1,25-dihydroxyvitamin D as Predictor of Renal Worsening Function in Chronic Kidney Disease. Results From the PASCaL-1,25D Study
- 1Renal Division, Azienda Socio Sanitaria Territoriale (ASST) Santi Paolo e Carlo, Department of Health Science, University of Milan, Milan, Italy
- 2DiaSorin SpA, Saluggia, Italy
- 3Consultant for DiaSorin, Lugano, Switzerland
- 4DiaSorin Inc, Stillwater, MN, United States
- 5Reply Data, Milan, Italy
Background: Heterogeneous progression of chronic kidney disease (CKD) toward dialysis advocates improving in renal care management. Diagnosis and staging of CKD relies on estimated glomerular filtration rate (eGFR) and albuminuria. Tubular biomarkers emerged as new predictors of worsening renal function (WRF), due to partial inaccuracy of eGFR and existing WRF in non-proteinuric patients. Active vitamin D is synthesized in renal tubules and participates to mineral adaptation in CKD. Circulating 1,25-dihydroxyvitamin D [1,25(OH)2D] was poorly investigated as a biomarker of endocrine tubular function and predictor of WRF.
Objective: Investigate capability of 1,25(OH)2D to predict parathormone (PTH) increase and WRF in CKD stage 3–4.
Methods: PASCaL-1,25D was an observational, prospective, monocentric study. Primary outcomes were absolute and 20% increase in PTH, and WRF defined as 20% reduction in eGFR or dialysis initiation at 6 months.
Results: Seventy-one patients completed follow up. Absolute increase in PTH (1–84) was independently predicted by lower 1,25(OH)2D levels (p = 0.0134). No association was detected between 1,25(OH)2D and iPTH increase. Higher 1,25(OH)2D was associated with reduced risk of WRF at univariate analysis [OR 0.89 (95% CI 0.86–0.93), p = 0.006]. The 1,25(OH)2D/PTH (1–84) ratio was associated with non-significant 84% risk reduction for WRF [OR 0.16 (95% CI 0.06–0.41), p = 0.05]. Low 1,25(OH)2D reached 100% sensitivity in predicting WRF in CKD stage 3 (AUC 9.909, p < 0.0001) and non-elderly patients (AUC 0.883, p < 0.0001). Machine learning models retained 1,25(OH)2D/PTH (1–84) as relevant predictor of WRF together with eGFR and albuminuria. Age influenced interaction between renal and mineral biomarkers.
Conclusion: 1,25(OH)2D deserves attention as biomarker of tubular health, and sensible predictor of WRF on the short run among non-elderly patients affected by stage 3 CKD. The 1,25(OH)2D/PTH (1–84) ratio may represent a composite biomarker of tubular reserve/endocrine response to the transition from adaptive to maladaptive equilibrium in CKD-MBD.
Introduction
Chronic kidney disease (CKD) represents a major public health problem, associated with poor quality of life, reduced survival and considerable economic impact (1–3). Recognizing individuals at risk for progression toward end stage renal disease (ESRD) remains challenging, although being essential to prevent patients from advancing to dialysis and renal transplant (4, 5).
Stratification of the risk for CKD progression currently relies on estimated glomerular filtration rate (eGFR) and albuminuria (6). However, their accuracy is limited by intrinsic unreliability of serum creatinine (sCr) and by the existence of non-albuminuric patients, who proceed toward ESRD (7, 8). Furthermore, eGFR and albuminuria do not reflect tubular injury (9), which on the contrary was associated with the risk of worsening renal function (WRF) in biopsy proven CKD (10, 11).
Although biomarkers of tubular damage are emerging as new predictors of WRF (12–16), a gold standard for non-invasive assessment of tubular health has not been validated. Nonetheless, renal tubules respond to alterations in mineral homeostasis secondary to lowered GFR. Hydroxylation of 25 hydroxyvitamin D [25(OH)D] into the active form 1,25-dihydroxyvitamin D [1,25(OH)2D] by the renal 1-α-hydroxylase (CYP27B2) represents a major endocrine function of the proximal renal tubule. Expression of CYP27B2 is up and downregulated by parathormone (PTH) and fibroblast growth factor-23 (FGF-23) respectively in the context of chronic kidney disease and mineral bone disorder (CKD-MBD) (17). Hence, it could be argued that CYP27B2 activity might be taken as biomarker of tubular endocrine reserve and of homeostatic response to mineral derangement.
Expression of 1-α-hydroxylase (CYP27B2) declines in CKD, secondary to the loss of nephron mass and to the direct inhibition elicited by increasing levels of FGF-23 (18–20). Consequent reduction in 1,25(OH)2D triggers the compensatory PTH increase, leading to secondary hyperparathyroidism (SHPT) (21, 22). In the impossibility to directly assess CYB27B2, circulating levels of 1–25(OH)2D might be considered a proxy of tubular synthesis, and 1,25(OH)2D/PTH ratio as a composite marker of tubular endocrine reserve and setpoint of transition from adaptive to maladaptive response in CKD-MBD.
It has been suggested that a composite risk factor, derived from the aggregation of both tubular synthesis biomarkers and hormones involved in mineral metabolism, may predict WRF. Post-hoc analysis from the SPRINT trial reported on how intact PTH (iPTH) and FGF-23, included with uromodulin (UMOD) into a single tubule reserve/mineral metabolism factor, resulted as unique independent predictor of eGFR decline (13). Thus, 1,25(OH)2D and 1,25(OH)2D/PTH ratio might deserve attention as negative risk factors of WRF.
However, reliable assessment of 1,25(OH)2D and of biologically active PTH remains an unmet need in clinical practice (23–25). DiaSorin LIAISON® XL analyzer is a validated and fully-automated method, which provides both the-third generation assay for the only biologically active PTH (1–84) whole peptide (26) and the accurate assessment of 1,25(OH)2D (27, 28). The 1,25(OH)2D/PTH (1–84) ratio, measured by aforementioned assay, independently predicted WRF in heart failure (29) and CKD stage G3b to G5 patients (30).
Results of PASCaL-1,25D study (PredictAbility of Secondary hyperparathyroidism and Ckd progression by circulating Levels of 1–25(OH)2 vitamin D) are herein presented. The study was designed to investigate the capability of low 1,25(OH)2D levels in predicting WRF and PTH increase, among adult patients affected by CKD stage G3 to G4 during 6-months follow up.
Methods
Study Design
PASCaL-1,25D study was an observational, prospective, monocentric study, conducted from September 2017 to November 2018 at the Renal Unit of San Paolo Hospital in Milan (Italy).
All adult patients, attending follow up at Renal Clinic due to CKD stage G3 to G4, were enrolled from September 2017 to May 2018. Subjects receiving nutritional vitamin D or vitamin D receptor activators (VDRA) were included. Exclusion criteria were age ≥85 or age <8 years, primary hyperparathyroidism, previous parathyroidectomy, pregnancy, lactation, ongoing bisphosphonates, denosumab, teriparatide, strontium ranelate, and inability or unwilling to subscribe written informed consent.
Primary outcomes consisted in: analyzing capability of basal 1,25(OH)2D to predict absolute and 20% increase in PTH (1–84) and iPTH levels at 6-months, and investigating basal 1–25(OH)2D as predictor of WRF, defined as 20% reduction in eGFR or dialysis initiation at 6-months follow up.
