- 1Facultad de Farmacia y Bioquímica, Instituto de Investigaciones en Bacteriología y Virología Molecular (IBaViM), Universidad de Buenos Aires, Buenos Aires, Argentina
- 2Consejo Nacional de Investigaciones Científicas y Técnicas (CONICET), Buenos Aires, Argentina
- 3Laboratorio de Virología, Hospital de Niños Dr. Ricardo Gutiérrez, Buenos Aires, Argentina
- 4Instituto de Investigación de la Cadena Láctea (IDICAL) INTA-CONICET, Rafaela, Argentina
- 5Laboratorio de Biología Celular y Retrovirus, Hospital de Pediatría “Prof. Juan P. Garrahan”, Buenos Aires, Argentina
- 6Instituto de Patología Vegetal – Centro de Investigaciones Agropecuarias – Instituto Nacional de Tecnología Agropecuaria (IPAVE-CIAP-INTA), Córdoba, Argentina
- 7CIBIC Laboratorio, Rosario, Argentina
- 8Grupo Virología Humana, Instituto de Biología Molecular y Celular de Rosario CONICET, Rosario, Argentina
- 9Instituto de Biotecnología/Instituto de Agrobiotecnología y Biología Molecular INTA-CONICET, Hurlingham, Argentina
- 10Instituto de Medicina Regional, Universidad Nacional del Nordeste, Resistencia, Argentina
- 11Laboratorio Central Ciudad de Neuquén, Ministerio de Salud, Neuquén, Argentina
- 12Instituto de Botánica del Nordeste, Universidad Nacional del Nordeste-CONICET, Resistencia, Argentina
- 13Instituto de Virología e Innovaciones Tecnológicas INTA-CONICET, Hurlingham, Argentina
- 14Laboratorio de Diagnóstico-UNIDAD COVID- Universidad Nacional de Hurlingham, Hurlingham, Argentina
- 15Instituto de Cálculo, Facultad de Ciencias Exactas y Naturales, Universidad de Buenos Aires, Buenos Aires, Argentina
- 16Departamento de Ciencias Básicas, Universidad Nacional de Luján, Luján, Argentina
- 17Unidad de Genómica del Instituto de Biotecnología/Instituto de Agrobiotecnología y Biología Molecular, INTA-CONICET, Hurlingham, Argentina
- 18Instituto de Virología “Dr. J. M. Vanella”, Facultad de Ciencias Médicas, Universidad Nacional de Córdoba, Córdoba, Argentina
- 19Laboratorio Mixto de Biotecnología Acuática, Facultad de Ciencias Bioquímicas y Farmacéuticas, Universidad Nacional de Rosario, Rosario, Argentina
- 20Instituto de Química Biológica de la Facultad de Ciencias Exactas y Naturales (IQUIBICEN), CONICET, Ciudad Universitaria, Buenos Aires, Argentina
- 21Laboratorio de Virología Molecular, Hospital Blas L. Dubarry, Mercedes, Argentina
- 22Centro de Tecnología en Salud Pública, Facultad de Ciencias Bioquímicas y Farmacéuticas, Universidad Nacional de Rosario, Rosario, Argentina
- 23Laboratorio del Hospital Zonal Dr. Ramón Carrillo, San Carlos de Bariloche, Argentina
- 24Comité Operativo de Emergencia COVID, Ministerio de Salud de la Ciudad Autónoma de Buenos Aires, Buenos Aires, Argentina
- 25Laboratorio de Salud Pública, Facultad de Ciencias Exactas, Universidad Nacional de La Plata, La Plata, Argentina
- 26Laboratorio de Virología, HIEAyC “San Juan de Dios”, La Plata, Argentina
- 27Laboratorio de Virología del Hospital JJ Urquiza, Concepción del Uruguay, Argentina
- 28Laboratorio Central de Salud Pública del Chaco, Resistencia, Argentina
- 29Secretaria de Prevención y Promoción, Ministerio de Salud de la Provincia de Córdoba, Córdoba, Argentina
- 30Laboratorio de la Dirección de Epidemiología, Santa Rosa, Argentina
- 31Laboratorio de Biología Molecular del Hospital Centenario, Gualeguaychú, Argentina
- 32Unidad de Emergencia COVID-19, Plataforma de Servicios Biotecnológicos, Universidad Nacional de Quilmes, Bernal, Argentina
- 33Laboratorio Central de la Provincia de Córdoba, Ministerio de Salud la Provincia de Córdoba, Córdoba, Argentina
- 34Laboratorio de Virología, Facultad de Veterinaria, Universidad Nacional del Centro de la Provincia de Buenos Aires, Buenos Aires, Argentina
- 35Instituto Nacional de Epidemiología “Dr. Jara”, Mar del Plata, Argentina
- 36Centro de Investigaciones Básicas y Aplicadas, Universidad Nacional del Noroeste de la Provincia de Buenos Aires, Junín, Argentina
- 37Dirección de Epidemiología y Red de Laboratorios del Ministerio de Salud de la Provincia de Mendoza, Mendoza, Argentina
- 38Servicio de Inmunología, Hospital Dr. Julio C Perrando, Resistencia, Argentina
- 39Laboratorio de Biología Molecular Bolívar, LABBO, Bolívar, Argentina
- 40Programa Laboratorio de Salud Pública “Dr Dalmiro Pérez Laborda”, Ministerio de Salud de la Provincia de San Luis, San Luis, Argentina
- 41Laboratorio de Salud Pública, Godoy Cruz, Argentina
- 42Laboratorio del Hospital Interzonal General de Agudos “Evita”, Lanús, Argentina
- 43Laboratorio Pediátrico Avelino Castelán, Resistencia, Argentina
- 44Laboratorio de Biología Molecular Hospital Pedro de Elizalde, Buenos Aires, Argentina
- 45Laboratorio Central, Santa Fe, Argentina
- 46Servicio de Microbiología, Hospital 4 de Junio, Presidencia Roque Saenz Peña, Argentina
- 47Laboratorio de Biología Molecular, Hospital Cosme Argerich, Buenos Aires, Argentina
- 48Laboratorio de Hospital El Cruce Dr. Néstor C. Kirchner, Florencio Varela, Argentina
- 49Instituto de Investigaciones Biomédicas en Retrovirus y Sida, CONICET-UBA, Buenos Aires, Argentina
- 50Laboratorio de Biología Molecular, Hospital Dr. Héctor Cura, Olavarría, Argentina
- 51Departamento de Biología y Genética Molecular; IACA Laboratorios, Bahía Blanca, Argentina
- 52Laboratorio de Biología Molecular del Hospital General de Agudos Dr. Carlos G. Durand, Buenos Aires, Argentina
- 53Ministerio de Ciencia, Tecnología e Innovación, Buenos Aires, Argentina
- 54Comisión de Investigaciones Científicas de la Provincia de Buenos Aires, La Plata, Argentina
SARS-CoV-2 variants with concerning characteristics have emerged since the end of 2020. Surveillance of SARS-CoV-2 variants was performed on a total of 4,851 samples from the capital city and 10 provinces of Argentina, during 51 epidemiological weeks (EWs) that covered the end of the first wave and the ongoing second wave of the COVID-19 pandemic in the country (EW 44/2020 to EW 41/2021). The surveillance strategy was mainly based on Sanger sequencing of a Spike coding region that allows the identification of signature mutations associated with variants. In addition, whole-genome sequences were obtained from 637 samples. The main variants found were Gamma and Lambda, and to a lesser extent, Alpha, Zeta, and Epsilon, and more recently, Delta. Whereas, Gamma dominated in different regions of the country, both Gamma and Lambda prevailed in the most populated area, the metropolitan region of Buenos Aires. The lineages that circulated on the first wave were replaced by emergent variants in a term of a few weeks. At the end of the ongoing second wave, Delta began to be detected, replacing Gamma and Lambda. This scenario is consistent with the Latin American variant landscape, so far characterized by a concurrent increase in Delta circulation and a stabilization in the number of cases. The cost-effective surveillance protocol presented here allowed for a rapid response in a resource-limited setting, added information on the expansion of Lambda in South America, and contributed to the implementation of public health measures to control the disease spread in Argentina.
