- 1Department of Nephrology and Rheumatology, University Medical Center Göttingen, Göttingen, Germany
- 2Institute of Pathology, University Medical Center Göttingen, Göttingen, Germany
- 3Institute of Neuropathology, University Medical Center Göttingen, Göttingen, Germany
- 4Max Planck Institute for Experimental Medicine, Göttingen, Germany
- 5Campus Institute for Dynamics of Biological Networks, University of Göttingen, Göttingen, Germany
- 6Infection Biology Unit, German Primate Center, Leibniz Institute for Primate Research Göttingen, Göttingen, Germany
- 7Faculty of Biology and Psychology, University Göttingen, Göttingen, Germany
- 8Department of Intensive Care Medicine, University Medical Center Hamburg-Eppendorf, Hamburg, Germany
- 9Department of Anesthesiology, Emergency and Intensive Care Medicine, University Medical Center Göttingen, Göttingen, Germany
Background: Acute kidney injury (AKI) is very common in severe acute respiratory syndrome coronavirus-2 (SARS-CoV-2) disease 2019 (COVID-19) and considered as a risk factor for COVID-19 severity. SARS-CoV-2 renal tropism has been observed in COVID-19 patients, suggesting that direct viral injury of the kidneys may contribute to AKI. We examined 20 adult cases with confirmed SARS-CoV-2 infection requiring ICU supportive care in a single-center prospective observational study and investigated whether urinary markers for viral infection (SARS-CoV-2 N) and shedded cellular membrane proteins (ACE2, TMPRSS2) allow identification of patients at risk for AKI and outcome of COVID-19.
Objectives: The objective of the study was to evaluate whether urinary markers for viral infection (SARS-CoV-2 N) and shedded cellular membrane proteins (ACE2, TMPRSS2) allow identification of patients at risk for AKI and outcome of COVID-19.
Results: Urinary SARS-CoV-2 N measured at ICU admission identified patients at risk for AKI in COVID-19 (HR 5.9, 95% CI 1.4–26, p = 0.0095). In addition, the combination of urinary SARS-CoV-2 N and plasma albumin measurements further improved the association with AKI (HR 11.4, 95% CI 2.7–48, p = 0.0016). Finally, combining urinary SARS-CoV-2 N and plasma albumin measurements associated with the length of ICU supportive care (HR 3.3, 95% CI 1.1–9.9, p = 0.0273) and premature death (HR 7.6, 95% CI 1.3–44, p = 0.0240). In contrast, urinary ACE2 and TMPRSS2 did not correlate with AKI in COVID-19.
Conclusions: In conclusion, urinary SARS-CoV-2 N levels associate with risk for AKI and correlate with COVID-19 severity.
Introduction
Acute kidney injury (AKI) is very common in severe acute respiratory syndrome coronavirus-2 (SARS-CoV-2) disease 2019 (COVID-19), particularly among patients requiring intensive care unit (ICU) supportive care and is considered as an independent risk factor for premature death (1–5). Rates of reported AKI vary considerably with higher rates reported in countries outside of China (6). Therefore, there is an urgent need for early identification of patients at risk for AKI in COVID-19. SARS-CoV-2 renal tropism and detection of SARS nucleocapsid protein (SARS-CoV-2 N) in renal tubules has been described in COVID-19 patients, suggesting that direct viral injury of the kidneys may contribute to AKI (7–9). This is in line with two autopsy studies suggesting renal SARS-CoV-2 infection as a possible cause of AKI in COVID-19 (7, 8). SARS-CoV-2 renal tropism has been in part attributed to the intrarenal presence of cellular membrane proteins essential for viral entry including angiotensin converting enzyme 2 (ACE2) and transmembrane protease serine subtype 2 (TMPRSS2) (9–11). Based on the assumption that renal injury is caused by direct viral infection of the kidneys, it remains unclear if markers for viral infection (SARS-CoV-2 N) and shedded cellular membrane proteins (ACE2, TMPRSS2) in urinary samples are useful for early identification of COVID-19 patients at risk for AKI. Therefore, we investigated whether risk for AKI in COVID-19 is associated with urinary SARS-CoV-2 N, ACE2, and TMPRSS2 levels, thus allowing for simple and fast identification of patients at risk. We report that urinary SARS-CoV-2 N levels are linked to AKI and COVID-19 severity.
Materials and Methods
Study Populations
All patients with PCR-based SARS-CoV-2 infection confirmed by nasopharyngeal swabs were admitted to the Department of Anesthesiology, Emergency and Intensive Care Medicine, University Medical Center Göttingen, Germany and those requiring ICU supportive care were included. The institutional ethical board of the University Medical Center Göttingen, Germany approved the study (reference number 25/4/19Ü), and informed written consent was obtained. All urinary samples were collected at the University Medical Center Göttingen, Germany between March and June 2020. Urinary samples from severe septic shock patients collected in 2015 served as control and have been previously described and recruited at the Department of Intensive Care Medicine, University Medical Center Hamburg-Eppendorf, Germany (12). The study was approved by the ethical committee Hamburg (Aerztekammer Hamburg, reference number PV4550). A detailed Strengthening the Reporting of Observational Studies in Epidemiology (STROBE) flow chart of patient disposition is shown in Supplementary Figure 1.
