- 1Service of General Internal Medicine, Geneva University Hospitals (HUG), Geneva, Switzerland
- 2Genomic Research Laboratory, Service of Infectious Diseases, Geneva University Hospitals, Geneva, Switzerland
- 3Bacteriology Laboratory, Service of Laboratory Medicine, Geneva University Hospitals, Geneva, Switzerland
- 4Service of Infectious Diseases, Geneva University Hospitals, Geneva, Switzerland
- 5Institute of Medical Microbiology, University of Zurich, Zurich, Switzerland
- 6Service of Cardiovascular Surgery, Geneva University Hospitals, Geneva, Switzerland
Diagnosis of culture-negative infective endocarditis usually implies indirect pathogen identification by serologic or molecular techniques. Clinical metagenomics, relying on next-generation sequencing (NGS) is an emerging approach that allows pathogen identification in challenging situations, as evidenced by a clinical case. We sequenced the DNA extracted from the surgically-removed frozen valve tissue from a patient with suspected infective endocarditis with negative blood and valve cultures. Mapping of the sequence reads against reference genomic sequences, a 16S rRNA gene database and clade-specific marker genes suggested an infection caused by Cardiobacterium hominis.
Pathogen identification is a cornerstone for the diagnosis of infective endocarditis (IE) and guides treatment duration and antibiotic choice. Yet, in up to 31% of patients with IE, cultures performed on blood and valve remain negative (1). Previous antibiotic treatment and fastidious growth requirements are main reasons for failure of microorganism identification (2). Bacteria of the HACEK group, intracellular germs or nutritionally variant streptococci require particular conditions for the growth in the laboratory, such as specific complex media or lysis-centrifugation blood culture system (2).
Broad-range PCR amplification which screens for bacterial pathogens using universal primers provides an adjunct in diagnosis of IE. Multimodal diagnostic assays, including serologies and specific real-time PCR amplification, further increase the diagnostic yield in blood-culture negative IE (3, 4).
Recently, metagenomic approach using high-throughput culture-independent techniques based on next-generation sequencing (NGS) has emerged and opened new perspectives for microbiological diagnosis in the clinical setting. Here, we report the identification of Cardiobacterium hominis by NGS in the vegetation of a culture negative IE, in which broad-range PCR amplification did not detect any microorganism.
In May 2018, a 58-year-old man, independent painter, consulted the emergency room complaining about breathlessness, chest tightness and chills for a couple of weeks. He had a good dental status with no history of recent dental procedure. Physical examination revealed signs of heart failure with no splenomegaly, peripheral embolic lesions, large-vessel emboli, cutaneous lesions, or retinal/conjunctival lesions. Transthoracic and then transesophageal echocardiographies showed a moderate to severe aortic regurgitation and a 15 × 8 cm mobile vegetation on the right leaflet. Laboratory tests showed leukocyte count 12.4 × 109 cells/L (reference range 4–11 × 109 cells/L), hemoglobin 130 g/L (reference range 140–180 g/L) and C-reactive protein at 87.3 mg/L (reference range 0–10 mg/L). Suspecting an infective endocarditis, empiric treatment was started with amoxicillin, amoxicillin/clavulanic acid and gentamicin (day 0). On day 3, an aortic valve replacement was performed, and a biological valve was placed without complications. Before pathogen identification, antibiotic treatment was already adjusted by probabilistic means to ceftriaxone, a third-generation cephalosporin. After detection of C. hominis, this regimen was pursued for a total duration of 6 weeks. The patient recovered well and went back to his former performance status.
Five sets of blood cultures, taken at 30 min intervals on day 0, i.e., before antibiotic treatment, remained negative on day 7. Culture of the excised valve and vegetation on standard enriched media came back negative. Results of indirect detection of infective agents were also negative. These included: (i) Specific PCR tests for Chlamydophila pneumoniae, Mycoplasma pneumoniae, and Bordetella spp. in oropharyngeal samples, Treponema pallidum, Tropheryma whipplei, Brucella spp., Bartonella henselae, and Bordetella quintana in the heart-valve and Brucella spp. in blood; (ii) Legionella pneumophila urinary antigen test, and (iii) serologies for Coxiella burnetti, Bartonella henselae, and Brucella spp.