Secondary objectives consisted in: correlating 1,25(OH)2D with eGFR and measured GFR (mGFR) at baseline, and correlating baseline 1,25(OH)2D to relevant analytes in the context of CKD-MBD, including iPTH, PTH (1–84), 25(OH)D, serum calcium (sCa), serum phosphorus (sP), intact FGF-23 (iFGF-23), bone specific alkaline phosphatase (BSAP) and sclerostin.
Exploratory analysis was included, for testing 1,25(OH)2D/PTH (1–84) and 1,25(OH)2D/iPTH ratios as predictors of 6-month WRF.
Age, gender, mGFR, history of cardiovascular disease (CVD), diabetes, arterial hypertension, cancer and the cause of CKD were collected at baseline. Following clinical and biochemical data were recorded at study entry, 3 and 6 months clinical visits: height, weight, blood pressure, heart rate, ongoing therapies for hypertension and diabetes, prescription of nutritional vitamin D and VDRA, sCr, 25(OH)D, 1,25(OH)2)D, PTH (1–84), iPTH, total sCa, sP, iFGF-23, sclerostin, alkaline phosphatase (ALP), bone specific alkaline phosphatase (BSAP), glycated hemoglobin (Hba1c) in diabetic patients, 24 h urinary analysis for creatinine, albumin, sodium, P and Ca. Medical therapies, including vitamin D, were modifiable during study period, based on nephrologists' clinical judgement.
Seventy patients were estimated as the sample size required, for detecting 20 PTH increase and 20% WRF at 6 months with 95 significance and 80% study power, based on 15–25% prevalence of CKD stage G3 to G4 observed at Renal Unit of San Paolo Hospital during a 2-months pre-study investigation (data not shown). Patients were censored whenever lost to follow up or due to voluntary withdrawal from the study.
Estimated GFR was assessed by CKD-EPI formula (31) at baseline, 3 and 6 months. Measured GFR was also assessed at study entry by 99 mTc-diethylenetriamine-pentaacetic acid renal scintigraphy, performed at the Nuclear Medicine Unit of San Paolo Hospital (32).
All the blood samples were collected at fasting between 7:00 and 10:00 a.m. Routine biochemical measurements were performed at the corresponding local accredited laboratories by standardized assays. Assessment of bone mineral biomarkers was performed at DiaSorin Inc. (Stillwater MN, USA) on a LIAISON® XL analyzer using the fully-automated LIAISON® assays for serum 1,25(OH)2D (#310980), total 25(OH)D (#310600), plasmatic PTH (1–84) (#310630), plasmatic iFGF-23 (#318700), serum sclerostin (#310930), and BAP OSTASE® (#310970). Samples for immunoassays assessment were prepared for testing and frozen (−20°C), until testing, conducted at DiaSorin laboratories (Stillwater, MN). All other blood and urinary analysis were performed at the San Paolo Hospital Laboratory by standardized routine assays. Second-generation assay, adopted for iPTH assessment, was t by VITROS 5600 integrated system.
PASCAL-1,25D study was approved by San Paolo Hospital ethical committee.
Statistical Analysis
Categorical and continuous variables were reported as mean ± SD or median (IQR) and frequencies (%) as appropriate. Patients' characteristics at baseline were stratified according to: basal eGFR (higher-equal or lower than 30 ml/min/1.73 m2), WRF and 20% increase in PTH (1–84). Differences between strata were tested for significance by ANOVA or Mann-Whitney U Test and Chi-squared or Fisher-exact test for continuous and categorical variables, respectively, as appropriate for normality of distribution.
Predictability of absolute and 20% increase in PTH (1–84) and iPTH was tested by separated multivariate regression models. Backward elimination procedure was adopted for best model selection. The following parameters were included as predictors at first step, based on clinical judgment in addition to the variables associated with PTH increase at univariate analysis: age, gender, 1,25(OH)2D, 25(OH)D, basal eGFR, any vitamin D therapy along the study period, urine albumin creatinine ratio (uACR) as continuous variable, iFGF23, sclerostin, sCa, sP, BSAP, diabetes and body mass index (BMI). Secondary models were performed, adopting 1,25(OH)2D as categorical variable, stratified according to threshold derived by Receiver Operating Characteristic analysis (ROC).
Univariate regression models were built to assess the association between 20% WRF and biochemical predictors. ROC analysis was performed to predict 20% WRF by 1,25(OH)2D, and eGFR in the whole cohort. DeLong and binomial exact methodology were applied to ROC analysis, which was further stratified according to basal eGFR and elderly.
Repeated measure analysis of variance was applied, for comparing renal and mineral parameters between baseline and 6-month follow up, stratified according to basal eGFR and to the presence of any ongoing vitamin D therapy.
Linear correlation between GFR, 1,25(OH)2D and PTH (1–84) at baseline was assessed by Pearson coefficient analysis. Spearman correlation matrix was performed between parameters of renal function and mineral metabolism at baseline, stratified according to age.
Classic statistical analysis was conducted by the programs R and MedCalc v20.
Non-prespecified post-hoc analysis was performed by machine learning (ML) techniques, for compensating unbalance derived by the low rate of WRF events observed. XGBoost methodology was applied to train a regression model in a supervised setting, for ascertaining which biomarkers may elicit biggest impact in predicting eGFR at 3-months follow up (33–36). In the training phase, 80% of the dataset was used to train the algorithm. The performance of resulting model was further tested on the remaining 20% of the dataset. Since XGBoost and other ML algorithms rely on a set of hyper parameters to optimally solve the machine learning problem, an extensive hyper parameter tuning phase was performed (37). A grid search, guided by squared error metric over 2-fold cross validation, was used to selected best values for each hyper parameter. GridSearchCV API from the scikit-learn package was adopted in conjunction with the XGBRegressor API from the XGboost library (38). The resulting values were 0.1 for the learning rate, 4 for the trees max depth, 0.7 for the observations subset sampling rate of each tree and 50 for the number of XGboost estimators. The final XGBoost. The model was trained by with these hyper parameters values. Model was evaluated on the test set by the mean absolute percentage error (MAPE) (39), which represents the percentual absolute error of model prediction. The scaling compared to the actual value allowed to properly weigh errors for low values of eGFR (39). Variables were finally sorted, according to their single weight in eGFR prediction after ex-post analysis.
Results
A total of 74 patients were enrolled, of whom three were subsequently lost to follow up. Analysis was performed on 71 patients.
In the overall population median age was 75 years (69–80 IQR), with 73% patients being older than 70 years (Table 1). Baseline eGFR and urinary albumin to creatinine ratio (uACR) were 31.2 ml/min/1.73 m2 (21.6–41.7 IQR) and 144 mg/g (49.0–620 IQR), respectively. Mild SHPT was highly represented [iPTH 112 pg/ml (85.4–157 IQR), PTH (1–84) 37.6 pg/ml (28.9–50.3 IQR)] in the presence of median 25(OH)D levels at the bottom threshold of normality range [31.0 ng/ml (22.7–41.8 IQR)] and normal serum levels of Ca, P, ALP, BSAP, and sclerostin. Forty (56%) patients received renin angiotensin receptor inhibitors. Vitamin D was prescribed in 46 (65%) as nutritional formulation (45%) or VDRA (3%) alone, or as combination of both nutritional and VDRA compounds (17%).
At 6-month follow up 29 (41%) and 40 (56%) patients reached 20% increase in iPTH and PTH (1–84), respectively. Fifteen (21%) patients developed 20% WRF, respectively (Figure 1).
