- 1Department of Environmental and Prevention Sciences, University of Ferrara, Ferrara, Italy
- 2Department of Public Health and Infectious Diseases, Sapienza University of Rome, Rome, Italy
- 3Department of Medical and Surgical Sciences, University of Bologna, Bologna, Italy
The addictive protection against SARS-CoV-2 reinfection conferred by vaccination, as compared to natural immunity alone, remains to be quantified. We thus carried out a meta-analysis to summarize the existing evidence on the association between SARS-CoV-2 vaccination and the risk of reinfection and disease. We searched MedLine, Scopus and preprint repositories up to July 31, 2022, to retrieve cohort or case-control studies comparing the risk of SARS-CoV-2 reinfection or severe/critical COVID-19 among vaccinated vs. unvaccinated subjects, recovered from a primary episode. Data were combined using a generic inverse-variance approach. Eighteen studies, enrolling 18,132,192 individuals, were included. As compared to the unvaccinated, vaccinated subjects showed a significantly lower likelihood of reinfection (summary Odds Ratio—OR: 0.47; 95% CI: 0.42–0.54). Notably, the results did not change up to 12 months of follow-up, by number of vaccine doses, in studies that adjusted for potential confounders, adopting different reinfection definitions, and with different predominant strains. Once reinfected, vaccinated subjects were also significantly less likely to develop a severe disease (OR: 0.45; 95% CI: 0.38–0.54). Although further studies on the long-term persistence of protection, under the challenge of the new circulating variants, are clearly needed, the present meta-analysis provides solid evidence of a stronger protection of hybrid vs. natural immunity, which may persist during Omicron waves and up to 12 months.
Introduction
Clarifying the frequency and predictors of SARS-CoV-2 reinfections is crucial to determine the course of the pandemic, and to optimize restriction and vaccination policies (1–3). Solid evidence is currently available on the frequency of reinfections after the emergence of the Omicron variant: a recent proportion meta-analysis including 15 million subjects recovered from a first infection estimated an overall reinfection rate of 3.3% in the first 3 months of Omicron predominance, likely increasing (2). However, the potential addictive protection conferred by hybrid immunity, generated by the combination of prior infection and vaccination, as compared to the sole natural immunity, remains to be fully disclosed (4, 5). A few population-based studies suggested that reinfection is less likely in vaccinated vs. unvaccinated subjects, but the magnitude of the association varied across studies, which differed for patients’ characteristics, exposure risk, type of SARS-CoV-2 vaccine received, definition of reinfection adopted, and extent of measured confounding (4, 6–8). In a recent meta-analysis, the overall reinfection rate among vaccinated subjects was quantified to be as low as 0.32%, as compared to 0.74% among previously infected, unvaccinated individuals, but these estimates were obtained from raw, unadjusted data (2). Additionally, only limited data are available on the time course of natural and hybrid immunity (9), and the extent of its waning, particularly due to Omicron infections, is not yet well characterized (4, 9).
We carried out a meta-analysis to summarize the existing evidence from adjusted analyses on the association between SARS-CoV-2 vaccination and reinfection, in subjects who recovered from a first episode of SARS-CoV-2 infection.
Methods
Bibliographic search, data extraction and quality assessment
The reporting of this meta-analysis was guided by the standards of the Preferred Reporting Items for Systematic Review and Meta-Analysis (PRISMA) 2020 Statement (10). We searched MedLine and Scopus databases, up to July 31, 2022, for studies evaluating the risk of SARS-CoV-2 reinfection (either asymptomatic or symptomatic and requiring hospital admission) among vaccinated subjects of all ages (with hybrid immunity resulting from a combination of natural and vaccine immunization), vs. unvaccinated subjects (with natural immunity only). Vaccinated subjects were defined as those receiving ≥ 1 dose of the COVID-19 vaccines currently approved ≥ 14 days before the reinfection. The following search strategy was adopted, without language restrictions: (coronavirus* or coronovirus* or coronavirinae* or Coronavirus* or Coronovirus* or Wuhan* or Hubei* or Huanan or “2019-nCoV” or 2019nCoV or nCoV2019 or “nCoV-2019” or “COVID-19” or COVID19 or “WN-CoV” or WNCoV or “HCoV-19” or HCoV19 or CoV or “2019 novel*” or Ncov or “n-cov” or “SARS-CoV-2” or “SARSCoV-2” or “SARSCoV2” or “SARS-CoV2” or SARSCov19 or “SARS-Cov19” or “SARSCov-19” or “SARS-Cov-19” or Ncovor or Ncorona* or Ncorono* or NcovWuhan* or NcovHubei* or NcovChina* or NcovChinese*) AND (reinfection* or re-infection* or second episode or recurrence* or recrudescence* or relapse* or RCOVID19) (2). The reference lists of reviews and retrieved articles was also screened, for additional pertinent papers (11). Given that several relevant clinical databases have been shared in public preprint repositories in the context of a public health emergency, we also searched for potential studies among those submitted in medRxiv.org. In case of re-analyses published from the same cohort, we extracted the data of the publication with the longer follow-up or, if the length of follow-up was identical, with the largest sample size.
