Enhancement of dieckol extraction yield from Ecklonia cava through optimization of major variables in generally recognized as safe solvent-based process
- 1Department of Chemical Engineering, Kwangwoon University, Seoul, Republic of Korea
- 2Department of Biotechnology, Sangmyung University, Seoul, Republic of Korea
- 3Department of Bio-Convergence Engineering, Dongyang Mirae University, Seoul, Republic of Korea
Ecklonia cava (EC), an edible brown macroalga abundant in intertidal areas of East Asia (Korea, Japan, and China), contains high-value bioactive compounds such as dieckol, which has antifungal, anti-inflammatory, antitumor, and antihyperlipidemic activities. However, no studies have been reported on the utilization of EC as a biorefinery feedstock, and the design of a more economical and high-yield process is required for the utilization of dieckol for the human healthcare industry. In this study, we designed a bioprocess for the high-yield recovery of dieckol from EC in a generally recognized as safe (GRAS) solvent to facilitate its application in the food and healthcare industries. Preliminary studies identified ethanol as an efficient solvent with the highest dieckol extraction yield (2.9 mg/g biomass). In order to maximize the recovery of dieckol from EC, the major extraction variables (solvent concentration, reaction temperature, and reaction time) were optimized based on statistical methods. Based on the predictive model, the numerical optimization determined that the solution with the highest dieckol content per weight of extract (62.6 vol% ethanol concentration, 54.2°C temperature, 13.2 min) was the optimal extraction condition. Under the determined conditions, the dieckol yield from EC achieved 6.4 mg dieckol/g EC (95.5% agreement with the predicted value). The designed process offers several advantages, including improving the utilization feasibility of EC, utilizing GRAS solvents with potential human applications, short extraction time (13.2 min), maximized process yield, and the highest dieckol recovery compared to previous reports.
1 Introduction
Biorefinery, defined as the production of biomaterials from biomass, is a vital concept for a circular bioeconomy (Yoo & Kim, 2021; Paliwal et al., 2022). Recently, the strategy of including marine resources as feedstock for biorefineries has gained attention to overcome the limited availability and low economic viability of lignocellulosic biomass due to limited agricultural land (Paliwal and Jutur, 2021; Vo et al., 2022; Yarkent and Oncel, 2022). In particular, marine biomass has a relatively high potential as a bioresource because it contains bioactive compounds that can be applied to related industries such as food, feed, cosmetics, and pharmaceuticals. For instance, polysaccharides from Sargassum dentifolium, a brown algal strain, can promote growth performance of fish (Abdelrhman et al., 2022), and Gammarus pulex, a freshwater amphipod, can be used as a partial replacement for fish meal due to its high protein content (Abo-Taleb et al., 2020). The extracts from Sinularia maxim, a soft coral, have antibacterial, antifungal, and anticancer activities, revealing potential pharmaceutical uses (Metwally et al., 2020).
In general, the economic feasibility of biorefineries can be improved by recovery of high-activity functional substances from biomass and application of high content carbohydrates. According to integrated or multi-step biorefineries, an extraction process is generally chosen as a first step because valuable components (e.g., carbohydrates) still remain in the residual biomass after the extraction, which can be further valorized through additional processes such as hydrothermal liquefaction and pyrolysis (Guo et al., 2019; Kim et al., 2021; López-Linares et al., 2021; de Almeida-Couto et al., 2022). Among various marine resources, macroalgae sequester carbon dioxide as primary producers, have high value as a sustainable resource due to their fast growth, and contain high content of carbohydrates and bioactive substances, thus are suitable for the described biorefinery feedstock (Choi, 2019; Lim et al., 2019; Woo et al., 2022).
Ecklonia cava (EC), an edible brown macroalga, is abundant in the subtidal areas of South Korea, Japan, and China (Lin et al., 2021). Globally, the economic values of Ecklonia (Laminariales, Phaeophyta) was reported to be about 920 kg ($28,000) per hectare per year (Eger et al., 2023). EC is used as feed for aquaculture operations (e.g., abalone) and as a raw material in the food, additive, and cosmetic industries (Choi et al., 2020). However, rejected materials generated during the harvesting and processing stages significantly impact environmental problems. In particular, most of the unmarketable seaweeds generated in the aquaculture stage are directly dumped into the sea (Kang and Kim, 2019). For more sustainable use of EC biomass, the identification and evaluation of functional materials in EC extractives have been actively studied in the research field of bioprocessing for EC (Kang et al., 2013; Hwang et al., 2021).
As one of the substances isolated from EC, dieckol is gaining attention as a high-value material with pharmacological effects (Abraham et al., 2021). Besides EC, dieckol has also been isolated from brown algae such as Eisenia bicyclis and Ecklonia stolonifera, but EC is the major feedstock for dieckol (Rajan et al., 2021). The nature of dieckol is as follows: chemical formula, C36H22O18; molecular weight, 742.52 g/mol; boiling point, 999.6°C; appearance, white to faint yellow; and stability, 2 years (Rajan et al., 2021; Jo et al., 2022).
Dieckol exhibits several bioactivities, including anti-fungal, anti-inflammatory, anti-tumorigenic, anti-hyperlipidemic, anti-platelet, anti-aging, and radioprotective activities, as reported by various studies (Lee et al., 2010a; Yoon et al., 2008; Jang et al., 2015; Lee et al., 2019; Abraham et al., 2021). Evaluating bioactivities of dieckol recovered from EC has been actively conducted, but research on process design and optimization for efficient recovery of dieckol from biomass is limited. Therefore, we hypothesized that designing and optimizing the dieckol extraction process would be a viable option as a first step for the valorization of EC. As mentioned earlier, the activities of dieckol enable its application in the food, cosmetic, and pharmaceutical industries, so the use of generally recognized as safe (GRAS)-grade solvents is recommended. Numerous studies have prepared microalgae (Spirulina platensis) and macroalgae (Chondrus crispus, Laminaria ochroleuca, and Ulva sp.) extracts using GRAS solvents such as ethanol, acetone, and ethyl acetate, considering potential human body applications (Herrero et al., 2005; Amaro et al., 2022).
The main objective of this study was to develop an eco-friendly process for efficient recovery of dieckol from EC and optimize the process variables using statistical methodology. The effects of various GRAS solvents, including distilled water (DW), ethanol, acetone, and ethyl acetate, on the extraction yield of dieckol from EC were investigated to determine the best solvent with the highest extraction efficiency. In addition, the process variables for solid-liquid extraction were optimized to maximize the dieckol yield from EC. The optimization process involved experimental design, modeling, and optimization. Finally, the potential of EC as a feedstock for dieckol production was evaluated. This study was the first attempt to maximize the yield of a GRAS solvent-based dieckol extraction to achieve economic feasibility and human safety.
2 Materials and methods
2.1 Materials
EC (Figure 1) was used as biomass in this study. EC powders were purchased from Myoungmunjungyakcho (Seoul, Republic of Korea). The obtained EC powders were freeze-dried using a freeze dryer (TFD8501, ilShinBioBase Co. Ltd., Dongducheon, Republic of Korea). Acetone (99.8% purity), ethanol (99.9% purity), and trifluoroacetic acid (TFA) were purchased from Daejung Chemicals & Metals Co., LTD. (Siheung, Republic of Korea). Ethyl acetate (99.8% purity) and potassium persulfate were purchased from Sigma-Aldrich (St. Louis, MO, USA). Methanol (99.9% purity) was purchased from J.T. Baker (New Jersey, USA). Dieckol standard was purchased from Aktin Chemical, Inc. (Chengdu, P.R. China). All chemicals and reagents used in this study were of analytical grade.
