- 1Biology Department, College of Science, Imam Mohammad Ibn Saud Islamic University (IMSIU), Riyadh, Saudi Arabia
- 2Department of Biology, College of Science, Princess Nourah Bint Abdulrahman University, Riyadh, Saudi Arabia
- 3Food Processing Programme Technical Science Vocational School Mersin University, Mersin, Türkiye
- 4Department of Bioinformatics, National Institute of Research and Development for Biological Sciences, Bucharest, Romania
- 5LR01ES14 Laboratory of Environment Biomonitoring, Coastal Ecology and Ecotoxicology Unit, Faculty of Sciences of Bizerte, University of Carthage, Zarzouna, Tunisia
Introduction: The current experiment investigated the multifaceted effects induced by microplastics and the statin Lipitor on marine benthic nematodes.
Methods: The nematodes were exposed to a single polystyrene and polyvinyl chlorides (both at 1 mg.kg-1 Dry Weight) and two Lipitor concentrations (0.1 and 1 mg.l-1), as well as to a mixture of both types of pollutants, for 30 days.
Results: The results highlighted a significant decrease in the abundance, individual biomass, and diversity of nematodes directly with the addition of polyvinyl chlorides and/or Lipitor. These treatments induced a greater mortality rate among microvores and diatom feeders compared to other feeding types of nematodes.
Discussion: The nematofauna underwent a strong restructuring phase following exposure to microplastics and Lipitor when added alone, leading to the disappearance of sensitive species and their replacement by more tolerant taxa. The toxicity of Lipitor is attenuated by the physical bonding with polystyrene when added to a mixture and has no negative effect on marine nematode species.
1 Introduction
Population growth and urbanization take place in many parts of the world, often accompanied by the local development of industry and technological advances, with detrimental effects on the environment (Avio et al., 2015). Various abiotic components, such as the atmosphere, water, soil, and sediments become the end recipients of traditional and emerging pollutants, with a negative impact on their ecological status (Menchaca et al., 2014).
One of the most widespread types of emergent contaminants of current concern comprises by- and end products of the plastic material industry (Abuwatfa et al., 2021). Two common types of plastics are Polyvinyl chlorides (hereafter PVC) and Polystyrene (hereafter PS). PVCs comprise cheap, easy-to-shape, and lightweight plastics. In recent history, the PVCs replaced traditional materials in various economic domains, but their efficient recycling remains a major challenge (Zhao et al., 2021). Besides, there are also a growing number of technical and economic issues associated with this, hindering progress in this field. The waste recycling technology improved the polymer market industry, but there is still room for improvement (Lange, 2021). Various types of common polymers, such as vinyl, are inherently difficult to recycle and to be transformed into an environmentally friendly end product. Thiolactones are a cleavable common additive used in the chemical degradation and recovery technology of vinyl polymers and produced another well-known free-radical polymer, polystyrene. PSs of variable molecular mass (i.e. 20–300 kDa) and randomly incorporated thioester backbone linkages (i.e. 2.5–55 mol %) are selectively depolymerized to produce well-defined thiol-terminated fragments (i.e. below 10 kDa). These fragments are suitable for efficient oxidative depolymerization, which produces recovered PS in good yields (i.e. 80–95%) with similar molecular mass (Wei et al., 2019).
Besides various end products of the plastic industry, another human activity with a detrimental impact on the environment is the drug industry. More recently, a particular drug type, called statins with a more special focus on Lipitor, caught the attention of specialists (Chow et al., 2010; Jaramillo-Madrid et al., 2020). Several countries with high development rates experienced during the past decade the occurrence of obesity and cardiovascular diseases. The frequency of occurrence of these human issues was proved to be reduced by Lipitor, a common lipid-modifying statin drug, which exerts multiple effects on lipoprotein metabolism and remains up to date the number-one-sold statin of all time.
It is known that large quantities of drugs and microplastics end up in the benthic marine areas and are transferred back to humans by bioaccumulation and biomagnification processes. The multifaceted detrimental effects induced by these xenobiotics on biota remain largely unknown and are insufficiently documented. In the current study, we have explored the impact that Lipitor and microplastics (PVC and PS) have on marine meiofauna, with far-reaching practical applications. The current bioassay aimed to fill the knowledge gap about meiofauna because of their lower use as ecological indicators compared to macrofauna (Borja et al., 2000). The current study supports, however, that utilizing these tiny organisms could be just as effective or potentially more beneficial and effective, thanks to their multi-species responsiveness, abundant availability, and constrained requirements for sampling (Semprucci et al., 2015). Yet, there remains an ongoing discussion regarding the possession of meiobenthic nematodes of traits that distinguish them as one of the most prosperous multicellular organisms on Earth, thriving in environments with very difficult circumstances (Sapir, 2021). Indeed, these worms form the lower part of marine food webs and comprise very abundant (up to 23 million per m2) and diverse invertebrates (~9000 species were described based on morphological traits) (Nemys, 2024). Global species richness of marine nematodes was even proposed based on the progress of DNA barcoding, being estimated between ~50,000 and one million or more (Ridall and Ingels, 2021). Numerous experimental studies have demonstrated that nematodes have an interesting potential as bioindicative tools in laboratory bioassays due to several characteristics, particularly their small size (generally 1–5 mm in length at the adult stage) and brief lifespans (weeks to months) (Moreno et al., 2011). Numerous studies made thus it possible to obtain ecotoxicological information that covers a large sensitivity/tolerance spectrum for nematodes against stress agents (Hägerbäumer et al., 2015).
The current work aimed to assess the ecotoxicological impact of three emergent contaminants, two microplastic types (i.e. PVC and PS) and Lipitor, on meiobenthic nematodes, by using single and mixture applications. The results obtained will be particularly useful with the goals of testing if one or both categories of microplastics could be used as remediators for statins’ contaminated sediments based on outcomes of treatment combinations.
2 Materials and methods
A graphic representation of steps, experimental design, and type of analyses are given in Figure 1. Details of all schematic steps are presented hereafter.
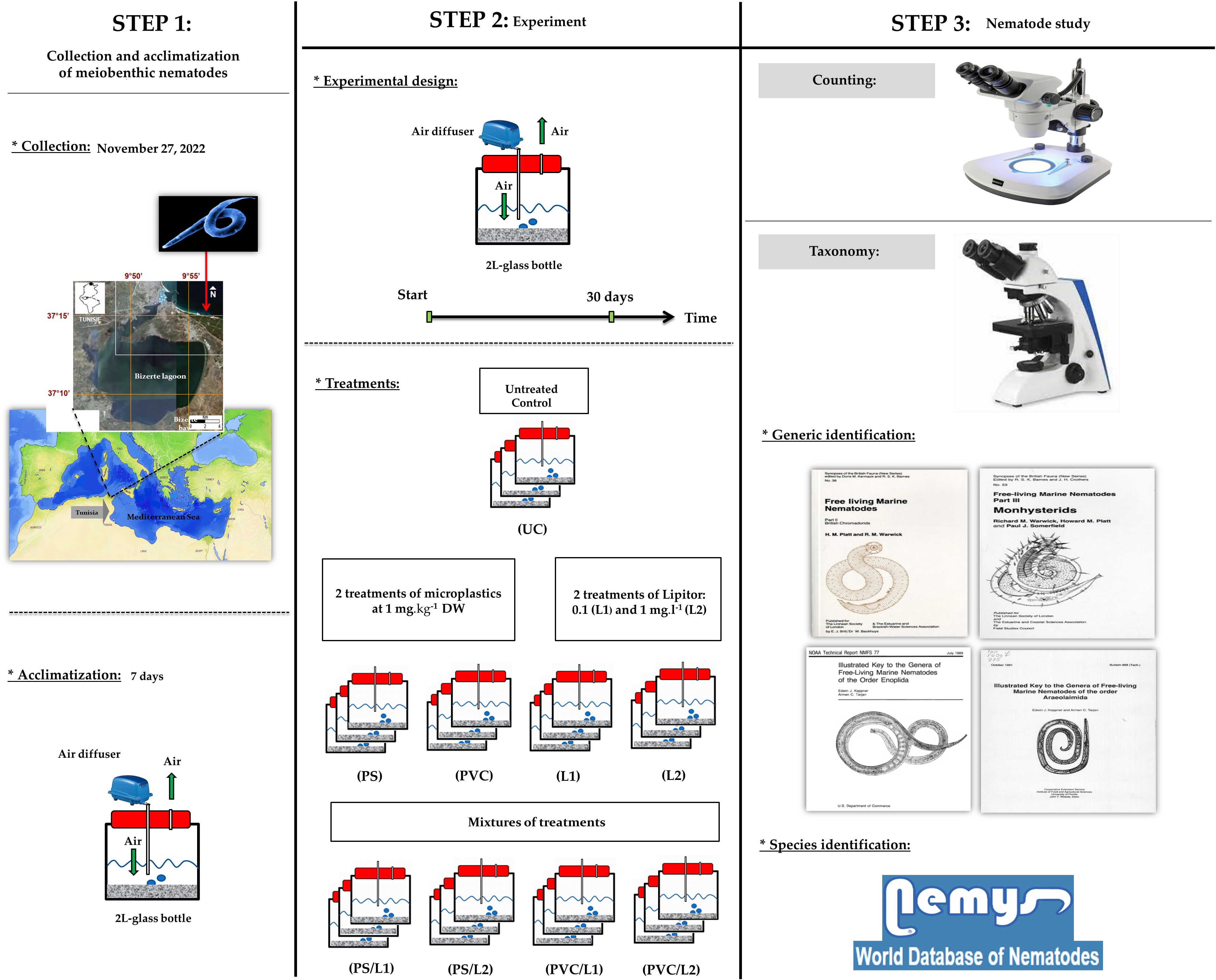
Figure 1 Graphical summary of steps and methodology adopted to experimentally study effects of Polystyrene, Polyvinyl chlorides, and Lipitor on meiobenthic nematodes.
