- 1Food4Future (F4F), c/o Leibniz Institute of Vegetable and Ornamental Crops (IGZ), Grossbeeren, Germany
- 2Department Plant Quality and Food Security, Leibniz Institute of Vegetable and Ornamental Crops, Großbeeren, Germany
- 3Institute of Nutritional Science, Food Chemistry, University of Potsdam, Nuthetal, Germany
- 4Food Metabolome, Faculty of Life Science: Food, Nutrition and Health, University of Bayreuth, Kulmbach, Germany
Driven by the global need of alternative food sources, there is a rising interest in edible macroalgae (seaweeds). In order to provide fresh and healthy seafood even in inland areas, land-based, environmentally controlled seaweed farming is on the rise. Facing several issues, especially the need of suitable cultivation media increases with growing distance from the coast. In order to provide regional solutions and make the cultivation approaches more sustainable, the present study investigates the potential use of natural saline ground water (brine) for the production of the macroalga Ulva compressa in inland cultivation systems. Over a period of 88 days, macroalgal thalli were cultivated in aerated experimental units (120 L) filled with brine-based media or commercial artificial sea water. Physicochemical properties of the cultivation media and the nutritional profile of macroalgal thalli were analyzed after 52 and 88 days of cultivation. The results underline not only the feasibility to use brine in the farming of sea vegetables (e.g. overcoming the lack of seawater and reduction of freshwater use), but also clearly indicate a nutrional value for the U. compressa cultivation, resulting in algal thalli, rich in polyunsaturated fatty acids and carotenoids (e.g. lutein, β carotene). The present findings might serve as a step stone for the further development of brine-based inner land aquaculture systems.
1 Introduction
Driven by the rise of human population, reaching about 9.7 billion in 2050 (PRB, 2021), global food security is considered as one of the main challenges of this century (FAO, 2021). As the intensification of conventional food production often correlates with shrinking environmental resources, for example, reduced supply of drinking water (Shukla et al., 2019), alternative food sources, and farming approaches are urgently required to create and stabilize sustainable food systems (Soria-Lopez et al., 2023). In this context, edible macroalgae (“seaweeds”) are playing an increasingly important role as a versatile natural product with a low carbon footprint (EuropeanComission, 2019; EuropeanComission, 2020; EuropeanComission, 2021), which can contribute to achieve the UN Sustainable Development Goals (SDGs) 2 (zero hunger), 3 (health and well-being), 7 (affordable and clean energy, 11 (sustainable cities and communities), 12 (responsible consumption and production patterns), 13 (climate action) and 14 (life under water) (Bermejo et al., 2022). Next to valuable carbohydrates, like alginate (E400-405), agar (E406), carrageenan (E407 and E407a), commonly used as emulsifier and thickener (Kraan, 2012), and high protein contents (Brien et al., 2022) additional health-promoting nutritional compounds, like unsaturated fatty acids, carotenoids and minerals make seaweeds attractive as food additives and as food on their own. Consequently, the interest in these “sea vegetables” is currently rising (Fleurence, 2016; Leandro et al., 2020). Moreover, other industries, such as cosmetics or pharmaceuticals, are increasingly interested in algae products due to their recognized potential for multiple uses (Lourenço-Lopes et al., 2020; Guo et al., 2022). It is estimated that the European demand for algal biomass will increase from around 270,000 tons in 2019 to 8 million tons in 2030 (Vincent et al., 2021). Driven by industrial innovation and increased research efforts, the EU algae sector faces an opportunity to expand in the coming years, a dynamic dubbed the “algal revolution” (Doumeizel et al., 2020).
The growing demand of healthy and fresh food and the recognized high potential of macroalgae lead to an increasing interest in environmentally controlled aquaculture able to provide fresh seafood also in inner-land areas (Lage-Yusty et al., 2014; Eismann et al., 2020). Here, the widely distributed green algal genus Ulva (“sea lettuce” and “green laver”), traditionally used as food and feed, plays an important role (Kim et al., 2011; Simon et al., 2022) and is already present in many commercial food products (Mendes et al., 2022). A possible way to shorten the transportation ways and allow the consumption of fresh (not preserved) biomass of such healthy sea vegetables also in inland areas, might be the establishment of appropriate environmentally controlled cultivation systems (Suthar et al., 2019; Fricke et al., 2022; Schmitz and Kraft, 2022). Supported by new technologies, allowing an energy efficient modulation of temperature and light regime [e.g., light-emitting diode (LED) technology] and development of resource saving techniques, like recirculating systems [e.g., recirculating aquaculture system (RAS)] or multi-species approaches [e.g., integrated multitrophic aquaculture (IMTA)], environmentally controlled land-based aquaculture facilities are becoming more applicable in the urban realm (Fricke et al., 2022). Nevertheless, originating from the sea, the quality and quantity of cultivation media provide a crucial element in most cultivation facilities. Overcome by recirculation (RAS) and organism combination (IMTA), there is always the need to refill the evaporated liquids and replace the used or depleting minerals. Consequently, we could expect a rising dependency from freshwater sources and artificial salt stocks with increasing coastal distance. However, this does not have to be the case; as in addition to the coastal seawater, there are other sources of water available in inland areas. In this context natural saline ground water, known as brine provide a promising media (Allan et al., 2009). Due to its unique geology and formation of natural aquifers, the area of Brandenburg (Germany) provides naturally occurring brines (Hoth, 1997). In general, the mineral composition of these subterranean waters strongly depend on the chemical composition of the surrounding rocks and soils (Stober et al., 2014). Given its rich iron deposits (e.g., bog iron ore), fostering mining activities dating back to the Germanic era (about 500 B.C.) (Sitschik et al., 2005), the natural brines are consequently characterized by high iron concentrations (Fricke et al., 2023). These brines showed to be promising cultivation media for sea vegetables, like edible halophytes (Fitzner et al., 2021). After testing brines as cultivation media for different macroalgae in-vitro flask approaches (Fricke et al., 2023), the present study aims to prove the feasibility of brine based media for the production of Ulva compressa in an urban macroalgal cultivation system. Growing U. compressa in parallel in commercial artificial seawater and brine-based media, we investigated differences in the uptake and incorporation of different minerals, as well as in the concentrations of photosynthetic pigments (carotenoids and chlorophylls) and fatty acids, altering the nutritional profile of the harvested algal biomass. The outcome of this study underlines not only the feasibility to use brine in the cultivation for sea vegetables, but also clearly show an additive nutritional value of brine-based media for the Ulva cultivation for food production.
2 Materials and methods
2.1 Experimental organisms and cultivation conditions
The green seaweed Ulva compressa (Ulvales, Ulvophyceae) was selected as experimental organism. Formerly known under the taxonomic name “Enteromorpha” and different trivial terms, such as flat gut weed, tape weed, awo-nori, parae, tarmalg, and linu ele ele (Pereira, 2016), this cosmopolite Chlorophyta species is a common sea vegetable in Asian countries. Also, in Europe, the alga was consumed (under the term Enteromorpha sp.) to a significant degree in Europe before 15 May 1997, and is, thus, not subject to the Novel Food Regulation (EU)2015/2283. The algal cultivar originated from field material collected and isolated in November 2019 in the intertidal area of the North Sea Island of Helgoland (GPS: 54°11′N, 7°53′E; Schleswig Holstein, Germany). Until experimental start, macroalgae were cultivated in aerated flask culture (1-L glass bottles) under stable artificial conditions in a cultivation room, at 18°C water temperature and illuminated with a photoperiod of 10h day and 14h night, using LEDs, emitting 35 µmol m-² s−1 photosynthetically active radiation (PAR, 400–700 nm wavelengths).
2.2 Experimental media
For cultivation, two different alternative cultivation media were selected: (i) an artificial seawater media (TM, Tropic Marine Classic, Tropic Marin, Wartenberg, Germany), successfully used in algal cultivation at 2.4 PSU salinity, and (ii) regional brine (BW), originating from the thermal bath Bad Saarow (GPS: N 52° 17.47 E 14° 3.62; Brandenburg, Germany). Brine was treated by the ozone plant of the thermal bath Bad Saarow (Type: OZ 50/80 V2-2K; Kaufmann Umwelttechnik, Wehr, Germany) before collection and stored >80 days in clean 60-L high density polyethylene (HDPE) barrels before experimental start. No visible precipitations were observed over storage time.
2.3 Experimental design
To test the effect of the two different cultivation media on the nutritional composition of the sea vegetable, we applied both media, in three replicates in our food4future developed macroalgal cultivation system (Figure 1A). The applied cultivation system, located in a greenhouse, consisted of six HDPE barrels, filled with 120 L, continently aerated with ambient gases from the bottom, connected with a temperature control unit (T4600, Lauda, Lauda-Königshofen, Germany) set on 18°C. Light regime was provided by natural irradiance through the greenhouse roof. To monitor the variable environment of the surrounding greenhouse, four PAR light sensor (LI-190SZ Quantum, LI-COR, Homburg, Germany) equipped with a logger device (Loggito Logger, Delphin Technology AG, Bergisch Gladbach, Germany) were exposed on the top of every second cultivation unit (Figures 1A, B). After a week of media aeration in the system, U. compressa thalli were applied to the systems in June 2022. To avoid the dilution of the biomass within the system and allow settlement of the thalli, floating “seeding nests” (composed of a floating Styrofoam ring of about 30 cm diameter wrapped with polyester fleece) were constructed and thalli concentrated in their sheltered center, comprising about 1 L Media (Figures 1C, D). To provide comparable conditions for the starting stock in relation to the two different media applied, we used macroalgal stocks germinated and grown in the two media prior experiment (Figure 1E) and equally distributed them between the treatments, resulting in a start weight of 1 g fresh weight (FW) per experimental unit. To support algal growth, 1.25 mL commercial fertilizer Algal (Aqualgae, Coruña, Spain) was applied weekly directly in the seeding nests. Salinity and electric conductivity were controlled weekly, using handheld meter (conductivity meter: G1400 Serie, Greisinger, Germany and pH meter: AL10, Carl Roth, Germany) and potential loses in water level through evaporation were counterbalanced with osmosis water. In addition, water samples were taken monthly from the experimental set up and stored at −60°C until analyses. To analyze differences in the nutritional composition of the grown biomass, macroalgal samples were taken after 7 weeks (day 52, T1, July, summer) when algal biomass more than doubled, and about a month later (day 88, T2, August, summer) (Figures 1F, G). Material was removed from the units by hand picking (wearing clean gloves), aiming to remove the highest possible amount. After removal, material was weighted, lyophilized, and stored in sterile 50 mL PP centrifuge tubes (Corning, New York, USA) at −60°C until further analysis of carotenoids, chlorophylls, fatty acids, and mineral composition.
