- 1Department of Emergency, The Second Hospital of Hebei Medical University, Shijiazhuang, China
- 2Translational Medicine Research Center, Medical Innovation Research Division and Fourth Medical Center of the Chinese PLA General Hospital, Beijing, China
Background: Sepsis can cause unpredictable harm, and early identification of risk for mortality may be conducive to clinical diagnosis. The present study proposes to assess the efficacy of the monocyte/high-density lipoprotein cholesterol ratio (MHR) combined with the neutrophil/lymphocyte ratio (NLR) on the day of admission in predictive efficacy in the 28-day mortality risk in critical patients with sepsis.
Material and Methods: We administered observational and retrospective cohort research from a single center. The correlation of the clinical variables, together with the system severity scores of APACHE II and SOFA, are displayed by correlation analysis, and a Cox regression model could be performed to screen the independent risk factors and estimate the capacity of multiple markers in predicting 28-day mortality. The receiver operating characteristic (ROC) curve served as an applied method to output cutoff values for the diagnosis and prognostic risk, and the area under the ROC curve and net reclassification improvement index (NRI), as well as integrated discrimination improvement index (IDI) were employed to assess the feasibility of multiple parameters for predictive value in 28-day mortality of septic patients.
Results: The study enrolled 274 eligible patients with sepsis. The correlation analysis indicated NLR and MHR were related to the sepsis severity. A multivariate Cox regression analysis indicated that NLR together with MHR displayed a close relation to death rate after adjusting for other potential confounders (NLR, HR = 1.404 [95% CI 1.170–1.684], P < 0.001; MHR, HR = 1.217 [95% CI 1.112–1.331], P < 0.001). The AUC of NLR, MHR, NLR_MHR was 0.827, 0.876, and 0.934, respectively. The addition on the biomarker NLR_MHR to the prediction model improved IDI by 18.5% and NRI by 37.8%.
Conclusions: Our findings suggest that NLR and MHR trend to an elevated level in non-surviving patients with sepsis. Evaluation of NLR_MHR, an independent risk factor for increased mortality, might improve the predictive efficacy for 28-day mortality risk in septic patients.
Introduction
Sepsis is a complicated, life-threatening disorder attributed to a dysregulated host response to infection; eventually acute multiorgan dysfunction develops with high morbidity and mortality (1–3). This syndrome, one of the major causes of death in the intensive care unit (ICU), is universally accepted as a public health issue with a considerable economic burden and tremendous concern for critical patients (4–6). According to the reports from the Centers for Disease Control, the incidence of sepsis is approximately more than 750,000 cases per year globally, and the morbidity of sepsis in all ICU admissions is as high as 27% (7, 8). Because of sepsis being the final pathway to death from most infections, it remains at a high mortality at around 25–30% in hospitalized sepsis patients that are equivalent to killing tens of millions of individuals worldwide annually (9–11). Despite constant progress in patient administration and therapeutic strategies, sepsis remains an intractable problem in clinical care because of the limitations in the gold standard of sepsis diagnosis as well as timely identification, which hinders the implement by reference in epidemiological studies.
The international consensus definition for sepsis and septic shock (Sepsis 3.0) has redefined sepsis as fatal multiple organ dysfunction with systemic interaction between excessive inflammatory response and a suppressive immune state in response to an infectious organism or tissue injury (12). The consensus of Sepsis 3.0 emphasizes the immune system as the foundation at which host-derived molecules and foreign products induced by pathogenic microorganism interact with pathogen recognition receptors expressed on immune cells, which cause unbalanced activation of innate immunity (13). Moreover, it emphasizes that the interactions between systemic inflammation and oxidative stress have particularly been accused of performing crucial impact on the pathogenesis of sepsis (14–16). Increasing evidence supports the viewpoint that both immune dysfunction and oxidative stress are critical in the pathogenesis of sepsis. Along with the continual amplification of immune dissonance, oxidative stress is exacerbated during sepsis, finally leading to the redox cascade of cell damage, impairment of mitochondrial function, and aggravation of inflammation (17). Thus, septic patients at risk of immune deterioration and oxidative storm should be identified prior to the onset of organ dysfunction. Identifications of immune and oxidative-related predictors in sepsis have great potential to improve the diagnosis, assessment, and treatment of septic complications.
Although various biomarkers have been improved and applied in evaluation of the capacity of early recognition and prediction in sepsis (18–20), their exact values are still uncertain or controversial. The neutrophil/lymphocyte ratio (NLR) is a rapidly available parameter that is previously reported as reflecting the severity of the disease in critically ill patients and notably correlates with in-hospital mortality in sepsis (21). However, whether NLR predicts septic prognosis in the long term remains controversial, and the reason is that NLR is representative only of the quantity of immune cells rather than functional and oxidative status when sepsis occurs. Monocyte/high-density lipoprotein cholesterol ratio (MHR) is proven to be a parameter of systemic inflammation and oxidative stress in many inflammatory diseases (22–24). Hence, it is speculated that MHR together with NLR might further improve predicting mortality risk in septic patients better than a single indicator. In light of this evidence, we propose to investigate the effectiveness of MHR combined with NLR in predicting 28-day mortality in patients with sepsis.
Materials and Methods
Subject Design and Patient Enrollment
This single-center retrospective observational study was administrated in septic patients who were admitted to the emergency ICU (EICU) of the Second Hospital of Hebei Medical University between January 2015 and December 2020; 2045 adult patients (aged >18 years) who conformed to the diagnostic standard of the Surviving Sepsis Guideline (Sepsis 3.0) (12) were enrolled in this study. We excluded patients with a reference standard that included (1) younger than 18 years old, (2) EICU hospitalized <24 h, (3) immunodeficient state: systemically solid tumor or hematological malignances [active stage or decubation within 5 years; recipients of autotransplantation or allotransplantation in stem cell; solid organ transplantation, long-term application for hormonotherapy (>30 days) or high dose (>1 mg/kg/day) of steroids (>14 days)] or currently on immunosuppressive drug for more than 30 days, (4) acute or chronic liver disease, (5) using antihyperlipidemic therapy, (6) synsemantic or missing medical data registers. The enrolled participants signed informed consent forms and were observed for at least 28 days. The patients accepted professional medical care in the whole hospitalized course and normative treatment complying with the Surviving Sepsis Campaign Guideline (12). The research was conducted in accordance with the principle of the Declaration of Helsinki and approved by the Medical Ethics Committee established in the Second Hospital of Hebei Medical University, Shijiazhuang, China (Figure 1).