2.1 Collection site and laboratory processing
Sediments were collected early in the morning (7 A.M.) of November 27, 2022, at a coastal site (37°15’07.34” N, 9°56’26.75” E) situated in the upper infralittoral domain of Bizerte Bay, Tunisia. Such a collection timing was chosen on purpose to avoid the vertical migration of nematodes to deeper sediment strata away from heat after sunrise (Wakkaf et al., 2020). The samples were taken from the upper 5 cm of the sediment layer with the aid of Plexiglass hand cores (inner diameter of 3.6 cm, section of 10 cm2). Coull and Chandler (1992) noted that the majority of meiobenthic organisms are found in the top substrate layers. The collection site was chosen following the recommendations of Boufahja (2010) and Hedfi et al (Hedfi et al., 2013, 2021), who classified it as pristine and with a highly diverse meiofauna community. Four abiotic parameters were measured at the sediment-water interface: the water depth, which was measured with a pendulum, salinity, and temperature, measured with a Thermo-Salinometer Model WTW LF 196 (Weilheim, Germany) and dissolved oxygen concentrations with a WTW Oxi 330/SET, WTW, Weilheim (Germany) Model Oximeter.
In the laboratory, aliquots of sediment were sieved (63 μm mesh size) and then dried at 45°C (Buchanan, 1971). Cumulative curves were plotted afterward to measure the mean grain size of the coarse fraction (Buchanan, 1971). Additional aliquots, of equal volumes, were used to quantify the total organic matter content by the mass loss method (450°C, 6 h) (Fabiano and Danovaro, 1994), and water content, after drying the sediment at 45°C until constant mass (Boufahja et al., 2011).
2.2 Sediment contamination and experimental setup
Following Schratzberger et al. (2004), some of the collected sediment was made azoic by repeated (three times) freezing (-20°C) and thawing (12 h/48 h). Following the removal of coarser particles (small rocks and microalgal debris) by sieving (1 mm), the remaining sediment was allowed to acclimatize for a week to adapt the meiofauna to the experimental conditions (darkness, 4°C) (Bellakhal et al., 2023).
Two types of microplastics were considered in this experiment: polystyrene and polyvinyl chloride. Spheres of PS microplastics measuring 10 μm in size and with a density of 1.05 g cm−3 were purchased from PolyScience, Europe (Fluoresbrite YG magnetic microspheres, Hirschberg an der Bergstraße, Germany, excitation: 441 nm, emission: 486 nm). These microplastics were previously identified and microscopically described by Weber et al. (2021). A high molecular weight PVC (MW = 48.000 g. mol−1, 16 mmol of Cl.g−1, 99%) was also acquired from Fluka. These microplastics consist of round particles that are smaller than 40 µm in diameter.
The amounts of PVC and PS to be introduced in the experimental units were chosen based on works of Hedfi et al. (2022) and Bellakhal et al. (2023), respectively. Based on these authors, a concentration of 1 mg.kg-1 Dry Weight ‘DW’ of microplastics will be not ecotoxic for meiobenthic nematodes in the case of PS, but with no known effect for PVC. The experiment lasted for 30 days (Essid et al., 2021) after applying eight treatments: polystyrene (PS: 1 mg.kg-1 DW), Polyvinyl chloride (PVC: 1 mg.kg-1 DW), and Lipitor (L1: 0.1 mg.l-1and L2: 1 mg.l-1), and their mixtures (PS/L1, PS/L2, PVC/L1, and PVC/L2). The control nematodes were topped up with only filtered seawater (0.7 µm pore-size Glas Microfibre GF/F, Whatman, Allouche et al., 2022) without the addition of Lipitor or microplastics.
2.3 Nematode study
The method of levigation-decantation-sieving (40 μm and 1 mm) was first applied to extract the meiobenthos, following Vitiello and Dinet (1979). Only the fraction coarser than 40 μm sieve was kept in 4% neutral formalin, and Rose Bengal (0.2 g.l-1) was added to better distinguish the pink-colored organisms from inert background material (Guo et al., 2001). Afterward, the meiobenthic nematodes were sorted with the aid of a 50x stereomicroscope (Wild-M3B type) with the aid of a tiled Dollfus tank (200 squares of 5 mm2, Hedfi et al., 2022). One hundred nematodes were then picked from each microcosm (Ishak et al., 2022) and mounted on microscope slides as described by Seinhorst (1959). The worms were identified to genus level with a Nikon DS-Fi2 camera coupled to a Nikon microscope (Image Software NIS Elements Analysis Version 4.0 Nikon 4.00.07–build 787–64 bit) based on the generic keys of Platt and Warwick (1983, 1988), Warwick et al. (1998) and Keppner and Tarjan (Keppner and Tarjan, 1989, 1991, 1994, 1999). Morphological descriptions of the species were downloaded from the NeMys database (Nemys, 2024). Four community-based qualitative tools were considered, namely: the Species number (S), Margalef’s species richness (d), Shannon-Wiener’s Index (H’), and Pielou’s Evenness (J’).
Two functional traits were distinguished for each nematode genus (Schratzberger et al., 2007): feeding type and tail shape. Following Wieser (1953), four trophic groups were separated: the selective deposit feeders (1A), which are mainly microcores, non-selective deposit feeders (1B) that consume detritus, the epistratum feeders (2A) that feed on benthic diatoms, and the omnivores-carnivores (2B) that prey on smaller meiobenthic invertebrates. Moreover, four tail shapes were discriminated according to Thistle et al. (1995): conical (co), clavate/conico-cylindrical (cla), elongated/filiform (e/f), and short/rounded (s/r).
2.4 Statistical processing
Data were tested for normality (i.e. Kolmogorov-Smirnov test) and equality of variances (i.e. Bartlett test) to satisfy the normal distribution and log-transformed afterward when necessary. Six univariate metrics were measured for each microcosm: abundance, individual biomass, number of species (S), Margalef’s species richness (d), Shannon-Weaner index (H’), and Pielou’s evenness (J’). One-way analysis of variance (ANOVA) and Tukey’s HSD (Honestly Significant Difference) tests were performed using STATISTICA v.8 software to detect significant global and for multiple comparisons, respectively. Several multivariate analyses were also performed using PRIMER v.5 package (Plymouth Routines in Multivariate Ecological Research) (see Clarke, 1993; Clarke and Glorley, 2001). First, a similarity matrix was constructed based on single-linkage Bray-Curtis similarity scores obtained from square-root transformed nematode abundances. Subsequently, hierarchical cluster analysis (hereinafter referred to as CA) and non-metric multidimensional scaling (hereinafter referred to as nMDS) sorting were applied. Analysis of similarity (ANOSIM) was necessary to identify significant taxonomic or functional differences, followed by SIMPER analysis (SIMilarity PERcentages) to determine the contribution of taxa or functional groups to ~50% to the average difference between treatments (Clarke, 1993; Mahmoudi et al., 2005).
3 Results
3.1 Abiotic features of the collection site
In-situ dissolved oxygen concentration was 12.6 mg.l-1 at 50 cm of water column, whereas the temperature and salinity were 14°C and 38.4 PSU, respectively. The sediments were coarse (> 63 µm, 87.23 ± 4.11%) and the mean grain size was 0.56 ± 0.09%. Finally, the total organic matter and water content of sediment were 2.5 ± 0.21% and 36.7 ± 2.19%, respectively.
3.2 Abundance and individual biomass of nematodes
Table 1 showed significant fluctuations in terms of abundances and individual biomass of meiobenthic nematodes. Thus, a maximum of 1239 ± 37 individuals and 2.23 ± 0.11 µg DW distinguished discernibly nematodes of the control community UC from those of all the other ones. It should be said that the maximum differences were found between the assemblage UC and those exposed to PVC, L1, and L2, for both abundance and individual biomass (Tukey’s HSD test: p < 0.0001).
