- 1Second Affiliated Hospital of Dalian Medical University, Dalian, China
- 2Department of General Practice, Xi'an People's Hospital (Xi'an Fourth Hospital), Xi'an, China
- 3Department of Health Examination Center, The Second Affiliated Hospital of Dalian Medical University, Dalian, China
Carnitine is an amino acid-derived substance that coordinates a wide range of biological processes. Such functions include transport of long-chain fatty acids from the cytoplasm to the mitochondrial matrix, regulation of acetyl-CoA/CoA, control of inter-organellar acyl traffic, and protection against oxidative stress. Recent studies have found that carnitine plays an important role in several diseases, including non-alcoholic fatty liver disease (NAFLD). However, its effect is still controversial, and its mechanism is not clear. Herein, this review provides current knowledge on the biological functions of carnitine, the “multiple hit” impact of carnitine on the NAFLD progression, and the downstream mechanisms. Based on the “multiple hit” hypothesis, carnitine inhibits β-oxidation, improves mitochondrial dysfunction, and reduces insulin resistance to ameliorate NAFLD. L-carnitine may have therapeutic role in liver diseases including non-alcoholic steatohepatitis, cirrhosis, hepatocellular carcinoma, alcoholic fatty liver disease, and viral hepatitis. We also discuss the prospects of L-carnitine supplementation as a therapeutic strategy in NAFLD and related diseases, and the factors limiting its widespread use.
Introduction
Carnitine (3-hydroxy-4-N-trimethylammoniobutanoate) is an essential water-soluble molecule with multiple functions in the human body (1). Examples of such functions include reducing oxidative stress, increasing expression of pro-inflammatory cytokines (2–4), and improving mitochondrial dysfunction (5) and insulin resistance (IR) (6, 7). Moreover, it plays an important role in the development of many metabolic diseases, such as hypertension, diabetes, polycystic ovary syndrome (8), and osteoarthritis (9). Besides, carnitine has been reported to be closely associated with the development of non-alcoholic fatty liver diseases (NAFLD). Numerous studies have shown that NAFLD has become a major healthcare concern and economic burden worldwide (10); therefore, its prevention and treatment have gained increased attention among researchers. In this review, we summarize and discuss the relationship between carnitine and NAFLD, effects of L-carnitine (the biologically active form of carnitine) supplementation in NAFLD, and related diseases, including non-alcoholic steatohepatitis (NASH), cirrhosis, hepatic cellular cancer (HCC), alcoholic fatty liver disease, and viral hepatitis.
Biological Characteristics of Carnitine
Classification and Distribution in Human Body
Carnitine is an amino acid belonging to a quaternary ammonium cationic complex. It has two stereoisomers: bioactive L-carnitine and abiotic enantiomeric isomer D-carnitine. L-carnitine is the predominant carnitine used in biological and medical fields and is commonly referred to as “carnitine.” The human body contains about 300 mg/kg of L-carnitine, 98% of which is intracellular, with 80% present in the muscles, 5–10% in the gastrointestinal tract, and 3% in the liver (11). Although D-carnitine has no biological activity in humans, it adversely impacts biochemical processes by inhibiting the carnitine acetyltransferase (12). D-carnitine supplementation has been found to induce liver inflammation, oxidative stress, and apoptosis in animal studies (13). In addition, D-carnitine can cause secondary carnitine deficiency (SCD), so researchers often use D-carnitine supplements to feed mice to establish carnitine lack animal models (14, 15). For these reasons, D-carnitine has been rarely studied in humans.
Endogenous Synthesis and Exogenous Sources
In the average adult diet, it is estimated that about 75% of the daily carnitine requirement mainly comes from meat, fish, and dairy products (16), while the remaining 25% is derived from endogenous synthesis (1). In strict vegetarians, more than 90% of the daily carnitine requirement is obtained by endogenous synthesis (17–19), whereby humans can synthesize ~1–2 μmol carnitine/kg/day (20). The synthesized carnitine is formed when 6-N-trimethyl-lysine (TML) is released during protein degradation (Figure 1) (21). After release, TML is hydroxylated into 3-hydroxyl-6-N-trimethyl-lysine (HTML) by trimethyl dioxygenase (TMLD), which is then broken down into 4-N-trimethyl-butylaldehyde (TMABA) and glycine by HTML aldolase (HTMLA) (1). TMABA produces 4-N-trimethylaminobutyrate (γ-butyrobetaine) under the action of dehydrogenase (11). Finally, γ-butyrobetaine dioxygenase (BBD) is used to hydroxylate γ-butyrobetaine to produce endogenous carnitine (Figure 1) (22, 23). BBD is confined to the human liver, kidneys, testis and brain, thus the biosynthesis of carnitine occurs only at these locations (24). Other tissues, such as skeletal muscle, obtain carnitine from the blood (25).
The distribution and homeostasis of carnitine within the body is controlled by organic cationic transporters (OCTN) (26). OCTN act on intestinal absorption and renal reabsorption of carnitine, and plays an important role in tissue distribution by catalyzing carnitine to enter cells in vivo (27). Among these, OCTN2 is the most important physiologically transporter of carnitine due to its high affinity and wide expression (27). OCTN2 plays a crucial role in carnitine homeostasis. Notably, BB, a direct precursor of carnitine, is also a good substrate for OCTN2. The liver and kidneys have a strong ability to convert BB to carnitine. Loss or mutation of OCTN2 function results in primary systemic carnitine deficiency (PCD) with severe clinical consequences such as cardiac and skeletal myopathy, cardiac hypertrophy and NAFLD (28).
Biological Functions
Transport of Long-Chain Fatty Acids Into the Mitochondrial Matrix
The essential function of carnitine is to transport LCFAs from the cytoplasm to the mitochondrial matrix for subsequent degradation by β-oxidation, known as “carnitine shuttle” (5). LCFA activation occurs in the cytosol, but the enzymes required to catalyze LCFA oxidation exist in the mitochondrial matrix (29). In this process, LCFA must be first activated into lipoyl-CoA via acyl-CoA synthetase (ACS) (30). Then, lipoyl-CoA is transported into the mitochondria. Since the inner mitochondrial membrane is impermeable to lipoyl-CoA (29), the entry of lipoyl-CoA relies on a shuttle system, which requires carnitine.
The carnitine shuttle has three main steps. First, CoA must be transferred from lipoyl-CoA to the hydroxyl group of carnitine to form lipoyl-carnitine. This transesterification is catalyzed by carnitine palmitoyl transferase I (CPT I) in the outer membrane (1). Second, the lipoyl-carnitine ester enters the matrix by facilitated diffusion through carnitine-acylcarnitine translocase (CACT) located in the inner mitochondrial membrane (31). In the final step, lipoyl-CoA is enzymatically transferred from carnitine to intramitochondrial CoA by carnitine palmitoyl transferase II (CPT II) (32). This isozyme, located on the inner face of the inner mitochondrial membrane, regenerates lipoyl-CoA and releases free carnitine into the matrix. Carnitine enters the intermembrane space again via CACT (Figure 2).
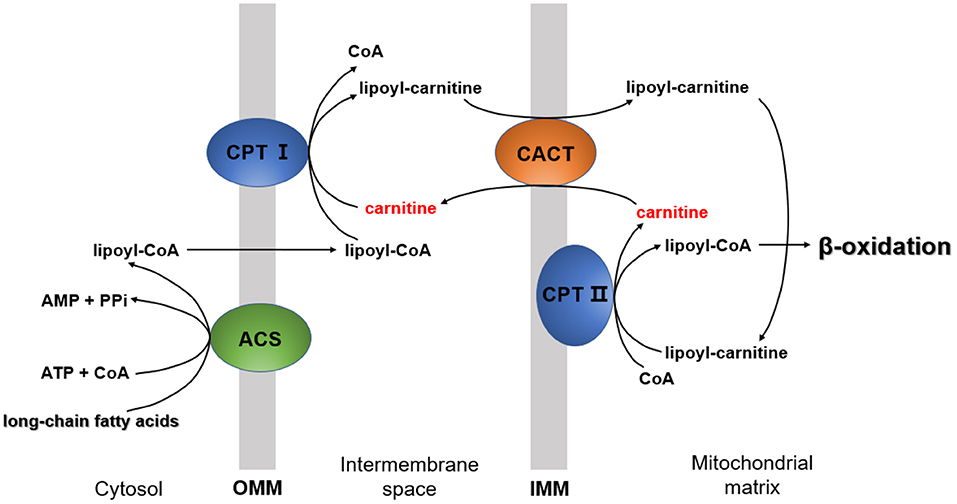
Figure 2. The mechanism by which long-chain fatty acids enter the mitochondria. CPT I, carnitine palmitoyltransferase I; CPT II, carnitine palmitoyltransferase II; ACS, acyl-CoA synthetase; CACT, carnitine-acylcarnitine translocase; OMM, outer mitochondrial membrane; IMM, inner mitochondrial membrane.
Regulation of Acetyl-CoA/CoA Ratio
Under physiological conditions, carnitine can buffer excess acetyl-CoA in the mitochondria via the formation of acetyl-carnitine (33), which requires the presence of carnitine acyltransferase and carnitine acylcarnitine translocase. Acetyl-CoA is either metabolized through the tricarboxylic acid cycle (TCA cycle) or exported as acetyl-carnitine by carnitine. When there is persistent excess or underutilization of certain fatty acids, non-metabolizable acyl-CoAs accumulate. In such situations, carnitine acts as a receiver for these acyl groups by removing them from the tissues and excreting them in the urine (20), or they get separated from carnitine and reused (34). Carnitine regulation of acetyl-CoA/CoA reduces the inhibition of many intramitochondrial enzymes involved in glucose and amino acid catabolism (35).