Basal eGFR <30 ml/min/1.73 m2 was associated with significantly higher levels of serum P, iPTH, PTH (1–84), iFGF-23 and lower 24 h renal excretion of both Ca and P (Table 1). The 1,25(OH)2D/iPTH and 1,25(OH)2D/PTH (1–84) ratios were significantly lower in patients with eGFR <30 ml/min/1.732 (p < 0.001), despite similar 1,25(OH)2D levels between eGFR strata (Table 1). Vitamin D was more frequently prescribed within lower eGFR group (85 vs. 47%) (p- for trend = 0.001). Rates of WRF and PTH increase were not influenced by the basal eGFR.
Basal PTH (1–84) was lower among patients who further achieved ≥20% increase in PTH (1–84) at 6 months [34.3 pg/ml (21.7–45.9 IQR) vs. 41.5 pg/ml (32.0–58.4 IQR), p = 0.01] (Supplementary Table 1). Absolute PTH (1–84) increase was independently predicted by unitary lower 1,25(OH)2D levels (p = 0.0134), absence of diabetes (p = 0.017), older age (p = 0.022), and higher uACR (p = 0.023) (Table 2). However, 1,25(OH)2D taken as categorical variable (cut-off set at 29 pg/ml) was unable to predict PTH increase (data not shown). None of the basal parameters was retained for predicting 20% PTH (1–84) increase in multivariate models. The increase in iPTH levels was not predicted by 1,25(OH)2D as continuous or categorical variable (Supplementary Table 2). Rate of iPTH and PTH (1–84) increase was not associated with WRF (Supplementary Table 3).
Lower baseline 1,25(OH)2D was the only patients' characteristics descriptively associated with WRF [25.7 pg/ml (16.2–28.9 IQR) vs. 31.4 pg/ml (28.1–39.4 IQR), respectively, p = 0.001] (Supplementary Table 3). At univariate regression model, unitary increase in basal 1,25(OH)2D levels was associated with 11% risk reduction for developing WRF [OR 0.89 (95% CI 0.86–0.93), p = 0.006] (Table 3). Higher 1,25(OH)2D/PTH (1–84) ratio was associated with 84% risk reduction for reaching the renal outcome at the limit of statistical significance [OR 0.16 (95% CI 0.06–0.41), p = 0.05]. Basal 1,25(OH)2D levels leq 29.0 pg/ml resulted moderately sensitive (86.7%) and poorly specific (66%) in predicting WRF in the whole cohort (p = 0.0002) (Table 4). Notably, sensitivity reached 100% together with mild improvement in specificity among patients with higher eGFR and lower age. On the contrary, 1,25(OH)2D was unreliable in predicting WRF in CKD stage 4 to 5. Basal eGFR di not predict WRF in the whole and the stratified cohorts (Table 4).
ML model, limited to standard clinical data, had a MAPE of 29.1% in predicting 3-months eGFR (Figure 2). MAPE reduced by 3.8% after inclusion of bone and mineral markers. The 1,25(OH)2D/PTH (1–84) ratio was retained as informative factor on renal outcome with the stronger weight, only second to eGFR independently from albuminuria. MAPE was even lower in patients younger than 70 years (MAPE = 23.2%) and with eGFR ≥ 30 ml/min/1.73 m2 (MAPE = 23.9%) (data not shown).
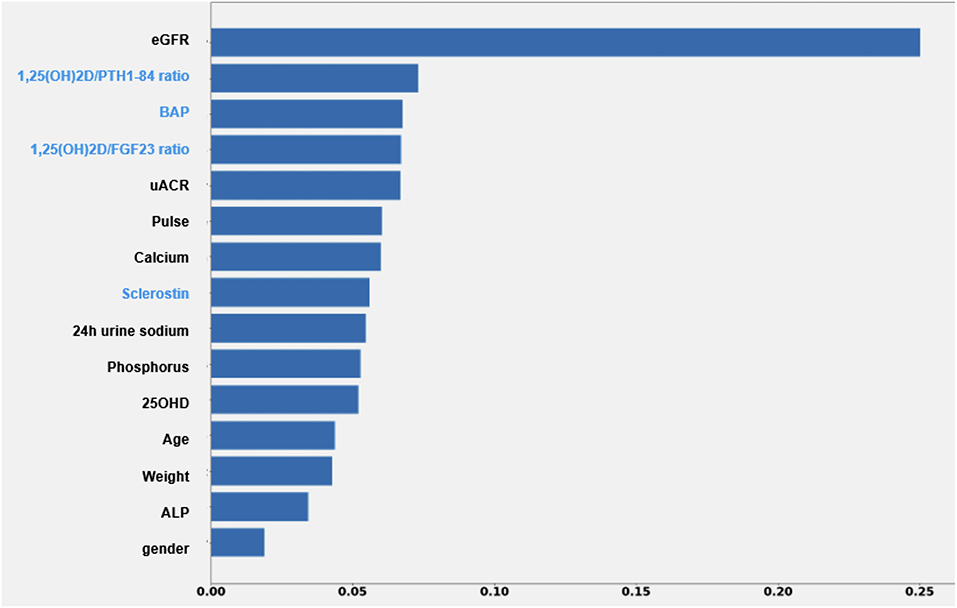
Figure 2. Impact of each feature of the XGBoost model on the prediction of eGFR value after 3 months. Each importance score attempts to quantify the relative importance of each feature in the prediction of the output variable. ALP, alkaline phosphatase; BAP, bone specific alkaline phosphatase; eGFR, estimated glomerular filtration rate; UACR, urine albumin creatinine ratio.
Renal scintigraphy was performed in 69 (97%) patients. Measured GFR was linearly correlated with both eGFR and PTH (1–84) (Figure 3). The strength of correlation was attenuated among elderly patients. No correlation was detected between 1,25(OH)2D, mGFR, and mineral parameters at baseline (Figure 3, Supplementary Figure 1). Moderate correlation was observed between GFR and mineral parameters in patients aged ≤ 70 years.