Definitions
Comorbidities were assessed using reported information by hospitals or private practices. Arterial hypertension, diabetes, hyperlipoproteinemia, or obesity was considered as metabolic diseases. Liver diseases, malignancies, and autoimmune diseases were considered as other diseases. Group allocation into AKI was defined according to KDIGO by laboratory findings, oliguria, or the need for dialysis. The simplified acute physiology score (SAPS) II and sequential organ failure assessment (SOFA) score were calculated according to published guidelines (13, 14). Requirement of intensive care treatment was defined at admission and calculated by the time between admission to the intensive care unit (ICU) or intermediate care unit (IMC) and relocation to the non-ICU/non-IMC medical ward; all patients required critical care treatment >24 h.
Urinary ELISA Measurements
Investigators were blinded to clinical data collection and urinary enzyme-linked immunosorbent assay (ELISA) measurements. According to the manufacturer's protocols, the urinary levels of SARS-CoV-2 N (KIT40588, Sino Biological), human ACE2 (NBP2-78734, Novus Biologicals), and human TMPRSS2 (NBP2-89170, Novus Biologicals) were analyzed. In brief, 100 μL of native patient urine was analyzed by sandwich ELISA using precoated plates with antibodies specific to SARS-CoV-2 N, human ACE2, and human TMPRSS2. After adding a biotinylated detection antibody, avidin-horseradish peroxidase conjugate was added successively to each microplate well and incubated. After washing, the substrate solution was added to each well. The enzyme–substrate reaction was terminated by addition of a stop solution, and the optical density was measured spectrophotometrically at a wavelength of 450 nm. Measurements were done in triplicates for each urinary sample and compared to the standard curve. Negative test results were declared as not detectable. Photomicrographs of urinary SARS-CoV-2 N ELISA measurements in 20 cases with confirmed SARS-CoV-2 infection requiring ICU supportive care are shown in Supplementary Figure 2.
SARS-CoV-2 RNA Detection
Tissues were dissolved in TRIzol (Zymo) and shredded using QiaShredder (Qiagen). RNA was extracted according to the manufacturer's protocol. RNA (0.5–1 μg) was added to the final PCR reaction. SARS-CoV-2 genome equivalents were detected by quantitative RT–PCR targeting the SARS-CoV-2 E gene as previously reported using the following primers: E_Sarbeco_F, ACAGGTACGTTAATAGTTAATAGCGT; E_Sarbeco_P1, FAM-ACACTAGCCATCCTTACTGCGCTTCG-BBQ; E_Sarbeco_R, ATATTGCAGCAGTACGCACACA (15, 16). The quantitative RT-PCR experiment and data processing were carried out using the LightCycler 480 Real-Time PCR System (Roche) and LightCycler 480 Software (version 1.5, Roche Molecular Systems). Absolute quantification was performed using SARS-CoV-2-specific in vitro–transcribed RNA standards, as previously described (15, 16).
Sample Size and Statistical Analysis
At the time of the study design, data on markers for viral infection (SARS-CoV-2 N) and shedded cellular membrane proteins (ACE2, TMPRSS2) in urinary samples of AKI in COVID-19 patients were lacking. Hence, a convenience sample of 20 consecutive patients was chosen to provide a timely report. Variables were tested for normal distribution using the Shapiro–Wilk test. Non-normally distributed continuous variables are expressed as median and interquartile range (IQR); categorical variables are presented as frequency and percentage. Statistical comparisons were not formally powered or prespecified. For group comparisons, the Mann–Whitney U-test was used to determine differences in medians. Non-parametric between-group comparisons were performed with Pearson's chi-square test. Correlation between parameters was calculated by Spearman's rank correlation and is shown by heatmap reflecting the mean values of Spearman's ρ; asterisks indicate p < 0.05. We retained those covariates found to be significantly associated with AKI in a multivariable regression model (limiting the model to four covariates to avoid model overfit). To establish a cutoff for each parameter, the ability of prognostic factors to discriminate groups was evaluated by receiver operator curves (ROC) and the area under the curve (AUC), as well as sensitivity and specificity. Sensitivity and specificity were based on selection of the cutoff point on the ROC that maximized Youden's index (sensitivity+specificity-1); comparison of survival curves was performed with log rank (Mantel–Cox) testing. For multiple logistic regression, comparison of models was performed with the likelihood ratio test. We reported the hazard ratio (HR) with 95% confidence interval (CI) for each covariate of interest to assess the association between AKI and subsequent mortality. Data analyses were performed with GraphPad Prism (version 8.4.0 for MacOS, GraphPad Software, San Diego, California, USA).
Results
Urinary SARS-CoV-2 N Levels at ICU Admission Associate With AKI in COVID-19
Among the 20 cases with confirmed SARS-CoV-2 infection requiring ICU supportive care, 10/20 (50%) had AKI of whom 2 presented already at ICU admission and 8 developed AKI within 8 days after admission (Table 1). Interestingly, risk of AKI in severe COVID-19 was not associated with disease severity including respiratory failure at ICU admission (Table 1), implicating specific pathomechanisms that contribute to AKI in COVID-19. The urinary levels of SARS-CoV-2 N, ACE2, and TMPRSS2 were measured at ICU admission (day 1), at day 3, and at day 8 during further clinical course. The AKI stage in COVID-19 was associated with elevated urinary levels of SARS-CoV-2 N measured at ICU admission (Figure 1A, Table 2). A similar association was observed for day 3 and 8 measurements, but the effect was less pronounced (Table 2). The specificity of urinary SARS-CoV-2 N measurements was validated by analyzing an organ failure matched historical ICU cohort with no SARS-CoV-2 infection and AKI in 12/22 (54.5%) of the cases, in which urinary SARS-CoV-2 N was not detectable (Supplementary Table 1) (12). ROC analysis revealed that a cutoff urinary SARS-CoV-2 N level higher than 512.2 pg/mL at ICU admission identified patients with AKI (AUC 0.81, p = 0.0211, Figure 1B). Survival analysis for cumulative incidence of AKI confirmed that urinary SARS-CoV-2 N levels at ICU admission identified patients at risk for AKI (HR 5.9, 95% CI 1.4–26, p = 0.0095, Figure 1C). In contrast, urinary ACE2 or TMPRSS2 levels did not correlate with urinary SARS-CoV-2 N measurements or AKI at any time point (Table 2), implicating that urinary clearance of SARS-CoV-2 N more reflects systemic inflammation due to viral spread rather than renal cell death by direct viral infection of the kidneys. This is further supported by post-mortem analysis from deceased patients with AKI and high urinary SARS-CoV-2 N, where SARS-CoV-2 RNA was not detectable in corresponding kidneys (Supplementary Table 2). These findings suggested that urinary SARS-CoV-2 N occurred independently of shedded cellular membrane proteins essential for viral entry, associated with risk of AKI in severe COVID-19.