Inter-organellar Acyl Transfer
Long-chain fatty acids (LCFA) and branched chain fatty acids are oxidized in peroxisomes. In contrast to mitochondrial β-oxidation, incomplete peroxisomal oxidation of fatty acids yields acetyl-CoA and shortened medium-chain acyl-CoAs. In order to completely oxidize these substances into CO2, the products of peroxisome fatty acid oxidation must be transported to the mitochondria (20). Since CoA and CoA esters cannot penetrate the cell membrane, they must be converted into their respective carnitine esters by catalase and carnitine octyltransferase (COT) in peroxisomes. Therefore, the carnitine esters are transported from peroxisomes to mitochondria through peroxisome and mitochondrial carnitine-acylcarnitine translocase (CACT), then reconverted into CoA esters by mitochondrial CPT II in the mitochondrial matrix (20). These are then oxidized into CO2 and H2O through mitochondrial β-oxidation, TCA cycle, and electron transfer.
Reduction of Oxidative Stress
Carnitine has several protective effects on oxidative stress. These include direct scavenging of free radicals, such as 2,2-diphenyl-1-picrylhydrazyl (DPPH), superoxide dismutase and hydrogen peroxide, and metal chelation to catalyze free radical formation, such as Fe2+; inhibition of reactive oxygen species-producing enzymes such as xanthine oxidase (XO) and nicotinamide adenine dinucleotide phosphate oxidase (NOX); upregulation of antioxidant enzymes like catalase (CAT), superoxide dismutase (SOD), glutathione reductase (GR) glutathione peroxidase (GPx), heme oxygenase, endothelial nitric oxide synthase, and other protective proteins (5).
In addition to the above biological functions, carnitine also plays an important role in anti-apoptosis and protection of mitochondrial biogenesis and integrity, which are beyond the scope of this study (5).
Application in Diseases
Carnitine, a natural compound closely related to the above-mentioned functions, has recently been found to alter the underlying disease pathology with fewer side effects (5, 36). It has been reported that L-carnitine as a supplement can be useful in the treatment of hypertension (37), diabetes mellitus (6, 7), NAFLD (34), heart failure (38), coronary artery disease (39), liver cirrhosis (40), muscle injury (41), dyslipidemia (42), migraine (43), Alzheimer's disease (44), and other chronic diseases.
Role of Carnitine in the Pathogenesis of NAFLD and Associated Diseases
Carnitine and “Multiple Hit” Hypothesis in the Pathogenesis of NAFLD
The prevalence of NAFLD is increasing at a tremendous rate, currently affecting about 24% of the world's population. It has been pinpointed as the most common cause of liver disease globally (45). In the last decade, the clinical burden of NAFLD has been associated to liver-related morbidity and mortality and also to the extra-hepatic manifestations involving other organs and regulatory pathways. The pathogenesis of NAFLD is multifactorial and only partially understood (46). At present, the highly recognized pathogenic mechanism is a “multiple hit” hypothesis, which considers the combined effects of multiple insults on genetically susceptible subjects to induce NAFLD and provides a reasonable explanation for the development of NAFLD (47). The first hit is the accumulation of fat in the liver, followed by the development of necrotic inflammation and fibrosis. In addition, nutritional factors, intestinal microflora, and genetic and epigenetic factors exhibit significant influence (48). Inhibition of β-oxidation, mitochondrial dysfunction, and insulin resistance (IR) are the three most important links in these “hits” as well as targets for carnitine to ameliorate NAFLD. Herein, we attempt to explain the role of carnitine in the pathogenesis of NAFLD, as shown in Figure 3.
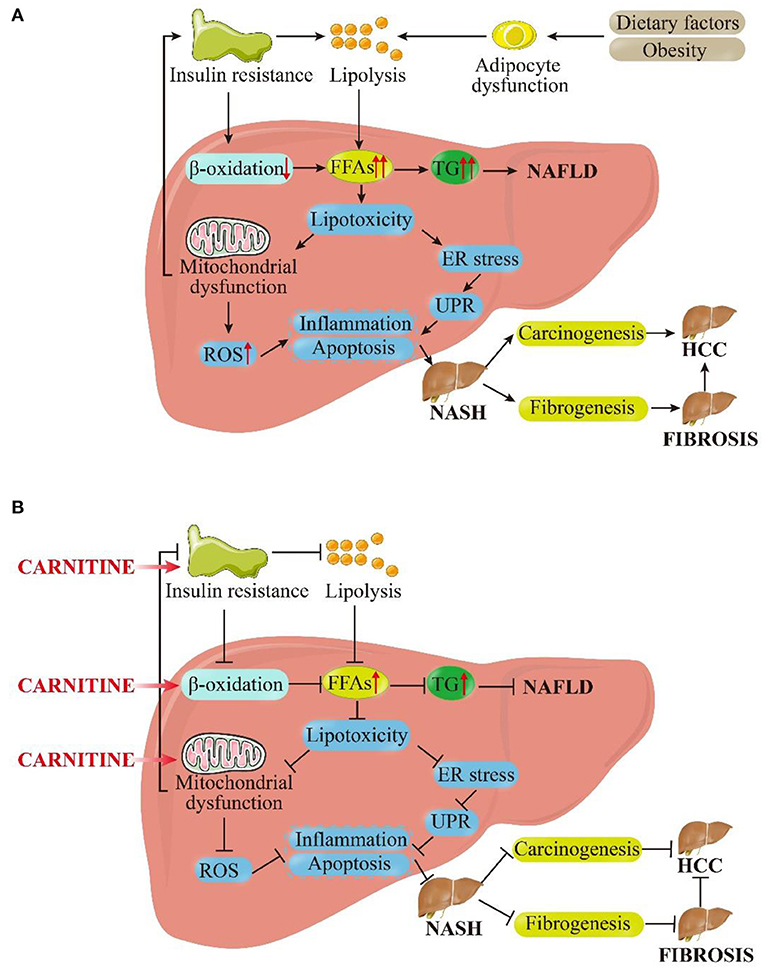
Figure 3. Pictorial representation of the (A) pathophysiological mechanism of NAFLD based on the “multiple hit” hypothesis and (B) inhibitory effects of carnitine on NAFLD and its progression. As shown in (A), dietary and environmental factors, together with obesity, lead to the proliferation and dysfunction of adipocytes. Insulin resistance acts on adipose tissue and worsens adipocyte dysfunction, which in turn worsens lipolysis. In the liver, insulin resistance inhibits β-oxidation. Under the dual attack of increased lipolysis and weakened β-oxidation, the influx FFAs to hepatocytes is increased greatly, leading to the synthesis and accumulation of TG and enhanced liver lipotoxicity. Excessive TG eventually induces NAFLD. Increased lipotoxicity leads to mitochondrial dysfunction and oxidative stress on endoplasmic reticulum (ER) by the activation of ROS, which leads to liver inflammation and fibrosis. At the same time, mitochondrial dysfunction promotes insulin resistance, exacerbating above process in a vicious circle. These pathological processes can cause the liver to persist in the stable stage of disease (NAFLD) or develop in to NASH. In the late stage of disease progression, NASH can progress to fibrosis or even HCC under the stimulation of certain factors. As shown in (B), carnitine supplementation reduces insulin resistance, promotes β-oxidation and improves mitochondrial function. Subsequently, the cellular concentration of FFAs and TG in hepatocytes get reduced and lipotoxicity is alleviated. The level of ROS is restrained to a certain extent, and inflammation and apoptosis were also improved. These above interlocking effects could alleviate NAFLD and NASH, and they may even have positive therapeutic effects on liver fibrosis and HCC. FFAs, free fatty acids; TG, triglycerides; ER, endoplasmic reticulum; UPR, unfolded protein response; ROS, reactive oxygen species; NASH, non-alcoholic steatohepatitis; HCC, hepatocellular carcinoma.
Supplementation with carnitine may have a positive effect on the β-oxidation of fatty acids in mitochondria, thereby promoting lipid metabolism by boosting the uptake of fatty acids and eventually reducing fat accumulation in hepatocytes. It is known that NAFLD is associated with an imbalance in a variety of metabolic networks, among which abnormal lipid metabolism is the core pathological metabolic process (49). The immediate cause of NAFLD is high levels of triglycerides (TG) and serum free fatty acids (FFAs). Excess TG comes from hepatic fat production and dietary fat supply, while FFAs accumulate due to lipolysis of visceral adipose tissues (50). The most important strategy to reduce NAFLD is to decrease dietary fat consumption and promote the catabolism of FFAs. β-oxidation is the core process of FFA decomposition, and carnitine acts as an FFA transporter. Carnitine plays an extremely important role in β-oxidation through the “carnitine shuttle,” as described above (51). The carnitine-mediated entry process is a rate-limiting step for mitochondrial LCFA oxidation and, thus, a major regulating point. Increased levels of carnitine may improve the originally inhibited β-oxidation, which is a great way to reduce fat accumulation in the liver (34). Recent studies have emphasized the key role of mitochondrial dysfunction in the occurrence and development of NAFLD (52). The effect of carnitine on mitochondrial dysfunction has been confirmed by various experiments (5). Increased FFAs can lead to hepatic lipotoxicity, generation of reactive oxygen, and damage to the mitochondrial membrane (52). Carnitine treatment could increase the mRNA expression of carnitine palmitoyltransferase 1A and peroxisome proliferator-activated receptor-γ (PPAR-γ), while preventing lipid membrane peroxidation and ROS. This further leads to the reversal of mitochondrial dysfunction, thereby increasing mitochondrial β-oxidation and reducing intracellular oxidative stress to prevent hepatic lipotoxicity (53, 54).