2.2 Procedure for EC extraction
A 1 g portion of freeze-dried EC was soaked in 10 mL of each of the different solvents (acetone, DW, ethanol, and ethyl acetate or ethanol-DW mixture) in a 50 mL plastic tube. To evaluate the extraction capacity of the selected pure GRAS solvents (acetone, DW, ethanol, and ethyl acetate), the reactions were performed at 30°C for 3 hr in a water bath (C-WBD3, Changshin Science, Seoul, Republic of Korea). In a preliminary study investigating the effects of ethanol concentration (mixed with DW) and extraction time on dieckol yield, reactions were carried out at 30°C for either 1 or 3 hours in the water bath. In the optimization study, the extraction reaction followed the designed conditions. Subsequently, each EC extract was centrifuged for 10 min at 12,000 × g, and the supernatant was used for dieckol detection via high-performance liquid chromatography (HPLC) analysis. All experiments were performed in triplicate, and data were expressed as mean ± standard deviation.
2.3 Experimental design and statistical optimization
Design-Expert software (version 7, Stat-Ease, Inc., USA) was used for experimental design, empirical model development, and numerical optimization in order to optimize extraction conditions for efficient dieckol extraction from EC (Rizqullah et al., 2022). Here, the experimental design was based on the central composite rotatable design (CCRD) of response surface methodology (RSM). A 5-level-3-factor CCRD was chosen to optimize extraction conditions for dieckol recovery from EC (Table 1). The levels of several variables (i.e., major extraction parameters) were set based on the results of the preliminary study mentioned above. Then, twenty extraction conditions were created by the CCRD, and the EC was extracted according to each of them. The dieckol yield (mg/g biomass) was set as the response value (i.e., dependent variable), and finally, an empirical model for predicting the dieckol yield was developed. A model equation was generated according to the following Eq. (1):

Table 1 A central composite rotatable design (CCRD) with three variables affecting dieckol recovery from Ecklonia cava.
where Y is the response value (i.e., dieckol yield [mg/g biomass]), β0 is the constant coefficient, βi, βii, and βij are the linear coefficients, quadratic coefficients, and the interaction coefficients, respectively, and Xi and Xj are the coded values of the variables (Kim et al., 2022a).
Analysis of variance (ANOVA) was carried out on the developed model to investigate statistical significance (Esfe et al., 2022). A reduced model was then created by eliminating the model terms that were not statistically significant (Mamelkina et al., 2019; Jin et al., 2022). Finally, numerical optimization was performed using the reduced model to derive the optimal extraction conditions for maximizing Y.
2.4 HPLC analysis
The dieckol concentration in the supernatant prepared according to the extraction procedure was determined using HPLC analysis (Agilent, 1260 Infinity II, Santa Clara, CA, USA). The samples were diluted 5 times and then filtered through a syringe filter (DISMIC-13HP, Advantec, Tokyo, Japan). The analysis was performed using an INNO column C18 (5 μm, 4.6 mm × 250 mm); a variable wavelength detector at 230 nm; and a mobile phase of 0.1vol% TFA in water (A) and 0.1vol% TFA in acetonitrile (B) with a flow rate of 1.0 mL/min. The gradient elution for the analysis was as follows: start, 90% A and 10% B; 40 min, 60% A and 40% B; 55 min, 90% A and 10% B. The sample injection volume was 5 μL. A standard curve (R2 = 0.9999) was prepared by analyzing various concentrations of dieckol solution and used for dieckol quantification. Based on the results of HPLC analysis, dieckol yield from biomass was calculated according to the following Eq. (2):
where solvent volume (mL) and biomass weight (g) were 10 mL and 1 g dry wt (of EC), respectively.
2.5 FTIR analysis
In order to investigate the chemical properties of the EC extracts and dieckol (standard material), Fourier-transform infrared (FTIR) analysis was performed using FTIR spectroscopy (JASCO FTIR-4600). The spectra were monitored in the range of 4000–650 cm-1.
3 Results and discussion
3.1 Extraction capacity of the selected GRAS solvents for dieckol recovery from EC
The effects of selected GRAS solvents on dieckol yield from EC were investigated in order to identify the most efficient solvent for dieckol recovery. Acetone, DW, ethanol, and ethyl acetate were tested in the experiment, and the dieckol yields were analyzed, as shown in Figure 2A. The dieckol yield was highest in the following order of solvents: ethanol (2.9 mg/g biomass), acetone (1.1 mg/g biomass), DW (0.7 mg/g biomass), and ethyl acetate (0.3 mg/g biomass). Among the selected pure GRAS solvents, ethanol demonstrated the highest extraction capacity for dieckol, and it is the most favored solvent in bioindustries due to its low toxicity (Fathordoobady et al., 2016; Ma et al., 2019). Although methanol exhibited a dieckol yield of 3.6 mg/g biomass (data not shown), it is considered a toxic solvent, leading us to choose ethanol for further process design and optimization. The dieckol yield in the ethanol extraction process can be improved by (1) preparing ethanol-based solvents with enhanced extraction capacity for dieckol, and (2) optimizing process variables. Aqueous organic solvents are generally used as efficient solvents for recovering polar phenolic compounds (Kim et al., 2022b). According to previous reports, binary solvents are more efficient in recovering phenolic compounds than mono-solvent, and water-solvent mixtures create a more polar medium, which can improve the extraction of phenolic compounds (Chaves et al., 2020; Sonar and Rathod, 2020). Therefore, we further investigated the effect of ethanol concentration on dieckol yield from EC and subsequently optimized extraction variables.
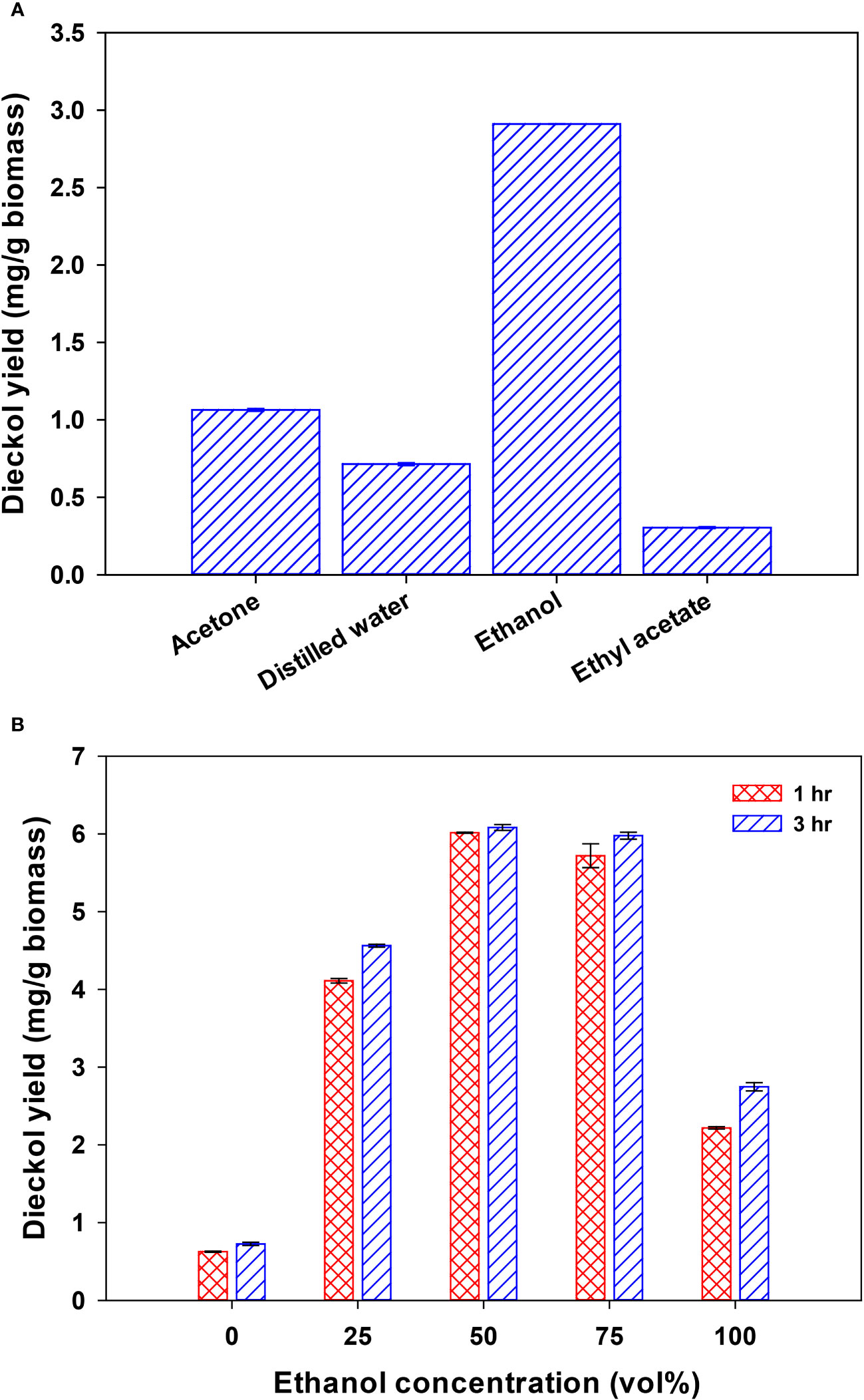
Figure 2 Dieckol extraction capacity in pure GRAS solvents (acetone, distilled water, ethanol, and ethyl acetate) (A) and ethanol-distilled water mixtures (B) from Ecklonia cava.