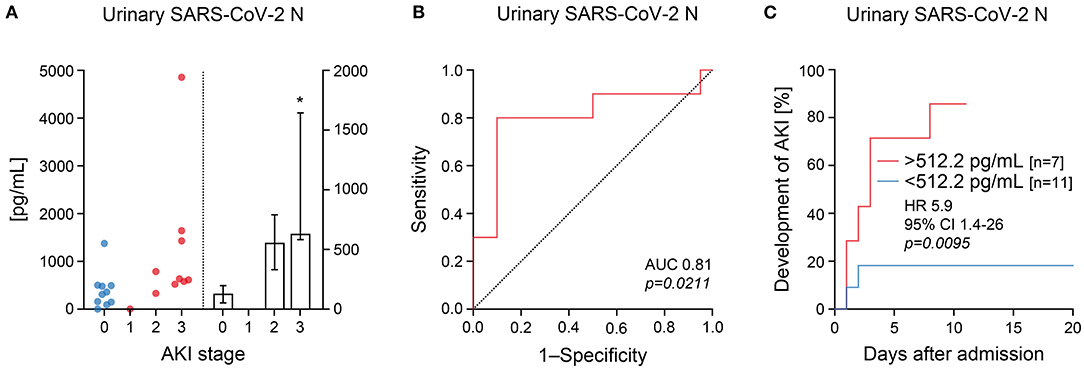
Figure 1. Urinary SARS-CoV-2 N levels at ICU admission associate with AKI in COVID-19. (A) Relation between the AKI stage (according to KDIGO) and urinary levels of SARS-CoV-2 N is shown by scatter dot plots (plotted on the left Y-axis) and bar graphs reflecting median with IQR (plotted on the right Y-axis). (B) ROC analysis for urinary SARS-CoV-2 N assessed at ICU admission for association with AKI in COVID-19. (C) Frequency and survival analysis for cumulative incidence of AKI after group separation for urinary SARS-CoV-2 N at 512.2 pg/mL. AKI, acute kidney injury; AUC, area under the curve; CI, confidence interval; HR, hazard ratio; ICU, intensive care unit; ROC, receiver operator curve; SARS-CoV-2 N, severe acute respiratory syndrome coronavirus 2 nucleocapsid protein.
Combining Urinary SARS-CoV-2 N and Plasma Albumin Measurements Identify Patients at Risk for AKI
Plasma albumin levels at the time of ICU admission have previously been established as a risk marker for AKI and COVID-19 severity (17–19). Therefore, we next compared clinical and routine laboratory parameters assessed at ICU admission for association with AKI. In line with previous findings, hypoalbuminemia at the time of ICU admission also identified patients at risk for AKI in our cohort (Figure 2A, Table 3). Interestingly, the levels of urinary SARS-CoV-2 N and plasma albumin did not correlate directly (Figure 2A), suggesting that combining urinary SARS-CoV-2 N and plasma albumin could further improve risk stratification for AKI in COVID-19. Furthermore, hypoalbuminemia did not correlate with proteinuria including albuminuria (Table 3), indicating that observed hypoalbuminemia reflects systemic inflammation rather than urinary loss. Confirmed by ROC analysis, combining urinary SARS-CoV-2 N higher than 512.2 pg/mL and hypoalbuminemia below 2.05 g/dL (two-variable model, AUC 0.94, p = 0.0009, Figure 3A) outperformed urinary SARS-CoV-2 N alone (AUC 0.81, p = 0.0211, comparison of models: p = 0.0016) or plasma albumin alone (AUC 0.78, p = 0.03, comparison of models: p = 0.0061). Thus, combined urinary SARS-CoV-2 N and plasma albumin levels assessed at ICU admission identified patients at risk for AKI (two-variable model, HR 11.4, 95% CI 2.7-48, p = 0.0016, Figure 3B). In summary, combining urinary SARS-CoV-2 N and plasma albumin levels at ICU admission allowed for a more robust identification of patients at risk for AKI in COVID-19 as compared to analysis of the single markers alone.
