- 1Department of Anesthesiology, Chi Mei Medical Center, Liouying, Tainan City, Taiwan
- 2Department of Emergency Medicine, E-Da Hospital, Kaohsiung City, Taiwan
- 3College of Medicine, I-Shou University, Kaohsiung City, Taiwan
- 4Department of Medical Imaging, Chi Mei Medical Center, Tainan City, Taiwan
- 5Department of Health and Nutrition, Chia Nan University of Pharmacy and Science, Tainan City, Taiwan
- 6Institute of Biomedical Sciences, National Sun Yat-sen University, Kaohsiung City, Taiwan
- 7Department of Anesthesiology, Chi Mei Medical Center, Tainan City, Taiwan
Background: To evaluate the analgesic efficacy and risk of low-to-medium dose intrathecal morphine (ITM) (i.e., ≤0.5 mg) following cardiac surgery.
Methods: Medline, Cochrane Library, Google scholar and EMBASE databases were searched from inception to February 2022. The primary outcome was pain intensity at postoperative 24 h, while the secondary outcomes included intravenous morphine consumption (IMC), extubation time, hospital/intensive care unit (ICU) length of stay (LOS), and ITM-associated side effects (e.g., respiratory depression). Subgroup analysis was performed on ITM dosage (low: <0.3 mg vs. medium: 0.3–0.5 mg).
Results: Fifteen RCTs involving 683 patients published from 1988 to 2021 were included. Pooled results showed significantly lower postoperative 24-h pain scores [mean difference (MD) = −1.61, 95% confidence interval: −1.98 to −1.24, p < 0.00001; trial sequential analysis: sufficient evidence; certainty of evidence: moderate] in the ITM group compared to the controls. Similar positive findings were noted at 12 (MD = −2.1) and 48 h (MD = −1.88). Use of ITM was also associated with lower IMC at 24 and 48 h (MD: −13.69 and −14.57 mg, respectively; all p < 0.05) and early tracheal extubation (i.e., 48.08 min). No difference was noted in hospital/ICU LOS, and nausea/vomiting in both groups, but patients receiving ITM had higher risk of pruritus (relative risk = 2.88, p = 0.008). There was no subgroup difference in IMC except a lower pain score with 0.3–0.5 mg than <0.3 mg at postoperative 24 h. Respiratory depression events were not noted in the ITM group.
Conclusion: Our results validated the analgesic efficacy of low-to-medium dose ITM for patients receiving cardiac surgery without increasing the risk of respiratory depression.
Introduction
Cardiac surgery, which is traditionally performed via median sternotomy and involves extensive tissue retraction and dissection, can be associated with severe pain within postoperative 2 days (1, 2). Not only does uncontrolled pain activate the sympathetic nervous system and increase myocardial oxygen demand by triggering tachycardia, increased cardiac contractility, and hypertension (3), but it could also increase the risks of pulmonary infections and other complications through restricting respiratory capacity, hampering breathing mechanism, and impairing clearance of respiratory secretions (4). Notwithstanding the analgesic effectiveness of high-dose opioid, the associations with potential adverse side effects including prolonged mechanical ventilation, postoperative respiratory complications, and lengthened intensive care unit (ICU) stay have precluded its incorporation into the standard care protocol for patients undergoing cardiac surgery (5, 6). To address this issue, previous studies have shown that central neuraxial blocks (i.e., epidural and intrathecal analgesia) combined with general anesthesia (GA) could attenuate the severity of pain and adrenergic stress response as well as analgesic consumption more effectively compared to parenteral analgesia (7, 8). Indeed, intrathecal analgesia has been gaining popularity for pain control among patients receiving cardiac surgery to alleviate stress response and enhance postoperative recovery (9–11).
Intrathecal morphine (ITM), which enables rapid action of morphine on the central nervous system by enhancing its access to the cerebrospinal fluid, is being increasingly used in a variety of surgeries to provide effective analgesia and decrease opioid consumption (12–15). In addition to its analgesic advantages, other beneficial effects may include a potentially reduced hospital length of stay (LOS) and enhanced recovery after surgery (15–17). However, its use may be associated with side effects such as nausea, vomiting, itching, and even respiratory depression (12–15). Although ITM has been used for decades in patients undergoing cardiac surgery, its possible association with respiratory depression as reported in a previous meta-analysis (i.e., odds ratio of 7.86) has raised a clinical concern that may restrict its application in this patient population (18). On the other hand, pooled evidence has revealed that ITM-associated adverse events are dose-dependent (15, 19). In that meta-analysis involving patients undergoing cardiac and non-cardiac surgeries, a relatively high dosage of ITM (e.g., >0.5 mg or >7 μg/kg) was adopted in over 40% of the included studies (i.e., 11 out of 27 trials) (18). In contrast, focusing on patients undergoing abdominal surgeries, a meta-analysis suggested that ITM with a dose less than 0.5 mg would not increase the risk of respiratory depression (15).
Although ITM has been reported to be a promising analgesic approach for non-cardiac surgery (15), the analgesic efficacy and associated risks of a relatively low-dose ITM remain unclear in those receiving cardiac procedures. As previous meta-analyses have reported an association of an ITM dose of less than 0.5 mg with a prompt extubation without increasing the risk of respiratory depression (8, 14), we investigated the analgesic efficacy and safety of ITM dosage of ≤0.5 mg or ≤7 μg/kg (i.e., based on a total dose ≤0.5 mg for an average adult with a body weight of 70 kg). In the current meta-analysis, a low-dose ITM was defined as that of <0.3 mg as previously reported (20), while we defined a medium-dose ITM as 0.3–0.5 mg. By hypothesizing that low-to-medium dose ITM (i.e., ≤0.5 mg) may provide favorable analgesic efficacy without increasing the risk of respiratory depression in patients undergoing cardiac surgery, this updated meta-analysis attempted to provide updated evidence for clinical guidance through reviewing the currently available clinical trials.
Methods
This meta-analysis was conducted in accordance with the recommendations of the PRISMA statement and registered with the International Prospective Register of Systematic Reviews (CRD42022310647).
Data sources and searches
We searched the Cochrane Library, Embase, Google scholar, and Medline databases from inception to February 11, 2022 using the following search terms: [“coronary artery bypass surger*” or “cardiopulmonary bypass surger*” or “cardiovascular surger*” or “cardiac surger*” or “CABG” or “off-pump coronary artery surger*” or “coronary artery bypass graft surger*” or “Heart Surger*” or “Cardiac Surgical Procedure*” or “(Aortic or Mitral or Heart Valve Prosthesis Implantation or Aortic Valve or Mitral Valve) adj4 (procedure* or operation* or surger*)”] and [(“Spinal” or “intraspinal” or “intradural” or “lumbar*” or “theca*” or “intrathecal” or “subarachnoid*” or “sub arachnoid*” or “regional”) adj4 (puncture* or inject* or anesth* or anaesth* or needle*)] limited to randomized controlled trials (RCTs). No restriction was placed on gender, language, study location, and sample size during literature search. The search strategies for one of these databases are demonstrated in Supplementary Table 1. Additional records identified by scrutinizing the reference lists of the retrieved studies were also reviewed for eligibility of being included in the current study.
Inclusion criteria
To identify articles eligible for the present meta-analysis, we adopted the following criteria: (a) Population: adult patients (age ≥18 years) undergoing a variety of cardiac surgeries with or without cardiopulmonary bypass, (b) Intervention: the use of a low-to-medium dose ITM with or without adjuncts (e.g., local anesthetics or short-acting opioids) as the intervention approach., (c) Comparison: ITM was not administered for postoperative pain control, (d) Outcomes: pain score, intravenous morphine consumption, length of hospital/ICU stay, extubation time, and ITM-associated side effects. We only included RCTs for analysis and contacted the authors of the included articles in which necessary information was missing in an attempt to access the original data.
Exclusion criteria
Exclusion criteria were: (1) studies which adopted a relatively high-dose ITM (i.e., >0.5 mg or 7 μg/kg); (2) those without a control group; (3) those in which information regarding outcomes was unavailable, and (4) RCTs presented only as letters or abstracts, or (5) those published as reviews, case reports, or other forms instead of original research.
Study selection
Two authors first independently reviewed the titles and abstracts of the retrieved articles for eligibility of being incorporated into the current study. The same two authors then independently scrutinized the full texts of the potentially eligible studies according to the inclusion and exclusion criteria. Discrepancies in opinions about the suitability of inclusion for a particular RCT were settled through consulting a third reviewer.
