- 1Department of Cell Biology, Institute of Biological Sciences, University of Brasilia, Brasília, Brazil
- 2Department of Pathology and Laboratory Medicine, University of Calgary, Calgary, AB, Canada
- 3Department of Clinical, Toxicological and Bromatological Analysis, School of Pharmaceutical Sciences of Ribeirão Preto, University of São Paulo, Ribeirão Preto, Brazil
- 4School of Dentistry, University of Ribeirão Preto, Ribeirão Preto, Brazil
We report a fatal bacteremia caused by Klebsiella pneumoniae in a 60–70-year-old patient from Brazil. The genomic analysis of three isolates (from blood culture, nasal and anal swabs) showed that the bacteremia was caused by a KPC-2 producing extensively drug-resistant K64-ST11 hypermucousviscous K. pneumoniae (hmKP) harboring several virulence and antimicrobial resistance genes. Although the isolates did not present virulence markers associated with hypervirulent K. pneumoniae (hvKP), they showed invasion and toxicity to epithelial Hep-2 cells; resistance to cell microbicidal mechanisms; and blood and human serum survival, evidencing their pathogenic potential. This study highlights the risk of infection caused by hmKp strains not characterized as hvKP as well as the clinical implications and difficulty of treatment, especially in elderly or immunocompromised patients.
Introduction
Case Presentation
A 60–70-year-old patient, presenting a transverse colon neoplasm, underwent a left hemicolectomy at a tertiary hospital located in Brazil. For 9 months prior to surgery, the patient presented severe diarrhea (five to six episodes a day) and weight loss (14 kg). At anesthetic induction, the patient received venous ciprofloxacin, metronidazole, and tazobactam. Four days later, after discharge from the intensive care unit (ICU), he presented acute kidney injury, systemic arterial hypertension, chronic atrial flutter and suspected septic shock. Explorative laparoscopy showed anastomotic dehiscence and enteric fluid in the peritoneal cavity. The patient was promptly transferred back to the ICU. A blood culture was requested and returned a negative result. At this time, meropenem and amphotericin B were administered as antimicrobial therapy. Two days later, infectious surveillance and dialysis were initiated. For 4 days the patient remained non-febrile, clinically stable and septic shock was considered resolved. Therefore, amphotericin B was discontinued and meropenem was maintained as antibiotic therapy. Five days later, the patient had a cardiac arrest followed by a bradycardia episode. A cardiopulmonary resuscitation with noradrenaline was performed with a positive response. The patient was submitted to mechanical ventilation, and subsequently developed a pulmonary purulent infection in the orotracheal tube. Polymyxin B was introduced as antimicrobial therapy. Eighteen days after hospitalization, the patient showed deterioration in general health, presented bradycardia (not atropine reversible), and eventually died due to cardiac arrest.
Bacterial Isolates
Seventeen days after patient hospitalization, blood, anal and nasal swabs were collected and all three bacterial cultures were positive for K. pneumoniae by the VITEK-2 system (bioMerieux Brasil) (KpBSB-A, KpBSB-B, and KpBSB-C). All isolates showed the hypermucoviscosity phenotype determined by the string test (1).
Bacterial Survival in Blood and Serum
All isolates were used to evaluate their capacity for survival in human blood and serum (2). For this approach, bacterial suspensions previously grown at 37°C in Luria-Bertani (LB) medium for 18 h, were incubated in 550 μL of human blood (107 bacterial cells) and in 550 μL human serum (108 bacterial cells) at 37°C. Aliquots of 10 μL from each inoculum were removed at times 0, 30, 60, and 120 min and plated in Agar MacConkey medium (Kasvi). All plates were incubated at 37°C for 18 h for colony forming unit (CFU) recovery quantification. For the assays, blood and serum were collected from five health donors. All isolates were tested in triplicate for each donor.
The results showed that the CFU recovery increased 2 times (2x) after 30 min of incubation in blood and serum. After 60 min, the recovery decreased in blood for isolates BSB-A and BSB-C, and increased for BSB-B (Figure 1A). All isolates showed an increase in CFU count after 60 min of incubation, and two of them (BSB-A and BSB-C) after 120 min of incubation in human serum (Figure 1B). These results indicate that the isolates were able to survive and grow in human serum and blood.