The broad-range 16S rDNA PCR performed on a fragment of the aortic valve revealed negative. A portion of the valve was further analyzed by clinical metagenomics (Supplementary Methods). DNA was extracted from two fragments (S1 and S2) of the valve specimen using a procedure that removes a significant amount of human DNA in order to allow deeper assessment of the bacterial component by NGS (5–8). One of the two fragments (S2) was mechanically grinded prior to DNA extraction. DNA quantification by qPCR revealed higher bacterial and lower human DNA concentrations in the mechanically pre-treated valve fragment as compared to the sample without this pre-treatment (Table 1). To identify bacterial species in the valve specimen, we performed NGS of both DNA extracts and analyzed sequence data by classifying sequence reads against: (i) reference genomic sequences using CLARK (9); (ii) clade-specific marker genes with MetaPhlAn2 (10), and (iii) curated 16S rRNA gene database (11) with mothur (12) and UBLAST (13). The results obtained by either approach suggested an infection caused by Cardiobacterium hominis (Figure 1; Supplementary Table 1). The reads assigned to this species were by far the most abundant (>97%) among the bacterial reads, as identified by CLARK. Similarly, most (>99.2%) reads that mapped to the 16S rRNA genes belonged to genus Cardiobacterium. Cardiobacterium was the only genus identified by mapping of reads against clade-specific markers, with a dominance of C. hominis for both DNA extracts. Cardiobacterium was not identified by sequencing of negative extraction controls (NEC1 and NEC2) by any of the three analysis methods used.
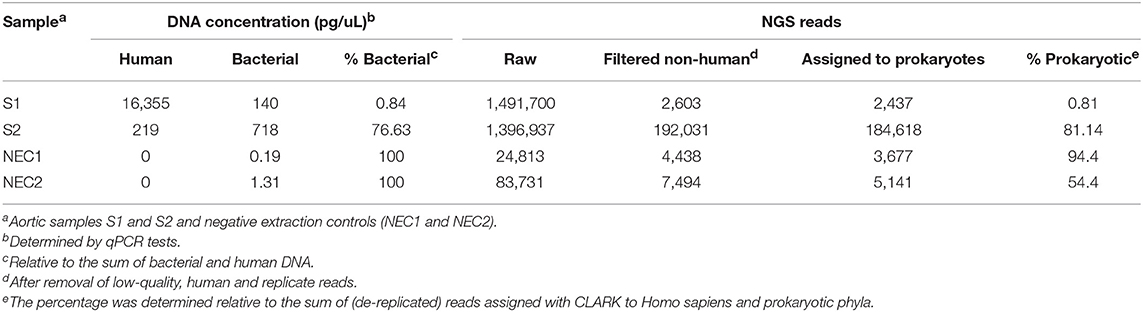
Table 1. Load of bacterial and human DNA in DNA extracts of the heart valve and in negative controls determined by qPCR and NGS.
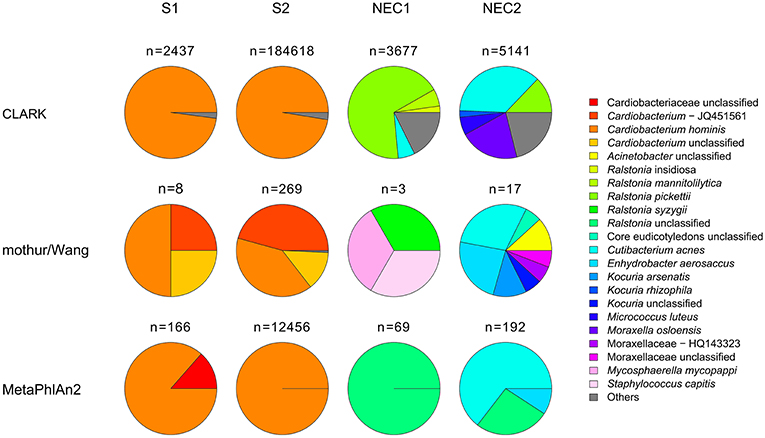
Figure 1. Relative abundance of prokaryotic taxa determined by classification of genome fragment sequences (CLARK), classification of 16S rRNA gene fragments (mothur/Wang) and read mapping to clade markers (MetaPhlAn2). All taxa with relative abundance <1% were summed up and represented as “others.” For simplicity, in the mothur/Wang approach, only results obtained with forward sequence reads are presented. n, number of hits obtained for each sample and method used.