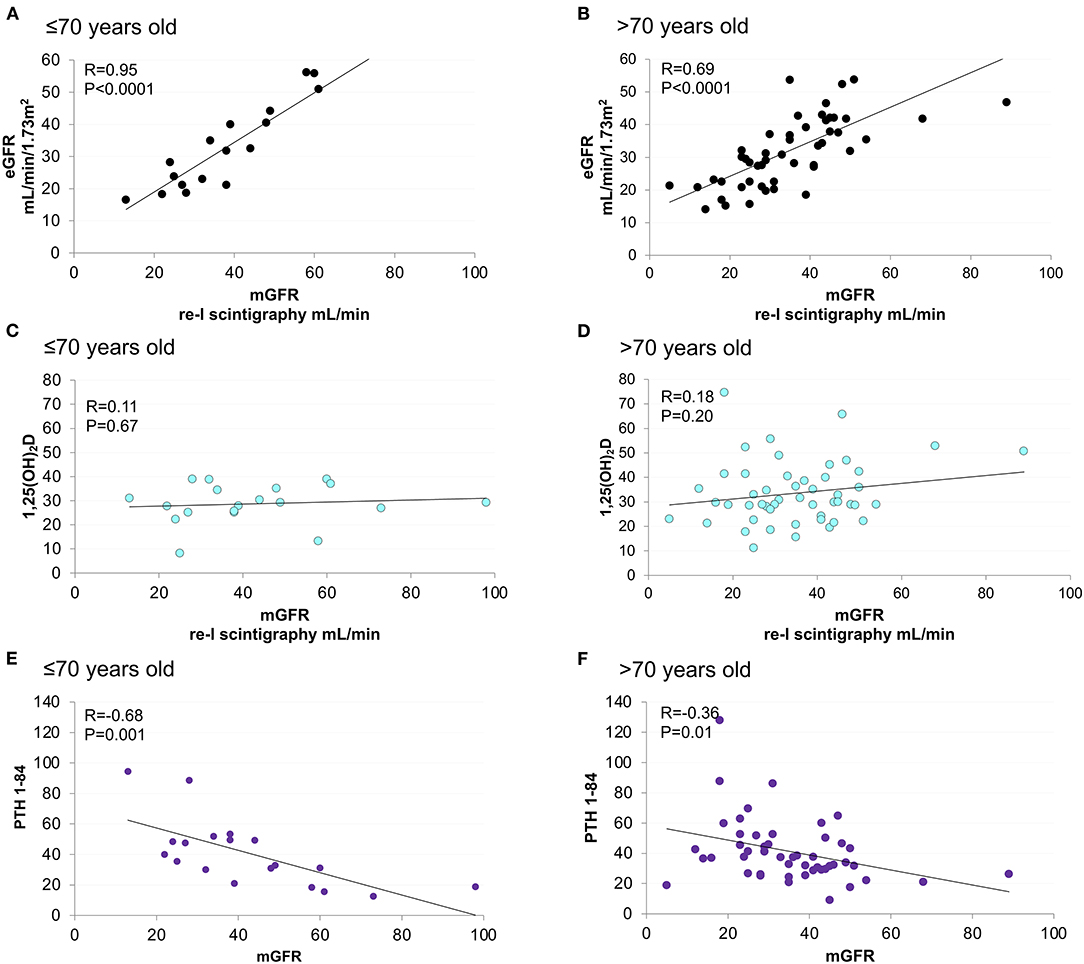
Figure 3. Linear correlation between mGFR and eGFR, 1,25(OH)2D and PTH (1–84), stratified according to age. Age < = 70 years old figures (A,C,E). Age > 70 years old figures (B,D,F).
At 6-month iFGF-23, and sclerostin significantly increased, while BSAP, 1,25(OH)2D/PTH (1–84) and 1,25(OH)2D/iFGF-23 ratios were reduced (Table 5, Supplementary Table 4). Sclerostin increase was more pronounced in patients undergoing vitamin D therapy (Supplementary Table 4).
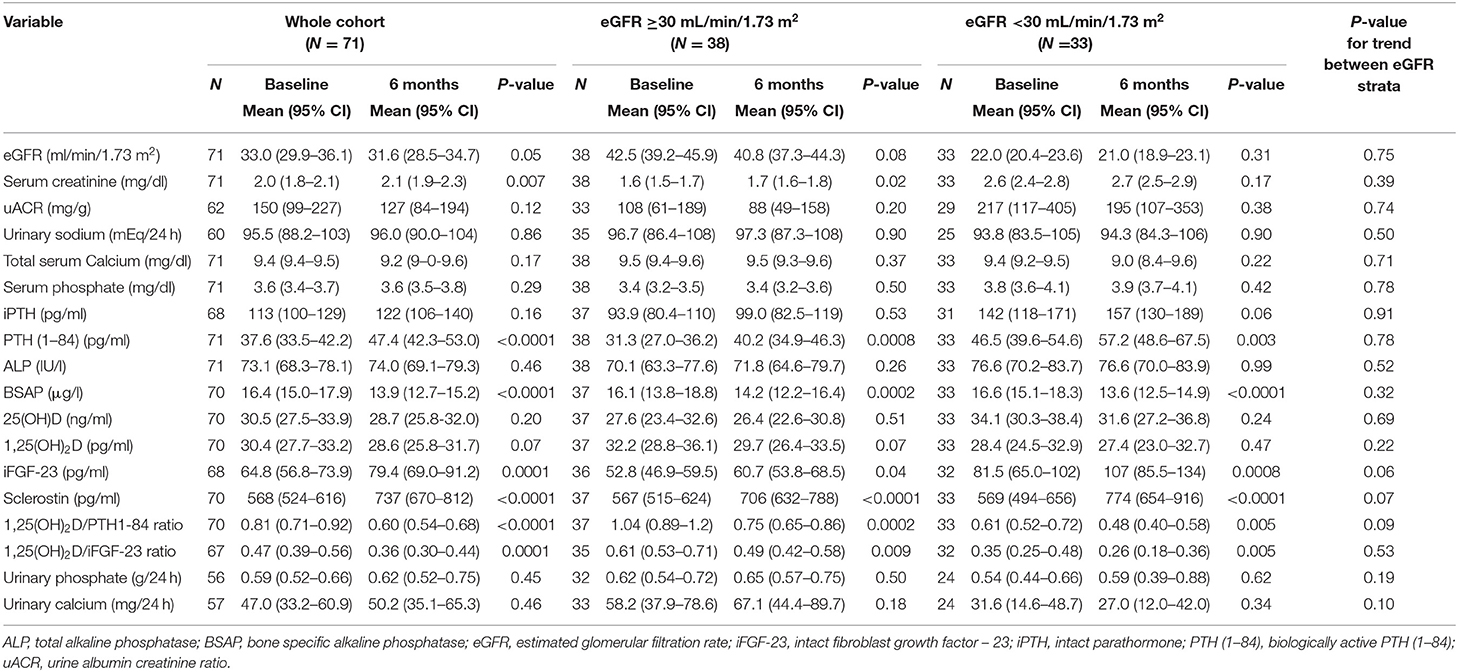
Table 5. Changes in renal and mineral parameters between baseline and 6-months follow up, stratified according to basal eGFR.
Discussion
CKD diagnosis and staging are currently based on eGFR and uACR (6, 40). However, eGFR and uACR are informative on glomerular rather than on tubular function/injury, which has been linked to the risk of CKD progression (41–43). Furthermore, tubular fibrosis was not associated with GFR, limiting its predictability by glomerular markers (44).
A growing body of evidence supports new biomarkers of tubular damage as independent predictors of WRF (12, 15, 16, 45, 46). Few molecules were classified as reliable markers of tubular injury, inflammation/fibrosis and repair (14, 46–48). More recently, biomarkers of tubular reabsorption and reserve (UMOD) were added into the panel, and factorial analysis was applied for aggregating single markers into clusters, based on their pathophysiologic role and cumulative association with clinical outcomes (13, 48, 49). FGF-23 and PTH were finally included into a single tubule reserve/mineral metabolism factor, together with UMOD, as biomarkers of tubular response to hormones (13, 49). Post-hoc analysis of the SPRINT trial observed that UMOD, FGF-23, PTH factor was associated with faster WRF independently from basal eGFR and albuminuria (13). The same factor was associated with increased risk of cardiovascular disease and heart failure in the same cohort (49). Hence, non-invasive tubular health assessment, including biomarkers of structural damage and cellular function, was suggested as important tool for better stratifying the risk of CKD progression (47).