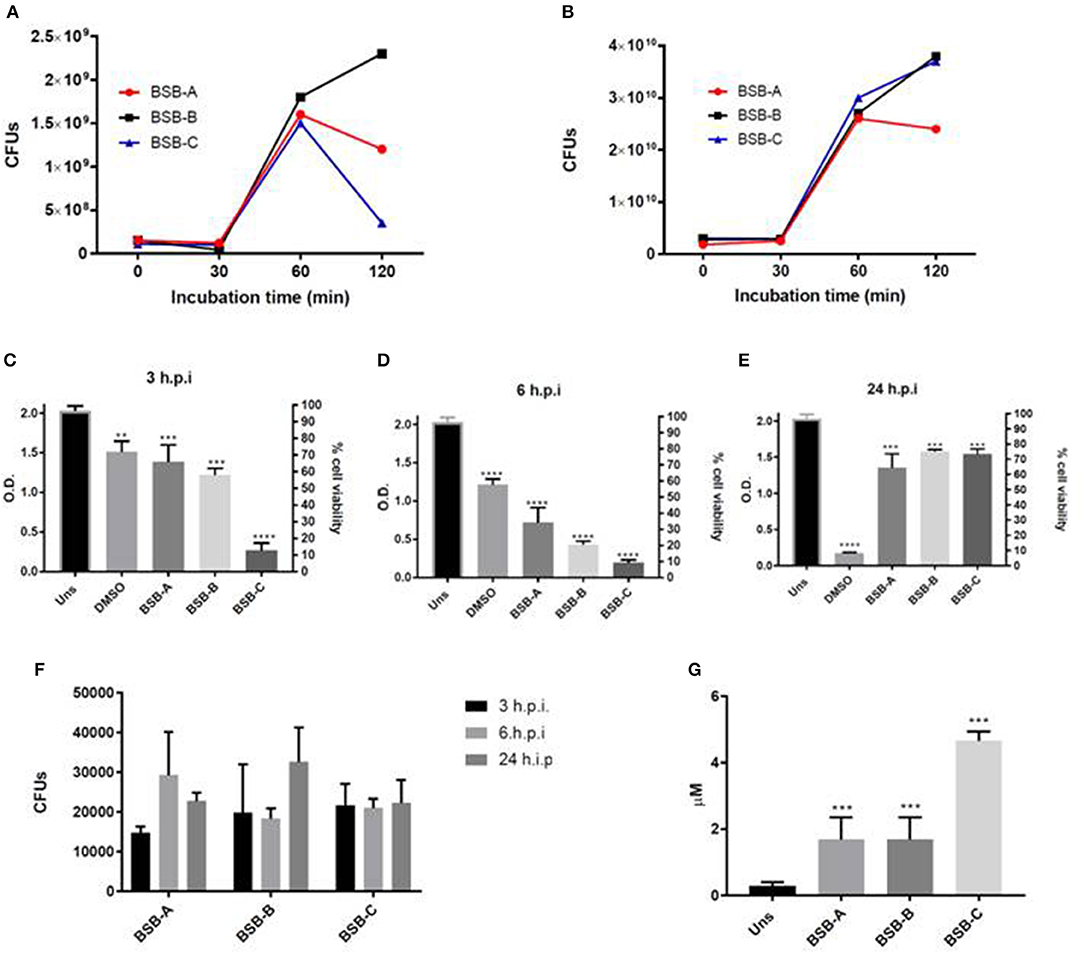
Figure 1. Biological characterization of the three hypermucousviscous Klebsiella pneumoniae isolates (BSB-A; BSB-B, BSB-C). (A) Bacterial blood survival evaluation of CFUs (colony forming units) recovery after isolate incubation in blood for 30, 60, and 120 min. (B) Bacterial human serum survival evaluation of CFUs recovery after isolate incubation in serum for 30, 60, and 120 min. (C–E) Hep-2 cells viability after different periods of infection by K. pneumoniae isolates. (F) Bacterial invasion of Hep-2 cells by K. pneumoniae isolates evaluated by CFUs recovery after 3, 6, and 24 h of infection. (G) Nitric Oxide quantification in Hep-2 cells after 24 h of infection by K. pneumonia isolates. Uns: Unstimulated cells (not submitted to bacterial infection). Control: unstimulated cells (not submitted to bacterial infection). DMSO: positive control for citotoxicity. *p < 0.05; **p < 0.01; ***p < 0.001. All infected cells were compared to unstimulated cells (non infected cells).