An important mechanism by which carnitine improves IR is enhancing the oxidation of mitochondrial long-chain acyl-CoAs (Figure 2) (55). Accumulation of long-chain acyl-CoAs and other FA metabolites impairs insulin signaling and leads to the development of IR, a crucial pathophysiological factor for carbohydrate metabolism disorders consistent with the development of NAFLD (49). Since abnormal mitochondrial function is associated with IR (56), carnitine therapy can also reduce IR by improving mitochondrial function (56).
Other possible mechanisms to improve insulin sensitivity in NAFLD reported in the literature include the regulation of the acetyl-CoA/CoA ratio in mitochondria, modulation of the pyruvate dehydrogenase complex (PDHC) activity, altering the expression of glycolytic and gluconeogenic enzymes as well as the expression of genes associated with the insulin signaling cascade, and stimulation of the insulin-like growth factor-1 (IGF-1) axis and IGF-1 signaling cascade (34, 55). As mentioned above, carnitine improves the glucose metabolism by reducing acetyl-CoA/CoA and reduces IR. Both insulin and IGF-1 act through their homologous receptor tyrosine kinases to coordinate metabolism and cellular responses to nutrient supply (57). When IGF-1 axis is activated, multiple signaling pathways corresponding to its kinase domain are also activated, including PI3K/Akt pathway and Raf/MEK/ERK level linkage pathway, which can ultimately reduce IR and prevent cell apoptosis (58, 59), thereby improving NAFLD.
Role of Carnitine in NAFLD Progression and Development of Associated Diseases
The liver is the main organ responsible for detoxification and metabolism of various compounds that produce reactive oxygen species (ROS). As such, liver disease may lead to increased ROS production and, subsequently, increased peroxidation of lipid membranes and inflammatory factors production, leading to hepatocyte injury and cell death (60–63). Injury or death of hepatocytes results in increased release of liver enzymes (64). Patients with chronic liver disease, especially liver cirrhosis, usually suffer from secondary carnitine deficiency (65, 66). Because the liver is one of the main sites for carnitine synthesis, liver disease can impair carnitine synthesis, which further aggravates the above-mentioned pathological processes and promotes disease progression. A recent meta-analysis showed that L-carnitine supplementation improves liver function by reducing histological steatosis and NAS scores in patients with NASH (67). In addition, Okabayashi et al. demonstrated that L-carnitine could improve postoperative liver function in hepatectomized patients (68). L-carnitine reduces inflammatory response via the transfer of β-oxidized long-chain fatty acids in mitochondria and the excretion of toxic substances in fatty acid metabolism (69). It also plays the role of free radical scavenger by reducing the production of ROS (70). Hence, L-carnitine may contribute by reducing oxidative stress, decreasing inflammatory response, and activating enzymes involved in the defense against oxidative damage, thereby reducing elevated serum liver enzymes in patients with liver disease.
Primary carnitine deficiency (PCD) is an autosomal recessive FA oxidation disease (34). Both serum and intracellular carnitine levels are very low in PCD patients. Therefore, FAs cannot be accumulated as an energy source (71), leading to increased ROS production (72). Different from PCD, secondary carnitine deficiency (SCD) is mostly caused by drugs (such as valproic acid, anticancer drugs, omeprazole, amphoteric drugs, etc.) or diseases (such as fatty acid oxidation disorder, organic acidemia, etc.) (1). These drugs or diseases increase the excretion of carnitine in the form of acylcarnitine in urine, reduce renal tubule reabsorption and endogenous biosynthesis, and inhibit carnitine transporters, resulting in increased carnitine consumption (73, 74). Carnitine deficiency impairs the mitochondrial β-oxidation of FAs, leading to acute metabolic decompensation, elevated aminotransferase, and hepatic encephalopathy similar to NAFLD (75). It has been reported that long-term oral carnitine treatment for 6 months in a patient with carnitine deficiency resulted in increased muscle strength, significant reduction in heart size, relief from cardiomyopathy, and partial repletion of carnitine levels in plasma and muscle with complete repletion of liver functions (71). L-carnitine has been approved by the US Food and Drug Administration for the treatment of PCD and SCD (76).
Alcoholic fatty liver disease is another major cause of fatty liver disease, for which carnitine supplementation may also be beneficial for improvement (77). The endogenous biosynthesis of FAs is the main cause of hepatic steatosis in chronic alcoholism (78). Hepatic steatosis is further aggravated by impaired fatty acid oxidation in an ethanol-impaired liver (79). Sachan et al. evaluated the lipid-lowering effects of carnitine and its precursors (lysine and methionine) in rats with chronic alcoholism and concluded that carnitine effectively prevented alcohol-induced hyperlipidemia and liver fat accumulation. Carnitine was also found to be more effective than its precursors (77).
The efficacy of L-carnitine supplements in the treatment of chronic hepatitis B (CHB) remains controversial. A Korean study compared the combination of entecavir and carnitine with entecavir alone to determine their therapeutic effects in CHB. Results indicated that the ALT normalization rate in the combination group was higher than that in the entecavir group, but the HBV-DNA normalization rate and change in serum HBV-DNA levels were similar (80). However, a recent trial showed that besifovir dipivoxil maleate combined with L-carnitine did not lead to any improvement in hepatic steatosis in CHB patients (81). On the other hand, carnitine has a higher recognition for the therapeutic effect in chronic hepatitis C (CHC). A recent meta-analysis showed that long-term use of low-dose (≤2,000 mg/day) L-carnitine could reduce TC and TG in overweight patients with liver disease, especially those with chronic hepatitis C (82). Another study also suggested that L-carnitine may be an effective adjuvant for anti-HCV therapy, because it reduces the oxidative stress induced by JFH-1 infection, inhibits HCV assembly, and exhibits anti-HCV activity through its anti-adipogenic activity in HCV-infected cells (83).
Downstream Mechanism of Carnitine in NAFLD and Related Diseases
The molecular regulation mechanism of the role of carnitine in fatty acid metabolism has been preliminarily clarified. Typical genes, such as OCTN2 (encoded by SLC22A5) and /or BBD (encoded by BBOX1), regulated by PPARα play regulatory roles in the cellular fatty acid uptake, fatty acid activation, intracellular fatty acid transport, and β-oxidation of mitochondrial and peroxisomal fatty acids (84). With the increase of FFA oxidation, PPARα is activated, and the expression of BBD and OCTN2 in oxidative tissue is simulated to facilitate disposal (85). At the same time, increased expression of BBD and OCTN2 also plays a role in regulating carnitine homeostasis by stimulating the intake and biosynthesis of the carnitine, thus increasing the concentration of carnitine (86). The transcriptional regulation of PPARα on carnitine homeostasis related genes is consistent with their basic role in fatty acid catabolism (85).
Many experimental studies have been conducted to understand the impact of carnitine supplementation in NAFLD. For instance, one study employed a mouse model to demonstrate that L-carnitine supplementation could oppose the NAFLD progression. Results revealed a reduction in hepatic lipid accumulation and oxidative stress, hepatic fibrosis via modulation of α-smooth muscle actin (αSMA), peroxisome-activated receptor gamma (PPARγ), and nuclear factor kappa B (Nf-κB) expression (87). Among them, PPARγ may regulate cell apoptosis through the p21, p53 and p27 pathways, and exert an inhibitory effect on the progression of HCC (88). Another research using a mouse model showed that L-carnitine supplementation can inhibit the NAFLD development by decreasing TNF (receptor superfamily member 9 or CD137), CCL23 (C-C motif chemokine 23), MMP1 (matrix metalloproteinase 1), and FGF21 (fibroblast growth factor 21) in the plasma (89). Another study on hepatoma HepG2 cells revealed the distinct benefits of L-carnitine in fructose-mediated lipid accumulation through adenosine monophosphate-activated protein kinase (AMPK) activation (90). L-carnitine was found to increase PGC1α expression and ameliorate mitochondrial damage (90). Numerous works have revealed that the combination of L-carnitine and nicotinamide nucleoside can enhance the transfer of fatty acids across mitochondrial inner membrane and increase the content of nicotinamide adenine nucleotide (NAD +), which is necessary for β-oxidation and the TCA cycle. It can also reverse the harmful effects of a high-fat diet on liver metabolic pathways and related regulators, such as ACOX, SCAP, SREBF, PPARGC1B, and INSR (91). These mechanisms support the administration of L-carnitine as a novel drug to reduce NAFLD.
Treatment of NAFLD With L-Carnitine Supplementation
Since the effects of NAFLD are reversible, it is important to control the disease in its early stages (92). Unfortunately, there are no drugs specifically approved for the treatment of NAFLD at present (93). Available treatments for NAFLD mainly focus on changing lifestyle habits and encouraging weight loss. In addition, several molecules have been studied as an adjuvant therapy, including L-carnitine, CoQ10, vitamin E, vine tea polyphenol, cytoprotective agents (ursodeoxycholic acid), and insulin sensitizers (pioglitazone and metformin) (94).
L-carnitine, the only biologically active form of carnitine, has been one of the most widely studied molecules for the treatment of NAFLD. Several meta-analyses showed that L-carnitine supplementation improved steatosis and NASH (67) and carnitine supplementation in NAFLD patients could reduce AST, ALT, TG, and HOMA-IR (95). In yet another meta-analysis, L-carnitine supplementation was found to significantly improve the circulating levels of ALT, AST, and gamma-glutamyl transpeptidase (γ-GT), which may have a positive impact on liver function (96).