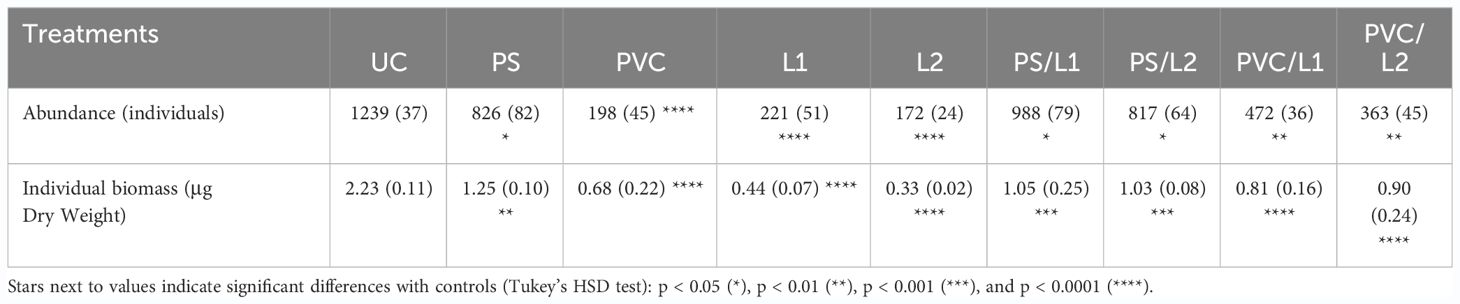
Table 1 Abundance and individual biomass of meiobenthic nematodes exposed to the control treatment (UC) and those enriched with Polystyrene (PS), polyvinyl chlorides (PVC), Lipitor (L1 and L2), and their mixtures (PS/L1, PS/L2, PVC/L1, and PVC/L2).
3.3 Taxonomic census and community composition of nematodes
At the end of the bioassay, the free-living marine nematodes comprised 7 orders, 18 families, 23 genera, and 27 species (Tables 2, 3). The most diverse families were Xyalidae (S = 6), Cyatholaimidae (S = 3), Oncholaimidae (S = 2), and Comesomatidae (S = 2). The remaining 14 families comprised just one species (Table 2). The control UC was populated by 27 species, whereas the treatments comprised as follows: PS - 22 species, PVC - 20 species, L1 - 22 species, L2 - 21 species, PS/L1 - 26 species, PS/L2 - 26 species, PVC/L1 - 25 species, PVC/L2 - 19 species.
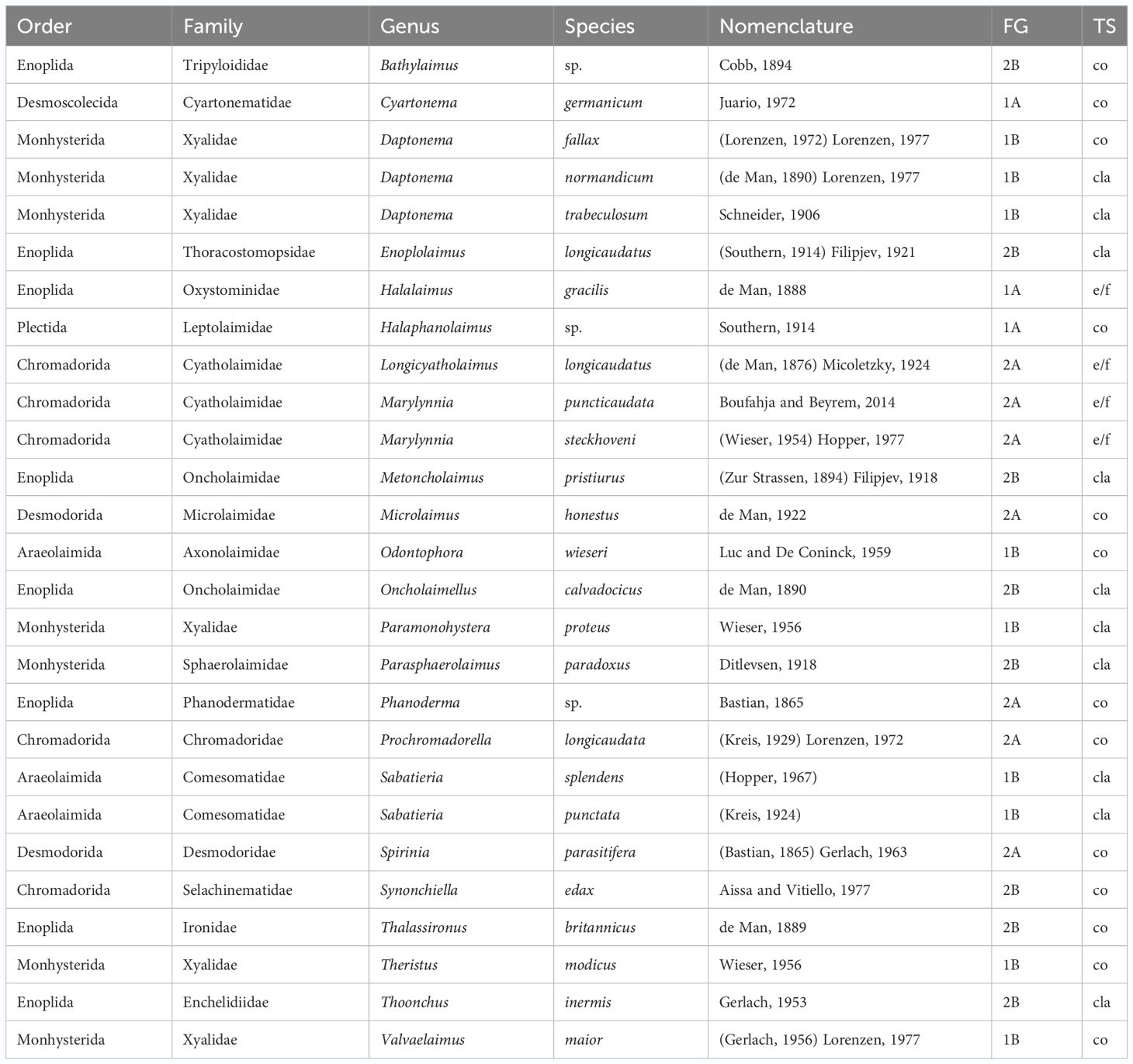
Table 2 Alphabetical listing of nematode taxa from microcosms spiked or not with Polyvinyl chlorides, polystyrene, and/or Lipitor and their taxonomic nomenclature and classification, feeding types (FG), and tail shapes (TS).
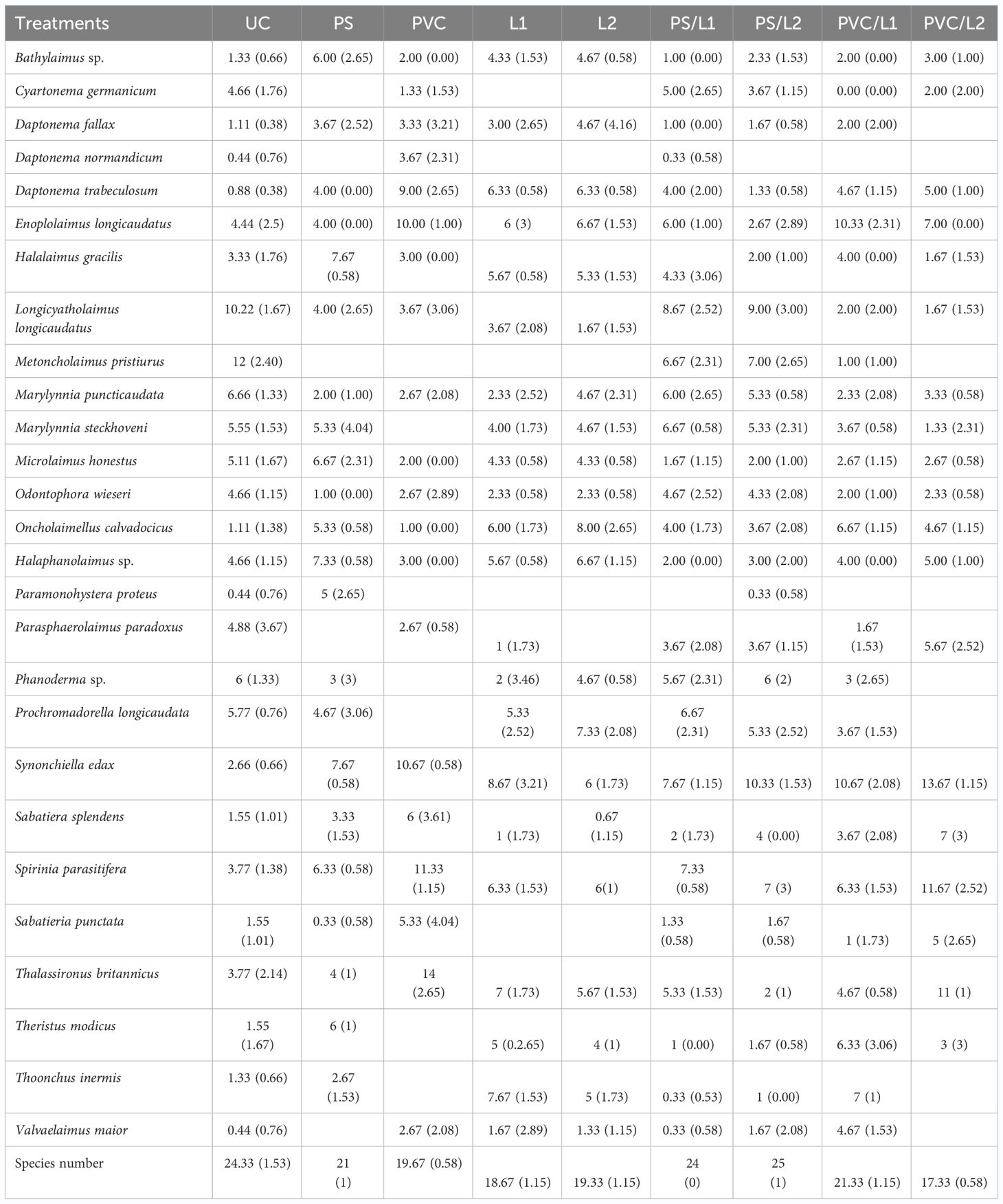
Table 3 Relative abundances (%) of the free-living nematode species identified in the control treatment (UC) and those enriched with Polystyrene (PS), polyvinyl chlorides (PVC), Lipitor (L1 and L2), and their mixtures (PS/L1, PS/L2, PVC/L1, and PVC/L2).