Introduction
The emergence of SARS-CoV-2 variants with concerning characteristics to public health has attracted the attention of the scientific community and governments both regionally and globally since the end of 2020. The most relevant variants described so far include: Alpha (lineage B.1.1.7), first detected in the United Kingdom; Beta (lineage B.1.351), initially detected in South Africa; Gamma (lineage P.1), initially detected in Manaus, Brazil, and Japan; Delta (lineage B.1.627.2), initially detected in India; Lambda (lineage C.37), initially detected in Peru; Mu (lineage B.1.621), first detected in Colombia; Epsilon (lineages B.1.427 and B.1.429), initially detected in California, United States; and Zeta (lineage P.2), first detected in Rio de Janeiro, Brazil (1). Four of these variants (Alpha to Delta) have been defined as variants of concern (VOCs) given their increased transmissibility and other characteristics, while Lambda and Mu have been defined as variants of interest (VOIs). The VOCs have also been associated with an increased risk of hospitalization (2, 3) and, in the case of Beta, Gamma, and Delta, with a moderate to a substantial reduction in neutralizing activity of monoclonal antibodies, convalescent, and vaccine sera (4–6). Gamma and Lambda are particularly relevant for Argentina due to their major presence in the South American region during the time of this study.
Importantly, some of these variants share mutations in the Spike protein—several of them in the receptor-binding domain region—that potentially affect transmissibility, pathogenesis, and/or response to vaccination and immune-based therapies (7, 8).
PAIS is the interinstitutional federal consortium of SARS-CoV-2 genomics in Argentina. It was created by the Ministry of Science and Technology to monitor SARS-CoV-2 diversity and evolution in the country, including surveillance of SARS-CoV-2 variants of public health interest (http://pais.qb.fcen.uba.ar/).
The objective of this work was to implement a SARS-CoV-2 molecular surveillance strategy, in a context of limited resources, which allowed an assessment of the dynamic situation of circulation of viral variants, and at the same time, to perform genomic and evolutionary analyzes to study their origin and dispersion in our country.
Materials and Methods
Samples
Between October 26th, 2020 and October 12th, 2021 (epidemiological week (EW) 44/2020 to EW41/2021), molecular surveillance was performed on a total of 4,851 samples from the capital city and 10 provinces of the country, including the four most populated districts (Figure 1, Supplementary Table 1). This period covers the end of the first wave and the second and largest wave of the COVID-19 pandemic in Argentina (Figure 2A). During this period, the frontiers were mostly open for Argentinean residents, but the foreigners had severe restrictions to enter the country as tourists.
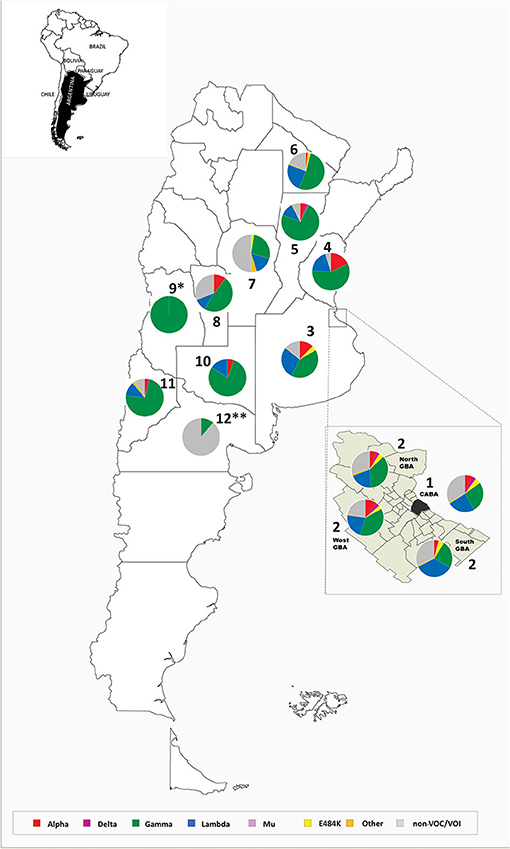
Figure 1. Location of cases analyzed in this work and pie charts representing the frequency of each variant detected in every region between EW44/2020 and EW41/2021. 1. Buenos Aires city, 2. Great Buenos Aires (North, West, South), 3. Province of Buenos Aires, 4. Province of Entre Ríos, 5. Province of Santa Fe, 6. Province of Chaco, 7. Province of Córdoba, 8. Province of San Luis, 9. Province of Mendoza, 10. Province of La Pampa, 11. Province of Neuquén, and 12. Province of Río Negro. *cases associated with an outbreak (n = 15) (Supplementary Table 1). **cases (n = 9) mainly associated with the first part of the period analyzed (Supplementary Table 1).