The research trials conducted to study the effects of L-carnitine supplements in NAFLD, its progression, and the associated diseases are summarized in Tables 1, 2. Most of these studies reported that L-carnitine supplementation can normalize or reduce the serum levels of liver enzymes (94, 97, 98, 101–104). Some have confirmed that L-carnitine supplementation could reduce the incidence and severity of NAFLD (103) and, thus, improve liver attenuation index on CT (101), NAFLD sonographic grade (97), and histological scores (104). Other studies propose that L-carnitine supplementation could improve blood glucose (including FBG and HbA1c) and IR (including HOMA-IR) (103), blood lipid profile (104), and mitochondrial function (98, 102) in NAFLD patients with diabetes. Two studies demonstrated that L-carnitine can improve liver function and prognosis in patients with liver cancer after surgery (68, 106). Several randomized controlled clinical trials conducted by Malaguarnera et al. in patients with cirrhosis and hepatic encephalopathy (ranging from mild hepatic encephalopathy to coma) confirmed that L-carnitine supplementation significantly improved hepatic encephalopathy parameters (107–111). The results of these trials suggest that L-carnitine supplementation is advantageous for the treatment of cirrhosis with hepatic encephalopathy. Experimental trials in animals further conclude that L-carnitine supplementation can reduce lipid deposition and increase the metabolites related to β-oxidation, which confirm the importance of L-carnitine in controlling oxidative stress, steatosis, and fibrosis in the liver (87, 99).
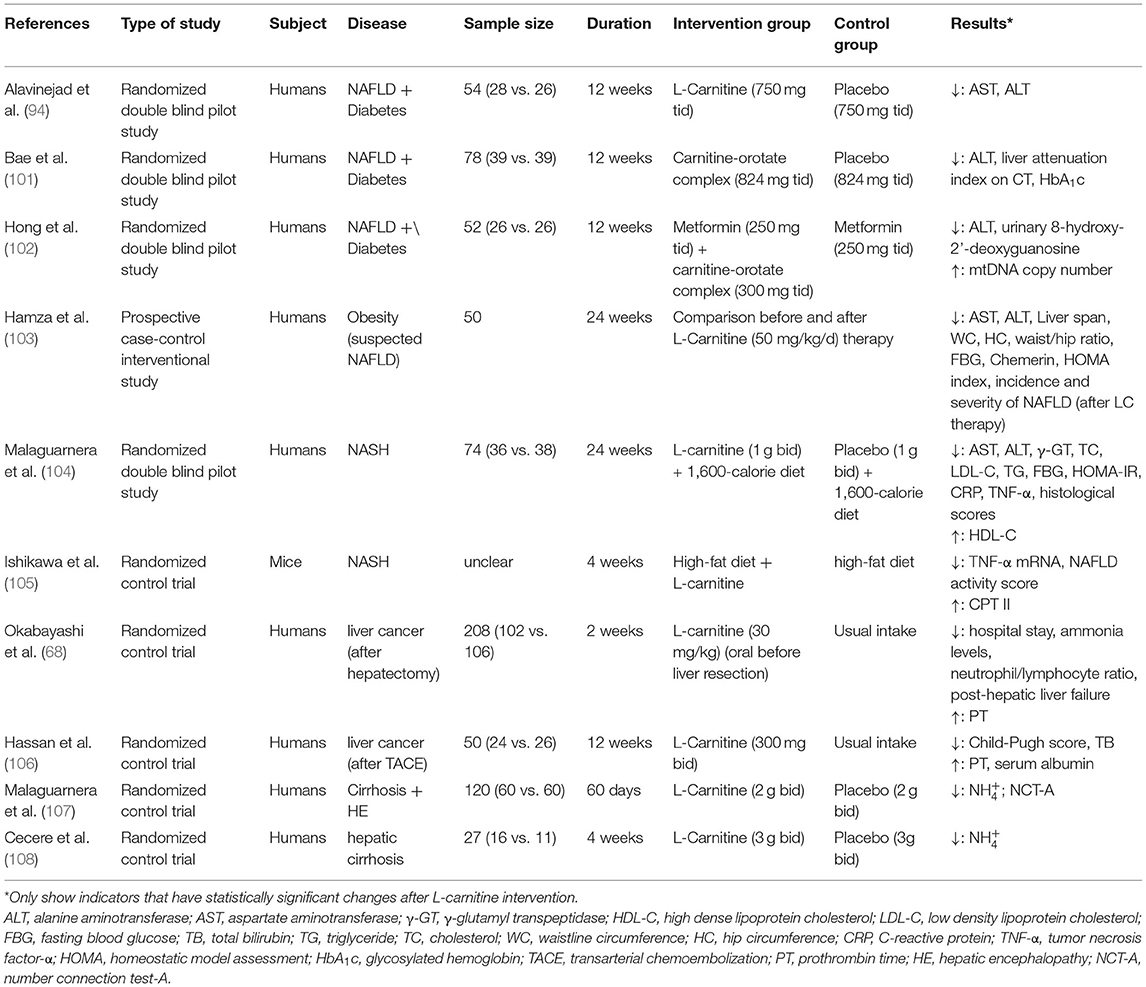
Table 2. Studies reporting the effects of L-carnitine supplementation on NAFLD progression and related diseases.
At least 11 studies reported no obvious adverse reactions in humans (112–122) and support the use of L-carnitine as an ingredient in dietary supplements. As recognized by several experiments, L-carnitine has also attracted widespread attention since it can be easily obtained from meat at a low-cost.
The recommended dose of L-carnitine is 15 g/day for healthy individuals (123) and 100–400 mg/kg/day for patients with carnitine deficiency (96). Some side effects have been observed after high-dose supplementation of L-carnitine, such as diarrhea, intestinal problems, and trimethylamine production that causes a fishy odor. However, these can be effectively addressed by appropriately reducing the supplemental dosage (124). Hence, it is important to measure the plasma L-carnitine levels to determine the optimal dose of L-carnitine for each patient.
Limitations and Prospects of Carnitine for the Treatment of NAFLD
Despite promising results, the studies on the role of L-carnitine in NAFLD, listed in Tables 1, 2, face many limitations. First, most trials used the compound preparation of L-carnitine and other substances in the intervention group. The presence of other substances can influence the outcomes of L-carnitine, making it difficult to assess its role in isolation. Second, the bioavailability of L-carnitine was not assessed in the reported studies, so there is no clear reference value for the amount of L-carnitine present in the blood after ingestion. Third, the sample sizes of the reported trials were small, which affects the credibility of the results.
In fact, there are still many unclear aspects of L-carnitine supplementation. For instance, the suggested improvement of metabolism by L-carnitine supplements is based primarily on short-term supplementation (125). A meta-analysis reported that the body weight of obese patients decreased significantly after supplementation with L-carnitine (126). However, in the subgroup analysis, it was found that L-carnitine had no effect on the body weight of subjects whose BMI was <25 kg/m2 or when L-carnitine supplementation was administered for more than 24 weeks (126). Thus, it is not explicit whether the effects of L-carnitine supplementation on NAFLD will result in similar time-dependent effects. Although carnitine supplementation was given orally in most of the studies (Tables 1, 2), some trials administered carnitine intravenously (127, 128). Therefore, the optimal route to administer L-carnitine supplements for NAFLD remains unclear. While many substances can be used as dietary supplements to improve NAFLD, no studies have directly compared them to determine which is most effective. Furthermore, the studies on the effects of L-carnitine supplements in NAFLD have yielded conflicting results. Bruls et al. showed that L-carnitine infusion could neither alleviate lipid-induced insulin resistance and metabolic inflexibility nor change the availability of skeletal muscle carnitine (129). Fujiwara et al. found that high-fat diet feeding and L-carnitine supplementation increased STAT3 phosphorylation in HCC tissues and could synergistically promote the development of liver cancer (130).
To improve the accuracy of future studies on the effects of L-carnitine supplementation in NAFLD treatment, the following aspects could be considered: use of L-carnitine in isolation in the intervention group; assessment of the bioavailability of L-carnitine; multicenter trials with larger sample size; longer follow-up to evaluate time-dependent effects; and use of intravenous L-carnitine supplementation. In addition, experimental studies are recommended to compare the effects of other substances that have been proven effective in enhancing NAFLD via L-carnitine and to understand the downstream mechanism.
Conclusion
Carnitine plays an important role in transporting FAs to the mitochondrial matrix for β-oxidation, regulating acetyl-CoA/CoA, exporting acetyl- and chain-shortened acyl products from peroxisomes, and reducing oxidative stress. L-carnitine supplementation can normalize or reduce serum levels of liver enzymes, decrease the incidence and severity of NAFLD, and improve both the lipid profile and mitochondrial function. L-carnitine may have therapeutic effects on liver diseases, including NASH, cirrhosis, HCC, alcoholic fatty liver disease, and viral hepatitis. In addition, L-carnitine supplementation is safe, low cost, and easy to administer. Due to the limited and inadequate studies on the effects of L-carnitine supplementation on NAFLD, future research should aim to determine the exact role of L-carnitine for the treatment of NAFLD.
Author Contributions
NL: study design and drafting. HZ: study design and critical review/revision. All authors contributed to the article and approved the submitted version.
Funding
This work was supported by the United Fund of the Second Hospital of Dalian Medical University and Dalian Institute of Chemical Physics, Chinese Academy of Sciences (UF-ZD-202011) and Project of Education Department of Liaoning Province (LZ2020009).
Conflict of Interest
The authors declare that the research was conducted in the absence of any commercial or financial relationships that could be construed as a potential conflict of interest.
Publisher's Note
All claims expressed in this article are solely those of the authors and do not necessarily represent those of their affiliated organizations, or those of the publisher, the editors and the reviewers. Any product that may be evaluated in this article, or claim that may be made by its manufacturer, is not guaranteed or endorsed by the publisher.