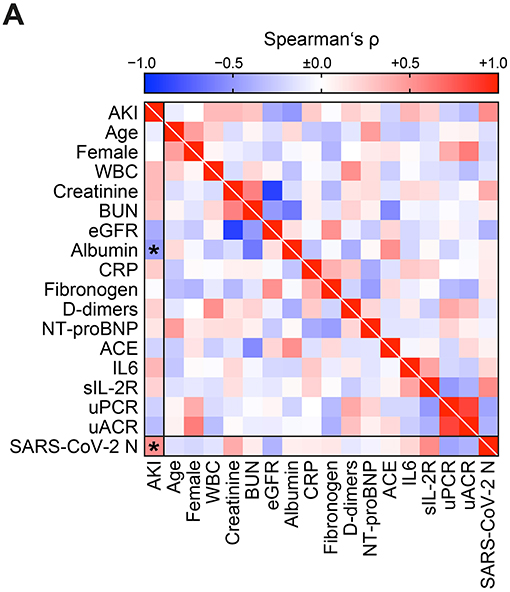
Figure 2. Hypoalbuminemia at time of ICU admission identifies patients at risk for AKI. (A) Correlation between AKI, clinical, and routine laboratory parameters assessed at ICU admission is shown by heatmap reflecting the mean values of Spearman's ρ; asterisks indicate p < 0.05. ACE, angiotensin converting enzyme; AKI, acute kidney injury; BUN, blood urea nitrogen; CRP, C-reactive protein; eGFR, estimated glomerular filtration rate (CKD-EPI); IL-6, interleukin-6; NT-proBNP, N-terminal pro-B-type natriuretic peptide; SARS-CoV-2 N, severe acute respiratory syndrome coronavirus 2 nucleocapsid protein; sIL-2R, soluble interleukin-2 receptor; uACR, urinary albumin-to-creatinine ratio; uPCR, urinary protein-to-creatinine ratio; WBC, white blood cells.
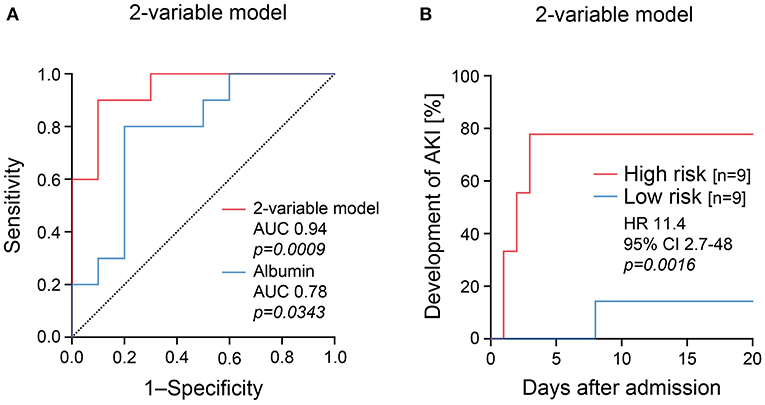
Figure 3. Combining urinary SARS-CoV-2 N and plasma albumin measurements identifies patients at risk for AKI. (A) ROC analysis for combining urinary SARS-CoV-2 N and plasma albumin measurements at ICU admission (two-variable model) for association with AKI in COVID-19. (B) Frequency and survival analysis for cumulative incidence of AKI after group separation for established two-variable model. AKI, acute kidney injury; AUC, area under the curve, CI: confidence interval; HR, hazard ratio; ROC: receiver operator curve; SARS-CoV-2 N, severe acute respiratory syndrome coronavirus 2 nucleocapsid protein.
Combining Urinary SARS-CoV-2 N and Plasma Albumin Measurements Associate With Length of ICU Supportive Care and Premature Death in COVID-19
AKI has previously been considered as an independent risk factor for premature death in patients with COVID-19 (1–5). Therefore, we next compared the association between AKI, disease course, outcome, and urinary SARS-CoV-2 N and plasma albumin levels assessed at ICU admission. AKI was associated with a prolonged ICU supportive care before patients could be relocated to a non-ICU medical ward (HR 2.8, 95% CI 0.97–8.1, p = 0.0269, Figure 4A). Urinary SARS-CoV-2 N and plasma albumin measured at ICU admission were equally associated with the length of ICU supportive care (two-variable model, HR 3.3, 95% CI 1.1–9.9, p = 0.0273, Figure 4B). In addition, combining urinary SARS-CoV-2 N and plasma albumin levels at ICU admission identified patients at risk for premature death in COVID-19 (two-variable model, HR 7.6, 95% CI 1.3–44, p = 0.0240, Figure 4C). In summary, AKI was associated with disease severity reflected by prolonged ICU length of stay. Furthermore, urinary SARS-CoV-2 N and plasma albumin levels at ICU admission equally allowed for a robust identification of patients at risk for prolonged ICU length of stay and premature death in COVID-19.
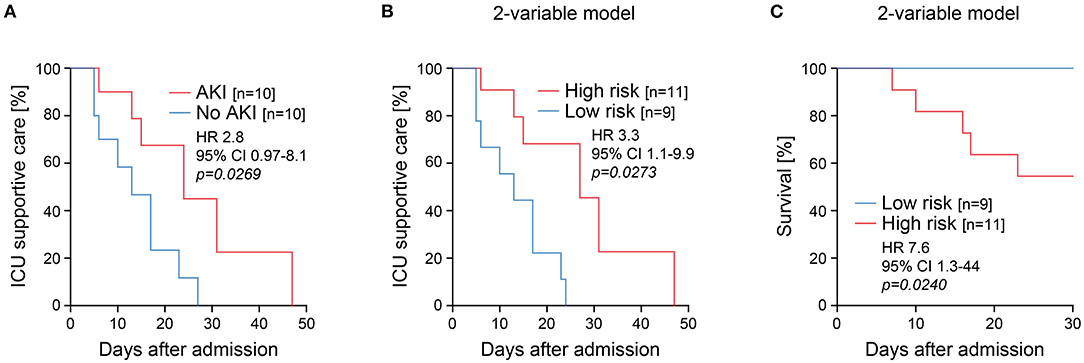
Figure 4. Combining urinary SARS-CoV-2 N and plasma albumin measurements associates with length of ICU supportive care and premature death in COVID-19. (A) Duration of ICU supportive care grouped for AKI. (B) Duration of ICU supportive care grouped for established two-variable model. (C) Premature death in COVID-19 grouped for established two-variable model. AKI, acute kidney injury; CI, confidence interval; HR, hazard ratio; ICU, intensive care unit.