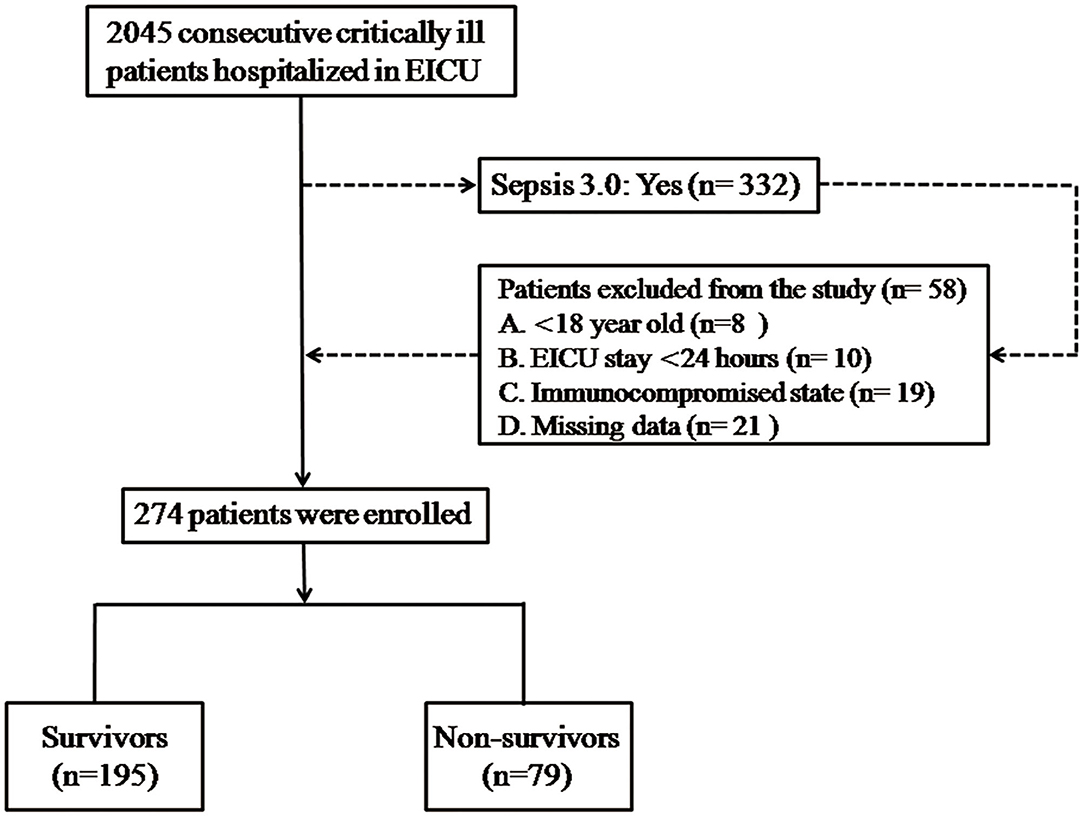
Figure 1. Flowchart of the enrolled patients. There were 2,045 adult patients who met the diagnostic criteria of the Surviving Sepsis Guideline (Sepsis 3.0), who were tested in this study, and 264 enrolled patients were stratified into the survivor and non-survivor groups. Demographic data and clinical and laboratory parameters were compared between the survivor and non-survivor groups.
Data Extraction Process
Demographic data and clinical and laboratory parameters of enrolled patients were collected from the electronic medical record system in the hospital. Patients' demographic characteristics are documented in detail in the records, and complications; source of infection; and vital signs, including body temperature, systolic blood pressure (SBP), and heart rate are collected. Laboratory parameters were obtained from the examination of blood samples in the antecubital vein within 3 h after admission to the EICU. Routine hemogram was determined with the EB-10 (F4) mechanized hematology analytical facility (Sysmetix, Mobe, Japan), and procalcitonin (PCT) level was tested by the luminescence immunoassay instrument (Goche, socobas e211). Electromagnetic biochemical analysis equipment 730-128 (Mitachi High Technologies, Japan) was used to determine the biochemical parameters. C-reactive protein (CRP) level was measured by applying the CRP mensurable device (Quickly Read system). In addition, MHR was obtained by calculating a ratio of absolute monocyte count to high-density lipoprotein (HDL) cholesterol content. NLR was computed by distributing the neutrophil-to-lymphocyte count. Of note, each participant is evaluated as to severity degree by means of Sequential Organ Failure Assessment (SOFA) and Acute Physiology and Chronic Health Evaluation II (APACHE II) scores. We employed the all-cause mortality of hospitalization as a primary endpoint in the current study.
Statistical Analysis
All statistical processes were performed by using SPSS software (version 26.0; IBM Corporation, St. Louis, Missouri, USA). Enrolled patients, who were stratified into survivor and non-survivor groups in line with 28-day survival status, are compared by the baseline characteristics accordingly. Continuous data that conformed to normal distribution were expressed as mean ± standard deviation (SD), non-normally distributed variables were presented as median (interquartile range), and categorical data were shown as counts (percentages). We adopted Student's t-test or the Mann–Whitney U test to evaluate the differences between continuous variables in the two groups, and the chi-squared test was applied to the comparison of categorical variables.
Prior to the analysis of regression on model risk factors, we selected boxplots to demonstrate the correlation between normal numerical variables (CRP, PCT, NLR, MHR) and severity of sepsis. Univariate and multivariate analyses were conducted by Cox regression model to assess the predicted potential of the abovementioned markers on 28-day mortality in sepsis. All the variables in the Cox regression models are shown as hazard ratio (HR) within 95% confidence intervals (CIs). Univariate analysis was performed preferentially, and variables with significance determined as P < 0.01 should subsequently be incorporated into the multivariate model, which is adjusted for the factors of age, sex, BMI, SBP, APACHE II, and SOFA scores. In a multiple Cox regression model, we compare the predictive value of PCT, CRP, NLR, and MHR.