Several characteristics may link UMOD to 1,25(OH)2D as protective biomarkers of tubular health. UMOD, otherwise known as Tamm-Horsfall protein, is expressed in the thick ascending limb of the loop of Henle and early distal convoluted tubule (50). UMOD is supposed to protect renal tubules from kidney stone formation (51), urinary tract infection (52), and ischemia (53). Higher UMOD levels were associated with lower risk of kidney function decline (14, 45, 50, 54) and were suggested as biomarker of tubular mass (45) and tubular pathology (55). Active vitamin D is synthesized in renal tubule, and deserves protective functions against inflammatory, fibrotic and oxidative insults (56). Low levels of 1,25(OH)2D trigger SHPT while VDRA administration improved albuminuria in experimental and clinical studies (57, 58). Hence, the pathophysiology of SHPT, the relevance of tubular health in CKD and the renal protection elicited by VDR activation support 1,25(OH)2D as both marker of tubular synthesis and favorable predictor of WRF, nonetheless responsive to adaptive hormonal feed-backs in the context of CKD-MBD. However, predictability of WRF by serum 1,25(OH)2D remains unexplored up to date.
In the PASCaL-1,25D study 1,25(OH)2D levels were not correlated with mGFR at baseline (Figure 2). However, higher 1,25(OH)2D was associated with 11% risk reduction in 6-months WRF at univariate analysis (p = 0.006) (Table 3). Renal outcome was not associated with any other variable. Furthermore, basal 1,25(OH)2D lower than 29.0 pg/ml was highly sensitive in predicting WRF in the whole cohort, reaching 100% sensitivity in younger patients and in those with better renal function at study entry (Table 4). Unfortunately, independency of association was impossible to be tested by multivariate regression models due to low rate of renal events.
Assessing biologically active PTH is relevant to analyze the clinical impact of mineral derangement in CKD. However, the most commonly used second-generation PTH assay, erroneously called iPTH, detects both the full-length biologically active PTH (1–84) and the large C-terminal PTH fragments, otherwise named “non-(1–84) PTH” (24). C-terminal PTH fragments accumulate in CKD (59), where they account for up to 50% of PTH levels assessed by second-generation assay (60) and participate to PTH resistance mediated by uremic toxins (61). On the contrary, third-generation assays detects biologically active PTH (1–84) (62). Compared with iPTH, biologically active PTH (1–84) was commonly 50–70% lower and more reliably associated with bone health in renal patients (63). However, PTH (1–84) is not routinely assessed in clinical practice, and its association with 1,25(OH)2D has been minimally investigated.
In the present study baseline PTH (1–84) was 2–4 times lower than iPTH (Table 1). Of note, the rate of 20% increase in PTH levels was descriptively higher when referred to PTH (1–84) (56%) compared with iPTH (41%) independently from eGFR strata (Table 1). Although 1,25(OH)2D and PTH were not correlated at baseline (Supplementary Figure 1), 1,25(OH)2D independently predicted PTH (1–84) (but not iPTH) increase at 6 months (Table 2, Supplementary Table 2), suggesting that lower 1,25(OH)2D levels may help in recognizing patients at increased risk of progression toward biologically-relevant SHPT.
Vitamin D deficiency and SHPT were associated with the risk of CKD progression in observational studies (64, 65). SHPT alters bone turnover and mineralization, and participates to vascular and renal aging, mediated by P and FGF-23 toxicity (66). Based on physiology driven approach, the lower the 1,25(OH)2D and the higher the biologically active PTH, the most maladaptive could be the endocrine response to CKD-MBD. The 1,25(OH)2D/PTH ratio was previously tested as negative predictor of CKD progression in post-hoc analysis from the GISSI-HF and CanPREDDICT studies. The GISSI-HF trial was a randomized control study, conducted among 6.975 patients with stable heart failure (67). The study included middle aged patients (66.8 ± 10.8 years) with mild to moderate CKD (eGFR 68.6 + 23.5 ml/min/1.73 m2) (29). In post-hoc analysis by Masson et al. (29) 335 (29.6%) experienced WRF, defined as ≥0.3 mg/dl and ≥25% increase in sCr at two consecutive study visits during 60-month follow up. Lower 1,25(OH)2D/PTH (1–84) ratio was independently associated with 25 and 35% risk reduction for the renal outcome and all-cause mortality, respectively. Notably, a weaker association was detected when taking 1,25(OH)2D and PTH (1–84) as individual predictors. In the present study older age, stronger severity of CKD, SHPT, and 1,25(OH)2D depletion may account for significant association between WRF and 1,25(OH)2D taken as single predictor. The observational CanPREDDICT study was conducted in Canada among CKD stage 3–4 patients (68) with demographic and renal characteristics comparable with the PASCaL-1,25D study. In the post-hoc analysis, conducted by Levin A. et al. among 1.784 patients, lower 1,25(OH)2D and lower 1,25(OH)2D/PTH (1–84) ratio were associated with 50% decline in eGFR over 5-years follow up independently from albuminuria (30).
In the PASCAL-1,25D study 1,25(OH)2D/PTH (1–84) ratio was significantly lower in patients with eGFR <30 ml/min/1.73 m2 at baseline, mainly attributable to higher PTH (1–84) levels (Table 1). Higher 1,25(OH)2D/PTH (1–84) ratio was associated with 84% reduction in the risk for WRF at the limit of statistical significance at univariate analysis (Table 3). Although regression models did not retain albuminuria and eGFR as predictors of renal outcome, ML analysis selected 1,25(OH)2D/PTH (1–84) as informative factor on 3-months eGFR prediction with the best relevance only secondary to the baseline eGFR. Albuminuria was retained in the same model, although with mildly lower weight compared with 1,25(OH)2D/PTH (1–84) ratio (Figure 2). The small sample size, the low rate of renal events and the shortness of follow up in the PASCAL-1,25D study may partially explain discrepant results obtained by classic statistics compared with ML approach.
Interpretation of biomarkers must be contextualized to clinical scenarios and patients' characteristics (48). Although elderly accounted for more than 70% of the entire cohort, younger patients deserved the strongest association between mineral and glomerular biomarkers (Figure 3, Supplementary Figure 1) as the more accurate predictability of WRF by 1,25(OH)2D (Table 4). This reminds the urgent need of further studies to investigate peculiarities of CKD-MBD in the elderly (69).
Several limitations hampered the PASCaL-1,25D study, including small sample size, short follow-up, low achievement of renal outcome, uncontrolled vitamin D administration, and unavailable assessment of other tubule biomarkers and oxidated PTH (25). Furthermore, comparisons between present data and post-hoc analysis from the SPRINT trial must be taken cautiously, due to exclusion of patients with diabetes and proteinuria > 1 g/24 h from that study (70). Present data remain underpowered to orient decisions on vitamin D therapy in CKD.
In conclusion, PASCaL-1,25D study suggests 1,25(OH)2D as a promising biomarker of tubular health, and sensible predictor of WRF on the short run among non-elderly patients affected by stage-3 CKD. The 1,25(OH)2D/PTH (1–84) ratio might represent a composite biomarker of tubular reserve/endocrine response to the transition from adaptive to maladaptive equilibrium in CKD-MBD. The aforesaid conclusions must be taken as hypothetical, in expectance of validation by further research.
Data Availability Statement
The raw data supporting the conclusions of this article will be made available by the authors, without undue reservation.
Ethics Statement
The studies involving human participants were reviewed and approved by Comitato Etico Interaziendale Milano Area A. The patients/participants provided their written informed consent to participate in this study.