3.2 A preliminary study for experimental design
Figure 2B depicts the effect of ethanol concentration on dieckol recovery from EC. The dieckol yield (mg/g biomass) in extraction processes using various concentrations of ethanol were as follows (for 1 hr and 3 hr extraction, respectively): 0 vol% (0.6 and 0.7), 25 vol% (4.1 and 4.6), 50 vol% (6.0 and 6.1), 75 vol% (5.7 and 6.0), and 100 vol% (2.2 and 2.7). From 100 vol% to 50 vol% ethanol concentration, the dieckol yield gradually increased as more water was added to ethanol. This finding supports the notion that the extraction capacity of the ethanol solvent was enhanced by the addition of water, in line with earlier reports (Kim et al., 2022b; Brahmi et al., 2022). According to this preliminary study, ethanol concentration significantly affects the dieckol yield from EC, prompting us to designate “ethanol concentration” as one of the variables in the optimization study (Table 1). Preliminary investigations of dieckol stability under severe conditions (70°C, 99% ethanol) showed that about 99% of the initial dieckol was detected at 2 hr, indicating that it was stable. In contrast, about 50% of the initial dieckol was detected at 24 hr, suggesting that close to half of the component was degraded (data not shown). Therefore, extraction time is considered an important parameter in the dieckol extraction process. The parameter of extraction time was designed within a stable range based on this finding. On the other hand, extraction times exceeding 1 hr did not result in a substantial increase in dieckol yield (Figure 2B). In the extraction using 50 vol% ethanol, which demonstrated the highest dieckol extraction capacity, the increase was a mere 0.1 mg/g biomass. Consequently, we planned to investigate the effect of extraction time by setting level 0 of the CCRD as 1 hr.
3.3 Development of empirical model for predicting dieckol yield
In order to develop the empirical model for predicting dieckol yield from EC, experiments were performed according to the designed extraction conditions. The designed conditions and corresponding experimental results are listed in Table 2. Regression analysis based on the experimental data resulted in model Eq. (3) in terms of actual factors:
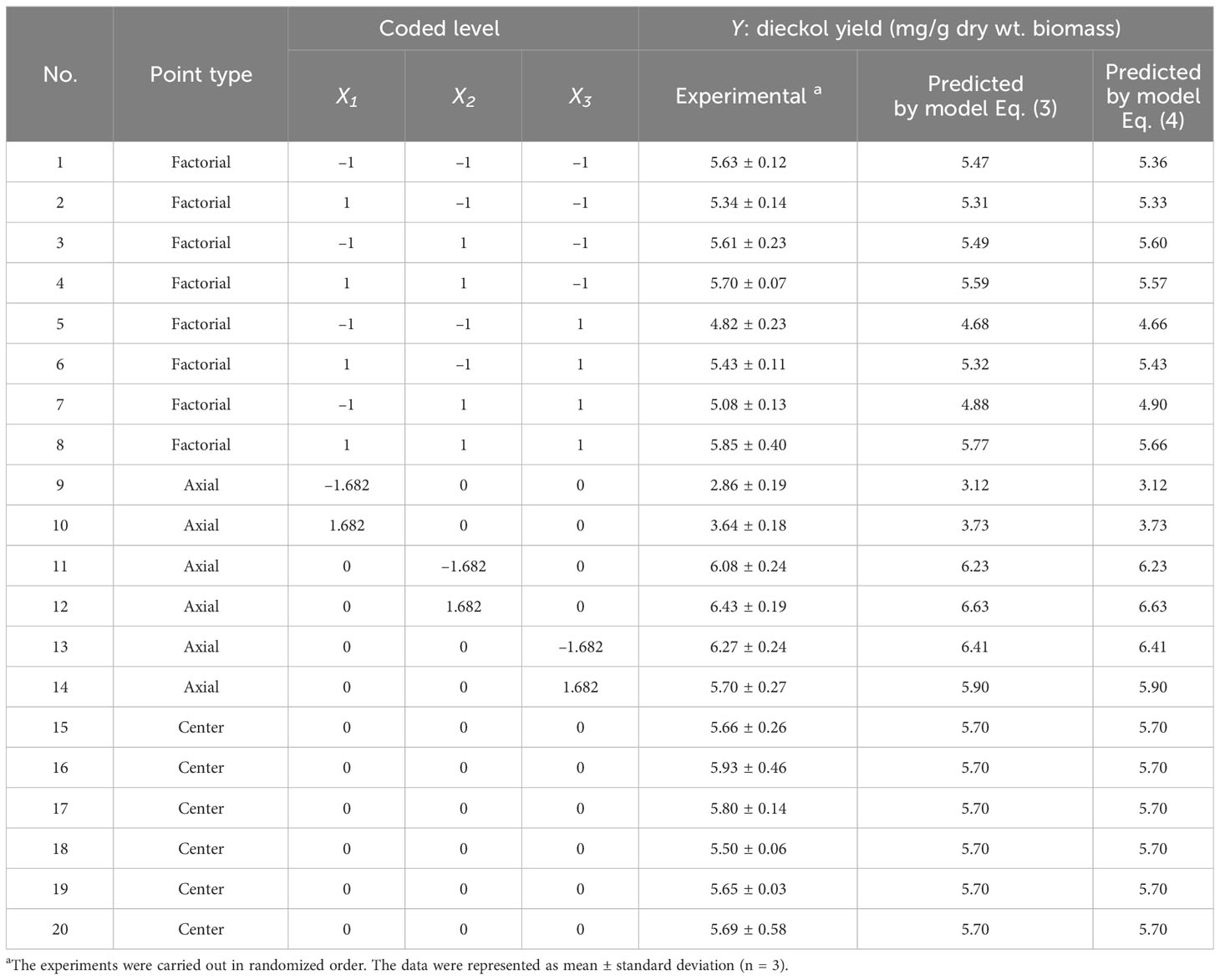
Table 2 Extraction conditions according to the CCRD and corresponding response values (dieckol yield).
where Y is the predicted dieckol yield (mg/g biomass), X1, X2, and X3 are ethanol concentration (vol%), extraction temperature (°C), and extraction time (min), respectively. The Y values predicted by Eq. (3) are listed in Table 2.