Infection of Hep-2 Cells by Bacterial Isolates
Bacterial invasion, cytotoxicity, resistance to epithelial cells and microbicidal mechanisms were evaluated with Hep-2 cells which were infected by the isolates with multiplicity of infection 1:1,000 (Hep-2 cells: isolates) as described by Favre-Bonte et al. (3). Following infection, the Hep-2 cells were incubated at 37°C and 5% CO2. After 30 min, the cells were washed with phosphate-buffered saline and the DMEM (Dulbecco's Modified Eagle's medium) (Gibco) medium was replaced by DMEM with 10 mg/mL of Gentamicin (Sigma Aldrich). Bacterial invasion and intracellular survival were evaluated by CFU quantification at 3, 6, and 24 h post infection (h.p.i.). Bacterial cytotoxicity was determined by MTT (3-(4, 5-dimethylthiazolyl-2)-2, 5-diphenyltetrazolium bromide) assays (Sigma Aldrich) at 3, 6, 24, and 48 h.p.i; and Hep-2 microbicidal mechanisms by nitric oxide (NO) quantification using Griess reagent (Promega) 24 h.p.i. All assays were carried out in triplicate for all isolates.
All isolates showed cytotoxic activity by reduced Hep-2 viability at 3, 6, and 24 h.p.i. (Figures 1C–E). Interestingly, after 24 h.p.i., all Hep-2 infected cells recovered their cell viability. About 15,000–20,000 CFUs were recovered from cells infected by the three isolates at 3 h.p.i. (Figure 1F). For cells infected by KpBSB-A, CFU recovery increased about 2x at 6 h.p.i (30,000) and 0.5x at 24 h.p.i. (2,000) (Figure 1F). For cells infected by KpBSB-B, CFU recovery remains at about 15,000 at 6 h.p.i and increased 1.5x at 24 h.p.i (Figure 1F). Finally, for cells infected by the KpBSB-C isolate, CFU recovery did not present alterations (Figure 1F). These results showed that all isolates were able to invade and survive within Hep-2 cells. Furthermore, KpBSB-A and KpBSB-B also presented growth in Hep-2 cells.
All infected cells showed higher levels of NO at 24 h.p.i. indicating the activity of microbicide mechanisms by Hep-2 cells (Figure 1G). Together with invasion assays (Figure 1F) these results suggest that all isolates are resistant to these microbicidal cell mechanisms.
Antimicrobial Susceptibility Test
Antimicrobial susceptibility tests were performed for 38 different antibiotic disks according to the Clinical & Laboratory Standards Institute (CLSI) (4) and the results showed extensively drug resistant (XDR) isolates (5), remaining susceptible only to aminoglycosides (amikacin, gentamicin, tobramycin, streptomycin and kanamycin). Minimum inhibitory concentration (MIC) was determined for polymyxin B and colistin by the microdilution method in a 96-well plate according to the CLSI (4), and the MIC values obtained for the three isolates were 0.5 μg/mL for both antibiotics, indicating that the isolates were susceptible to them.
PCR Screening for Antimicrobial Resistance Genes and Molecular Typing Methods
Initial PCR screening for antimicrobial resistance genes (6, 7) showed isolates producing blaKPC and blaSHV genes. Plasmid-mediated colistin resistance genes, mcr-1 and mcr-2, were not found (Table 1). According to multilocus sequence typing (MLST) analysis (http://bigsdb.pasteur.fr/klebsiella/klebsiella.html), the isolates belonged to ST11 associated with the clonal group CG258 which is considered an international high-risk sequence type of KPC-producing K. pneumoniae. Enterobacterial Repetitive Intergenic Consensus-PCR (ERIC-PCR) analysis (8) showed the isolates presented 100% genetic similarity (Supplementary Material). The combination of the ERIC-PCR, MLST, and antimicrobial susceptibility results indicated that the three isolates were the same strain.

Table 1. Data on the virulence and resistance genes and plasmid replicons, obtained by draft genome analysis of K. pneumoniae isolates from the study.
Whole Genome Sequence
Two isolates (KpBSB-A and KpBSB-B) were submitted to next-generation sequencing on an Illumina NextSeq500 (150-bp paired-end) using the Nextera XT DNA sample preparation kit (Illumina, San Diego, CA, United States). The obtained sequences were assembled using SPAdes version 3.9.0 (9). The final assembly contained 92 scaffolds (>1,000 bp) and a total length of 5.6 Mb. The genomic sequences enabled the assignment of K. pneumoniae belonging to ST11, confirming our previous observations.