Data extraction
The following information was retrieved from each study: first author, year of publication, patient characteristics, sample size, dosage of ITM, type of surgery, extubation time, intravenous morphine consumption, postoperative pain score, ITM-related side effects (e.g., pruritus, respiratory depression, nausea/vomiting), hospital LOS, ICU LOS. Disagreements were solved through discussion with a third author.
Outcomes and definitions
The primary outcome was the analgesic efficacy of low-to-medium dose ITM as reflected by the postoperative pain score at postoperative 24 h, while the secondary outcomes included intravenous morphine consumption, extubation time, and hospital/ICU LOS as well as the risks of pruritus, respiratory depression, and nausea/vomiting. The definition of respiratory depression was in accordance with that of each study. If one study did not clearly define this event, we regarded postoperative reintubation or the use of non-invasive ventilation as an indicator of respiratory depression. Subgroup analysis based on the dosage of ITM (i.e., <0.3 mg vs. 0.3–0.5 mg) was also performed to assess possible dose-dependent analgesic efficacy and side effects. Regarding the possible influence of other factors on postoperative 24-h pain score, we conducted subgroup analyses focusing on the impacts of three confounders: (1) the type of surgery [e.g., coronary artery bypass graft surgery (CABG), valve surgery, combined procedures], (2) the use of cardiopulmonary bypass (i.e., yes vs. no), and (3) the use of other intrathecal agents (i.e., ITM alone vs. ITM combined with other agents).
Assessment of risk of bias
Using the Cochrane’s tool (RoB 2), two authors independently assessed the risks of different biases of the included RCTs, namely, allocation, performance, attrition, measurement, and reporting biases as well as the overall bias (21). The risk of bias of each RCT was reported as “low,” “some concern,” or “high.” Disagreement between the two authors was settled through arbitration that involved a third reviewer.
Data synthesis and analysis
Cochrane Review Manager (RevMan 5.3; Copenhagen: The Nordic Cochrane Centre, The Cochrane Collaboration, 2014) was used for the present meta-analysis. The pooled risk ratios (RRs) and mean difference (MD) with 95% confidence intervals (CIs) were computed for binary and continuous outcomes, respectively. For the current study, visual analog scale (VAS) 0–10 cm or 0–100 mm, numerical rating scale (NRS) 0–10, and visual numeric scale (VNS) 0–10 were converted into VAS 0–10 cm for pain severity comparison (22). Regarding the comparison of opioid dosage across different studies, we converted all opioid dosages to morphine equivalents as previously described (23). We assessed heterogeneity with I2 statistics and defined substantial heterogeneity as an I2 over 50%. Assuming the existence of heterogeneity across the included studies, we adopted a priori a random-effects model for outcome evaluation (22, 24). The potential publication bias was assessed by visual inspection of a funnel plot on encountering 10 or more trials sharing a particular outcome. For equivocal findings from funnel plots, Egger’s test was conducted to investigate the possibility of bias using Comprehensive Meta-Analysis version 3.3.070 (BioSTAT, United States). Sensitivity analysis was performed with a leave-one-out approach to weigh the potential influence of the data from an individual trial on the overall outcome. The level of significance was set at <0.05 for all outcome analyses.
Robustness of the conclusion and reliability of the pooled evidence were evaluated with trial sequential analysis (TSA) to reduce false-positive or false-negative outcomes from multiple testing and sparse data (25, 26). TSA was conducted with TSA viewer version 0.9.5.10 Beta1. We calculated the required information size as well as the trial sequential monitoring boundaries for all outcomes. The variance was obtained from the retrieved data of our included studies.
If the cumulative Z-curve crosses the TSA boundary, there is sufficient evidence for the expected intervention effect with no need for support from further studies. In contrast, if the Z-curve fails to cross the TSA boundaries or attain the required information size, the level of evidence is inadequate to support a conclusion. Setting a type I error at 5%, a power at 80%, and a relative risk reduction at 20% for dichotomous outcomes, we computed the required information size with two-sided tests (27).
Certainty assessment
The certainty of the evidence from our primary and secondary outcomes was assigned to four grades (i.e., high, moderate, low, and very low) by two independent authors based on the probability of study limitations, publication bias, effect consistency, imprecision, and indirectness as described in GRADE. In case of disagreements about certainty ratings, consensus was reached through discussion.
Results
Study selection and characteristics
The study selection process is shown in Figure 1. A total of 740 records were acquired from database search. After removing duplicates and records that did not meet the inclusion criteria, we identified 39 potentially eligible trials for a more detailed review. After analyzing the full text, 24 studies were excluded because of being non-RCTs (review article, n = 2), availability only as an abstract (n = 1), no control group (n = 1), and use of ITM > 0.5 mg or 7 μg/kg (n = 20) (Supplementary Table 2). Finally, 15 RCTs published between 1988 and 2021 met our inclusion criteria (3, 28–41). The characteristics of the included trials are shown in Table 1. The mean or median age ranged from 25.9 to 67.3 years with a male predominance (>70%, 11 trials). CABG and mixed CABG/valve surgery were performed in eight (3, 31, 34–36, 39–41) and four (28, 29, 33, 37) trials, respectively, while the other three trials were focused on minimally invasive cardiac surgery (n = 2) (30, 38) and valve surgery (n = 1) (32). ITM was administered preoperatively in all studies with a maximum dose of 0.5 mg or 7 μg/kg and a minimum dose of 0.25 mg or 0.4 μg/kg. Intrathecal morphine was used as a single agent in 12 trials (3, 28, 30–34, 36, 38–41) and as a component of a combined regimen in three studies (29, 35, 37). Patients in the control groups received local anesthesia of the back, no treatment, or placebo (e.g., intrathecal normal saline). Analysis of the occurrence of respiratory depression including postoperative reintubation or the use of non-invasive ventilation in the five trials with available information (3, 30–33) showed no such incidence in a total of 234 patients, suggesting the safety of its clinical use. Nevertheless, because of the absence of events indicating respiratory depression in all of the five studies, statistical analysis could not be performed.
Risk of bias assessment
The assessment of the risk of bias is shown in Figure 2. The overall risk of bias was considered to be low in 11 studies (3, 28–32, 35–37, 39, 41), and high in four trial (33, 34, 38, 40). High risk of bias was associated with bias arising from the randomization process.
Results of syntheses
Primary outcome: Impact of intrathecal morphine on severity of pain at postoperative 24 h
By adopting a random-effects model, ITM was associated with a lower pain score compared to that in the control group at postoperative 24 h (MD = −1.61, 95% CI: −1.98 to −1.24, p < 0.00001, I2 = 90%, 11 trials, 578 participants) (Figure 3). There were similar findings at postoperative 12 h (MD = −2.1, 95% CI: −2.83 to −1.36, p < 0.00001, I2 = 96%, 10 trials, 517 participants) and 48 h (MD = −1.88, 95% CI: −2.83 to −0.93, p = 0.0001, I2 = 80%, 4 trials, 259 participants). Subgroup analysis demonstrated a superior analgesic efficacy associated with a dosage of 0.3–0.5 mg compared to that with <0.3 mg (p = 0.03) at postoperative 24 h, but not at 12 or 48 h (Supplementary Figures 1, 2).