Before further assessing the accuracy of the parameters in predicting the prognostic value of sepsis with the measure of AUC in the ROC analysis (25) and classification of mortality risk categories in the following step (26, 27), we adopted logistic regression to calculate and output a proportion of combination between NLR and MHR, which constructed a new model defined as NLR_MHR. The predictive value of each model of CRP, PCT, NLR, MHR, and NLR_MHR was evaluated by ROC analysis. Additionally, the sensitivity and specificity of the optimal cutoff value, positive predictive value (PPV) and negative predictive value (NPV) as well as Youden index were calculated to evaluate the accuracy of the predictors. The effects of classified model mortality risk categories were analyzed with net reclassification improvement index (NRI), which represents the reformative ability in differentiation and reclassification as well as the integrated discrimination improvement index (IDI) that was appropriate for the new model in prediction (27). The values of NRI and IDI were calculated by R Statistical Software (version 4.0.3, Vienna, Austria). P value <0.05 was considered statistically significant.
Results
Baseline Characteristics and Clinical Outcomes
A total of 274 eligible patients admitted to the EICU in the period of study were enrolled with reference to the detailed flow diagram shown in Figure 1 and should be classified into survivor (n = 195) and non-survivor groups (n = 79), according to the eventual state. The difference in demographic data of groups and comparative results are represented in Table 1. Of all septic patients, 195 (71.2%) patients survived more than 28 days. In comparison to those in the survivor group, patients in the non-survivor group, respectively, presented older ages (59.47 ± 7.44 vs. 56.96 ± 11.67; P < 0.05), higher body temperatures (38.58 ± 0.89 vs. 38.17 ± 0.88; P < 0.05), and lower levels of SBP (107.22 ± 15.97 vs. 112.35 ± 14.90; P < 0.05). Meanwhile, patients of the non-survivor group were more likely to be complicated with hypertension and coronary heart disease than those of the survivor group. With regard to the laboratory data, the levels of neutrophils (6.69 ± 1.42 vs. 6.12 ± 1.26) and monocytes (0.67 ± 0.66 vs. 0.41 ± 0.07) in the non-survivor group were significantly higher, whereas the levels of lymphocyte (1.12 ± 0.39 vs. 1.57 ± 0.65) and HDL (46.53 ± 2.4 vs. 52.11 ± 2.16) were markedly lower than those of the survivor group. For bioindicators, the levels of PCT (10.39 ± 4.21 vs. 7.71 ± 3.63; P = 0.001), NLR (6.28 ± 1.37 vs. 4.28 ± 1.18; P = 0.001), and MHR (14.29 ± 2.52 vs. 9.76 ± 2.85; P = 0.001) in the non-survivor group were markedly elevated than those in the survivor group. Otherwise, there was no significant difference of CRP level between the survivor and non-survivor groups. Apparently, patients in the non-survivor group presented poorer prognoses with the outcomes of APACHE II scores (27.11 ± 3.86 vs. 17.05 ± 4.13; P = 0.001) and SOFA scores (12 (10–14) vs. 7 (6–9); P = 0.001). Nevertheless, we failed to observe significant differences in terms of BMI, heart rate, and source of infection.
Correlation Analysis of Investigated Variables and Severity Scores
Scatterplots were distributed to describe the correlation between laboratory bioindicators and severity of sepsis. In antecedent of the statistical analysis, we stratified septic patients into three groups complying with the following levels of scores: (1) APACHE II scores: <16, 16–24, >24 and (2) SOFA scores: <6, 6–10, >10 (28, 29). As shown in Figure 2, MHR presented the closest correlation with both APACHE II and SOFA scores, followed by NLR and PCT, whereas CRP showed absence of relevance to severity scores.
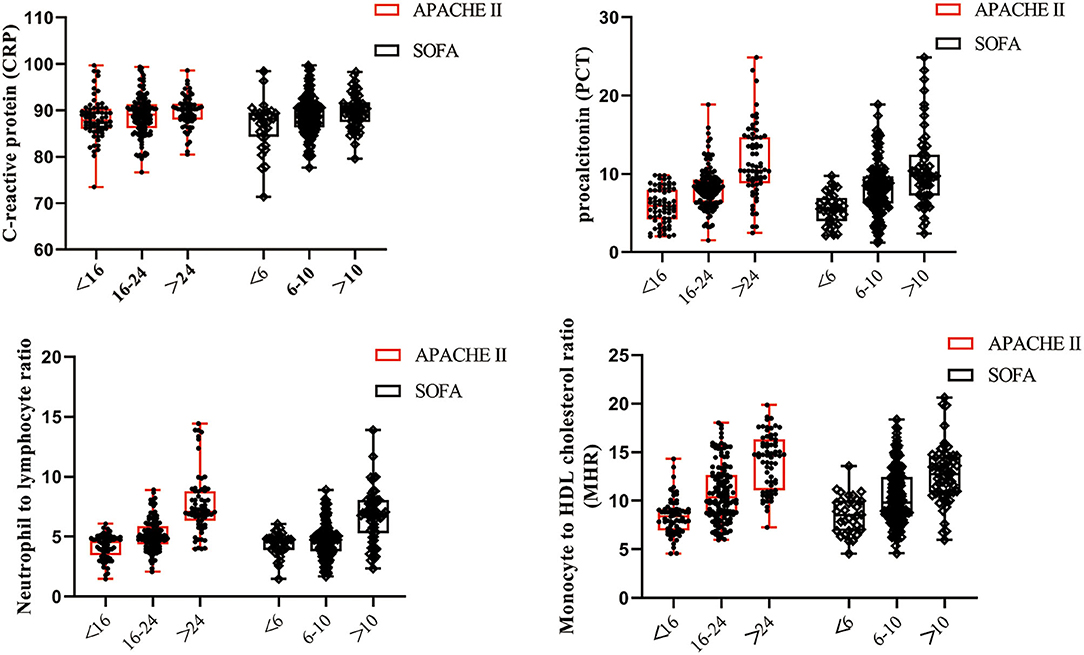
Figure 2. Levels of CRP, PCT, NLR, and MHR in patients with severity classifications. Scatterplots were conducted to demonstrate the correlation between laboratory bioindicators and severity of sepsis, which was evaluated by APACHE II and SOFA scores. MHR and NLR were more closely relevant to the severity of sepsis than CRP and PCT.