Inclusion criteria were: (a) cohort or case-control design; (b) laboratory confirmation of SARS-CoV-2 initial episode through a positive reverse-transcriptase polymerase chain reaction (RT-PCR) test, and/or an initial positive serology investigated with the use of an anti-trimeric spike IgG enzyme-linked immunosorbent assay (ELISA) (12); (c) data available to compare SARS-CoV-2 reinfection by vaccination status in subjects who recovered from a primary infection; (d) explicit reinfection definition criteria. In accordance with CDC (12), a reinfection was defined by the presence of:
(a) two positive PCR samples detected ≥ 45 days apart with ≥ 1 negative RT-PCR test collected between the first and second episode (13), and/or confirmation of infection with two different phylogenetic strains by viral genomic sequencing;
(b) two positive PCR samples detected ≥ 45 days apart in subjects with a symptomatic second episode or in close contact with a laboratory-confirmed COVID-19 case (12);
(c) a positive PCR test ≥ 45 days after the first positive serology (detection of anti-S1 domain of spike protein IgG antibodies using an enzyme-linked immunosorbent assay—ELISA) (12, 14).
Each included article was independently evaluated by 2 reviewers (MEF, CAM), who extracted the main study characteristics and measures of effect. In case of discrepancies in data extraction, a third author was contacted (LM), and consensus achieved through discussion.
Individual study quality was evaluated using an adapted version of the Newcastle Ottawa Quality Assessment Scale, assessing the comparability across groups for confounding factors, the appropriateness of outcome assessment, length of follow-up and missing data handling and reporting (15).
Data analysis
The units of the meta-analysis were single comparisons of vaccinated vs. unvaccinated subjects in predicting (a) SARS-CoV-2 reinfection; (b) severe COVID-19 disease—requiring hospital admission with no use of an intensive care unit; (c) critical/lethal COVID-19 disease—requiring admission in an intensive care unit and/or causing death (2). The likelihood of each outcome was assessed: (a) using ≥ 45 days as the minimum time-lag between two positive episodes; (b) adopting a more stringent time-lag of 90 days (2); (c) including only studies with adjusted estimates. When data were available, we also performed several additional meta-analyses stratified by: (d) number of vaccine doses (“fully vaccinated” subjects—those receiving ≥ 2 doses of mRNA-1273, BNT162b2, ChAdOx1 nCoV-19, BBV152, BBIBP-CorV, Gam-COVID-Vac, CoronaVac, or 1 dose of JNJ-78436735 ≥ 14 days before reinfection—or “partially vaccinated” subjects—those receiving 1 dose of mRNA-1273, BNT162b2, ChAdOx1 nCoV-19, BBV152, BBIBP-CorV, Gam-COVID-Vac, or CoronaVac ≥ 14 days before reinfection—vs. unvaccinated) (13). When data were available, we also extracted separate estimates for those who received 3 doses of mRNA-1273, BNT162b2, ChAdOx1 nCoV-19, BBV152, BBIBP-CorV, Gam-COVID-Vac, or CoronaVac vaccines (“boosted subjects”); (e) time between first episode and reinfection (<6 vs. ≥ 6 months); (f) dominant viral strain (Delta or Omicron); (g) exposure risk (healthcare workers or general population); (h) study design (cohort or case-control).
Data were combined using a random-effect generic inverse variance approach (16, 17), in order to account for between-study heterogeneity (18). If a study reported the results of different multivariable models, the most stringently controlled estimates (those from the model adjusting for more factors) were extracted. If different models controlled for the same number of covariates, the model containing the most clinically meaningful covariates was used for the analysis (19). When a study only reported separate estimates by vaccine dose, the overall estimate of risk was computed from the separate relative risks using the fixed-effect model for generic inverse-variance outcomes (19).
Between-study heterogeneity was quantified using the I2 statistic. Potential publication bias was assessed graphically, using funnel plots [displaying the Odds Ratios—ORs from individual comparisons vs. their precision (1/SE)], and formally, using Egger’s regression asymmetry test (16).
All meta-analyses were performed using RevMan software, version 5.3 [The Cochrane Collaboration, (20)].