In control treatments, the nematofauna was co-dominated (5% ≤, Engelmann (1978)) by seven taxa, as follows: Metoncholaimus pristiurus (12 ± 2.40%), Longicyatholaimus longigicaudatus (10.22 ± 1.67%), Marylynnia puncticaudata (6.66 ± 1.33%), Phanoderma sp. (6 ± 1.3%), Prochromadorella longicaudata (5.7 ± 0.76), M. steckhoveni (5.55 ± 1.53%), and Microlaimus honestus (5.11 ± 1.67%).
The changes observed in the nematode diversity in treatments are graphically representation in Figure 2. The communities comprising the lower number of species were the treatments exposed to Polyvinyl chlorides (PVC) and Lipitor (L1 and L2). The rest of the treatments were associated with higher taxonomic diversity, statistically not different from controls (Tukey-HSD test: p-values > 0.05, Figure 2). In contrast, the metrics H’ amd/or d were significantly lower in PVC, L1, and L2 compared to UC (Tukey-HSD test: p-values < 0.01, Figure 2). The Pielou’s Evenness did not differ among treatments (1-ANOVA: p-values = 0.7135, Figure 2).
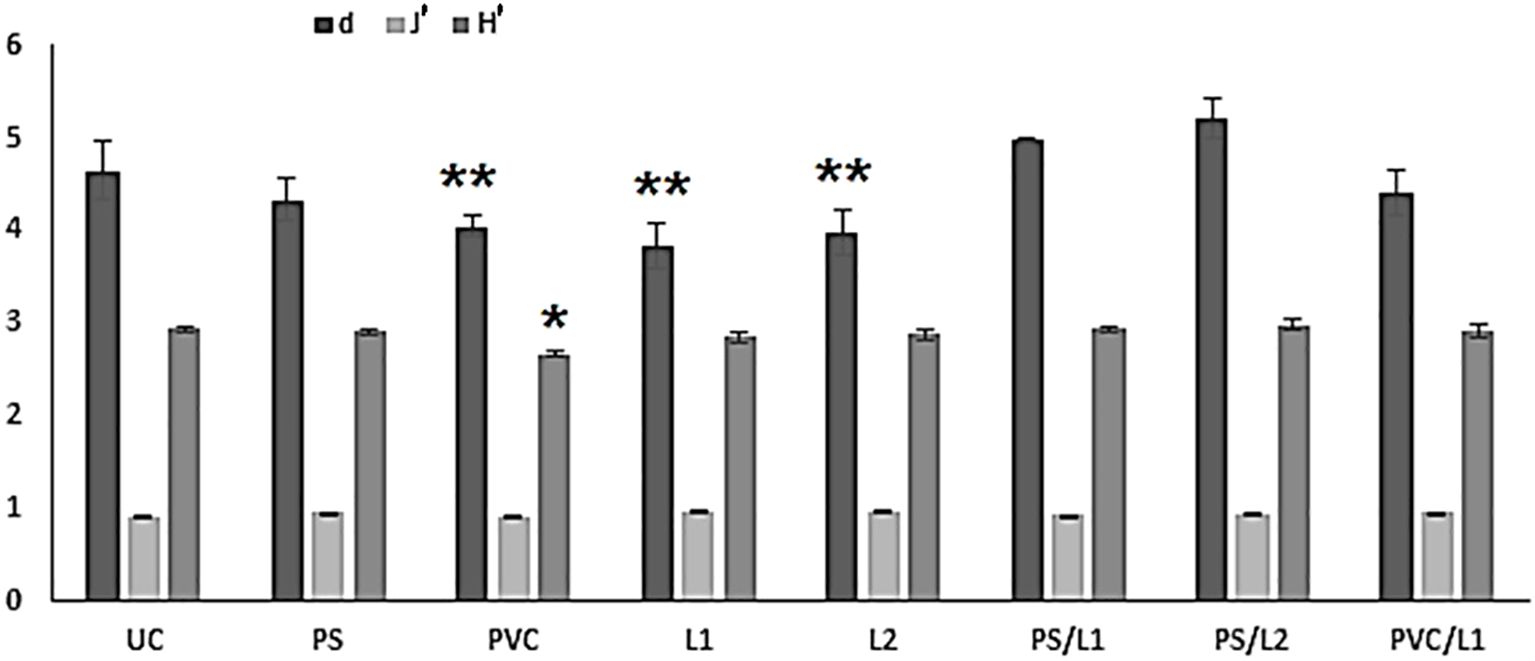
Figure 2 Graphical summary of univariate taxonomic indices of the control microcosms (UC) and those enriched with polystyrene (PS), Polyvinyl chlorides (PVC), Lipitor (L1 and L2) and their mixtures (PS/L1, PS/L2, PVC/L1, and PVC/L2). d = Margalef’s Species Richness, J’ = Pielou’s evenness, H’ = Shannon-Wiener index. The stars indicate the significant differences compared to the controls UC (*p < 0.05; **p < 0.01; Tukey’s HSD test, log-transformed data).
The Hierarchical Cluster Analysis and the nMDS orientation based on the Bray-Curtis similarity matrix, revealed at the cutoff at ~70%, a similar two-dimensional pattern of the nematode assemblages (Figure 3). The nematofauna was divided into three groups. The first group belonged to treatments exposed to PVC and PVC/L2 and was located on the top left of Figure 3. The second group comprised the nematodes exposed to UC, PS/L1, and PS/L2 and was located at the bottom of Figure 3. The third group was exposed to PVC/L1, L1, L2, and PS and was located at the top right of Figure 3. This clustering was also closely supported by the dissimilarity values, which linked treatments to controls (Table 4). Overall, based on the ANOSIM outcomes and dissimilarity values compared to controls (Table 4), the treatments followed the ranking PS/L2 < PS/L1 < L2 < PS < PVC/L1 < L1 < PVC/L2 < PVC. The minimum values of the R-statistics were observed also for PS/L2 (0.889) and PS/L1 (0.926); all the remaining treatments had a value of 1. SIMPER results (Table 4) supported also the overall nMDS outcomes, by specifying the contributions of taxa to ~50% of dissimilarities among assemblages exposed to PS, PVC, and L and control treatments, as follows:
- The first pattern: the abundances of three species were significantly reduced at PS: L. longicaudatus, M. puncticaudata, and Odontophora wieseri (Table 4). The following species were deleted: M. pristiurus, Cyartonema germanicum, and Parasphaerolaimus paradoxus.
- The second pattern: the abundances of three species were significantly reduced at PVC: L. longicaudatus, C. germanicum, and M. puncticaudata (Table 4). The following species were deleted: M. pristiurus, Phanoderma sp., P. longicaudata, and M. puncticaudata.
-The third pattern: the abundances of one species were significantly reduced at L1 and L2: L. longicaudatus (Table 4). The following species were deleted: M. pristiurus, C. germanicum, and S. punctata, but the P. paradoxus in L1 declined and in L2 disappeared.
- The fourth taxonomic pattern was apparent in the remaining mixtures: PS/L1, PS/L2, PVC/L1, and PVC/L2. M. pristiurus declined in all mixtures, except for PVC/L2, from where it disappeared. L. longicaudatus declined in all treatments.