Abbreviations
ACS, acyl-CoA synthetase; ALT, alanine aminotransferase; AMPK, adenosine monophosphate-activated protein kinase; AST, aspartate aminotransferase; BMI, body mass index; CACT, carnitine-acylcarnitine translocase; CAT, catalase; CHB, chronic hepatitis B; CHC, chronic hepatitis C; COT, carnitine octyltransferase; CPT I, carnitine palmitoyltransferase I; CPT II, carnitine palmitoyltransferase II; CRP, C-reactive protein; ER, endoplasmic reticulum; FBG, fasting blood glucose; FFAs, free fatty acids; GPx, glutathione peroxidase; GR, glutathione reductase; HbA1c, glycosylated hemoglobin; HC, hip circumference; HCC, hepatocellular carcinoma; HDL-C, high dense lipoprotein cholesterol; HE, hepatic encephalopathy; HOMA, homeostatic model assessment; IGF-1, insulin-like growth factor-1; IMM, inner mitochondrial membrane; IR, insulin resistance; LCFA, long-chain fatty acids; LDL-C, low density lipoprotein cholesterol; NAFLD, non-alcoholic fatty liver disease; NASH, non-alcoholic steatohepatitis; NCT-A, number connection test-ANf-κB, nuclear factor kappa B; NOX, nicotinamide adenine dinucleotide phosphate oxidase; OMM, outer mitochondrial membrane; PCD, primary carnitine deficiency; PDHC, pyruvate dehydrogenase complex; PPARγ, peroxisome-activated receptor-γ; PT, prothrombin time; ROS, reactive oxygen species; SOD, superoxide dismutase; SOD2, superoxide dismutase 2; TACE, transarterial chemoembolization; TB, total bilirubin; TC, cholesterol; TG, triglyceride; TNF-α, tumor necrosis factor-α; UPR, unfolded protein response; WC, waistline circumference; XO, xanthine oxidase; αSMA, α-smooth muscle actin; γ-GT, γ-glutamyl transpeptidase.
References
1. Almannai M, Alfadhel M, El-Hattab AW. Carnitine inborn errors of metabolism. Molecules. (2019) 24:3251. doi: 10.3390/molecules24183251
2. Ribas GS, Vargas CR, Wajner M. L-carnitine supplementation as a potential antioxidant therapy for inherited neurometabolic disorders. Gene. (2014) 533:469–76. doi: 10.1016/j.gene.2013.10.017
3. Lee B-J, Lin J-S, Lin Y-C, Lin P-T. Effects of L-carnitine supplementation on oxidative stress and antioxidant enzymes activities in patients with coronary artery disease: a randomized, placebo-controlled trial. Nutr J. (2014) 13:79. doi: 10.1186/1475-2891-13-79
4. Komlósi K, Havasi V, Bene J, Süle N, Pajor L, Nicolai R, et al. Histopathologic abnormalities of the lymphoreticular tissues in organic cation transporter 2 deficiency: evidence for impaired B cell maturation. J Pediatr. (2007) 150:109–111.e2. doi: 10.1016/j.jpeds.2006.09.042
5. Modanloo M, Shokrzadeh M. Analyzing mitochondrial dysfunction, oxidative stress, and apoptosis: potential role of L-carnitine. Iran J Kidney Dis. (2019) 13:74–86.
6. Bene J, Hadzsiev K, Melegh B. Role of carnitine and its derivatives in the development and management of type 2 diabetes. Nutr Diabetes. (2018) 8:8. doi: 10.1038/s41387-018-0017-1
7. Xu Y, Jiang W, Chen G, Zhu W, Ding W, Ge Z, et al. L-carnitine treatment of insulin resistance: a systematic review and meta-analysis. Adv Clin Exp Med. (2017) 26:333–8. doi: 10.17219/acem/61609
8. Jamilian H, Jamilian M, Samimi M, Afshar Ebrahimi F, Rahimi M, Bahmani F, et al. Oral carnitine supplementation influences mental health parameters and biomarkers of oxidative stress in women with polycystic ovary syndrome: a randomized, double-blind, placebo-controlled trial. Gynecol Endocrinol. (2017) 33:442–7. doi: 10.1080/09513590.2017.1290071
9. Malek Mahdavi A, Mahdavi R, Kolahi S. Effects of l-Carnitine supplementation on serum inflammatory factors and matrix metalloproteinase enzymes in females with knee osteoarthritis: a randomized, double-blind, placebo-controlled pilot study. J Am Coll Nutr. (2016) 35:597–603. doi: 10.1080/07315724.2015.1068139
10. Younossi Z, Anstee QM, Marietti M, Hardy T, Henry L, Eslam M, et al. Global burden of NAFLD and NASH: trends, predictions, risk factors and prevention. Nat Rev Gastroenterol Hepatol. (2018) 15:11–20. doi: 10.1038/nrgastro.2017.109
11. Adeva-Andany MM, Calvo-Castro I, Fernández-Fernández C, Donapetry-García C, Pedre-Piñeiro AM. Significance of l-carnitine for human health. IUBMB Life. (2017) 69:578–94. doi: 10.1002/iub.1646
12. Vashistha VK, Bhushan R. Bioanalysis and enantioseparation of dl-carnitine in human plasma by the derivatization approach. Bioanalysis. (2015) 7:2477–88. doi: 10.4155/bio.15.155
13. Li J-M, Li L-Y, Zhang Y-X, Jiang Z-Y, Limbu SM, Qiao F, et al. Functional differences between l- and d-carnitine in metabolic regulation evaluated using a low-carnitine Nile tilapia model. Br J Nutr. (2019) 122:625–38. doi: 10.1017/S000711451900148X
14. Sayed-Ahmed MM, Darweesh AQ, Fatani AJ. Carnitine deficiency and oxidative stress provoke cardiotoxicity in an ifosfamide-induced Fanconi Syndrome rat model. Oxid Med Cell Longev. (2010) 3:266–74. doi: 10.4161/oxim.3.4.12859
15. Arafa HMM. Carnitine deficiency aggravates carboplatin nephropathy through deterioration of energy status, oxidant/anti-oxidant balance, and inflammatory endocoids. Toxicology. (2008) 254:51–60. doi: 10.1016/j.tox.2008.09.010
16. Zhu C, Petracci M, Li C, Fiore E, Laghi L. An untargeted metabolomics investigation of Jiulong Yak (Bos grunniens) meat by H-NMR. Foods. (2020) 9:481. doi: 10.3390/foods9040481
17. Rebouche CJ. Carnitine function and requirements during the life cycle. FASEB J. (1992) 6:3379–86. doi: 10.1096/fasebj.6.15.1464372
18. Stanley CA. Carnitine deficiency disorders in children. Ann N Y Acad Sci. (2004) 1033:42–51. doi: 10.1196/annals.1320.004
19. Tein I, Bukovac SW, Xie ZW. Characterization of the human plasmalemmal carnitine transporter in cultured skin fibroblasts. Arch Biochem Biophys. (1996) 329:145–55. doi: 10.1006/abbi.1996.0203
20. Steiber A, Kerner J, Hoppel CL. Carnitine: a nutritional, biosynthetic, and functional perspective. Mol Aspects Med. (2004) 25:455–73. doi: 10.1016/j.mam.2004.06.006
21. Bremer J. Carnitine–metabolism and functions. Physiol Rev. (1983) 63:1420–80. doi: 10.1152/physrev.1983.63.4.1420
22. Vaz FM, Wanders RJA. Carnitine biosynthesis in mammals. Biochem J. (2002) 361 (Pt 3):417–29. doi: 10.1042/bj3610417
23. Strijbis K, Vaz FM, Distel B. Enzymology of the carnitine biosynthesis pathway. IUBMB Life. (2010) 62:357–62. doi: 10.1002/iub.323
24. Rebouche CJ. Kinetics, pharmacokinetics, and regulation of L-carnitine and acetyl-L-carnitine metabolism. Ann N Y Acad Sci. (2004) 1033:30–41. doi: 10.1196/annals.1320.003
25. Schooneman MG, Vaz FM, Houten SM, Soeters MR. Acylcarnitines: reflecting or inflicting insulin resistance? Diabetes. (2013) 62:1–8. doi: 10.2337/db12-0466
26. Lahjouji K, Mitchell GA, Qureshi IA. Carnitine transport by organic cation transporters and systemic carnitine deficiency. Mol Genet Metab. (2001) 73:287–97. doi: 10.1006/mgme.2001.3207
27. Koch A, König B, Luci S, Stangl GI, Eder K. Dietary oxidised fat up regulates the expression of organic cation transporters in liver and small intestine and alters carnitine concentrations in liver, muscle and plasma of rats. Br J Nutr. (2007) 98:882–9. doi: 10.1017/S000711450775691X
28. Kou L, Sun R, Ganapathy V, Yao Q, Chen R. Recent advances in drug delivery via the organic cation/carnitine transporter 2 (OCTN2/SLC22A5). Expert Opin Ther Targets. (2018) 22:715–26. doi: 10.1080/14728222.2018.1502273
29. Kerner J, Hoppel C. Fatty acid import into mitochondria. Biochim Biophys Acta. (2000) 1486:1–17. doi: 10.1016/S1388-1981(00)00044-5
30. Yan S, Yang X-F, Liu H-L, Fu N, Ouyang Y, Qing K. Long-chain acyl-CoA synthetase in fatty acid metabolism involved in liver and other diseases: an update. World J Gastroenterol. (2015) 21:3492–8. doi: 10.3748/wjg.v21.i12.3492
31. Indiveri C, Iacobazzi V, Tonazzi A, Giangregorio N, Infantino V, Convertini P, et al. The mitochondrial carnitine/acylcarnitine carrier: function, structure and physiopathology. Mol Aspects Med. (2011) 32:223–33. doi: 10.1016/j.mam.2011.10.008
32. McGarry JD, Brown NF. The mitochondrial carnitine palmitoyltransferase system. From concept to molecular analysis. Eur J Biochem. (1997) 244:1–14. doi: 10.1111/j.1432-1033.1997.00001.x
33. Rinaldo P, Cowan TM, Matern D. Acylcarnitine profile analysis. Genet Med. (2008) 10:151–6. doi: 10.1097/GIM.0b013e3181614289
34. Savic D, Hodson L, Neubauer S, Pavlides M. The importance of the fatty acid transporter L-carnitine in non-alcoholic fatty liver disease (NAFLD). Nutrients. (2020) 12:2178. doi: 10.3390/nu12082178
35. Tanphaichitr V, Leelahagul P. Carnitine metabolism and human carnitine deficiency. Nutrition. (1993) 9:246–54.
36. Nicolson GL. Mitochondrial dysfunction and chronic disease: treatment with natural supplements. Integr Med. (2014) 13:35–43.