Resistance genes and plasmid replicons were determined in silico using the ResFinder and PlasmidFinder web services, (10, 11) and the Bacterial Isolate Genome Sequence Database (https://pubmlst.org/software/database/bigsdb/) was used to predict pathogenicity. Genome analysis showed that the isolates presented capsular type K64 and detected resistance genes for beta-lactam (blaKPC−2, blaSHV−11), sulfonamide (sul1, sul2), tetracycline (tetA, tetD), and trimethoprim (dfrA1). Also, a plasmid-mediated (qnrS1) and efflux pump complex (oqxA, oqxB) for quinolone resistance were detected. The following plasmid replicons were observed: IncR and ColRNAI, (IncN, IncA/C2, and IncFIB-like) (Table 1). In addition, the search for virulence factors detected genes related to the phenolate siderophore Yersiniabactin (ybtA, ybtE, ybtP, ybtQ, ybtS, ybtT, ybtU, ybtX), irp1, irp2 and fyuA. The irp2 gene is observed in strains derived from urinary infections, bacteremia and other sources, and is considered an important virulence factor for extraintestinal infection establishment (12). Furthermore, mannose-specific adhesin subunit of type 3 fimbriae mrkABCDF and mrkHIJ loci, able to mediate bacterial adhesion and biofilm formation for several surface structures (13) were also detected (Table 1). Previous studies have demonstrated that many strains belonging to ST11 do not present the mucoviscosity-associated genes rpmA/magA (14)
Statistical Analysis
For bacterial blood and serum survival and for Hep-2 bacterial infection assays, the results were analyzed by ANOVA one way using Prism GraphPad software version 6. P ≤ 0.05 were considered significant.
Background
K. pneumoniae is a Gram-negative bacilli associated with opportunistic and nosocomial infections such as pneumonia, meningitis and bacteremia. These strains are named classic K. pnemoniae and have a polyssacharide capsule that promotes their pathogenicty by phagocyte evasion. However, in the last decades, a new variant named hypermucousviscous K. pneumoniae (hmKP) has emerged and this scenario has become extremely complicated by be frequent association with abscess and devastating disseminated infections (15).
The hmKP strains produce a hypercapsule that promotes the ability of bacterial spread and a propensity for metastatic infection (1). Initially, these strains were also considered hypervirulent (named hvKP/hmKP) by being able to infect the non-immunocompromised population. Nowadays, several researches have reported non-hypervirulent hmKP strains (16).
Among hvKP/hmKP strains, hypermucousviscous phenotype is typically due to the increase of production of capsular polysaccharide mediated primarily by the presence of specific genes such as rmpA and magA (17). K1 and K2 are the serotypes most associated with hvKP/hmKP, with K1 ST23 CC23 being considered a clonal group harboring specific virulence factors. These strains are also considered hypervirulent, primarily because of the association with the KPHPI patogenicity island and with virulence factors such as aerobactin, and salmochelin encoded by the pLVPK plasmid (17, 18). K2 isolates were also considered hypervirulent, however they are genetically more diverse (19).
Besides K1 and K2 serotypes, the virulence markers associated with hvKP/hmKP strains are rmpA, rmpA2, aerobactin, yersiniabactin, pld1, KpnO porin and a higher content of capsular sialic acid. Despite hvKP/hmKP not belonging to K1 or K2 and/or not harboring the rmpA/rmpA2 genes, they represent a puzzle regarding the genetic factors responsible for their particular phenotype (16). At the same time, evidence suggests that hmKP strains may harbor other virulence factors that promote their survival and spread in the host (16).
The prevalence of antibiotic resistance in hmKP and hvKP/hmKP strains is rare compared with the high prevalence presented by classic K. pneumoniae isolates (15, 17). However, reports of antibiotic-resistant hmKP and hvKP/hmKP are increasing worldwide and multidrug-resistance has been reported in Asia, Europe and America (17). Difficulties on managing carbapenem-resistant hmKP and hmKP/hvKP infections could turn these strains into the next worldwide “superbug” (17). Here we characterized three hmKP KPC-XDR isolates from a patient with bacteremia after a sepsis due to hemicolectomy surgery. The bacteremia was fatal and the isolates were obtained from blood, nasal and anal swabs. The results here obtained can contribute to understanding of the pathogenic mechanisms related to hmKP.
Discussion
Sepsis is a common complication observed after colectomy surgery among elderly persons (20). An implication of this scenario is surgical site infections, where K. pneumoniae is one of the most likely organisms to be encountered (21). These infections are successfully treated by antibiotic therapy, however the presence of resistant or multidrug resistant strains can be associated with bloodstream bacterial dissemination (22). Here we reported a case of a sepsis episode in a 60–70-year-old patient, after a hemicolectomy surgery followed by bacteremia caused by a KPC-2 XDR K. pneumoniae strain.