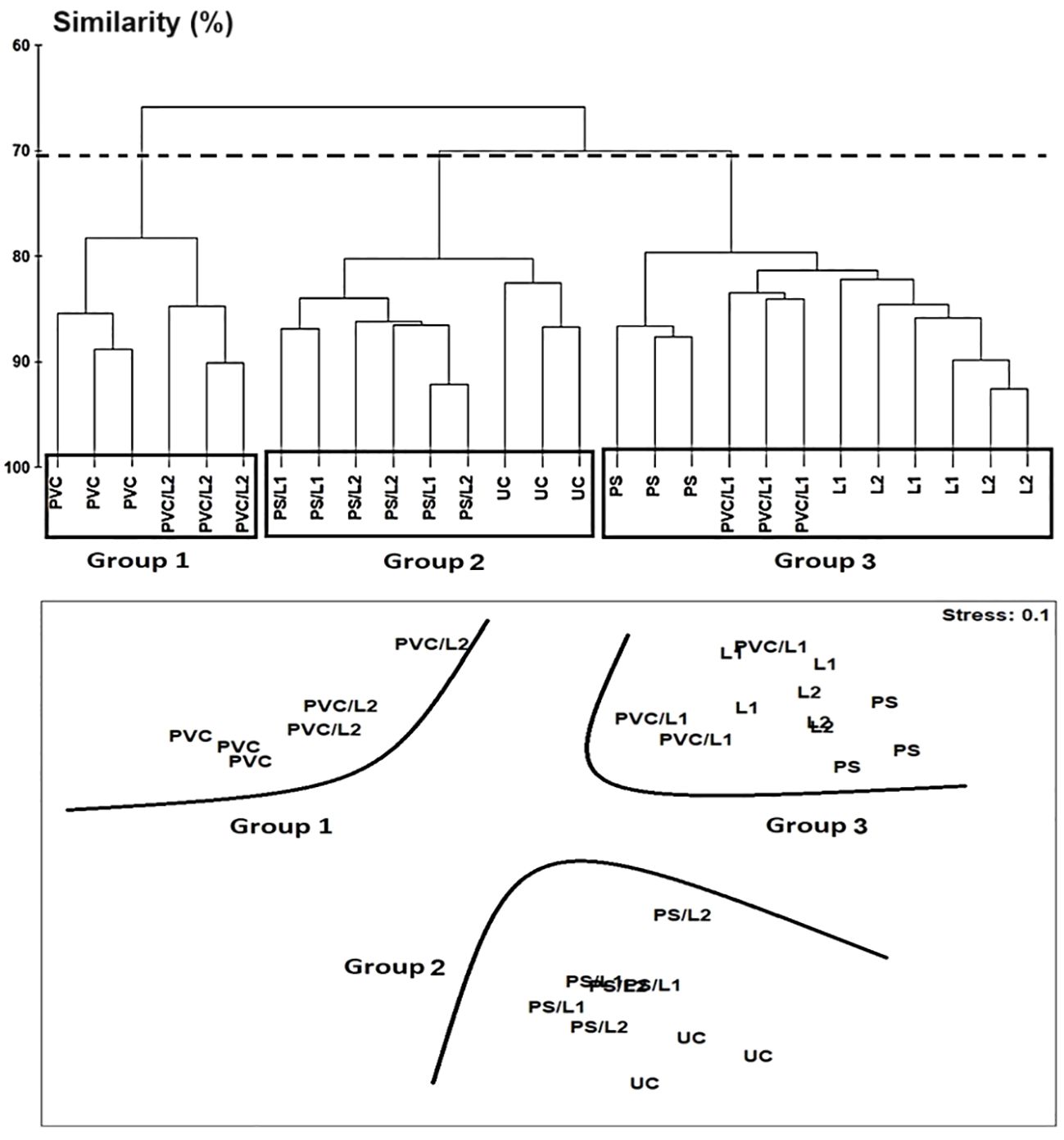
Figure 3 Above: Hierarchical Cluster Analysis (single linkage Bray-Curtis similarity values) of nematode assemblages based on square-root transformed abundances of species from a nematofauna the control nematode assemblage (UC) and those enriched with polystyrene (PS), Polyvinyl chlorides (PVC), Lipitor (L1 and L2) and their mixtures (PS/L1, PS/L2, PVC/L1, and PVC/L2) where groups are separated by fixing the cutoff at ~70% of Bray-Curtis similarity, as shown by the dotted horizontal line. Below: non-metric Multidimensional Scaling (nMDS) 2D plot based on Bray-Curtis similarity.
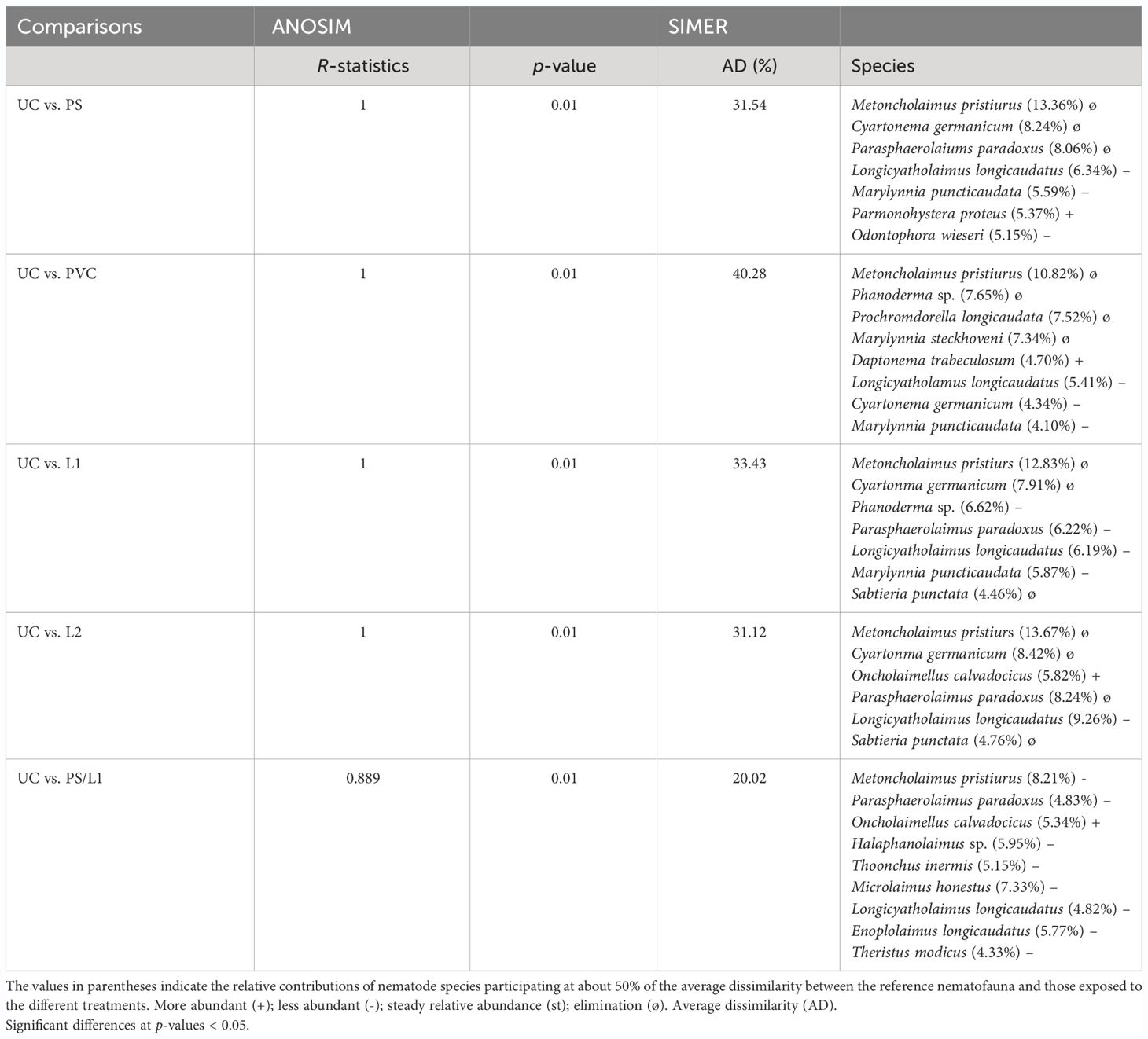
Table 4 Analysis of Similarity (ANOSIM) and SIMPER (Similarity Percentage) procedures based on taxonomic composition between the control nematode assemblage (UC) and those enriched with polystyrene (PS), Polyvinyl chlorides (PVC), Lipitor (L), and their mixtures (PS/L1, PS/L2, PVC/L1, and PVC/L2).
4 Discussion
The current experiment aimed to assess the separate and combined effects of PS, PVC, and Lipitor on meiobenthic nematodes. A comparable magnitude of the negative impact of PVC, L1, and L2 was significantly found on the abundance, individual biomass, and taxonomic diversity at the end of the current experiment. The microcosms with the highest concentrations of PVC microplastics and Lipitor were significantly less populated, and less rich in large individuals and species compared to controls and all the rest of the assemblages. The species L. longicaudatus (2A) and C. germanicum (1A) appeared to be sensitive, whereas Daptonema trabeculosum (1B) and Oncholaimellus calvadocicus (2B) were tolerant/opportunistic for PCV and Lipitor, respectively. The special case of the omnivore-carnivore M. pristiurus, which appeared extremely sensitive to both types of pollutants, could be related to the fact that most specimens collected were juveniles, so at a vulnerable stage of their life.
