- 1Department of Obstetrics and Gynecology, The Second XIANGYA Hospital of Central South University, Changsha, China
- 2Laboratory Medicine Center, Zhu Zhou Hospital Affiliated to Xiangya School of Medicine, Central South University (CSU), Zhuzhou, China
- 3Department of Obstetrics and Gynecology, Zhu Zhou Hospital Affiliated to Xiangya School of Medicine, CSU, Zhuzhou, China
Globally, cervical cancer (CC) is the most common malignant tumor of the female reproductive system and its incidence is only second after breast cancer. Although screening and advanced treatment strategies have improved the rates of survival, some patients with CC still die due to metastasis and drug resistance. It is considered that cancer is driven by somatic mutations, such as single nucleotide, small insertions/deletions, copy number, and structural variations, as well as epigenetic changes. Previous studies have shown that cervical intraepithelial neoplasia is associated with copy number variants (CNVs) and/or mutations in cancer-related genes. Further, CC is also related to genetic mutations. The present study analyzed the data on somatic mutations of cervical squamous cell carcinoma (CESC) in the Cancer Genome Atlas database. It was evident that the Apolipoprotein B mRNA editing enzyme-catalyzed polypeptide-like (APOBEC)-related mutation of the FLG gene can upregulate the expression of the JUN gene and ultimately lead to poor prognosis for patients with CC. Therefore, the findings of the current study provide a new direction for future treatment of CC.
Introduction
Cervical cancer (CC) is the most common malignant tumor of the female reproductive system in the world and its incidence is only second after breast cancer (1). Cervical squamous cell carcinoma (CESC) is the primary subtype of CC, accounting for between 80 and 85% of all its diagnoses (2). According to the 2020 Global Cancer Statistics, CC ranks fourth in morbidity and mortality among women with cancer whereby there were 604,127 new cases and 41,831 deaths in the year (3). The highest global prevalence of CC occurs in developing countries (87%), of which it is the foremost form of gynecological cancer (4) whereas China accounts for 12% of the global incidence of CC and 11% of deaths (5). The CC screening program aims to reduce the incidence and mortality of the cancer (6). CC screening can detect abnormalities in the cells of the cervix, such as precancerous lesions, and early stages of CC (7). Screening for cervical cytology and human papilloma virus (HPV) is the most common method of CC screening (8). Although screening and advanced treatment strategies have improved the survival rates of patients with CC, some patients still die due to metastasis and drug resistance (9).
Copy number variants (CNVs) are genome rearrangements that lead to different copy numbers of genome segments, such as deletions, amplifications, and unbalanced translocations (10). Genomic structural variants are prevalent in the human genome (11) and represent an important source of genotype and phenotypic variation. CNVs usually play a functional role in regulating gene expression (12). Somatic mutations and other forms of genomic instabilities, such as single nucleotide variations (SNVs), small insertions/deletions (Indels), CNVs, structural variations, and epigenetic changes, can cause cancer (13).
Recently, the use of next-generation sequencing (NGS) has dramatically increased the number of somatic mutations detected in human cancers (14). For DNA-based analysis, Sanger sequencing remains the gold standard for the identification of somatic mutations (15). Currently, genomic studies have produced a wealth of data, including gene expression, copy number variation, and single nucleotide polymorphisms (SNPs) (16). The Cancer Genome Atlas (TCGA) is a large public database that includes 33 cancer types and matched clinical data (17). It provides a wide range of data types for patients with cancer, such as copy number variation, mRNA expression, and methylation.
The persistent infection of HPV has been recognized as an important cause of CC and precancerous lesions (18). However, only 10% of women infected with high-risk HPV develop precancerous lesions with less than 1% of the women progressing to CC (19). Although the occurrence and development of CC requires infection with certain types of HPV, other multiple factors are also involved but are not fully understood (20). Studies have shown that cervical intraepithelial neoplasia (CIN) is commonly associated with CNVs and/or mutations, such as KRAS and BRAF in cancer-related genes (21). Similarly, CC is also associated with genetic mutations (22, 23).
Recently, the relationship between vaginal microbiology and CC has attracted attention of many researchers (24). Viral infections can induce the expression of Apolipoprotein B mRNA editing enzyme-catalyzed polypeptide-like (APOBEC) enzymes (25). Further, in addition to attacking viral genetic material, APOBEC enzymes can also attack human ssDNA, resulting in mutations that may contribute to cancer development (26). A previous study has reported that APOBEC-mediated mutations are the most significant contributor of the occurrence and development of CC (27). The present study analyzed the somatic mutation data of CESC in the TCGA database to evaluate the potential of APOBEC-associated mutated genes and target genes that are closely related to CC as well as provide new ideas for the future treatment of CC. The workflow of the study is shown in Figure 1.
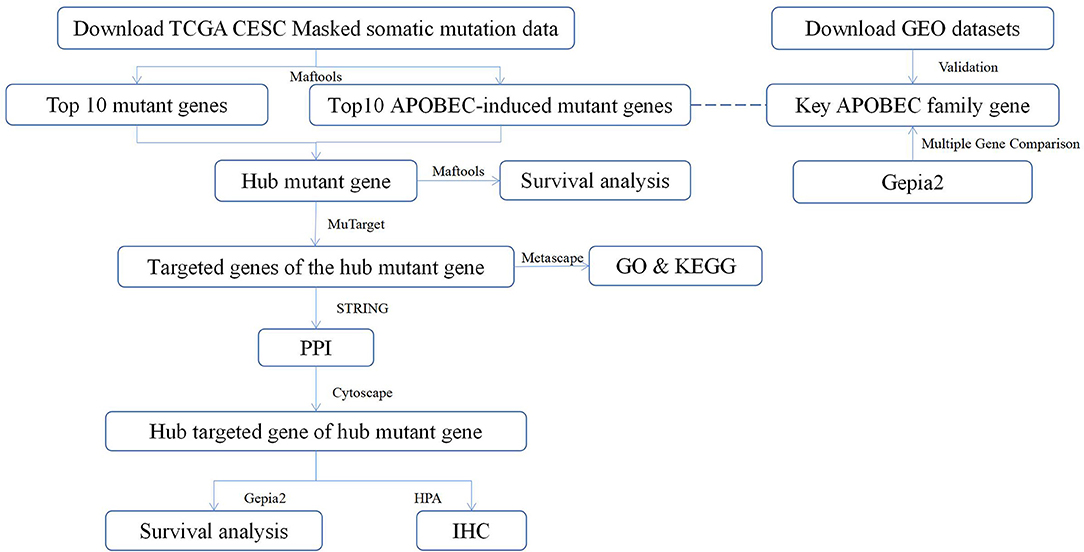
Figure 1. The workflow of the study. TCGA, The Cancer Genome Atlas; CESC, cervical squamous cell carcinoma; Maftools, a package in R language; MuTarget, an online platform, which could help us to identify the gene(s) showing altered expression in samples harboring a mutated input gene or identify mutations resulting in the expression change in the input gene (https://mutarget.com/); GO, gene oncology; KEGG, Kyoto Encyclopedia of Genes and Genomes; STRING, functional protein association networks platform (https://cn.string-db.org); PPI, protein–protein interaction; Cytoscape, an open-source software platform for dynamic, graphical visualization, manipulation, and analysis of networks; Gepia2, an online analysis platform based on TCGA database (http://gepia.cancer-pku.cn/); HPA, human protein atlas (https://www.proteinatlas.org) GEO, Gene Expression Omnibus; IHC, Immunohistochemistry.