Four days after presenting sepsis the patient remained non-febrile and clinically stable and the sepsis episode was considered resolved. However, the bacterial infection showed high progression and after 15 days, and K. pneumoniae were isolated from blood, nasal and anal cultures. All isolates were identified as KPC-XDR, ST11, and presented the same ERIC-PCR profile (Supplementary Material) and hypermucousviscous phenotype, suggesting that the bacteremia was caused by one strain presenting high spread potential.
The anastomotic dehiscence and enteric fluid in the peritoneal cavity indicate that the source of bacteremia could be associated with the surgical site infection due to the colectomy, where the XDR-KPC strain could be present in the intestine of the patient. On the other hand, the pulmonary purulent infection in the orotracheal tube developed after mechanical ventilation suggests that bacteremia could be acquired due to hospital equipment. KPC infections have been reported in hospitals in Brazil, including those located in the geographic area of the isolates (23). However, we cannot confirm these hypotheses because we do not have previous bacterial culture from the patient and/or other isolates studied from the ICU hospital.
Previous case reports from bacteremia caused by XDR K. pneumoniae strains showed that these infections were mostly observed in elderly patients, mainly among those exposed to invasive procedures harboring malignant and chronic diseases (24–26). As observed in our report, the strains belonged to high spreading STs such as ST258 (26) and ST11 (25), which we frequently reported as causing bloodstream infections (BSI). MLST analysis showed that the isolates belonged to ST11 CG258. ST11 is a high-risk clone associated with KPC dissemination and is commonly found in Brazil. This ST has been associated with dissemination of KPC-KP by hmKP and hvKP strains from different regions of Brazil (27). In our case report, we also identified several virulence markers, primarily iron capitation systems frequently associated with hypervirulent K. pneumoniae (15). The association between the risk of KPC and multidrug resistance dissemination makes this ST even more worrying.
XDR- K. pneumoniae BSI are considered a huge health concern because of the low or absent options for treatment. Genomic analysis showed that strains harbored beta-lactamases (SHV), carbapemases (KPC-2) and genes conferring resistance to fosfomicin (fosA), sulfamtoxazole (sul1, sul2), trimethoprim (dfrA1), tetracycline (tetDb) and to quinolones (oqxA, oqxB,: qnrS1) (Table 1). This analysis confirmed the resistance profile detected by antimicrobial susceptibility: that bacteremia was caused by an XDR K. pneumoniae, and aminoglycosides could be the unique option for therapy. However the negative result obtained from the first blood culture and the patient's clinical stability had masked the KPC-XDR infection. Therefore, meropenen prescription was continued and may contribute to the spread of infection. For sepsis, clinical symptoms frequently manifest in the absence of a positive culture resulting in diagnosis delay (28). As observed here, negative culture contributed to the wrong antibiotic prescription and was fatal to the patient. Periodical blood collection for bacterial culture could be an approach used for similar cases in order to detect silent infections, mainly in areas with previous KPC K. pneumoniae reports.
For BSI, monotherapy has been considered low efficacy and antibiotic combinations have shown better effectiveness due to synergic action (29). Therefore, carbapenem monotherapy should be avoided, manly if its resistance was previously reported in the hospital. Triple combination with polymyxin-colistin has shown improved efficacy to carbapenamases-producing K. pneumoniae infections treatment (29). On the other hand the hypercapsule of the isolates could be an obstacle to this approach since the increase of capsular polysaccharide production has been associated with polymyxin susceptibility reduction (30).
All isolates were classified as K64, a capsular serotype not yet reported with hypervirulence. Furthermore, these strains did not present several molecular virulence markers associated with hvKP strains (16). However, all isolates presented abilities to invade epithelial cells, to resist their microbicidal mechanisms, and showed cytotoxic potential (Figures 1C–E). These features suggest that the isolates harbor factors that promote epithelial host cells colonization and infection dissemination. Additionally, it has been shown that this strain may carry different virulence determinants from those already described for hmKP/hvKP. More characterization is needed to identify whether the strain here studied is also an hvKP with pathogenicity mechanisms not yet described.
The diagnosis difficulties after a sepsis episode, the high pathogenic potential presented by the isolates, the antibiotic resistance promoted by genes and by hypercapsule production, and the patient's debility contributed to the fatal character of the bacteremia. Moreover, the high prevalence of KPC-2 in Brazil demonstrates the high spread and colonization capacity presented by these strains (31). Considering all these variants, immunization programs for high-risk patients can be a precious tool to decrease the fatal potential of XDR-KPC K. pneumoniae. Successful immunization approaches such as the Egyptian vaccine offered broad spectrum coverage of more than 85% to all tested isolates (32) and can be a good alternative to improve the outcome for a high risk patient.