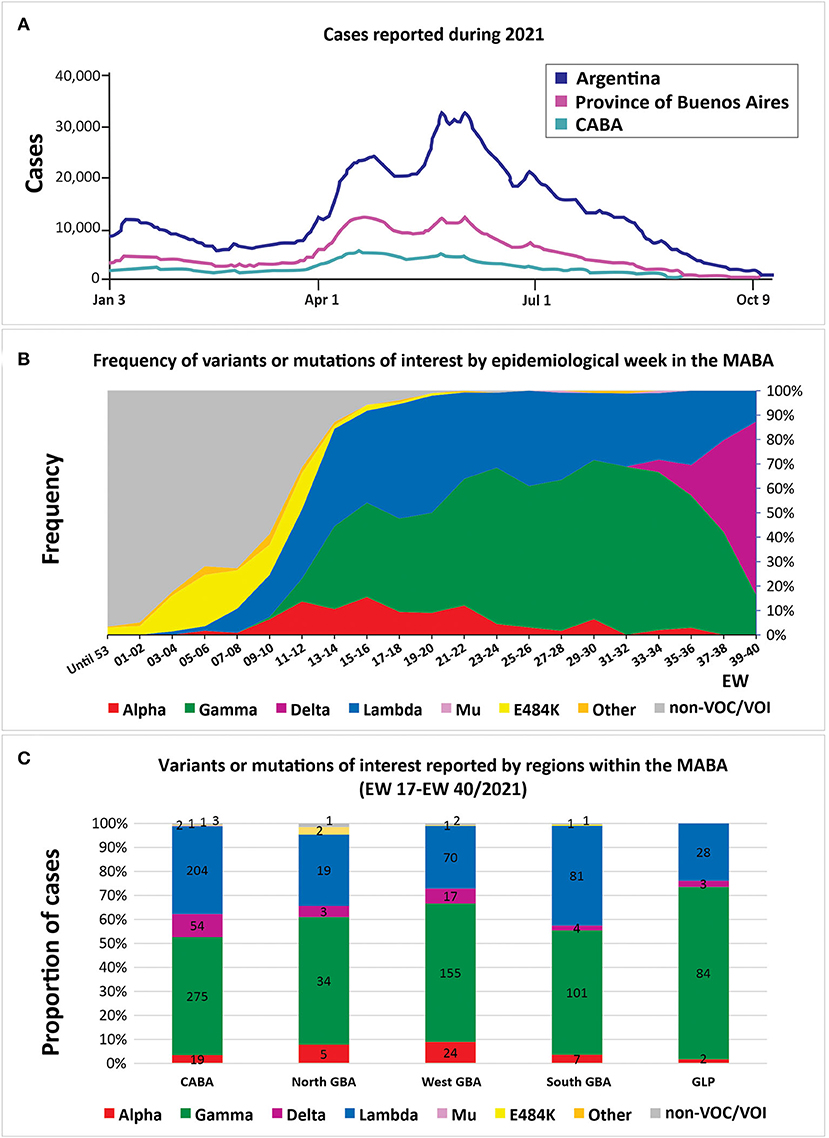
Figure 2. (A) Confirmed cases in Argentina, the city of Buenos Aires (CABA) and the province of Buenos Aires in the year 2021. (B) Frequency of SARS-CoV-2 variants and sequences with or without mutations of interest by epidemiological week (n = 2,625). Only cases from the metropolitan area of Buenos Aires (MABA) that did not present a history of travel or close contact with travelers are included; in cases with registered epidemiological links, only one case was considered representative. (C) The cumulative number of cases between EW 17/2021 and EW 40/2021 of SARS-CoV-2 variants and sequences with or without mutations of interest within the different regions of the MABA: CABA, Great Buenos Aires (GBA), and Great La Plata (GLP). Only cases that did not present a history of travel or close contact with travelers are included; in cases sharing an epidemiological link, only one was included. (A,B) are on the same time scale.
For this work, samples analyzed included a randomly selected 2.5–50% fraction of the total positive cases weekly detected in different healthcare centers. The proportion of samples analyzed in each week was associated with the absolute number of samples that could be processed by the methodology implemented according to the number of cases reported at the beginning, at the peak, and toward the end of the second wave in the country. Regular sampling from four sentinel laboratories located in the metropolitan area of Buenos Aires (MABA) was performed along with sporadic sampling from other locations. Most of the samples corresponded to randomly selected cases with no epidemiological link among them or with international travel (n = 4,632), while a minor share corresponded to outbreak studies, special cases, or confirmatory studies of specific real time-PCR methodologies for the detection of variants (n = 219) (Supplementary Table 1).
Sequencing
The surveillance strategy was based on Sanger sequencing of a 970 nt region of Spike encoding amino acids 428–750 (Supplementary Figure 1) (9). This region allows the identification of signature mutations associated with variants Alpha, Beta, Gamma, Delta, Lambda, and Mu. In addition, complete SARS-CoV-2 genome sequences were obtained from 637 samples using the Quick protocol (10) with Oxford Nanopore or Illumina platforms. Both strategies combined allowed to perform the analysis of 4,851 sequences (Supplementary Table 1).
Statistical Analysis
The frequencies of detection of VOI, VOC, or mutations of interest and the 95% CIs were estimated with the Wilson/Brown method, implemented in the Graph Pad Prism v.9.2 program (San Diego, CA, United States, www.graphpad.com).
Phylogenetic Analysis
Phylogenetic analysis was carried out for VOCs Alpha and Gamma to study their introduction and initial spread in Argentina. Datasets included the Argentine sequences from the first part of the second wave, their best five BLAST hits sequences (against the GISAID database on June 2nd, 2021), reference sequences of B.1.1.7 or P.1 lineages, and B.1.1.1 or B.1.1.28 sequences as outgroups, respectively. Alignments were built using MAFFT v7.486 (11) and maximum likelihood trees were built using IQ-TREE v.2.1 (12). The SH-like approximate likelihood ratio test (1,000 replicates) (13) and ultrafast bootstrap approximation (1,000 replicates) (14) were used as methods to evaluate the reliability of the groups and branches obtained. We gratefully acknowledge the authors from the originating laboratories responsible for obtaining the specimens and the submitting laboratories where genetic sequence data were generated and shared via the GISAID Initiative, on which part of this research is based (Supplementary Tables 4, 5).
Ethics Statement
The study was revised and approved by the Medical Ethics and Research Committees of “Ricardo Gutiérrez” Children's Hospital, Buenos Aires, Argentina (DI-2020-165-GCABA-HGNRG). Informed consent was not obtained, as patient information was anonymized and de-identified before analysis.
Results
Alpha
Alpha was identified in 307 cases. This variant was detected in the city of Buenos Aires (CABA) and in the provinces of Buenos Aires, Córdoba, Chaco, Entre Ríos, Santa Fe, San Luis, La Pampa, and Neuquén. Its frequency in the MABA region (the CABA, Great Buenos Aires (GBA), Great La Plata (GLP), and surrounding areas) reached 15.5% (95% CI = 11.2–21.0) in EW 15-16/2021 (April 11th−24th) but decreased to 0% by EW 37-38/2021 (September 12th−25th, 2021) (Supplemetary Tables 1, 2, Figure 2).
To study the introduction of Alpha in our country, the dominant variant of the second wave throughout the northern hemisphere and other locations, a phylogenetic analysis of complete genome sequences was carried out. This analysis included 127 sequences from Argentina (43 from this work) and showed at least 35 independent introductions to the country, being the most related sequences from the USA, Central America, Europe, and the Middle East. Besides, five highly supported groups with at least three Argentinean sequences were observed (Figure 3). The largest group included 53 sequences from the CABA and nine provinces, from north to south of the country, suggesting a widespread local transmission and diversification.