Discussion
AKI is common in critically ill patients with severe infection and sepsis, associated with mortality rates between 15 and 60% and up to 10 times higher as compared to general ICU cohorts (20–24). Hemodynamic support and guided volume therapy to optimize renal perfusion and to limit intrarenal injury are fundamental preventive strategies. Established laboratory markers to identify AKI do not provide insights into the underlying pathological mechanisms. Based on the assumption that AKI in COVID-19 is caused by direct viral infection of the kidneys, it was unclear whether markers for viral infection (SARS-CoV-2 N) and shedded cellular membrane proteins essential for viral entry (ACE2, TMPRSS2) are detectable in urinary samples of patients with AKI in COVID-19.
Here, we demonstrate for the first time that urinary SARS-CoV-2 N is an early and easily assessable marker to identify patients at risk for AKI and premature death in COVID-19. SARS N has been detected in renal tubules, and SARS-CoV-2 renal tropism has been demonstrated in COVID-19 autopsy studies, suggesting that renal SARS-CoV-2 infection may cause AKI in COVID-19 (7–9). Whether SARS-CoV-2 directly infects the kidneys and related AKI in COVID-19 is induced by direct viral infection of the kidney and responsible for poor outcome or a consequence of systemic viral spread is still controversial (25–28). Our observations that urinary ACE2 and TMPRSS2 do not associate with AKI or disease severity suggest that urinary clearance of SARS-CoV-2 N more reflects systemic inflammation due to viral spread rather than renal cell death by direct viral infection of the kidneys. This is supported by failure to detect SARS-CoV-2 RNA in corresponding kidneys of deceased patients with AKI and high urinary SARS-CoV-2 N and in line with previous studies reporting that urinary SARS-CoV-2 viral load was not more frequently detected in patients who died or developed AKI, suggesting that direct viral infection is unlikely an important mechanism of AKI in COVID-19 (29–31). This is further supported by unspecific post-mortem findings despite multiorgan viral spread in COVID-19 (32).
A variety of potential mechanisms contributing to AKI in COVID-19 have been suggested. The receptor-binding domain of the SARS-CoV-2 spike protein gains entry to host cells by binding to membrane-bound ACE2 that is also present on kidney tubular epithelial cells and podocytes (33, 34). In this context, recent reports suggest that polymorphisms in ACE2 might alter the ability of SARS-CoV-2 to enter cells in the kidney (33, 34). Furthermore, endothelial dysfunction and microvascular damage indicated by higher levels of D-dimers indicate an important risk factor for coagulopathy associated with COVID-19. Other pro-thrombotic conditions such as thrombotic microangiopathy (TMA) by direct viral activation of the complement system might also contribute to endothelial dysfunction and risk of AKI in patients with COVID-19 (35, 36). In addition, infection with SARS-CoV-2 is associated with induction of an inflammatory response resulting in hyperinflammation resembling a cytokine release syndrome (CRS) that might also contribute to the pathogenesis of multiorgan dysfunction associated with COVID-19, including AKI (37–44). While identification of mechanisms contributing to AKI related to COVID-19 is of relevance and requires further investigation, our findings that urinary SARS-CoV-2 N associates with AKI and COVID-19 severity are also of importance. The accuracy of urinary SARS-CoV-2 N levels to identify patients at risk for AKI in COVID-19 was further improved by plasma albumin measurements, also previously reported as a risk marker for AKI and COVID-19 severity (17–19). Finally, urinary SARS-CoV-2 N and plasma albumin levels identified patients at risk for premature death in COVID-19. Hypoalbuminemia has previously been associated with disease severity and mortality across numerous clinical settings (45). The pathophysiology behind hypoalbuminemia is thought to be secondary to increased capillary permeability, decreased protein synthesis, and decreased half-life of serum albumin, also described in severe COVID-19 (17–19). Furthermore, inflammation may be responsible for the extravasation of serum albumin into the interstitial space due to capillary permeability, with an increased volume distribution of albumin (46). Our observation that hypoalbuminemia did not correlate with proteinuria including albuminuria further supports that hypoalbuminemia in severe COVID-19 is attributed to systemic inflammation and extravasation rather than urinary loss. In summary, our findings that urinary levels of SARS-CoV-2 N associate with AKI and COVID-19 severity might be of great relevance for risk stratification and can potentially lead to early recognition of severe disease to assist clinicians in making informed decision for their patients.
The primary limitation of this study is the small sample size limiting the strength of correlations and the modeling or prediction analyses. Multiple logistic regression was performed as exploratory analyses and should be interpreted with caution due to the study being underpowered. This might limit the results of the multivariate analyses and lead to a certain overfitting. In addition, our findings need further validation in independent cohorts and comparison with other experimental markers of AKI. However, the strong association of urinary SARS-CoV-2 N with AKI and COVID-19 severity in this single-center observational study is promising and requires further investigation.