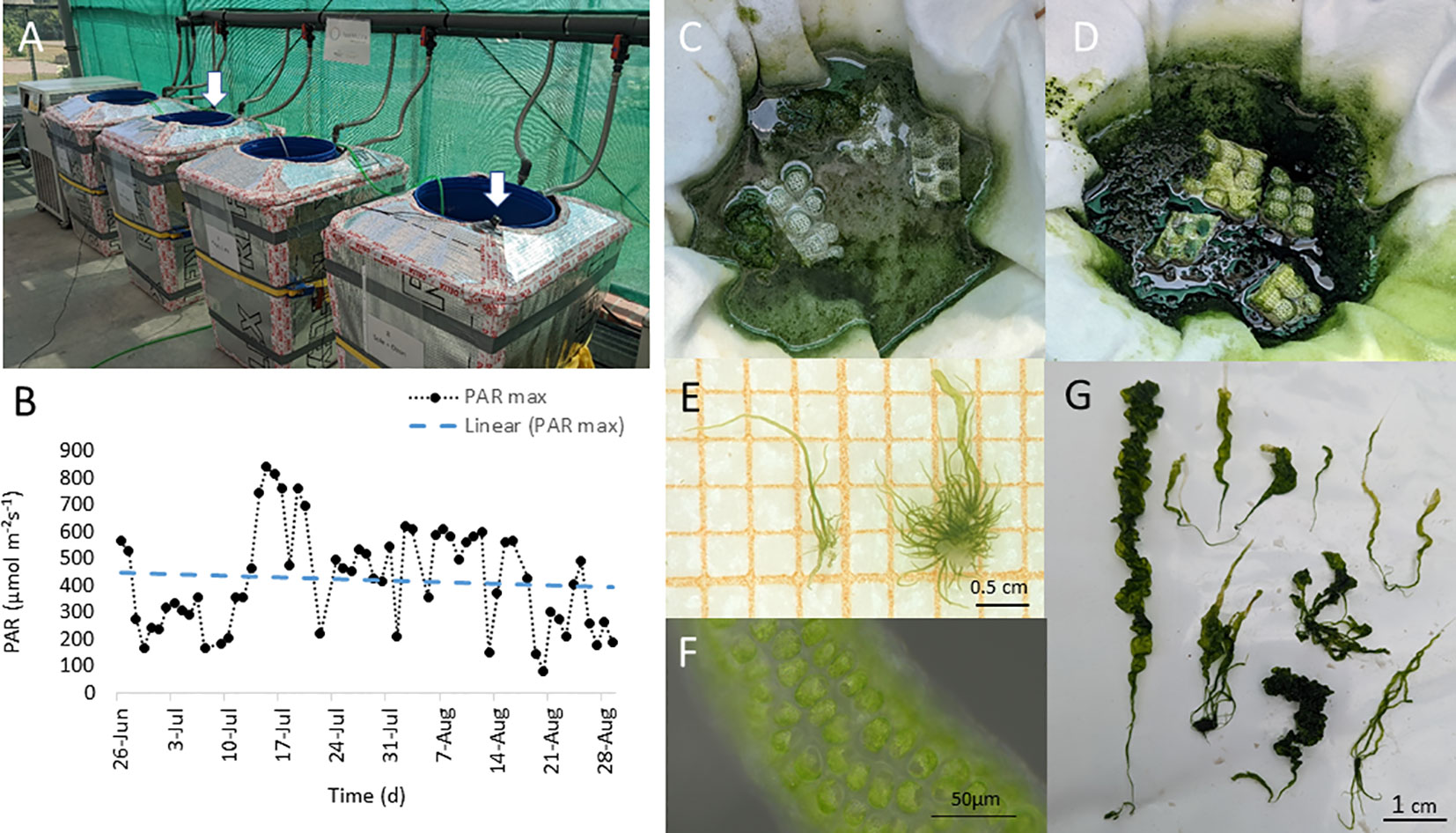
Figure 1 Ulva compressa. (A) Macroalgal cultivation system in the IGZ greenhouse facility. White arrows indicate position of photosynthetic active radiation (PAR) light loggers (LI-190SZ Quantum, LI-COR, equipped with a logger device, Loggito Logger, Delphin), exposed on the surface of the cultivation system and (B) measured daily PAR maxima over experimental time (26.6. to 29.8.2022), with decreasing linear trend indicated by dashed line (slope: m = -0.7752). (C) Experimental units, alternating filled with different media: (D) artifical seawater (Tropic Marine Classic, Tropic Marine, Germany) 2.4 PSU salinity and (C) brine-based media, orginating from the Thermal bath Bad Saarow (Brandenburg, Germany). (E–G) germlings (E), mature cells (F) and grown thalli (G) of the macroalga U. compressa grown in brine based medium.
2.4 Mineral composition of cultivation media and harvested macroalgal tissue
The anion concentrations were measured in the different media collected at days 52 and 88, using an ion chromatograph 930 Compact IC Flex equipped with a conductivity detector with suppression system (Metrohm AG, Herisau, Swisse). One hundred twenty microliter of samples was diluted up to 12 mL using ultrapure water, including 400 µL sodium bromide (0.6 mg mL−1) as an internal standard. An injection volume of 20 µL at a flow rate of 0.7 mL min−1 was used with an eluent consisting of sodium carbonate (3.2 mmol L−1) and sodium bicarbonate (1 mmol L−1) and a Metrosep a Supp5 column (Metrohm AG, Hersiau, Swisse). The final concentration of anions fluoride (F-), chloride (Cl-), sulfate ([SO4]2−), nitrate (NO3-), and phosphate (PO₄³⁻) were calculated with external calibrations using MagIC Net 3.2 software (Metrohm AG, Swisse). Salinity (PSU NaCl) was calculated from the measured chloride concentration. Cation concentrations were measured for media at Days 52 and 88 and for macroalgal biomass harvested at day 88. The cations were measured using inductively coupled plasma—optical emission spectrometry (ICP-OES) (iCAP 7400, Thermo Fisher Scientific, Henningsdorf, Germany), following the DIN EN ISO 11885 (ISO11885, 2007) lyophilized algal material homogenized to a fine powder, acidified with nitric acid and hydrogen peroxide was extracted in a microwave heated pressure digestion system (MARS XPRESS, CEM GmbH in Kamp-Lintfort, Germany), following the methodology of (VDLUFA, 2014). For the cultivation media and macroalgal biomass six macro (Na, Cl, Mg, S, K, and Ca) and six trace elements (Fe, Mn, Cu, Zn, B, and Si) were analyzed, whereas Si was only determined for the media. In addition, eight potential toxic metals (Al, Ni, Cd, Co, Cr, Pb, and Ti) and semimetals (As) and were analyzed to investigate potential contamination of the media, whereas Al showing the highest concentrations in the media was also analyzed for the algal tissue. Furthermore, to provide additional nutritional information, also phosphorous (P) concentrations, was measured in the algal tissue.
2.5 Determination of concentrations of carotenoids and chlorophylls
Carotenoid and chlorophyll concentrations were determined for U. compressa based on the methodology described by (Fricke et al., 2023). About 5 mg lyophilized algal material was homogenized to a fine powder (≤1mm grain size) and extracted 3 times in 0.5 mL methanol/tetrahydrofuran (THF)(1:1, v/v). Samples were dried under nitrogen stream, re-dissolved in 50 µL dichloromethane and 200 µL isopropanol, and filtered through PTFE-filter tubes (0.2 µm, Thermo Fischer Scientific Inc., Wilmington, NC, USA) before it was transferred to HPLC vials. The chromatographic separation of the different analytes was performed with an Agilent Technologies 1290 Infinity UHPLC (Agilent Technologies Sales and Services, GmbH & Co. KG, Waldbronn, Germany) on a C30 Carotenoid column (YMC Co. Ltd., Kyoto, Japan; 100 mm × 2.1 mm, 3 µm) at 15°C. The analytes were detected using DAD–detector (UV/VIS spectra) and mass spectrometry (HR-MS data), using a coupled Agilent Technologies 6230 ToF LC/MS. Identification and quantification was achieved by external calibration with carotenoid standards of all-trans-isomers from α-carotene, β-carotene, and lutein, as well as chlorophyll a and b standards at the wavelength of 450 nm. Data analyses were performed using the software package Mass Hunter (Agilent, Technologies, Santa Clara, USA). Total chlorophyll and total carotenoid content were calculated based on the summed concentrations of single analytes.
2.6 Determination of concentrations in fatty acids
Fatty acids were analyzed as corresponding methyl esters for U. compressa following the protocol of (Harbart et al., 2022), using 5 mg lyophilized algal material homogenized to a fine powder, extracted and derivatized using 1,000-µL methanolic hydrochlorid acid (HCl/MeOH (1:3, v/v + 5% 2,2-Dimethoxypropane) with the addition of 250 µL internal standard (0.02 mg mL−1 margaric acid in THF/MeOH (1:1, v/v). After an hour incubation at 300 rpm on a thermos shaker, under nitrogen atmosphere and temperature of 80°C samples were cooled down to room temperature and 750 µL n-hexane and 1,000 µL of a saturated sodium chloride solution were added. Samples were vortexed and centrifuged at 4500 rpm for 5 min. Five hundred microliter of the upper hexane phase was collected and filtrated over a Pasteur pipette column, filled with sodium sulfate. Two hundred microliter of the eluent was transferred in 1.5 mL glass vials with inserts and put under nitrogen atmosphere. Fatty acid methyl esters (FAMES) were analyzed with an Agilent 8890 GC System equipped with a J&W DB-23 GC Column, 5977B GC/MSD (Agilent Technologies, Santa Clara, USA) at a flow rate of 1.2 mL min−1 and helium as carrier gas (230°C, 76.7 kPa 15.3 mL min−1). The following temperature program was used for elution: 80°C for 2 min, 80°C–120°C with 5°C min−1, 120°C–220°C with 2°C min−1, held at 220°C for 5 min. Compounds were detected with an Agilent 5973 mass selective detector. The source temperature was set to 230°C, the quadrupole temperature was set to 150°C and the voltage was set to 953 V. Analysis was performed in scan mode using a mass range between m/z 60 and 400. Fatty acids were identified as their methyl esters by comparing retention time and mass spectra with those of standards (FAME, Merck KGaA, Darmstadt, Germany). For quantification the internal standard magarinic acid (≥ 98 %) was used and response factors of the fatty acids of interest were determined. The data were analyzed with the software Quant-My-Way v10.2 (Mass Hunter, Agilent Technologies, Santa Clara, USA). For comparison, fatty acids were grouped according their saturation state in saturated fatty acids (SFAs), monounsaturated fatty acids (MUFAs) and polyunsaturated fatty acids (PUFAs). In addition to evaluate the nutritional value, we calculated the ω-6/ω-3 ratio based on (Simopoulos, 2010).
2.7 Data evaluation and statistics
To evaluate the measured mineral data in terms of food grade conditions, we compared the mineral media concentrations with limit concentrations of the German drinking water ordinance from 2001 last changed 22.9.2021 (Trinkw, 2001). Likewise, to evaluate the potential nutritive value of the cultivated algal biomass we compared the data with records from field derived data (Circuncisao et al., 2018) and nutritive profile from lettuce (Lactuca sativa) (Souci et al., 2015) and related the values toward recommended dietary allowances for adults (RDA > 19 years old) and tolerable upper intake level (UL) for adults according to standards of the European Food Safety Authority (EFSA, 2018). To analyze the potential impact of cultivation media composition on the growing algal biomass, we compared mineral composition measured in cultivation media after 52 days with the mineral composition measured in the algal thalli harvested after 88 days. Differences between treatments were statistically evaluated using analyses of variance (ANOVAs; significant levels of p < 0.05) or corresponding nonparametric Kruskall Wallis test, in cases homogeneity of data was not reached after log (n + 1) transformation, confirmed by Cochran homogeneity test. Subsequent pairwise comparisons were conducted for significant differences using Tukey post-hoc test. Data were analyzed using Statistica v10 software (Statsoft, Tuls, USA) and figures created using GraphPrism version 5.03 (Graph Pad software, Boston, USA). In addition to analyses of potential differences between mineral compositions in media and algal thalli, data were analyzed with permutional multivariate ANOVA (PERMANOVA) based on Euclidean distances, log transformed prior analyses to down weight the impact of components with high concentrations. Similarity percentage (SIMPER) were conducted to identify the specific differences, which were visualized using a principal coordinate analysis (PCO) and subsequent SIMPROF analyses. In addition, differences in media mineral composition were related to Ulva thallus composition based on Pearson correlation and projected as vectors. All these non-parametric analyses data were conducted with the PRIMER v7 + PERMOVA software package (Primer-e, Auckland, NZ).
