- 1Instituto de Ciências da Atividade Física e Esportes, Universidade Cruzeiro do Sul, São Paulo, Brazil
- 2Seção de Produção de Imunobiológicos, Centro Bioindustrial, Instituto Butantan, São Paulo, Brazil
Coronavirus disease 2019 (COVID-19) features hyper-inflammation, cytokine storm, neutrophil function changes, and sodium chloride (NaCl) homeostasis disruption, while the treatment with NaCl hypertonic solutions (HS) controls electrolytic body homeostasis and cell functions. HS treatment is a simple, popular, economic, and feasible therapy to regulate leukocyte function with a robust anti-inflammatory effect in many inflammatory diseases. The purpose of this narrative review is to highlight the knowledge on the use of HS approaches against viral infection over the past years and to describe the mechanisms involved in the release of neutrophil extracellular traps (NETs) and production of cytokine in severe lung diseases, such as COVID-19. We reported the consequences of hyponatremia in COVID-19 patients, and the immunomodulatory effects of HS, either in vitro or in vivo. We also described the relationship between electrolyte disturbances and COVID-19 infection. Although there is still a lack of clinical trials, hypertonic NaCl solutions have marked effects on neutrophil function and NETs formation, emerging as a promising adjuvant therapy in COVID-19.
Introduction
The World Health Organization (WHO) determined the severe acute respiratory syndrome coronavirus 2 (SARS-CoV-2) disease (COVID-19) as a pandemic in March 2020. Even after efficient vaccines are made available, the new variants of SARS-CoV-2 may reduce their effectiveness, and COVID-19 caused by different variants can still affect the respiratory tract (1).
COVID-19 patients have also been reported to have superinfections and coinfections with SARS-CoV-2 (2) and a recent meta-analysis found coinfections (19%) and superinfections (24%) of these patients, both being related to the risk of higher mortality (3). In this sense, superinfections and respiratory coinfections in SARS-CoV-2-positive patients were more prevalent in critically ill COVID-19 patients (4) and Paolucci et al. found a correlation between elevated viral load (especially Epstein-Barr virus) and lymphopenia, which demonstrates the relationship between viral prevalence and immunosuppression (5). Thus, alternative practices are needed to prevent hyper-inflammation and the respective worsening of severe respiratory illness in COVID-19 patients.
The vaccine minimizes the risk of coronavirus infections and reduces the severity of the disease, while the heterologous and homologous sera treatments block the virus. In general, antivirals block the activity of a specific enzyme/protein needed for the virus to replicate. In the chronology of disease, vaccine administration must be before contamination, thus, treatment with serums is effective in the high viral load.
The evolution of COVID-19 to lung inflammation results in severe cases. At pulmonary complications, anti-inflammatory drugs (cortisone/dexamethasone) are administrated to patients and, theoretically, in a critical inflammatory period, hypertonic saline could be a potential adjuvant therapy. Hypertonic sodium chloride solution (HS) has hemodynamic and electrolyte homeostasis maintenance properties. It improves blood viscosity, causes fast distension of intravascular volume, and decreases endothelial and tissue edema (6, 7). HS also regulates leukocyte function and exhibits an anti-inflammatory effect to improve inflammatory disease conditions such as sepsis and cystic fibrosis (8, 9).
Sputum induction by HS inhalation has been suggested for use in chronic obstructive pulmonary disease (10), while HS inhalation enhances the effectiveness of respiratory physiotherapy in patients with bronchiectasis (11). HS also stimulates mucociliary clearance in healthy individuals and patients with asthma, bronchiectasis, and cystic fibrosis (12, 13).
Recent studies reported that hyponatremia is associated with poor outcomes in COVID-19 patients as an independent predictor of in-hospital mortality (14, 15). Kimura et al. studied outpatients with COVID-19 without acute respiratory distress syndrome, one of the leading causes of mortality in COVID-19 patients, and suggested considerable symptom resolution with HS administration (16). However, they only enrolled patients who could self-isolate and perform irrigation in a bathroom separate from other household contacts and similar precautions would need to be taken by any COVID-19 patient considering this type of intervention in other studies. It is also worth noting that their study had a reduced number of participants (only 14 participants in the hypertonic saline group; 16). Therefore, despite the positive HS effects in other inflammatory diseases (17, 18), it has not been used in COVID-19 patients, and the mechanisms by which HS regulates mucociliary clearance and neutrophil functions remain unknown. We explored how HS modulates leukocyte function and attenuates severe respiratory illness aggravation in COVID-19 patients. We also discuss how HS may regulate neutrophil functions and NETosis formation in the interaction with SARS-CoV-2.