Prognosticators of 28-Day Mortality Risk in Septic Patients
To identify the risk factors for 28-day mortality in patients suffering from sepsis, we implemented Cox regression analysis. As revealed in Table 2, high levels of PCT, NLR, and MHR potentially aggravated the 28-day mortality risk of septic patients (P < 0.001). In multivariate analyses, it indicated that MHR remained statistically significant after adjusting for age, sex, BMI, SBP, and APACHE II as well as SOFA scores (HR = 1.217, 95% CI 1.112–1.331, P < 0.001). Additionally, NLR was confirmed to be independently associated with 28-day mortality of patients diagnosed with sepsis (HR = 1.404, 95% CI 1.170–1.684, P < 0.001).
The Predictive Accuracy of Parameters for 28-Day Mortality in Septic Patients
We compared the model performance–discrimination, overall fit, and reclassification to further evaluate the predictive potency of 28-day mortality in sepsis. For the model discrimination displayed in Figure 3, outcomes in the AUC diagram indicate that the MHR_NLR model had the largest AUC (0.934 [0.898–0.960]), followed by MHR (0.876 [0.831–0.913]), NLR (0.827 [0.777–0.870]), and PCT (0.705 [0.647–0.758]). The AUC value of CRP (0.569 [0.508–0.628]) was found to be smaller than the above predictors, which demonstrate the serum CRP levels might be irrelevant to the mortality risk of septic complications.
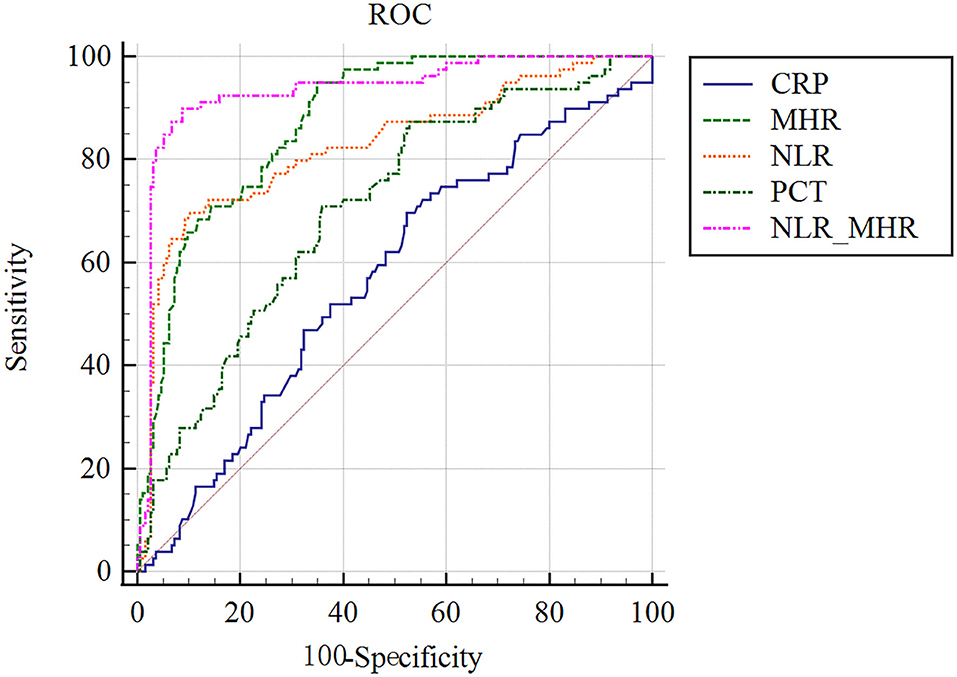
Figure 3. ROC analysis of parameters for predicting the prognosis of 28-day mortality in sepsis. MHR_NLR model displayed the largest AUC than other indicators. The AUC value of CRP was found to be the minimum, which were talentless in predicting sepsis prognostic risk.
Next, the model of overall reclassification improvement was assessed by NRI and IDI, which are more sensitive tests for improving model discrimination than ROC curves. When compared with CRP, it is noteworthy that PCT failed to indicate the significantly higher NRI and IDI. NLR shows a better NRI compared with PCT. Additionally, MHR with high IDI and NRI could better regrade patients to a more proper mortality risk classification than CRP, PCT, and NLR. Notably, we identified that NLR_MRH could better reclassify patients as indicated by significantly higher NRI and IDI in comparison to the single of NLR or MHR (Table 3).
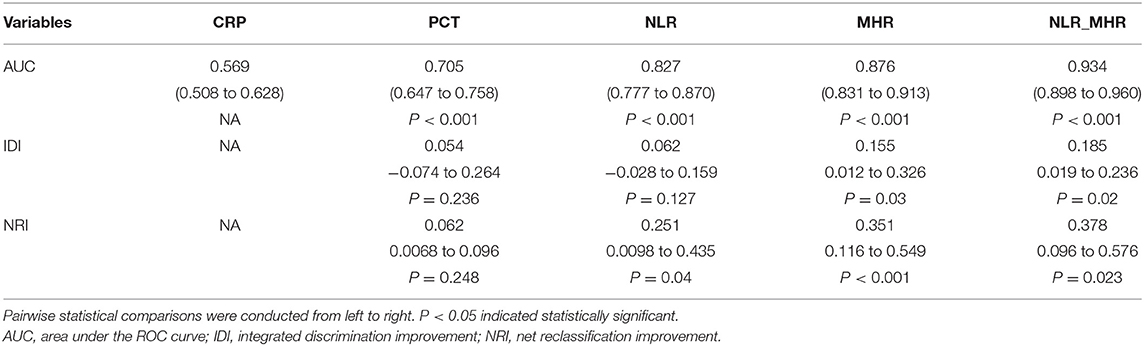
Table 3. Evaluating the efficiency of parameters in improving to predict 28-day death risk of sepsis.