Materials and Methods
Date Download and Procession
The TCGA is the largest database of genetic information on cancer (https://portal.gdc.cancer.gov/). Masked somatic mutations and associated clinicopathological data of patients with CC were downloaded from the TCGA database on 6 April 2021 for use in the current study. The samples included 302 cancer tissues and 8 adjacent tissue samples. The data were obtained, analyzed, and visualized using the “maftools” in the R software package (28). The Gene Expression Omnibus (GEO) datasets (GSE63514, GES6791, and GSE63678) obtained from the GEO database (http://www.ncbi.nlm.nih.gov/geo) were used as validation datasets for APOBEC family genes. These three datasets contain 24 normal cervix and 28 CC tissues; 5 normal cervix and 5 CC tissues; 8 normal cervix and 20 CC tissues, respectively.
Identification of APOBEC-Induced Mutant Genes and Hub Mutant Genes
APOBEC-induced mutations are more common in solid tumors and are mainly associated with C>T transition events occurring in the TCW motif. Therefore, the present study analyzed the differences in mutation patterns between APOBEC-enriched and non-APOBEC-enriched samples using plotApobecDiff in the maftools package. Then, we overlapped the top 10 mutant genes and the top 10 APOBEC-induced mutant genes in the samples.
Relationship Between Hub Mutant Genes and Survival
In the present study, Kaplan–Meier analysis was performed using the mafSurvive function in the Maftools package to investigate the prognostic value of hub mutant genes in CC.
Identifying the Targeted Genes of Hub Mutant Genes
MuTarget (https://mutarget.com/) is an online platform, which can help in the identification of gene(s) showing altered expression in samples harboring a mutated input gene or mutations resulting in expression change in the input gene (29). The platform was used to identify the targeted genes associated with hub mutant genes whereby all target genes with p < 0.05 and mean fold change >1.44 were exported.
Identification of the Hub Targeted Gene of Hub Mutant Genes
The targeted gene upregulation of the hub mutant gene was analyzed for protein–protein interaction (PPI) using online analysis website STRING (https://string-db.org/) (30), and the results were then entered into Cytoscape software (31) to calculate the hub targeted gene of hub mutant genes by using the Mcode plugin.
Gene Oncology Analysis and Kyoto Encyclopedia of Genes and Genomes Pathway Enrichment
The gene oncology (GO) analysis and Kyoto Encyclopedia of Genes and Genomes (KEGG) pathway enrichment of the targeted genes of the hub mutant genes were carried out in the current study using Metascape (http://metascape.ncibi.org/).
Identification of key APOBEC Family Genes
The current study analyzed the differential expression of APOBEC family genes using the online analysis tool—gepia2 (32) and the APOBEC family genes were validated in three independent datasets.
Relationship Between the Hub Targeted Gene of Hub Mutant Genes and Survival
The present study analyzed the relationship between the hub targeted gene of hub mutant genes and overall survival of patients with CC using gepia2, an online analysis tool (32).
Validation of the Hub Targeted Gene of Hub Mutant Genes
Protein expression of the hub targeted gene of hub mutant genes was validated by the Human Protein Atlas (https://www.proteinatlas.org/) (33).
Results
SNP Mutation and CNV Results in CESC Samples
A summary of the TCGA CESC maf file in the present study is presented in Figure 2. After combining the mutation data with the corresponding clinical data, only 289 samples remained for follow-up analysis. In total, there were 55,768 mutations in all 289 samples. It was noted that the most common mutation in the CESC samples was missense mutation, followed by non-sense mutation, frame shift del, frame shift ins, splice site, in-frame ins, in-frame del, and translation start site. Result of the present study showed that the most common variant type was SNP, followed by INS and DEL. Further, it was noted that the most common SNV variant was C>T, followed by C>G, C>A, T>C, T>G, and T>A, whereas the number of variants per sample was 85 and the median missense mutation per sample was 74. In addition, the results of the current study found that the top 10 mutant genes were TNN, MUC4, PIK3CA, MUC16, KMT2C, KMT2D, SYNE1, FLG, EP300, and DMD and the mutations were detected in 224 out of the 289 samples (Figure 3).
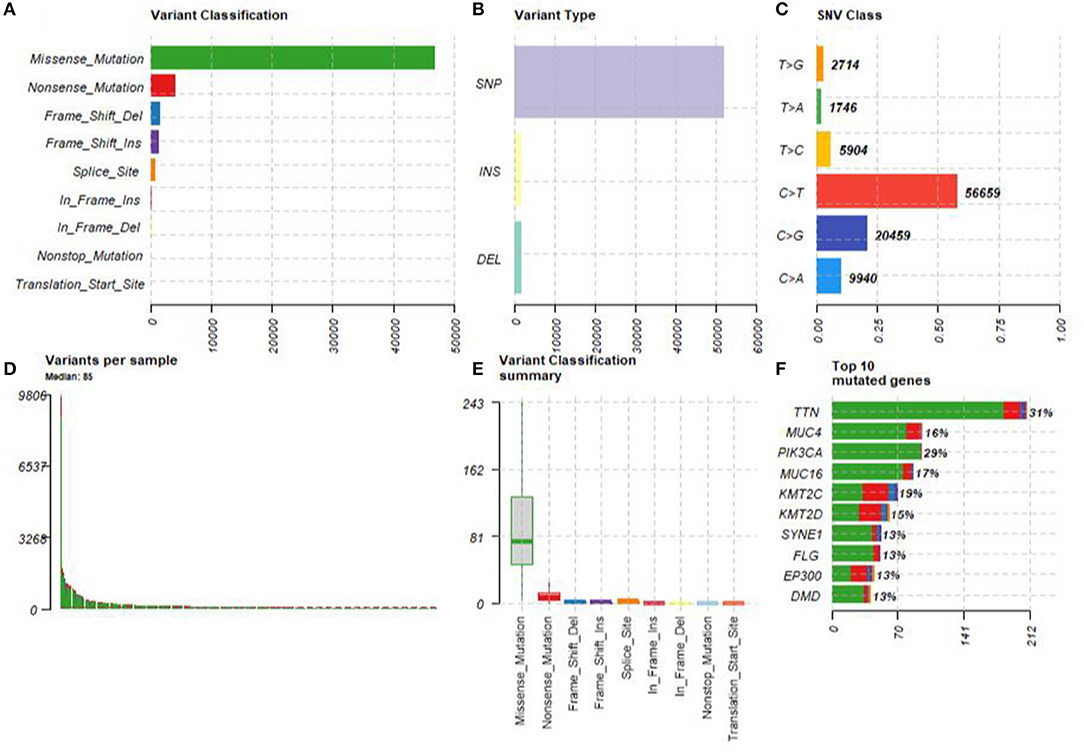
Figure 2. The summary of the TCGA CESC maf file. (A) The most common mutation was missense mutation, followed by nonsense mutation, frame shift del, frame shift ins, splice site, in-frame ins, in-frame del, and translation start site. (B) The most common variant type was single nucleotide polymorphism (SNP), followed by INS and DEL. (C) The most common SNV variant was C>T, followed by C>G, C>A, T>C, T>G, and T>A. (D) The number of variants per sample was 85. (E) The median missense mutation per sample was 74, followed by nonsense mutation, frame shift del, frame shift ins, splice site, in-frame ins, in-frame del, and translation start site. (F) The top 10 mutant genes were TNN, MUC4, PIK3CA, MUC16, KMT2C, KMT2D, SYNE1, FLG, EP300, and DMD.