Results
Of the 3,470 papers initially retrieved, seven case-control (4, 21–26) and 11 cohort studies (6–8, 27–34) were included in the analyses (Supplementary Figure 1 and Supplementary Table 1). Three studies contributed with two dataset (24, 27, 31), as the same publication provided separate data for healthcare workers and the general population (31), and for Delta and Omicron waves (24, 27): this led to a total of 21 datasets that were included in the analyses (Table 1).
Six studies were carried out in Europe (4, 6, 8, 24, 27–29), six in the USA (21, 22, 24–26, 31), five in Asia (7, 30, 32–34) and one in South Africa (23). Thirteen studies evaluated the general population (4, 6–8, 21, 22, 24–28, 30, 31), and six assessed the healthcare workers (23, 29, 31–34). In most studies, the analyses were adjusted for age, gender, and comorbidities, as a minimum set of potential confounders of the association between vaccination status and reinfections (4, 6–8, 21, 23, 26, 28–32, 34).
The mean age of the participants ranged from 15 to 46 years, and the mean follow-up ranged from a minimum of 60 up to 340 days. In 13 studies (4, 7, 21–26, 29, 31–34) the minimum time-lag between infection and reinfection was set at 90 days, and only three (28, 29, 34) strictly followed the CDC criteria to define a reinfection (≥1 intermediate negative PCR and/or viral genomic sequencing) (12). Most reinfections were reported during the Delta (7, 8, 22, 24, 25, 27, 31–33) and the Omicron waves (4, 6, 23, 24, 27, 28, 30).
The methodological characteristics of the included studies are summarized in Table 2: the selection of the cohort of patients, the ascertainment of the exposure, and the evaluation of the comparability of subjects were adequate in all studies, while 15 out of 18 adequately addressed the items pertaining to outcome assessment and follow-up (length and missing data).
Twenty-one datasets including a total of 18,132,192 individuals were included in the overall meta-analysis comparing the risk of SARS-CoV-2 reinfection in vaccinated vs. unvaccinated subjects (Table 3) (4, 6–8, 21–34). In 20 out of 21 datasets, the vaccinated subjects were significantly less likely to be reinfected, with a summary OR of 0.47 (95% confidence interval—CI – 0.42–0.54) (Figure 1). When the only study reporting a significantly higher risk among vaccinated subjects (and no data on underlying comorbidities) was excluded (6), the estimates were virtually identical (OR: 0.45; 95% CI: 0.39–0.50). Also, the results did not substantially change after the exclusion of the three studies with unadjusted estimates (OR: 0.47; 95% CI: 0.39–0.56) (25, 27, 33), and when only the 17 datasets with a more conservative time-lag of 90 days were considered (OR: 0.47; 95% CI: 0.41–0.54) (4, 7, 21, 23, 24, 26, 29, 31–34).
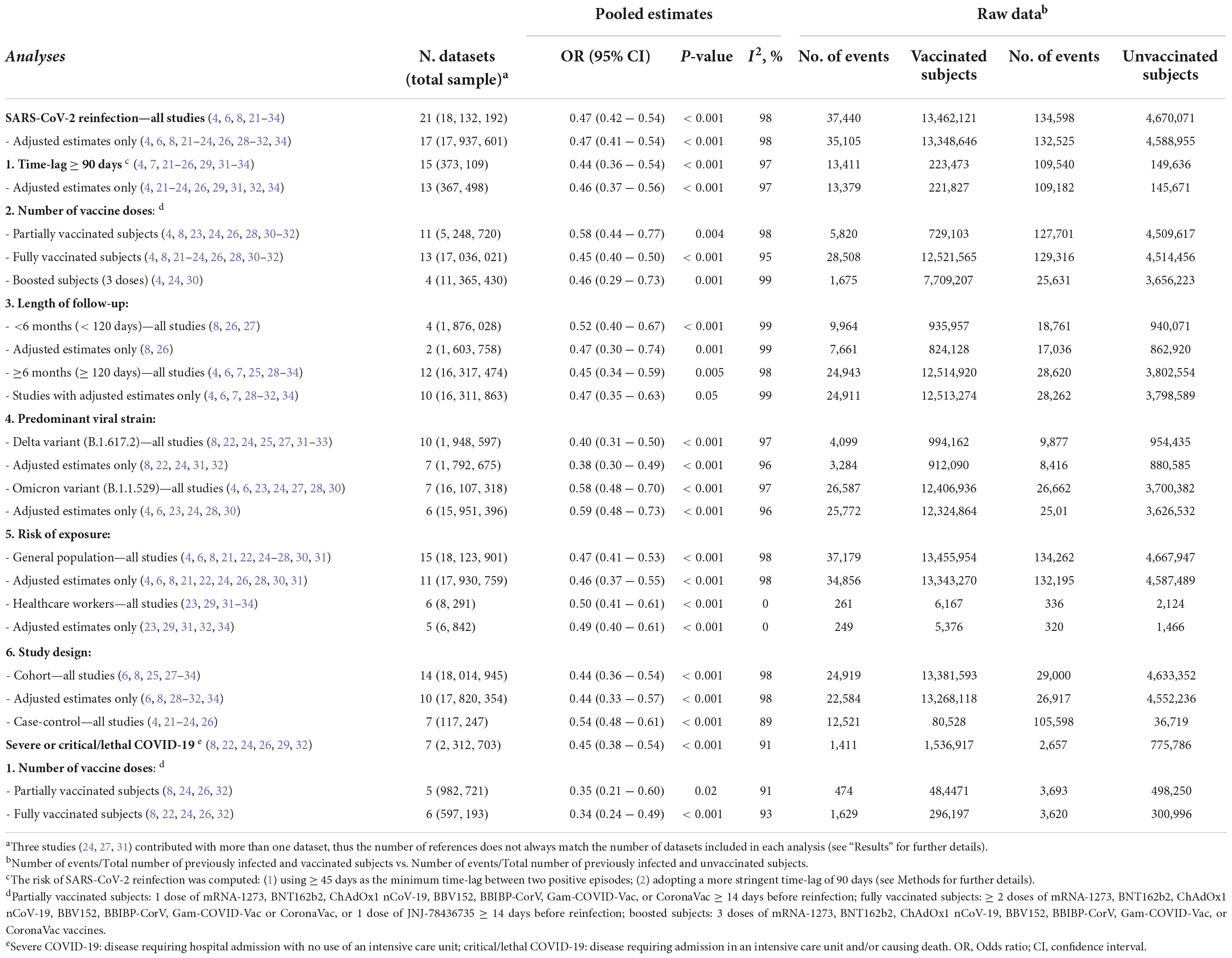
Table 3. Risk of SARS-CoV-2 reinfection and severe/critical COVID-19 among vaccinated vs. unvaccinated subjects, overall, and stratified by definition of reinfection, number of vaccine doses, length of follow-up, predominant strain, study design and risk exposure.