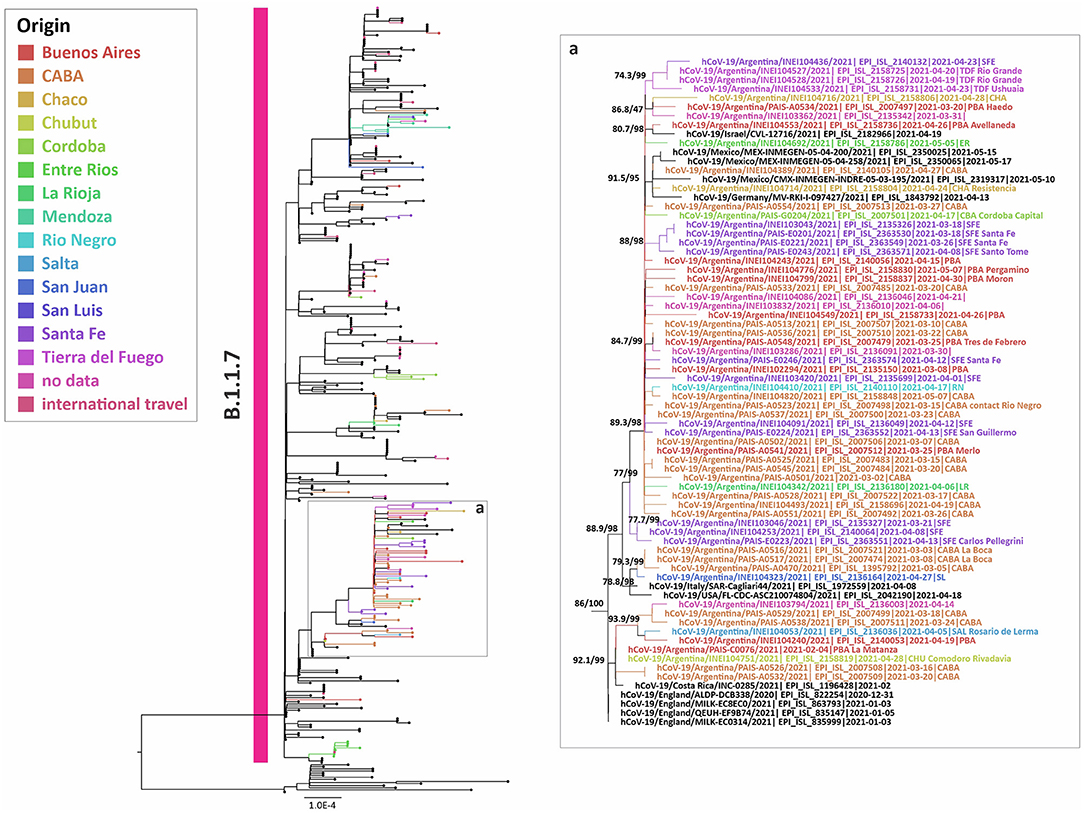
Figure 3. Phylogenetic tree of SARS-CoV-2 whole-genome sequences of Alpha (lineage B.1.1.7). B.1.1.1 sequences were used as outgroup. Only the largest group with Argentinean sequences is shown. The SH-like/UFB values for the relevant groups are indicated for some groups. UFB, ultrafast bootstrap. The right panel (a) represents an inset of the largest B.1.1.7 group from the tree.
Gamma
Gamma was predominant in the second wave of the pandemic in Argentina, found in 2,123 out of 4,851 (43.8%) analyzed cases. This variant was detected in all the regions analyzed. Its frequency in the MABA remained at values over 30% since EW 13-14/2021, reaching a maximum of 68.9% (95% CI = 58.7–77.5) during EW 31-32/2021 (August 1st−14th, 2021). The study of the introduction of Gamma to Argentina through phylogenetic analysis with complete genomic sequences (including 238 from Argentina, 50 from this work) showed at least 50 introductions to the country, with the most related sequences from Brazil and the USA. Besides, 18 supported groups with at least three Argentinean sequences were observed (Figure 4). The largest one included 72 sequences from the CABA and 14 provinces, from north to south of the country, which suggests a wide local transmission and diversification of a common transmission chain together with the establishment of other more geographically limited chains (Figure 4d). Eight sequences from Argentina were located in a clade that showed a basal diversification within the P.1 lineage [previously named as P.1-like-II, (15)]. This clade was composed of sequences from South America only (Brazil, Chile, and Argentina) (Figure 4), which shows a diversification process at a regional level.
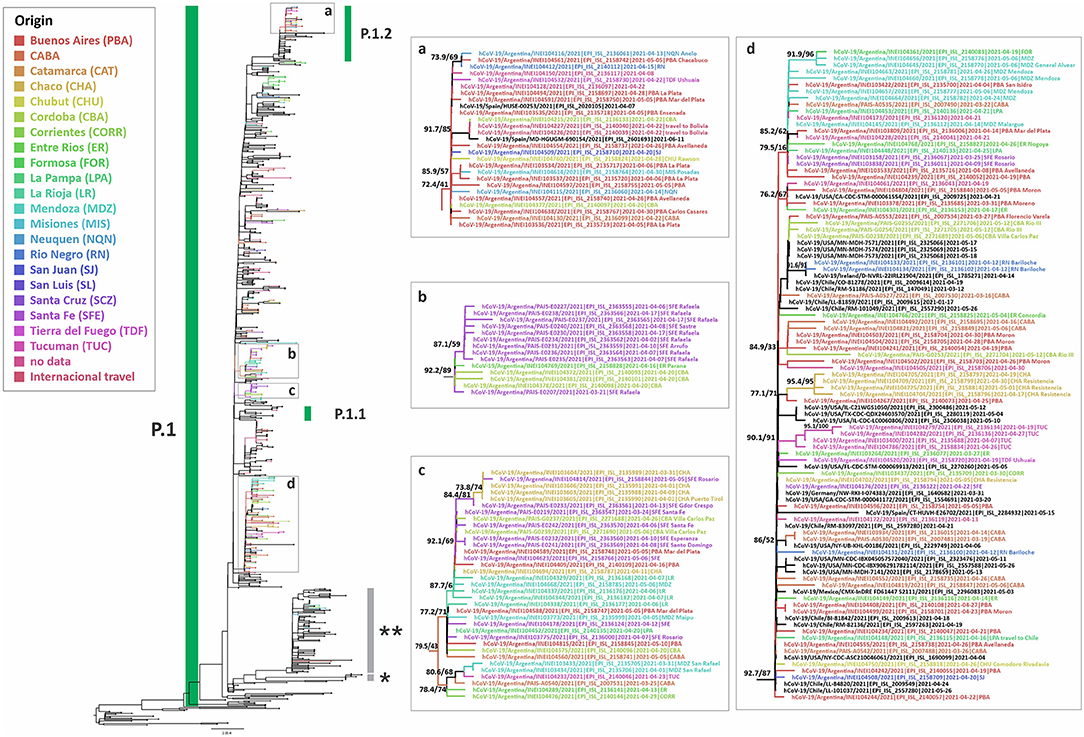
Figure 4. Phylogenetic tree of SARS-CoV-2 whole-genome sequences of Gamma (lineage P.1). B.1.1.28 sequences were used as outgroup. Only selected groups with Argentinean sequences are shown. The SH-like/UFB values for the relevant groups are indicated for some groups. *P.1-like-I and **P.1-like-II described by Gräf et al. (15). UFB, ultrafast bootstrap. The panels (a–d) represent insets of some groups from the tree.