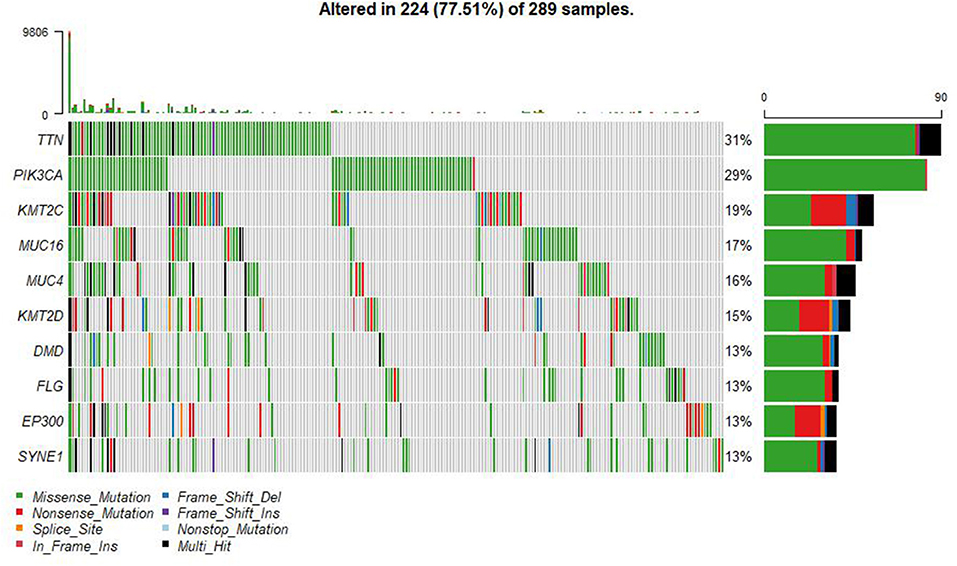
Figure 3. Summary of the TCGA CESC mutations. There were 55,768 mutations in all 289 samples. The top 10 mutant genes were TNN, MUC4, PIK3CA, MUC16, KMT2C, KMT2D, SYNE1, FLG, EP300, and DMD.
Identification of APOBEC-Induced Mutant Genes and Hub Mutant Genes
The present study used the maftools package to enrich C>T mutations occurring within TCW motifs on all C>T mutations in a given sample. Further, the C>T mutations were compared with background cytosines and TCWs occurring within 20 bp of the mutated base. In addition, the APOBEC-enriched and non-enriched sample sizes were 211 and 78, respectively (Figure 4). The top 10 of 192 differentially APOBEC-related mutant genes were FOLH1, FLG, ADCY6, ALPK1, ARHGEF1, LRRIQ3, PCGF5, QSOX1, SLC5A2, and TEAD2. Further, it was found that FLG was the hub mutant gene from the overlapped top 10 mutant genes and the top 10 APOBEC-related mutant genes.
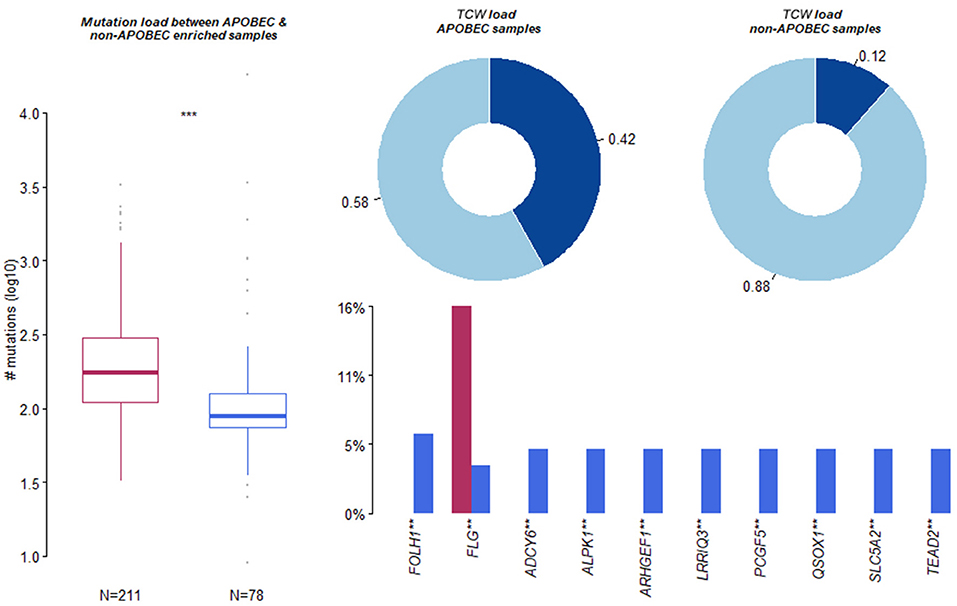
Figure 4. Summary of Apolipoprotein B mRNA editing enzyme-catalyzed polypeptide-like (APOBEC)-induced mutation. Apolipoprotein B mRNA editing enzyme-catalyzed polypeptide-like -enriched and non-enriched sample sizes were 211 and 78, respectively. The top 10 of 192 differentially APOBEC-induced mutant genes were FOLH1, FLG, ADCY6, ALPK1, ARHGEF1, LRRIQ3, PCGF5, QSOX1, SLC5A2, and TEAD2. **, *** means significant difference.
Identification of the Targeted Genes of the Hub Mutant Gene
A total of 124 genes with altered expression associated with FLG gene mutations were identified through muTarget website analysis. It was found that among the 124 genes, there were 99 downregulated genes and 25 upregulated genes.
Identification of the Hub Targeted Gene of the Hub Mutant Gene
A PPI network analysis constructed the interaction networks for the upregulated targeted gene of hub mutant genes. It was found that JUN was the hub targeted gene among the hub mutant genes identified in the current study using the Mcode plugin.
GO Analysis and KEGG Pathway Enrichment
The result of GO analysis and KEGG pathway enrichment in the current study is presented in Figure 5. GO analysis showed that 54 terms were significantly over represented (17 MF, 29 BP, and 8 CC). GO terms that JUN was involved in were DNA-binding transcription activator activity, response to drug, regulation of leukocyte differentiation, response to cAMP, and viral gene expression. Kyoto Encyclopedia of Genes and Genomes (KEGG) pathway analysis revealed that six pathways were significantly enriched. Moreover, the KEGG pathways that JUN was involved in were the IL-17 signaling pathway and endocrine resistance.
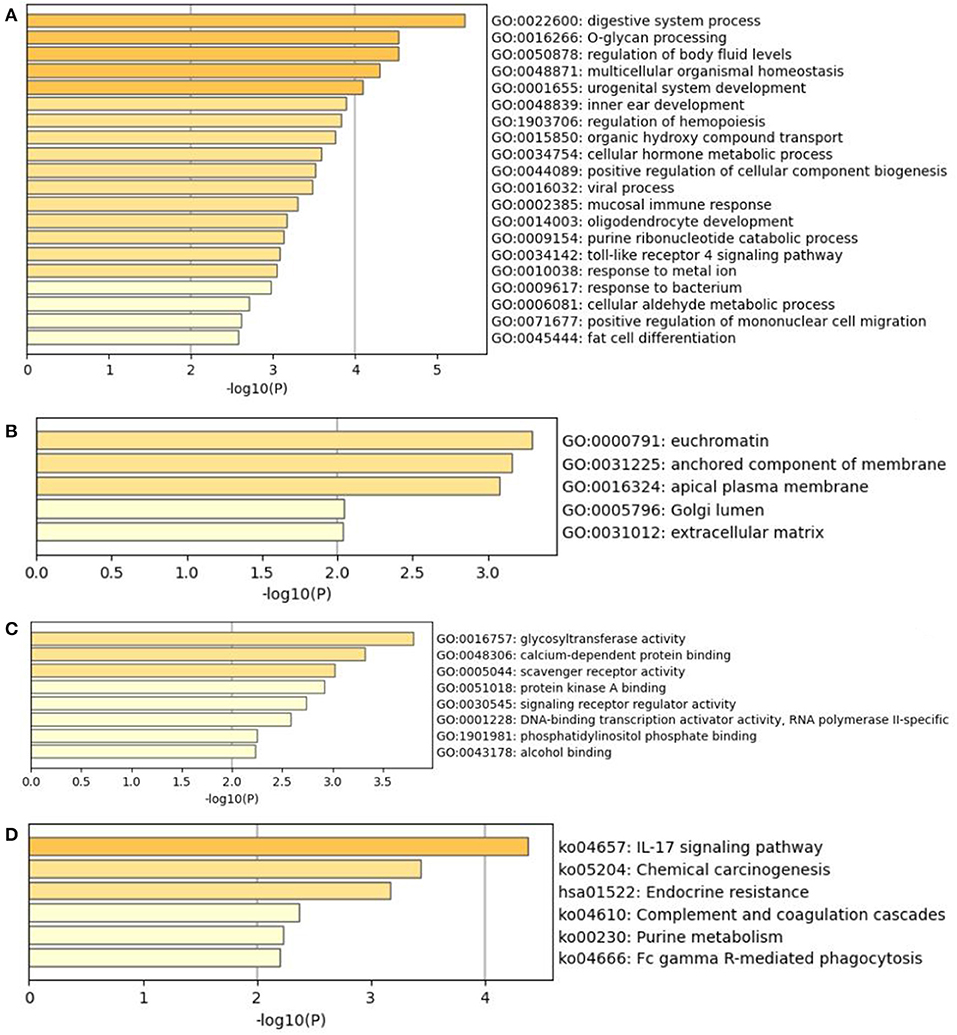
Figure 5. The GO analysis and KEGG pathway enrichment of hub mutant gene's targeted genes. (A) Biological processes (GO-BP). (B) Cellular components (GO-CC). (C) Molecular functions (GO-MF). (D) KEGG pathway of hub mutant gene's targeted genes.