Discussion
Hyponatremia in Coronavirus Diseases 2019 Patients and Hypertonic Solution Administration Approaches
The infection of human cells by SARS-CoV-2 occurs through virus Spike protein binding to angiotensin I-converting enzyme 2 (ACE2) receptor (19). ACE-2 is one of the major anti-regulatory proteins of the main axis of the renin-angiotensin system (RAS), a fundamental determinant for the regulation of electrolyte balance and blood pressure. The binding of SARS-CoV-2 to ACE2 results in increased angiotensin II activity, as well as inflammation, and reduces the counter-response of ACE2 on RAS, which influences electrolyte control and elevates blood pressure (20). Moreover, approximately 60% of COVID-19 patients with watery diarrhea have moderate hyponatremia (21). As a result, SARS-CoV-2 infection promotes disturbances in the homeostasis of pH and electrolytes in vivo.
Hyponatremia is the most common electrolyte disturbance and, even when mild, is related to higher mortality (22). This electrolyte disorder is as high as 30% in inpatient settings (23). It is categorized in euvolemic, hypovolemic, and hypervolemic hyponatremia, each managed differently (24). Regarding severe acute respiratory syndrome (SARS), retrospective research in 77 medical, surgical, and mixed intensive care units showed that hyponatremia is described as an independent predictive indicator of poor outcomes in critically ill patients (25) and it is associated with poor prognosis (26). In addition to the impact on lung function, the clinical evolution of patients with COVID-19 can be unpredictable, leading to systemic complications and affecting different organs, as shown in Supplementary Table 1.
Direct endothelial cell infection by SARS-CoV-2 and the following endothelitis can induce platelet activation, high vascular permeability, raised thrombin generation, and reduced fibrinolysis, leading to a hypercoagulable state (27). In severe COVID-19, the high concentration of cytokines is related to systemic and local endothelial dysfunction and injury (28). In turn, endothelial activation induces downregulation of thrombomodulin expression, elevated tissue factor expression, and loss of heparin sulfate – all defensive mechanisms against thrombosis (29), contributing substantially to mortality and morbidity (30). Moreover, unregulated inflammatory markers production can induce blood-brain-barrier disruption, hence favoring the entry of cytokines, (or even SARS-CoV-2) into the central nervous system (31), promoting neurologic damage through either the complement or macrophages (32).
Extra-pulmonary manifestations such as gastrointestinal (GI) symptoms are also common in patients with COVID-19. Since ACE2 is expressed by various tissues, including epithelial cells of the GI tract (33), GI symptoms are usual in COVID-19 and the most ordinary GI presentation in COVID-19 patients is diarrhea, followed by vomiting and/or nausea and abdominal pain (34). Other usual GI symptoms reported in COVID-19 patients are anosmia, anorexia, and dysgeusia (35).
Since SARS-CoV-2 moves into the mucous membranes, it can access the biliary system through the portal vein. Thus, SARS-CoV-2 can induce direct immune injury to hepatocytes (cytopathic effect). In this sense, direct viral cytopathy with micro-vesicular steatosis, mild lobular, or portal implication has been observed in other studies (36). Elements that may influence the hepatic involvement in COVID-19 include exacerbated immune responses/systemic inflammatory response syndrome (37), direct viral cytopathic effects, hypoxia-induced alterations, endothelitis (38), vascular changes due to coagulopathy, and drug-induced liver injury (37). Regarding the severity, the prevalence of liver damage in severe COVID-19 cases (74.4%) was higher than that of patients with mild disease (43%), while the prevalence of liver injury in COVID-19-related deaths was 58% (39).
The etiology of hyponatremia seems to have multiple causes in COVID-19 patients, possibly including the syndrome of inappropriate secretion of antidiuretic hormone (SIADH) and digestive losses of sodium by vomiting or diarrhea (40). COVID-19 aggravation has been related to the reduction in potassium, calcium, and sodium serum levels (41, 42). Berni et al. (43) reported that hyponatremia is related to a more severe outcome in COVID-19 patients (43).
Hyponatremia may also be a consequence of the increased inflammatory biomarkers and interleukin (IL)-6 being one of the most relevant cytokines involved in COVID-19 infection (43). IL-6 may induce hyponatremia by causing vasopressin release (44). Besides, the proposed mechanisms for SIADH in COVID-19 patients involve inflammatory cytokine production (45). This issue requires prospective multicenter research and search for chronic underlying hyponatremia and evaluation of cytokines associated with urinary osmolarity to find out the mechanisms involved in the SIADH and COVID-19 linkage.
In vitro assays show that intracellular energy deprivation and membrane depolarization are potential pathways by which the HS usefully avoids virus replication. This possibility was recently raised by Machado et al., who studied non-human primate kidney cell line Vero and found that 1.2% NaCl restrained virus replication by 90%, achieving 100% restraining at mildly HS (1.5%). They also found that 1.1% NaCl was enough to restrain virus replication by 88% in human epithelial lung cell line Calu-3 (46).
In addition to the possible protection against viral replication, several NaCl solutions promote clear patient clinical improvements. HS improves the efficacy of respiratory physiotherapy in patients with bronchiectasis or cystic fibrosis (47, 48), stimulating cough (49), and restraining epithelial sodium channels (6).