Herein, we present the case of a culture-negative IE in which the all-round standard multimodal diagnostic work-up, aimed at identifying causative agents, came back negative. The final diagnosis was achieved by NGS of the aortic native valve DNA extract, which identified C. hominis, a member of the HACEK group that accounts for 1.2–3% of IE cases (14). Only 61 cases of C. hominis IE have been reported in the English-language literature between 1962 and 2005 (15).
C. hominis is a facultatively anaerobic, slow-growing, fastidious, non-motile, gram-negative rod of low virulence, commonly present in normal oral and nasal human microbiota. It is the third most common agent responsible for HACEK endocarditis (14). Failure to identify C. hominis in blood cultures, which were taken before antibiotic treatment of our patient, may be ascribed to the fastidious nature of the organism.
In previous studies, testing heart valves for bacterial pathogens in patients with suspected IE revealed higher specificity of broad-range PCR as compared to standard cultures, even though some patients with positive cultures tested negative by PCR (16, 17). Various reasons have been attributed to false negative broad-range PCR results: low bacterial load due to the time lapsing between antibiotic therapy and surgical intervention, use of a non-representative valve fragment (with low local bacterial density), and PCR inhibition (17) which may depend on DNA extraction procedure and PCR conditions. In our case, antibiotic treatment could be a possible cause of the culture-negative results of the valve tissue but unlikely to have caused failure in pathogen detection by PCR, because bacterial DNA load (which may originate from either live or dead cells) in the extracts of valve fragments used for NGS was relatively high (>2 × 106 and >107 rRNA gene copies for S1 and S2, respectively; Table 1). It is more likely that the broad-range PCR detection was compromised by the presence of PCR inhibitors. Yet, a sampling bias cannot be ruled out, since broad-range PCR and NGS assessed different specimen fragments. In addition, it has been recently shown that specific steps in sample preparation of the valve specimen may affect the diagnostic yield (18).
In a previous study, Imai et al. found good correlation between NGS and culture data obtained on the native valve from two patients with blood culture-positive endocarditis (19). Two other cases of blood culture-negative endocarditis were reported where the pathogen could be identified by NGS (19, 20). In our case, the identification of the causative agent of IE by NGS was reinforced by the use of three different bioinformatics tools relying on different reference databases. We believe this point is of crucial importance and guarantees for a curated and redundant signal and therefore a more reliable diagnosis.
Currently, the application of NGS approach in routine diagnostic testing is relatively costly unless larger batches of samples are processed. The application of NGS to various samples is of increasing clinical interest because it allows faster pathogen identification than standard culture. A complete metagenomics analysis of a clinical sample is now possible in <30 h using Illumina sequencing platforms. This turn-around time is longer than that of PCR and qPCR assays; however, it circumvents the need for multiple assays, e.g. target-specific PCRs aimed at confirming broad range PCR results (21), and has the potential to (i) detect multiple organisms (including uncommon pathogens), (ii) perform bacterial typing and (iii) predict antibiotic resistance profile (6, 22), all in the same test. Mechanical pre-treatment of valve tissue followed by the removal of DNA from selectively lysed human cells seem to increase the likelihood of pathogen identification. NGS of cell-free circulating plasma or serum DNA is another promising diagnostic tool for the detection of bacteremia in patients with IE (23). Our case clearly demonstrates that there is a lack in conventional techniques and that there is a clinical benefit to perform NGS in selected situations such as culture- and PCR-negative results. In particular the implementation of multiple analysis approaches and the use of orthogonal assessment to validate the obtained results should be promoted further.
Data Availability
The datasets generated for this study can be found in European Nucleotide Archive (ENA), PRJEB25228.
Ethics Statement
Written informed consent has been obtained from the patient for the publication of this case report.