Identification of APOBEC3B as a Key APOBEC Family Gene
The present study performed multiple gene expression analysis of the APOBEC family. Among the gene expressions, it was found that the expression of APOBEC3A and APOBEC3B was significantly higher in tumor tissues than in normal tissues (Figure 6). Only the expression of APOBEC3B correlated with overall survival (OS) and disease-free survival (DFS) (Figure 7). Further, it was noted that only the expression of APOBEC3B was significantly different among the groups in the validation datasets (p < 0.05; Figure 8).
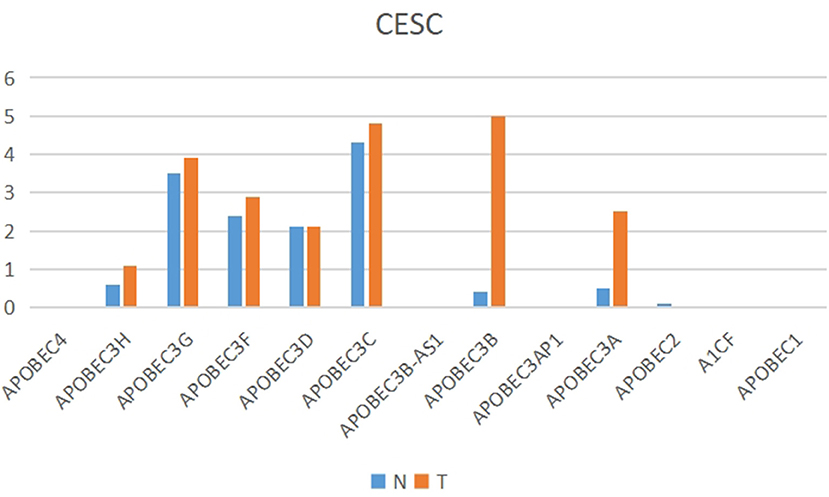
Figure 6. Multiple gene expression analysis of the APOBEC family in CESC. Among the APOBEC gene family, the expression of APOBEC3A and APOBEC3B was significantly higher in tumor tissues than in normal tissues.
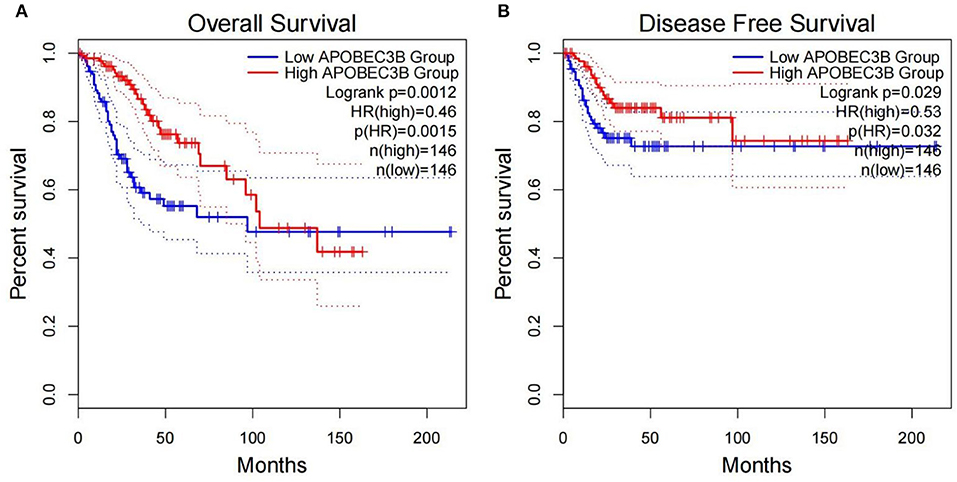
Figure 7. Correlation between the expression of APOBEC3B and overall survival (OS) and disease-free survival (DFS). (A) Overall survival was inferior in APOBEC3B-low patients; (B) DFS was inferior in APOBEC3B-low patients.
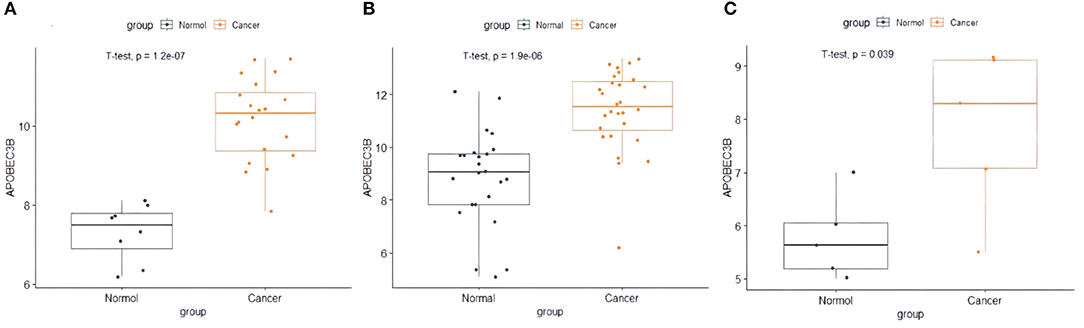
Figure 8. The expression of APOBEC3B in validation datasets. (A) The expression of APOBEC3B in GSE63678, (B) the expression of APOBEC3B in GSE63541, and (C) the expression of APOBEC3B in GES6791.
Relationship Between Hub Mutant Gene and Hub Targeted Gene of the Hub Mutant Gene and Survival
Furthermore, survival analysis was performed in the current study using the mafsurvival function in the maftools software package. Results of the analysis found that FLG mutations were associated with prognosis in patients with CC (Figure 9). The patients were then classified into stages 1, 2, 3, and 4 according to FIGO staging, and it was found that the FLG mutations were associated with the prognosis of CC stage 1 and not with the other stages (not shown). In addition, the survival analysis of JUN was performed using online analysis tool-gepia2 and it was found that JUN was linked with prognosis of CC (Figure 10).