Conclusions
In conclusion, urinary SARS-CoV-2 N levels associate with risk for AKI and COVID-19 severity. Therefore, we propose that urinary SARS-CoV-2 N could be used as an early and easily assessable marker to identify patients at risk for AKI and premature death in COVID-19.
Data Availability Statement
The original contributions presented in the study are included in the article/Supplementary Material, further inquiries can be directed to the corresponding author/s.
Ethics Statement
The studies involving human participants were reviewed and approved by the institutional review board of the University Medical Center Göttingen, Germany (reference number 25/4/19Ü). The patients/participants provided their written informed consent to participate in this study.
Author Contributions
BT conceived the study, collected and analyzed data, and wrote the first draft. DT performed urinary ELISA measurements and co-wrote the first draft. HH-W, SP, SK, OM, and MW collected human specimens. MW and BT contributed equally as senior authors. All authors participated in the construction and editing of the manuscript.
Funding
This study was supported by grants of the Deutsche Forschungsgemeinschaft (DFG) transregional collaborative research center (CRC) 274 Checkpoints of CNS recovery, the DFG under Germany's Excellence Strategy (EXC 2067/1-390729940). JF is partly financed by the clinician scientist module of the CRC274. OM and MW received funding of Sartorius AG, Göttingen, Germany. BT was supported by the Research program, University Medical Center, University of Göttingen (1403720). The funding sources had no involvement in the design, collection, analysis, interpretation, writing, or decision to submit the article.
Conflict of Interest
The authors declare that the research was conducted in the absence of any commercial or financial relationships that could be construed as a potential conflict of interest.
Acknowledgments
The authors thank the patients and their families for informed consent. We thank Ulrike Ehbrecht, René Müller, Caroline Obergfell, and Katja Schulz for expert technical assistance.
Supplementary Material
The Supplementary Material for this article can be found online at: https://www.frontiersin.org/articles/10.3389/fmed.2021.644715/full#supplementary-material
References
1. Gabarre P, Dumas G, Dupont T, Darmon M, Azoulay E, Zafrani L. Acute kidney injury in critically ill patients with COVID-19. Intensive Care Med. (2020) 46:1339–48. doi: 10.1007/s00134-020-06153-9
2. Chan L, Chaudhary K, Saha A, Chauhan K, Vaid A, Zhao S, et al. AKI in hospitalized patients with COVID-19. J Am Soc Nephrol. (2020) 32:151–60. doi: 10.1681/ASN.2020050615
3. Gupta S, Coca SG, Chan L, Melamed ML, Brenner SK, Hayek SS, et al. AKI treated with renal replacement therapy in critically ill patients with COVID-19. J Am Soc Nephrol. (2020) 32:161–76. doi: 10.1681/ASN.2020060897
4. Xu J, Yang X, Yang L, Zou X, Wang Y, Wu Y, et al. Clinical course and predictors of 60-day mortality in 239 critically ill patients with COVID-19: a multicenter retrospective study from Wuhan, China. Crit Care. (2020) 24:394. doi: 10.1186/s13054-020-03098-9
5. Joseph A, Zafrani L, Mabrouki A, Azoulay E, Darmon M. Acute kidney injury in patients with SARS-CoV-2 infection. Ann Intensive Care. (2020) 10:117. doi: 10.1186/s13613-020-00734-z
6. Nadim MK, Forni LG, Mehta RL, Connor MJ Jr, Liu KD, et al. COVID-19-associated acute kidney injury: consensus report of the 25th Acute Disease Quality Initiative (ADQI) Workgroup. Nat Rev Nephrol. (2020) 16:747–64. doi: 10.1038/s41581-020-00356-5
7. Braun F, Lutgehetmann M, Pfefferle S, Wong MN, Carsten A, Lindenmeyer MT, et al. SARS-CoV-2 renal tropism associates with acute kidney injury. Lancet. (2020) 396:597–8. doi: 10.1016/S0140-6736(20)31759-1
8. Puelles VG, Lutgehetmann M, Lindenmeyer MT, Sperhake JP, Wong MN, Allweiss L, et al. Multiorgan and renal tropism of SARS-CoV-2. N Engl J Med. (2020) 383:590–2. doi: 10.1056/NEJMc2011400
9. Su H, Yang M, Wan C, Yi LX, Tang F, Zhu HY, et al. Renal histopathological analysis of 26 postmortem findings of patients with COVID-19 in China. Kidney Int. (2020) 98:219–27. doi: 10.1016/j.kint.2020.04.003
10. Pan XW, Xu D, Zhang H, Zhou W, Wang LH, Cui XG. Identification of a potential mechanism of acute kidney injury during the COVID-19 outbreak: a study based on single-cell transcriptome analysis. Intensive Care Med. (2020) 46:1114–6. doi: 10.1007/s00134-020-06026-1
11. Hoffmann M, Kleine-Weber H, Schroeder S, Kruger N, Herrler T, Erichsen S, et al. SARS-CoV-2 cell entry depends on ACE2 and TMPRSS2 and is blocked by a clinically proven protease inhibitor. Cell. (2020) 181:271–80 e8. doi: 10.1016/j.cell.2020.02.052