Author Contributions
MK, VL, SE, ACa, ACh, CH, and JS analyzed and interpreted patient data. MG, NG, PK, and ACh performed the experiments. NG and VL analyzed the metagenomics data. MK, VL, YC, and JS wrote the manuscript. All authors read and approved the final manuscript.
Conflict of Interest Statement
The authors declare that the research was conducted in the absence of any commercial or financial relationships that could be construed as a potential conflict of interest.
Supplementary Material
The Supplementary Material for this article can be found online at: https://www.frontiersin.org/articles/10.3389/fmed.2019.00203/full#supplementary-material
References
1. Habib G, Lancellotti P, Antunes MJ, Bongiorni MG, Casalta JP, Del Zotti F, et al. 2015 ESC guidelines for the management of infective endocarditis: The Task Force for the Management of Infective Endocarditis of the European Society of Cardiology (ESC). Endorsed by: European Association for Cardio-Thoracic Surgery (EACTS), the European Association of Nuclear Medicine (EANM). Eur Heart J. (2015) 36:3075–128. doi: 10.1093/eurheartj/ehv319
2. Mylonakis E, Calderwood SB. Infective endocarditis in adults. N Engl J Med. (2001) 345:1318–30. doi: 10.1056/NEJMra010082
3. Raoult D, Casalta JP, Richet H, Khan M, Bernit E, Rovery C, et al. Contribution of systematic serological testing in diagnosis of infective endocarditis. J Clin Microbiol. (2005) 43:5238–42. doi: 10.1128/JCM.43.10.5238-5242.2005
4. Fournier PE, Gouriet F, Casalta JP, Lepidi H, Chaudet H, Thuny F, et al. Blood culture-negative endocarditis: improving the diagnostic yield using new diagnostic tools. Medicine. (2017) 96:e8392. doi: 10.1097/MD.0000000000008392
5. Leo S, Gaïa N, Ruppé E, Emonet S, Girard M, Lazarevic V, et al. Detection of bacterial pathogens from broncho-alveolar lavage by next-generation sequencing. Int J Mol Sci. (2017) 18:E2011. doi: 10.3390/ijms18092011
6. Lazarevic V, Gaia N, Girard M, Leo S, Cherkaoui A, Renzi G, et al. When bacterial culture fails, metagenomics can help: a case of chronic hepatic brucelloma assessed by next-generation sequencing. Front Microbiol. (2018) 9:1566. doi: 10.3389/fmicb.2018.01566
7. Horz HP, Scheer S, Huenger F, Vianna ME, Conrads G. Selective isolation of bacterial DNA from human clinical specimens. J Microbiol Methods. (2008) 72:98–102. doi: 10.1016/j.mimet.2007.10.007
8. Horz HP, Scheer S, Vianna ME, Conrads G. New methods for selective isolation of bacterial DNA from human clinical specimens. Anaerobe. (2010) 16:47–53. doi: 10.1016/j.anaerobe.2009.04.009
9. Ounit R, Wanamaker S, Close TJ, Lonardi S. CLARK: fast and accurate classification of metagenomic and genomic sequences using discriminative k-mers. BMC Genomics. (2015) 16:236. doi: 10.1186/s12864-015-1419-2
10. Truong DT, Franzosa EA, Tickle TL, Scholz M, Weingart G, Pasolli E, et al. MetaPhlAn2 for enhanced metagenomic taxonomic profiling. Nat Methods. (2015) 12:902–3. doi: 10.1038/nmeth.3589
11. Yoon SH, Ha SM, Kwon S, Lim J, Kim Y, Seo H, et al. Introducing EzBioCloud: a taxonomically united database of 16S rRNA gene sequences and whole-genome assemblies. Int J Syst Evol Microbiol. (2017) 67:1613–7. doi: 10.1099/ijsem.0.001755
12. Schloss PD, Westcott SL, Ryabin T, Hall JR, Hartmann M, Hollister EB, et al. Introducing mothur: open-source, platform-independent, community-supported software for describing and comparing microbial communities. Appl Environ Microbiol. (2009) 75:7537–41. doi: 10.1128/AEM.01541-09