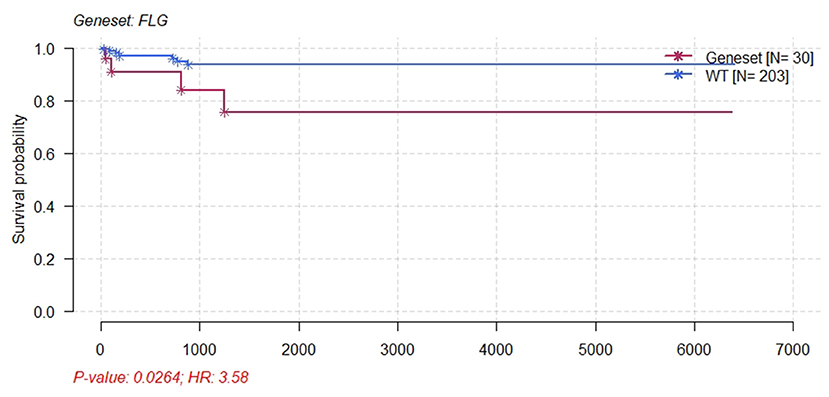
Figure 9. Kaplan–Meier survival plot for FLG wild-type and patients with CESC mutants. Patients with CESC and FLG mutations had significant poor survival.
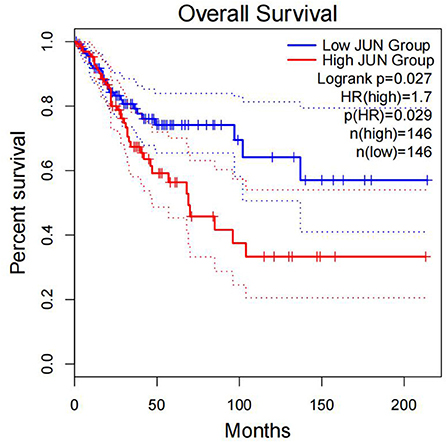
Figure 10. Correlation between the expression of JUN and OS. Overall survival was inferior in patients with high JUN expression.
Immunohistochemical Staining
In the Human Protein Atlas website, it was found that the JUN protein was not shown in normal cervical tissues. However,the protein was expressed at moderate to high levels in CC tissue with two different antibodies (antibody CAB003801 and antibody CAB007780) (Figure 11).
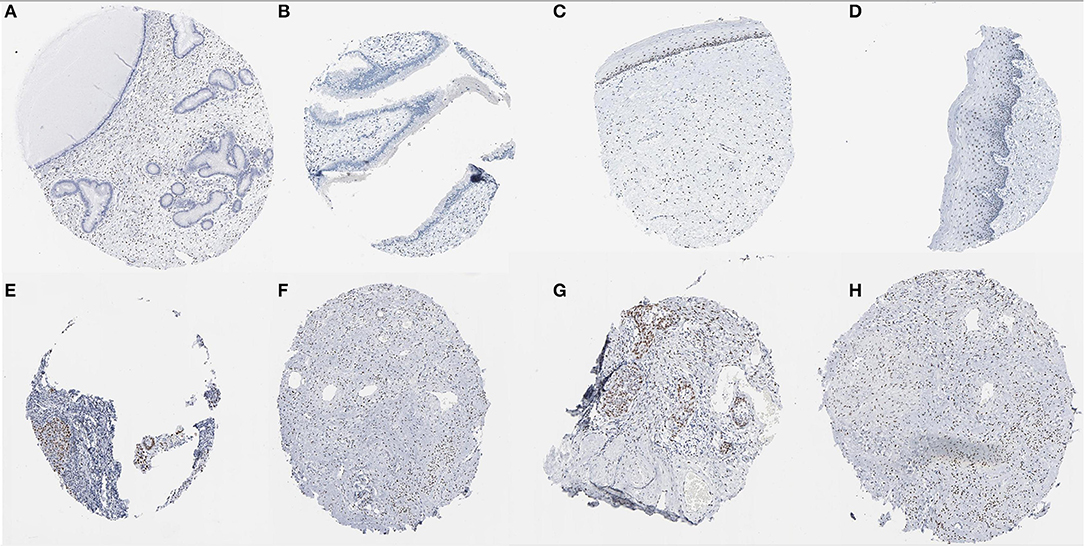
Figure 11. Immunohistochemical images of the JUN gene in cervical tissue. (A–D) Normal cervix JUN protein was not shown in normal cervical tissues; (E–H) JUN protein showed moderate to high levels of staining in CESC (Image available from v21.0. proteinatlas.org).
Discussion
The present study analyzed the masked number segment data of CECS in TCGA. It was evident that APOBEC-induced FLG gene mutations were associated with CC prognosis. Further, it was noted that the patients with FLG mutations had significantly lower survival rates as compared with patients with WT (p = 0.0246, hazard ratio [HR] = 3.58). In addition, online analysis through muTargets found that the expression of JUN was positively correlated with the mutation of FLG and the patients with elevated expression of JUN also had a poor OS rate. Results of the online analysis showed that APOBEC3B and JUN expression was higher in CC tissues than in normal tissues. Only the expression of APOBEC3B was correlated with OS and DFS. We validated that the expression of APOBEC3B was higher in CC tissues than in normal tissues across multiple independent datasets. Therefore, it is suggested that the APOBEC-induced mutation of FLG could upregulate the expression of JUN and then lead to a poor prognosis of patients with CC. Interestingly in this study, OS and DFS were higher in patients with high APOBEC3B than in those with low expression. The exact reason for this is unclear; it is possible that APOBEC3B plays an active role in cervical carcinogenesis, but when its expression exceeds a certain value, it regulates a certain gene or pathway to play a protective role, which needs further investigation.
The Flaggin gene is located in chromosome 1q.21.3 and its coding product is the 4,061 amino acid polypeptide chain. To the best of our knowledge, there are only two studies on FLG mutation of CC. First, a study conducted by Skaaby reported that FLG loss-of-function mutations were associated with a higher incidence of HPV-associated and precancerous cancers. Further, it was reported that the association between the FLG genotype and CC was statistically significant with a HR of 2.0 (95%; CI: 1.0, 4.0) (34). Elsewhere, Bager (35) reported that FLG mutations were not associated with CC (6.3% of cases and 7.7% of controls were carriers: OR adjusted 0.81, 95% CI 0.57–1.14; OR adjusted weighted 0.96, 95% CI 0.58–1.57). It was noted that although FLG mutations increased the CC mortality (HR 4.55, 95% CI 1.70–12.2), the association decreased after cancer stratification (HR 2.53, 95% CI 0.84–7.59) (35). However, the two studies were conducted on two mutant loci (c.1537C>T and c.2318-2321del) that are peculiar to the Danish population and did not address other mutant loci. Furthermore, both studies only focused on germ line mutations in the FLG gene.
The FLG gene is an important epidermal protein that promotes skin barrier function and its mutations remain the most easily replicated and important risk factor for atopic dermatitis (36). It has been reported that mutation of the FLG gene reduces or completely eliminates epidermal filament proteins and their degradation products (37). Further, the loss of FLG function results in a decrease in natural water, leading to an abnormal epithelial barrier (38). Oral mucosa of the defective FLG gene can also be prone to dryness and infection hence leading to dental caries (39). All these suggest that the FLG gene plays an important role in barrier function. Moreover, the filaggrin protein is notably not only expressed in the skin and oral epithelium, but also in the esophageal and cervical mucosa (34).
The FLG gene has been found to be significantly mutated or amplified in some types of cancer, such as colorectal carcinomas (40), differentiated thyroid cancer (41), gastric cancer (42), breast cancer (43), colorectal cancer (44), penile squamous cell carcinoma (45), glioblastoma (46), nasopharyngeal carcinoma (47), malignant melanoma (48), hepatocellular carcinoma (49), and uterine leiomyosarcoma (50). It has been reported that hairdressers are exposed to the higher levels of chemicals that result in FLG mutations, which may affect their risk of cancer (51). Therefore, this also suggests that FLG mutation takes place in response to the stimulation by external factors, thereby increasing susceptibility to cancer for people under high exposure to the factors.