The terms with a positive sign imply the synergistic effect that increases dieckol yield, while the terms with a negative sign imply a hostile effect (Nayak & Vyas, 2019). Table 3 presents the ANOVA result for regression validation of Eq. (3). The model F-value of 33.12 implies that the model is statistically significant, with only a 0.01% chance that a model F-value this large could occur due to noise. In general, a low p-value (< 0.05) of a model term means that the corresponding term has a statistically significant effect (Lee et al., 2022a). Therefore, it was confirmed that the following model terms all have significant effects on dieckol yield from EC: the linear term of ethanol concentration (X1) and extraction time (X3), the quadratic term of ethanol concentration (X12), extraction temperature (X22), and extraction time (X32), and the interaction term of ethanol concentration-extraction time (X1X3). On the other hand, the interaction term of ethanol concentration-extraction temperature (X1X2) and extraction temperature-extraction time (X2X3) had no significant effect on the dieckol yield (Table 3).
The empirical model can be improved by excluding insignificant model terms from the initial quadratic model Eq. (3) (Mamelkina et al., 2019; Jin et al., 2022). Thus, the insignificant model terms (X1X2 and X2X3) were removed from the model, while the linear term X2 was not removed because its significant quadratic term (X22) should be included in the final model (Yu and Kim, 2018). The final empirical model was finally developed by model reduction using backward elimination, as shown in Eq. (4) in terms of actual factors:
where Y is the predicted dieckol yield (mg/g biomass), X1, X2, and X3 are ethanol concentration (vol%), extraction temperature (°C), and extraction time (min), respectively. The Y values predicted by Eq. (4) are listed in Table 2.
Table 4 shows the ANOVA result for regression validation of Eq. (4). The ANOVA result proved that the R2, adjusted R2, and predicted R2 of the model are 0.96, 0.94, and 0.85, respectively. In general, an R2 close to 1 indicates a good degree of correlation between predicted and experimental values (Lee et al., 2023a). Because the R2 always increases by adding variables, in the statistical areas, the adjusted R2 is generally used to judge the accuracy of a model (Lee et al., 2022b). Additionally, the difference between the adjusted R2 and the predicted R2, which implies the accuracy of the model in predicting the response value (Y) for the new trials, should be less than 0.2 (Haji and Rahimi, 2020; Li et al., 2022). The predicted R2 of the final model Eq. (4) is in reasonable agreement with the adjusted R2 (with a difference value of 0.09). Compared to the difference value (0.14) between adjusted R2 and predicted R2 in the initial model Eq. (3), the value of the final model was decreased, resulting in improved model accuracy, which may be a result of model reduction. The model F-value was also improved from 33.12 to 45.66, with a p-value of< 0.0001 (Table 2). In the reduced model, all linear terms and quadratic terms were found to be statistically significant. A lack of fit F-value should be insignificant to make the model fit well (Rajewski and Dobrzyńska-Inger, 2021), and in the final model Eq. (4), the lack of fit F-value of 2.49 (p-value = 0.17) indicates that the lack of fit is insignificant relative to the pure error. As shown in Figure 3A, the predicted dieckol yields match the experimental values well. Further, the adequate precision (AP) and coefficient of variation (CV) of the final model were 27.64 (> 4) and 3.69% (< 10%), respectively. Relatively high AP implies that the model is suitable for exploring the designed space, and relatively low CV indicates the accuracy and reliability of the predicted response value (Kim et al., 2022b). Overall, the ANOVA results demonstrated that the final model is more improved than the initial model, and thus, the final model is suitable for predicting dieckol yield from EC.
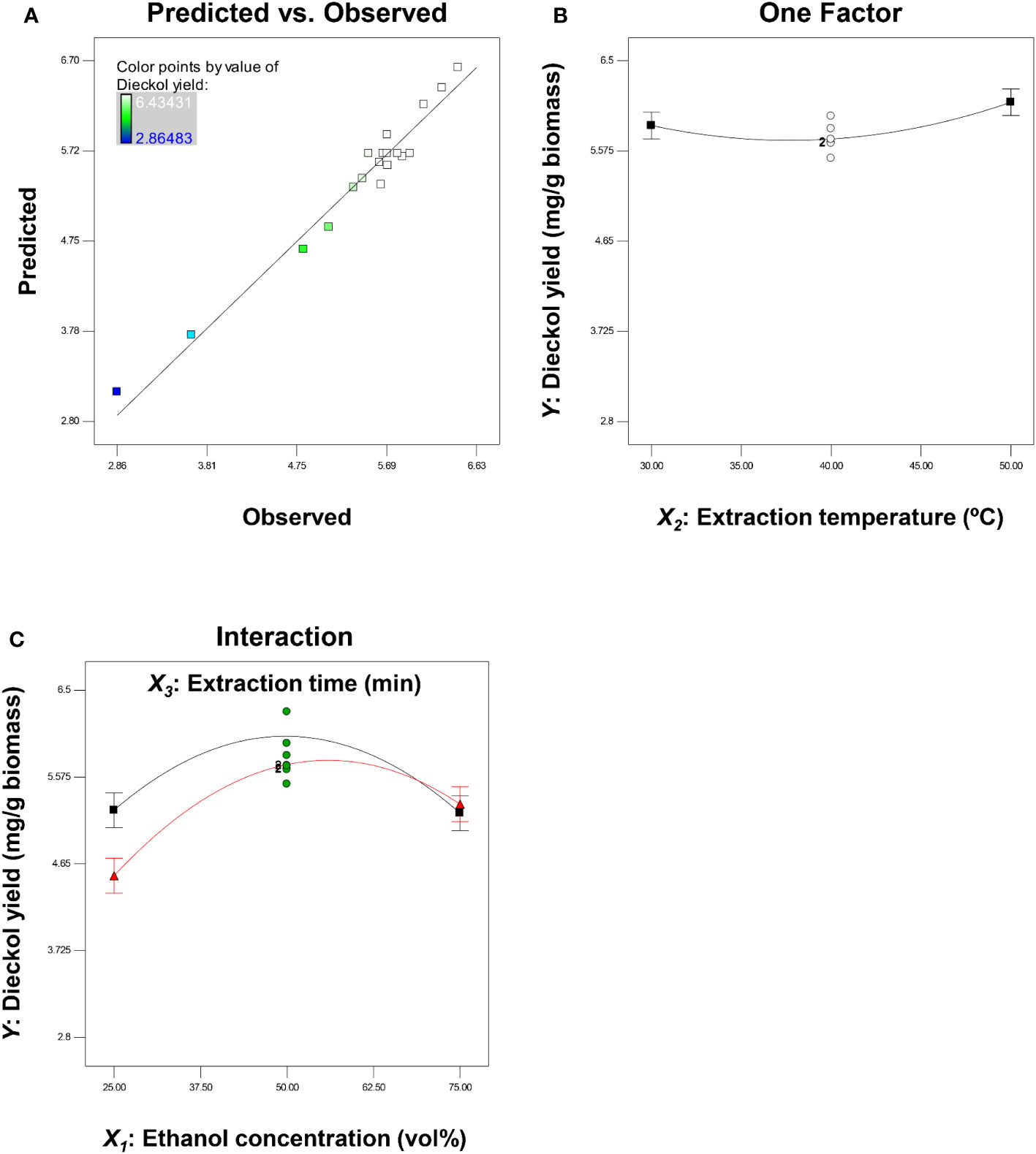
Figure 3 Plots representing the predicted dieckol yield versus observed values (A), one factor effect of extraction temperature on dieckol yield (B), and interaction effect of ethanol concentration and extraction time on dieckol yield (C).