When the analyses were stratified by number of doses, the summary OR of reinfection was lower among fully vaccinated than partially vaccinated subjects (summary OR 0.45 and 0.58, respectively). The confidence intervals, however, largely overlapped. In the analyses restricted to the subjects who received three doses (a booster dose), the summary OR was comparable to that of the fully vaccinated individuals (OR: 0.46; 95% CI: 0.29–0.73). As shown in Table 3, the association between vaccination and reinfection did not show a substantial variation by length of follow-up: the summary OR of the studies with a follow-up shorter than 6 months (OR: 0.52; 95% CI: 0.40–0.67) was comparable with the OR (0.45; 95% CI: 0.34–0.59) of the studies with a longer follow-up (up to 340 days).
The likelihood of a reinfection remained significantly lower among vaccinated subjects both in the studies that were carried out during Delta predominance (summary OR: 0.40; 95% CI: 0.31–0.50) (7, 8, 19, 22–24, 27–29) and during Omicron predominance (OR: 0.58; 95% CI: 0.48–0.60) (2, 4, 6, 23, 24, 27, 30). Again, in the analyses stratified by risk of exposure (general population or healthcare workers) and by study design (cohort or case-control) the likelihood of reinfection was comparably, significantly lower among vaccinated subjects, with summary ORs ranging from 0.44 to 0.54, and overlapping confidence intervals.
The Egger test was not significant (p = 0.3), and the funnel plot displaying the ORs of the individual comparisons vs. the logarithm of their SE (precision) did not show asymmetry, suggesting the absence of publication bias (Supplementary Figure 2).
A total of seven datasets and 2,312,703 individuals provided specific data and were included in the meta-analysis comparing the risk of severe/lethal COVID-19 of the vaccinated vs. the unvaccinated subjects (8, 22, 24, 26, 29, 32). Compared with the unvaccinated, those receiving ≥ 1 dose were significantly less likely to develop a severe disease, once reinfected (OR: 0.45; 95% CI: 0.38–0.54—Table 3 and Figure 2). The risk remained comparably and significantly lower when only the subset of studies evaluating partial vaccination (OR: 0.35; 95% CI: 0.21–0.60) or those evaluating full vaccination (OR: 0.34; 95% CI: 0.24–0.49) vs. no vaccination, were included.
Discussion
This meta-analysis, which included the data of more than 18 million previously infected and recovered subjects, has two main findings. First, as compared to natural immunity alone, the addition of vaccination approximately halved the odds of severe COVID-19, and the degree of protection was similar after a single or multiple doses. Second, the likelihood of reinfection was also reduced by approximately 50% among the vaccinated, and this finding was consistent in all stratified analyses, either extracting estimates adjusted for potential confounders or unadjusted, with follow-ups shorter or longer than 6 months, adopting different reinfection definitions, in both case-control and cohort studies, in the general population and healthcare workers alone, after a single or multiple vaccine doses, and irrespective of the predominant strain.