Apolipoprotein B mRNA editing enzyme-catalyzed polypeptide-like (APOBEC)-induced mutations across the cancer genome are common and correlated with the levels of APOBEC mRNA. Results of TCGA-based data analysis showed that APOBEC mutation patterns were evident in bladder, cervical, breast, and lung as well as head and neck cancers, reaching 68% of all mutations in some samples (52). An endogenous mutational process associated with APOBEC deaminase dominates recessive dystrophic epidermolysis bullosa squamous cell carcinomas (RDEB SCC) (53).
The mutation of the FLG gene in the current study is a form of APOBEC-induced mutation. This mutation can result in the loss of function of the FLG gene. It has been noted that inflammation activates APOBEC-1 and stabilizes multiple anti-apoptotic mRNAs (54). APOBEC expression plays an important role in HPV-induced CC (55). According to a study conducted by Qiong et al., APOBEC3B is highly expressed in CC specimens as compared with CIN III with high-risk HPV. The mechanism of APOBEC3B is that HPV-16 E6 can upregulate APOBEC3B by directly binding to the promoter of APOBEC3B in CC cells (56).
C-Jun is an important cytokine that is closely linked to the HPV life cycle (57). It has been found that the overexpression of C-Jun is associated with low-risk specific HPV infection in condyloma acuminatum (58). Further, the stable transfection of HPV-16 and 18 with C-Jun mutants reduces anchorage-independent growth (59). In addition, JNK/c-Jun signaling is necessary for the constitutive expression of HPV E6 and E7 that are critical for the growth and survival of CC cells (60). Therefore, JNK/c-Jun signaling is necessary for the survival of CC cells which suggests that JUN can promote HPV infection and hence lead to the development of CC.
Conclusion
In conclusion, FLG gene mutation induced by APOBEC3B can upregulate the expression of the JUN gene leading to the development and progression of CC, and ultimately resulting in poor prognosis in patients with CC (Figure 12). Therefore, it is possible that the upregulation of APOBEC3B is due to HPV infection and this provides a new direction for the future treatment of CC.
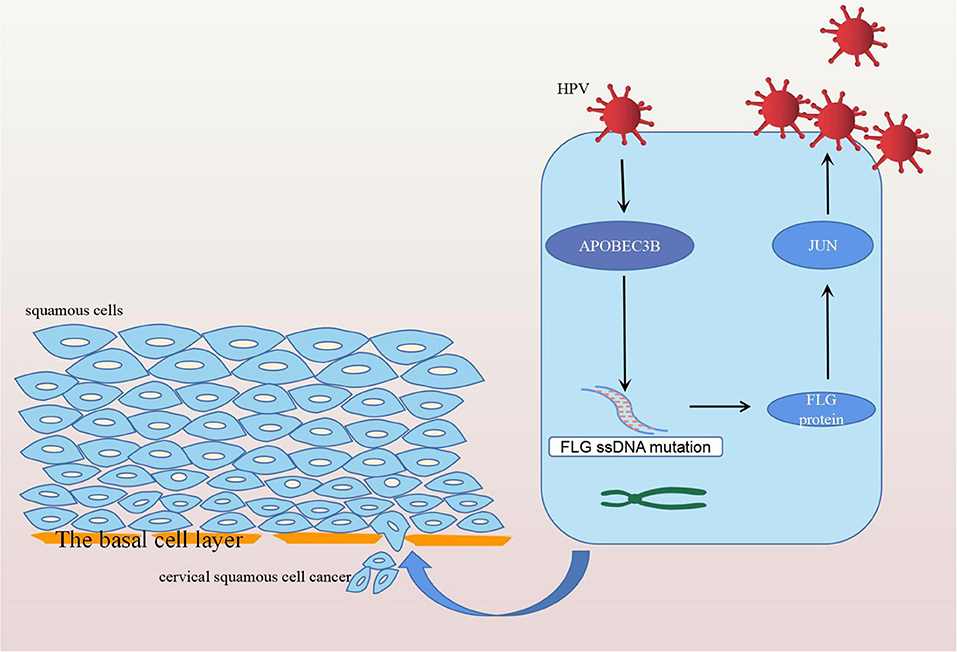
Figure 12. Diagram of the mechanism of action of FLG gene mutation. Human papilloma virus infection stimulates the expression of APOBEC3B, which induces the APOBEC-related mutation of FLG ssDNA. The mutated FLG protein acts on JUN, resulting in its upregulation, and ultimately leading to the development and progression of CC.
Data Availability Statement
The datasets presented in this study can be found in online repositories. The names of the repository/repositories and accession number(s) can be found in the article/Supplementary Material.
Author Contributions
HC participated in the design, performed statistical analyses, and drafted the manuscript. XX conceived the study, participated in the design, and helped to draft the manuscript. LZ, JL, HZ, XW, and XF helped to draft the manuscript. All authors contributed to the article and approved the submitted version.
Funding
This study was supported by the Fundamental Research Funds for the Central Universities of Central South University (2021zzts0391), Major Scientific and Technological Projects for collaborative prevention and control of birth defects in Hunan Province (2019SK1010), the National Natural Science Foundation of China (Grant Number 81771558), and Natural Science Foundation of Hunan Province (Grant Number 2020JJ4814).
Conflict of Interest
The authors declare that the research was conducted in the absence of any commercial or financial relationships that could be construed as a potential conflict of interest.
Publisher's Note
All claims expressed in this article are solely those of the authors and do not necessarily represent those of their affiliated organizations, or those of the publisher, the editors and the reviewers. Any product that may be evaluated in this article, or claim that may be made by its manufacturer, is not guaranteed or endorsed by the publisher.
Supplementary Material
The Supplementary Material for this article can be found online at: https://www.frontiersin.org/articles/10.3389/fmed.2022.815450/full#supplementary-material
References
1. Cao G, Cui R, Liu C, Zhang G, Zhang Z. MTBHsp70-exFPR1-pulsed dendritic cells enhance the immune response against cervical cancer. J Cancer. (2019) 10:6364–73. doi: 10.7150/jca.29779
2. Li S, Liu N, Piao J, Meng F, Li Y. CCNB1 expedites the progression of cervical squamous cell carcinoma via the regulation by FOXM1. Onco Targets Ther. (2020) 13:12383–95. doi: 10.2147/OTT.S279951
3. Sung H, Ferlay J, Siegel RL, Laversanne M, Soerjomataram I, Jemal A, et al. Global cancer statistics 2020: GLOBOCAN estimates of incidence and mortality worldwide for 36 cancers in 185 countries. CA Cancer J Clin. (2021) 71:209–49. doi: 10.3322/caac.21660
4. Li N, Cheng C, Wang T. MiR-181c-5p mitigates tumorigenesis in cervical squamous cell carcinoma via targeting glycogen synthase kinase 3β interaction protein (GSKIP). Onco Targets Ther. (2020) 13:4495–505. doi: 10.2147/OTT.S245254
5. Liu Y, Fan P, Yang Y, Xu C, Huang Y, Li D, et al. Human papillomavirus and human telomerase RNA component gene in cervical cancer progression. Sci Rep. (2019) 9:15926. doi: 10.1038/s41598-019-52195-5
6. Ryan M, Waller J, Marlow LA. Could changing invitation and booking processes help women translate their cervical screening intentions into action? A population-based survey of women's preferences in Great Britain. BMJ Open. (2019) 9:e028134. doi: 10.1136/bmjopen-2018-028134
7. Getachew S, Getachew E, Gizaw M, Ayele W, Addissie A, Kantelhardt EJ. Cervical cancer screening knowledge and barriers among women in Addis Ababa, Ethiopia. PLoS ONE. (2019) 14:e0216522. doi: 10.1371/journal.pone.0216522
8. Wang Z, Gu Y, Wang H, Chen J, Zheng Y, Cui B, et al. Distribution of cervical lesions in high-risk HPV (hr-HPV) positive women with ASC-US: a retrospective single-center study in China. Virol J. (2020) 17:185. doi: 10.1186/s12985-020-01455-2
9. Lin M, Ye M, Zhou J, Wang ZP, Zhu X. Recent advances on the molecular mechanism of cervical carcinogenesis based on systems biology technologies. Comput Struct Biotechnol J. (2019) 17:241–50. doi: 10.1016/j.csbj.2019.02.001