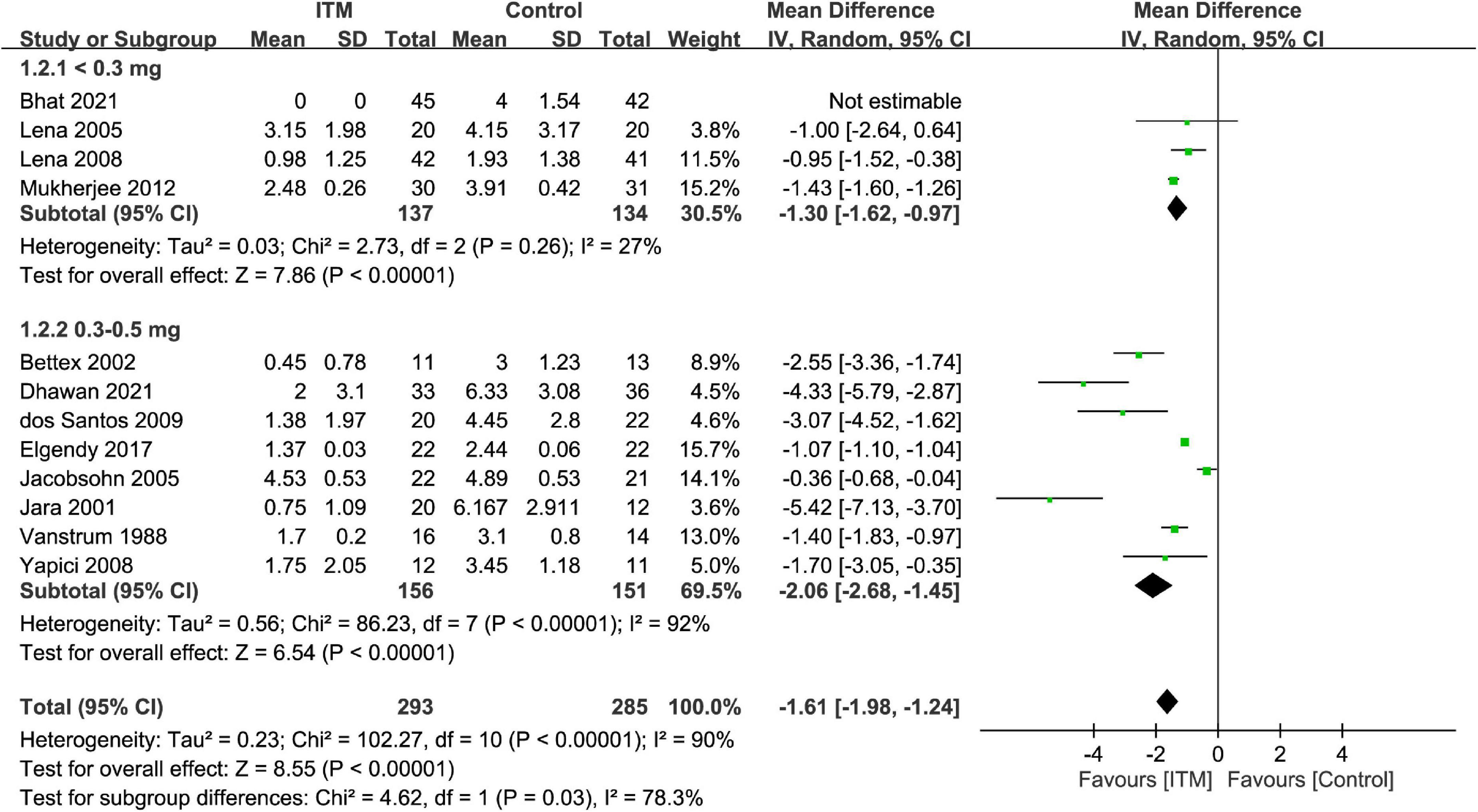
Figure 3. Forest plot comparing the pain score at postoperative 24 h between intrathecal morphine (ITM) and control groups. CI, confidence interval; IV, inverse variance; SD, standard deviation.
The results of subgroup analyses based on the type of cardiac surgery, the use of cardiopulmonary bypass, and combination with other intrathecal agents are demonstrated in Figures 4–6, respectively. Despite the absence of notable subgroup variation in 24-h pain score among different types of cardiac surgery (p = 0.14) (Figure 4), those not subjected to cardiopulmonary bypass (Figure 5) and those receiving ITM alone instead of a combined regimen (Figure 6) were found to have a more significant reduction in 24-h pain score (p < 0.00001 and p = 0.02, respectively).
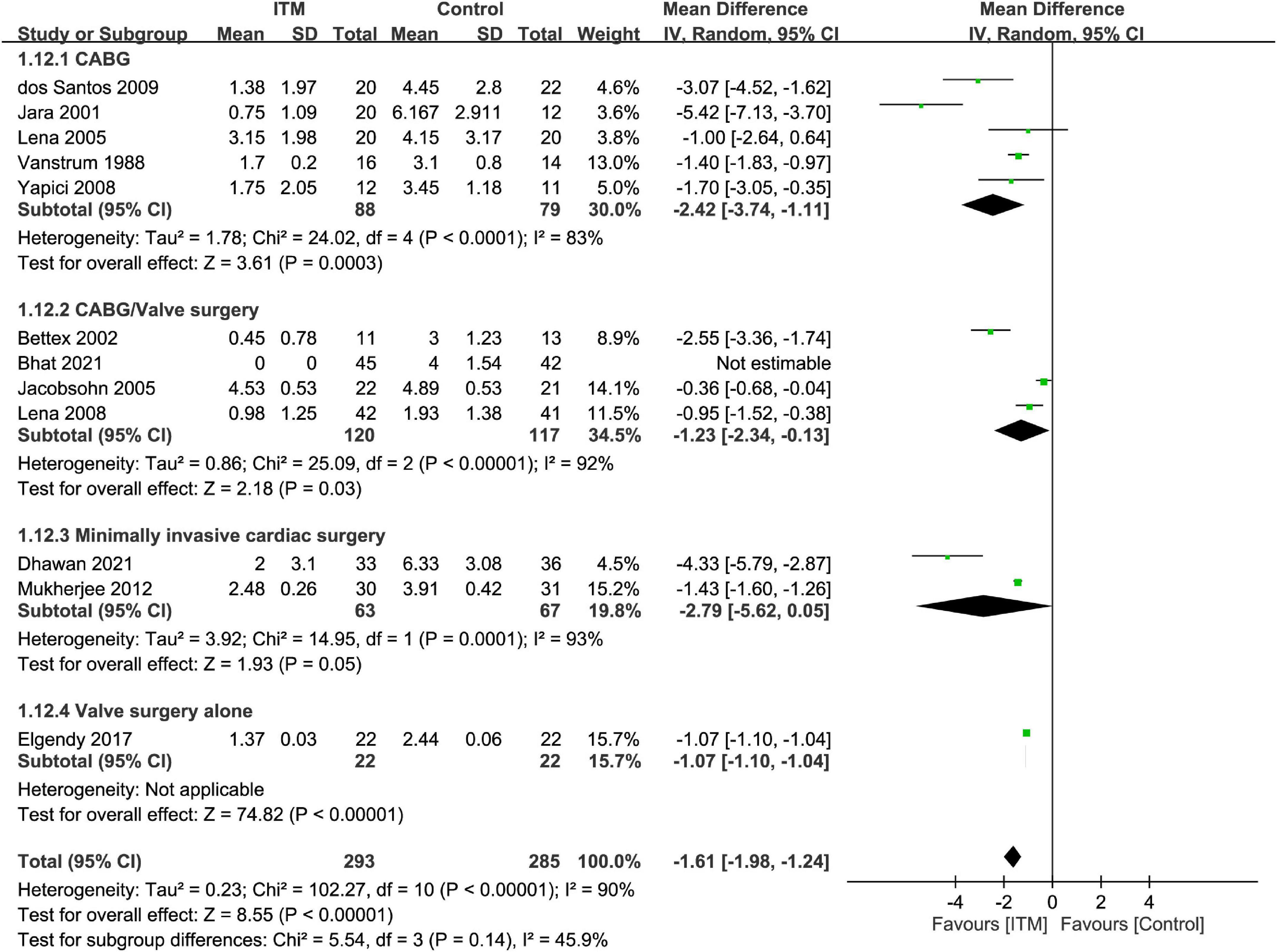
Figure 4. Subgroup analysis comparing postoperative 24-h pain score between intrathecal morphine (ITM) and control groups based on type of cardiac surgery. CI, confidence interval; IV, inverse variance; SD, standard deviation.