Ho et al. investigated a case of SARS-CoV-2 induced SIADH manifesting as new-onset seizures using a pro-active desmopressin strategy (3% HS infusion with concomitant fluid restriction). On day 4, the authors found a clinical recovery with resolution and normalization of natremia. They suggested that high cytokine concentrations promote osmoregulation impairment, leading to hyponatremia (50). Nevertheless, this research is a case study without a control group and based on just one patient’s clinical and biochemical data. A randomized controlled trial (RCT) also reported the efficiency of HS (3, 2.5, 2.0, and 1.5%; nasal irrigation and gargle versus standard care) as therapy on adults within 48 h of the upper respiratory tract infection (URTI) onset. The authors found a reduction in the time of illness, transmission within household contacts, over-the-counter medications use, and viral shedding (51). Accordingly, the analysis from the Edinburgh and Lothians Viral Intervention Study RCT demonstrated that HS (gargling and nasal irrigation) decreases the time of URTI by an average of two-and-a-half days (52), however, since the results are a post hoc secondary analysis of data from a pilot RCT, they need to be interpreted with caution. In a systematic review, Singh et al. pointed out that HS with gargles and nasal wash may be beneficial in the prevention and care of COVID-19 patients (53).
Hypertonic Saline as an Immunomodulatory Agent
During SARS-CoV-2 infection, hyper-inflammation and pulmonary edema are the most worrying clinical conditions (54). COVID-19 in the severe phase presents a cytokine storm with increased plasma concentrations of tumor necrosis factor (TNF)-α, IL-1β, IL-2, IL-6, IL-7, IL-8, IL-10, IL-17, chemokine ligand 2 (CCL2), chemokine C-C motif ligand 3 (CCL3), granulocyte colony-stimulating factor (G-CSF), interferon (IFN)γ, and IFNγ-inducible protein 10 (55, 56). High plasma concentrations of cytokines and chemokines in patients with COVID-19 are associated with the aggravated state of the disease compared to non-severe patients (57). Huang et al. reported that COVID-19 patients in the intensive care unit, compared with non-intensive care unit patients, have increased plasma levels of CCL2, CCL3, interferon-inducible protein 10, TNF-α, IL-2, IL-7, IL-10, and G-CSF (58). Patients with COVID-19 and hyponatremia have a worse prognosis than individuals without electrolyte disbalance (59).
The reduction in cytokine production by leukocytes may be a supplementary beneficial effect of HS on the exacerbated immune response in COVID-19 patients. The HS modulates the expression and release of adhesion molecules, such as beta2-integrins and intercellular adhesion molecule (ICAM)-1, and cytokines (e.g., TNF and IL-10) in leukocytes (60–62).
Aerosolized HS elevates IL-8 release by cystic fibrosis gland cells via nuclear factor (NF)-κB pathway (63) and IL-8 expression via p38 mitogen-activated protein kinases in human bronchial epithelial cells (64). On a cellular level, the favorable outcomes of aerosolized HS were also reported regarding suppressing mTOR activity in mononuclear cells (65) and the decreased arachidonic acid leukotriene-B4-induced priming of the respiratory burst in neutrophils (66). Oreopoulos et al. reported that HS decreases TNF and increases IL-10 stimulated by lipopolysaccharide (LPS) at the gene expression level, independent of NF-κB signaling. HS treatment might exert its effects by independently modulating pro- and anti-inflammatory molecules, explaining the reduced degree of injury in multiple organs after HS administration (62).
The potential anti-inflammatory effects of HS are still unconcluded in humans. Paff et al. carried out a double-blind RCT study on the impact of HS inhalation (7%, twice daily) in 22 patients with primary ciliary dyskinesia. The authors evaluated inflammatory parameters [serum C-reactive protein, erythrocyte sedimentation rate, white blood cell count and cell differentiation, sputum cell differentiation, sputum neutrophil elastase (NE), IL-1β, IL-6, IL-8, IL-10, TNF-α, myeloperoxidase, IFN-α, and –β] and the quality of life (QoL) of the patients. The authors reported that the QoL-bronchiectasis health perception scale improved with HS. However, there was no alteration in the inflammatory measurements even after 12 weeks of treatment (67). Similarly, Aitken et al. did not find a reduction in IL-8 levels after sputum induction (3% HS at 5-time points over 20 min) in 10 clinically stable patients with cystic fibrosis (68). Elkins et al. also did not find differences in pro-inflammatory cytokines (IL-10, IL-6, IL-8, and TNFα) in the sputum of 164 patients with stable cystic fibrosis (7% HS inhaled twice daily) after 48 weeks of intervention (69). Nevertheless, it is essential to mention that all samples from Aitken et al. and Elkins et al. studies were in the post nebulization condition. There was no direct comparison between participants pre and post-nebulization (68, 69). In contrast, Reeves et al. found that HS reduces neutrophil chemotaxis and IL-8 levels in the sputum of 18 cystic fibrosis patients (nebulized 7% HS) compared to 14 non-cystic fibrosis control subjects, thereby assisting resolution of inflammation in the lower airways (18).