The suggested sensitivity of nematodes to both Lipitor and PVC could also be related to the feeding mode, given that the communities from all treatments comprised a low frequency of epistratum feeders (or diatom consumers) and microvores. Thus, it was deduced that the thresholds were reached as low as 1 mg.l-1 of Lipitor and 1 mg.kg-1 of PVC. The scientific literature on the uptake and toxicity of statins and PVC by diatoms and microbes seems to support the above findings. According to Wang et al. (2020), PVC microplastics negatively impact marine diatoms in a manner that correlates with the dosage, leading to a decrease in chlorophyll levels, hindering photosynthesis, and causing harm to algal cells. Moreover, they indicated that PVC microplastics that have been exposed to UV light are more harmful than new PVC microplastics, causing harm to the growth and chlorophyll levels of freshwater algae Chlamydomonas reinhardtii. Overall, it appears that exposure to microplastics PVC has a detrimental impact on the growth of freshwater algae by particularly hindering the photosynthesis process (Wu et al., 2019).
On the other hand, Zhao et al. (2021) mentioned that PVC microplastics play a crucial role in the transportation and spread of pathogenic bacteria and resistance genes in sewage, aiding in the dissemination of antibiotics and heavy metals. Indeed, PVC microplastics have a detrimental effect on the anaerobic digestion of waste-activated sludge due to the release of toxic bisphenol-A, resulting in inhibitory impacts on the processes of hydrolysis-acidification and methanation (Wei et al., 2019). Moreover, as reported Dai et al. (2020), this type of microplastics inhibits nitrogen removal and promotes antibiotic resistance gene propagation.
As mentioned above, significant toxicity was also observed after exposure to Lipitor for both algae (Jaramillo-Madrid et al., 2020) and microorganisms (Chow et al., 2010; Ting et al., 2016), suggesting at least a negative indirect impact of statins on feeding-type nematodes 2A and 1A. Indeed, it is known that these drugs have antimicrobial effects on a range of oral microorganisms, such as periodontal pathogens and bacterial species resistant to viruses and fungi (Ting et al., 2016). Also, treating diatoms with statins is known to block the enzymes 3-hydroxy-3-methyl glutaryl CoA reductase and disrupt the metabolism of isoprenoids, leading to a reduction in total sterol content (Jaramillo-Madrid et al., 2020).
By the end of the experiment, two feeding groups seem to have been favored: the non-selective deposit feeders (1B) and omnivore-carnivores (2B). The explanation could be related to the toxicity of PVC and Lipitor on sensitive nematodes, mostly appurtenant to feeding groups made with microvores and epistrate-consumers. The nematodes 1B and 2B may have ingested detritus comprising corpses of decomposing prey 1A and 2A. This feeding mode is common for 1B nematodes (Wieser, 1953), and facultative for 2B, the latter becoming omnivorous if necessary (Moens and Vincx, 1997).
Another functional aspect is likely to have played an important role in the current experiment: the tail shape. The worms with elongated and conical tails were disadvantaged in the presence of both pollutants (i.e. PVC and Lipitor). It could be hypothesized that the movements of the elongated morphotype were hampered by the gluey nature of the organically enriched substrate with decomposing bodies. The worms with conical caudal shape proved to be more sensitive to pollution because of their larger support lateral contact surface and, as a consequence, the lower jumping potential, associated with the frequency and speediness, leading overall to longer exposure time to pollution. An additional potential explanation for such a pattern would also be the possibility of corpses acting as glue themselves, therefore binding the worms with higher leaning surfaces in their caudal part (co).
The mixture of polystyrene and Lipitor had a less toxic impact on meiobenthic nematodes when combined compared to their individual effects. The abundance, individual biomass, and taxonomic diversity of nematodes in treatments PS/L1 and PS/L2 were comparable to control. Such an effect could be related to an antagonist interaction between the two xenobiotics. Particularly, the nMDS ordination showed different clustering between PVC/L2 and PS/L2 groups. The former treatment appears to have had a higher toxicity on meiobenthic nematodes compared to the latter. Thus, it could be evoked that the polystyrene was able to neutralize the toxicity of Lipitor and a plausible explanation for the similarity in abundances and species richness among PS/L1 and PS/L2 treatments and control, with just 19.5% dissimilarity. Following the rationale highlighted by Wakkaf et al. (2020) and Hedfi et al. (2022), the occurrence of aggregates of polystyrene and Lipitor on sediment particles seems to distance the latter pollutant away from nematodes (Figure 4). Therefore, these aggregates may comprise the substrate itself, making it coarser and reducing at least in part the availability of toxicant compounds for nematodes. This agglutination may also affect their bioavailability, by reducing the cuticular uptake rate by nematodes, as naturally larger aggregates are less efficiently internalized.
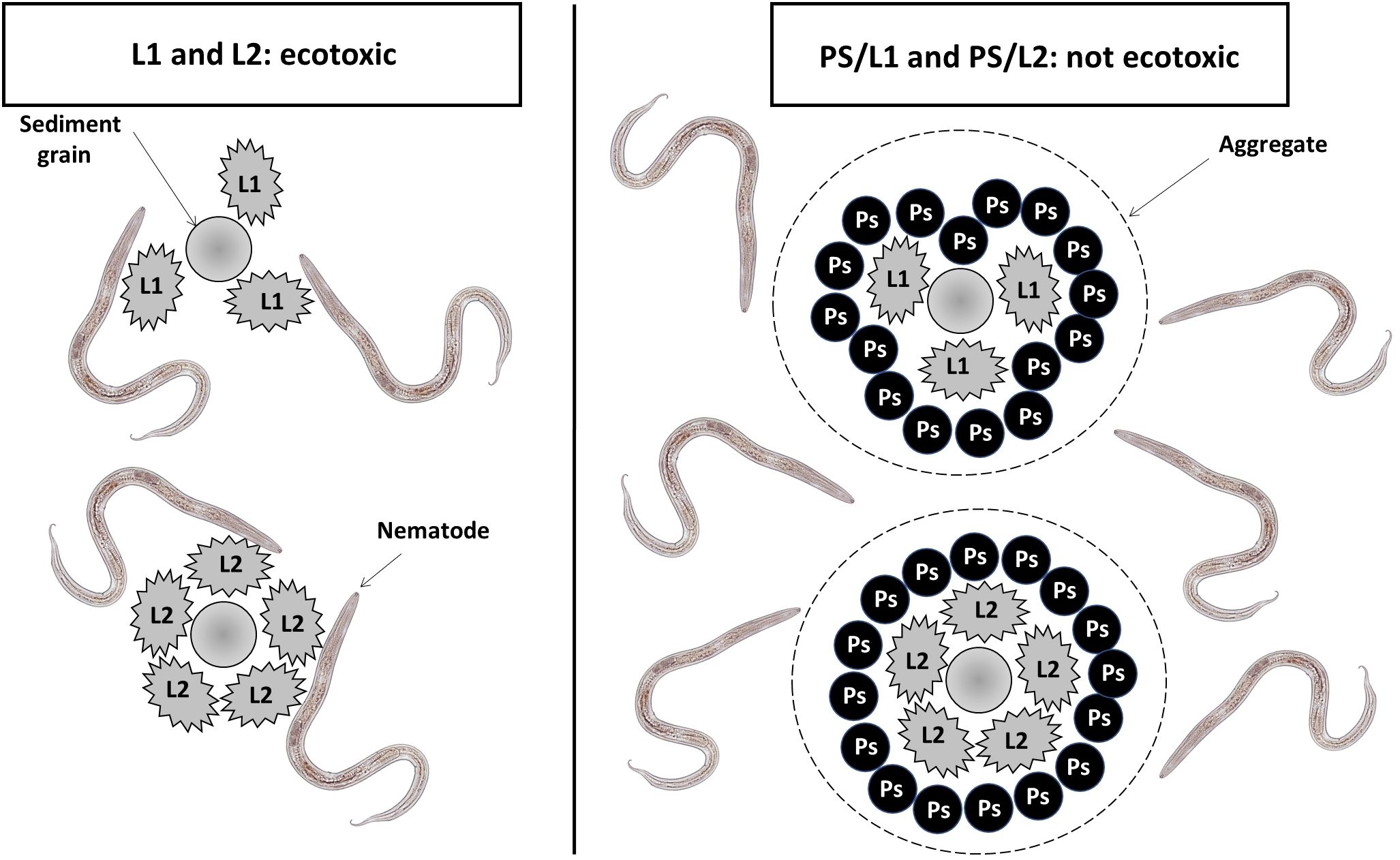
Figure 4 Graphical representation showing the hypothetical behavior of Lipitor (L1 = 0.1 mg.l-1, L2 = 1 mg.l-1) in the presence of polystyrene particles (PS = 1 mg.kg-1 Dry Weight).