3 Results
3.1 Cultivation conditions
The cultivation conditions in the greenhouse cabinet, showed strong environmental alterations over the experimental time. Incident natural PAR intensities varied according to daily variations, peaking at day 12 with a maximum of 839 µmol m−²s−1and slightly decreased in PAR intensity over the following experimental time (Figure 1B, Supplementary Figure 1). Despite ambient greenhouse temperature showed strong daily and seasonal variations, reaching temperatures >40°C, water temperature was kept stable between 18°C and 19°C in the cultivation system, providing a temperature similar to the cultivation room (18°C) throughout the experiment.
3.2 Differences in media mineral composition over cultivation period
Mineral composition not only strongly differed between the two media but was also significantly altered during the cultivation process (Tables 1 and 2, Supplementary Table 1). Concerning potential contamination, all measured potential element concentrations laid below the thresholds provided by the German Drinkwater Ordinance (TrinkwV, Table 1), whereas some of the nutritive elements like chloride, sulfate and corresponding sulfur, fluoride, sodium, boron, and manganese exceeded these levels. Comparing the elementary composition, the media differed significantly from each other throughout the cultivation period (Table 2), mainly due to elevated sulfur, phosphorous and magnesium concentrations in the artificial seawater (TM), whereas the brine-based medium (BW) exceeded the TM especially in its silicon concentrations of about 38 times (Table 1). Except for the macroelements florid and sodium, significant decreases in concentrations were measured for most of the different elements over cultivation time (Tables 1, 2). Whereas a decrease in minerals was only visible for the medium which showed comparable higher concentrations, thus phosphorous, manganese and zinc decreased only in TM, whereas calcium and silicon lowered their concentration only in BW (Table 1). These different depletions lead to significant time wise shifts in the media composition (Table 2).
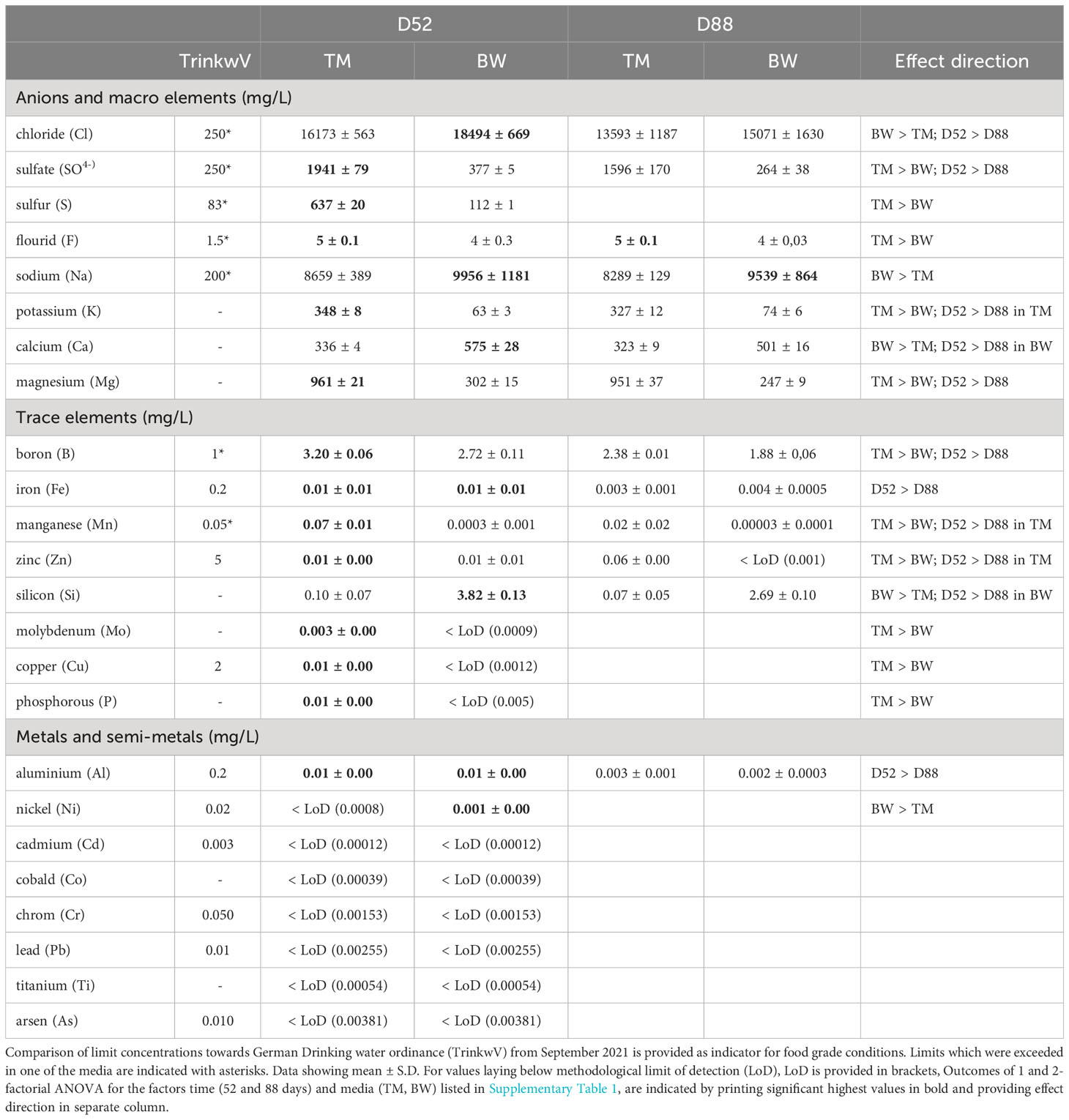
Table 1 Mineral composition of different cultivation media (TM - artificial seawater and BW- brine-based medium), after 52 and 88 days of cultivation with the macroalga Ulva compressa in comparison to the German Drinking water ordinance (TrinkwV).
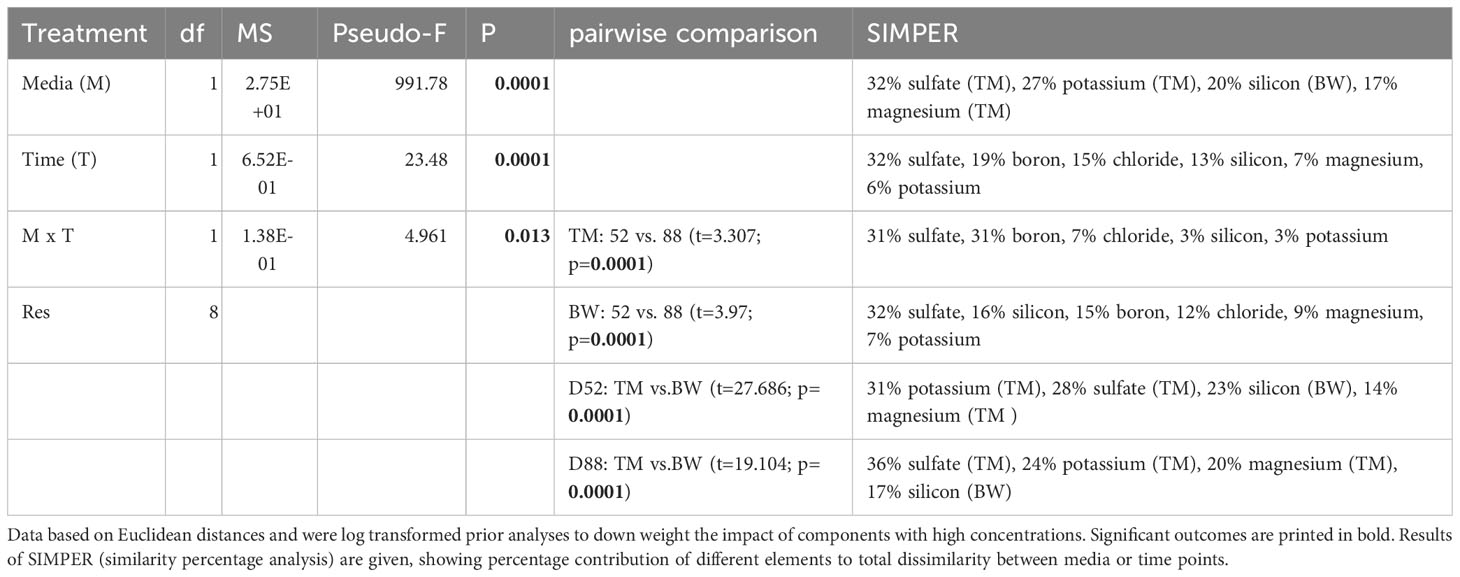
Table 2 Outcome of 2-way PERMANOVA and subsequent SIMPER analyses, investigating the effect of the factors media (TM-artificial seawater and BW- brine-based medium) and time (52, 88 days) on the elementary composition of exposed media during cultivation with the macroalga Ulva compressa.
3.3 Nutritional composition of cultivated macroalgal biomass
3.3.1 Macroalgal growth
After a period of 7 weeks, biomass of the cultivated macroalgae more than doubled and an average of 2.3 g fresh weight (FW) was harvested from the cultivation units of the different experimental treatments (TM = 2.3 ± 0.7 g FW and BW = 2.3 ± 0.4 g FW). After additional 4 weeks, the average harvested amount doubled (TM = 4.7 ± 1.0 g FW) and was slightly but not significantly higher in the BW (9.4 ± 3.9 g FW) (Figures 1C, D). Overall algal thalli grown in the BW show normal growth and morphology (Figures 1E–G).
3.3.2 Mineral composition of macroalgal thalli
For the minerals, the most striking difference was observed in the calcium thalli concentration, reaching about 1.2 g 100 g−1 FW in the BW, exceeding the TM by 9.8 times (Table 3). Considering its nutritional value, the brine-treated Ulva exceeded the calcium content of lettuce (Lactuca sativa) of about 57 times and covered over 100% of the recommended dietary allowance for adults (RDA), without exceeding the tolerable upper intake level (UL) of 2.5 g 100 g−1 FW, defined by EFSA (Table 3). Likewise, two times higher boron concentrations of 1.79 mg 100 g−1 FW in the BW exceeded L. sativa concentrations and covered the required RDA by 100%. In addition, also other minerals, in the U. compressa tissue strongly differed, related to the different media applied. Thus, BW grown thalli showed significant lower magnesium, manganese, copper, phosphorous, sulfur concentrations compared to TM exposed thalli (Supplementary Table S2 and Table 3). Overall, apart from phosphorous, which only reached about 7 mg 100 g−1FW, concentrations of the macro- and microminerals measured in the BW treated U. compressa exceeded the recorded values of L. sativa and thus better supported the RDA, without exceeding the recommended upper intake levels (UL) defined by EFSA (Table 3). Comparing the mineral composition of the BW grown U. compressa biomass with data taken from field records (Circuncisao et al., 2018) (Supplementary Table S3), strong differences were found between the cultivated and field-derived biomass. Thus, we calculated about 3–6 times higher concentrations in sodium and about 4–44 times higher concentrations in calcium, whereas in terms of iron and magnesium, about 1.6 and 2 times lower concentrations were reached in the brine-based cultivation.