13. Edgar RC. Search and clustering orders of magnitude faster than BLAST. Bioinformatics. (2010) 26:2460–1. doi: 10.1093/bioinformatics/btq461
14. Revest M, Egmann G, Cattoir V, Tattevin P. HACEK endocarditis: state-of-the-art. Expert Rev Anti Infective Therapy. (2016) 14:523–30. doi: 10.1586/14787210.2016.1164032
15. Malani AN, Aronoff DM, Bradley SF, Kauffman CA. Cardiobacterium hominis endocarditis: two cases and a review of the literature. Eur J Clin Microbiol Infect Dis. (2006) 25:587–95. doi: 10.1007/s10096-006-0189-9
16. Breitkopf C, Hammel D, Scheld HH, Peters G, Becker K. Impact of a molecular approach to improve the microbiological diagnosis of infective heart valve endocarditis. Circulation. (2005) 111:1415–21. doi: 10.1161/01.CIR.0000158481.07569.8D
17. Gauduchon V, Chalabreysse L, Etienne J, Celard M, Benito Y, Lepidi H, et al. Molecular diagnosis of infective endocarditis by PCR amplification and direct sequencing of DNA from valve tissue. J Clin Microbiol. (2003) 41:763–6. doi: 10.1128/JCM.41.2.763-766.2003
18. Gomes A, van Oosten M, Bijker KLB, Boiten KE, Salomon EN, Rosema S, et al. Sonication of heart valves detects more bacteria in infective endocarditis. Sci Rep. (2018) 8:12967. doi: 10.1038/s41598-018-31029-w
19. Imai A, Gotoh K, Asano Y, Yamada N, Motooka D, Fukushima M, et al. Comprehensive metagenomic approach for detecting causative microorganisms in culture-negative infective endocarditis. Int J Cardiol. (2014) 172:e288–9. doi: 10.1016/j.ijcard.2013.12.197
20. Fukui Y, Aoki K, Okuma S, Sato T, Ishii Y, Tateda K. Metagenomic analysis for detecting pathogens in culture-negative infective endocarditis. J Infect Chemother. (2015) 21:882–4. doi: 10.1016/j.jiac.2015.08.007
21. Greub G, Lepidi H, Rovery C, Casalta JP, Habib G, Collard F, et al. Diagnosis of infectious endocarditis in patients undergoing valve surgery. Am J Med. (2005) 118:230–8. doi: 10.1016/j.amjmed.2004.12.014
22. Ruppe E, Cherkaoui A, Lazarevic V, Emonet S, Schrenzel J. Establishing genotype-to-phenotype relationships in bacteria causing hospital-acquired pneumonia: a prelude to the application of clinical metagenomics. Antibiotics. (2017) 6:E30. doi: 10.3390/antibiotics6040030
Keywords: next-generation sequencing, culture-negative endocarditis, clinical metagenomics, Cardiobacterium hominis, diagnosis
Citation: Kolb M, Lazarevic V, Emonet S, Calmy A, Girard M, Gaïa N, Charretier Y, Cherkaoui A, Keller P, Huber C and Schrenzel J (2019) Next-Generation Sequencing for the Diagnosis of Challenging Culture-Negative Endocarditis. Front. Med. 6:203. doi: 10.3389/fmed.2019.00203
Received: 13 June 2019; Accepted: 29 August 2019;
Published: 20 September 2019.
Edited by:
Vitali Sintchenko, University of Sydney, AustraliaReviewed by:
Matthew Watts, New South Wales Health Pathology, AustraliaJianfeng Xie, Southeast University, China
Copyright © 2019 Kolb, Lazarevic, Emonet, Calmy, Girard, Gaïa, Charretier, Cherkaoui, Keller, Huber and Schrenzel. This is an open-access article distributed under the terms of the Creative Commons Attribution License (CC BY). The use, distribution or reproduction in other forums is permitted, provided the original author(s) and the copyright owner(s) are credited and that the original publication in this journal is cited, in accordance with accepted academic practice. No use, distribution or reproduction is permitted which does not comply with these terms.
*Correspondence: Jacques Schrenzel, amFjcXVlcy5zY2hyZW56ZWxAaGN1Z2UuY2g=
†These authors have contributed equally to this work