Lambda
Lambda variant showed a continuous increase in MABA since EW 7-8/2021, reaching its maximum frequency of 48.0% (95% CI = 38.5–57.7) during EW 19-20/2021 (May 9th−22nd). After Gamma, this VOI was the most frequent one in the second wave of the country, until its recent displacement by the Delta variant.
Delta
Delta variant has been detected in 103 randomly collected samples, with a frequency in the MABA that increased from 5.1% (95% CI % = 2.2–11.3) in EW 31-32/2021 to 70.9% (95% CI = 57.9–81.2%) in EW 39-40/2021 (from September 26th to October 9th, 2021). Its entry was also noticed in the provinces of Santa Fe and Neuquén, where Gamma was also the predominant variant throughout the second wave. In the last weeks analyzed, Delta was found with a frequency of 26.3% (95% CI = 11.8–48.8) in Santa Fe (EW 39-40/2021) and of 18.2% (95% CI % = 3.2–47.7) in Neuquén (EW 41/2021).
Variant Distribution
The distribution of variants of epidemiological interest was heterogeneous within the MABA region. This could be evidenced when the VOIs/VOCs reached 95% of the total detections in the MABA (between EW 17/2021 and EW 40/2021), a time that marks the beginning of the characteristic pattern of the second wave in the country.
The North GBA presented a marked predominance of Gamma (34/64 cases, 53.1%), followed by Lambda (19/64 cases, 29.7%), Alpha (5/64, 7.9%), and Delta (3/64, 4.7%), similar to the West GBA that presented Gamma in 155/269 cases (57.6%), Lambda in 70/269 cases (21.5%), Alpha in 24/269 cases (8.9%), and Delta in 17/269 cases (6.3%). On the other hand, in the same period, the CABA presented a more homogeneous distribution of Gamma and Lambda, with 275/559 cases (49.2%) and 204/559 cases (36.5%), respectively, followed by Delta in 54/559 cases (9.7%)—detected since EW 35-36/2,021—and Alpha, in 19/559 cases (3.4%). It should be mentioned that the Mu variant was marginally detected in 2/559 cases (EW 27-28/2021 and EW 33-34/2021). Likewise, the South GBA presented Gamma in 101/195 cases (51.8%), followed by Lambda in 81/195 cases (41.5%), Alpha in 7/195 cases (3.6%), and Delta in 4/195 cases (2.1%). Finally, GLP showed a remarkable predominance of Gamma, with 84/117 (71.8%) (Figure 2C).
The analysis of sporadic sampling from various parts of the country showed Gamma as the predominant variant in most of the provinces studied, although it also revealed a heterogeneous distribution of other variants and mutations of interest (Supplementary Table 1).
Mutations of Interests
The E484K mutation—not associated with VOC/VOI signatures—was found in 144 cases. Most of the samples were from the CABA and the GBA regions from individuals without travel history, suggesting local circulation, at least, since the last week of December 2020, when it was first detected by our surveillance program. Nevertheless, a reduction in its frequency was observed since EW 12/2021 in the MABA, being replaced by VOCs and VOIs of more recent emergence, such as Lambda (Figure 2B, Supplementary Table 2). So far, 53 of 144 cases were analyzed by full-length sequencing and all of them have been identified as Zeta.
Other mutations of interest, such as L452R—as the unique mutation of interest detected in the sequenced region—have been occasionally observed in several regions, mainly in the MABA (Supplementary Table 1), although it never exceeded 5% of detections (Figure 1). The complete genome analysis of 14 cases allowed the identification of the Epsilon variant in 12 (one from the lineage B.1.429 and 11 from the lineage B.1.427) and the lineage A.2.5 in two cases.
Discussion
In this work, we show genomic evidence of the introduction and local transmission of the SARS-CoV-2 variants Gamma, Lambda, and Delta, and to a lesser extent, of Alpha, Epsilon, and Zeta in Argentina, the sporadic detection of Mu and the circulation of mutations of interests (such as L452R and E484K in Spike), in different geographical regions of the country.
The main lineages that circulated toward the end of the first wave were completely replaced by emerging variants at the regional (such as Gamma and Lambda) and global (such as Alpha and Delta) levels within a few weeks, as was previously observed in other countries of the region (16–18).
These results demonstrate the impact of the regional circulation of SARS-CoV-2 variants on the epidemiology of Argentina. Importantly, these data have contributed to the WHO declaration of Lambda as a global VOI (19). The neutralization capacity of sera, transmission, clinical behavior, and impact on vaccine effectiveness of this VOI is being studied. The first results have shown that the neutralization capacity of convalescent sera from the first wave in Argentina and sera from individuals vaccinated with Sputnik V was not compromised (6, 20). On the other hand, increased infectivity and resistance to neutralizing antibodies produced by individuals immunized with CoronaVac (SinoVac) and mRNA-1273 (Moderna) vaccines were observed (21–23).
Mutations at aa positions 484 and 452 of Spike protein have also been detected, with several samples confirmed as Zeta or Epsilon variants, respectively. These mutations have been associated with possible immune escape and modified affinity to the human receptor and may occur in various newly emerging lineages worldwide (22, 24, 25). Both positions are located within the receptor-binding motif. On one hand, the E484K mutation, constitutively present in Beta, Gamma, Mu, and Zeta variants, was associated with resistance to neutralization by monoclonal antibodies, convalescent, and vaccinated sera (4, 26–28). On the other hand, mutations at position 452 of the Spike protein, were associated with decreased neutralization by monoclonal antibodies, convalescent, and some vaccine sera (22, 24, 29). Another important mutation in Spike is P681R, recently associated with an enhanced viral fusion, which would result in a more efficient entry of the virus into the cell and a greater capacity for the virus to spread through cell-cell passages (30–32).