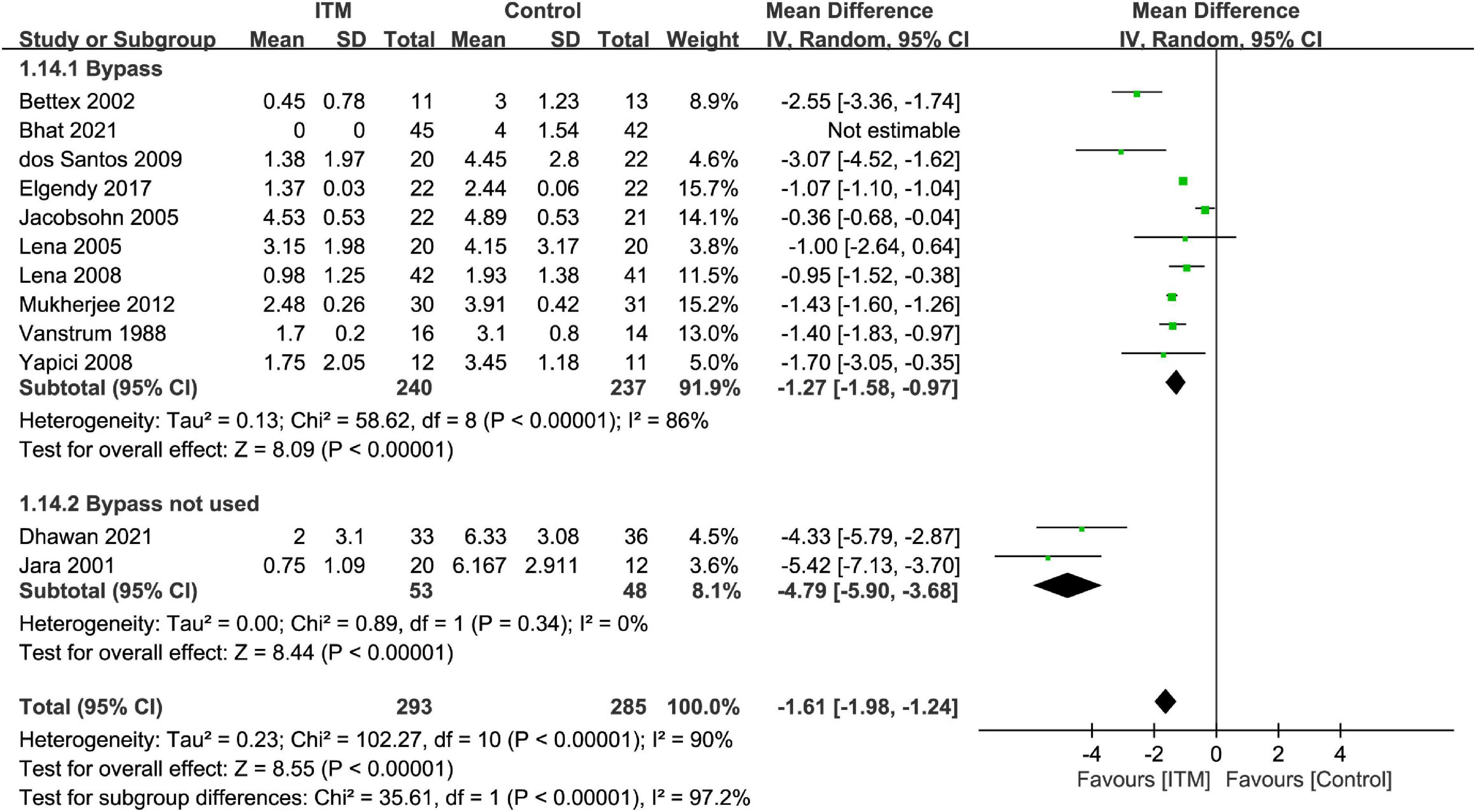
Figure 5. Subgroup analysis comparing postoperative 24-h pain score between intrathecal morphine (ITM) and control groups based on the use of cardiopulmonary bypass. CI, confidence interval; IV, inverse variance; SD, standard deviation.
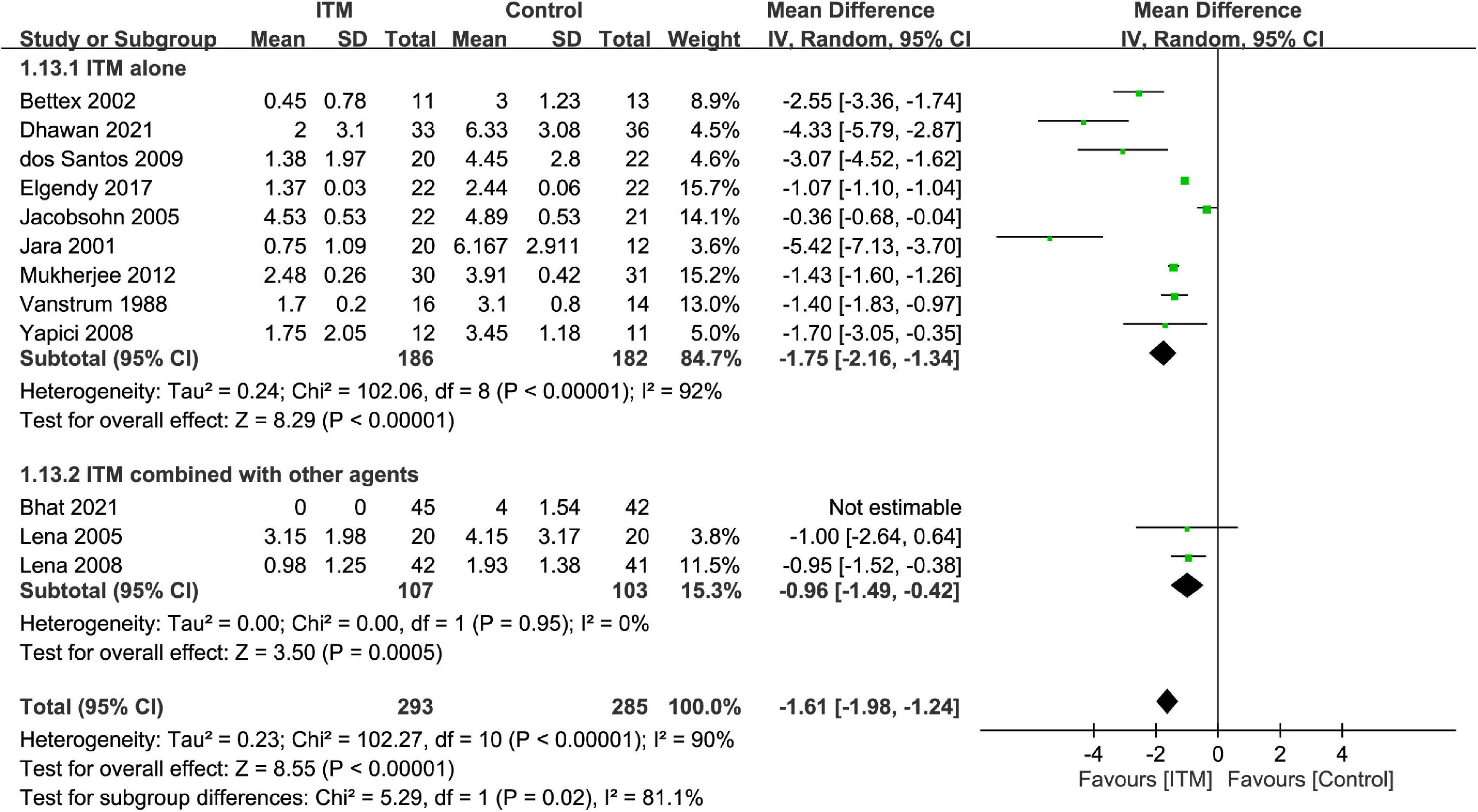
Figure 6. Subgroup analysis comparing postoperative 24-h pain score between intrathecal morphine (ITM) and control groups based on the use of intrathecal agents. CI, confidence interval; IV, inverse variance; SD, standard deviation.
Secondary outcomes: Association of intrathecal morphine with intravenous morphine consumption, early extubation time, and length of stay
Forest plot showed a lower intravenous morphine consumption in the ITM groups than that in the control groups at postoperative 24 h (MD = −13.69, 95% CI: −22.29 to −5.08, p = 0.002; I2 = 88%, 355 participants) (Figure 7) and 48 h (MD = −14.57, 95% CI: −26.98 to −2.17, p = 0.02; I2 = 98%, 289 participants) (Supplementary Figure 3). There were no subgroup differences between the doses of 0.3–0.5 mg and <0.3 mg at these two time points.