Concluding Remarks
In summary, we described a fatal case of a hypermucousviscous KPC-2 producing XDR ST11 K. pneumoniae, which occurred in Brazil. Resistance to carbapenems and KPC-2 are extensively reported in K. pneumoniae isolates from this region (30). As shown by the antimicrobial susceptibility test results, the use of aminoglycosides could have been a good therapeutic choice. However, the initial patient clinical stability, after meropenem treatment for sepsis control, may have hidden the bacteremia progress by XDR-K. pneumoniae. Moreover, the virulence may have also contributed to the bacteremia evolution. Periodical blood collection for bacterial culture could be an approach used for similar cases in order to detect silent infections, mainly in areas with previous KPC K. pneumoniae infection reports.
This Whole Genome Shotgun project has been deposited in ENA (European Nucleotide Archive) under the accession number PRJEB24576.
Ethics Statement
Ethical approval was received from the Faculdade de Ciências Farmacêuticas de Ribeirão Preto, Universidade de São Paulo, Ribeirão Preto, SP, Brazil [approval no:CEP/FCFRP 362; CAEE 36031914.9.0000.5403] and from the Faculdade de Medicina, Universidade de Brasília, Brasília, DF, Brazil [approval no. CEP/FMUnB 1.131.054;CAEE: 44867915.1.0000.558]. Extensive efforts have been made to obtain consent for the publication of this case report from the next of kin; however, as this could not be obtained, this case report has been thoroughly de-identified.
Author Contributions
TC and AP-S conceived, designed the experiments and wrote the paper. LG and VP performed blood and serum survival assays. LG and KM performed Hep-2 cell infection assays. GG, JF, and ES performed the antimicrobial susceptibility tests, PCR and molecular typing methods. GP, JP, and GPJ performed the whole genome sequence analysis.
Conflict of Interest Statement
The authors declare that the research was conducted in the absence of any commercial or financial relationships that could be construed as a potential conflict of interest.
Acknowledgments
We are thankful for the financial support from Fundação de Amparo à Pesquisa do Estado de São Paulo-FAPESP [grant number 2013/22581-5] and Fundação de Apoio ao Desenvolvimento Científico e Tecnológico do Hospital Universitário de Brasília-FAHUB [grant number 01/2015]. We also would like to thank John Carpenter (Ribeirão Preto, SP, Brazil) for the English revision.
Supplementary Material
The Supplementary Material for this article can be found online at: https://www.frontiersin.org/articles/10.3389/fmed.2018.00265/full#supplementary-material
Supplementary Material. Agarose gel (1.5% w/v) electrophoresis with ERIC-PCR (Enterobacterial Repeats Intergenic Consensus Sequences–PCR) profile. 1: 1 Kb Ladder (Gibco); 2: BSB-A ERIC-profile; 3: BSB-B ERIC-profile; 4: BSB-C ERIC-profile.
References
1. Shon AS, Bajwa RPS, Russo TA. Hypervirulent (hypermuscoviscous) Klebsiella pneumoniae: a new and dangerous breed. Virulence (2013) 4:107–13. doi: 10.4161/viru.22718
2. DeLeo FR, Kobayashi SD, Porter AR, Freedman D, Dorward DW, Chen L, et al. Survival of carbapenem-resistant Klebsiella pneumonia sequence type 258 in human blood. Antimicrob Agents Chemother. (2017) 61:e02533–16. doi: 10.1128/AAC.02533-16
3. Favre-Bonte S, Bernand J, Forestier C. Consequences of reduction of Klebsiella pneumoniae capsule expression on interactions of this bacterium with epithelial cells. Infect Immun. (1999) 67:554–61.
4. CLSI. Performance Standards for Antimicrobial Susceptibility Testing, M100-S26. Twenty-Sixth Informational Supplement. Wayne PA: Clinical and Laboratory Standards Institute (2016).