Author Contributions
AG and MGC: concept, methodology, supervision, and editing. EF and RC: patient enrolment and data collection. MAP, CM, and PC: data analysis. FB and CZ: data analysis, writing original draft, and editing. FAB: writing original draft and editing. MC: data curation and project administration. All authors contributed to the article and approved the submitted version.
Funding
This study was funded and supported by DiaSorin SpA manufacturer of bone and mineral biomarker assays.
Conflict of Interest
FB, MC, and FAB are employees of DiaSorin the manufacturer of the LIAISON® bone and mineral tests. CZ is an independent consultant to DiaSorin. MGC has received consulting fees from DiaSorin in the past for advisory board membership. AG and MGC have received speaking honoraria from Abbvie, Amgen, Shire, and Vifor Fresenius. CM and MAP are employed by Reply Data.
The remaining authors declare that the research was conducted in the absence of any commercial or financial relationships that could be construed as a potential conflict of interest.
Publisher's Note
All claims expressed in this article are solely those of the authors and do not necessarily represent those of their affiliated organizations, or those of the publisher, the editors and the reviewers. Any product that may be evaluated in this article, or claim that may be made by its manufacturer, is not guaranteed or endorsed by the publisher.
Acknowledgments
We thank all of the patients who participated in the study, and the coordinators for data and sample collection, as well Luisa Facheris for trial organization, and Jeremy Seeman for performing the sample testing on the DiaSorin analyzer platforms. In addition, we would like to thank Douglas M. Hawkins for assistance with statistical analyses.
Supplementary Material
The Supplementary Material for this article can be found online at: https://www.frontiersin.org/articles/10.3389/fmed.2022.840801/full#supplementary-material
References
1. Saran R, Robinson B, Abbott KC, Bragg-Gresham J, Chen X, Gipson D, et al. US renal data system 2019 annual data report: epidemiology of kidney disease in the United States. Am J Kidney Dis. (2020) 75(1 Suppl. 1):A6–7. doi: 10.1053/j.ajkd.2019.09.003
2. Li PK, Garcia-Garcia G, Lui SF, Andreoli S, Fung WW, Hradsky A, et al. Kidney health for everyone everywhere: from prevention to detection and equitable access to care. J Nephrol. (2020) 33:201–10. doi: 10.1159/000506966
3. Brown EA, Zhao J, McCullough K, Fuller DS, Figueiredo AE, Bieber B, et al. Burden of kidney disease, health-related quality of life, and employment among patients receiving peritoneal dialysis and in-center hemodialysis: findings from the DOPPS Program. Am J Kidney Dis. (2021) 78:489–500 e1. doi: 10.1053/j.ajkd.2021.02.327
4. Ku E, Johansen KL, McCulloch CE. Time-centered approach to understanding risk factors for the progression of CKD. Clin J Am Soc Nephrol. (2018) 13:693–701. doi: 10.2215/CJN.10360917
5. Chen TK, Knicely DH, Grams ME. Chronic kidney disease diagnosis and management: a review. JAMA. (2019) 322:1294–304. doi: 10.1001/jama.2019.14745
6. Kidney Disease: Improving Global Outcomes (KDIGO) CKD Work Group. KDIGO 2012 clinical practice guideline for the evaluation and management of chronic kidney disease. Kidney Int Soppl. (2013) 3:1–150. doi: 10.1038/kisup.2012.73
7. Porrini E, Ruggenenti P, Luis-Lima S, Carrara F, Jimenez A, de Vries APJ, et al. Estimated GFR: time for a critical appraisal. Nat Rev Nephrol. (2019) 15:177–90. doi: 10.1038/s41581-018-0080-9
8. Kramer HJ, Nguyen QD, Curhan G, Hsu CY. Renal insufficiency in the absence of albuminuria and retinopathy among adults with type 2 diabetes mellitus. JAMA. (2003) 289:3273–7. doi: 10.1001/jama.289.24.3273
9. Kassirer JP. Clinical evaluation of kidney function–tubular function. N Engl J Med. (1971) 285:499–502. doi: 10.1056/NEJM197108262850906
10. Striker GE, Schainuck LI, Cutler RE, Benditt EP. Structural-functional correlations in renal disease. I A method for assaying and classifying histopathologic changes in renal disease. Hum Pathol. (1970) 1:615–30. doi: 10.1016/S0046-8177(70)80060-0
11. Risdon RA, Sloper JC, De Wardener HE. Relationship between renal function and histological changes found in renal-biopsy specimens from patients with persistent glomerular nephritis. Lancet. (1968) 2:363–6. doi: 10.1016/S0140-6736(68)90589-8
12. Malhotra R, Katz R, Jotwani V, Ambrosius WT, Raphael KL, Haley W, et al. Urine markers of kidney tubule cell injury and kidney function decline in sprint trial participants with CKD. Clin J Am Soc Nephrol. (2020) 15:349–58. doi: 10.2215/CJN.02780319
13. Bullen AL, Katz R, Jotwani V, Garimella PS, Lee AK, Estrella MM, et al. Biomarkers of kidney tubule health, CKD progression, and acute kidney injury in SPRINT (systolic blood pressure intervention trial) participants. Am J Kidney Dis. (2021) 783:61–8. doi: 10.1053/j.ajkd.2021.01.021
14. Bullen AL, Katz R, Lee AK, Anderson CAM, Cheung AK, Garimella PS, et al. The SPRINT trial suggests that markers of tubule cell function in the urine associate with risk of subsequent acute kidney injury while injury markers elevate after the injury. Kidney Int. (2019) 96:470–9. doi: 10.1016/j.kint.2019.03.024
15. Greenberg JH, Abraham AG, Xu Y, Schelling JR, Feldman HI, Sabbisetti VS, et al. Plasma biomarkers of tubular injury and inflammation are associated with CKD progression in children. J Am Soc Nephrol. (2020) 31:1067–77. doi: 10.1681/ASN.2019070723
16. Schrauben SJ, Shou H, Zhang X, Anderson AH, Bonventre JV, Chen J, et al. Association of multiple plasma biomarker concentrations with progression of prevalent diabetic kidney disease: findings from the chronic renal insufficiency cohort (CRIC) study. J Am Soc Nephrol. (2021) 32:115–26. doi: 10.1681/ASN.2020040487
17. Galassi A, Ciceri P, Porata G, Iatrino R, Boni Brivio G, Fasulo E, et al. Current treatment options for secondary hyperparathyroidism in patients with stage 3 to 4 chronic kidney disease and vitamin D deficiency. Expert Opin Drug Saf. (2021) 20:1333–49. doi: 10.1080/14740338.2021.1931117
18. Levin A, Le Barbier M, Er L, Andress D, Sigrist MK, Djurdjev O. Incident isolated 1,25(OH)(2)D(3) deficiency is more common than 25(OH)D deficiency in CKD. J Nephrol. (2012) 25:204–10. doi: 10.5301/JN.2011.8429