Consensus opinions of experts in hyponatremia by the Hyponatremia Treatment Guidelines (24) indicate that the treatment of hyponatremia depends on two factors: (a) etiology and (b) the volume status and comorbidities of the patient. Besides, usual saline therapies involve two different approaches: fluid restriction treatment and electrolytic substitution treatment. In the first scenario, the fluid limitation is necessary in the case of hyponatremia secondary to SIADH, and the HS administration may be associated in this case (depending on the stage of neurological impairment). This approach is recommended to prevent iatrogenic problems, such as lung injury aggravation secondary to SARS-COV-2 infection and pulmonary edema. On the other hand, general guidelines establish the beginning of electrolyte replacement treatment in the case of hypovolemic hyponatremia secondary to GI fluid losses and decreased fluid intake (24). Therefore, personalized pathophysiological judgment is crucial in this pandemic since there are no official clinical guidelines for treating hyponatremia in COVID-19 patients.
As discussed above, the ideal concentrations of NaCl solutions in the different stages of COVID-19 and the associative consequences of NaCl unbalance and clinical relevance in COVID-19 patients remains unestablished. The ongoing RCTs on COVID-19 and HS approaches are summarized in Table 1. All trials are being applied to adults (≥18) or older adults among the RCTs. Three studies (NCT04465604, NCT04382131, and NCT05104372) are with the status of “recruiting” and one study had the status of completed (NCT04755972), whereas two others are in the level of “not yet recruiting” (Table 1).
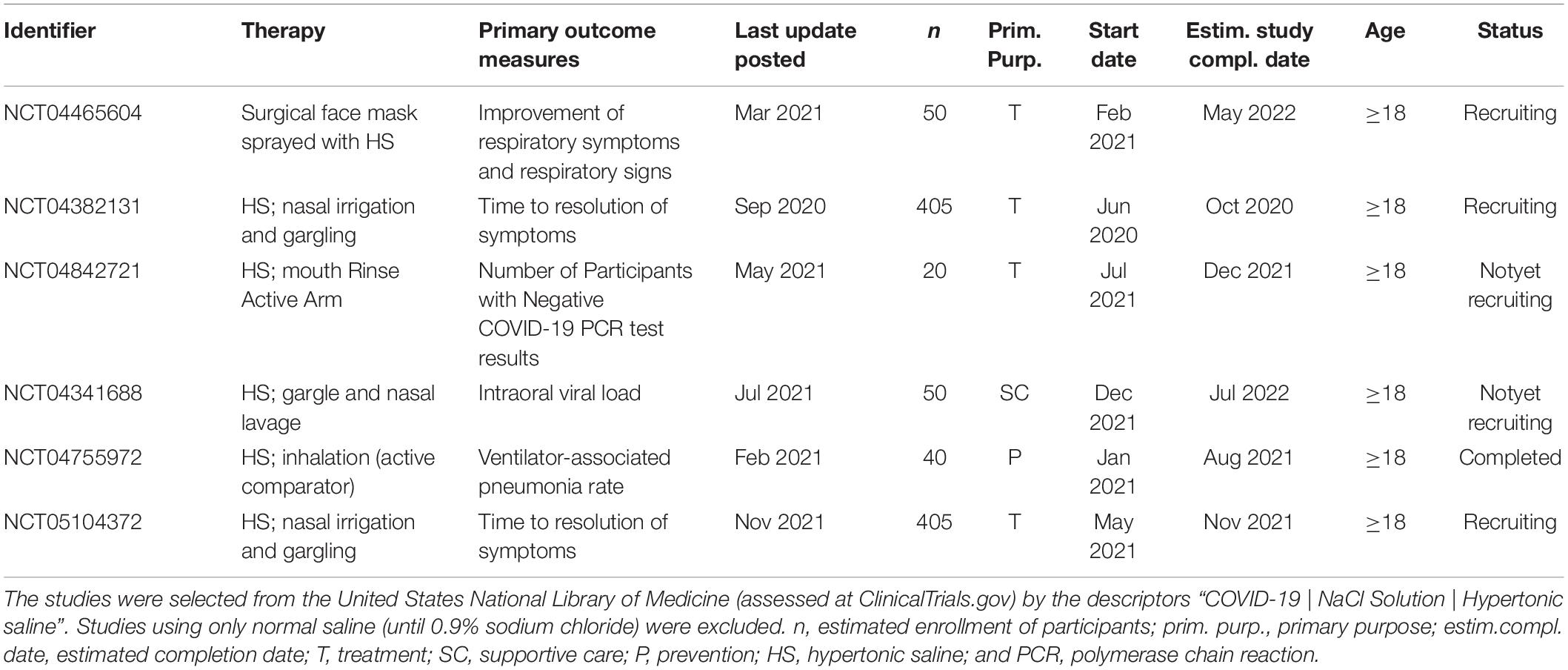
Table 1. Summary of ongoing randomized controlled trials that included hypertonic saline approaches in the COVID-19 treatment.
Hypertonic Saline and Neutrophils
Neutrophils’ altered responsiveness is likely to be a risk factor for severe COVID-19 considering increased mortality in the elderly, diabetic, and obese patients. These patients exhibit leukocyte dysfunction and chronic inflammation that predispose them to an excessive release of cytokines (70, 71). Impaired leukocyte function and reduced cell number are indicators of the progress from mild to severe clinical disease phases (72), and hyponatremia was recently associated with the high neutrophil count in SARS-CoV-2 patients (15).