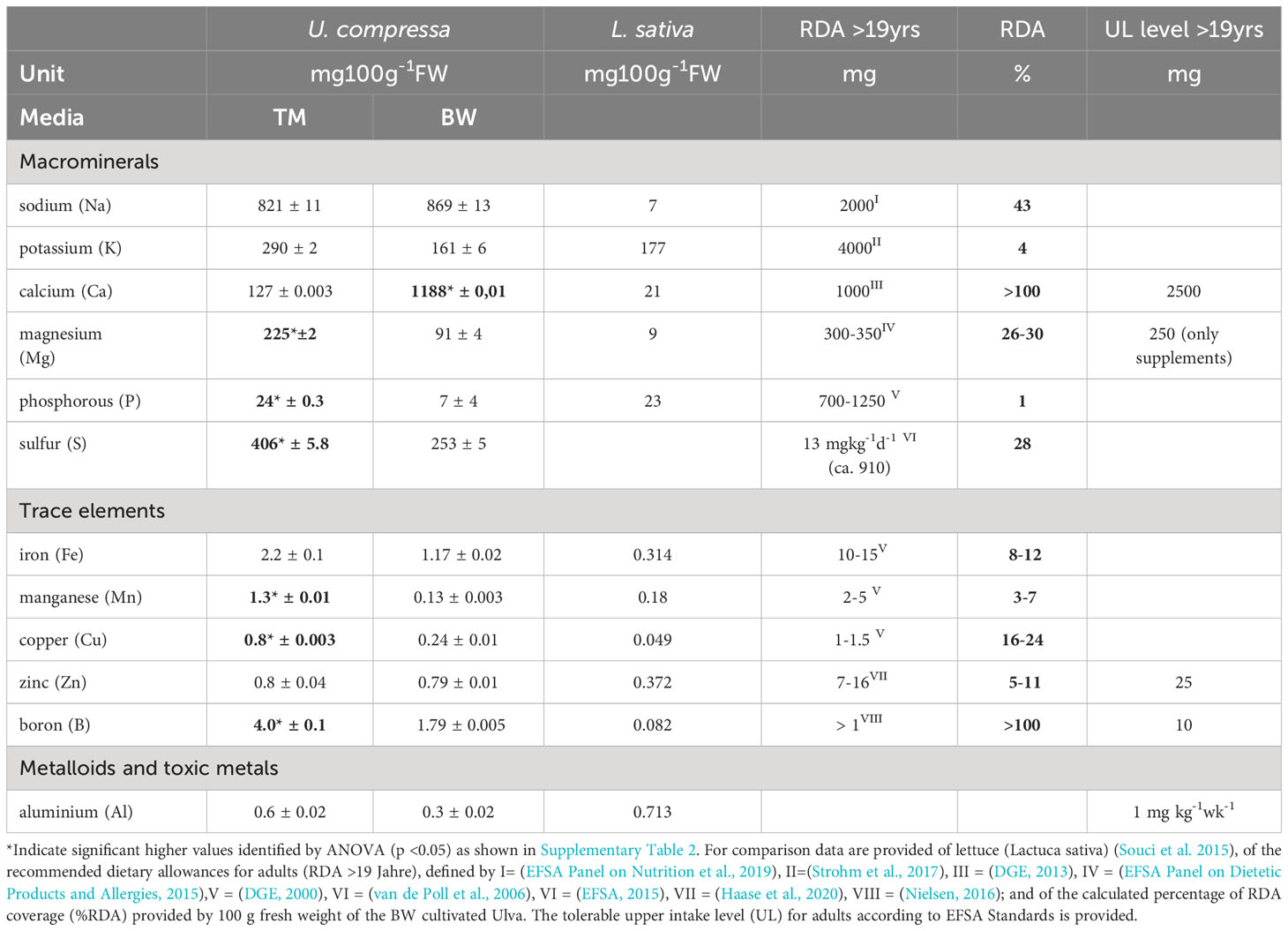
Table 3 Mineral composition of Ulva compressa cultivated for 88 days in artificial seawater (TM) and brine-based medium (BW) in comparison to lettuce (Lactuca sativa) and related to different dietary allowance (RDA) and tolerable upper intake levels (UL).
3.3.3 Composition of photosynthetic active pigments
Likewise, the mineral composition, strong differences were also found in the photosynthetic pigments. Concerning the chlorophylls 6 times higher total concentrations were found in the algal thalli cultivated in the BW compared to the TM, reaching average concentrations of 8.53 ± 0.71 µg mg−1 dry mass (59.88 ± 10.17 mg 100 g−1 FW) after 52 days of cultivation (Figure 2A and Supplementary Table S4). The chlorophyll composition changed over time showing differences between the applied. In the BW chlorophyll b, chlorophyll b′ and chlorophyll a′ decreased significantly over time (Table 4). In contrast no changes in chlorophyll b or b′ were observed in the TM, but alterations in the chlorophyll a/chlorophyll a′ pool. Thus, the relative concentrations of chlorophyll a′ decreased, while chlorophyll a increased over time. Corresponding to the chlorophylls also the carotenoids showed strong differences between cultivation media and over time. Highest concentrations of all measured carotenoids were found in the BW media, reaching average concentrations of 0.86 ± 0.1 µg mg−1 dry mass (6.01 ± 1.15 mg 100 g−1 FW) after 52 days and exceeding the TM cultivated concentration of about 150 times (Figure 2B and Supplementary Table S5). Over cultivation time concentrations of total and individual carotenoids decreased in the BW media (Table 4), whereas total carotenoid concentration in the TM media increased with time, due to significant increase in β-carotene and 9-cis-β-carotene. These patterns lowered the difference between the media, which showed still a 6 times higher total carotenoid concentration in the BW after 88 days of cultivation.
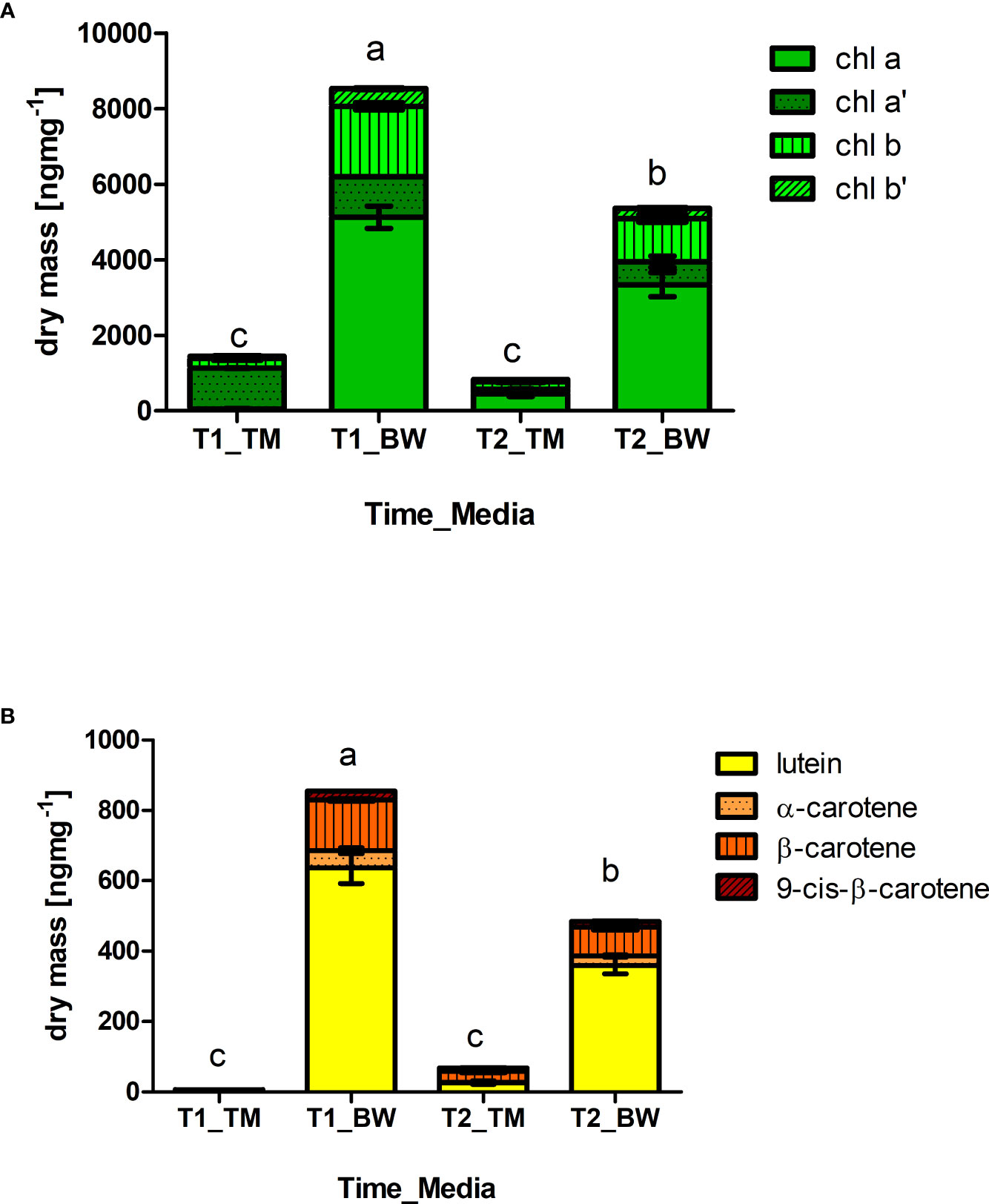
Figure 2 Differences in photosynthetic active pigments. Staggered columns showed concentrations of (A) chlorophylls (Chla, chla′, Chlb, Chlb′) and (B) carotenoids (lutein, α-carotene, β-carotene, 9-cis- β-carotene) measured in the thalli of Ulva compressa exposed to different cultivation media (TM: artificial seawater and BW: brine-based medium) over a period of 52 (T1) and 88 (T2) days. Data showing mean ± SE (n = 3).
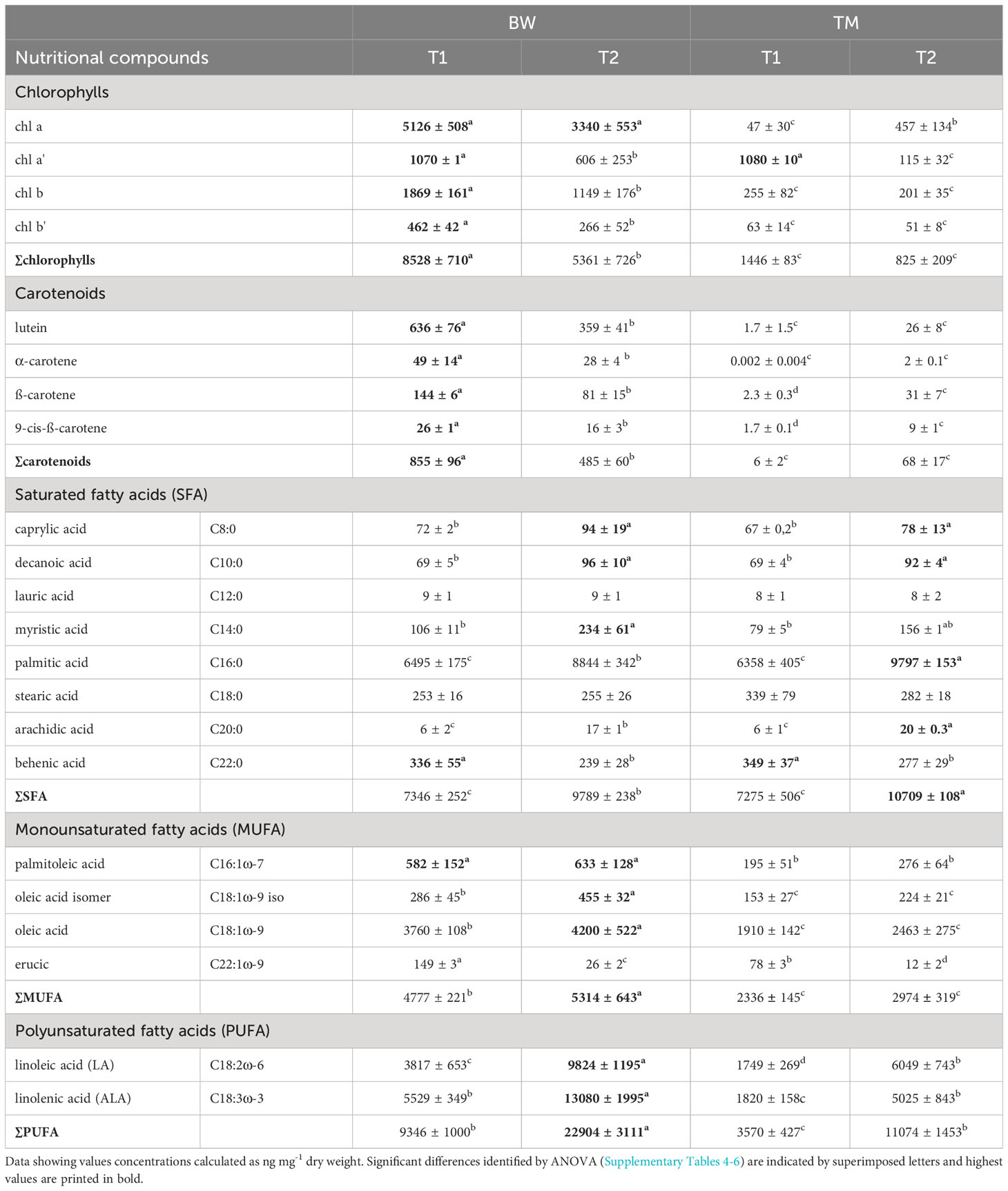
Table 4 Nutritional profile of Ulva compressa cultivated in brine water-based media (BW) and artificial seawater (TM) over a period of T1 = 52 and T2 = 88 days.