10. Ritz A, Paris PL, Ittmann MM, Collins C, Raphael BJ. Detection of recurrent rearrangement breakpoints from copy number data. BMC Bioinformatics. (2011) 12:114. doi: 10.1186/1471-2105-12-114
11. Zhang L, Hong H. Genomic discoveries and personalized medicine in neurological diseases. Pharmaceutics. (2015) 7:542–53. doi: 10.3390/pharmaceutics7040542
12. Steenwyk JL, Soghigian JS, Perfect JR, Gibbons JG. Copy number variation contributes to cryptic genetic variation in outbreak lineages of Cryptococcus gattii from the North American Pacific Northwest. BMC Genomics. (2016) 17:700. doi: 10.1186/s12864-016-3044-0
13. Zhou Z, Wu S, Lai J, Shi Y, Qiu C, Chen Z, et al. Identification of trunk mutations in gastric carcinoma: a case study. BMC Med Genomics. (2017) 10:49. doi: 10.1186/s12920-017-0285-y
14. Försti A, Kumar A, Paramasivam N, Schlesner M, Catalano C, Dymerska D, et al. Pedigree based DNA sequencing pipeline for germline genomes of cancer families. Hered Cancer Clin Pract. (2016) 14:16. doi: 10.1186/s13053-016-0058-1
15. Catteau A, Girardi H, Monville F, Poggionovo C, Carpentier S, Frayssinet V, et al. A new sensitive PCR assay for one-step detection of 12 IDH1/2 mutations in glioma. Acta Neuropathol Commun. (2014) 2:58. doi: 10.1186/2051-5960-2-58
16. Gusenleitner D, Howe EA, Bentink S, Quackenbush J, Culhane AC. iBBiG. iterative binary bi-clustering of gene sets. Bioinformatics. (2012) 28:2484–92. doi: 10.1093/bioinformatics/bts438
17. Tang W, Cao Y, Ma X. Novel prognostic prediction model constructed through machine learning on the basis of methylation-driven genes in kidney renal clear cell carcinoma. Biosci Rep. (2020) 40:BSR20201604. doi: 10.1042/BSR20201604
18. Zhu FC, Hu SY, Hong Y, Hu YM, Zhang X, Zhang YJ, et al. Efficacy, immunogenicity and safety of the AS04-HPV-16/18 vaccine in Chinese women aged 18-25 years: End-of-study results from a phase II/III, randomised, controlled trial. Cancer Med. (2019) 8:6195–6211. doi: 10.1002/cam4.2399
19. Silva NNT, Santos ACS, Nogueira VM, Carneiro CM, Lima AA. 3'UTR polymorphism of Thymidylate Synthase gene increased the risk of persistence of pre-neoplastic cervical lesions. BMC Cancer. (2020) 20:323. doi: 10.1186/s12885-020-06811-7
20. Amorim AT, Marques LM, Campos GB, Lobão TN, de Souza Lino V, Cintra RC, et al. Co-infection of sexually transmitted pathogens and Human Papillomavirus in cervical samples of women of Brazil. BMC Infect Dis. (2017) 17:769. doi: 10.1186/s12879-017-2835-5
21. Nguyen HT, Le DT, Duong QH, Tatipamula VB, Van Nguyen B. High frequency of microsatellite instability and its substantial co-existence with KRAS and BRAF mutations in Vietnamese patients with colorectal cancer. Oncol Lett. (2021) 21:41. doi: 10.3892/ol.2020.12302
22. Cai T, Wu W, Guo L, Xia Y, Jiang X, Zhang L, et al. Notoginsenoside R1 induces DNA damage via PHF6 protein to inhibit cervical carcinoma cell proliferation. Mol Med Rep. (2021) 23:242. doi: 10.3892/mmr.2021.11881
23. Cao CH, Liu R, Lin XR, Luo JQ, Cao LJ, Zhang QJ, et al. LRP1B mutation is associated with tumor HPV status and promotes poor disease outcomes with a higher mutation count in HPV-related cervical carcinoma and head & neck squamous cell carcinoma. Int J Biol Sci. (2021) 17:1744–56. doi: 10.7150/ijbs.56970
24. Li L, Ding L, Gao T, Lyu Y, Wang M, Song L, et al. Association between vaginal micro-environment disorder and cervical intraepithelial neoplasia in a community based population in China. J Cancer. (2020) 11:284–91. doi: 10.7150/jca.35022
25. Xu WK, Byun H, Dudley JP. The role of APOBECs in viral replication. Microorganisms. (2020) 8:1899. doi: 10.3390/microorganisms8121899
26. Elango R, Osia B, Harcy V, Malc E, Mieczkowski PA, Roberts SA, et al. Repair of base damage within break-induced replication intermediates promotes kataegis associated with chromosome rearrangements. Nucleic Acids Res. (2019) 47:9666–84. doi: 10.1093/nar/gkz651
27. Shi MJ, Meng XY, Fontugne J, Chen CL, Radvanyi F, Bernard-Pierrot I. Identification of new driver and passenger mutations within APOBEC-induced hotspot mutations in bladder cancer. Genome Med. (2020) 12:85. doi: 10.1186/s13073-020-00781-y
28. Mayakonda A, Lin DC, Assenov Y, Plass C, Koeffler HP. Maftools: efficient and comprehensive analysis of somatic variants in cancer. Genome Res. (2018) 28:1747–56. doi: 10.1101/gr.239244.118
29. Nagy Á, Gyorffy B. muTarget: a platform linking gene expression changes and mutation status in solid tumors. Int J Cancer. (2021) 148:502–11. doi: 10.1002/ijc.33283
30. Szklarczyk D, Gable AL, Lyon D, Junge A, Wyder S, Huerta-Cepas J, et al. STRING v11: protein-protein association networks with increased coverage, supporting functional discovery in genome-wide experimental datasets. Nucleic Acids Res. (2019) 47:D607–13. doi: 10.1093/nar/gky1131
31. Shannon P, Markiel A, Ozier O, Baliga NS, Wang JT, Ramage D, et al. Cytoscape: a software environment for integrated models of biomolecular interaction networks. Genome Res. (2003) 13:2498–504. doi: 10.1101/gr.1239303
32. Tang Z, Li C, Kang B, Gao G, Li C, Zhang Z, et al. a web server for cancer and normal gene expression profiling and interactive analyses. Nucleic Acids Res. (2017) 45:W98–W102. doi: 10.1093/nar/gkx247
33. Uhlén M, Fagerberg L, Hallström BM, Lindskog C, Oksvold P, Mardinoglu A, et al. Tissue-based map of the human proteome. Science. (2015) 347:1260419. doi: 10.1126/science.1260419
34. Skaaby T, Husemoen LL, Jørgensen T, Johansen JD, Menné T, Szecsi PB, et al. Associations of filaggrin gene loss-of-function variants and human papillomavirus-related cancer and pre-cancer in Danish adults. PLoS ONE. (2014) 9:e99437. doi: 10.1371/journal.pone.0099437
35. Bager P, Wohlfahrt J, Sørensen E, Ullum H, Høgdall CK, Palle C, et al. Common filaggrin gene mutations and risk of cervical cancer. Acta Oncol. (2015) 54:217–23. doi: 10.3109/0284186X.2014.973613
36. Debińska A. New treatments for atopic dermatitis targeting skin barrier repair via the regulation of FLG expression. J Clin Med. (2021) 10:2506. doi: 10.3390/jcm10112506
37. Kezic S, O'Regan GM, Yau N, Sandilands A, Chen H, Campbell LE, et al. Levels of filaggrin degradation products are influenced by both filaggrin genotype and atopic dermatitis severity. Allergy. (2011) 66:934–40. doi: 10.1111/j.1398-9995.2010.02540.x