19. Gal-Moscovici A, Sprague SM. Role of vitamin D deficiency in chronic kidney disease. J Bone Miner Res. (2007) 22(Suppl. 2):V91–4. doi: 10.1359/jbmr.07s203
20. Gutierrez O, Isakova T, Rhee E, Shah A, Holmes J, Collerone G, et al. Fibroblast growth factor-23 mitigates hyperphosphatemia but accentuates calcitriol deficiency in chronic kidney disease. J Am Soc Nephrol. (2005) 16:2205–15. doi: 10.1681/ASN.2005010052
21. Levin A, Bakris GL, Molitch M, Smulders M, Tian J, Williams LA, et al. Prevalence of abnormal serum vitamin D, PTH, calcium, and phosphorus in patients with chronic kidney disease: results of the study to evaluate early kidney disease. Kidney Int. (2007) 71:31–8. doi: 10.1038/sj.ki.5002009
22. Galassi A, Cupisti A, Santoro A, Cozzolino M. Phosphate balance in ESRD: diet, dialysis and binders against the low evident masked pool. J Nephrol. (2015) 28:415–29. doi: 10.1007/s40620-014-0142-4
23. Altieri B, Cavalier E, Bhattoa HP, Perez-Lopez FR, Lopez-Baena MT, Perez-Roncero GR, et al. Vitamin D testing: advantages and limits of the current assays. Eur J Clin Nutr. (2020) 74:231–47. doi: 10.1038/s41430-019-0553-3
24. Kritmetapak K, Pongchaiyakul C. Parathyroid hormone measurement in chronic kidney disease: from basics to clinical implications. Int J Nephrol. (2019) 2019:5496710. doi: 10.1155/2019/5496710
25. Hocher B, Zeng S. Clear the fog around parathyroid hormone assays: what Do iPTH assays really measure? Clin J Am Soc Nephrol. (2018) 13:524–6. doi: 10.2215/CJN.01730218
26. Valcour A, Zierold C, Blocki FA, Hawkins DM, Martin KJ, Rao SD, et al. Trueness, precision and stability of the LIAISON 1–84 parathyroid hormone (PTH) third-generation assay: comparison to existing intact PTH assays. Clin Chem Lab Med. (2018) 56:1476–82. doi: 10.1515/cclm-2018-0217
27. Valcour A, Zierold C, Podgorski AL, Olson GT, Wall JV, DeLuca HF, et al. A novel, fully-automated, chemiluminescent assay for the detection of 1,25-dihydroxyvitamin D in biological samples. J Steroid Biochem Mol Biol. (2016) 164:120–6. doi: 10.1016/j.jsbmb.2015.08.005
28. van Helden J, Weiskirchen R. Experience with the first fully automated chemiluminescence immunoassay for the quantification of 1alpha, 25-dihydroxy-vitamin D. Clin Chem Lab Med. (2015) 53:761–70. doi: 10.1515/cclm-2014-0698
29. Masson S, Barlera S, Colotta F, Magnoli M, Bonelli F, Moro M, et al. A low plasma 1,25(OH)2 vitamin D/PTH (1–84) ratio predicts worsening of renal function in patients with chronic heart failure. Int J Cardiol. (2016) 224:220–5. doi: 10.1016/j.ijcard.2016.09.014
30. Levin A, Ognjenka D., Mila T, et al. Ratios of parathyroid hormone, fibroblast growth factor 23, amd 1,25 dihydroxylvitamin D and CKD progression. J Am Soc Nephrol. (2017) 28:467.
31. O'Callaghan CA, Shine B, Lasserson DS. Chronic kidney disease: a large-scale population-based study of the effects of introducing the CKD-EPI formula for eGFR reporting. BMJ Open. (2011) 1:e000308. doi: 10.1136/bmjopen-2011-000308
32. Perrone RD, Steinman TI, Beck GJ, Skibinski CI, Royal HD, Lawlor M, et al. Utility of radioisotopic filtration markers in chronic renal insufficiency: simultaneous comparison of 125I-iothalamate, 169Yb-DTPA, 99mTc-DTPA, and inulin. The modification of diet in renal disease study. Am J Kidney Dis. (1990) 16:224–35. doi: 10.1016/S0272-6386(12)81022-5
33. Friedman J. Greedy function approximation: a gradient boosting machine. Ann Stat. (2001) 29:1189–232. doi: 10.1214/aos/1013203451
34. Pedregosa F VGGramfort, et al. Scikit-learn: machine learning in python. J Mach Learn Res. (2011) 12:2825–30.
36. XGboost developers. XGBoost Documentation 2021. Available online at: https://xgboost.readthedocs.io/en/stable/index.html (2021).
37. Probst P BA, Bischl B. Tunability: importance of hyper parameters of machine learning algorithms. J Mach Learn Res. (2018) 9:20:1–32.
38. Chen T, Xgboost GC. A scalable tree boosting system. Proceedings of the 2nd acm sigkdd interational conference on knowledge discovery and data mining. KDD 16. (2016):785–94. doi: 10.1145/2939672.2939785
40. Ix JH, Shlipak MG. The promise of tubule biomarkers in kidney disease: a review. Am J Kidney Dis. (2021) 78:719–27. doi: 10.1053/j.ajkd.2021.03.026
41. Nath KA. Tubulointerstitial changes as a major determinant in the progression of renal damage. Am J Kidney Dis. (1992) 20:1–17. doi: 10.1016/S0272-6386(12)80312-X
42. Howie AJ, Ferreira MA, Adu D. Prognostic value of simple measurement of chronic damage in renal biopsy specimens. Nephrol Dial Transplant. (2001) 16:1163–9. doi: 10.1093/ndt/16.6.1163
43. Ong AC, Fine LG. Loss of glomerular function and tubulointerstitial fibrosis: cause or effect? Kidney Int. (1994) 45:345–51. doi: 10.1038/ki.1994.44
44. Rule AD, Amer H, Cornell LD, Taler SJ, Cosio FG, Kremers WK, et al. The association between age and nephrosclerosis on renal biopsy among healthy adults. Ann Intern Med. (2010) 152:561–7. doi: 10.7326/0003-4819-152-9-201005040-00006
45. Steubl D, Buzkova P, Garimella PS, Ix JH, Devarajan P, Bennett MR, et al. Association of serum uromodulin with ESKD and kidney function decline in the elderly: the cardiovascular health study. Am J Kidney Dis. (2019) 74:501–9. doi: 10.1053/j.ajkd.2019.02.024
46. Gutierrez OM, Shlipak MG, Katz R, Waikar SS, Greenberg JH, Schrauben SJ, et al. associations of plasma biomarkers of inflammation, fibrosis, and kidney tubular injury with progression of diabetic kidney disease: a cohort study. Am J Kidney Dis. (2021). doi: 10.1053/j.ajkd.2021.09.018
47. Walther CP, Shah M, Navaneethan SD. Estimated GFR decline and tubular injury biomarkers with intensive blood pressure control. Am J Kidney Dis. (2019) 73:4–7. doi: 10.1053/j.ajkd.2018.09.008
48. Walther CP, Benoit JS. Tubular kidney biomarker insights through factor analysis. Am J Kidney Dis. (2021) 78:335–7. doi: 10.1053/j.ajkd.2021.03.016
49. Lee AK, Katz R, Jotwani V, Garimella PS, Ambrosius WT, Cheung AK, et al. Distinct dimensions of kidney health and risk of cardiovascular disease, heart failure, and mortality. Hypertension. (2019) 74:872–9. doi: 10.1161/HYPERTENSIONAHA.119.13339