Overall, two objectives appeared behind the current work: (1) determining the threshold related to the exposure to Lipitor, and (2) testing PS beads as a potential remediation tool for reducing sediment contamination with statins. However, despite the results found are promising, it will be very difficult to obtain governmental authorization to spill microplastics in contaminated areas with Lipitor. As perspectives, more engineering studies should be designed to give more details on how and by using which amount and size of polystyrene particles will be added to contaminated sediments.
5 Conclusion
Meiobenthic nematodes are known to be one the most abundant and diverse groups of invertebrates from marine benthic areas. Despite numerous published studies using these worms as bioindicators and models in ecotoxicology, no work has focused up to date on the toxic impact of microplastic and Lipitor on marine benthic meiofauna. The results of the current experiment identified important interactions between these types of xenobiotics and the abundances, individual biomasses, and taxonomic traits of nematodes, particularly at concentrations of 1 mg.kg-1 DW of PVC and Lipitor (L1 (0.1 mg.l-1) and L2 (1 mg.l-1). A potential list of sensitive (i.e. 1A and 2A) and tolerant/opportunistic (i.e. 1B and 2B) taxa for each type of tested xenobiotic was suggested. However, the nematodes exposed particularly to PS/L1 and PS/L2 treatments were not significantly affected. The interactions between Lipitor and complexing polystyrene can serve as a depollution method to protect living organisms. It is essential to further explore the link between both types of contaminants to mitigate the ecotoxicological risks and for governmental policy purposes. The results obtained in the current study encourage the use of polystyrene for cleaning subtidal marine areas of Lipitor and statins in general, due to its strong adsorbent and electro-magnetic power, but also lightness, which makes the collection of contaminated microplastic particles floating on the water easier.
Data availability statement
The raw data supporting the conclusions of this article will be made available by the authors, without undue reservation.
Ethics statement
The manuscript presents research on animals that do not require ethical approval for their study.
Author contributions
BA: Writing – original draft, Software, Formal analysis, Funding acquisition. MA: Writing – original draft, Software, Funding acquisition, Formal analysis. NA-H: Writing – review & editing, Methodology, Investigation, Conceptualization, Software, Formal analysis. SÖ: Writing – original draft, Funding acquisition, Writing – review & editing. OP: Supervision, Software, Resources, Data curation, Conceptualization, Writing – review & editing. MN: Visualization, Validation, Project administration, Writing – review & editing, Software, Resources, Data curation. EM: Investigation, Conceptualization, Writing – review & editing, Validation, Software. AH: Writing – original draft, Supervision, Formal analysis, Data curation, Writing – review & editing, Software. HR: Project administration, Funding acquisition, Conceptualization, Writing – review & editing, Formal analysis, Data curation. FB: Writing – original draft, Validation, Supervision, Writing – review & editing, Funding acquisition, Conceptualization.
Funding
The authors declare financial support was received for the research, authorship, and/or publication of this article. This work was supported and funded by the Deanship of Scientific Research at Imam Mohammad Ibn Saud Islamic University (IMSIU) (grant number IMSIU-RP23071).
Conflict of interest
The authors declare that the research was conducted in the absence of any commercial or financial relationships that could be construed as a potential conflict of interest.
Publisher’s note
All claims expressed in this article are solely those of the authors and do not necessarily represent those of their affiliated organizations, or those of the publisher, the editors and the reviewers. Any product that may be evaluated in this article, or claim that may be made by its manufacturer, is not guaranteed or endorsed by the publisher.
References
Abuwatfa W. H., Dana A., Al-Othman A., Halalsheh N., Tawalbeh M. (2021). Insights into the removal of microplastics from water using biochar in the era of COVID-19: a mini review. Case Stud. Chem. Environ. Eng. 4, 100151. doi: 10.1016/j.cscee.2021.100151
Allouche M., Ishak S., Ben Ali M., Hedfi A., Almalki M., Karachle P. K., et al. (2022). Molecular interactions of polyvinyl chloride microplastics and beta-blockers (diltiazem and bisoprolol) and their effects on marine meiofauna: combined in vivo and modeling study. J. Hazard. Mat. 431, 128609. doi: 10.1016/j.jhazmat.2022.128609
Avio C. G., Gorbi S., Milan M., Benedetti M., Fattorini D., d’ Errico G., et al. (2015). Pollutants bioavailability and toxicological risk from microplastics to marine mussels. Environ. pollut. 198, 211–222. doi: 10.1016/j.envpol.2014.12.021
Bellakhal M., Ishak S., Al-Hoshani N., Qurtam A. A., Al-Zharani M., Pacioglu O., et al. (2023). The multifaceted effects of fluoranthene and polystyrene on the taxonomic composition and associated functional traits of marine meiofauna, by using single and mixture applications. Mar. pollut. Bull. 194, 115390. doi: 10.1016/j.marpolbul.2023.115390
Borja Á., Franco J., Pérez V. (2000). A marine biotic index to establish the ecological quality of soft bottom benthos within European estuarine and coastal environments. Mar. pollut. Bull. 40, 1100–1114. doi: 10.1016/S0025-326X(00)00061-8
Boufahja F. (2010). Approches communautaires et populationnelles de biosurveillance du milieu marin chez les nématodes libres (lagune et baie de Bizerte, Tunisie). PhD thesis in Biological Sciences, Faculty of Sciences of Bizerte (Tunisia: University of Carthage), 300.
Boufahja F., Hedfi A., Amorri J., Aïssa P., Beyrem H., Mahmoudi E. (2011). Examination of the bioindicator potential of Oncholaimus campylocercoides (Nematoda, Oncholaimidae) from Bizerte bay (Tunisia). Ecol. Indic. 11, 1139–1148. doi: 10.1016/j.ecolind.2010.12.014
Buchanan J. B. (1971). “Measurement of the physical and chemical environment: Sediments,” in Methods for the Study of Marine Benthos. International Biological Programme Handbook No. 16. Eds. Holme N. A., McIntyre A. D. (Blackwell Scientific Publications, Oxford). 334 pp.
Chow O., Köckritz-Blickwede M., Bright A., Hensler M., Zinkernagel A., Cogen A., et al. (2010). Statins enhance formation of phagocyte extracellular traps. Cell Host Microbe 8 5, 445–454. doi: 10.1016/j.chom.2010.10.005
Clarke K. R. (1993). Non-parametric multivariate analyses of changes in community structure. Austral. Ecol. 18, 117–143. doi: 10.1111/j.1442-9993.1993.tb00438.x
Clarke K. R., Glorley R. N. (2001). PRIMER v5: User Manual/Tutorial (Polymouth., UK: PRIMER-E). 91 p.
Coull B. C., Chandler G. T. (1992). Pollution and meiofauna: field, laboratory and mesocosm studies. Oceanogr. Mar. Biol. 30, 191–271.
Dai H., Gao J., Wang Z., Zhao Y., Zhang D. (2020). Behavior of nitrogen, phosphorus and antibiotic resistance genes under polyvinyl chloride microplastics pressures in an aerobic granular sludge system. J. Cean. Prod. 256, 120402. doi: 10.1016/j.jclepro.2020.120402
Engelmann H. D. (1978). Zur dominanzklassifizierung von bodenarthropoden. Pedobiologia 18, 378–380. doi: 10.1016/S0031-4056(23)00612-1
Essid N., Faiza M., Hedfi A., Almalki M., Urkmez D., Boufahja F. (2021). Toxicity of synthetic Endocrine Disrupting Compounds on meiofauna: Estradiol benzoate as a case study. Environ. pollut. 286, 117300. doi: 10.1016/j.envpol.2021.117300
Fabiano M., Danovaro R. (1994). Composition of organic matter in sediment facing a river estuary (Tyrrhenian Sea): relationships with bacteria and microphytobenthic biomass. Hydrobiologia 277, 71–84. doi: 10.1007/BF00016755
Guo Y., Somerfield P. J., Warwick R. M., Zhang Z. (2001). Large-scale patterns in the community structure and biodiversity of free-living nematodes in the Bohai Sea.China. J. Mar. Biolog. Assoc. 81, 755–763. doi: 10.1017/S0025315401004568
Hägerbäumer A., Höss S., Heininger P., Traunspurger W. (2015). Experimental studies with nematodes in ecotoxicology: an overview. J. Nematol. 47 (1), 11–27.