37. Askarpour M, Hadi A, Dehghani Kari Bozorg A, Sadeghi O, Sheikhi A, Kazemi M, et al. Effects of L-carnitine supplementation on blood pressure: a systematic review and meta-analysis of randomized controlled trials. J Hum Hypertens. (2019) 33:725–34. doi: 10.1038/s41371-019-0248-1
38. Kinugasa Y, Sota T, Ishiga N, Nakamura K, Kamitani H, Hirai M, et al. l-Carnitine supplementation in heart failure patients with preserved ejection fraction; a pilot study. Geriatr Gerontol Int. (2020) 20:1244–45. doi: 10.1111/ggi.14060
39. Nachvak SM, Shabanpur M, Mostafai R, Heidari Moghaddam R, Moludi J. L-Carnitine supplementation reduces biomarkers of inflammatory and oxidative stress in patients with coronary artery disease: a randomised controlled trial. Arch Physiol Biochem. (2020). doi: 10.1080/13813455.2020.1797102. [Epub ahead of print].
40. Hanai T, Shiraki M, Imai K, Suetugu A, Takai K, Shimizu M. Usefulness of carnitine supplementation for the complications of liver cirrhosis. Nutrients. (2020) 12:1915. doi: 10.3390/nu12071915
41. Yarizadh H, Shab-Bidar S, Zamani B, Vanani AN, Baharlooi H, Djafarian K. The effect of L-carnitine supplementation on exercise-induced muscle damage: a systematic review and meta-analysis of randomized clinical trials. J Am Coll Nutr. (2020) 39:457–68. doi: 10.1080/07315724.2019.1661804
42. Askarpour M, Hadi A, Symonds ME, Miraghajani M, Omid S, Sheikhi A, et al. Efficacy of l-carnitine supplementation for management of blood lipids: a systematic review and dose-response meta-analysis of randomized controlled trials. Nutr Metab Cardiovasc Dis. (2019) 29:1151–67. doi: 10.1016/j.numecd.2019.07.012
43. Hajihashemi P, Askari G, Khorvash F, Reza Maracy M, Nourian M. The effects of concurrent Coenzyme Q10, L-carnitine supplementation in migraine prophylaxis: a randomized, placebo-controlled, double-blind trial. Cephalalgia. (2019) 39:648–54. doi: 10.1177/0333102418821661
44. Kepka A, Ochocinska A, Borzym-Kluczyk M, Skorupa E, Stasiewicz-Jarocka B, Chojnowska S, et al. Preventive role of L-carnitine and balanced diet in Alzheimer's disease. Nutrients. (2020) 12:1987. doi: 10.3390/nu12071987
45. Wegermann K, Suzuki A, Mavis AM, Abdelmalek MF, Diehl AM, Moylan CA. Tackling NAFLD: three targeted populations. Hepatology. (2020) 73:1199–206. doi: 10.1002/hep.31533
46. Draijer LG, Froon-Torenstra D, van Weeghel M, Vaz FM, Bohte AE, Holleboom AG, et al. Lipidomics in nonalcoholic fatty liver disease: exploring serum lipids as biomarkers for pediatric nonalcoholic fatty liver disease. J Pediatr Gastroenterol Nutr. (2020) 71:433–9. doi: 10.1097/MPG.0000000000002875
47. Buzzetti E, Pinzani M, Tsochatzis EA. The multiple-hit pathogenesis of non-alcoholic fatty liver disease (NAFLD). Metabolism. (2016) 65:1038–48. doi: 10.1016/j.metabol.2015.12.012
48. Tsochatzis EA, Papatheodoridis GV, Archimandritis AJ. Adipokines in nonalcoholic steatohepatitis: from pathogenesis to implications in diagnosis and therapy. Mediators Inflamm. (2009) 2009:831670. doi: 10.1155/2009/831670
49. Shao M, Ye Z, Qin Y, Wu T. Abnormal metabolic processes involved in the pathogenesis of non-alcoholic fatty liver disease (Review). Exp Ther Med. (2020) 20:26. doi: 10.3892/etm.2020.9154
50. Ibrahim SH, Kohli R, Gores GJ. Mechanisms of lipotoxicity in NAFLD and clinical implications. J Pediatr Gastroenterol Nutr. (2011) 53:131–40. doi: 10.1097/MPG.0b013e31822578db
51. Knottnerus SJG, Bleeker JC, Wüst RCI, Ferdinandusse S, Ijlst L, Wijburg FA, et al. Disorders of mitochondrial long-chain fatty acid oxidation and the carnitine shuttle. Rev Endocr Metab Disord. (2018) 19:93–106. doi: 10.1007/s11154-018-9448-1
52. Meex RCR, Blaak EE. Mitochondrial dysfunction is a key pathway that links saturated fat intake to the development and progression of NAFLD. Mol Nutr Food Res. (2020) 65:e1900942. doi: 10.1002/mnfr.201900942
53. Jun DW, Cho WK, Jun JH, Kwon HJ, Jang K-S, Kim H-J, et al. Prevention of free fatty acid-induced hepatic lipotoxicity by carnitine via reversal of mitochondrial dysfunction. Liver Int. (2011) 31:1315–24. doi: 10.1111/j.1478-3231.2011.02602.x
54. Perez-Carreras M, Del Hoyo P, Martin M, Rubio J, Martin A, Castellano G, et al. Defective hepatic mitochondrial respiratory chain in patients with non-alcoholic steatohepatitis. Hepatology. (2003) 38:999–1007. doi: 10.1002/hep.1840380426
55. Ringseis R, Keller J, Eder K. Role of carnitine in the regulation of glucose homeostasis and insulin sensitivity: evidence from in vivo and in vitro studies with carnitine supplementation and carnitine deficiency. Eur J Nutr. (2012) 51:1–18. doi: 10.1007/s00394-011-0284-2
56. Choi JW, Ohn JH, Jung HS, Park YJ, Jang HC, Chung SS, et al. Carnitine induces autophagy and restores high-fat diet-induced mitochondrial dysfunction. Metabolism. (2018) 78:43–51. doi: 10.1016/j.metabol.2017.09.005
57. Belfiore A, Frasca F, Pandini G, Sciacca L, Vigneri R. Insulin receptor isoforms and insulin receptor/insulin-like growth factor receptor hybrids in physiology and disease. Endocr Rev. (2009) 30:586–623. doi: 10.1210/er.2008-0047
58. Bailes J, Soloviev M. Insulin-like growth factor-1 (IGF-1) and its monitoring in medical diagnostic and in sports. Biomolecules. (2021) 11:217. doi: 10.3390/biom11020217
59. Cubbon RM, Kearney MT, Wheatcroft SB. Endothelial IGF-1 receptor signalling in diabetes and insulin resistance. Trends Endocrinol Metab. (2016) 27:96–104. doi: 10.1016/j.tem.2015.11.009
60. Li S, Tan H-Y, Wang N, Zhang Z-J, Lao L, Wong C-W, et al. The role of oxidative stress and antioxidants in liver diseases. Int J Mol Sci. (2015) 16:26087–124. doi: 10.3390/ijms161125942
61. Muriel P, Gordillo KR. Role of oxidative stress in liver health and disease. Oxid Med Cell Longev. (2016) 2016:9037051. doi: 10.1155/2016/9037051
62. Cortez-Pinto H, Chatham J, Chacko VP, Arnold C, Rashid A, Diehl AM. Alterations in liver ATP homeostasis in human nonalcoholic steatohepatitis: a pilot study. JAMA. (1999) 282:1659–64. doi: 10.1001/jama.282.17.1659
63. Serviddio G, Bellanti F, Tamborra R, Rollo T, Romano AD, Giudetti AM, et al. Alterations of hepatic ATP homeostasis and respiratory chain during development of non-alcoholic steatohepatitis in a rodent model. Eur J Clin Invest. (2008) 38:245–52. doi: 10.1111/j.1365-2362.2008.01936.x
64. Limdi JK, Hyde GM. Evaluation of abnormal liver function tests. Postgrad Med J. (2003) 79:307–12. doi: 10.1136/pmj.79.932.307
65. Selimoglu MA, Aydogdu S, Yagci RV, Huseyinov A. Plasma and liver carnitine status of children with chronic liver disease and cirrhosis. Pediatr Int. (2001) 43:391–5. doi: 10.1046/j.1442-200X.2001.01423.x
66. Rudman D, Sewell CW, Ansley JD. Deficiency of carnitine in cachectic cirrhotic patients. J Clin Invest. (1977) 60:716–23. doi: 10.1172/JCI108824
67. Musso G, Gambino R, Cassader M, Pagano G. A meta-analysis of randomized trials for the treatment of nonalcoholic fatty liver disease. Hepatology. (2010) 52:79–104. doi: 10.1002/hep.23623
68. Okabayashi T, Sui K, Mastumoto T, Iwata J, Morita S, Iiyama T, et al. l-Carnitine improves postoperative liver function in hepatectomized patients. J Parenter Enteral Nutr. (2020) 44:823–30. doi: 10.1002/jpen.1720
69. Famularo G, De Simone C, Trinchieri V, Mosca L. Carnitines and its congeners: a metabolic pathway to the regulation of immune response and inflammation. Ann N Y Acad Sci. (2004) 1033:132–8. doi: 10.1196/annals.1320.012
70. Quillet-Mary A, Jaffrézou JP, Mansat V, Bordier C, Naval J, Laurent G. Implication of mitochondrial hydrogen peroxide generation in ceramide-induced apoptosis. J Biol Chem. (1997) 272:21388–95. doi: 10.1074/jbc.272.34.21388
71. Chapoy PR, Angelini C, Brown WJ, Stiff JE, Shug AL, Cederbaum SD. Systemic carnitine deficiency–a treatable inherited lipid-storage disease presenting as Reye's syndrome. N Engl J Med. (1980) 303:1389–94. doi: 10.1056/NEJM198012113032403