50. Garimella PS, Katz R, Ix JH, Fried LF, Kritchevsky SB, Devarajan P, et al. Association of urinary uromodulin with kidney function decline and mortality: the health ABC study. Clin Nephrol. (2017) 87:278–86. doi: 10.5414/CN109005
51. Liu Y, Mo L, Goldfarb DS, Evan AP, Liang F, Khan SR, et al. Progressive renal papillary calcification and ureteral stone formation in mice deficient for Tamm-Horsfall protein. Am J Physiol Renal Physiol. (2010) 299:F469–78. doi: 10.1152/ajprenal.00243.2010
52. Serafini-Cessi F, Monti A, Cavallone D. N-Glycans carried by Tamm-Horsfall glycoprotein have a crucial role in the defense against urinary tract diseases. Glycoconj J. (2005) 22:383–94. doi: 10.1007/s10719-005-2142-z
53. El-Achkar TM, Wu XR, Rauchman M, McCracken R, Kiefer S, Dagher PC. Tamm-Horsfall protein protects the kidney from ischemic injury by decreasing inflammation and altering TLR4 expression. Am J Physiol Renal Physiol. (2008) 295:F534–44. doi: 10.1152/ajprenal.00083.2008
54. Steubl D, Block M, Herbst V, Nockher WA, Schlumberger W, Kemmner S, et al. Urinary uromodulin independently predicts end-stage renal disease and rapid kidney function decline in a cohort of chronic kidney disease patients. Medicine. (2019) 98:e15808. doi: 10.1097/MD.0000000000015808
55. Webster AC, Nagler EV, Morton RL, Masson P. Chronic Kidney Disease. Lancet. (2017) 389:1238–52. doi: 10.1016/S0140-6736(16)32064-5
56. Gembillo G, Cernaro V, Siligato R, Curreri F, Catalano A, Santoro D. Protective role of vitamin d in renal tubulopathies. Metabolites. (2020) 10:115. doi: 10.3390/metabo10030115
57. de Zeeuw D, Agarwal R, Amdahl M, Audhya P, Coyne D, Garimella T, et al. Selective vitamin D receptor activation with paricalcitol for reduction of albuminuria in patients with type 2 diabetes (VITAL study): a randomised controlled trial. Lancet. (2010) 376:1543–51. doi: 10.1016/S0140-6736(10)61032-X
58. de Borst MH, Hajhosseiny R, Tamez H, Wenger J, Thadhani R, Goldsmith DJ. Active vitamin D treatment for reduction of residual proteinuria: a systematic review. J Am Soc Nephrol. (2013) 24:1863–71. doi: 10.1681/ASN.2013030203
59. Brossard JH, Lepage R, Cardinal H, Roy L, Rousseau L, Dorais C, et al. Influence of glomerular filtration rate on non-(1-84) parathyroid hormone (PTH) detected by intact PTH assays. Clin Chem. (2000) 46:697–703. doi: 10.1093/clinchem/46.5.697
60. Brossard JH, Cloutier M, Roy L, Lepage R, Gascon-Barre M, D'Amour P. Accumulation of a non-(1–84) molecular form of parathyroid hormone (PTH) detected by intact PTH assay in renal failure: importance in the interpretation of PTH values. J Clin Endocrinol Metab. (1996) 81:3923–9. doi: 10.1210/jcem.81.11.8923839
61. Slatopolsky E, Finch J, Clay P, Martin D, Sicard G, Singer G, et al. A novel mechanism for skeletal resistance in uremia. Kidney Int. (2000) 58:753–61. doi: 10.1016/S0085-2538(15)47156-X
62. Gao P, Scheibel S, D'Amour P, John MR, Rao SD, Schmidt-Gayk H, et al. Development of a novel immunoradiometric assay exclusively for biologically active whole parathyroid hormone 1–84: implications for improvement of accurate assessment of parathyroid function. J Bone Miner Res. (2001) 16:605–14. doi: 10.1359/jbmr.2001.16.4.605
63. O'Flaherty D, Sankaralingam A, Scully P, Manghat P, Goldsmith D, Hampson G. The relationship between intact PTH and biointact PTH (1-84) with bone and mineral metabolism in pre-dialysis chronic kidney disease (CKD). Clin Biochem. (2013) 46:1405–9. doi: 10.1016/j.clinbiochem.2013.06.023
64. Ravani P, Malberti F, Tripepi G, Pecchini P, Cutrupi S, Pizzini P, et al. Vitamin D levels and patient outcome in chronic kidney disease. Kidney Int. (2009) 75:88–95. doi: 10.1038/ki.2008.501
65. Bozic M, Diaz-Tocados JM, Bermudez-Lopez M, Forne C, Martinez C, Fernandez E, et al. Independent effects of secondary hyperparathyroidism and hyperphosphatemia on chronic kidney disease progression and cardiovascular events: an analysis from the NEFRONA cohort. Nephrol Dial Transplant. (2021). doi: 10.1093/ndt/gfab184
66. Gutierrez OM. Fibroblast growth factor 23, Klotho, and disordered mineral metabolism in chronic kidney disease: unraveling the intricate tapestry of events and implications for therapy. J Ren Nutr. (2013) 23:250–4. doi: 10.1053/j.jrn.2013.01.024
67. Tavazzi L, Maggioni AP, Marchioli R, Barlera S, Franzosi MG, Latini R, et al. Effect of n-3 polyunsaturated fatty acids in patients with chronic heart failure (the GISSI-HF trial): a randomised, double-blind, placebo-controlled trial. Lancet. (2008) 372:1223–30. doi: 10.1016/S0140-6736(08)61239-8
68. Levin A, Rigatto C, Brendan B, Madore F, Muirhead N, Holmes D, et al. Cohort profile: Canadian study of prediction of death, dialysis and interim cardiovascular events (CanPREDDICT). BMC Nephrol. (2013) 14:121. doi: 10.1186/1471-2369-14-121
69. Galassi A, Ciceri P, Fasulo E, Carugo S, Cianciolo G, Cozzolino M. Management of secondary hyperparathyroidism in chronic kidney disease: a focus on the elderly. Drugs Aging. (2019) 36:885–95. doi: 10.1007/s40266-019-00696-3
Keywords: CKD, 1, 25-dihydroxyvitamin D, PTH, PTH (1–84), vitamin D, tubular biomarkers, CKD-MBD
Citation: Galassi A, Fasulo EM, Ciceri P, Casazza R, Bonelli F, Zierold C, Calleri M, Blocki FA, Palmieri MA, Mastronardo C and Cozzolino MG (2022) 1,25-dihydroxyvitamin D as Predictor of Renal Worsening Function in Chronic Kidney Disease. Results From the PASCaL-1,25D Study. Front. Med. 9:840801. doi: 10.3389/fmed.2022.840801
Received: 21 December 2021; Accepted: 07 February 2022;
Published: 02 March 2022.
Edited by:
Claudia Torino, Italian National Research Council, ItalyReviewed by:
Matthias Zeiler, C. e G. Mazzoni Hospital, ItalyMassimo Torreggiani, Centre Hospitalier Le Mans, France
Copyright © 2022 Galassi, Fasulo, Ciceri, Casazza, Bonelli, Zierold, Calleri, Blocki, Palmieri, Mastronardo and Cozzolino. This is an open-access article distributed under the terms of the Creative Commons Attribution License (CC BY). The use, distribution or reproduction in other forums is permitted, provided the original author(s) and the copyright owner(s) are credited and that the original publication in this journal is cited, in accordance with accepted academic practice. No use, distribution or reproduction is permitted which does not comply with these terms.
*Correspondence: Andrea Galassi, andrea.galassi@asst-santipaolocarlo.it