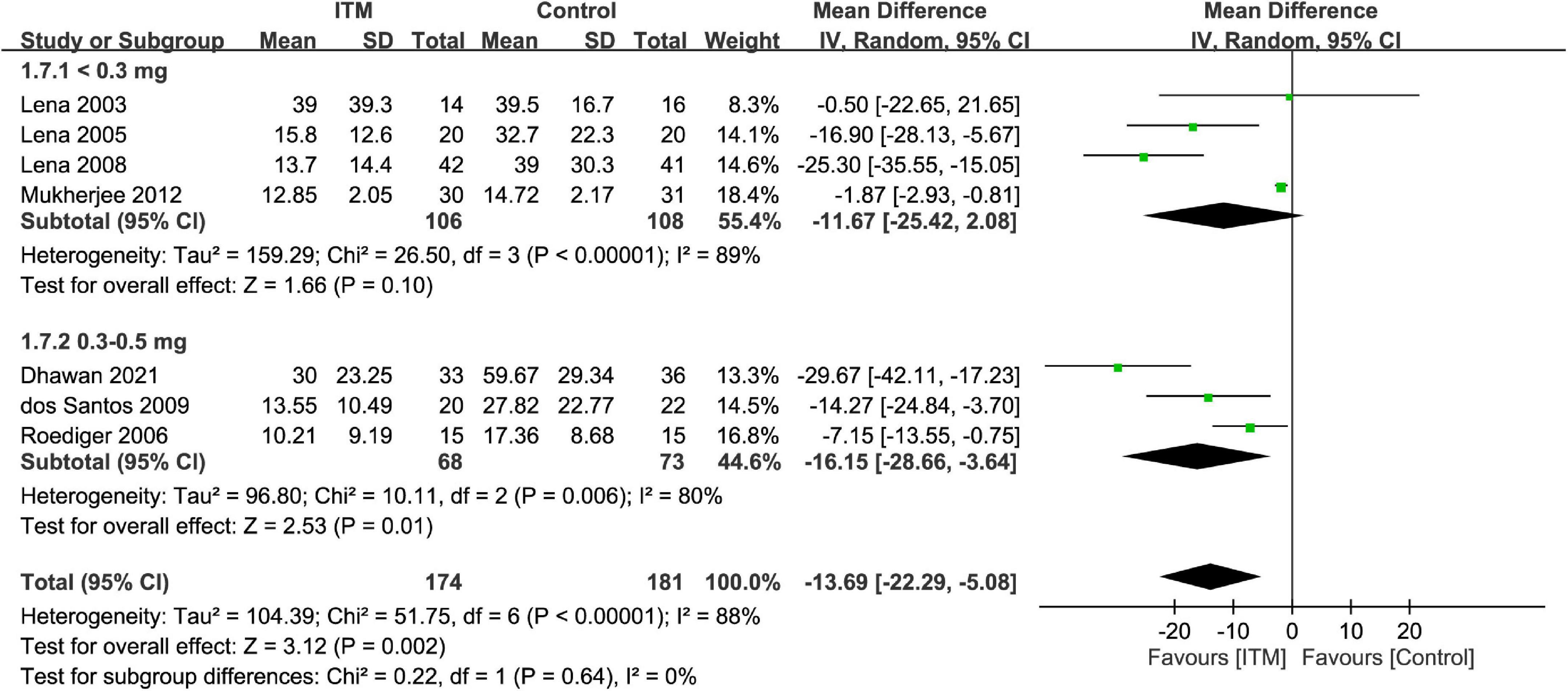
Figure 7. Forest plot comparing intravenous morphine consumption at postoperative 24 h between intrathecal morphine (ITM) and control groups. CI, confidence interval; IV, inverse variance; SD, standard deviation.
The extubation time was 41.4–355 and 39.2–396 min in the ITM and control groups, respectively. Merged results demonstrated a shorter time for tracheal extubation in the ITM group than that in the control group (MD = −48.08 min, 95%: −78.49 to −17.68, p = 0.002, I2 = 75%, 10 trials, 483 participants) (Figure 8). Subgroup analysis revealed no impact of ITM dosage on extubation time (p = 0.2).
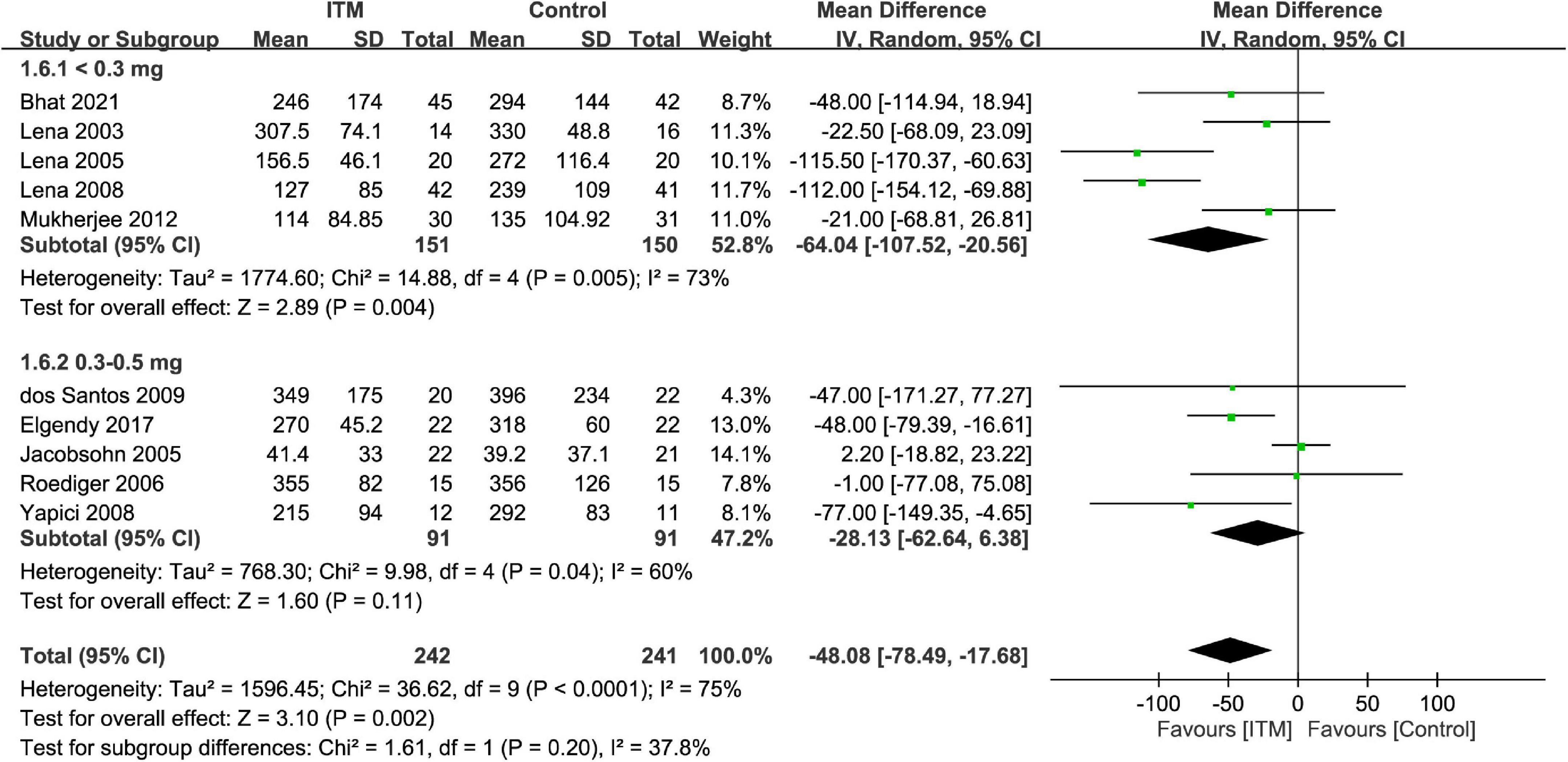
Figure 8. Forest plot comparing extubation time between intrathecal morphine (ITM) and control groups. CI, confidence interval; IV, inverse variance; SD, standard deviation.
Our results showed no significant beneficial effect of using ITM on shortening ICU LOS (MD = −5.69 h, 95% CI: −11.83 to 0.46, p = 0.07, I2 = 87%, four trials, 158 participants) (Supplementary Figure 4) or hospital LOS (MD = −0.53 days, 95% CI: −1.16 to 0.1, p = 0.1, I2 = 0, four trials, 178 participants) (Supplementary Figure 5). Subgroup analysis also demonstrated no dose-related impact of ITM on hospital/ICU LOS.
Secondary outcomes: Impact of intrathecal morphine on risks of nausea/vomiting and pruritus
Merged results demonstrated no association between ITM and the risk of PONV (RR = 1.13, 95% CI: 0.73 to 1.74, p = 0.59, I2 = 29%, nine trials, 495 participants) (Supplementary Figure 6). Consistently, subgroup analysis showed no impact of ITM on the risk of PONV (p = 0.75).
Forest plot revealed a higher risk of pruritus in patients receiving ITM compared to that in the control group (RR = 2.88, 95% CI: 1.31 to 6.31, p = 0.008, I2 = 0%, eight trials, 411 participants) (Supplementary Figure 7). Nevertheless, subgroup analysis demonstrated no correlation between ITM dosage and the risk of pruritus (p = 0.92).