The primary role of neutrophils in infections is the clearance of pathogens and debris through phagocytosis (73). The liberation of neutrophil-chemoattractant agents and the consequent recruitment of neutrophils is a vital host action against viral infection (74). Barnes et al. described an extensive lung infiltration of neutrophils in an autopsy specimen from a patient who succumbed to COVID-19 (75). Moreover, a high neutrophil-to-lymphocyte ratio indicates a poor prognosis for these patients (76).
Hypertonic solutions resuscitation has been reported as a potential strategy to decrease tissue damage and neutrophil activation in trauma patients (77), which mechanisms for neutrophil adhesion and sequestration vary with the inflammatory state. Chen et al. found that HS resuscitation has anti-inflammatory effects on panx1, CD39, CD73, and other ectonucleotidases. The authors also reported adenosine production induced by HS blocks neutrophil function through A2a receptors (78). Rizoli et al. investigated the effect of HS resuscitation on the progress of lung injury in a hemorrhagic shock model. They found suppression of LPS-stimulated activation and expression of CD11b. They demonstrated that CD11b integrin could be essential for neutrophil–endothelial interactions under the conditions studied (79). Reports also indicate that HS decreases lung injury by avoiding neutrophil adhesion to endothelium and suggests a mechanism for HS resuscitation. HS resuscitation decreases neutrophil margination by suppressing neutrophil L-selectin expression (80) and HS not only reduces post-shock mesenteric lymph release but also suppresses neutrophil priming by mesenteric lymph (81).
Hypertonic solutions may have clinical relevance by decreasing neutrophil-mediated intestinal damage. Tillinger et al. investigated HS treatment of neutrophils in vitro. They noted a dose-dependent effect involving decreased cell migration and the disruption of T84 monolayers compared with untreated control cells (82). Compared with physiological saline, Oreopoulos et al. found inhibition of ischemia/reperfusion-induced hepatic expression of ICAM-1 mRNA with HS from in vivo model of hepatic ischemia-reperfusion and in vitro model from the activated endothelial cell. The authors postulated hypertonicity minimizes neutrophil-mediated injury by regulating endothelial ICAM-1 expression (61).
Hatanaka et al. reported that hypertonic NaCl solution strongly inhibits LPS-mediated cytokines released by neutrophils and mononuclear cells in vitro (83). These findings were corroborated by research showing that the blockage of surface integrins or selectin molecules expression by HS prevents the accumulation of neutrophils in the sites of inflammation (17). Besides, HS inhibits neutrophil’s function regarding the expression of adhesion molecules (84), reactive oxygen species (ROS) production (7), neutrophil migration (85), and exocytosis (86). However, the mechanism by which HS stimulates mucociliary clearance is not fully clarified yet. In addition, investigations on the effects of hypertonic NaCl solution on neutrophil death and ROS production should be encouraged. Figure 1 illustrates the possible beneficial effects of hypertonic saline solution decreasing neutrophils’ hyperresponsiveness in the coronavirus disease.
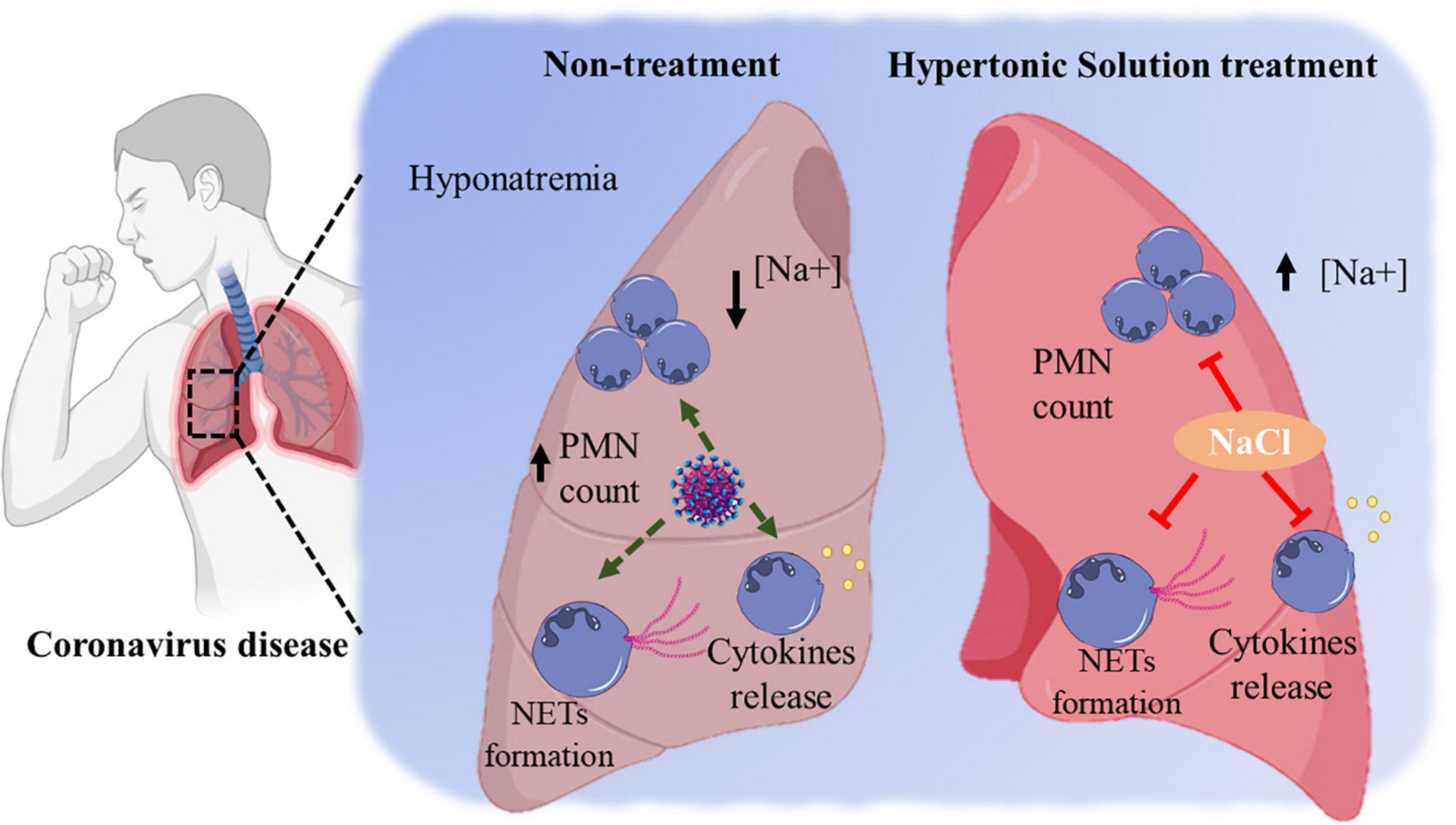