ROC Curve Analysis
To achieve more precise progress in predictive value for 28-day mortality of sepsis, we figured out the sensitivity, specificity, cutoff point, PPV, NPV, and Youden index in ROC analysis. As shown in Table 4, the sensitivity and specificity of MHR at the optimal cutoff value of 10.15 were 94.94% (87.5–98.6%) and 65.13% (58.0–71.8%), respectively. The optimal cutoff value of NLR was 5.51, which gave a sensitivity of 69.62% (58.2–79.5%) and a specificity of 89.74% (84.6–93.6%). Of note, MHR_NLR trended toward a high specificity of 91.28% (86.4–94.8%). Based on the cutoff values listed in Table 4, NLR_MHR obtained a best PPV (80.7, 72.5–86.9%), and MHR showed a best NPV (96.9, 92.4–98.8%). Furthermore, we found NLR_MHR presented the highest Youden index of 0.8116. Collectively, NLR_MHR was determined to be the most reliable diagnostic accuracy for predictive value of 28-day mortality in septic patients.
Discussion
Sepsis has recently been redefined as a syndrome of physiological, pathological, and biochemical abnormalities that induce an uncontrollable host reaction to inflammation that causes fatal multiple organ dysfunction (1, 12). A vicious circle of inflammation and oxidative stress ultimately inducing immunosuppression is supposed to be the essence of the pathophysiological process. The incidence of sepsis has been gradually trending toward ascension, and conservative estimates indicate that sepsis may be a leading cause of death in ICU hospitalization (30, 31). Therefore, early identification of septic risk is critical to improving the diagnosis, therapeutic intervention, and prognosis in this serious complication. Although a variety of predictive models for sepsis risk factors are established in clinical studies, the practical values of these biomarkers are still disputed. Accordingly, the current study was conducted to explore a novel death risk screening indicator defined as MHR combined with NLR in predicting 28-day mortality in sepsis, and further evaluate the predictive efficacy of the parameters.
It is demonstrated that sepsis is induced by a dysregulated host response to infection, and the innate immune system is triggered when microbial molecular patterns are identified by specific receptors expressed on immune cells (32). This interaction can activate immune cells, including neutrophils and lymphocytes, to release both proinflammatory and anti-inflammatory mediators. Neutrophils as the first line of defense play an indispensable role in elimination of pathogens by phagocytosis and T cell activation, and lymphocytes as an indicator of immunosuppression play a role in mediating apoptosis (33, 34). Therefore, NLR appears to represent a balanced state between innate and adaptive immunity. Recently, several reports have documented the potential utility of NLR as a diagnostic parameter that is closely related to miscellaneous diseases, including inflammation, ischemic cerebrovascular disease, cancer, and trauma (20, 35–40). In our observational study, we found that NLR in patients who died were remarkably elevated compared with those with mild cases (P < 0.01) (41), which is in accordance with the previous study showing that an inflammatory reactive state typically led to neutrophilia and relative lymphocytopenia (42). The AUC value of NLR corresponded to 0.827 with 69.62% sensitivity and 89.74% specificity at the optimal cutoff value of 5.51. Theoretically, increased neutrophils reflect a response to microbial infection and migration to the infected region, whereas a reduced number in apoptotic lymphopenia contributes to the development of immunosuppression (43). A high level of neutrophils in the circulation in patients with sepsis indicate overactivation of the innate immune response (44). Conversely, exhaustion of lymphopenia may induce inefficiency in initiating an adaptive immune response and activating T cells. Hence, the results in our study verify the inference that NLR could be a prognostic sign of impending sepsis and predicting mortality risk in septicemia.
Oxidative stress is considered to be another crucial element involved in progression of sepsis. Increasing evidence suggests that both the incapacity of cells to consume oxygen and accumulation of peroxide may severely exacerbate the pathological process of sepsis (45). Sepsis-induced multiple organ dysfunctions finally occur when it suffers from an imbalance of oxidants and antioxidants due to capillary penetrability damage, deteriorative organic property, and hyporespiratory function induced by mitochondrial malfunction (46). During the process of oxidative stress, monocytes, the main source of proinflammatory and oxidative mediators, reveal the responsive capacity of the innate immune system (46). Although HDL cholesterol (HDL-C) inhibits hyperoxidation of low-density lipoprotein cholesterol (LDL-C) and can exert a protective effect on the endothelium. In the early stage of sepsis, circulating monocytes migrate to vascular endothelium and mature into macrophages, which then oxidize liposomes and differentiate into foam cells to release inflammatory cytokines and activate T lyphocytes and more monocytes. In contrast, HDL-C inhibits the activation and transformation of monocytes, thereby resulting in a suppression of inflammatory response (47, 48). Based on this view, it is rational to unite these two parameters into a single index (MHR), which is cost-effective and consists of easily available laboratory parameters reflecting anti-inflammatory and antioxidant effects. Recent studies propose a high level of MHR as an unfavorable prognostic marker, indicating systemic inflammatory and oxidative diseases, including Behçet's disease, psoriasis, and spondylarthritis (49–51). The present study was consistent with the abovementioned clinical observations in that the MHR level was noticed to be significantly higher in the non-survivors group with the average level than the survivor group, which demonstrated MHR was a more precise parameter than others for evaluating systematic inflammation in sepsis. Kanbay and colleagues analyzed the level of MHR in critical patients on admission, and they suggest that MHR might be an early predictor in cardiovascular emergency in patients with chronic kidney disease (24). Similar to previous reports, the scatter diagram in our results found that a high level of MHR was significantly correlated with APACHE II and SOFA scores. The fatality in patients with a high level of MHR was more serious than in patients with a low level of MHR, which indicates that MHR was independently related to prognosis risk for a septic event. Moreover, the current data supports previous findings that MHR is better than NLR in determining prognosis due to its larger AUC. When the cutoff value of MHR was set at 10.15, a higher sensitivity was obtained, and correspondingly, 52.4% of dead patients were effectively classified in the non-survivor group, and 3.1% of the deceased could be assigned to the surviving patients group. Strikingly, the addition of MHR could efficaciously improve the early diagnosis capacity according to the high IDI and NRI. These findings illustrate that MHR may be advantageous in diagnosing sepsis and predicting prognostic risk of 28-day mortality.
Other markers, including CRP and PCT, were also analyzed in our study, and their predictive abilities appeared negative, and they failed to forecast progressive bacterial infection in terms of sepsis in early diagnostic settings. Cox analysis indicated that PCT was incapable of assessing septic mortality after adjusting partial factors (HR = 1.024, 95% CI 0.954–1.098, P = 0.517). Clinically, due to the trend of PCT reaching a plateau slowly at 8–24 h, the evaluated capacity of PCT in predicting septic prognosis was obviously disadvantaged with an AUC of 0.705, smaller than those of NLR and MHR. Nevertheless, CRP released in the acute phase of inflammation indicated no difference between the survivor and non-survivor groups and were irrelevant to the severity of sepsis. The potential cause might be that the CRP level peaked within only 48 h and failed to reflect the terminal state of sepsis (52).