Sensitivity analysis and publication bias
Sensitivity analysis confirmed the robustness of most results except three secondary outcomes (i.e., intravenous morphine consumption at postoperative 24 h, ICU LOS, and risk of pruritus). The potential publication bias was assessed by visual inspection of a funnel plot in three outcomes (i.e., pain score at postoperative 12-, 24 h, and extubation time) (Supplementary Figures 8–10). There is a low risk of publication bias on extubation time (Supplementary Figure 10), while there was uncertainty on pain score at postoperative 12 and 24 h (Supplementary Figures 8, 9). Egger’s test revealed p-values of 0.68 and 0.086 for pain score at 12 and 24 h, respectively, indicating no publication bias for the two outcomes.
Trial sequence analysis
Trial sequential analysis demonstrated sufficient evidence to support a robust conclusion for pain score at postoperative 24 h (i.e., primary outcome) (Figure 9). In addition, TSA in the current study also suggested a robust conclusion for postoperative pain score (i.e., at 12 and 48 h), intravenous morphine consumption at postoperative 24 h, and extubation time by demonstrating the crossing of cumulative Z-curve through the trial sequential monitoring boundary and reaching the required information size (Supplementary Figures 11–13,15). For intravenous morphine consumption at postoperative 48 h, failure of the cumulative Z-curve to cross the trial sequential monitoring boundary or reach the required information size on TSA suggested inadequate evidence for this outcome (Supplementary Figure 14). Similarly, the cumulative Z-curve did not cross the futility boundary for hospital/ICU LOS and risk of nausea/vomiting, implicating inconclusive evidence for these outcomes (Supplementary Figures 16–18). TSA was not conducted for risk of pruritus due to insufficient information (Supplementary Figure 19).
Certainty of evidence
Table 2 summarizes the quality of evidence for outcome measures in accordance with the GRADE system. The levels of evidence were graded as low, moderate, and high in two (intravenous morphine consumption at 24 and 48 h), five (pain score at 12–48 h, extubation time, ICU stay), and three (hospital stay, risk of nausea/vomiting, risk of pruritus) outcomes, respectively. The level of evidence was downgraded due to a high degree of inconsistency and imprecision.
Discussion
Satisfactory postoperative pain control is essential to patient recovery after cardiothoracic surgery because inadequate analgesia may contribute to prolonged immobilization as well as impaired lung expansion and respiratory function, especially in those undergoing median sternotomy (4, 42–44). Our results demonstrated an association of low-to-medium dose ITM with a lower pain score and intravenous morphine consumption compared to the control group up to postoperative 48 h without increasing the risks of PONV and respiratory depression. Besides, a shorter extubation time (i.e., 48.08 min) was noted in patients receiving low-to-medium dose ITM despite the absence of a positive impact of ITM on ICU/hospital LOS. On the other hand, ITM-associated pruritus was noted regardless of the dosage used in the current meta-analysis.
Although two previous meta-analyses recruiting patients receiving cardiac (8) or cardiac/non-cardiac (18) surgery reported the effectiveness of ITM for reducing pain score and intravenous morphine consumption, most trials in one meta-analysis (i.e., 13 out of 17) (8) and a significant proportion in the other (i.e., 11 out of 27) (18) used a relatively high dose of ITM (i.e., 8 μg/kg–4 mg). Therefore, the relatively high risk of respiratory depression (odds ratio: 7.86) in one of the meta-analyses (18), which may partly be attributed to a high ITM dosage, raises the concern over the possibility of a dose-related increase in the risk of respiratory complications. Similarly, despite focusing on patients receiving CABG, another meta-analysis including mostly trials adopting a high-dose ITM (8) could not reflect the efficacy of low-to-medium dose ITM in the cardiac surgery setting. Accordingly, the present study, which systematically reviewed the evidence from currently available clinical trials, is the first to investigate the impacts of low-to-medium dose ITM on the efficacy of postoperative analgesia as well as the risks of adverse side-effects in patients after cardiac surgery.
In general, surgical pain after cardiac procedures is most intense during the first 2 days, especially in the younger population (1). Compared with previous meta-analyses which did not investigate the analgesic efficacy of ITM at postoperative 48 h (8, 18), our finding of a significant reduction in pain intensity associated with low-to-medium dose ITM at postoperative 12–48 h (range of mean difference: −1.61 to −2.1) highlighted its efficacy during the acute painful period. In addition, subgroup analysis indicated no impact of ITM dosage on analgesic efficacy at postoperative 12 and 48 h, implying the feasibility of adopting a low-dose ITM (i.e., <0.3 mg or 4 μg/kg) in the cardiac operation setting.
Despite the lack of a significant beneficial impact of ITM on mortality or the incidence of myocardial infarction following cardiac surgery from pooled evidence (8, 45), optimization of acute pain management with ITM not only may enhance postoperative recovery and minimize the possibility of persistent chronic pain following cardiac surgery (46, 47) but could also reduce the risk of postoperative delirium, which has been identified as a potential sequela of acute pain (48, 49) possibly associated with long-term cognitive decline (50). Hence, our findings suggested that incorporation of ITM into the standard pain management strategy may be recommended for this patient population.
In the present study, ITM was related to a lower intravenous morphine consumption compared with the control groups at postoperative 24 (MD = −13.69 mg) and 48 (MD = −14.57 mg) hours. Consistent with our findings, a previous meta-analysis in which the majority of included trials used a high-dose ITM (i.e., 8 μg/kg–4 mg) reported that ITM decreased intravenous morphine consumption by 11 mg after cardiac surgery (8). The comparable reductions in intravenous morphine dosage between the present study and the previous meta-analysis (8) suggested similar opioid-sparing effects between high-dose (i.e., 8 μg/kg–4 mg) and low-to-medium dose (i.e., ≤0.5 mg) ITM in the cardiac surgery setting. Furthermore, we also found no impact of ITM dosage on intravenous morphine consumption during subgroup analysis (i.e., <0.3 mg vs. 0.3–0.5 mg), implying the feasibility of using a low-dose ITM in clinical practice. Nevertheless, compared with the control group with a median intravenous morphine consumption of 32.7 mg at postoperative 24 h, our study showed a reduction in intravenous morphine dosage only by only 13.69 mg in those receiving low-to-medium dose ITM. Therefore, our findings implied the need for additional postoperative analgesic strategies in patients after cardiac surgery.
Despite the lack of clinical significance, we revealed a shorter extubation time in the ITM group compared to that in the control group (i.e., MD = −48.08 min). This finding may be attributed to a decreased pain intensity and reduced intravenous morphine consumption in the immediate postoperative period (51). In contrast, the use of a relatively high-dose ITM, which could be associated with respiratory depression (15), may mask the beneficial effect of ITM on early tracheal extubation in a previous meta-analysis (8). Taking into account the recommended practice of early extubation (defined as within postoperative 6 h) after cardiac surgery (52) that was demonstrated in our control group, a further reduction of 48.08 min within such a relatively short period by using ITM as shown in the present study could be of clinical significance. Such a tendency for early tracheal extubation in the current meta-analysis may partially explain the relatively minor shortening in extubation time with low-to-medium dose ITM. As early tracheal extubation has been found to be associated with a decreased risk of infections, stroke, renal failure, and mortality (53–55), our results suggested that adoption of low-to-medium dose ITM in patients with a high risk of delayed extubation [e.g., the elderly (56)] may be recommended.
There are several limitations that need to be addressed in the current meta-analysis. First, the relatively small sample size of each trial included in the present study may potentially bias our results. Second, the recruitment of predominantly males (i.e., ≥70% in 11 out of 15 trials) with a relatively young age (i.e., ≤65 years) in our study may restrict the applicability of our findings to females and the aged population. Third, heterogeneity in study design, procedure, drug dosage, and institute-based practices across the included studies may bias our study outcomes. In fact, our finding of a high heterogeneity in pain score and intravenous morphine consumption implicated a potential adverse effect on the reliability of our results. Fourth, the availability of only five trials that provided information about the absence of respiratory depression warrants further investigations into the potential influence. Fifth, because the analgesic efficacy of ITM may be affected by the use of other adjuncts or cardiopulmonary bypass, further studies are needed to address this issue. Finally, the beneficial effects of low-to-medium dose ITM on the risk of mortality and myocardial infarction were not investigated because of limited information available from the included studies.