38. Gruber R, Sugarman JL, Crumrine D, Hupe M, Mauro TM, Mauldin EA, et al. Sebaceous gland, hair shaft, and epidermal barrier abnormalities in keratosis pilaris with and without filaggrin deficiency. Am J Pathol. (2015) 185:1012–21. doi: 10.1016/j.ajpath.2014.12.012
39. Shim JS, Yang MS. Identification of oral symptoms associated with atopic dermatitis in adolescents: Results from the Korea national representative survey 2009-2017. Sci Rep. (2020) 10:19461. doi: 10.1038/s41598-020-76532-1
40. Ge W, Hu H, Cai W, Xu J, Hu W, Weng X, et al. High-risk Stage III colon cancer patients identified by a novel five-gene mutational signature are characterized by upregulation of IL-23A and gut bacterial translocation of the tumor microenvironment. Int J Cancer. (2020) 146:2027–35. doi: 10.1002/ijc.32775
41. Hou S, Xie X, Zhao J, Wu C, Li N, Meng Z, et al. Downregulation of miR-146b-3p inhibits proliferation and migration and modulates the expression and location of sodium/iodide symporter in dedifferentiated thyroid cancer by potentially targeting MUC20. Front Oncol. (2021) 10:566365. doi: 10.3389/fonc.2020.566365
42. Wang H, Shen L, Li Y, Lv J. Integrated characterisation of cancer genes identifies key molecular biomarkers in stomach adenocarcinoma. J Clin Pathol. (2020) 73:579–86. doi: 10.1136/jclinpath-2019-206400
43. Midha MK, Huang YF, Yang HH, Fan TC, Chang NC, Chen TH, et al. Comprehensive cohort analysis of mutational spectrum in early onset breast cancer patients. Cancers. (2020) 12:2089. doi: 10.3390/cancers12082089
44. Peng L, Li Y, Gu H, Xiang L, Xiong Y, Wang R, et al. Mucin 4 mutation is associated with tumor mutation burden and promotes antitumor immunity in colon cancer patients. Aging (Albany NY). (2021) 13:9043–55. doi: 10.18632/aging.202756
45. Wang Y, Wang K, Chen Y, Zhou J, Liang Y, Yang X, et al. Mutational landscape of penile squamous cell carcinoma in a Chinese population. Int J Cancer. (2019) 145:1280–9. doi: 10.1002/ijc.32373
46. Wipfler K, Cornish AS, Guda C. Comparative molecular characterization of typical and exceptional responders in glioblastoma. Oncotarget. (2018) 9:28421–33. doi: 10.18632/oncotarget.25420
47. Yang Y, Liu W, Zhao Z, Zhang Y, Xiao H, Luo B. Filaggrin gene polymorphism associated with Epstein-Barr virus-associated tumors in China. Virus Genes. (2017) 53:532–7. doi: 10.1007/s11262-017-1463-x
48. Thyssen JP, Andersen YMF, Balslev E, Szecsi PB, Stender S, Kaae J, et al. Loss-of-function mutations in filaggrin gene and malignant melanoma: a case-control study. J Eur Acad Dermatol Venereol. (2018) 32:242–4. doi: 10.1111/jdv.14532
49. Zhu P, Ren Q, He N, Zhou C, Jin Q, Gong Z. Construction and validation of an N6-methyladenosine-associated prognostic signature in hepatocellular carcinoma. Oncol Lett. (2021) 21:221. doi: 10.3892/ol.2021.12482
50. Cho YL, Bae S, Koo MS, Kim KM, Chun HJ, Kim CK, et al. Array comparative genomic hybridization analysis of uterine leiomyosarcoma. Gynecol Oncol. (2005) 99:545–51. doi: 10.1016/j.ygyno.2005.07.017
51. Liljedahl ER, Wahlberg K, Lidén C, Albin M, Broberg K. Genetic variants of filaggrin are associated with occupational dermal exposure and blood DNA alterations in hairdressers. Sci Total Environ. (2019) 653:45–54. doi: 10.1016/j.scitotenv.2018.10.328
52. Roberts SA, Lawrence MS, Klimczak LJ, Grimm SA, Fargo D, Stojanov P, et al. An APOBEC cytidine deaminase mutagenesis pattern is widespread in human cancers. Nat Genet. (2013) 45:970–6. doi: 10.1038/ng.2702
53. Cho RJ, Alexandrov LB, den Breems NY, Atanasova VS, Farshchian M, Purdom E, et al. APOBEC mutation drives early-onset squamous cell carcinomas in recessive dystrophic epidermolysis bullosa. Sci. Transl Med. (2018) 10:9668. doi: 10.1016/j.jid.2017.07.734
54. Wright N. Inflammation activates Apobec-1 and stabilizes multiple anti-apoptotic mRNAs. Gastroenterology. (2004) 127:1259. doi: 10.1053/j.gastro.2004.08.062
55. Chen L, Qiu X, Zhang N, Wang Y, Wang M, Li D, et al. APOBEC-mediated genomic alterations link immunity and viral infection during human papillomavirus-driven cervical carcinogenesis. Biosci Trends. (2017) 11:383–8. doi: 10.5582/bst.2017.01103
56. Fan Q, Huang T, Sun X, Wang YW, Wang J, Liu Y, et al. HPV-16/18 E6-induced APOBEC3B expression associates with proliferation of cervical cancer cells and hypomethylation of Cyclin D1. Mol Carcinog. (2021) 60:313–30. doi: 10.1002/mc.23292
57. Prasad CK, Meyers C, Zhan DJ, You H, Chiriva-Internati M, Mehta JL, et al. The adeno-associated virus major regulatory protein Rep78-c-Jun-DNA motif complex modulates AP-1 activity. Virology. (2003) 314:423–31. doi: 10.1016/S0042-6822(03)00439-2
58. Yang YF, Tsao YP, Yin CS, Chen SL, Chu TY. Overexpression of the proto-oncogene c-jun in association with low-risk type specific human papillomavirus infection in condyloma acuminata. J Med Virol. (1996) 48:302–7. doi: 10.1002/(SICI)1096-9071(199604)48:4<302::AID-JMV2>3.0.CO;2-B
59. Li JJ, Rhim JS, Schlegel R, Vousden KH, Colburn NH. Expression of dominant negative Jun inhibits elevated AP-1 and NF-kappaB transactivation and suppresses anchorage independent growth of HPV immortalized human keratinocytes. Oncogene. (1998) 16:2711–21. doi: 10.1038/sj.onc.1201798
Keywords: APOBEC, FLG, mutation, JUN, cervical cancer
Citation: Chen H, Zhao L, Liu J, Zhou H, Wang X, Fang X and Xia X (2022) Bioinformatic Analyzes of the Association Between Upregulated Expression of JUN Gene via APOBEC-Induced FLG Gene Mutation and Prognosis of Cervical Cancer. Front. Med. 9:815450. doi: 10.3389/fmed.2022.815450
Received: 15 November 2021; Accepted: 15 March 2022;
Published: 18 April 2022.
Edited by:
Francesco Cosentino, University of Molise, ItalyReviewed by:
Mei Qiang, Shanxi Medical University, ChinaArtem G. Lada, University of California, Davis, United States
Copyright © 2022 Chen, Zhao, Liu, Zhou, Wang, Fang and Xia. This is an open-access article distributed under the terms of the Creative Commons Attribution License (CC BY). The use, distribution or reproduction in other forums is permitted, provided the original author(s) and the copyright owner(s) are credited and that the original publication in this journal is cited, in accordance with accepted academic practice. No use, distribution or reproduction is permitted which does not comply with these terms.
*Correspondence: Xiaomeng Xia, eGlheGlhb21lbmdAY3N1LmVkdS5jbg==