In recent months, the Delta variant has spread to multiple countries, causing new waves of infections around the world. Although South America is at the end of its second wave and is the region with the lowest prevalence of Delta in the period studied, this variant has already been detected in all the countries of the region and many of them, showing community transmission. The latest data from neighboring countries indicate that Delta is already dominant in Brazil, Chile, and Uruguay, and that community circulation has been verified in Paraguay. The latest reports indicate that Delta is found in ~87–92% of the cases in Chile during EW 38-39/2021 (18), in ~91.8% of the cases in Brazil during September 2021 (33), and 100% cases in Uruguay during October 2021 (34).
In this work, the proportion of Delta increased from 5% to more than 70% of MABA cases in eight epidemiological weeks, displacing the Gamma and Lambda variants, which were predominant in the second wave in the country. Similarly, an increase in Delta frequency detection, displacing Gamma, began to be observed in Santa Fe and Neuquén provinces. In this context, it is expected that eventually, Delta will be dominant in Argentina as in most countries.
These results showed a shared regional epidemiological situation between Argentina and neighboring countries, up to now characterized by a concurrent increase in Delta detection frequency, simultaneously with the report of a stable number of cases. However, the latest data would indicate that this trend could begin to reverse both in Chile and in some regions of Argentina, where cases have started to slowly increase, accompanied by the opening of massive social activities.
South American countries are facing the verge of a potential third wave, which could be restricted and/or favored by specific population groups that have not yet been vaccinated. The uncertainties in terms of the future epidemiological landscape in the region, the unknown lasting protection of the vaccines already applied, or the potential emergence of novel variants leading to antigenic drift, reinforce the importance of the molecular surveillance studies of viral circulation with a focus on the regional level.
In conclusion, the surveillance strategy implemented over 51 epidemiological weeks in Argentina, based on Spike and complete genome sequencing, allowed us to describe the introduction, establishment, dispersion, and evolution of SARS-CoV-2 variants of interest and concern in the second wave of the COVID-19 pandemic in Argentina and contributed to the description of variants of importance for South America. The main variants found were Gamma, Lambda, Delta, and to a lesser extent, Alpha, Zeta, and Epsilon. This implementation allowed a rapid response in a limited-resources scenario and contributed to the implementation of public health measures to control disease spread in the country.
Data Availability Statement
The datasets presented in this study can be found in online repositories. The names of the repository/repositories and accession number(s) can be found in the article/Supplementary Material.
Author Contributions
CTo, PAu, and GK analyzed data and co-wrote the article. LMoj and HD analyzed data and provided critical revision of the article. DA, SA, MN, and LV performed experiments, analyzed data, and provided critical revision of the article. SGoy designed analysis tools, performed experiments, and provided critical revision of the article. AAm, AGi, ACe, BB, DC, EB, FF, GV, GOr, HL, LF, LP, MD, JM, ML, AFe, PC, PF, MG, SL, NM, MMu, MNa, BP, APu, VP, VR, RA, SZ, GB, GC, MM, CP, AS, GT, ET, and CZ performed experiments and analyzed data. MCa and MI designed analysis tools and performed experiments. DF and JZ provided computing resources. ES provided computing resources and analyzed data. ACa, SB, CCa, ME, MAc, CA, MAl, AAn, APi, MA, ABe, ABo, IB, VC, ACa, CCe, CCi, GM, JC, MCo, ACo, CCr, SD, GD, GV, RE, YE, CE, AFa, FFM, AGa, SGon, MF, MJ, JA, JJ, NA, NL, ML, RL, VL, GL, EL, MMa, CMa, CMe, BM, LMon, VM, AMu, VN, MNi, GOj, MP, MR, CR, FR, GR, VS, LS, JSp, CS, JSu, JT, CTh, MT, RT, OU, MZa, and MZu collected samples and provided data. PAn and PS provided data. AMi provided and analyzed data and provided critical revision of the article. MV analyzed data, co-wrote the paper, and coordinated the project. All authors contributed to the article and approved the submitted version.
Funding
This work was supported by the Proyecto IP COVID-19 N°08 (Ministerio de Ciencia, Tecnología e Innovación, Argentina) and the Focem COF 03/11 Covid-19 (Fondo para la Convergencia Estructural del MERCOSUR). Funding had no role in the study design, collection, analysis, or interpretation of data, in the writing or in the decision to submit the article for publication.
Conflict of Interest
The authors declare that the research was conducted in the absence of any commercial or financial relationships that could be construed as a potential conflict of interest.
Publisher's Note
All claims expressed in this article are solely those of the authors and do not necessarily represent those of their affiliated organizations, or those of the publisher, the editors and the reviewers. Any product that may be evaluated in this article, or claim that may be made by its manufacturer, is not guaranteed or endorsed by the publisher.
Acknowledgments
A preliminary version of this manuscript has been posted as a preprint at medRxiv (35), doi: 10.1101/2021.07.19.21260779.