Figure 1. The possible beneficial effects of hypertonic saline solution decreasing neutrophils’ hyperresponsiveness in the coronavirus disease.
Neutrophil Extracellular Traps: A Promising Path?
Neutrophil extracellular traps (NETs) play a role in immune defense, autoimmunity, and sepsis (87). NETs are constituted of antimicrobial agents and decondensed chromatin, including myeloperoxidase (MPO) and NE, which capture and kill bacteria, parasites, and fungi (88). Considering the high production of ROS and the cytokine storm, COVID-19 cases can be greatly worsened by the tissue-damaging actions of NETs (89). Conditions closely associated with NETosis are coagulopathy, severe tissue damage, and barrier dysfunction of the lungs (90). For an in-depth look at the subject, Borges et al. previously described the mechanisms related to NETs formation in the pathophysiology of COVID-19 (91).
Hypertonic solutions induces water to come out from the cell, activating various cellular processes (92), such as dehydration of neutrophils that mitigate their role to restrain ROS and sequential NETosis. Nadesalingam et al. described that HS usually used in therapies (509 mM or 3% saline) restrains NOX2-dependent NETosis promoted by phorbol-12-myristate-13-acetate (PMA) and LPS. They also reported that the suppressive action of HS on NETosis is in part controlled by restraining liberation of ROS, which is mainly exerted by an elevation in osmolarity (93).
Myeloperoxidase has been closely associated with hyper-inflammation tissue damage (94). Although the role of MPO in SARS-CoV-2 is still uncertain, NaCl on MPO may be clinically associated with less severe conditions. Delgado-Enciso et al. investigated ambulatory COVID-19 patients and therapy efficacy with nebulized and/or intravenous neutral electrolyzed saline (containing hypochlorous acid) associated with usual medical care versus routine medical care only. They found no adverse severe symptoms and showed an increased effect on SARS-CoV-2 clearance (95). The authors hypothesized that increased osmolarity influences oxidative processes related to the death and health deterioration of COVID-19 patients. However, the precise MPO mechanism still requires specific in-depth research to establish the effect of saline regarding the regulation of SARS-CoV-2 infectivity by MPO. This knowledge could assist the planning of novel salt approaches in intensive care unit-related inflammatory illnesses, such as COVID-19, in different stages of the disease.
Final Considerations
Although the relationship between the electrolyte disturbances and COVID-19 infection is not fully clear yet, the electrolytic imbalance is often reported among COVID-19 patients, and hyponatremia is associated with a bad prognosis. HS therapies are simple, popular, economic, and feasible therapy to regulate leukocyte function with a robust anti-inflammatory effect in many inflammatory diseases, such as sepsis and cystic fibrosis. However, the potential anti-inflammatory action of HS in vivo requires more studies, the mechanisms by which HS stimulates mucociliary clearance are not fully clear and the cause-and-effect relationship of these events in patients with COVID-19 can only be confirmed by RCT. Therefore, there is a compelling need to investigate the effects of HS on neutrophil function and NETs formation in COVID-19 as promising targets for pharmacological treatment of the disease in the current pandemic scenario.
