hearing and sound production in health and illness." In: Perspectives on modern auditory research: papers in honor of Fay R. R., Gourevitch G.. (Groton: Amphora Press), 247–296.
Sayigh L. S., El Haddad N., Tyack P. L., Janik V. M., Wells R. S., Jensen F. H. (2023). Bottlenose dolphin mothers modify signature whistles in the presence of their own calves. Proc. Natl. Acad. Sci. 120 (27), e2300262120. doi: 10.1073/pnas.2300262120
Sayigh L. S., Esch H. C., Wells R. S., Janik V. M. (2007). Facts about signature whistles of bottlenose dolphins. Tursiops truncatus. Anim. Behav. 74, 1631–1642. doi: 10.1016/j.anbehav.2007.02.018
Sayigh L. S., Janik V. M., Jensen F. H., Scott M. D., Tyack P. L., Wells R. S. (2022). The Sarasota Dolphin Whistle Database: A unique long-term resource for understanding dolphin communication. Front. Mar. Sci. 9. doi: 10.3389/fmars.2022.923046
Sayigh L. S., Tyack P. L., Wells R. S., Solow A. R., Scott M. D., Irvine A. B. (1999). Individual recognition in wild bottlenose dolphins: A field test using playback experiments. Anim. Behav. 57, 41–50. doi: 10.1006/anbe.1998.0961
Sayigh L. S., Tyack P. L., Wells R. S., Scott M. D. (1990). Signature whistles of free-ranging bottlenose dolphins Tursiops truncatus: stability and mother-offspring comparisons. Behav. Ecol. Sociobiol. 26, 247–260. doi: 10.1007/BF00178318
Sehrsweeney M., Wilson D. R., Bain M., Boutin S., Lane J. E., McAdam A. G., et al. (2018). Is physiological stress state reflected in acoustic structure of vocalizations? An experimental test in wild North American red squirrels. BioRxiv, 456830. doi: 10.1101/456830
Sehrsweeney M., Wilson D. R., Bain M., Boutin S., Lane J. E., McAdam A. G., et al. (2019). The effects of stress and glucocorticoids on vocalizations: a test in North American red squirrels. Behav. Ecol. 30 (4), 1030–1040. doi: 10.1093/beheco/arz044
Shapiro A. D. (2006). ). Preliminary evidence for signature vocalizations among free-ranging narwhals (Monodon monoceros). J. Acoustical Soc. America 120, 1695–1705. doi: 10.1121/1.2226586
Sportelli J. J., Jones B. L., Ridgway S. H. (2023). Non-linear phenomena: a common acoustic feature of bottlenose dolphin (Tursiops truncatus) signature whistles. Bioacoustics 32 (3), 241–260. doi: 10.1080/09524622.2022.2106306
Struntz W. D., Kucklick J. R., Schantz M. M., Becker P. R., McFee W. E., Stolen M. K. (2014). Persistent organic pollutants in rough-toothed dolphins (Steno bredanensis) sampled during an unusual mass stranding event. Mar. Pollut. Bull. 48 (1), 164–173. doi: 10.1016/j.marpolbul.2003.09.002
Tyack P. L., Recchia C. A. (1991). A datalogger to identify vocalizing dolphins. J. Acoust. Soc. Am. 90(3), 1668–1671. doi: 10.1121/1.401908
Tyson R. B., Nowacek D. P., Miller P. J. (2007). Nonlinear phenomena in the vocalizations of North Atlantic right whales (Eubalaena glacialis) and killer whales (Orcinus orca). J. Acoust. Soc. Am. 122 (3), 1365–1373. doi: 10.1121/1.2756263
Van Parijs S. M., Corkeron P. J. (2001). Evidence for signature whistle production by a Pacific humpback dolphin, Sousa chinensis. Mar. Mammal Sci. 4, 944–949. doi: 10.1111/j.1748-7692.2001.tb01308.x
Watwood S. L., Owen E. C., Tyack P. L., Wells R. S. (2005). Signature whistle use by temporarily restrained and free-swimming bottlenose dolphins, Tursiops truncatus. Anim. Behav. 69(6), 1373–1386. doi: 10.1016/j.anbehav.2004.08.019
Wemelsfelder F., Mullan S. (2014). Applying ethological and health indicators to practical animal welfare assessment. OIE Sci. Tech. Rev. 33 (1), 111–120. doi: 10.20506/rst.33.1.2259
Keywords: Steno bredanensis, acoustics, communication, health correlates, wildlife rehabilitation
Citation: Ramos EA, Jones BL, Austin M, Eierman L, Collom KA, Melo-Santos G, Castelblanco-Martínez N, Arreola MR, Sánchez-Okrucky R and Rieucau G (2023) Signature whistle use and changes in whistle emission rate in a rehabilitated rough-toothed dolphin. Front. Mar. Sci. 10:1278299. doi: 10.3389/fmars.2023.1278299
Received: 16 August 2023; Accepted: 12 October 2023;
Published: 30 October 2023.
Edited by:
Francesco Caruso, Anton Dohrn Zoological Station Naples, ItalyReviewed by:
Frants Havmand Jensen, Woods Hole Oceanographic Institution, United StatesJavier Almunia, Loro Parque Foundation, Spain
Copyright © 2023 Ramos, Jones, Austin, Eierman, Collom, Melo-Santos, Castelblanco-Martínez, Arreola, Sánchez-Okrucky and Rieucau. This is an open-access article distributed under the terms of the Creative Commons Attribution License (CC BY). The use, distribution or reproduction in other forums is permitted, provided the original author(s) and the copyright owner(s) are credited and that the original publication in this journal is cited, in accordance with accepted academic practice. No use, distribution or reproduction is permitted which does not comply with these terms.
*Correspondence: Eric A. Ramos, ZXJpYy5hbmdlbC5yYW1vc0BnbWFpbC5jb20=