Hedfi A., Ben Ali M., Korkobi M., Allouche M., Harrath A. H., Beyrem H., et al. (2022). The exposure to polyvinyl chloride microplastics and chrysene induces multiple changes in the structure and functionality of marine meiobenthic communities. J. Hazard. Mat. 436, 12916. doi: 10.1016/j.jhazmat.2022.129161
Hedfi A., Ben Ali M., Noureldeen A., Darwish H., Mahmoudi E., Plăvan G., et al. (2021). Effects of benzo(a)pyrene on meiobenthic assemblage and biochemical biomarkers in an Oncholaimus campylocercoides (Nematoda) microcosm. Environ. Sci. pollut. Res. 29, 16529–16548. doi: 10.1007/s11356-021-16885-w
Hedfi A., Boufahja F., Ben Ali M., Aïssa P., Mahmoudi E., Beyrem H. (2013). Do trace metals (chromium, copper and nickel) influence toxicity of diesel fuel for free-living marine nematodes? Environ. Sci. pollut. Res. 20, 3760–3770. doi: 10.1007/s11356-012-1305-2
Ishak S., Allouche M., Nasri A., Harrath A. H., Alwasel S., Plavan G., et al. (2022). The antidepressants amitriptyline and paroxetine induce changes in the structure and functional traits of marine nematodes. Sustainability 14, 6100. doi: 10.3390/su14106100
Jaramillo-Madrid A. C., Abbriano R., Ashworth J., Fabris M., Pernice M., Ralph P. J. (2020). Overexpression of key sterol pathway enzymes in two model marine diatoms alters sterol profiles in phaeodactylum tricornutum. Pharmaceuticals 13, 481. doi: 10.3390/ph13120481
Keppner E. J., Tarjan A. C. (1989). Illustrated key to the genera of free living marine nematodes of the order Enoplida Vol. 77 (USA: U.S. Department of Commerce. NOAA Technical Report NMFS), 1–26.
Keppner E. J., Tarjan A. C. (1991). Illustrated key to the genera of free-living marine nematodes of the order Araeolaimida Vol. 885 (USA: Corporative Extension service. Institute of Federal Agricultural Sciences. University of Florida. Technical Bulletin), 1–18.
Keppner E. J., Tarjan A. C. (1994). Illustrated key to the genera of free-living marine nematodes of the Microlaimoidea and Desmodoroidea (Nematoda, Chromadorida: Chromadorina) Vol. 890 (USA: University of Florida, Institute of Food and Agricultural Sciences. Technical Bulletin), 1–17.
Keppner E. J., Tarjan A. C. (1999). Illustrated key to the genera of free-living marine nematodes in the Superfamily Chromadoroidea - Exclusive of the Chromadoridae. Extension Publication EENY-82 (USA: University of Florida), 39.
Lange J.-P. (2021). Managing plastic waste-sorting, recycling, disposal, and product redesign. ACS Sustain. Chem. Eng. 9, 15722–15738. doi: 10.1021/acssuschemeng.1c05013
Mahmoudi E., Essid N., Beyrem H., Hedfi A., Boufahja F., Vitiello P., et al. (2005). Effects of hydrocarbon contamination on a free-living marine nematode community: results from microcosm experiments. Mar. pollut. Bull. 50, 1197–1204. doi: 10.1016/j.marpolbul.2005.04.018
Menchaca I., Rodriguez J. G., Borja A., Jesus Belzunce-Segarra M., Franco J., Garmendia J. M., et al. (2014). Determination of polychlorinated biphenyl and polycyclic aromatic hydrocarbon marine regional sediment quality guidelines with the European water framework directive. Chem. Ecol. 30, 693–700. doi: 10.1080/02757540.2014.917175
Moens T., Vincx M. (1997). Observations on the feeding ecology of estuarine nematodes. J. Mar. Biol. Ass. U. K. 77, 211–227. doi: 10.1017/S0025315400033889
Moreno M., Semprucci F., Vezzulli L., Balsamo M., Fabiano M., Albertelli G. (2011). The use of nematodes in assessing ecological quality status in the Mediterranean coastal ecosystems. Ecol. Indic. 11, 328–336. doi: 10.1016/j.ecolind.2010.05.011
Nemys (2024) Nemys: World Database of Nematodes. Available online at: https://nemys.ugent.be.
Platt H. M., Warwick R. M. (1983). Free-living Marine Nematodes. Part I. British Enoploids. (London, UK: Cambridge University), 307.
Platt H. M., Warwick R. M. (1988). Free-living marine nematodes. Part II. British Chromadorids. Synopsis of the British fauna (New Series). No. 38: E.J. Brill/W. Backhuys, Leiden.
Ridall A., Ingels J. (2021). Suitability of free-living marine nematodes as bioindicators: status and future considerations. Front. Mar. Sci. 8, 685327. doi: 10.3389/fmars.2021.685327
Sapir A. (2021). Why are nematodes so successful extremophiles? Commun. Integr. Biol. 14, 24–26. doi: 10.1080/19420889.2021.1884343
Schratzberger M., Warr K. J., Rogers S. I. (2007). Functional diversity of nematode communities in the southwestern North Sea. Mar. Environ. Res. 63, 368–389. doi: 10.1016/j.marenvres.2006.10.006
Schratzberger M., Whomersley P., Warr K., Bolam S. G., Rees H. L. (2004). Colonisation of various types of sediment by estuarine nematodes via lateral infaunal migration: a laboratory study. Mar. Biol. 145, 69–78. doi: 10.1007/s00227-004-1302-1
Seinhorst J. W. (1959). A rapid method for the transfer of nematodes from fixative to anhydrous glycerin. Nematologica. 4, 67–69.
Semprucci F., Losi V., Moreno M. (2015). A review of Italian research on free-living marine nematodes and the future perspectives on their use as Ecological Indicators (EcoInds). Mediterr. Mar. Sci. 16, 352. doi: 10.12681/mms.1072
Thistle D., Lambshead P. J. D., Sherman K. M. (1995). Nematode tail-shape groups respond to environmental differences in the deep sea. Vie Milieu 45, 107–115.
Ting M., Whitaker E., Albandar J. (2016). Systematic review of the in vitro effects of statins on oral and perioral microorganisms. Eur. J. Oral. Sci. 124, 4–10. doi: 10.1111/eos.12239
Vitiello P., Dinet A. (1979). Définition et échantillonnage du méiobenthos. Rapp. Commun. Int. Expl. Sci. Mer. Médit. 25, 279–283.
Wakkaf T., Allouche M., Harrath A. H., Mansour L., Alwasel S., Ansari K. G. M. T., et al. (2020). The individual and combined effects of cadmium, Polyvinyl chloride (PVC) microplastics and their polyalkylamines modified forms on meiobenthic features in a microcosm. Environ. pollut. 266, 115263. doi: 10.1016/j.envpol.2020.115263
Wang S., Wang Y., Liang Y., Cao W., Sun C., Ju P., et al. (2020). The interactions between microplastic polyvinyl chloride and marine diatoms: Physiological, morphological, and growth effects. Ecotoxicol. Environ. Saf. 203, 111000. doi: 10.1016/j.ecoenv.2020.111000
Warwick R., Platt H., Somerfield P., Shrewsbury U. K. (1998). Free-living marine nematodes. Part III. British Monhysterida.
Weber A., von Randow M., Voigt A.-L., von der Au M., Fischer E., Meermann B., et al. (2021). Ingestion and toxicity of microplastics in the freshwater gastropod Lymnaea stagnalis: no microplastic-induced effects alone or in combination with copper. Chemosphere 263, 128040. doi: 10.1016/j.chemosphere.2020.128040
Wei W., Huang Q., Sun J., Wang J., Wu S., Ni B. (2019). Polyvinyl chloride microplastics affect methane production from the anaerobic digestion of waste activated sludge through leaching toxic bisphenol-A. Environ. Sci. Technol. 53, 2509–2517. doi: 10.1021/acs.est.8b07069
Wieser W. (1953). Die Besiehung zwischen Mundhchlen-gestalt, Ernährungsweise und. Vorkommen beifreilbenden marinen Nematoden. Ark. Zoop. Ser. 2, 439–484.
Wu Y., Guo P., Zhang X., Zhang Y., Xie S., Deng J. (2019). Effect of microplastics exposure on the photosynthesis system of freshwater algae. J. Hazard. Mat. 374, 219–227. doi: 10.1016/j.jhazmat.2019.04.039
Keywords: polystyrene, polyvinyl chloride, Lipitor, meiofauna, ecotoxicity
Citation: Aldraiwish BM, Alaqeel MM, Al-Hoshani N, Özdemir S, Pacioglu O, Necula M, Milea EC, Hedfi A, Rudayni HA and Boufahja F (2024) Are microplastics efficient remediation tools for removing the statin Lipitor? A laboratory experiment with meiobenthic nematodes. Front. Mar. Sci. 11:1390700. doi: 10.3389/fmars.2024.1390700
Received: 23 February 2024; Accepted: 29 April 2024;
Published: 21 May 2024.
Edited by:
Yohei Shimasaki, Kyushu University, JapanReviewed by:
Gabriel-Ionut Plavan, Alexandru Ioan Cuza University, RomaniaMohamed Nejib Daly Yahia, Qatar University, Qatar
Copyright © 2024 Aldraiwish, Alaqeel, Al-Hoshani, Özdemir, Pacioglu, Necula, Milea, Hedfi, Rudayni and Boufahja. This is an open-access article distributed under the terms of the Creative Commons Attribution License (CC BY). The use, distribution or reproduction in other forums is permitted, provided the original author(s) and the copyright owner(s) are credited and that the original publication in this journal is cited, in accordance with accepted academic practice. No use, distribution or reproduction is permitted which does not comply with these terms.
*Correspondence: Fehmi Boufahja, ZmFib3VmYWhqYUBpbWFtdS5lZHUuc2E=
†These authors have contributed equally to this work