12. Winkler MS, Nierhaus A, Holzmann M, Mudersbach E, Bauer A, Robbe L, et al. Decreased serum concentrations of sphingosine-1-phosphate in sepsis. Crit Care. (2015) 19:372. doi: 10.1186/s13054-015-1089-0
13. Le Gall JR, Lemeshow S, Saulnier F. A new simplified acute physiology score (SAPS II) based on a European/North American multicenter study. JAMA. (1993) 270:2957–63. doi: 10.1001/jama.270.24.2957
14. Vincent JL, de Mendonca A, Cantraine F, Moreno R, Takala J, Suter PM, et al. Use of the SOFA score to assess the incidence of organ dysfunction/failure in intensive care units: results of a multicenter, prospective study. Working group on “sepsis-related problems” of the European Society of Intensive Care Medicine. Crit Care Med. (1998) 26:1793–800. doi: 10.1097/00003246-199811000-00016
15. Hoffmann M, Mosbauer K, Hofmann-Winkler H, Kaul A, Kleine-Weber H, Kruger N, et al. Chloroquine does not inhibit infection of human lung cells with SARS-CoV-2. Nature. (2020) 585:588–90. doi: 10.1038/s41586-020-2575-3
16. Corman VM, Landt O, Kaiser M, Molenkamp R, Meijer A, Chu DK, et al. Detection of 2019 novel coronavirus (2019-nCoV) by real-time RT-PCR. Euro Surveill. (2020) 25:23–30. doi: 10.2807/1560-7917.ES.2020.25.3.2000045
17. Huang J, Cheng A, Kumar R, Fang Y, Chen G, Zhu Y, et al. Hypoalbuminemia predicts the outcome of COVID-19 independent of age and co-morbidity. J Med Virol. (2020) 92:2152–8. doi: 10.1002/jmv.26003
18. Aziz M, Fatima R, Lee-Smith W, Assaly R. The association of low serum albumin level with severe COVID-19: a systematic review and meta-analysis. Crit Care. (2020) 24:255. doi: 10.1186/s13054-020-02995-3
19. Paliogiannis P, Mangoni AA, Cangemi M, Fois AG, Carru C, Zinellu A. Serum albumin concentrations are associated with disease severity and outcomes in coronavirus 19 disease (COVID-19): a systematic review and meta-analysis. Clin Exp Med. (2021). doi: 10.1007/s10238-021-00686-z
20. Liano F, Pascual J. Epidemiology of acute renal failure: a prospective, multicenter, community-based study. Madrid Acute Renal Failure Study Group. Kidney Int. (1996) 50:811–8. doi: 10.1038/ki.1996.380
21. Brivet FG, Kleinknecht DJ, Loirat P, Landais PJ. Acute renal failure in intensive care units–causes, outcome, and prognostic factors of hospital mortality; a prospective, multicenter study. French Study Group on acute renal failure. Crit Care Med. (1996) 24:192–8. doi: 10.1097/00003246-199602000-00003
22. Uchino S, Kellum JA, Bellomo R, Doig GS, Morimatsu H, Morgera S, et al. Acute renal failure in critically ill patients: a multinational, multicenter study. JAMA. (2005) 294:813–8. doi: 10.1001/jama.294.7.813
23. Cheng Y, Luo R, Wang K, Zhang M, Wang Z, Dong L, et al. Kidney disease is associated with in-hospital death of patients with COVID-19. Kidney Int. (2020) 97:829–38. doi: 10.1016/j.kint.2020.03.005
24. Pei G, Zhang Z, Peng J, Liu L, Zhang C, Yu C, et al. Renal involvement and early prognosis in patients with COVID-19 pneumonia. J Am Soc Nephrol. (2020) 31:1157–65. doi: 10.1681/ASN.2020030276
25. Calomeni E, Satoskar A, Ayoub I, Brodsky S, Rovin BH, Nadasdy T. Multivesicular bodies mimicking SARS-CoV-2 in patients without COVID-19. Kidney Int. (2020) 98:233–4. doi: 10.1016/j.kint.2020.05.003
26. Khan S, Chen L, Yang CR, Raghuram V, Khundmiri SJ, Knepper MA. Does SARS-CoV-2 infect the kidney? J Am Soc Nephrol. (2020) 31:2746–8. doi: 10.1681/ASN.2020081229
27. Kudose S, Batal I, Santoriello D, Xu K, Barasch J, Peleg Y, et al. Kidney biopsy findings in patients with COVID-19. J Am Soc Nephrol. (2020) 31:1959–68. doi: 10.1681/ASN.2020060802
28. Santoriello D, Khairallah P, Bomback AS, Xu K, Kudose S, Batal I, et al. Postmortem kidney pathology findings in patients with COVID-19. J Am Soc Nephrol. (2020) 31:2158–67. doi: 10.1681/ASN.2020050744
29. Frithiof R, Bergqvist A, Jarhult JD, Lipcsey M, Hultstrom M. Presence of SARS-CoV-2 in urine is rare and not associated with acute kidney injury in critically ill COVID-19 patients. Crit Care. (2020) 24:587. doi: 10.1186/s13054-020-03302-w
30. Akilesh S, Nast CC, Yamashita M, Henriksen K, Charu V, Troxell ML, et al. Multicenter clinicopathologic correlation of kidney biopsies performed in COVID-19 patients presenting with acute kidney injury or proteinuria. Am J Kidney Dis. (2020) 77:82–93.e1. doi: 10.1053/j.ajkd.2020.10.001
31. Nasr SH, Alexander MP, Cornell LD, Herrera LH, Fidler ME, Said SM, et al. Kidney biopsy findings in patients with COVID-19, kidney injury, and proteinuria. Am J Kidney Dis. (2020) 77:465–8. doi: 10.1053/j.ajkd.2020.11.002