Supplementary Material
The Supplementary Material for this article can be found online at: https://www.frontiersin.org/articles/10.3389/fmed.2021.755463/full#supplementary-material
References
1. World Health Organization. Tracking SARS-CoV-2 Variants. (2021). Available online at: https://www.who.int/en/activities/tracking-SARS-CoV-2-variants/
2. Funk T, Pharris A, Spiteri G, Bundle N, Melidou A, Carr M, et al. Characteristics of SARS-CoV-2 variants of concern B.1.1.7, B.1.351 or P.1: data from seven EU/EEA countries, weeks 38/2020 to 10/2021. Eurosurveillance. (2021) 26:1–10. doi: 10.2807/1560-7917.ES.2021.26.16.2100348
3. Sheikh A, McMenamin J, Taylor B, Robertson C. SARS-CoV-2 Delta VOC in Scotland: demographics, risk of hospital admission, and vaccine effectiveness. Lancet. (2021) 397:2461–2. doi: 10.1016/S0140-6736(21)01358-1
4. Garcia-Beltran WF, Lam EC St., Denis K, Nitido AD, Garcia ZH, Hauser BM, et al. Multiple SARS-CoV-2 variants escape neutralization by vaccine-induced humoral immunity. Cell. (2021) 184:2372–83.e9. doi: 10.1016/j.cell.2021.03.013
5. Wang P, Casner RG, Nair MS, Wang M, Yu J, Cerutti G, et al. Increased resistance of SARS-CoV-2 variant P.1 to antibody neutralization. Cell Host & Microbe. (2021) 29:747–51. doi: 10.1016/j.chom.2021.04.007
6. Biobanco de Enfermedades Infecciosas,. Seroneutralización de variantes SARS-CoV-2 Gamma (P.1, Manaos), Alpha (B.1.1.7, Reino Unido) y Lambda (C.37, Andina) en individuosvacunados con Sputnik V e individuos recuperados de la infección por SARS-CoV-2. (2021). Available online at: http://pais.qb.fcen.uba.ar/files/reportes/informe_seroneutralizacion_C37_P1.pdf
7. Harvey WT, Carabelli AM, Jackson B, Gupta RK, Thomson EC, Harrison EM, et al. SARS-CoV-2 variants, spike mutations and immune escape. Nat Rev Microbiol. (2021) 19:409–24. doi: 10.1038/s41579-021-00573-0
8. Tao K, Tzou PL, Nouhin J, Gupta RK, de Oliveira T, Kosakovsky Pond SL, et al. The biological and clinical significance of emerging SARS-CoV-2 variants. Nat Rev Genet. (2021) 22:757–73. doi: 10.1038/s41576-021-00408-x
9. Paden C, Tao Y, Queen K, Zhang J, Li Y, Uehara A, et al. Rapid, sensitive, full-genome sequencing of severe acute respiratory syndrome coronavirus 2. Emerg Infect Dis J. (2020) 26:2401. doi: 10.3201/eid2610.201800
10. Quick J,. nCoV-2019 Sequencing Protocol v3 (LoCost) V.3. (2020). Available online at: https://www.protocols.io/view/ncov-2019-sequencing-protocol-v3-locost-bh42j8ye doi: 10.17504/protocols.io.bbmuik6w
11. Katoh K, Standley DM. MAFFT multiple sequence alignment software version 7: improvements in performance and usability. Mol Biol Evol. (2013) 30:772–80. doi: 10.1093/molbev/mst010
12. Minh BQ, Schmidt HA, Chernomor O, Schrempf D, Woodhams MD, von Haeseler A, et al. IQ-TREE 2: new models and efficient methods for phylogenetic inference in the genomic era. Mol Biol Evol. (2020) 37:1530–4. doi: 10.1093/molbev/msaa015
13. Guindon S, Dufayard JF, Lefort V, Anisimova M, Hordijk W, Gascuel O. New algorithms and methods to estimate maximum-likelihood phylogenies: assessing the performance of PhyML 3.0. Syst Biol. (2010) 59:307–21. doi: 10.1093/sysbio/syq010
14. Hoang DT, Chernomor O, von Haeseler A, Minh BQ, Le SV, Vinh LS. UFBoot2: improving the ultrafast bootstrap approximation. Mol Biol Evol. (2018) 35:518–22. doi: 10.1093/molbev/msx281
15. Gräf T, Bello G, Martins Venas TM, Cavalcante Pereira E, Dias Paixão AC, Reis Appolinario L, et al. Identification of SARS-CoV-2 P.1-Related Lineages in Brazil Provides New Insights About the Mechanisms of Emergence of Variants of Concern. Available online at: https://virological.org/t/identification-of-sars-cov-2-p-1-related-lineages-in-brazil-provides-new-insights-about-the-mechanisms-of-emergence-of-variants-of-concern/694 (accessed October 20, 2021).
16. Romero P, Dávila-Barclay A, Salvatierra G, González L, Cuicapuza D, Solís L, et al. The emergence of Sars-CoV-2 Variant Lambda (C.37) in South America. Microbiol Spectr. (2021) 9:e00789–21. doi: 10.1128/Spectrum.00789-21
17. Faria NR, Mellan TA, Whittaker C, Claro IM, Candido D da S, Mishra S, et al. Genomics and epidemiology of the P.1 SARS-CoV-2 lineage in Manaus, Brazil. Science. (2021) 372:815–21. doi: 10.1126/science.abh2644
18. Instituto de Salud Pública Chile,. INFORME DE VARIANTES SARS-CoV-2. (2021). Available online at: https://vigilancia.ispch.gob.cl/app_direct/varcovid/ (accessed October 29, 2021).
19. WHO. COVID-19 Weekly Epidemiological Update - 15 June 2021. (2021). Available online at: https://www.who.int/publications/m/item/weekly-epidemiological-update-on-covid-19-−15-june-2021 (accessed October 20, 2021).
20. Gonzalez Lopez Ledesma MM, Sanchez L, Ojeda DS, Rouco SO, Rossi AH, Varese A, et al. Longitudinal study after Sputnik V vaccination shows durable SARS-CoV-2 Neutralizing antibodies and reduced viral variant escape over time. medRxiv. (2021) 1–19. doi: 10.1101/2021.08.22.21262186
21. Acevedo ML, Alonso-Palomares L, Bustamante A, Gaggero A, Paredes F, Cortés CP, et al. Infectivity and immune escape of the new SARS-CoV-2 variant of interest Lambda. medRxiv. (2021) 1–18. doi: 10.1101/2021.06.28.21259673
22. Tada T, Zhou H, Dcosta BM, Samanovic MI, Mulligan MJ, Landau NR. SARS-CoV-2 lambda variant remains susceptible to neutralization by mRNA vaccine-elicited antibodies and convalescent serum. bioRxiv. (2021) 1–18. doi: 10.1101/2021.07.02.450959
23. Kimura I, Kosugi Y, Wu J, Yamasoba D, Butlertanaka EP, Tanaka YL, et al. SARS-CoV-2 Lambda variant exhibits higher infectivity and immune resistance. bioRxiv. (2021) 1–31. doi: 10.1101/2021.07.28.454085
24. Liu Z, VanBlargan LA, Bloyet L-MM, Rothlauf PW, Chen RE, Stumpf S, et al. Identification of SARS-CoV-2 spike mutations that attenuate monoclonal and serum antibody neutralization. Cell Host Microbe. (2021) 29:477–88.e4. doi: 10.1016/j.chom.2021.01.014
25. Deng X, Garcia-Knight MA, Khalid MM, Servellita V, Wang C, Morris MK, et al. Transmission, infectivity, and antibody neutralization of an emerging SARS-CoV-2 variant in California carrying a L452R spike protein mutation. medRxiv. (2021) 1–47. doi: 10.1101/2021.03.07.21252647
26. Weisblum Y, Schmidt F, Zhang F, DaSilva J, Poston D, Lorenzi JCC, et al. Escape from neutralizing antibodies by SARS-CoV-2 spike protein variants. Elife. (2020) 9:e61312. doi: 10.7554/eLife.61312
27. Greaney AJ, Starr TN, Gilchuk P, Zost SJ, Binshtein E, Loes AN, et al. Complete mapping of mutations to the SARS-CoV-2 spike receptor-binding domain that escape antibody recognition. Cell Host Microbe. (2021) 29:44–57.e9. doi: 10.1016/j.chom.2020.11.007
28. Baum A, Fulton BO, Wloga E, Copin R, Pascal KE, Russo V, et al. Antibody cocktail to SARS-CoV-2 spike protein prevents rapid mutational escape seen with individual antibodies. Science. (2020) 369:1014–8. doi: 10.1126/science.abd0831
29. Starr TN, Greaney AJ, Hilton SK, Ellis D, Crawford KHD, Dingens AS, et al. Deep mutational scanning of SARS-CoV-2 receptor binding domain reveals constraints on folding and ACE2 binding. Cell. (2020) 182:1295–310.e20. doi: 10.1016/j.cell.2020.08.012
30. Peacock TP, Sheppard CM, Brown JC, Goonawardane N, Zhou J, Whiteley M, et al. The SARS-CoV-2 variants associated with infections in India, B.1.617, show enhanced spike cleavage by furin. bioRxiv. (2021) 1–10. doi: 10.1101/2021.05.28.446163
31. Saito A, Nasser H, Uriu K, Kosugi Y, Irie T, Shirakawa K, et al. SARS-CoV-2 spike P681R mutation enhances and accelerates viral fusion. bioRxiv. (2021) 1–40. doi: 10.1101/2021.06.17.448820
32. Liu Y, Liu J, Johnson BA, Xia H, Ku Z, Schindewolf C, et al. Delta spike P681R mutation enhances SARS-CoV-2 fitness over Alpha variant. bioRxiv. (2021) 1–29. doi: 10.1101/2021.08.12.456173
33. Rede Genômica Fiocrruz. Vigilância genômica do SARS-CoV-2 no Brasil. (2021). Available online at: http://www.genomahcov.fiocruz.br/dashboard/ (accessed October 29, 2021).