3.3.4 Fatty acids
Also, in the fatty acids, profound differences were identified between media and sampling times. For the saturated fatty acids (SFA), we found strong interactions between the applied media and sampling times (Supplementary Table S6). In both media, the SFA concentrations significantly increased over time (Table 4) but differed between the media only at the experimental end after 88 days (Figure 3A), when thalli in TM were about 1.1 times higher, reaching values of 10.7 ± 0.1 µg mg−1 dry mass. This pattern was mainly supported by palmitic acid (C16:0), which clearly dominated the SFA, and arachidic acid (C20:0). In contrast, myristic acid (C14:0) showed significant higher concentration in the BW exposed thalli (Supplementary Table S6). For the monounsaturated fatty acids (MUFA), mainly composed by oleic acid (C18:1ω-9), significant higher values were measured in the BW media exposed thalli in all MUFAs (Supplementary Table S6 and Figure 3B). Additionally, the total values significantly increased over time (Supplementary Table S6). About 1.9 times higher average MUFA values of 5.05 ± 0.52 µg mg−1 dry mass were measured in the BW media throughout the experiment. This pattern was supported by oleic acid (C18:1ω-9) and its isomer (C18:1ω-9 iso), and but not by erucic acid (C22:1ω-9) which decreased in concentration over time (Table 4). No time wise alterations were observed in the concentrations for palmitoleic acid (C16:1). For the polyunsaturated fatty acids (PUFA) we observed a strong interaction between the applied media and the sampling times (Supplementary Table S6). Under both treatments the total PUFA, composed by linolenic acid (ALA, C18:3ω-3) and linoleic acid (LA; C18:2ω-6), concentration increased with time (Table 4) and was at both sampling events significantly higher in the BW media grown thalli. After 88 days PUFA values peaked in the brine-based media with 22.9 ± 3.1 µg mg−1 dry mass, 2.1 times higher than in the TM media (Figure 3C). Also, ALA and LA followed this pattern, whereas for LA no interaction was found (Supplementary Table S6). Concerning the LA/ALA ratio (ω-6/ω-3), we measured a significant increase over cultivation time (ANOVA: F = 39.05, p < 0.000) and significant lower values in the BW exposed thalli (ANOVA: F = 7.81, p = 0.02), with average values ranging from 0.69 ± 0.08 to 0.75 ± 0.05 (Supplementary Table S6).
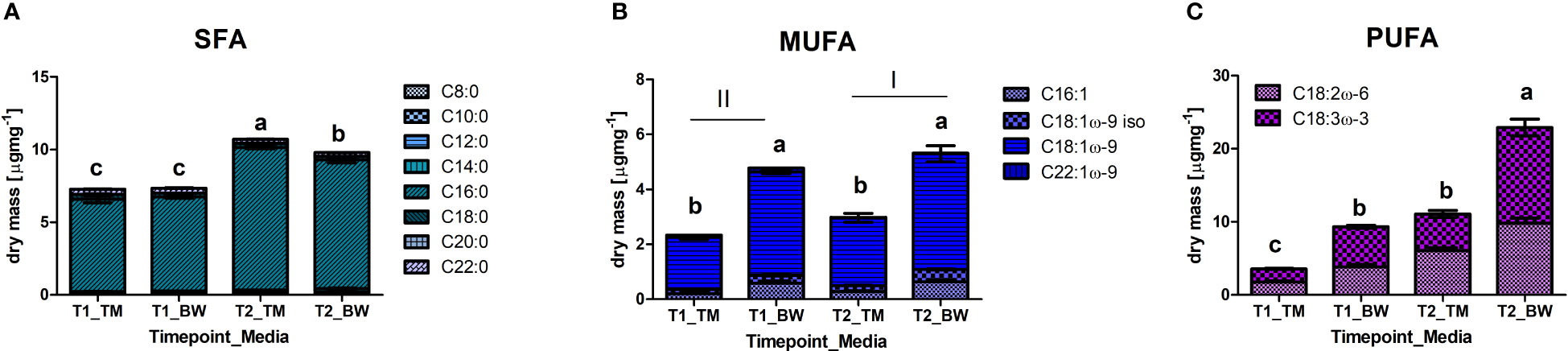
Figure 3 Differences in fatty acid methyl esters (FAME), grouped according to their saturation state in (A) saturated fatty acids (SFA), (B) monounsaturated fatty acids (MUFA) and (C) polyunsaturated fatty acids (PUFA), measured in the thalli of Ulva compressa exposed to different cultivation media (TM - artificial seawater and BW - brine-based medium) over a period of 52 (T1) and 88 (T2) days. Data showing mean ± SE (n = 3). Small letters and roman numbers indicate significant differences between treatments, analysed by ANOVA presented in Supplementary Tables 4–6.
3.3.5 Mineral media concentrations and nutritional Ulva thalli composition
Investigating the potential role of different minerals to the nutritional composition of U. compressa, we related the composition of cultivation media after 52 days (T1) to the mineral concentrations measured in the Ulva thalli harvested after 88 days (T2). The results showed a strong negative correlation (r < −0.5) for calcium, silicon, chloride and nickel and a strong positive (>0.5) correlation for molybdenum and copper, all correlated (Pearson correlation) to the principal coordination axis (PCO1, 97.2% explained variation) (Figure 4A and Supplementary Table S7). From this minerals Ca, showing high concentration in the brine-based media contributed to the main difference (93.8%) between the different treated thalli (Figure 4B and Table 5).
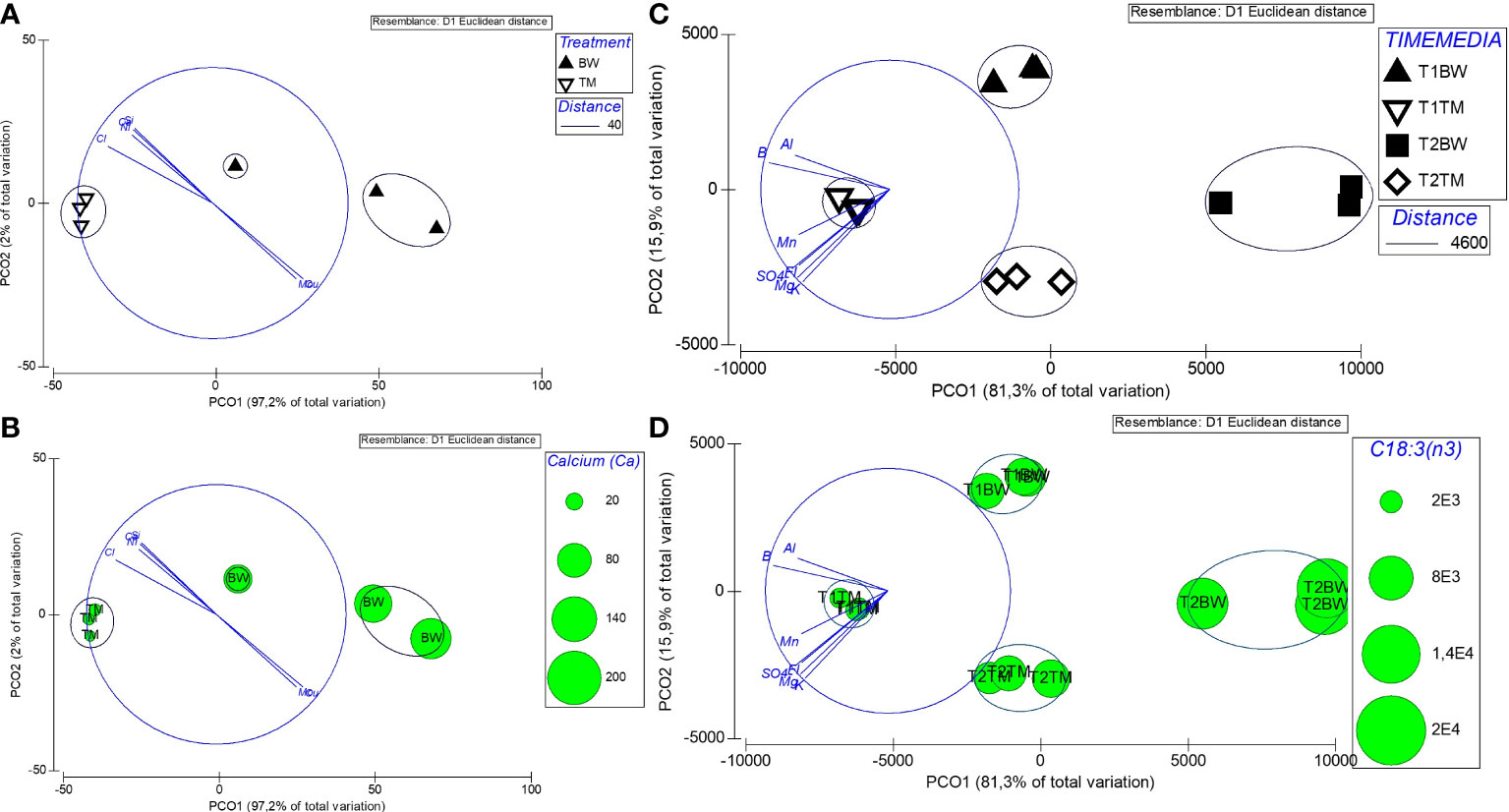
Figure 4 Differences in media and Ulva compressa thallus composition. (A) Principal coordinates analysis (PCO), showing differences in mineral composition of U. compressa exposed over 88 days (T2) to different cultivation media [artificial seawater (TM)—white; brine-based medium (BW)—black); overlayed with SIMPROF cluster analysis defining differences between groups. (B) PCO as shown before with overlaid Ca concentration extracted from U. compressa biomass presented as bubbles. (C) PCO showing differences in nutritional composition (carotenoids, chlorophylls, fatty acids) of U. compressa exposed to BW and TM after T1 and T2; (D) PCO as shown in (C) with overlaid chlorophyll concentrations extracted from U. compressa biomass presented as bubbles. Overlaying vectors illustrate mineral compounds of the media measured at T1 (C, D) and T1 and T2 (C, D) strong correlated (r > 0.5, Pearson correlation) with the ordination axis PCO1. Vectors indicate the strength (by length) and direction of different media compound to the gradients reflected by each ordination axis.