72. Pike LS, Smift AL, Croteau NJ, Ferrick DA, Wu M. Inhibition of fatty acid oxidation by etomoxir impairs NADPH production and increases reactive oxygen species resulting in ATP depletion and cell death in human glioblastoma cells. Biochim Biophys Acta. (2011) 1807:726–34. doi: 10.1016/j.bbabio.2010.10.022
73. Flanagan JL, Simmons PA, Vehige J, Willcox MD, Garrett Q. Role of carnitine in disease. Nutr Metab. (2010) 7:30. doi: 10.1186/1743-7075-7-30
74. Lheureux PER, Hantson P. Carnitine in the treatment of valproic acid-induced toxicity. Clin Toxicol. (2009) 47:101–11. doi: 10.1080/15563650902752376
75. Mahale RR, Mehta A, Timmappaya A, Srinivasa R. Primary carnitine deficiency as a cause of metabolic leukoencephalopathy: report of one case. Neurol India. (2016) 64:166–8. doi: 10.4103/0028-3886.173650
76. Buist NRM. Historical perspective on clinical trials of carnitine in children and adults. Ann Nutr Metab. (2016) 68(Suppl. 3):1–4. doi: 10.1159/000448320
77. Sachan DS, Rhew TH, Ruark RA. Ameliorating effects of carnitine and its precursors on alcohol-induced fatty liver. Am J Clin Nutr. (1984) 39:738–44. doi: 10.1093/ajcn/39.5.738
78. DeCarli LM, Lieber CS. Fatty liver in the rat after prolonged intake of ethanol with a nutritionally adequate new liquid diet. J Nutr. (1967) 91:331–6. doi: 10.1093/jn/91.3_Suppl.331
79. Blomstrand R, Kager L, Lantto O. Studies on the ethanol-induced decrease of fatty acid oxidation in rat and human liver slices. Life Sci. (1973) 13:1131–41. doi: 10.1016/0024-3205(73)90380-9
80. Jun DW, Kim BI, Cho YK, Kim HJ, Kwon YO, Park SY, et al. Efficacy and safety of entecavir plus carnitine complex (GODEX®) compared to entecavir monotherapy in patient with ALT elevated chronic hepatitis B: randomized, multicenter open-label trials. The GOAL study. Clin Mol Hepatol. (2013) 19:165–72. doi: 10.3350/cmh.2013.19.2.165
81. Jung YW, Kim M, Kim BK, Park JY, Kim DY, Ahn SH, et al. Influence of besifovir dipivoxil maleate combined with L-carnitine on hepatic steatosis in patients with chronic hepatitis B. J Korean Med Sci. (2020) 35:e104. doi: 10.3346/jkms.2020.35.e104
82. Abbasnezhad A, Hasanavand A, Falahi E, Kashkooli S, Asbaghi O, Choghakhori R. Effect of L-carnitine supplementation on lipid profiles of patients with liver disease: a systematic review and meta-analysis. Prev Nutr Food Sci. (2020) 25:124–32. doi: 10.3746/pnf.2020.25.2.124
83. Tsukuda Y, Suda G, Tsunematsu S, Ito J, Sato F, Terashita K, et al. Anti-adipogenic and antiviral effects of l-carnitine on hepatitis C virus infection. J Med Virol. (2017) 89:857–66. doi: 10.1002/jmv.24692
84. Mandard S, Müller M, Kersten S. Peroxisome proliferator-activated receptor alpha target genes. Cell Mol Life Sci. (2004) 61:393–416. doi: 10.1007/s00018-003-3216-3
85. Couturier A, Ringseis R, Most E, Eder K. Pharmacological doses of niacin stimulate the expression of genes involved in carnitine uptake and biosynthesis and improve the carnitine status of obese Zucker rats. BMC Pharmacol Toxicol. (2014) 15:37. doi: 10.1186/2050-6511-15-37
86. Ringseis R, Wen G, Eder K. Regulation of genes involved in carnitine homeostasis by PPARα across different species (rat, mouse, pig, cattle, chicken, and human). PPAR Res. (2012) 2012:868317. doi: 10.1155/2012/868317
87. Mollica G, Senesi P, Codella R, Vacante F, Montesano A, Luzi L, et al. L-carnitine supplementation attenuates NAFLD progression and cardiac dysfunction in a mouse model fed with methionine and choline-deficient diet. Dig Liver Dis. (2020) 52:314–23. doi: 10.1016/j.dld.2019.09.002
88. Scalera A, Tarantino G. Could metabolic syndrome lead to hepatocarcinoma via non-alcoholic fatty liver disease? World J Gastroenterol. (2014) 20:9217–28. doi: 10.3748/wjg.v20.i28.9217
89. Zhang C, Bjornson E, Arif M, Tebani A, Lovric A, Benfeitas R, et al. The acute effect of metabolic cofactor supplementation: a potential therapeutic strategy against non-alcoholic fatty liver disease. Mol Syst Biol. (2020) 16:e9495. doi: 10.15252/msb.209495
90. Montesano A, Senesi P, Vacante F, Mollica G, Benedini S, Mariotti M, et al. L-Carnitine counteracts in vitro fructose-induced hepatic steatosis through targeting oxidative stress markers. J Endocrinol Invest. (2020) 43:493–503. doi: 10.1007/s40618-019-01134-2
91. Salic K, Gart E, Seidel F, Verschuren L, Caspers M, van Duyvenvoorde W, et al. Combined treatment with L-carnitine and nicotinamide riboside improves hepatic metabolism and attenuates obesity and liver steatosis. Int J Mol Sci. (2019) 20:4359. doi: 10.3390/ijms20184359
92. Li N, Liu Y, Yu S, Hu B, Zhao H. Composite BMI and waist-to-height ratio index for risk assessment of non-alcoholic fatty liver disease in adult populations. Hepat Mon. (2020) 20:e103607. doi: 10.5812/hepatmon.103607
93. Mahjoubin-Tehran M, De Vincentis A, Mikhailidis DP, Atkin SL, Mantzoros CS, Jamialahmadi T, et al. Non-alcoholic fatty liver disease and steatohepatitis: state of the art on effective therapeutics based on the gold standard method for diagnosis. Mol Metab. (2021) 50:101049. doi: 10.1016/j.molmet.2020.101049
94. Alavinejad P, Zakerkish M, Hajiani E, Hashemi SJ, Chobineh M, Moghaddam EK. Evaluation of l-carnitine efficacy in the treatment of non-alcoholic fatty liver disease among diabetic patients: a randomized double blind pilot study. J Gastroenterol Hepatol Res. (2016) 5:2191–5. doi: 10.17554/j.issn.2224-3992.2016.05.662
95. Abolfathi M, Mohd-Yusof B-N, Hanipah ZN, Mohd Redzwan S, Yusof LM, Khosroshahi MZ. The effects of carnitine supplementation on clinical characteristics of patients with non-alcoholic fatty liver disease: a systematic review and meta-analysis of randomized controlled trials. Comp Ther Med. (2020) 48:102273. doi: 10.1016/j.ctim.2019.102273
96. Pirmadah F, Ramezani-Jolfaie N, Mohammadi M, Talenezhad N, Clark CCT, Salehi-Abargouei A. Does L-carnitine supplementation affect serum levels of enzymes mainly produced by liver? A systematic review and meta-analysis of randomized controlled clinical trials. Eur J Nutr. (2020) 59:1767–83. doi: 10.1007/s00394-019-02068-4
97. Somi MH, Fatahi E, Panahi J, Havasian MR, Judaki A. Data from a randomized and controlled trial of L-carnitine prescription for the treatment for non- alcoholic fatty liver disease. Bioinformation. (2014) 10:575–9. doi: 10.6026/97320630010575
98. Lim CY, Jun DW, Jang SS, Cho WK, Chae JD, Jun JH. Effects of carnitine on peripheral blood mitochondrial DNA copy number and liver function in non-alcoholic fatty liver disease. Korean J Gastroenterol. (2010) 55:384–9. doi: 10.4166/kjg.2010.55.6.384
99. Fujisawa K, Takami T, Matsuzaki A, Matsumoto T, Yamamoto N, Terai S, et al. Evaluation of the effects of L-carnitine on medaka (Oryzias latipes) fatty liver. Sci Rep. (2017) 7:2749. doi: 10.1038/s41598-017-02924-5
100. Kathirvel E, Morgan K, French SW, Morgan TR. Acetyl-L-carnitine and lipoic acid improve mitochondrial abnormalities and serum levels of liver enzymes in a mouse model of nonalcoholic fatty liver disease. Nutr Res. (2013) 33:932–41. doi: 10.1016/j.nutres.2013.08.001
101. Bae JC, Lee WY, Yoon KH, Park JY, Son HS, Han KA, et al. Improvement of nonalcoholic fatty liver disease with carnitine-orotate complex in type 2 diabetes (CORONA): a randomized controlled trial. Diabetes Care. (2015) 38:1245–52. doi: 10.2337/dc14-2852
102. Hong ES, Kim EK, Kang SM, Khang AR, Choi SH, Park KS, et al. Effect of carnitine-orotate complex on glucose metabolism and fatty liver: a double-blind, placebo-controlled study. J Gastroenterol Hepatol. (2014) 29:1449–57. doi: 10.1111/jgh.12536
103. Hamza RT, Elkabbany ZA, Shedid AM, Hamed AI, Ebrahim AO. Serum chemerin in obese children and adolescents before and after L-carnitine therapy: relation to nonalcoholic fatty liver disease and other features of metabolic syndrome. Arch Med Res. (2016) 47:541–9. doi: 10.1016/j.arcmed.2016.11.010