Preliminary evidence suggested that the protection conferred by hybrid immunity against reinfection was similar, or only marginally better, than the infection-induced or vaccine-induced immunity alone (5, 35). More recently, however, a proportion meta-analysis including 15 million previously infected and recovered individuals reported markedly lower rates of reinfection among vaccinated vs. unvaccinated subjects (0.32% vs. 0.74%), but these findings were based upon raw data and needed confirmation from adjusted estimates (2). The present meta-analysis expanded the previous and included 15 studies that adjusted the analyses for age, gender, comorbidities, and other potential confounders, providing solid evidence of a stronger protection of hybrid vs. natural immunity, which may persist during Omicron waves and up to 12 months.
Indeed, concerning the waning of the immunity, a 20% decline in the effectiveness of vaccination against first infection after 6 months was first showed in a meta-analysis including studies up to December 2021 (36). Then, evidence of waning protection both with hybrid and natural immunity 4 months after immunization was reported in some large prospective studies, which showed corresponding upward trends in reinfection absolute rates during time (5, 8, 9). In the present meta-analysis, the reinfection rates of the cohort studies with follow-up longer than 6 months were not distinctly higher (0.17 and 0.65 × 100 individuals in vaccinated and unvaccinated subjects, respectively), as compared to those with short follow-up (0.39 and 0.50 × 100 individuals in vaccinated and unvaccinated subjects, respectively). Additionally, we did not observe a substantial reduction of the protection when the follow-up lasted 6–11 months: pooling the 12 datasets with a longer follow-up, the odds of reinfection were approximately 50% lower among the vaccinated. Inevitably, this information remains preliminary, as it is based upon studies in which the follow-up lasted up to 12 months, and the use of viral genomic sequencing was uneven.
These findings may offer a contribution to help planning tailored immunization strategies for previously infected subjects: if, on one side, the marked increase in the absolute number of reinfections with time is concerning, the significantly lower relative risk still observed among vaccinated subjects may be reassuring, thus vaccinating also this population may definitely play a role to control the pandemic (4). In this scenario, the strong protective effect exerted by a single dose (if confirmed during longer follow-up and toward different strains) might be taken into account when designing tailored vaccination schedules directed to lower-priority groups (4, 5). It should be also considered, however, that the degree of additional protection specifically conferred by further boosters (three or more doses) still remains uncertain, as our stratified meta-analyses did not show a clear benefit of a 3- vs. a 2-dose schedule.
The second main finding of the present meta-analysis was the significant reduction of the risk of hospitalization due to severe COVID-19 that was observed among the vaccinated subjects, either receiving one or more doses. This was crucial, as the primary aim of COVID-19 vaccination is to reduce the pressure on the healthcare systems preventing severe disease and hospitalization (37). Unfortunately, however, most of the studies included in the meta-analyses of this outcome were carried out before the emergence of Omicron strain. Therefore, this finding requires confirmation from more recent data with longer follow-up, as the large increase in the number of reinfections during the Omicron wave, and in turn the consequences on the healthcare systems still needs to be carefully evaluated.
In the first phases of the pandemic, there was uncertainty on the criteria to define a reinfection, especially on the time interval between the first and second episodes, and most initial studies defined a reinfection as a new PCR test occurring ≥ 90 days after complete resolution of the first infection (4, 7, 21–26, 29, 31–34). However, the CDC later expanded the definition, including also the subjects with COVID-19-like symptoms and detection of SARS-CoV-2 RNA ≥ 45 days since first infection (12). In the present analysis, we did not find substantial differences when a 90-day or a 45-day cutoff was adopted, suggesting that a low proportion of reinfections was missed using the longer threshold. Indeed, a recent cohort study reported a mean time between the first and second infection of 349 days, with less than 15% of the reinfections occurring in the first 6 months since the first episode (28).
Some limitations must be considered when interpreting the present findings. First, most meta-analyses showed an intermediate-to-high level of heterogeneity. However, a certain degree of heterogeneity across studies was inevitable, given the large variation in terms of setting and baseline patients characteristics. Also, when the analyses were repeated adopting a fixed approach, none of the results substantially differed (except for CIs, which were typically tighter). Second, although most studies provided analyses at least adjusted for age, gender, and several underlying comorbidities, some extent of residual confounding cannot be completely ruled out, as for any observational study (38). Third, the risk of reinfection could have been overestimated in several of the included studies adopting less stringent criteria to define a reinfection (2). Conversely, if previously infected people tended to seek fewer testing due to their presumed acquired natural immunity, the reinfection rate could have been underestimated (4). A sensitivity analysis based upon the average number of PCR tests as a proxy of health-seeking behavior would have increased the precision of our estimates (2), but these data were unfortunately not available. Fourth, it might have been interesting to evaluate if the results differed according to the sequence of events, whether vaccination was administered before or after the first infection. Unfortunately, however, the exact timeline of events could be determined only in two studies (4, 31), in which all the infections occurred before the start of the vaccination campaign.