Because MHR obtained higher sensitivity but lower specificity than NLR, we conducted model parameters of MHR together with NLR in predictive risk of sepsis, and further evaluated the efficiency in predicting 28-day mortality. In the predictive model of MHR_NLR, the AUC maximum value was 0.934 with a better sensitivity and specificity than the single variable. Moreover, we examined such parameter in terms of reclassifying improvement and discrimination by use of IDI and NRI, which showed significant improvement in the mean difference of predicted probabilities. Taken together, MHR combined with NLR as the parameter is not only appropriate for the early diagnosis of sepsis, but also for the prediction of its severity and prognosis.
There are many clinical implements in the original study. We conducted multiple indicators to evaluate their predictive efficacy to ensure the reliability and accuracy. It first illuminated the inchoate predictive value of MHR combined with NLR for prognosis in sepsis, which provided a more precise guideline for administration and management of septic patients as well as clinical follow-up during the late stage of development. Continuous monitoring of these laboratory variables contributes to enhancing septic prognosis and treatment. Nevertheless, several limitations still remain in our current study. First, this single retrospective study needs to be further proven by more prospective cohort studies or multicenter randomized clinical trials. Futhermore, we need to assess the possible impact of patient's characteristics, including dietary and smoking history, which can affect performance evaluation. Second, a larger sample size appears to be essential to reduce proportional error. Finally, we should collect more information about mechanical ventilation and hemodynamic-associated indicators to better reflect the eventual development of sepsis.
Conclusions
In summary, the current study suggests that MHR together with NLR are closely related to the severity of sepsis and might be independent predictors of 28-day mortality of septic patients. Notably, MHR combined with NLR can significantly improve the predictive efficiency of 28-day mortality in sepsis.
Data Availability Statement
The original contributions presented in the study are included in the article/Supplementary Material, further inquiries can be directed to the corresponding authors.
Ethics Statement
The studies involving human participants were reviewed and approved by Medical Ethic Committee of Second Hospital of Hebei Medical University, Shijiazhuang, China. The patients/participants provided their written informed consent to participate in this study.
Author Contributions
J-yL performed the major design and drafted the manuscript. R-qY and S-qL conducted the statistics. Y-fZ contributed to the data collection. Y-pT and Y-mY conceptualized, supervised, and revised. All authors read and approved the final manuscript.
Funding
This work was supported by grants from the National Natural Science Foundation of China (81730057, 81873946) and the Provincial Key Project of Medical Science Research of Hebei (20210013).
Conflict of Interest
The authors declare that the research was conducted in the absence of any commercial or financial relationships that could be construed as a potential conflict of interest.
Publisher's Note
All claims expressed in this article are solely those of the authors and do not necessarily represent those of their affiliated organizations, or those of the publisher, the editors and the reviewers. Any product that may be evaluated in this article, or claim that may be made by its manufacturer, is not guaranteed or endorsed by the publisher.
Acknowledgments
We would like to sincerely acknowledge all the patients for their understanding and support to this study.
Supplementary Material
The Supplementary Material for this article can be found online at: https://www.frontiersin.org/articles/10.3389/fmed.2021.741015/full#supplementary-material
References
1. Czura CJ. Merinoff symposium 2010: sepsis - speaking with one voice. Mol Med. (2011) 17:2–3. doi: 10.2119/molmed.2010.00001.commentary
2. Wu L, Feng Q, Ai ML, Deng SY, Liu ZY, Huang L, et al. The dynamic change of serum S100B levels from day 1 to day 3 is more associated with sepsis-associated encephalopathy. Sci Rep. (2020) 10:7718. doi: 10.1038/s41598-020-64200-3
3. Angus DC, van der Poll T. Severe sepsis and septic shock. N Engl J Med. (2013) 369:840–51. doi: 10.1056/NEJMra1208623
4. Fleischmann C, Scherag A, Adhikari NK, Hartog CS, Tsaganos T, Schlattmann P, et al. Assessment of global incidence and mortality of hospital-treated sepsis: current estimates and limitations. Am J Respir Crit Care Med. (2016) 193:259–72. doi: 10.1164/rccm.201504-0781OC
5. Tiru B, DiNino EK, Orenstein A, Mailloux PT, Pesaturo A, Gupta A, et al. The economic and humanistic burden of severe sepsis. Pharmacoeconomics. (2015) 33:925–37. doi: 10.1007/s40273-015-0282-y