104. Malaguarnera M, Gargante MP, Russo C, Antic T, Vacante M, Malaguarnera M, et al. L-carnitine supplementation to diet: a new tool in treatment of nonalcoholic steatohepatitis–a randomized and controlled clinical trial. Am J Gastroenterol. (2010) 105:1338–45. doi: 10.1038/ajg.2009.719
105. Ishikawa H, Takaki A, Tsuzaki R, Yasunaka T, Koike K, Shimomura Y, et al. L-carnitine prevents progression of non-alcoholic steatohepatitis in a mouse model with upregulation of mitochondrial pathway. PLoS ONE. (2014) 9:e100627. doi: 10.1371/journal.pone.0100627
106. Hassan A, Tsuda Y, Asai A, Yokohama K, Nakamura K, Sujishi T, et al. Effects of oral L-carnitine on liver functions after transarterial chemoembolization in intermediate-stage HCC patients. Mediators Inflamm. (2015) 2015:608216. doi: 10.1155/2015/608216
107. Malaguarnera M, Pistone G, Astuto M, Dell'Arte S, Finocchiaro G, Lo Giudice E, et al. L-Carnitine in the treatment of mild or moderate hepatic encephalopathy. Dig Dis. (2003) 21:271–5. doi: 10.1159/000073347
108. Malaguarnera M, Vacante M, Motta M, Giordano M, Malaguarnera G, Bella R, et al. Acetyl-L-carnitine improves cognitive functions in severe hepatic encephalopathy: a randomized and controlled clinical trial. Metab Brain Dis. (2011) 26:281–9. doi: 10.1007/s11011-011-9260-z
109. Malaguarnera M, Pistone G, Elvira R, Leotta C, Scarpello L, Liborio R. Effects of L-carnitine in patients with hepatic encephalopathy. World J Gastroenterol. (2005) 11:7197–202. doi: 10.3748/wjg.v11.i45.7197
110. Malaguarnera M, Pistone G, Astuto M, Vecchio I, Raffaele R, Lo Giudice E, et al. Effects of L-acetylcarnitine on cirrhotic patients with hepatic coma: randomized double-blind, placebo-controlled trial. Dig Dis Sci. (2006) 51:2242–7. doi: 10.1007/s10620-006-9187-0
111. Malaguarnera M, Gargante MP, Cristaldi E, Vacante M, Risino C, Cammalleri L, et al. Acetyl-L-carnitine treatment in minimal hepatic encephalopathy. Dig Dis Sci. (2008) 53:3018–25. doi: 10.1007/s10620-008-0238-6
112. Iliceto S, Scrutinio D, Bruzzi P, D'Ambrosio G, Boni L, Di Biase M, et al. Effects of L-carnitine administration on left ventricular remodeling after acute anterior myocardial infarction: the L-carnitine ecocardiografia digitalizzata infarto miocardico (CEDIM) trial. J Am Coll Cardiol. (1995) 26:380–7. doi: 10.1016/0735-1097(95)80010-E
113. Van Oudheusden LJ, Scholte HR. Efficacy of carnitine in the treatment of children with attention-deficit hyperactivity disorder. Prostaglandins Leukot Essent Fatty Acids. (2002) 67:33–8. doi: 10.1054/plef.2002.0378
114. Ellaway C, Williams K, Leonard H, Higgins G, Wilcken B, Christodoulou J. Rett syndrome: randomized controlled trial of L-carnitine. J Child Neurol. (1999) 14:162–7. doi: 10.1177/088307389901400306
115. Benvenga S, Ruggeri RM, Russo A, Lapa D, Campenni A, Trimarchi F. Usefulness of L-carnitine, a naturally occurring peripheral antagonist of thyroid hormone action, in iatrogenic hyperthyroidism: a randomized, double-blind, placebo-controlled clinical trial. J Clin Endocrinol Metab. (2001) 86:3579–94. doi: 10.1210/jcem.86.8.7747
116. Iyer R, Gupta A, Khan A, Hiremath S, Lokhandwala Y. Does left ventricular function improve with L-carnitine after acute myocardial infarction? J Postgrad Med. (1999) 45:38–41.
117. Löster H, Miehe K, Punzel M, Stiller O, Pankau H, Schauer J. Prolonged oral L-carnitine substitution increases bicycle ergometer performance in patients with severe, ischemically induced cardiac insufficiency. Cardiovasc Drugs Ther. (1999) 13:537–46. doi: 10.1023/A:1007883822625
118. Lenzi A, Sgrò P, Salacone P, Paoli D, Gilio B, Lombardo F, et al. A placebo-controlled double-blind randomized trial of the use of combined l-carnitine and l-acetyl-carnitine treatment in men with asthenozoospermia. Fertil Steril. (2004) 81:1578–84. doi: 10.1016/j.fertnstert.2003.10.034
119. Brooks JO, Yesavage JA, Carta A, Bravi D. Acetyl L-carnitine slows decline in younger patients with Alzheimer's disease: a reanalysis of a double-blind, placebo-controlled study using the trilinear approach. Int Psychogeriatr. (1998) 10:193–203. doi: 10.1017/S1041610298005304
120. Turpeinen AK, Kuikka JT, Vanninen E, Yang J, Uusitupa MI. Long-term effect of acetyl-L-carnitine on myocardial 123I-MIBG uptake in patients with diabetes. Clin Auton Res. (2000) 10:13–6. doi: 10.1007/BF02291384
121. Torrioli MG, Vernacotola S, Mariotti P, Bianchi E, Calvani M, De Gaetano A, et al. Double-blind, placebo-controlled study of L-acetylcarnitine for the treatment of hyperactive behavior in fragile X syndrome. Am J Med Genet. (1999) 87:366–8. doi: 10.1002/(SICI)1096-8628(19991203)87:4<366::AID-AJMG18>3.0.CO;2-F
122. Dal Lago A, De Martini D, Flore R, Gaetani E, Gasbarrini A, Gerardino L, et al. Effects of propionyl-L-carnitine on peripheral arterial obliterative disease of the lower limbs: a double-blind clinical trial. Drugs Exp Clin Res. (1999) 25:29–36.
123. Mosah HA, Khazaal FAK, Sahib HB, AS H. Effect of l-carnitine and raspberry ketones on metabolic parameters in iraqi obese females, a comparative study. Int J Pharm Sci Rev Res. (2015) 31:63–8.
124. Longo N, Amat di San Filippo C, Pasquali M. Disorders of carnitine transport and the carnitine cycle. Am J Med Genet C Semin Med Genet. (2006) 142C:77–85. doi: 10.1002/ajmg.c.30087
125. Brass EP. Supplemental carnitine and exercise. Am J Clin Nutr. (2000) 72(2 Suppl.):618S–23S. doi: 10.1093/ajcn/72.2.618S
126. Askarpour M, Hadi A, Miraghajani M, Symonds ME, Sheikhi A, Ghaedi E. Beneficial effects of l-carnitine supplementation for weight management in overweight and obese adults: an updated systematic review and dose-response meta-analysis of randomized controlled trials. Pharmacol Res. (2020) 151:104554. doi: 10.1016/j.phrs.2019.104554
127. Katalinic L, Krtalic B, Jelakovic B, Basic-Jukic N. The unexpected effects of L-carnitine supplementation on lipid metabolism in hemodialysis patients. Kidney Blood Press Res. (2018) 43:1113–20. doi: 10.1159/000491807
128. Delaney CL, Spark JI, Thomas J, Wong YT, Chan LT, Miller MD. A systematic review to evaluate the effectiveness of carnitine supplementation in improving walking performance among individuals with intermittent claudication. Atherosclerosis. (2013) 229:1–9. doi: 10.1016/j.atherosclerosis.2013.03.004
129. Bruls YMH, Op den Kamp YJM, Phielix E, Lindeboom L, Havekes B, Schaart G, et al. L-carnitine infusion does not alleviate lipid-induced insulin resistance and metabolic inflexibility. PLoS ONE. (2020) 15:e0239506. doi: 10.1371/journal.pone.0239506
Keywords: carnitine, non-alcoholic fatty liver disease, L-carnitine supplementation, targeted therapy, therapeutic diet
Citation: Li N and Zhao H (2021) Role of Carnitine in Non-alcoholic Fatty Liver Disease and Other Related Diseases: An Update. Front. Med. 8:689042. doi: 10.3389/fmed.2021.689042
Received: 31 March 2021; Accepted: 15 June 2021;
Published: 09 August 2021.
Edited by:
Oscar Escribano, Complutense University of Madrid, SpainReviewed by:
Adriaan Holleboom, Academic Medical Center, NetherlandsTom L. Broderick, Midwestern University, United States
Copyright © 2021 Li and Zhao. This is an open-access article distributed under the terms of the Creative Commons Attribution License (CC BY). The use, distribution or reproduction in other forums is permitted, provided the original author(s) and the copyright owner(s) are credited and that the original publication in this journal is cited, in accordance with accepted academic practice. No use, distribution or reproduction is permitted which does not comply with these terms.
*Correspondence: Hui Zhao, emhhb2h1aUBkbXUuZWR1LmNu
†ORCID: Na Li orcid.org/0000-0003-2567-3992
Hui Zhao orcid.org/0000-0001-6358-7978