Conclusion
Our results demonstrated that low-to-medium dose intrathecal morphine (i.e., ≤0.5 mg) was associated with a lower pain severity and intravenous morphine consumption without increasing the risk of respiratory depression. Nevertheless, our finding of only a moderate reduction in intravenous morphine consumption associated with the use of low-to-medium dose ITM warrants further studies to investigate the effectiveness of a multimodal analgesic approach in the post-cardiac surgery care setting.
Author contributions
I-WC and C-KS contributed to conceptualization and literature search. C-CK and W-CL contributed to methodology. C-CK and P-HF contributed to trial selection. K-CH contributed to data analysis. P-HF and I-CT contributed to data extraction. K-CH, C-ML, and C-KS contributed to writing—original draft preparation. K-CH and C-KS contributed to writing—review and editing. All authors have read and agreed to the published version of the manuscript.
Conflict of interest
The authors declare that the research was conducted in the absence of any commercial or financial relationships that could be construed as a potential conflict of interest.
Publisher’s note
All claims expressed in this article are solely those of the authors and do not necessarily represent those of their affiliated organizations, or those of the publisher, the editors and the reviewers. Any product that may be evaluated in this article, or claim that may be made by its manufacturer, is not guaranteed or endorsed by the publisher.
Supplementary material
The Supplementary Material for this article can be found online at: https://www.frontiersin.org/articles/10.3389/fmed.2022.1017676/full#supplementary-material
Footnotes
References
1. Mueller XM, Tinguely F, Tevaearai HT, Revelly JP, Chioléro R, von Segesser LK. Pain location, distribution, and intensity after cardiac surgery. Chest. (2000) 118:391–6.
2. Bignami E, Castella A, Pota V, Saglietti F, Scognamiglio A, Trumello C, et al. Perioperative pain management in cardiac surgery: a systematic review. Minerva anestesiol. (2018) 84:488–503.
3. Alhashemi JA, Sharpe MD, Harris CL, Sherman V, Boyd D. Effect of subarachnoid morphine administration on extubation time after coronary artery bypass graft surgery. J Cardiothorac vasc Anesth. (2000) 14:639–44.
4. Gottschalk A, Cohen SP, Yang S, Ochroch EA. Preventing and treating pain after thoracic surgery. Anesthesiology. (2006) 104:594–600.
5. Oderda GM, Said Q, Evans RS, Stoddard GJ, Lloyd J, Jackson K, et al. Opioid-related adverse drug events in surgical hospitalizations: impact on costs and length of stay. Ann Pharmacother. (2007) 41:400–6.
6. Meade MO, Guyatt G, Butler R, Elms B, Hand L, Ingram A, et al. Trials comparing early vs late extubation following cardiovascular surgery. Chest. (2001) 120:445s–53s.
7. Lee TW, Kowalski S, Falk K, Maguire D, Freed DH, HayGlass KT. High spinal anesthesia enhances anti-inflammatory responses in patients undergoing coronary artery bypass graft surgery and aortic valve replacement: randomized pilot study. PLoS One. (2016) 11:e0149942. doi: 10.1371/journal.pone.0149942
8. Liu SS, Block BM, Wu CL. Effects of perioperative central neuraxial analgesia on outcome after coronary artery bypass surgery: a meta-analysis. Anesthesiology. (2004) 101:153–61. doi: 10.1097/00000542-200407000-00024
9. Hanada S, Kurosawa A, Randall B, Van Der Horst T, Ueda K. Impact of high spinal anesthesia technique on fast-track strategy in cardiac surgery: retrospective study. Reg Anesth pain Med. (2020) 45:22–6. doi: 10.1136/rapm-2018-100213
10. Ellenberger C, Sologashvili T, Bhaskaran K, Licker M. Impact of intrathecal morphine analgesia on the incidence of pulmonary complications after cardiac surgery: a single center propensity-matched cohort study. BMC Anesthesiol. (2017) 17:109. doi: 10.1186/s12871-017-0398-z
11. Hall R, Adderley N, MacLaren C, McIntyre A, Barker R, Imrie D, et al. Does intrathecal morphine alter the stress response following coronary artery bypass grafting surgery? Canad J Anaesth. (2000) 47:463–6. doi: 10.1007/BF03018978
12. AbdelQadir YH, Nabhan AE, Abdelghany EA, Mohamed AG, Tokhey ASE, Elmegeed AA, et al. Efficacy and safety of intrathecal morphine in total knee arthroplasty: A systematic review and meta-analysis. J opioid Manag. (2021) 17:405–16.
13. Wang LM, Zhang Z, Yao RZ, Wang GL. The role of intrathecal morphine for postoperative analgesia in primary total joint arthroplasty under spinal anesthesia: a systematic review and meta-analysis. Pain Med (Malden, Mass). (2021) 22:1473–84. doi: 10.1093/pm/pnab089
14. Wang J, Sun H, Sun WT, Sun HP, Tian T, Sun J. Efficacy and safety of intrathecal morphine for pain control after spinal surgery: a systematic review and meta-analysis. Eur Rev Med Pharmacol Sci. (2021) 25:2674–84.
15. Koning MV, Klimek M, Rijs K, Stolker RJ, Heesen MA. Intrathecal hydrophilic opioids for abdominal surgery: a meta-analysis, meta-regression, and trial sequential analysis. Br J Anaesth. (2020) 125:358–72. doi: 10.1016/j.bja.2020.05.061
16. Koning MV, Teunissen AJW, van der Harst E, Ruijgrok EJ, Stolker RJ. Intrathecal morphine for laparoscopic segmental colonic resection as part of an enhanced recovery protocol: a randomized controlled trial. Reg Anesth Pain Med. (2018) 43:166–73. doi: 10.1097/AAP.0000000000000703
17. Koning MV, de Vlieger R, Teunissen AJW, Gan M, Ruijgrok EJ, de Graaff JC, et al. The effect of intrathecal bupivacaine/morphine on quality of recovery in robot-assisted radical prostatectomy: a randomised controlled trial. Anaesthesia. (2020) 75:599–608. doi: 10.1111/anae.14922
18. Meylan N, Elia N, Lysakowski C, Tramèr MR. Benefit and risk of intrathecal morphine without local anaesthetic in patients undergoing major surgery: meta-analysis of randomized trials. Br J Anaesth. (2009) 102:156–67. doi: 10.1093/bja/aen368
19. Palmer CM, Emerson S, Volgoropolous D, Alves D. Dose-response relationship of intrathecal morphine for postcesarean analgesia. J Am Soc Anesthesiol. (1999) 90:437–44.
20. Cole PJ, Craske DA, Wheatley RG. Efficacy and respiratory effects of low-dose spinal morphine for postoperative analgesia following knee arthroplasty. Br J Anaesth. (2000) 85:233–7. doi: 10.1093/bja/85.2.233
21. Sterne JAC, Savović J, Page MJ, Elbers RG, Blencowe NS, Boutron I, et al. RoB 2: a revised tool for assessing risk of bias in randomised trials. BMJ. (2019) 366:4898. doi: 10.1136/bmj.l4898
22. Hung K-C, Wu S-C, Chang P-C, Chen I, Hsing C-H, Lin C-M, et al. Impact of intraoperative ketamine on postoperative analgesic requirement following bariatric surgery: a meta-analysis of randomized controlled trials. Obesity Surg. (2021) 31:5446–57.
23. Hung K-C, Lin Y-T, Chen K-H, Wang L-K, Chen J-Y, Chang Y-J, et al. The effect of perioperative vitamin C on postoperative analgesic consumption: A meta-analysis of randomized controlled trials. Nutrients. (2020) 12:3109.
24. Hung KC, Hung CT, Poon YY, Wu SC, Chen KH, Chen JY, et al. The effect of cricoid pressure on tracheal intubation in adult patients: a systematic review and meta-analysis. Canad J Anaesth. (2021) 68:137–47.