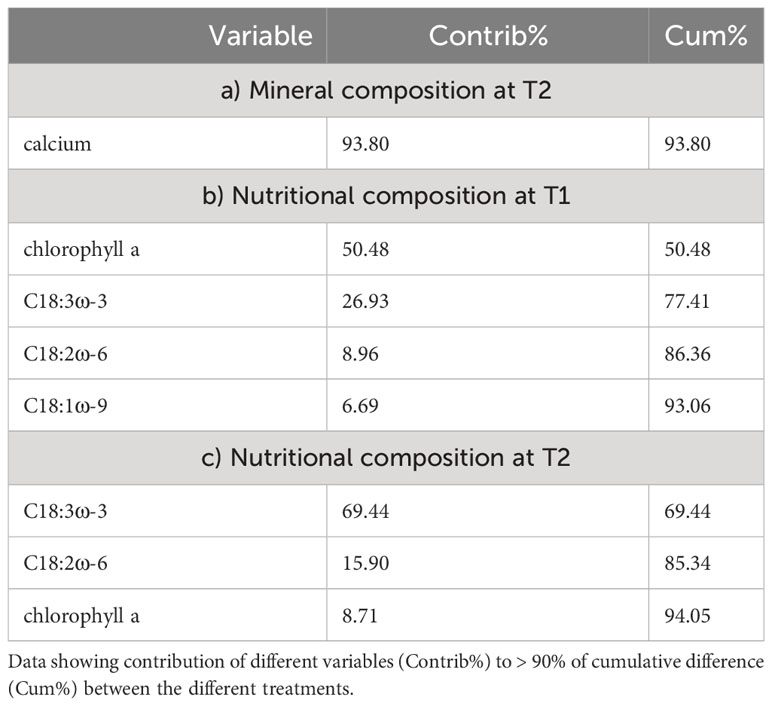
Table 5 Differences in composition of Ulva compressa exposed to different cultivation media (BW, TM) based on similarity percentage (SIMPER).
Likewise, relating the mineral composition of different cultivation media to the nutritional composition (carotenoids, chlorophylls, and fatty acids) of the different treated U. compressa, we found strong negative correlations (n > 0.5, Pearson correlation) for potassium, magnesium, manganese, boron, aluminum, sulfate and florid to the principal coordination axis (PCO1, 81.3% explained variation) (Supplementary Table S8 and Figure 4C). From the metabolites, PUFA C18:3ω-3 and chlorophyll a, both peaking in the brine-based media, contributed to the main differences the different treated Ulva thalli (Figure 4D and Table 5).
4 Discussion
In the present study, we proof the suitability of inland brine for the production of the marine macroalga U. compressa in an inland cultivation system. The macroalga were successfully cultivated and harvested in a semi-open system, using natural sunlight, in units of 120 L volume over a period of 3 months. The applied brine-based cultivation supported the development of a healthy nutritional profile, rich in bioactive compounds like carotenoids and unsaturated fatty acids.
The cultivated thallus provided a high content of ω-3 rich PUFA linolenic acid (ALA, C18:3ω-3) and a low ω-6 to ω-3 ratio, both indicating a high value for human nutrition (Simopoulos, 2010). Furthermore, the measured elevated concentrations of antioxidative acting carotenoids support the value for human nutrition and health, as for example lutein is known for its eye protective properties (Buscemi et al., 2018) and β-carotene is known as an important precursor for vitamin A (Grune et al., 2010). In addition, also the observed high chlorophyll concentrations can be considered to support human nutrition based on an in vitro Caco-2 cell study by Chen et al. (Chen and Roca, 2018).
Overall, the brine-based media strongly affected the mineral and nutritional composition of the cultivated macroalgae. A possible explanation of the observed strong differences seems to be the different mineral composition of the applied media. In this respect, especially the about 38 times higher silicon (Si) concentration of the brine-based media seem to play a crucial role for the observed nutritional fortification in the cultivated macroalgae.
Considered as the second most abundant element in the earth crust and present in most soils and aquatic environments, silicon is commonly taken up and incorporated as silicon dioxide (SiO2), also known as silica, or biogenic silica (bSi) in plant (Hodson and Evans, 2020) and algal tissues (Yacano et al., 2022). Despite its abundance and known beneficial role, the essentiality of silicon for plants was long-time discussed among scientists and, recently, become more evident, by detailed description of silicon transporters and successful fertilization studies (reviewed in (Pavlovic et al., 2021). Like for plants, for algae, silica play a crucial role not only for forming the supporting and protecting frustules of the microscopic diatoms (Raven and Waite, 2004) but also by promoting growth of green macroalgae like Cladophora glomerata (Chlorophyta)(Moore and Traquair, 1976). Overall, given their known high silicon uptake marine macroalgae could be considered as silicon sink, as proposed by (Yacano et al., 2022). Next to the stabilizing role in cell walls, silicon thallus content is discussed to improve stress tolerance, as observed in temperature responses in Pyropia yezoensis (Rhodophyta) (Le et al., 2019), or in Saccharina japonica (Phaeophyceae), where the silicate uptake was activated by the presence of reactive oxygen species (Mizuta et al., 2021).
Moreover, the uptake by active silicic acid transporters (SITs) (Knight et al., 2016) can be connected to other elemental transporters (e.g. N, P, K, Ca, Mg, S, Fe, Zn, Mn, B, Cl, Ni, Al, and Na Si) as described for higher plants (Pavlovic et al., 2021). This might correlate with some observations done in the present study. Thus, we found a strong negative correlation for silicon, calcium, chloride and nickel, and the algal mineral concentration, indicating a strong uptake in the algal thallus. This was partly confirmed by a measured about 9 times higher concentration of calcium in the silicon rich brine. A close relation of silicon and calcium driven mechanisms is very likely for macroalgae, given the fact that silicification and calcification are widespread mechanisms in marine algae (Koester et al., 2016). A silicon-promoted calcium uptake was also reported from studies of various field-grown crops (e.g., wheat, corn salad, and tomatoes) (Pavlovic et al., 2021; Li et al., 2022).
In addition, to its important role in plants and algae, calcium plays also an essential role for the human diet. Being the most abundant mineral in the human body, it supports the bone and teeth formation and many important vital functions (Raisz, 1981; Anderson, 1996). Based on the recommended dietary allowances formulated by the German Nutrition Society (DGE) the daily intake of calcium should be about 1,000-mg for adults, with elevated needs (about 1100 mg d−1–1200 mg d−1) for teenager (10–19 years) calcium carbonate (CaCO3) provides one of the widest distributed calcium forms, present in rocks as well as part of structural components in many biota, including macroalgae (Al Omari et al., 2016). Containing the comparable high amount of elementary calcium (40%), CaCO3 is considered as a cheap and accessible calcium source and therefore commonly used for food supplements (Shkembi and Huppertz, 2021).
Taken into account that any food product needs to be considered as source of various and not only one single nutrient, the embedding matrix plays a crucial role for human nutrition (Aguilera, 2018). Accompanying compounds, like vitamins, fatty acids or other minerals can have a direct impact on the bioavailability of the required calcium. The presence of vitamin D support the calcium absorption in the intestine (Khazai et al., 2008) and vitamin D rich milk and lactic products (e.g., cheese, butter, cream, yoghurt, kefir) are considered as an important dietary calcium source for the human diet (Zahedirad et al., 2019). Alternatively, and strongly supported by the rising interest in dairy free diets, other vitamin D rich calcium sources, like green leafy vegetables and also macroalgae are becoming in the focus of the food industry. Here, the calcareous red seaweed Phymatolithon calcareum (formerly Lithothamnoin calcareum) (Rhodophyta) have become a key element in the vegan market as a common Ca supplement and fortifier in plant-based milk substitutes over the past years. Moreover, it is also applied in bovine feed and thus serving indirectly as milk fortifier (Neville et al., 2022). Unfortunately, considered as a non-organic ingredient it was forbidden for the processing of organic rice- and soya-based drinks from the European court of justice in April 2021 (Union, 2021). The fact is that the rather slow growing macroalgae derived from field harvest, rather than controlled cultivation, seem to be part of this juristically issue. In this respect, potentially other more farmable and, thus, certifiable macroalgae, for example, by the Seaweed Standard of the Aquaculture Stewardship Council and Marine Stewardship Council, could provide suitable replacements.
Overall elevated silicon, as well as high calcium concentrations, have been related to elevated photosynthetic activity (Silva et al., 2012; Wang et al., 2019). Consequently, the silicon-calcium-rich brine grown macroalgae reached high pigment concentrations, exceeding records of fresh harvested lettuce (Lactuca sativa) grown under natural light in greenhouse conditions in Portugal (Cruz et al., 2012) by 3.7 times for total chlorophylls. Also, for carotenoids, strong differences were observed between the media, with about 150 times higher concentrations in the BW compared to the TM. Nevertheless, compared to greenhouse grown lettuce the values of lutein were only slightly 1.1 times elevated, whereas the values for β-carotene were even lower (about 0.3 times).
Interestingly, the measured high carotenoid and chlorophyll concentrations in the brine treated U. compressa decreased significantly over experimental time, possibly following the altered silicon and calcium concentrations. In contrast, the pigment pool of the artificial seawater (TM) treated macroalgae showed less variations and even increased for chlorophyll a, β-carotene and 9-cis β-carotene over the experimental time. In addition, also other nutritive compounds, like fatty acids showed alterations over cultivation time. Here, the observed overall increase in SFA, MUFA, and PUFA in the brine exposed thalli followed the measured time-wise decrease in different elements like iron and silicon, both considered to affect lipid content and fatty acid composition in different algae species (Jiang et al., 2016). Silicon-deficiency can induce lipid production, while reducing biomass growth the same time, a physiological response which is known from microalgae and valued in the biofuel industry (Gao et al., 2019).
The observed time-wise shifts in the concentration maxima of the different nutritional compound groups, for example, carotenoids and chlorophylls at T1 and fatty acids at T2, underline not only the need to identify the driving factors but also indicate the need of a harvest strategy. Thus, harvest time seems not only play a crucial role for field grown Ulva crops (Steinhagen et al., 2022) but also for environmental controlled land-based cultivation, as approached in the present study.
As differences in water temperatures were neglectable over the cultivation time, other environmental factors like changes in light intensity might be responsible for the observed alterations in the carotenoid production (Eismann et al., 2020). In fact, in the present study we observed an overall decrease in maximum PAR intensities over the second half of the experiment. As shown by different studies carotenoids play an important photoprotective role in Ulva species, as the conversion of different xanthophylls provide an effective way to exceed excess energy in terms of nonphotochemical quenching (NPQ) (Zhang et al., 2015; Gao et al., 2020). Nevertheless, given the recorded strong alterations in daily PAR intensities, light can only explain a part of the observed changes. In addition to the discussed external cultivation factors, also other more intrinsic parameters might provide possible explanations for the observed changes in the Ulva physiology and morphology, and affect the nutritional composition. Thus, as shown in prior experiments (Fricke et al., 2023) the growth of U. compressa strongly depends from its life- and fertility stage, measured in differences of daily specific growth rates (e.g., germling ~6.4% d, thalli ~2.3% d, drop from 3.1% d to about 0.7% d during sporulation). Moreover, the genus Ulva is known to be strongly dependent from its bacterial community affecting its morphogenesis (Wichard, 2015). To clarify whether the brine-based media is feasible to support a further economic development of the U. compressa cultivation it need to meet the two important requirements of controlled environment agriculture (Ting et al., 2016), supporting a stable and qualitative production. In this respect further studies have to consider the light regime as well as addressing the potential impact of brine-based media on different life stages (e.g., spores, germlings, gametophytes, sporophytes) and associated microbiomes. The underlaying physiological processes are urgently required to define a commercial cultivation approach based on this alternative cultivation media. Next to the supported alterations in nutritional composition of growing thalli, the effect of brine-based media on fertility and reproduction has to be investigated as additional key for a continuous cultivation. Moreover, in addition to the addressed bioactive compounds, further studies shall also address the potential impact on other commercial interesting algal components, like sulphated polysaccharides or proteins (Kidgell et al., 2019; Brien et al., 2022).