Figures 3B, C display the effect of extraction parameters on dieckol yield. As discussed previously, the extraction temperature (X2) was not included in interaction terms, while ethanol concentration (X1) and extraction time (X3) were included in interaction terms as well as linear terms. One factor plot for extraction temperature was plotted at a fixed solvent concentration (50 vol%) and extraction time (60 min). Extraction temperatures of 30°C, 40°C, and 50°C resulted in dieckol yields of 5.8, 5.7, and 6.1 mg/g biomass, respectively, which were minor differences (Figure 3B). This finding is presumably due to the low F-value. A higher model term F-value indicates a greater effect on the response value (Balasundram et al., 2023). In this context, although extraction temperature (X2) was significant in the final model Eq. (4), its F-value of 4.79 (p-value = 0.0491) was relatively lower than other variables (Table 4). In the case of the interaction effect of ethanol concentration and extraction time (X1X3), it was plotted at a fixed extraction temperature of 40°C. Figure 3C clearly shows that there is an interaction effect of those two variables. Decreasing the extraction time from 90 min (red line in Figure 3C) to 30 min (black line in Figure 3C) did not always increase the yield of dieckol. If there is no interaction effect of ethanol concentration and extraction time, the graph must plot the tendency irrespective of any ethanol concentration. Therefore, Eq. (4), which includes this interaction as a significant term, is promising as a tool for optimizing dieckol extraction conditions.
3.4 Optimization of dieckol extraction parameters using the final model
Numerical optimization was carried out using the final model Eq. (4) to determine the optimal extraction conditions for maximizing dieckol yield from EC. The goals were set as ethanol concentration, “in range”; extraction temperature, “in range”; extraction time, “in range”; and dieckol yield, “maximize”. As a result, RSM solutions for obtaining the maximized dieckol yield (mg/g biomass) were derived, and several solutions with the desirability of 1.0 and the highest dieckol yield were sorted in Table 5. Under the optimal suggested conditions, the dieckol yield was predicted as 6.7–7.0 mg/g biomass. For the model validation, extraction processes were performed according to each solution, and then the dieckol yield in each process was calculated. As a result, the maximum error was 15.25% and the minimum error was only 3.40%. The average error was about 7.0%, which can successfully predict the dieckol yield in the EC extraction process.
The experimental results show that RSM solutions 1, 2, 4, and 5 give similar dieckol yields (Table 5). In order to finally select the best one, we further investigated the dieckol content in the EC extract obtained under each condition. The high dieckol content at the extract level is expected to provide ease of separation and purification. Figure 4 shows the dieckol content in the EC extract obtained following each solution. The contents (mg dieckol/g freeze-dried extract from EC) were as follows: 28.0, 24.8, 24.2, 27.4, and 29.4 mg/g under the solution 1, 2, 3, 4, and 5, respectively. Solution 5 is considered to be a condition that can effectively recover dieckol from EC in a shorter time (13.2 min) than other solutions. Taken together, solution 5 was finally determined as the best extraction condition for efficient dieckol recovery from EC.
3.5 Characterization of EC extract
Figure 5 shows that the HPLC chromatogram of the EC extract from solution 5. The peak of dieckol was detected at a retention time of 32.4 min, which is the same as that of standard material, implying dieckol-rich EC extract.
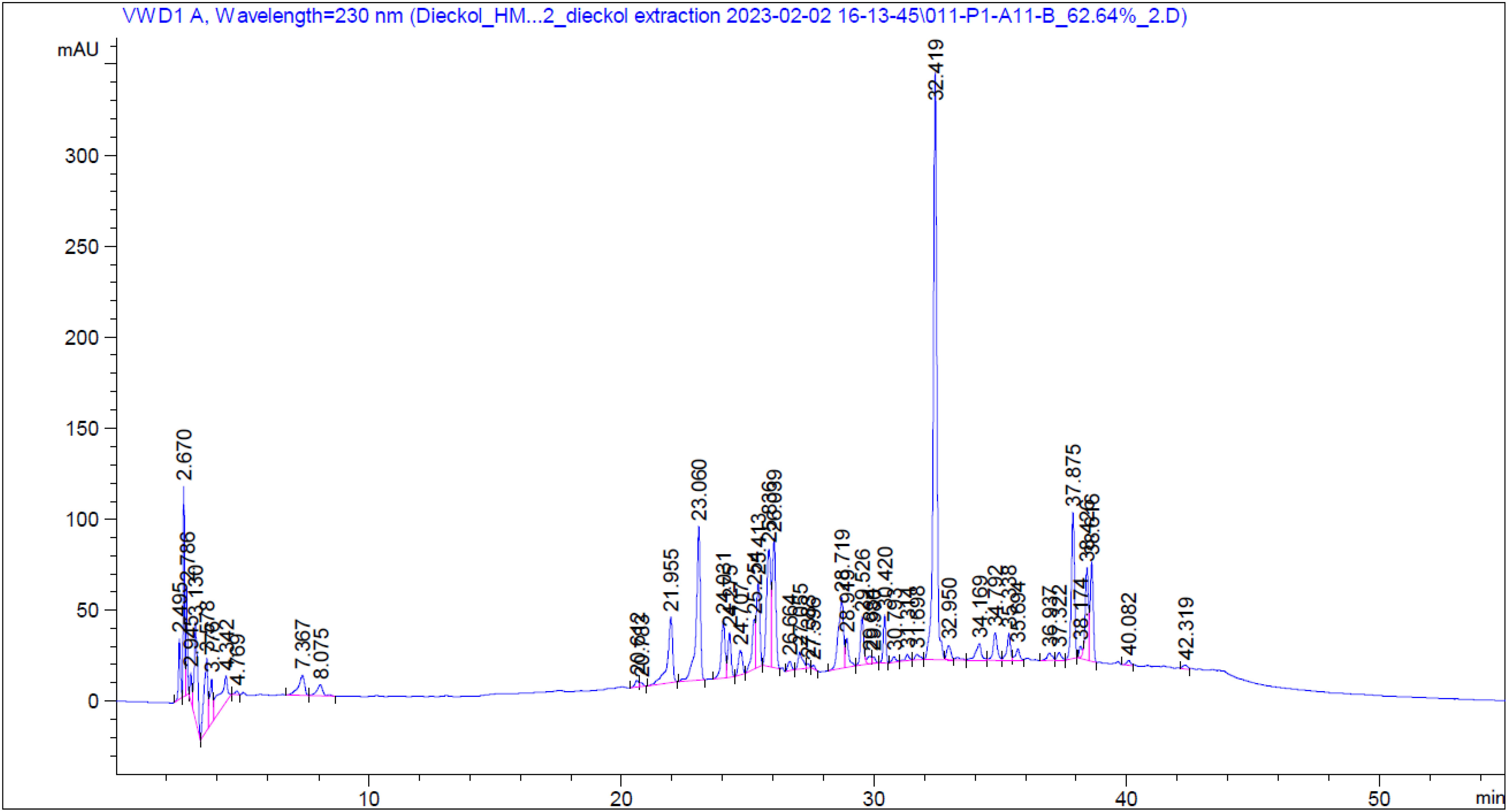
Figure 5 HPLC chromatogram of Ecklonia cava extract obtained in optimized conditions (62.6% ethanol solvent, 54.2°C temperature, and 13.2 min extraction time). Dieckol was detected at 32.4 min.
Figure 6 shows the FTIR spectra of the EC extract and dieckol standard. The broad peak at 3200 cm-1 was observed in both samples, especially strong in the standard compound. This peak is assigned to the phenolic hydroxyl band (Xing et al., 2020). Previous reports on FTIR studies of phlorotannins also observed this peak (Xiaojun et al., 1996; Yeo et al., 2012). Other significant peaks at 1607, 1482, and 1270 cm-1 were observed, which are assigned to the aromatic ring structure of phlorotannin including dieckol (Yeo et al., 2012). Overall, the FTIR pattern of the EC extract is similar to that of the dieckol standard and shows signature peaks, implying that the produced extract is dieckol-rich.