32. Remmelink M, De Mendonca R, D'Haene N, De Clercq S, Verocq C, Lebrun L, et al. Unspecific post-mortem findings despite multiorgan viral spread in COVID-19 patients. Crit Care. (2020) 24:495. doi: 10.1186/s13054-020-03218-5
33. Delanghe JR, Speeckaert MM, De Buyzere ML. The host's angiotensin-converting enzyme polymorphism may explain epidemiological findings in COVID-19 infections. Clin Chim Acta. (2020) 505:192–3. doi: 10.1016/j.cca.2020.03.031
34. Othman H, Bouslama Z, Brandenburg JT, da Rocha J, Hamdi Y, Ghedira K, et al. Interaction of the spike protein RBD from SARS-CoV-2 with ACE2: similarity with SARS-CoV, hot-spot analysis and effect of the receptor polymorphism. Biochem Biophys Res Commun. (2020) 527:702–8. doi: 10.1016/j.bbrc.2020.05.028
35. Goldstein SL, Dahale D, Kirkendall ES, Mottes T, Kaplan H, Muething S, et al. A prospective multi-center quality improvement initiative (NINJA) indicates a reduction in nephrotoxic acute kidney injury in hospitalized children. Kidney Int. (2020) 97:580–8. doi: 10.1016/j.kint.2019.10.015
36. Noris M, Benigni A, Remuzzi G. The case of complement activation in COVID-19 multiorgan impact. Kidney Int. (2020) 98:314–22. doi: 10.1016/j.kint.2020.05.013
37. Mehta P, McAuley DF, Brown M, Sanchez E, Tattersall RS, Manson JJ, et al. COVID-19: consider cytokine storm syndromes and immunosuppression. Lancet. (2020) 395:1033–4. doi: 10.1016/S0140-6736(20)30628-0
38. Ritchie AI, Singanayagam A. Immunosuppression for hyperinflammation in COVID-19: a double-edged sword? Lancet. (2020) 395:1111. doi: 10.1016/S0140-6736(20)30691-7
39. Moore JB, June CH. Cytokine release syndrome in severe COVID-19. Science. (2020) 368:473–4. doi: 10.1126/science.abb8925
40. Yang X, Yu Y, Xu J, Shu H, Xia J, Liu H, et al. Clinical course and outcomes of critically ill patients with SARS-CoV-2 pneumonia in Wuhan, China: a single-centered, retrospective, observational study. Lancet Respir Med. (2020) 8:475–81. doi: 10.1016/S2213-2600(20)30079-5
41. Xu Z, Shi L, Wang Y, Zhang J, Huang L, Zhang C, et al. Pathological findings of COVID-19 associated with acute respiratory distress syndrome. Lancet Respir Med. (2020) 8:420–2. doi: 10.1016/S2213-2600(20)30076-X
42. Winkler MS, Korsten P, Binder C, Tampe B. Correspondence on 'Interleukin-6 receptor blockade with subcutaneous tocilizumab in severe COVID-19 pneumonia and hyperinflammation: case-control study'. Ann Rheum Dis. (2020). doi: 10.1136/annrheumdis-2020-218836
43. Caricchio R, Gallucci M, Dass C, Zhang X, Gallucci S, Fleece D, et al. Preliminary predictive criteria for COVID-19 cytokine storm. Ann Rheum Dis. (2021) 80:88–95. doi: 10.1136/annrheumdis-2020-218323
44. Tampe D, Winkler MS, Korsten P, Hakroush S, Moerer O, Tampe B. Correspondence on 'Preliminary predictive criteria for COVID-19 cytokine storm'. Ann Rheum Dis. (2021). doi: 10.1136/annrheumdis-2020-219709
45. Akirov A, Masri-Iraqi H, Atamna A, Shimon I. Low albumin levels are associated with mortality risk in hospitalized patients. Am J Med. (2017) 130:1465 e11– e19. doi: 10.1016/j.amjmed.2017.07.020
Keywords: severe acute respiratory syndrome coronavirus-2, SARS-CoV-2 disease 2019, acute kidney injury, intensive care, risk prediction
Citation: Tampe D, Hakroush S, Bösherz M-S, Franz J, Hofmann-Winkler H, Pöhlmann S, Kluge S, Moerer O, Stadelmann C, Ströbel P, Winkler MS and Tampe B (2021) Urinary Levels of SARS-CoV-2 Nucleocapsid Protein Associate With Risk of AKI and COVID-19 Severity: A Single-Center Observational Study. Front. Med. 8:644715. doi: 10.3389/fmed.2021.644715
Received: 22 December 2020; Accepted: 19 April 2021;
Published: 25 May 2021.
Edited by:
Wei-Jun Qian, Pacific Northwest National Laboratory (DOE), United StatesReviewed by:
Adam C. Swensen, Pacific Northwest National Laboratory (DOE), United StatesShoji Saito, Nagoya University, Japan
Copyright © 2021 Tampe, Hakroush, Bösherz, Franz, Hofmann-Winkler, Pöhlmann, Kluge, Moerer, Stadelmann, Ströbel, Winkler and Tampe. This is an open-access article distributed under the terms of the Creative Commons Attribution License (CC BY). The use, distribution or reproduction in other forums is permitted, provided the original author(s) and the copyright owner(s) are credited and that the original publication in this journal is cited, in accordance with accepted academic practice. No use, distribution or reproduction is permitted which does not comply with these terms.
*Correspondence: Björn Tampe, YmpvZXJuLnRhbXBlQG1lZC51bmktZ29ldHRpbmdlbi5kZQ==
†These authors share senior authorship