5 Outlook
Given the rising interest and demand in macroalgal based products and importance of new cultivation techniques, the present study presents a promising approach to provide healthy and fresh harvested macroalgal material in inland areas. The finding that brine-based Ulva cultivation is not only possible to overcome the challenge of freshwater use (e.g., by preparing artificial salt solution) but even provide an additional nutritional benefit by fortifying the concentrations of desired compounds (e.g., minerals, photosynthetic pigments, PUFAs). The present findings might not only help to foster the use of such sea derived vegetables, but might also serve as a step stone for the further development of brine based inner land aquaculture systems. Following this path, it might not only help to diversify inland kitchens with fresh seafood but also offer the possibility of the development and combination of new regional flavors and tastes.
Data availability statement
The raw data supporting the conclusions of this article will be made available by the authors on request.
Author contributions
AF: Conceptualization, Data curation, Formal analysis, Investigation, Methodology, Writing – original draft, Writing – review & editing. VH: Formal analysis, Methodology, Writing – review & editing. MS: Funding acquisition, Project administration, Resources, Writing – review & editing, Supervision, Conceptualization. SB: Funding acquisition, Methodology, Project administration, Resources, Supervision, Writing – review & editing, Conceptualization.
Funding
The author(s) declare financial support was received for the research, authorship, and/or publication of this article. The work was performed in the frame of the project food4future (Funding code IGZ: 031B0730A) funded by the German Federal Ministry for Education and Research (BMBF within the Funding Line “Agricultural Systems of the Future”).
Acknowledgments
For helping hands in cultivation and laboratory we thank Andrea Maikath, Kersten Maikath, Toni Krause, Annett Platalla and Andrea Jankowsky. We specially thank Kerstin Schmidt supporting the work on mineral composition. We thank the staff at the thermal baths in Bad Saarow (Axel Walter) for their support.
Conflict of interest
The authors declare that the research was conducted in the absence of any commercial or financial relationships that could be construed as a potential conflict of interest.
Publisher’s note
All claims expressed in this article are solely those of the authors and do not necessarily represent those of their affiliated organizations, or those of the publisher, the editors and the reviewers. Any product that may be evaluated in this article, or claim that may be made by its manufacturer, is not guaranteed or endorsed by the publisher.
Supplementary material
The Supplementary Material for this article can be found online at: https://www.frontiersin.org/articles/10.3389/fmars.2023.1292947/full#supplementary-material
References
Aguilera J. (2018). The food matrix: implications in processing, nutrition and health. Crit. Rev. Food Sci. Nutr. 59, 1–43. doi: 10.1080/10408398.2018.1502743
Allan G. L., Fielder D., Fitzsimmons K., Applebaum S. L., Raizada S. (2009). “36 - Inland saline aquaculture,” in New Technologies in Aquaculture. Ed. Burnell G., Geoff A. (UK: Woodhead Publishing), 1119–1147. doi: 10.1533/9781845696474.6.1119
Al Omari M. M. H., Rashid I. S., Qinna N. A., Jaber A. M., Badwan A. A. (2016). “Chapter two - calcium carbonate,” in Profiles of drug substances, excipients and related methodology. Ed. Brittain H. G. (Elsevier, United States: Academic Press), 31–132.
Anderson J. J. B. (1996). “The role of calcium, phosphorus, and macronutrients in the maintenance of skeletal health,” in Osteoporosis: diagnostic and therapeutic principles. Ed. Rosen C. J. (Totowa, NJ: Humana Press), 17–27.
Bermejo R., Buschmann A., Capuzzo E., Cottier-Cook E., Fricke A., Hernández I., et al. (2022). State of knowledge regarding the potential of macroalgae cultivation in providing climate-related and other ecosystem services. Report prepared by an Eklipse Working Group for the European DG for Maritime Affairs and Fisheries. doi: 978-3-944280-28-8
Brien R., Hayes M., Sheldrake G., Tiwari B., Walsh P. (2022). Macroalgal proteins: A review. Foods 11 (4), 571. doi: 10.3390/foods11040571
Buscemi S., Corleo D., Di Pace F., Petroni M. L., Satriano A., Marchesini G. (2018). The effect of lutein on eye and extra-eye health. Nutrients 10 (9), 1321. doi: 10.3390/nu10091321
Chen K., Roca M. (2018). In vitro bioavailability of chlorophyll pigments from edible seaweeds. J. Funct. Foods 41, 25–33. doi: 10.1016/j.jff.2017.12.029
Circuncisao A. R., Catarino M. D., Cardoso S. M., Silva A. M. S. (2018). Minerals from macroalgae origin: health benefits and risks for consumers. Mar. Drugs 16 (11), 400. doi: 10.3390/md16110400
Cruz R., Baptista P., Cunha S., Pereira J. A., Casal S. (2012). Carotenoids of lettuce (Lactuca sativa L.) grown on soil enriched with spent coffee grounds. Molecules 17 (2), 1535–1547. doi: 10.3390/molecules17021535
DGE (2000). German nutrition society: The nutrition report 2000. Ed. Wolfram G. (Frankfurt am Main).
DGE (2013). German nutrition society - new reference values for calcium. Ann. Nutr. Metab. 63 (3), 186–192. doi: 10.1159/000354482
Doumeizel V., Aass K., McNevin A., Cousteau A., Yuri Yap A., Cai J., et al. (2020). Seaweed revolution - a manifesto for a sustainable future (L.s.R. Foundation, UK). https://ungc-communications-assets.s3.amazonaws.com/docs/publications/The-Seaweed-Manifesto.pdf.
EFSA (2015). Scientific opinion on dietary reference values for iron- EFSA panel on dietetic products, nutrition and allergies (NDA). EFSA J. 13. doi: 10.2903/j.efsa.2015.4254
EFSA Panel on Dietetic Products, N., and Allergies (2015). Scientific opinion on dietary reference values for magnesium. EFSA J. 13 (7), 4186. doi: 10.2903/j.efsa.2015.4186
EFSA (2018). Summary of tolerable upper intake levels – version 4. European Food and safety authority (EFSA), Parma, Italy.
EFSA Panel on Nutrition, N.F., Allergens F., Turck D., Castenmiller J., de Henauw S., Hirsch-Ernst K.-I., et al. (2019). Dietary reference values for sodium. EFSA J. 17 (9), e05778. doi: 10.2903/j.efsa.2019.5778
Eismann A. I., Perpetuo Reis R., Ferreira da Silva A., Negrão Cavalcanti D. (2020). Ulva spp. carotenoids: responses to environmental conditions. Algal Res. 48, 101916. doi: 10.1016/j.algal.2020.101916
EuropeanComission (2020). COM/2020/381 final: farm to fork strategy for a fair, healthy and environmentally-friendly food system. (Brussels, Belgium).
EuropeanComission (2021). COM(2021) 240 final: on a new approach for a sustainable blue economy in the EU Transforming the EU's Blue Economy for a Sustainable Future. (Brussels, Belgium).
FAO (2021). The state of food and agriculture 2021. Making agrifood systems more resilient to shocks and stresses (Food and Agriculture Organization of the United Nations, Rome, Italy).
Fitzner M., Fricke A., Schreiner M., Baldermann S. (2021). Utilization of regional natural brines for the indoor cultivation of Salicornia europaea. Sustainability 13 (21), 12105. doi: 10.3390/su132112105
Fleurence J. (2016). “Seaweeds as food", in Seaweed in Health and Disease Prevention. Ed. Fleurence J., Levine I. (Academic Press, Amsterdam; NL), 149–167. doi: 10.1016/B978-0-12-802772-1.00005-1
Fricke A., Harbart V., Schreiner M., Baldermann S. (2023). A proof of concept for inland production of the “sea-vegetable” Ulva compressa in Brandenburg (Central Europe) using regional saline groundwater. Algal Res. 74, 103226. doi: 10.1016/j.algal.2023.103226
Fricke A., Psarianos M., Sabban J., Fitzner M., Reipsch R., Schlüter O. K., et al. (2022). Composite materials for innovative urban farming of alternative food sources (macroalgae and crickets). Front. Sustain. Food Syst. 6. doi: 10.3389/fsufs.2022.1001769
Gao G., Wu M., Fu Q., Li X., Xu J. (2019). A two-stage model with nitrogen and silicon limitation enhances lipid productivity and biodiesel features of the marine bloom-forming diatom Skeletonema costatum. Bioresource Technol. 289, 121717. doi: 10.1016/j.biortech.2019.121717
Gao S., Zheng Z., Wang J., Wang G. (2020). Slow zeaxanthin accumulation and the enhancement of CP26 collectively contribute to an atypical non-photochemical quenching in macroalga Ulva prolifera under high light. J. Phycology 56 (2), 393–403. doi: 10.1111/jpy.12958
Grune T., Lietz G., Palou A., Ross A. C., Stahl W., Tang G., et al. (2010). Beta-carotene is an important vitamin A source for humans. J. Nutr. 140 (12), 2268s–2285s. doi: 10.3945/jn.109.119024
Guo J., Qi M., Chen H., Zhou C., Ruan R., Yan X., et al. (2022). Macroalgae-derived multifunctional bioactive substances: the potential applications for food and pharmaceuticals. Foods 11 (21), 3455. doi: 10.3390/foods11213455
Haase H., Ellinger S., Linseisen J., Neuhäuser-Berthold M., Richter M. (2020). Revised d-A-CH-reference values for the intake of zinc. J. Trace Elements Med. Biol. 61, 126536. doi: 10.1016/j.jtemb.2020.126536
Harbart V., Kläring H.-P., Baldermann S. (2022). Antifogging additives for greenhouse covers – effects on phytochemicals and nutritional quality of lettuce. J. Appl. Bot. Food Quality-Angewandte Botanik 95, 76–84. doi: 10.5073/JABFQ.2022.095.010
Hodson M. J., Evans D. E. (2020). Aluminium-silicon interactions in higher plants: an update. J. Exp. Bot. 71 (21), 6719–6729. doi: 10.1093/jxb/eraa024
Hoth P. (1997). Geowissenschaftliche Bewertungsgrundlagen zur Nutzung hydrogeothermaler Ressourcen in Norddeutschland. Geothermie Report 1. doi: 10.23689/fidgeo-482
ISO11885 (2007). Water quality - Determination of selected elements by inductively coupled plasma optical emission spectrometry (ICP-OES International Organization for Standardization (ISO), Geneva, Switzerland).