3.6 Mass balance
The potential of feedstocks can be estimated based on the mass balance. Figure 7 shows the mass balance in the extraction processes for recovering dieckol from 1 g of dried EC. The GRAS solvents recovered 0.3–2.9 mg dieckol, in which ethanol showed the best extraction capacity. It was found that dieckol extraction capacity can be enhanced by using an ethanol-DW mixture, and drastically improved dieckol yield (6.0 mg/g EC) was observed in the extraction using 50 vol% ethanol. Dieckol yield from EC could be further improved by optimizing process variables such as ethanol concentration, extraction temperature, and extraction time. The statistical model for dieckol yield suggested several solutions to maximize process yield, and finally, 6.4 mg of dieckol was recovered from 1 g EC. Previous studies have focused on the bioactivities of dieckol, and the results have scientifically proven the potential value of dieckol (Zhang et al., 2011; Lee et al., 2010a; Lee et al., 2008; Yoon et al., 2013; Yayeh et al., 2014; Lee et al., 2010b; Yoon et al., 2008; Kim et al., 2012; Jung et al., 2014). In previous studies, dieckol extracts were generally prepared for function evaluation but not extracted under the optimized processes. In earlier reports, dieckol extraction processes have been performed using methanol under room temperature for 3 hr–10 days, resulting the dieckol yields of up to 0.6 mg/g biomass. Therefore, the present study is significant in that it improved the extraction yield for the economic feasibility of the dieckol recovery process. EC is expected to be a sustainable feedstock for the production of dieckol for human use.
4 Conclusion
The current study designed and optimized a green process for dieckol recovery from EC as part of sustainable biorefinery strategies. Existing research has focused on the evaluation of bioactivities of EC extracts, but not maximizing dieckol extraction yield. Ethanol was efficient for dieckol extraction, with ethanol concentration demonstrating a significant impact on dieckol yield. Under severe conditions, dieckol was degraded by about 50% after 24 hr, although it was found to be stable up to 2 hr. Therefore, the RSM design to optimize the dieckol extraction parameters was set to less than 2 hr. RSM was successfully employed in the process optimization, leading to the determination of optimal conditions: ethanol concentration at 62.6%, extraction temperature at 54.2°C, and extraction time at 13.2 min. Under the optimal conditions, the dieckol yield was found to be 6.4 mg/g EC, which was the highest yield compared to previous reports. This study offers an economical dieckol production process from EC. In the near future, we aim to further valorize the residual biomass after EC extraction to contribute to a circular bioeconomy.
Data availability statement
The original contributions presented in the study are included in the article/supplementary material. Further inquiries can be directed to the corresponding authors.
Author contributions
HS: Conceptualization, Formal Analysis, Methodology, Writing – original draft. JL: Conceptualization, Formal Analysis, Methodology, Writing – original draft. JB: Investigation, Methodology, Visualization, Writing – original draft. KL: Investigation, Methodology, Validation, Writing – original draft. HY: Conceptualization, Project administration, Validation, Writing – review & editing. CP: Conceptualization, Funding acquisition, Project administration, Validation, Writing – review & editing.
Funding
The author(s) declare financial support was received for the research, authorship, and/or publication of this article. This research was supported by Korea Institute of Marine Science & Technology Promotion (KIMST) funded by the Ministry of Oceans and Fisheries (20220258).
Conflict of interest
The authors declare that the research was conducted in the absence of any commercial or financial relationships that could be construed as a potential conflict of interest.
Publisher’s note
All claims expressed in this article are solely those of the authors and do not necessarily represent those of their affiliated organizations, or those of the publisher, the editors and the reviewers. Any product that may be evaluated in this article, or claim that may be made by its manufacturer, is not guaranteed or endorsed by the publisher.
References
Abdelrhman A. M., Ashour M., Al-Zahaby M. A., Sharawy Z. Z., Nazmi H., Zaki M. A., et al. (2022). Effect of polysaccharides derived from brown macroalgae Sargassum dentifolium on growth performance, serum biochemical, digestive histology and enzyme activity of hybrid red tilapia. Aquacult. Rep. 25, 101212. doi: 10.1016/j.aqrep.2022.101212
Abo-Taleb H. A., Zeina A. F., Ashour M., Mabrouk M. M., Sallam A. E., El-feky M. M. M. (2020). Isolation and cultivation of the freshwater amphipod Gammarus pulex (Linnaeus 1758), with an evaluation of its chemical and nutritional content. Egypt. J. Aquat. Biol. Fish. 24, 69–82. doi: 10.21608/ejabf.2020.78232
Abraham R. E., Alghazwi M., Liang Q., Zhang W. (2021). Advances on marine-derived natural radioprotection compounds: historic development and future perspective. Mar. Life Sci. Technol. 3, 474–487. doi: 10.1007/s42995-021-00095-x
Amaro H. M., Pagels F., Tavares T. G., Costa I., Sousa-Pinto I., Guedes A. C. (2022). Antioxidant and anti-inflammatory potential of seaweed extracts as functional ingredients. Hydrobiology 1, 469–482. doi: 10.3390/hydrobiology1040028
Balasundram V., Zaman K. K., Ibrahim N., Kasmani R. M., Isha R., Hamid M. K. A., et al. (2023). Optimizing the catalytic performance of Ni-Ce/HZSM-5 catalyst for enriched C6–C8 hydrocarbons in pyrolysis oil via response surface methodology. Biomass Convers. Biorefinery 13, 8603–8613. doi: 10.1007/s13399-020-00873-0
Brahmi F., Mateos-Aparicio I., Garcia-Alonso A., Abaci N., Saoudi S., Smail-Benazzouz L., et al. (2022). Optimization of conventional extraction parameters for recovering phenolic compounds from potato (Solanum tuberosum L.) peels and their application as an antioxidant in yogurt formulation. Antioxidants 11, 1401. doi: 10.3390/antiox11071401
Chaves J. O., Souza M. C., Silva L. C., Lachos-Perez D., Torres-Mayanga P. C., MaChado A. P. F., et al. (2020). Extraction of flavonoids from natural sources using modern techniques. Front. Chem. 8. doi: 10.3389/fchem.2020.507887
Choi Y. (2019). Ecological restoration and networks realization in the East Sea Islands - Successful story of Toki and potential of Sea Lion. J. Asian Stud. 22, 257–288. doi: 10.21740/jas.2019.02.22.1.257
Choi S. K., Kang Y. H., Park S. R. (2020). Growth responses of kelp species Ecklonia cava to different temperatures and nitrogen sources. Korean J. Environ. Biol. 38, 404–415. doi: 10.11626/KJEB.2020.38.3.404