Author Contributions
MG-F and LB developed the idea and wrote the manuscript. AD, EW, RC, and TP-C collected and prepared the study data. EH reviewed and edited the manuscript. All authors have read and agreed to the published version of the manuscript.
Funding
This study was supported by the Brazilian research funding agencies CAPES (Coordenação de Aperfeiçoamento de Pessoal de Nível Superior – 88882.314890/2013-01, Finance Code 001), CNPq (Conselho Nacional de Desenvolvimento Científico e Tecnológico – 308700/2017-1), and FAPESP (Fundação de Amparo à Pesquisa do Estado de São Paulo).
Conflict of Interest
The authors declare that the research was conducted in the absence of any commercial or financial relationships that could be construed as a potential conflict of interest.
Publisher’s Note
All claims expressed in this article are solely those of the authors and do not necessarily represent those of their affiliated organizations, or those of the publisher, the editors and the reviewers. Any product that may be evaluated in this article, or claim that may be made by its manufacturer, is not guaranteed or endorsed by the publisher.
Supplementary Material
The Supplementary Material for this article can be found online at: https://www.frontiersin.org/articles/10.3389/fmed.2022.917008/full#supplementary-material
References
1. Karim SSA. Vaccines and SARS-CoV-2 variants: the urgent need for a correlate of protection. Lancet. (2021) 397:1263–4. doi: 10.1016/S0140-6736(21)00468-2
2. Lin D, Liu L, Zhang M, Hu Y, Yang Q, Guo J, et al. Co-infections of SARS-CoV-2 with multiple common respiratory pathogens in infected patients. Sci China Life Sci. (2020) 63:606–9. doi: 10.1007/s11427-020-1668-5
3. Musuuza JS, Watson L, Parmasad V, Putman-Buehler N, Christensen L, Safdar N. Prevalence and outcomes of co-infection and superinfection with SARS-CoV-2 and other pathogens: a systematic review and meta-analysis. PLoS One. (2021) 16:e0251170. doi: 10.1371/journal.pone.0251170
4. Kurra N, Woodard PI, Gandrakota N, Gandhi H, Polisetty SR, Ang SP, et al. Opportunistic infections in COVID-19: a systematic review and meta-analysis. Cureus. (2022) 14:e23687. doi: 10.7759/cureus.23687
5. Paolucci S, Cassaniti I, Novazzi F, Fiorina L, Piralla A, Comolli G, et al. EBV DNA increase in COVID-19 patients with impaired lymphocyte subpopulation count. Int J Infect Dis. (2021) 104:315–9. doi: 10.1016/j.ijid.2020.12.051
6. Goralski JL, Wu D, Thelin WR, Boucher RC, Button B. The in vitro effect of nebulised hypertonic saline on human bronchial epithelium. Eur Respir J. (2018) 51:1702652. doi: 10.1183/13993003.02652-2017
7. Hampton MB, Chambers ST, Vissers MC, Winterbourn CC. Bacterial killing by neutrophils in hypertonic environments. J Infect Dis. (1994) 169:839–46. doi: 10.1093/infdis/169.4.839
8. Corcoran TE, Godovchik JE, Donn KH, Busick DR, Goralski J, Locke LW, et al. Overnight delivery of hypertonic saline by nasal cannula aerosol for cystic fibrosis. Pediatr Pulmonol. (2017) 52:1142–9. doi: 10.1002/ppul.23749
9. Poli-de-Figueiredo LF, Cruz RJJ, Sannomiya P, Rocha-E-Silva M. Mechanisms of action of hypertonic saline resuscitation in severe sepsis and septic shock. Endocr Metab Immune Disord Drug Targets. (2006) 6:201–6. doi: 10.2174/187153006777442305
10. Toungoussova O, Migliori GB, Foschino Barbaro MP, Esposito LM, Dragonieri S, Carpagnano GE, et al. Changes in sputum composition during 15 min of sputum induction in healthy subjects and patients with asthma and chronic obstructive pulmonary disease. Respir Med. (2007) 101:1543–8. doi: 10.1016/j.rmed.2006.12.009
11. Kellett F, Redfern J, Niven RM. Evaluation of nebulised hypertonic saline (7%) as an adjunct to physiotherapy in patients with stable bronchiectasis. Respir Med. (2005) 99:27–31. doi: 10.1016/j.rmed.2004.05.006
12. Daviskas E, Anderson SD. Hyperosmolar agents and clearance of mucus in the diseased airway. J Aerosol Med. (2006) 19:100–9. doi: 10.1089/jam.2006.19.100
13. Sood N, Bennett WD, Zeman K, Brown J, Foy C, Boucher RC, et al. Increasing concentration of inhaled saline with or without amiloride: effect on mucociliary clearance in normal subjects. Am J Respir Crit Care Med. (2003) 167:158–63. doi: 10.1164/rccm.200204-293OC