Acknowledging these caveats, this meta-analysis showed that, among the subjects that recovered from a first SARS-CoV-2 infection, vaccination was associated with a significant and substantial reduction of the risk of both reinfection and severe COVID-19. This finding was confirmed when the analyses were adjusted for potential confounders, up to 12 months of follow-up, and after any vaccine dose. Further studies on the long-term persistence of protection, and assessing the reinfection and hospitalization rates under the challenge of the new circulating variants, are strongly warranted.
Data availability statement
The data presented in this study are available upon reasonable request from the corresponding author.
Author contributions
MF and LM: concept and design and statistical analysis. MF, CA, VB, and ER: acquisition, analysis, or interpretation of data. MF, CA, and LM: drafting of the manuscript. CD, PV, and LM: critical revision of the manuscript for important intellectual content. PV and LM: supervision. LM: full access to all the data in the study and take responsibility for the integrity of the data and the accuracy of the data analysis. All authors contributed to the article and approved the submitted version.
Conflict of interest
The authors declare that the research was conducted in the absence of any commercial or financial relationships that could be construed as a potential conflict of interest.
Publisher’s note
All claims expressed in this article are solely those of the authors and do not necessarily represent those of their affiliated organizations, or those of the publisher, the editors and the reviewers. Any product that may be evaluated in this article, or claim that may be made by its manufacturer, is not guaranteed or endorsed by the publisher.
Supplementary material
The Supplementary Material for this article can be found online at: https://www.frontiersin.org/articles/10.3389/fmed.2022.1023507/full#supplementary-material
References
1. European Centre for Disease Prevention and Control. Reinfection with SARS-CoV-2: Considerations for Public Health Response. Stockholm: ECDC (2020).
2. Flacco ME, Acuti Martellucci C, Baccolini V, De Vito C, Renzi E, Villari P, et al. Risk of reinfection and disease after SARS-CoV-2 primary infection: meta-analysis. Eur J Clin Invest. (2022) 52:e13845. doi: 10.1111/eci.13845
3. Yahav D, Yelin D, Eckerle I, Eberhardt CS, Wang J, Cao B, et al. Definitions for coronavirus disease 2019 reinfection, relapse and PCR re-positivity. Clin Microbiol Infect. (2021) 27:315–8. doi: 10.1016/j.cmi.2020.11.028
4. Medic S, Anastassopoulou C, Lozanov-Crvenkovic Z, Vukovic V, Dragnic N, Petrovic V, et al. Risk and severity of SARS-CoV-2 reinfections during 2020-2022 in Vojvodina, Serbia: a population-level observational study. Lancet Reg Health Eur. (2022) 20:100453. doi: 10.1016/j.lanepe.2022.100453
5. World Health Organization. Interim Statement on Hybrid Immunity and Increasing Population Seroprevalence Rates. Geneva: World Health Organization (2022).
6. Eythorsson E, Runolfsdottir HL, Ingvarsson RF, Sigurdsson MI, Palsson R. Rate of SARS-CoV-2 reinfection during an omicron wave in Iceland. JAMA Netw Open. (2022) 5:e2225320. doi: 10.1001/jamanetworkopen.2022.25320
7. Hammerman A, Sergienko R, Friger M, Beckenstein T, Peretz A, Netzer D, et al. Effectiveness of the BNT162b2 vaccine after recovery from Covid-19. N Engl J Med. (2022) 386:1221–9. doi: 10.1056/NEJMoa2119497
8. Nordstrom P, Ballin M, Nordstrom A. Risk of SARS-CoV-2 reinfection and COVID-19 hospitalisation in individuals with natural and hybrid immunity: a retrospective, total population cohort study in Sweden. Lancet Infect Dis. (2022) 22:781–90. doi: 10.1016/S1473-3099(22)00143-8
9. Goldberg Y, Mandel M, Bar-On YM, Bodenheimer O, Freedman LS, Ash N, et al. Protection and waning of natural and hybrid immunity to SARS-CoV-2. N Engl J Med. (2022) 386:2201–12. doi: 10.1056/NEJMoa2118946
10. Page MJ, McKenzie JE, Bossuyt PM, Boutron I, Hoffmann TC, Mulrow CD, et al. The PRISMA 2020 statement: an updated guideline for reporting systematic reviews. BMJ. (2021) 372:n71. doi: 10.1136/bmj.n71
11. Boccia S, Rothman KJ, Panic N, Flacco ME, Rosso A, Pastorino R, et al. Registration practices for observational studies on ClinicalTrials.gov indicated low adherence. J Clin Epidemiol. (2016) 70:176–82. doi: 10.1016/j.jclinepi.2015.09.009