6. Reinhart K, Daniels R, Kissoon N, Machado FR, Schachter RD, Finfer S. Recognizing sepsis as a global health priority: a WHO resolution. N Engl J Med. (2017) 377:414–7. doi: 10.1056/NEJMp1707170
7. Angus DC, Linde-Zwirble WT, Lidicker J, Clermont G, Carcillo J, Pinsky MR. Epidemiology of severe sepsis in the United States: analysis of incidence, outcome, and associated costs of care. Crit Care Med. (2001) 29:1303–10. doi: 10.1097/00003246-200107000-00002
8. Mayr FB, Yende S, Angus DC. Epidemiology of severe sepsis. Virulence. (2014) 5:4–11. doi: 10.4161/viru.27372
9. Cohen J, Vincent JL, Adhikari NK, Machado FR, Angus DC, Calandra T, et al. Sepsis: a roadmap for future research. Lancet Infect Dis. (2015) 15:581–614. doi: 10.1016/S1473-3099(15)70112-X
10. Jain S. Sepsis: an update on current practices in diagnosis and management. Am J Med Sci. (2018) 356:277–86. doi: 10.1016/j.amjms.2018.06.012
11. Cheng YW, Cao X, Qin LJ. Mesenchymal stem cell-derived extracellular vesicles: a novel cell-free therapy for sepsis. Front Immunol. (2020) 11:647. doi: 10.3389/fimmu.2020.00647
12. Singer M, Deutschman CS, Seymour CW, Shankar-Hari M, Annane D, Bauer M, et al. The third international consensus definitions for sepsis and septic shock (Sepsis-3). JAMA. (2016) 315:801–10. doi: 10.1001/jama.2016.0287
13. Dellinger RP, Carlet JM, Masur H, Gerlach H, Calandra T, Cohen J, et al. Surviving Sepsis Campaign guidelines for management of severe sepsis and septic shock. Crit Care Med. (2004) 32:858–73. doi: 10.1097/01.CCM.0000117317.18092.E4
14. Rhodes A, Evans LE, Alhazzani W, Levy MM, Antonelli M, Ferrer R, et al. Surviving sepsis campaign: international guidelines for management of sepsis and septic shock: 2016. Intensive Care Med. (2017) 43:304–77. doi: 10.1007/s00134-017-4683-6
15. Zhang JX, Wang XL, Vikash V, Ye Q, Wu DD, Liu Y, et al. ROS and ROS-mediated cellular signaling. Oxid Med Cell Longev. (2016) 2016:4350965. doi: 10.1155/2016/4350965
16. Meo SD, Reed TT, Venditti P, Victor VM. Role of ROS and RNS sources in physiological and pathological conditions. Oxid Med Cell Longev. (2016) 2016:1245049. doi: 10.1155/2016/1245049
17. Bajčetić M, Spasić S, Spasojević I. Redox therapy in neonatal sepsis: reasons, targets, strategy, and agents. Shock. (2014) 42:179–84. doi: 10.1097/SHK.0000000000000198
18. Jekarl DW, Kim JY, Lee S, Kim M, Kim Y, Han K, et al. Diagnosis and evaluation of severity of sepsis via the use of biomarkers and profiles of 13 cytokines: a multiplex analysis. Clin Chem Lab Med. (2015) 53:575–81. doi: 10.1515/cclm-2014-0607
19. Sandquist M, Wong HR. Biomarkers of sepsis and their potential value in diagnosis, prognosis and treatment. Expert Rev Clin Immunol. (2014) 10:1349–56. doi: 10.1586/1744666X.2014.949675
20. Ni J, Wang HY, Li Y, Shu YM, Liu YH. Neutrophil to lymphocyte ratio (NLR) as a prognostic marker for in-hospital mortality of patients with sepsis: a secondary analysis based on a single-center, retrospective, cohort study. Medicine. (2019) 98:e18029. doi: 10.1097/MD.0000000000018029
21. Park KS, Lee SH, Yun SJ, Ryu S, Kim K. Neutrophil-to-lymphocyte ratio as a feasible prognostic marker for pyogenic liver abscess in the emergency department. Eur J Trauma Emerg Surg. (2019) 45:343–51. doi: 10.1007/s00068-018-0925-8
22. Canpolat U, Aytemir K, Yorgun H, Sahiner L, Kaya EB, Çay S, et al. The role of preprocedural monocyte-to-high-density lipoprotein ratio in prediction of atrial fibrillation recurrence after cryoballoon-based catheter ablation. EP Europace. (2015) 17:1807–15. doi: 10.1093/europace/euu291
23. Canpolat U, Çetin EH, Cetin S, Aydin S, Akboga MK, Yayla C, et al. Association of monocyte-to-HDL cholesterol ratio with slow coronary flow is linked to systemic inflammation. Clin Appl Thromb Hemost. (2016) 22:476–82. doi: 10.1177/1076029615594002
24. Kanbay M, Solak Y, Unal HU, Kurt YG, Gok M, Cetinkaya H, et al. Monocyte count/HDL cholesterol ratio and cardiovascular events in patients with chronic kidney disease. Int Urol Nephrol. (2014) 46:1619–25. doi: 10.1007/s11255-014-0730-1
25. Jordan D, Steiner M, Kochs EF, Schneider G. A program for computing the prediction probability and the related receiver operating characteristic graph. Anesth Analg. (2010) 111:1416–21. doi: 10.1213/ANE.0b013e3181fb919e
26. Demler OV, Pencina MJ, Cook NR, D'Agostino RB Sr. Asymptotic distribution of ΔAUC, NRIs, and IDI based on theory of U-statistics. Stat Med. (2017) 36:3334–60. doi: 10.1002/sim.7333
27. Yu H, Nie L, Liu A, Wu K, Hsein YC, Yen DW, et al. Combining procalcitonin with the qSOFA and sepsis mortality prediction. Medicine. (2019) 98:e15981. doi: 10.1097/MD.0000000000015981
28. Yin YP, Tao J, Duan ZG, Chen FG, Dong LQ. APACHE II scoring used in evaluating the prognosis of patients with emergency critical value of research. Contemp Med. (2019) 25:85–7.