Jiang X., Han Q., Gao X., Gao G. (2016). Conditions optimising on the yield of biomass, total lipid, and valuable fatty acids in two strains of Skeletonema menzelii. Food Chem. 194, 723–732. doi: 10.1016/j.foodchem.2015.08.073
Khazai N., Judd S. E., Tangpricha V. (2008). Calcium and vitamin D: skeletal and extraskeletal health. Curr. Rheumatol Rep. 10 (2), 110–117. doi: 10.1007/s11926-008-0020-y
Kidgell J. T., Magnusson M., de Nys R., Glasson C. R. K. (2019). Ulvan: A systematic review of extraction, composition and function. Algal Research-Biomass Biofuels Bioproducts 39, 101422. doi: 10.1016/j.algal.2019.101422
Kim S.-K., Pangestuti R., Rahmadi P. (2011). “Chapter 5 - sea lettuces: culinary uses and nutritional value,” in Advances in food and nutrition research. Ed. Kim S.-K. (Academic Press, Elsevier, USA), 57–70.
Knight M. J., Senior L., Nancolas B., Ratcliffe S., Curnow P. (2016). Direct evidence of the molecular basis for biological silicon transport. Nat. Commun. 7 (1), 11926. doi: 10.1038/ncomms11926
Koester J., Brownlee C., Taylor A. R. (2016). Algal calcification and silicification. eLS, 1–10. doi: 10.1002/9780470015902.a0000313.pub2
Kraan S. (2012). “Algal polysaccharides, novel applications and outlook”, in Carbohydrates. Ed. Chang C.-F. (Taiwan, Intech Open) 489–532.
Lage-Yusty M. A., Alvarado G., Ferraces-Casais P., Lopez-Hernandez J. (2014). Modification of bioactive compounds in dried edible seaweeds. Int. J. Food Sci. Technol. 49 (1), 298–304. doi: 10.1111/ijfs.12277
Le B., Nadeem M., Yang S.-H., Shin J.-A., Kang M.-G., Chung G., Sangmi S., et al. (2019). Effect of silicon in Pyropia yezoensis under temperature and irradiance stresses through antioxidant gene expression. J. Appl. Phycology 31, 1297–1302. doi: 10.1007/s10811-018-1605-0
Leandro A., Pacheco D., Cotas J., Marques J., Pereira L., Gonçalves A. (2020). Seaweed's bioactive candidate compounds to food Industry and global food security. Life 10, 140. doi: 10.3390/life10080140
Li Z., Liu Z., Yue Z., Wang J., Jin L., Xu Z., et al. (2022). Application of exogenous silicon for alleviating photosynthetic inhibition in tomato seedlings under low and minus calcium Stress. Int. J. Mol. Sci. 23 (21), 13526. doi: 10.3390/ijms232113526
Lourenço-Lopes C., Fraga-Corral M., Jimenez-Lopez C., Pereira A. G., Garcia-Oliveira P., Carpena M., et al. (2020). Metabolites from macroalgae and its applications in the cosmetic industry: a circular economy approach. Resources 9 (9), 101. doi: 10.3390/resources9090101
Mendes M. C., Navalho S., Ferreira A., Paulino C., Figueiredo D., Silva D., et al. (2022). Algae as food in Europe: an overview of species diversity and their application. Foods 11 (13), 1871. doi: 10.3390/foods11131871
Mizuta H., Uji T., Yasui H. (2021). Extracellular silicate uptake and deposition induced by oxidative burst in Saccharina japonica sporophytes (Phaeophyceae). Algal Res. 58, 102369. doi: 10.1016/j.algal.2021.102369
Moore L. F., Traquair J. A. (1976). Silicon, a required nutrient for Cladophora glomerata (L) Kütz. (Chlorophyta). Planta 128 (2), 179–182. doi: 10.1007/bf00390321
Neville E. W., Fahey A. G., Meade K. G., Mulligan F. J. (2022). Effects of calcareous marine algae on milk production, feed intake, energy balance, mineral status, and inflammatory markers in transition dairy cows. J. Dairy Sci. 105 (8), 6616–6627. doi: 10.3168/jds.2021-21443
Nielsen F. H. (2016). “Boron,” in Encyclopedia of food and health. Eds. Caballero B., Finglas P. M., Toldrá F. (Oxford: Academic Press), 451–455.
Pavlovic J., Kostic L., Bosnic P., Kirkby E. A., Nikolic M. (2021). Interactions of silicon with essential and beneficial elements in plants. Front. Plant Sci. 12. doi: 10.3389/fpls.2021.697592
PRB (2021) World population data sheet. Available at: https://www.prb.org/news/2021-world-population-data-sheet-released/.
Raisz L. G. (1981). Calcium regulation. Clin. Biochem. 14 (5), 209–212. doi: 10.1016/s0009-9120(81)90904-8
Raven J. A., Waite A. M. (2004). The evolution of silicification in diatoms: inescapable sinking and sinking as escape? New Phytol. 162 (1), 45–61. doi: 10.1111/j.1469-8137.2004.01022.x
Schmitz T. A., Kraft E. (2022). Pilot scale photobioreactor system for land-based macroalgae cultivation. J. Appl. Phycology 34 (1), 507–516. doi: 10.1007/s10811-021-02617-7
Shkembi B., Huppertz T. (2021). Calcium absorption from food products: food matrix effects. Nutrients 14 (1), 180. doi: 10.3390/nu14010180
Shukla R. S., Skea J., Calvo Buendia E., Masson-Delmotte V., Pörtner H.-O., Roberts D. C., et al. (2019). IPCC 2019: Climate Change and Land: an IPCC special report on climate change, desertification, land degradation, sustainable land management, food security, and greenhouse gas fluxes in terrestrial ecosystems. (Intergovernmental Panel on Climate Change (IPCC), Geneva, Switzerland).
Silva O. N., Lobato A., Ávila F., Costa R. C. L., Neto C. F., Filho B., et al. (2012). Silicon-induced increase in chlorophyll is modulated by the leaf water potential in two water-deficient tomato cultivars. Plant Soil Environ. 58, 481–486. doi: 10.17221/213/2012-PSE
Simon C., McHale M., Sulpice R. (2022). Applications of Ulva biomass and strategies to improve Its yield and composition: a perspective for Ulva aquaculture. Biology 11 (11), 1593. doi: 10.3390/biology11111593
Simopoulos A. P. (2010). The omega-6/omega-3 fatty acid ratio: health implications. OCL 17 (5), 267–275. doi: 10.1051/ocl.2010.0325
Sitschik H., Ludwig F., Wetzel E., Luckert J., Höding T. H. (2005). Bog iron ore – also in Brandenburg a raw material with important economical history. Brandenbuurgische geowissenschaftliche Berichte 12, 119–128.
Soria-Lopez A., Garcia-Perez P., Carpena M., Garcia-Oliveira P., Otero P., Fraga-Corral M., et al. (2023). Challenges for future food systems: From the Green Revolution to food supply chains with a special focus on sustainability. Food Front. 4 (1), 9–20. doi: 10.1002/fft2.173
Souci S., Fachmann W., Kraut H. (2015). Die Zusammensetzung der Lebensmittel - Nährwert-Tabellen La composition des aliments - Tableaux des valeurs nutritives. Ed. Deutsche Forschungsanstalt für Lebensmittelchemie (Munich, Germany: Med Pharm), 1263.
Steinhagen S., Enge S., Cervin G., Larsson K., Edlund U., Schmidt A. E. M., et al. (2022). Harvest time can affect the optimal yield and quality of sea lettuce (Ulva fenestrata) in a sustainable sea-based cultivation. Front. Mar. Sci. 9. doi: 10.3389/fmars.2022.816890
Stober I., Birner J., Wolfgramm M. (2014). Hydrochemie der Tiefenwässer in Deutschland. Zeitschrift für Geologische Wissenschaften. 41/42, 339–380.
Strohm D., Ellinger S., Leschik-Bonnet E., Maretzke F., Heseker H., Society G. N. (2017). Revised reference values for potassium intake. Ann. Nutr. Metab. 71 (1-2), 118–124. doi: 10.1159/000479705
Suthar P., Gajaria T. K., Reddy C. R. K. (2019). Production of quality seaweed biomass through nutrient optimization for the sustainable land-based cultivation. Algal Research-Biomass Biofuels Bioproducts 42, 101583. doi: 10.1016/j.algal.2019.101583
Ting K., Lin T., Davidson P. (2016). “Integrated urban controlled environment agriculture systems”, in LED Lighting for Urban Agriculture. Ed. Kozai T., Fujiwara K., Runkle E. S. (Springer, Singapore). 19–36. doi: 10.1007/978-981-10-1848-0_2
Trinkw V. (2001). Verordnung über die Qualität von Wasser für den menschlichen Gebrauch (Trinkwasserverordnung - TrinkwV, Federal Ministry of Health, Bonn, Germany).
Union (2021). “Case C-815/19: EU law prohibits the addition of the alga Lithothamnium calcareum in the processing of organic foodstuffs such as rice- and soya-based organic drinks for the purpose of their enrichment with calcium,” in Judgment in case C-815/19 (EU Court of Justice (ECJ), Luxemburg). C.o.J.o.t.E.
van de Poll M. C., Dejong C. H., Soeters P. B. (2006). Adequate range for sulfur-containing amino acids and biomarkers for their excess: lessons from enteral and parenteral nutrition. J. Nutr. 136 (6 Suppl), 1694s–1700s. doi: 10.1093/jn/136.6.1694S
VDLUFA (2014). VDLUFA method book: volume VII environmental analysis. Association of German Agricultural Analytic and Research Institutes.
Vincent A., Stanley A., Ring J. (2021). Hidden champion of the ocean: seaweed as a growth engine for a sustainable European future Ed. Chung H. T., Jeffries E. (Seaweed for Europe).
Wang Q., Yang S., Wan S., Li X. (2019). The significance of calcium in photosynthesis. Int. J. Mol. Sci. 20 (6), 1353. doi: 10.3390/ijms20061353
Wichard T. (2015). Exploring bacteria-induced growth and morphogenesis in the green macroalga order Ulvales (Chlorophyta). Front. Plant Sci. 6. doi: 10.3389/fpls.2015.00086
Yacano M. R., Foster S. Q., Ray N. E., Oczkowski A., Raven J. A., Fulweiler R. W. (2022). Marine macroalgae are an overlooked sink of silicon in coastal systems. New Phytol. 233 (6), 2330–2336. doi: 10.1111/nph.17889
Zahedirad M., Asadzadeh S., Nikooyeh B., Neyestani T. R., Khorshidian N., Yousefi M., et al. (2019). Fortification aspects of vitamin D in dairy products: a review study. Int. Dairy J. 94, 53–64. doi: 10.1016/j.idairyj.2019.01.013
Keywords: urban farming, saline agriculture, land-based macroalgal cultivation, Ulva compressa, minerals, polyunsaturated fatty acids, carotenoids, saline groundwater
Citation: Fricke A, Harbart V, Schreiner M and Baldermann S (2023) Study on the nutritional composition of the sea vegetable Ulva compressa in a brine-based cultivation system. Front. Mar. Sci. 10:1292947. doi: 10.3389/fmars.2023.1292947
Received: 12 September 2023; Accepted: 26 October 2023;
Published: 07 December 2023.
Edited by:
Giovanna Romano, Anton Dohrn Zoological Station, ItalyReviewed by:
João Cotas, University of Coimbra, PortugalLeonel Pereira, University of Coimbra, Portugal
Copyright © 2023 Fricke, Harbart, Schreiner and Baldermann. This is an open-access article distributed under the terms of the Creative Commons Attribution License (CC BY). The use, distribution or reproduction in other forums is permitted, provided the original author(s) and the copyright owner(s) are credited and that the original publication in this journal is cited, in accordance with accepted academic practice. No use, distribution or reproduction is permitted which does not comply with these terms.
*Correspondence: Anna Fricke, ZnJpY2tlQGlnemV2LmRl