12. CDC. Common Investigation Protocol for Investigating Suspected SARS-CoV-2 Reinfection. Atlanta, GA: CDC (2020).
13. Jain VK, Iyengar K, Garg R, Vaishya R. Elucidating reasons of COVID-19 re-infection and its management strategies. Diabetes Metab Syndr. (2021) 15:1001–6. doi: 10.1016/j.dsx.2021.05.008
14. Lumley SF, O’Donnell D, Stoesser NE, Matthews PC, Howarth A, Hatch SB, et al. Antibody status and incidence of SARS-CoV-2 infection in health care workers. N Engl J Med. (2021) 384:533–40. doi: 10.1056/NEJMoa2034545
15. Wells G, Shea B, O’Connell D, Peterson J, Welch V, Losos M, et al. The Newcastle-Ottawa Scale (NOS) for Assessing the Quality of Nonrandomised Studies in Meta-Analyses. Ottawa ON: Ottawa Hospital Research Institute (2005).
16. Deeks JJ, Higgins JPT, Altman DG. Cochrane Handbook for Systematic Reviews of Interventions Version 5.1.0. London: The Cochrane Collaboration (2011).
17. Manzoli L, Flacco ME, Boccia S, D’Andrea E, Panic N, Marzuillo C, et al. Generic versus brand-name drugs used in cardiovascular diseases. Eur J Epidemiol. (2015) 31:351–68. doi: 10.1007/s10654-015-0104-8
18. DerSimonian R, Laird N. Meta-analysis in clinical trials. Control Clin Trials. (1986) 7:177–88. doi: 10.1016/0197-2456(86)90046-2
19. Flacco ME, Acuti Martellucci C, Bravi F, Parruti G, Cappadona R, Mascitelli A, et al. Treatment with ACE inhibitors or ARBs and risk of severe/lethal COVID-19: a meta-analysis. Heart. (2020) 106:1519–24. doi: 10.1136/heartjnl-2020-317336
20. Review Manager Web. Version 5.3. The Cochrane Collaboration. (2018). Available online at: revman.cochrane.org
21. Cavanaugh AM, Spicer KB, Thoroughman D, Glick C, Winter K. Reduced risk of reinfection with SARS-CoV-2 after COVID-19 vaccination – Kentucky, May-June 2021. MMWR Morb Mortal Wkly Rep. (2021) 70:1081–3. doi: 10.15585/mmwr.mm7032e1
22. Levin-Rector, A, Firestein L, McGibbon E, Sell J, Lim S, Lee EH, et al. Reduced odds of SARS-CoV-2 reinfection after vaccination among New York city adults, July-November 2021. Clin Infect Dis. (2022) ciac380. doi: 10.1101/2021.12.09.21267203 [Epub ahead of print].
23. Nunes MC, Mbotwe-Sibanda S, Baillie VL, Kwatra G, Aguas R, Madhi SA, et al. SARS-CoV-2 Omicron symptomatic infections in previously infected or vaccinated South African Healthcare workers. Vaccines. (2022) 10:459. doi: 10.3390/vaccines10030459
24. Plumb ID, Feldstein LR, Barkley E, Posner AB, Bregman HS, Hagen MB, et al. Effectiveness of COVID-19 mRNA vaccination in preventing COVID-19-Associated hospitalization among adults with previous SARS-CoV-2 infection – United States, June 2021-February 2022. MMWR Morb Mortal Wkly Rep. (2022) 71:549–55. doi: 10.15585/mmwr.mm7115e2
25. Spicer KB, Glick C, Cavanaugh AM, Thoroughman D. Association of severe acute respiratory syndrome Coronavirus 2 vaccination or a prior positive test result in adolescents during the delta variant surge in Kentucky. J Pediatr. (2022) 248:119–21. doi: 10.1016/j.jpeds.2022.05.034
26. Cerqueira-Silva T, Andrews JR, Boaventura VS, Ranzani OT, de Araujo Oliveira V, Paixao ES, et al. Effectiveness of CoronaVac, ChAdOx1 nCoV-19, BNT162b2, and Ad26.COV2.S among individuals with previous SARS-CoV-2 infection in Brazil: a test-negative, case-control study. Lancet Infect Dis. (2022) 22:791–801. doi: 10.1016/S1473-3099(22)00140-2
27. Bager P, Wohlfahrt J, Bhatt S, Stegger M, Legarth R, Moller CH, et al. Risk of hospitalisation associated with infection with SARS-CoV-2 omicron variant versus delta variant in Denmark: an observational cohort study. Lancet Infect Dis. (2022) 22:967–76.