34. MSP. Ministerio de Salud Pública de Uruguay. (2021). Available online at: https://www.gub.uy/ministerio-salud-publica/home (accessed October 29, 2021).
Keywords: SARS-CoV-2, variants, South America, surveillance, spike sequences, Gamma, Lambda, Delta
Citation: Torres C, Mojsiejczuk L, Acuña D, Alexay S, Amadio A, Aulicino P, Debat H, Fay F, Fernández F, Giri AA, Goya S, König G, Lucero H, Nabaes Jodar M, Pianciola L, Sfalcin JA, Acevedo RM, Bengoa Luoni S, Bolatti EM, Brusés B, Cacciabue M, Casal PE, Cerri A, Chouhy D, Dus Santos MJ, Eberhardt MF, Fernandez A, Fernández PdC, Fernández Do Porto D, Formichelli L, Gismondi MI, Irazoqui M, Campos ML, Lusso S, Marquez N, Muñoz M, Mussin J, Natale M, Oria G, Pisano MB, Posner V, Puebla A, Re V, Sosa E, Villanova GV, Zaiat J, Zunino S, Acevedo ME, Acosta J, Alvarez Lopez C, Álvarez ML, Angeleri P, Angelletti A, Arca M, Ayala NA, Barbas G, Bertone A, Bonnet A, Bourlot I, Cabassi V, Castello A, Castro G, Cavatorta AL, Ceriani C, Cimmino C, Cipelli J, Colmeiro M, Cordero A, Cristina C, Di Bella S, Dolcini G, Ercole R, Espasandin Y, Espul C, Falaschi A, Fernandez Moll F, Foussal MD, Gatelli A, Goñi S, Jofré ME, Jaramillo J, Labarta N, Lacaze MA, Larreche R, Leiva V, Levin G, Luczak E, Mandile M, Marino G, Massone C, Mazzeo M, Medina C, Monaco B, Montoto L, Mugna V, Musto A, Nadalich V, Nieto MV, Ojeda G, Piedrabuena AC, Pintos C, Pozzati M, Rahhal M, Rechimont C, Remes Lenicov F, Rompato G, Seery V, Siri L, Spina J, Streitenberger C, Suárez A, Suárez J, Sujansky P, Talia JM, Theaux C, Thomas G, Ticeira M, Tittarelli E, Toro R, Uez O, Zaffanella MB, Ziehm C, Zubieta M, Mistchenko AS, Valinotto L and Viegas M (2021) Cost-Effective Method to Perform SARS-CoV-2 Variant Surveillance: Detection of Alpha, Gamma, Lambda, Delta, Epsilon, and Zeta in Argentina. Front. Med. 8:755463. doi: 10.3389/fmed.2021.755463
Received: 08 August 2021; Accepted: 02 November 2021;
Published: 10 December 2021.
Edited by:
Susan Christina Welburn, University of Edinburgh, United KingdomReviewed by:
Diana Carolina Hernández Castro, Rosario University, ColombiaArif Ansori, Airlangga University, Indonesia
Copyright © 2021 Torres, Mojsiejczuk, Acuña, Alexay, Amadio, Aulicino, Debat, Fay, Fernández, Giri, Goya, König, Lucero, Nabaes Jodar, Pianciola, Sfalcin, Acevedo, Bengoa Luoni, Bolatti, Brusés, Cacciabue, Casal, Cerri, Chouhy, Dus Santos, Eberhardt, Fernandez, Fernández, Fernández Do Porto, Formichelli, Gismondi, Irazoqui, Campos, Lusso, Marquez, Muñoz, Mussin, Natale, Oria, Pisano, Posner, Puebla, Re, Sosa, Villanova, Zaiat, Zunino, Acevedo, Acosta, Alvarez Lopez, Álvarez, Angeleri, Angelletti, Arca, Ayala, Barbas, Bertone, Bonnet, Bourlot, Cabassi, Castello, Castro, Cavatorta, Ceriani, Cimmino, Cipelli, Colmeiro, Cordero, Cristina, Di Bella, Dolcini, Ercole, Espasandin, Espul, Falaschi, Fernandez Moll, Foussal, Gatelli, Goñi, Jofré, Jaramillo, Labarta, Lacaze, Larreche, Leiva, Levin, Luczak, Mandile, Marino, Massone, Mazzeo, Medina, Monaco, Montoto, Mugna, Musto, Nadalich, Nieto, Ojeda, Piedrabuena, Pintos, Pozzati, Rahhal, Rechimont, Remes Lenicov, Rompato, Seery, Siri, Spina, Streitenberger, Suárez, Suárez, Sujansky, Talia, Theaux, Thomas, Ticeira, Tittarelli, Toro, Uez, Zaffanella, Ziehm, Zubieta, Mistchenko, Valinotto and Viegas. This is an open-access article distributed under the terms of the Creative Commons Attribution License (CC BY). The use, distribution or reproduction in other forums is permitted, provided the original author(s) and the copyright owner(s) are credited and that the original publication in this journal is cited, in accordance with accepted academic practice. No use, distribution or reproduction is permitted which does not comply with these terms.
*Correspondence: Carolina Torres, Y3RvcnJlc0BmZnliLnViYS5hcg==; Mariana Viegas, dmllZ2FzbWFyaWFuYUBjb25pY2V0Lmdvdi5hcg==
†These authors have contributed equally to this work and share first authorship
‡These authors have contributed equally to this work and share second authorship
§These authors have contributed equally to this work and share third authorship
¶These authors have contributed equally to this work and share forth authorship