5. Magiorakos AP, Srinivasan A, Carey RB, Carmeli Y, Falagas ME, Giske CG, et al. Multidrug-resistant, extensively drug-resistant and pandrug-resistant bacteria: an international expert proposal for interim standard definitions for acquired resistance. Clin Microbiol Infect. (2012) 18:268–81. doi: 10.1111/j.1469-0691.2011.03570.x
6. Dallenne C, Da Costa A, Decré D, Favier C, Arlet G. Development of a set of multiplex PCR assays for the detection of genes encoding important beta-lactamases in Enterobacteriaceae. J Antimicrob Chemother. (2010) 65:490–5. doi: 10.1093/jac/dkp498
7. Doyle D, Peirano G, Lascols C, Lloyd T, Church DL, Pitout JD. Laboratory detection of Enterobacteriaceae that produce carbapenemases. J Clin Microbiol. (2012) 50:3877–80. doi: 10.1128/JCM.02117-12
8. Versalovic J, Schneider M, De Bruijn FJ, Lupski JR. Genomic fingerpriting of bacteria using repetitive sequence-based polymerase chain reaction. Met Mol Cell Biol. (1994) 5:25–40.
9. Nurk S, Bankevich A, Antipov D, Gurevich AA, Korobeynikov A, Lapidus A, et al. Assembling single-cell genomes and mini-metagenomes from chimeric MDA products. J Comput Biol. (2013) 20:714–37. doi: 10.1089/cmb.2013.0084
10. Zankari E, Hasman H, Cosentino S, Vestergaard M, Rasmussen S, Lund O, et al. Identification of acquired antimicrobial resistance genes. J Antimicrob Chemother. (2012) 67:2640–4. doi: 10.1093/jac/dks261
11. Carattoli A, Zankari E, Garcia-Fernandez A, Voldby Larsen M, Lund O, Villa L, et al. In silico detection and typing of plasmids using PlasmidFinder and plasmid multilocus sequence typing. Antimicrob Agents Chemother. (2014) 58:3895–903. doi: 10.1128/AAC.02412-14
12. Souza Lopes AC, Rodrigues JF, Cabral AB, da Silva ME, Leal NC, da Silveira VM, et al. Occurrence and analysis of irp 2 virulence gene in isolates of Klebsiella pneumoniae and Enterobacter spp. from microbiota and hospital and community-acquired infections. Microb Pathog (2016) 96:15–19. doi: 10.1016/j.micpath.2016.04.018
13. Wilksch JJ, Yang J, Clements A, Gabbe JL, Short KR, Cao H, et al. MrkH, a novel c-di-GMP-dependent transcriptional activator, controls Klebsiella pneumoniae biofilm formation by regulating type 3 fimbriae expression. PloS Pathog. (2011) 7:e1002204. doi: 10.1371/journal.ppat.1002204
14. Arena F, De Angelis LH, Cannatelli A, Fossati L, Di Pilato V, Giani T, et al. Infections caused by carbapenem-resistant Klebsiella pneumoniae with hypermucoviscous phenotype: a case report and literature review. Virulence (2017) 8:1900–08. doi: 10.1080/21505594.2017.1286439
15. Paczosa MK, Mecsas J. Klebsiella pneumoniae: going on the offense with a strong defense. Microbiol Mol Rev. (2016) 15:629–61. doi: 10.1128/MMBR.00078-15
16. Catalán-Nájera JC, Garza-Ramos U, Barrio s-Camacho H. Hypervirulence and hypermucoviscosity: two different but complemantery Klebsiella spp. phenotypes? Virulence (2017) 8:1111–23. doi: 10.1080/21505594.2017.1317412
17. Lee C-R, Lee JH, Park KS, Jeon JH, Kim YB, Chang-Jun C, et al. Antimicrobial resistance of hypervirulent Klebsiella pneumoniaie: edpidemiology, hypervirulence-associated determinants, and resistance mechanisms. Front Cell Infect Microbiol. (2017) 7:483. doi: 10.3389/fcimb.2017.00483
18. Struve C, Roe CC, Stegger M, Stahlhut SG, Hansen DS, Engelthaler DM, et al. Mapping the evolution of hypervirulent Klebsiella pneumoniae. MBio (2015) 6:e00630–15. doi: 10.1128/mBio.00630-15
19. Bialek-Davenet S, Criscuolo A, Ailloud F, Passet V, Jones L, Delannoy-Vieillard A-S, et al. Genomic definition of hypervirulent and multidrug-resistant Klebsiella pneumoniae clonal groups. Emerg Infect Dis. (2014) 20:1812–20. doi: 10.3201/eid2011.140206
20. Podnos YD, Jimenes JC, Wilson SE. Intra-abdominal sepsis in elderly persons. Clin Infect Dis. (2002) 35:62–68. doi: 10.1086/340866