28. Flacco ME, Soldato G, Acuti Martellucci C, Di Martino G, Carota R, Caponetti A, et al. Risk of SARS-CoV-2 reinfection 18 months after primary infection: population-level observational study. Front Public Health. (2022) 10:884121. doi: 10.3389/fpubh.2022.884121
29. Hall VJ, Foulkes S, Charlett A, Atti A, Monk EJM, Simmons R, et al. SARS-CoV-2 infection rates of antibody-positive compared with antibody-negative health-care workers in England: a large, multicentre, prospective cohort study (SIREN). Lancet. (2021) 397:1459–69.
30. Jang EJ, Choe YJ, Yun GW, Wang S, Cho UJ, Yi S, et al. Reinfection with SARS-CoV-2 in general population, South Korea; nationwide retrospective cohort study. J Med Virol. (2022) 94:5589–92. doi: 10.1002/jmv.28026
31. Lewis N, Chambers LC, Chu HT, Fortnam T, De Vito R, Gargano LM, et al. Effectiveness associated with vaccination after COVID-19 recovery in preventing reinfection. JAMA Netw Open. (2022) 5:e2223917. doi: 10.1001/jamanetworkopen.2022.23917
32. Malhotra S, Mani K, Lodha R, Bakhshi S, Mathur VP, Gupta P, et al. SARS-CoV-2 reinfection rate and estimated effectiveness of the inactivated whole virion vaccine BBV152 against reinfection among health care workers in New Delhi, India. JAMA Netw Open. (2022) 5:e2142210. doi: 10.1001/jamanetworkopen.2021.42210
33. Murugesan M, Mathews P, Paul H, Karthik R, Mammen JJ, Rupali P. Protective effect conferred by prior infection and vaccination on COVID-19 in a healthcare worker cohort in South India. PLoS One. (2022) 17:e0268797. doi: 10.1371/journal.pone.0268797
34. Nisha B, Dakshinamoorthy K, Padmanaban P, Jain T, Neelavarnan M. Infection, reinfection, and postvaccination incidence of SARS-CoV-2 and associated risks in healthcare workers in Tamil Nadu: a retrospective cohort study. J Family Community Med. (2022) 29:49–55. doi: 10.4103/jfcm.jfcm_318_21
35. Krammer F. Correlates of protection from SARS-CoV-2 infection. Lancet. (2021) 397:1421–3. doi: 10.1016/S0140-6736(21)00782-0
36. Feikin DR, Higdon MM, Abu-Raddad LJ, Andrews N, Araos R, Goldberg Y, et al. Duration of effectiveness of vaccines against SARS-CoV-2 infection and COVID-19 disease: results of a systematic review and meta-regression. Lancet. (2022) 399:924–44. doi: 10.1016/S0140-6736(22)00152-0
37. European Centre for Disease Prevention and Control. Objectives of Vaccination Strategies against COVID-19. Stockholm: ECDC (2021).
Keywords: SARS-CoV-2, COVID-19, vaccination, meta-analysis, Omicron (B.1.1.529), reinfection
Citation: Flacco ME, Acuti Martellucci C, Baccolini V, De Vito C, Renzi E, Villari P and Manzoli L (2022) COVID-19 vaccines reduce the risk of SARS-CoV-2 reinfection and hospitalization: Meta-analysis. Front. Med. 9:1023507. doi: 10.3389/fmed.2022.1023507
Received: 19 August 2022; Accepted: 24 October 2022;
Published: 09 November 2022.
Edited by:
Ying Zhang, The University of Sydney, AustraliaReviewed by:
Oana Sandulescu, Carol Davila University of Medicine and Pharmacy, RomaniaMatteo Riccò, IRCCS Local Health Authority of Reggio Emilia, Italy
Copyright © 2022 Flacco, Acuti Martellucci, Baccolini, De Vito, Renzi, Villari and Manzoli. This is an open-access article distributed under the terms of the Creative Commons Attribution License (CC BY). The use, distribution or reproduction in other forums is permitted, provided the original author(s) and the copyright owner(s) are credited and that the original publication in this journal is cited, in accordance with accepted academic practice. No use, distribution or reproduction is permitted which does not comply with these terms.
*Correspondence: Lamberto Manzoli, bG1hbnpvbGlAcG9zdC5oYXJ2YXJkLmVkdQ==
†These authors have contributed equally to this work