29. Vincent JL, de Mendonça A, Cantraine F, Moreno R, Takala J, Suter PM, et al. Use of the SOFA score to assess the incidence of organ dysfunction/failure in intensive care units: results of a multicenter, prospective study. Working group on “sepsis-related problems” of the European Society of Intensive Care Medicine. Crit Care Med. (1998) 26:1793–800. doi: 10.1097/00003246-199811000-00016
30. Vincent JL, Marshall JC, Namendys-Silva SA, François B, Martin-Loeches I, Lipman J, et al. ICON investigators. assessment of the worldwide burden of critical illness: the Intensive Care Over Nations (ICON) audit. Lancet Respir Med. (2014) 2:380–6. doi: 10.1016/S2213-2600(14)70061-X
31. Krishnamurthi RV, Moran AE, Forouzanfar MH, Bennett DA, Mensah GA, Lawes CM, et al. The global burden of hemorrhagic stroke: a summary of findings from the GBD 2010 study. Glob Heart. (2014) 9:101–6. doi: 10.1016/j.gheart.2014.01.003
32. Boyd JH, Russell JA, Fjell CD. The meta-genome of sepsis: host genetics, pathogens and the acute immune response. J Innate Immun. (2014) 6:272–83. doi: 10.1159/000358835
33. Hwang SY, Shin TG, Jo IJ, Jeon K, Suh GY, Lee TR, et al. Neutrophil-to-lymphocyte ratio as a prognostic marker in critically-ill septic patients. Am J Emerg Med. (2017) 35:234–9. doi: 10.1016/j.ajem.2016.10.055
34. Laukemann S, Kasper N, Kulkarni P, Steiner D, Rast AC, Kutz A, et al. Can we reduce negative blood cultures with clinical scores and blood markers? Results from an observational cohort study. Medicine. (2015) 94:e2264. doi: 10.1097/MD.0000000000002264
35. Tamelyte E, Vaičekauskiene G, Dagys A, Lapinskas T, Jankauskaite L. Early blood biomarkers to improve sepsis/bacteremia diagnostics in pediatric emergency settings. Medicina. (2019) 55:99. doi: 10.3390/medicina55040099
36. Ozdemir SA, Ozer EA, Ilhan O, Sutcuoglu S. Can neutrophil to lymphocyte ratio predict late-onset sepsis in preterm infants? J Clin Lab Anal. (2018) 32:e22338. doi: 10.1002/jcla.22338
37. Erre GL, Paliogiannis P, Castagna F, Mangoni AA, Carru C, Passiu G, et al. Meta-analysis of neutrophil-to-lymphocyte and platelet-to-lymphocyte ratio in rheumatoid arthritis. Eur J Clin Invest. (2019) 49:e13037. doi: 10.1111/eci.13037
38. Zhang YF, Jiang L, Yang P, Zhang YZ. Comparison of lymphocyte count, neutrophil to lymphocyte ratio and platelet to lymphocyte ratio in predicting the severity and the clinical outcomes of acute cerebral infarction patients. Clin Lab. (2019) 65. doi: 10.7754/Clin.Lab.2019.190102
39. Sarraf KM, Belcher E, Raevsky E, Nicholson AG, Goldstraw P, Lim E. Neutrophil/lymphocyte ratio and its association with survival after complete resection in non-small cell lung cancer. J Thorac Cardiovasc Surg. (2009) 137:425–8. doi: 10.1016/j.jtcvs.2008.05.046
40. Park JM. Neutrophil-to-lymphocyte ratio in trauma patients. J Trauma Acute Care Surg. (2017) 82:225–6. doi: 10.1097/TA.0000000000001266
41. Liu SQ, Wang XK, She F, Zhang W, Liu HS, Zhao XD. Effects of neutrophil-to-lymphocyte ratio combined with interleukin-6 in predicting 28-day mortality in patients with sepsis. Front Immunol. (2021) 12:639735. doi: 10.3389/fimmu.2021.639735
42. de Jager CP, van Wijk PT, Mathoera RB, de Jongh-Leuvenink J, van der Poll T, Wever PC. Lymphocytopenia and neutrophil-lymphocyte count ratio predict bacteremia better than conventional infection markers in an emergency care unit. Crit Care. (2010) 14:R192. doi: 10.1186/cc9309
43. Joshi VD, Kalvakolanu DV, Cross AS. Simultaneous activation of apoptosis and inflammation in pathogenesis of septic shock: a hypothesis. FEBS Lett. (2003) 555:180–4. doi: 10.1016/S0014-5793(03)01271-7
44. Tulzo YL, Pangault C, Gacouin A, Guilloux V, Tribut O, Amiot L, et al. Early circulating lymphocyte apoptosis in human septic shock is associated with poor outcome. Shock. (2002) 18:487–94. doi: 10.1097/00024382-200212000-00001
45. Karapetsa M, Pitsika M, Goutzourelas N, Stagos D, Becker TA, Zakynthinos E. Oxidative status in ICU patients with septic shock. Food Chem Toxicol. (2013) 61:106–11. doi: 10.1016/j.fct.2013.03.026
46. Bar-Or D, Bar-Or R, Rael LT, Brody EN. Oxidative stress in severe acute illness. Redox Biol. (2015) 4:340–5. doi: 10.1016/j.redox.2015.01.006
47. Ancuta P, Wang J, Gabuzda D. CD16+ monocytes produce IL-6, CCL2, and matrix metalloproteinase-9 upon interaction with CX3CL1-expressing endothelial cells. J Leukoc Biol. (2006) 80:1156–64. doi: 10.1189/jlb.0206125
48. Uca FM. A potential marker of bare metal stent restenosis: monocyte count-to-HDL cholesterol ratio. BMC Cardiovasc Disord. (2016) 16:186. doi: 10.1186/s12872-016-0367-3
49. Acikgoz N, Kurtoglu E, Yagmur J, Kapicioglu Y, Cansel M, Ermis N. Elevated monocyte to high-density lipoprotein cholesterol ratio and endothelial dysfunction in Behçet disease. Angiology. (2018) 69:65–70. doi: 10.1177/0003319717704748
50. Karabay EA, Demir D, Çerman AA. Evaluation of monocyte to high-density lipoprotein ratio, lymphocytes, monocytes, and platelets in psoriasis. An Bras Dermatol. (2020) 95:40–5. doi: 10.1016/j.abd.2019.05.002
51. Huang YK, Deng WM, Zheng SL, Feng F, Huang ZX, Huang QD, et al. Relationship between monocytes to lymphocytes ratio and axial spondyloarthritis. Int Immunopharmacol. (2018) 57:43–6. doi: 10.1016/j.intimp.2018.02.008
Keywords: neutrophil/lymphocyte ratio, monocyte/high-density lipoprotein cholesterol ratio, predictive value, mortality, sepsis
Citation: Li J-y, Yao R-q, Liu S-q, Zhang Y-f, Yao Y-m and Tian Y-p (2021) Efficiency of Monocyte/High-Density Lipoprotein Cholesterol Ratio Combined With Neutrophil/Lymphocyte Ratio in Predicting 28-Day Mortality in Patients With Sepsis. Front. Med. 8:741015. doi: 10.3389/fmed.2021.741015
Received: 14 July 2021; Accepted: 30 August 2021;
Published: 13 October 2021.
Edited by:
Penglin Ma, Guiqian International General Hospital, ChinaCopyright © 2021 Li, Yao, Liu, Zhang, Yao and Tian. This is an open-access article distributed under the terms of the Creative Commons Attribution License (CC BY). The use, distribution or reproduction in other forums is permitted, provided the original author(s) and the copyright owner(s) are credited and that the original publication in this journal is cited, in accordance with accepted academic practice. No use, distribution or reproduction is permitted which does not comply with these terms.
*Correspondence: Ying-ping Tian, tianyingping999@163.com; Yong-ming Yao, c_ff@sina.com