de Almeida-Couto J. M. F., Abrantes K. K. B., Stevanato N., Ramos da Silva W., Wisniewski A., da Silva C., et al. (2022). Oil recovery from defective coffee beans using pressurized fluid extraction followed by pyrolysis of the residual biomass: Sustainable process with zero waste. J. Supercrit. Fluids 180, 105432. doi: 10.1016/j.supflu.2021.105432
Eger A. M., Marzinelli E. M., Beas-Luna R., Blain C. O., Blamey L. K., Byrnes J. E. K., et al. (2023). The value of ecosystem services in global marine kelp forests. Nat. Commun. 14, 1894. doi: 10.1038/s41467-023-37385-0
Esfe M. H., Motallebi S. M., Toghraie D. (2022). Modeling and optimization of dynamic viscosity of oil-based nanofluids containing alumina particles and carbon nanotubes by response surface methodology (RSM). Korean J. Chem. Eng. 39, 2800–2809. doi: 10.1007/s11814-022-1156-6
Fathordoobady F., Mirhosseini H., Selamat J., Abd Manap M. Y. (2016). Effect of solvent type and ratio on betacyanins and antioxidant activity of extracts from Hylocereus polyrhizus flesh and peel by supercritical fluid extraction and solvent extraction. Food Chem. 202, 70–80. doi: 10.1016/j.foodchem.2016.01.121
Guo B., Yang B., Silve A., Akaberi S., Scherer D., Papachristou I., et al. (2019). Hydrothermal liquefaction of residual microalgae biomass after pulsed electric field-assisted valuables extraction. Algal Res. 6, 9018–9027. doi: 10.1016/j.algal.2019.101650
Haji A., Rahimi M. (2020). RSM optimization of wool dyeing with Berberis Thunbergii DC leaves as a new source of natural dye. J. Nat. Fibers 19, 2785–2798. doi: 10.1080/15440478.2020.1821293
Herrero M., Martín-Álvarez P. J., Senorans F. J., Cifuentes A., Ibáñez E. (2005). Optimization of accelerated solvent extraction of antioxidants from Spirulina platensis microalga. Food Chem. 93, 417–423. doi: 10.1016/j.foodchem.2004.09.037
Hwang J., Yang H. W., Lu Y. A., Je J. G., Lee H. G., Fernando K. H. N., et al. (2021). Phloroglucinol and dieckol isolated from Ecklonia cava suppress impaired diabetic angiogenesis; A study of in-vitro and in-vivo. Biomed. Pharmacother. 138, 111431. doi: 10.1016/j.biopha.2021.111431
Jang S. K., Yu J. M., Kim S. T., Kim G. H., Lee D. I., Joo S. S. (2015). An Aβ42 uptake and degradation via Rg3 requires an activation of caveolin, clathrin and Aβ-degrading enzymes in microglia. Eur. J. Pharmacol. 758, 1–10. doi: 10.1016/j.ejphar.2015.03.071
Jin X., Wang S., Shen Y. (2022). Effects of operating conditions and particle properties on mixing performance in an industrial-scale U-shape ribbon mixer. Powder Technol. 411, 117933. doi: 10.1016/j.powtec.2022.117933
Jo M.-H., Kim Y.-T., Park S. J. (2022). Dieckol inhibits autophagic flux and induces apoptotic cell death in A375 human melanoma cells via lysosomal dysfunction and mitochondrial membrane impairment. Int. J. Mol. Sci. 23, 14149. doi: 10.3390/ijms232214149
Jung H. A., Jung H. J., Jeong H. Y., Kwon H. J., Ali M. Y., Choi J. S. (2014). Phlorotannins isolated from the edible brown alga Ecklonia stolonifera exert anti-adipogenic activity on 3T3-L1 adipocytes by downregulating C/EBPα and PPARγ. Fitoterapia 92, 260–269. doi: 10.1016/j.fitote.2013.12.003
Kang J. H., Kim W. S. (2019). Study on industralization strategy for efficient reuse of seaweed by-products. J. Fish Bus. Adm. 50, 1–9. doi: 10.12939/FBA.2019.50.4.001
Kang M. C., Wijesinghe W. A. J. P., Lee S. H., Kang S. M., Ko S. C., Yang X., et al. (2013). Dieckol isolated from brown seaweed Ecklonia cava attenuates type ІІ diabetes in db/db mouse model. Food Chem. Toxicol. 53, 294–298. doi: 10.1016/j.fct.2012.12.012
Kim T. H., Ku S. K., Bae J. S. (2012). Antithrombotic and profibrinolytic activities of eckol and dieckol. J. Cell Biochem. 113, 2877–2883. doi: 10.1002/jcb.24163
Kim H. R., Lee J. H., Lee S. K., Chun Y., Yoo H. Y., Lee H. U., et al. (2021). High potential of microalgal sludge biochar for a flexible all-solid-state microsupercapacitor. J. Energy Storage 44, 103458. doi: 10.1016/j.est.2021.103458
Kim S., Son H., Pang S. Y., Yang J. J., Lee J., Lee K. H., et al. (2022b). Optimization of major extraction variables to improve recovery of anthocyanins from elderberry by response surface methodology. Processes 11, 72. doi: 10.3390/pr11010072
Kim J. H., Yeom S. H., Hwang Y. S., Kim S. H., Kim J. W. (2022a). Ultrasound-assisted extraction of polyphenols from Carthamus tinctorius seeds: Optimization of process variables. Biotechnol. Bioprocess Eng. 27, 869–878. doi: 10.1007/s12257-022-0092-y
Lee S. H., Han J. S., Heo S. J., Hwang J. Y., Jeon Y. J. (2010b). Protective effects of dieckol isolated from Ecklonia cava against high glucose-induced oxidative stress in human umbilical vein endothelial cells. Toxicol. Vitro 24, 375–381. doi: 10.1016/j.tiv.2009.11.002
Lee D. S., Kang M. S., Hwang H. J., Eom S. H., Yang J. Y., Lee M. S., et al. (2008). Synergistic effect between dieckol from Ecklonia stolonifera and β-lactams against methicillin-resistant Staphylococcus aureus. Biotechnol. Bioprocess Eng. 13, 758–764. doi: 10.1007/s12257-008-0162-9
Lee J., Kim M., Jung J., Heo J. W., Lee K. H., Kim S., et al. (2023a). Valorization of persimmon calyx, an industrial biowaste, as a potential resource for antioxidant production. Environ. Technol. Innov. 30, 103038. doi: 10.1016/j.eti.2023.103038
Lee J., Kim S., Lee K. H., Lee S. K., Chun Y., Kim S. W., et al. (2022a). Improvement of bioethanol production from waste chestnut shells via evaluation of mass balance-based pretreatment and glucose recovery process. Environ. Technol. Innov. 28, 102955. doi: 10.1016/j.eti.2022.102955
Lee K. H., Lee S. K., Lee J., Kim S., Kim S. W., Park C., et al. (2022b). Energy-efficient glucose recovery from chestnut shell by optimization of NaOH pretreatment at room temperature and application to bioethanol production. Environ. Res. 208, 112710. doi: 10.1016/j.envres.2022.112710
Lee M. H., Lee K. B., Oh S. M., Lee B. H., Chee H. Y. (2010a). Antifungal activities of dieckol isolated from the marine brown alga Ecklonia cava against Trichophyton rubrum. J. Korean Soc Appl. Biol. Chem. 53, 504–507. doi: 10.3839/jksabc.2010.076
Lee Y. J., Park J. H., Park S. A., Joo N. R., Lee B. H., Lee K. B., et al. (2019). Dieckol or phlorofucofuroeckol extracted from Ecklonia cava suppresses lipopolysaccharide-mediated human breast cancer cell migration and invasion. J. Appl. Phycol. 32, 631–640. doi: 10.1007/s10811-019-01899-2
Li L., Wan Y., Chen S., Tian W., Long W., Song J. (2022). Prediction of optimal ranges of mix ratio of self-compacting mortars (SCMs) based on response surface method (RSM). Constr. Build Mater. 319, 126043. doi: 10.1016/j.conbuildmat.2021.126043
Lim H. G., Kwak D. H., Park S., Woo S., Yang J. S., Kang C. W., et al. (2019). Vibrio sp. dhg as a platform for the biorefinery of brown macroalgae. Nat. Commun. 10, 2486. doi: 10.1038/s41467-019-10371-1