21. Fry DE. The prevention of surgical site infection in elective colon surgery. Scientifica (2013).2013:896297. doi: 10.1155/2013/896297
22. Girometti N, Lewis RE, Gianella M, Ambretti S, Bartoletti M, Tedeschi S, et al. Klebsiella pneumonia bloodstream infection: epidemiology and impact of inappropriated empirical therapy. Med (Baltimore) (2014) 93:298–309. doi: 10.1097/MD.0000000000000111
23. Sampaio JLM, Gales AC. Antimicrobial resistance in Enterobacteriaceae in Brazil: focus on β-lactams and polymyxins. Braz J Microbiol. (2016) 7(Suppl. 1):31–37. doi: 10.1016/j.bjm.2016.10.002
24. Zhao F, Zhang J, Fu Y, Ruan Z, Xie X. Dissemination of extensively drug-resistant and KPC-2 producing Klebsiella pneumoniae isolated from bloodstream infections. J Infect Dev Ctries. (2015). 9:1016–21. doi: 10.3855/jidc.6679
25. Bi W, Liu H, Dunstan RA, Li B, Torres VVL, Cao J, et al. Extensively drug-resistant Klebsiella pneumoniae causing nosocomial bloodstream infections in China: molecular investigation of antibiotic resistance determination, informing therapy, and clinical outcomes. Front Microbiol. (2017) 8:1230. doi: 10.3389/fmicb.2017.01230
26. Krapp F, Ozer EA, Qi C, Hauser AR. Case report of an extensively drug-resistant Klebsiella pneumoniae infection with genomic characterization of the strain and review of similar cases in the United States. Open Forum Infect. Dis. (2018). 5:ofy074. doi: 10.1093/ofid/ofy074
27. Araújo BF, Ferreira ML, Campos PA, Royer S, Gonçalves IR, da Fonseca Bastião DW, et al. Hypervirulence and biofilm production in KPC-2-producing Klebsiella pneumoniaie CG258 isolated in Brazil. J Med Microbiol. (2018). 67:523–8. doi: 10.1099/jmm.0.000711
28. Jacobs DM, Safir MC, Huang D, Minhaj F, Parker A, Rao GG. Triple combination antibiotic therapy for carbapenemase-producion Klebsiella pneumoniae: a systematic review. Ann Clin Microbiol Antimicrob. (2017) 16:76. doi: 10.1186/s12941-017-0249-2
29. Carrigan SD, Scott G, Tabrizian M. Toward resolving the challenges of sepsis diagnosis. Clin Chem. (2004) 50:1301–14. doi: 10.1373/clinchem.2004.032144
30. Llobet E, Tomás JM, Bengoechea JA. Capsule polysaccharide is a bacterial decoy for antimicrobial peptides. Microbiology (2008) 154:3877–86. doi: 10.1099/mic.0.2008/022301-0
31. Biberg CA, Rodrigues AC, do Carmo SF, Chaves CE, Gales AC, Chang MR. KPC-2-producing Klebsiella pneumoniae in a hospital in the Midwest region of Brazil. Braz J Microbiol. (2015) 46:501–4. doi: 10.1590/S1517-838246246220140174
Keywords: Klebsiella pneumoniae, hypermucousviscous, KPC-2, XDR, ST11, virulence, antimicrobial resistance
Citation: de Campos TA, Gonçalves LF, Magalhães KG, de Paulo Martins V, Pappas Júnior GJ, Peirano G, Pitout JDD, Gonçalves GB, Furlan JPR, Stehling EG and Pitondo-Silva A (2018) A Fatal Bacteremia Caused by Hypermucousviscous KPC-2 Producing Extensively Drug-Resistant K64-ST11 Klebsiella pneumoniae in Brazil. Front. Med. 5:265. doi: 10.3389/fmed.2018.00265
Received: 27 April 2018; Accepted: 31 August 2018;
Published: 21 September 2018.
Edited by:
Dongsheng Zhou, Beijing Institute of Microbiology and Epidemiology, ChinaReviewed by:
Tarek Adnan Ahmad, Bibliotheca Alexandrina, EgyptGeorge Dimopoulos, University General Hospital Attikon, Greece
Copyright © 2018 de Campos, Gonçalves, Magalhães, de Paulo Martins, Pappas Júnior, Peirano, Pitout, Gonçalves, Furlan, Stehling and Pitondo-Silva. This is an open-access article distributed under the terms of the Creative Commons Attribution License (CC BY). The use, distribution or reproduction in other forums is permitted, provided the original author(s) and the copyright owner(s) are credited and that the original publication in this journal is cited, in accordance with accepted academic practice. No use, distribution or reproduction is permitted which does not comply with these terms.
*Correspondence: Tatiana Amabile de Campos, dGF0aWFuYWRlY2FtcG9zQHVuYi5icg==