25. Brok J, Thorlund K, Gluud C, Wetterslev J. Trial sequential analysis reveals insufficient information size and potentially false positive results in many meta-analyses. J Clin Epidemiol. (2008) 61:763–9. doi: 10.1016/j.jclinepi.2007.10.007
26. Brok J, Thorlund K, Wetterslev J, Gluud C. Apparently conclusive meta-analyses may be inconclusive–Trial sequential analysis adjustment of random error risk due to repetitive testing of accumulating data in apparently conclusive neonatal meta-analyses. Int J Epidemiol. (2009) 38:287–98. doi: 10.1093/ije/dyn188
27. Jiao XF, Lin XM, Ni XF, Li HL, Zhang C, Yang CS, et al. Volatile anesthetics versus total intravenous anesthesia in patients undergoing coronary artery bypass grafting: An updated meta-analysis and trial sequential analysis of randomized controlled trials. PLoS One. (2019) 14:e0224562. doi: 10.1371/journal.pone.0224562
28. Bettex DA, Schmidlin D, Chassot PG, Schmid ER. Intrathecal sufentanil-morphine shortens the duration of intubation and improves analgesia in fast-track cardiac surgery. Canad J Anaesth. (2002) 49:711–7. doi: 10.1007/BF03017451
29. Bhat I, Arya VK, Mandal B, Jayant A, Dutta V, Rana SS. Postoperative hemodynamics after high spinal block with or without intrathecal morphine in cardiac surgical patients: a randomized-controlled trial. Canad J Anaesth. (2021) 68:825–34.
30. Dhawan R, Daubenspeck D, Wroblewski KE, Harrison JH, McCrorey M, Balkhy HH, et al. Intrathecal morphine for analgesia in minimally invasive cardiac surgery: a randomized, placebo-controlled, double-blinded clinical trial. Anesthesiology. (2021) 135:864–76. doi: 10.1097/ALN.0000000000003963
31. dos Santos LM, Santos VCJ, Santos SRCJ, Malbouisson LMS, Carmona MJC. Intrathecal morphine plus general anesthesia in cardiac surgery: Effects on pulmonary function, postoperative analgesia, and plasma morphine concentration. Clinics. (2009) 64:279–85. doi: 10.1590/s1807-59322009000400003
32. Elgendy H, Helmy HAR. Intrathecal morphine improves hemodynamic parameters and analgesia in patients undergoing aortic valve replacement surgery: a prospective, double-blind, randomized trial. Pain Phys. (2017) 20:405–12.
33. Jacobsohn E, Lee TW, Amadeo RJ, Syslak PH, Debrouwere RG, Bell D, et al. Low-dose intrathecal morphine does not delay early extubation after cardiac surgery. Canad J Anaesth. (2005) 52:848–57.
34. Jara FM, Klush J, Kilaru V. Intrathecal morphine for off-pump coronary artery bypass patients. Heart Surg Forum. (2001) 4:57–60.
35. Lena P, Balarac N, Arnulf JJ, Bigeon JY, Tapia M, Bonnet F. Fast-track coronary artery bypass grafting surgery under general anesthesia with remifentanil and spinal analgesia with morphine and clonidine. J Cardiothorac Vasc Anesth. (2005) 19:49–53. doi: 10.1053/j.jvca.2004.11.009
36. Lena P, Balarac N, Arnulf JJ, Teboul J, Bonnet F. Intrathecal morphine and clonidine for coronary artery bypass grafting. Br J Anaesth. (2003) 90:300–3.
37. Lena P, Balarac N, Lena D, De La Chapelle A, Arnulf JJ, Mihoubi A, et al. Fast-Track anesthesia with remifentanil and spinal analgesia for cardiac surgery: the effect on pain control and quality of recovery. J Cardiothorac Vasc Anesth. (2008) 22:536–42. doi: 10.1053/j.jvca.2008.04.012
38. Mukherjee C, Koch E, Banusch J, Scholz M, Kaisers UX, Ender J. Intrathecal morphine is superior to intravenous PCA in patients undergoing minimally invasive cardiac surgery. Ann Cardiac Anaesth. (2012) 15:122–7. doi: 10.4103/0971-9784.95075
39. Roediger L, Joris J, Senard M, Larbuisson R, Canivet JL, Lamy M. The use of pre-operative intrathecal morphine for analgesia following coronary artery bypass surgery. Anaesthesia. (2006) 61:838–44. doi: 10.1111/j.1365-2044.2006.04744.x
40. Vanstrum GS, Bjornson KM, Ilko R. Postoperative effects of intrathecal morphine in coronary artery bypass surgery. Anesth Analg. (1988) 67:261–7.
41. Yapici D, Altunkan ZO, Atici S, Bilgin E, Doruk N, Cinel I, et al. Postoperative effects of low-dose intrathecal morphine in coronary artery bypass surgery. J Cardiac Surg. (2008) 23:140–5.
42. Charlesworth M, Klein A. Enhanced recovery after cardiac surgery. Anesthesiol Clin. (2022) 40:143–55.
43. Myles PS, Daly DJ, Djaiani G, Lee A, Cheng DC. A systematic review of the safety and effectiveness of fast-track cardiac anesthesia. Anesthesiology. (2003) 99:982–7. doi: 10.1097/00000542-200310000-00035
44. Roediger L, Larbuisson R, Lamy M. New approaches and old controversies to postoperative pain control following cardiac surgery. Eur J Anaesthesiol. (2006) 23:539–50.
45. Zangrillo A, Bignami E, Biondi-Zoccai GG, Covello RD, Monti G, D’Arpa MC, et al. Spinal analgesia in cardiac surgery: a meta-analysis of randomized controlled trials. J Cardiothorac Vasc Anesth. (2009) 23:813–21.
46. Harrogate SR, Cooper JA, Zawadka M, Anwar S. Seven-year follow-up of persistent postsurgical pain in cardiac surgery patients: A prospective observational study of prevalence and risk factors. Eur J Pain (London, England). (2021) 25:1829–38. doi: 10.1002/ejp.1794
47. Krakowski JC, Hallman MJ, Smeltz AM. Persistent pain after cardiac surgery: prevention and management. Semin Cardiothorac Vasc Anesth. (2021) 25:289–300.
48. Inouye SK, Westendorp RG, Saczynski JS. Delirium in elderly people. Lancet (London, England). (2014) 383:911–22.
50. Saczynski JS, Marcantonio ER, Quach L, Fong TG, Gross A, Inouye SK, et al. Cognitive trajectories after postoperative delirium. New Engl J Med. (2012) 367:30–9.
51. Cheng D. Anesthetic techniques and early extubation: does it matter? J Cardiothorac Vasc Anesth. (2000) 14:627–30. doi: 10.1053/jcan.2000.18677
52. Camp S, Stamou S, Stiegel R, Reames M, Skipper E, Madjarov J, et al. Can timing of tracheal extubation predict improved outcomes after cardiac surgery? HSR Proc Intensive Care Cardiovasc Anesth. (2009) 1:39.
53. Khandelwal N, Dale CR, Benkeser DC, Joffe AM, Yanez ND III, Treggiari MM. Variation in tracheal reintubations among patients undergoing cardiac surgery across Washington state hospitals. J Cardiothorac Vasc Anesth. (2015) 29:551–9. doi: 10.1053/j.jvca.2014.11.009
54. Wong P, Iqbal R, Light KP, Williams E, Hayward J. Head and neck surgery in a tertiary centre: predictors of difficult airway and anaesthetic management. Proc Singapore Healthc. (2016) 25:19–26.
55. Chamchad D, Horrow JC, Nachamchik L, Sutter FP, Samuels LE, Trace CL, et al. The impact of immediate extubation in the operating room after cardiac surgery on intensive care and hospital lengths of stay. J Cardiothorac Vasc Anesth. (2010) 24:780–4.
Keywords: analgesia, cardiac surgery, intrathecal morphine, tracheal extubation, respiratory depression
Citation: Chen I-W, Sun C-K, Ko C-C, Fu P-H, Teng I-C, Liu W-C, Lin C-M and Hung K-C (2022) Analgesic efficacy and risk of low-to-medium dose intrathecal morphine in patients undergoing cardiac surgery: An updated meta-analysis. Front. Med. 9:1017676. doi: 10.3389/fmed.2022.1017676
Received: 12 August 2022; Accepted: 23 September 2022;
Published: 05 October 2022.
Edited by:
Zhongheng Zhang, Sir Run Run Shaw Hospital, ChinaReviewed by:
Chong Lei, Fourth Military Medical University, ChinaKe Peng, The First Affiliated Hospital of Soochow University, China
Copyright © 2022 Chen, Sun, Ko, Fu, Teng, Liu, Lin and Hung. This is an open-access article distributed under the terms of the Creative Commons Attribution License (CC BY). The use, distribution or reproduction in other forums is permitted, provided the original author(s) and the copyright owner(s) are credited and that the original publication in this journal is cited, in accordance with accepted academic practice. No use, distribution or reproduction is permitted which does not comply with these terms.
*Correspondence: Kuo-Chuan Hung, ZWQxMDI2MDVAZ21haWwuY29t
†These authors share first authorship
‡These authors have contributed equally to this work