Lin L., Yang S., Xiao Z., Hong P., Sun S., Zhou C., et al. (2021). The inhibition effect of the seaweed polyphenol, 7-phloro-eckol from Ecklonia cava on alcohol-induced oxidative stress in HepG2/CYP2E1 cells. Mar. Drugs 19, 158. doi: 10.3390/md19030158
López-Linares J. C., García-Cubero M. T., Coca M., Lucas S. (2021). A biorefinery approach for the valorization of spent coffee grounds to produce antioxidant compounds and biobutanol. Biomass Bioenerg. 147, 106026. doi: 10.1016/j.biombioe.2021.106026
Ma Y., Wang Y., Li X., Hou L. X., Wei A. Z. (2019). Sensory characteristics and antioxidant activity of Zanthoxylum bungeanum Maxim. Pericarps. Chem. Biodivers. 16, e1800238. doi: 10.1002/cbdv.201800238
Mamelkina M. A., Vasilyev F., Tuunila R., Sillanpää M., Häkkinen A. (2019). Investigation of the parameters affecting the treatment of mining waters by electrocoagulation. J. Water Process Eng. 32, 100929. doi: 10.1016/j.jwpe.2019.100929
Metwally A. S., El-Naggar H. A., El-Damhougy K. A., Bashar M. A. E., Ashour M., Abo-Taleb H. A. H. (2020). GC-MS analysis of bioactive components in six different crude extracts from the Soft Coral (Sinularia maxim) collected from Ras Mohamed, Aqaba Gulf, Red Sea. Egypt. J. Aquat. Biol. Fish. 24, 425–434. doi: 10.21608/ejabf.2020.114293
Nayak M. G., Vyas A. P. (2019). Optimization of microwave-assisted biodiesel production from Papaya oil using response surface methodology. Renew. Energy 138, 18–28. doi: 10.1016/j.renene.2019.01.054
Paliwal C., Jutur P. P. (2021). Dynamic allocation of carbon flux triggered by task-specific chemicals is an effective non-gene disruptive strategy for sustainable and cost-effective algal biorefineries. Chem. Eng. J. 418, 129413. doi: 10.1016/j.cej.2021.129413
Paliwal C., Rehmanji M., Shaikh K. M., Zafar S. U., Jutur P. P. (2022). Green extraction processing of lutein from Chlorella saccharophila in water-based ionic liquids as a sustainable innovation in algal biorefineries. Algal Res. 66, 102809. doi: 10.1016/j.algal.2022.102809
Rajan D. K., Mohan K., Zhang S., Ganesan A. R. (2021). Dieckol: A brown algal phlorotannin with biological potential. Biomed. Pharmacother. 142, 111988. doi: 10.1016/j.biopha.2021.111988
Rajewski J., Dobrzyńska-Inger A. (2021). Application of response surface methodology (RSM) for the optimization of chromium (III) synergistic extraction by supported liquid membrane. Membranes 11, 854. doi: 10.3390/membranes11110854
Rizqullah H., Yang J., Lee J. W. (2022). Temperature-swing transesterification for the coproduction of biodiesel and ethyl levulinate from spent coffee grounds. Korean J. Chem. Eng. 39, 2754–2763. doi: 10.1007/s11814-022-1145-9
Sonar M. P., Rathod V. K. (2020). Microwave assisted extraction (MAE) used as a tool for rapid extraction of Marmelosin from Aegle marmelos and evaluations of total phenolic and flavonoids content, antioxidant and anti-inflammatory activity. Chem. Data Coll. 30, 100545. doi: 10.1016/j.cdc.2020.100545
Vo T. K., Kim S.-S., Kim J. (2022). Pyrolysis characteristics and quantitative kinetic model of microalgae Tetralselmis sp. Korean J. Chem. Eng. 39, 1478–1486. doi: 10.1007/s11814-022-1064-9
Woo S., Moon J. H., Sung J., Baek D., Shon Y. J., Jung G. Y. (2022). Recent advances in the utilization of brown macroalgae as feedstock for microbial biorefinery. Biotechnol. Bioprocess Eng. 27, 879–889. doi: 10.1007/s12257-022-0301-8
Xiaojun Y., Xiancui L., Chengxu Z., Xiao F. (1996). Prevention of fish oil rancidity by phlorotannins from Sargassum kjellmanianum. J. Appl. Phycol. 8, 201–203. doi: 10.1007/BF02184972
Xing Y., He X., Yang R., Zhang K., Yang S. (2020). Design of high-performance polybenzoxazines with tunable extended networks based on resveratrol and allyl functional benzoxazine. Polymers 12, 2794. doi: 10.3390/polym12122794
Yarkent Ç., Oncel S. S. (2022). Recent progress in microalgal squalene production and its cosmetic application. Biotechnol. Bioprocess Eng. 27, 295–305. doi: 10.1007/s12257-021-0355-z
Yayeh T., Im E. J., Kwon T. H., Roh S. S., Kim S., Kim J. H., et al. (2014). Hemeoxygenase 1 partly mediates the anti-inflammatory effect of dieckol in lipopolysaccharide stimulated murine macrophages. Int. Immunopharmacol. 22, 51–58. doi: 10.1016/j.intimp.2014.06.009
Yeo M., Jung W. K., Kim G. (2012). Fabrication, characterisation and biological activity of phlorotannin-conjugated PCL/β-TCP composite scaffolds for bone tissue regeneration. J. Mater. Chem. 22, 3568–3577. doi: 10.1039/C2JM14725D
Yoo H. Y., Kim S. W. (2021). The next-generation biomass for biorefining. BioResources 16, 2188–2191. doi: 10.15376/biores.16.2.2188-2191
Yoon N. Y., Kim H. R., Chung H. Y., Choi J. S. (2008). Anti-hyperlipidemic effect of an edible brown algae, Ecklonia stolonifera, and its constituents on poloxamer 407-induced hyperlipidemic and cholesterol-fed rats. Arch. Pharm. Res. 31, 1564–1571. doi: 10.1007/s12272-001-2152-8
Yoon J. S., Yadunandam A. K., Kim S. J., Woo H. C., Kim H. R., Kim G. D. (2013). Dieckol, isolated from Ecklonia stolonifera, induces apoptosis in human hepatocellular carcinoma Hep3B cells. J. Nat. Med. 67, 519–527. doi: 10.1007/s11418-012-0709-0
Yu J., Kim D. (2018). Effects of welding current and torch position parameters on minimizing the weld porosity of zinc-coated steel. Int. J. Adv. Manuf. Technol. 95, 551–567. doi: 10.1007/s00170-017-1180-6
Keywords: Ecklonia cava, dieckol, macroalgal biorefinery, green process, mass balance, optimization
Citation: Shin H, Lee J, Bae J, Lee KH, Yoo HY and Park C (2023) Enhancement of dieckol extraction yield from Ecklonia cava through optimization of major variables in generally recognized as safe solvent-based process. Front. Mar. Sci. 10:1287047. doi: 10.3389/fmars.2023.1287047
Received: 01 September 2023; Accepted: 24 November 2023;
Published: 08 December 2023.
Edited by:
El-Sayed Salama, Lanzhou University, ChinaReviewed by:
Mohamed Ashour, National Institute of Oceanography and Fisheries (NIOF), EgyptChetan Paliwal, Centrum Algatech, Czechia
Copyright © 2023 Shin, Lee, Bae, Lee, Yoo and Park. This is an open-access article distributed under the terms of the Creative Commons Attribution License (CC BY). The use, distribution or reproduction in other forums is permitted, provided the original author(s) and the copyright owner(s) are credited and that the original publication in this journal is cited, in accordance with accepted academic practice. No use, distribution or reproduction is permitted which does not comply with these terms.
*Correspondence: Chulhwan Park, chpark@kw.ac.kr; Hah Young Yoo, y2h2000@smu.ac.kr
†These authors have contributed equally to this work and share first authorship