14. Frontera JA, Valdes E, Huang J, Lewis A, Lord AS, Zhou T, et al. Prevalence and impact of hyponatremia in patients with coronavirus disease 2019 in New York City. Crit Care Med. (2020) 48:e1211–7. doi: 10.1097/CCM.0000000000004605
15. Hu W, Lv X, Li C, Xu Y, Qi Y, Zhang Z, et al. Disorders of sodium balance and its clinical implications in COVID-19 patients: a multicenter retrospective study. Intern Emerg Med. (2021) 16:853–62. doi: 10.1007/s11739-020-02515-9
16. Kimura KS, Freeman MH, Wessinger BC, Gupta V, Sheng Q, Huang LC, et al. Interim analysis of an open-label randomized controlled trial evaluating nasal irrigations in non-hospitalized patients with coronavirus disease 2019. Int Forum Allergy Rhinol. (2020) 10:1325–8. doi: 10.1002/alr.22703
17. Pascual JL, Khwaja KA, Ferri LE, Giannias B, Evans DC, Razek T, et al. Hypertonic saline resuscitation attenuates neutrophil lung sequestration and transmigration by diminishing leukocyte-endothelial interactions in a two-hit model of hemorrhagic shock and infection. J Trauma. (2003) 54:121–2. doi: 10.1097/00005373-200301000-00015
18. Reeves EP, Williamson M, O’Neill SJ, Greally P, McElvaney NG. Nebulized hypertonic saline decreases IL-8 in sputum of patients with cystic fibrosis. Am J Respir Crit Care Med. (2011) 183:1517–23. doi: 10.1164/rccm.201101-0072OC
19. Chen L, Li X, Chen M, Feng Y, Xiong C. The ACE2 expression in human heart indicates new potential mechanism of heart injury among patients infected with SARS-CoV-2. Cardiovasc Res. (2020) 116:1097–100. doi: 10.1093/cvr/cvaa078
20. Scialo F, Daniele A, Amato F, Pastore L, Matera MG, Cazzola M, et al. ACE2: the major cell entry receptor for SARS-CoV-2. Lung. (2020) 198:867–77. doi: 10.1007/s00408-020-00408-4
21. Gheorghe G, Ilie M, Bungau S, Stoian AMP, Bacalbasa N, Diaconu CC. Is There a Relationship between COVID-19 and Hyponatremia? Medicina. (2021) 57:55. doi: 10.3390/medicina57010055
22. Waikar SS, Mount DB, Curhan GC. Mortality after hospitalization with mild, moderate, and severe hyponatremia. Am J Med. (2009) 122:857–65. doi: 10.1016/j.amjmed.2009.01.027
23. Upadhyay A, Jaber BL, Madias NE. Incidence and prevalence of hyponatremia. Am J Med. (2006) 119:S30–5. doi: 10.1016/j.amjmed.2006.05.005
24. Verbalis JG, Goldsmith SR, Greenberg A, Korzelius C, Schrier RW, Sterns RH, et al. Diagnosis, evaluation, and treatment of hyponatremia: expert panel recommendations. Am J Med. (2013) 126:S1–42. doi: 10.1016/j.amjmed.2013.07.006
25. Funk G-C, Lindner G, Druml W, Metnitz B, Schwarz C, Bauer P, et al. Incidence and prognosis of dysnatremias present on ICU admission. Intensive Care Med. (2010) 36:304–11. doi: 10.1007/s00134-009-1692-0
26. Leong H-N, Earnest A, Lim H-H, Chin C-F, Tan CSH, Puhaindran ME, et al. SARS in Singapore–predictors of disease severity. Ann Acad Med Singap. (2006) 35:326–31.
27. Jin Y, Ji W, Yang H, Chen S, Zhang W, Duan G. Endothelial activation and dysfunction in COVID-19: from basic mechanisms to potential therapeutic approaches. Signal Transduct Target Ther. (2020) 5:293. doi: 10.1038/s41392-020-00454-7
28. Teuwen L-A, Geldhof V, Pasut A, Carmeliet P. COVID-19: the vasculature unleashed. Nat Rev Immunol. (2020) 20:389–91. doi: 10.1038/s41577-020-0343-0
29. Levi M, van der Poll T, Büller HR. Bidirectional relation between inflammation and coagulation. Circulation. (2004) 109:2698–704. doi: 10.1161/01.CIR.0000131660.51520.9A
30. Malas MB, Naazie IN, Elsayed N, Mathlouthi A, Marmor R, Clary B. Thromboembolism risk of COVID-19 is high and associated with a higher risk of mortality: a systematic review and meta-analysis. EClinicalMedicine. (2020) 29:100639. doi: 10.1016/j.eclinm.2020.100639
31. Kempuraj D, Selvakumar GP, Ahmed ME, Raikwar SP, Thangavel R, Khan A, et al. COVID-19, mast cells, cytokine storm, psychological stress, and neuroinflammation. Neuroscientist. (2020) 26:402–14. doi: 10.1177/1073858420941476
32. Alexopoulos H, Magira E, Bitzogli K, Kafasi N, Vlachoyiannopoulos P, Tzioufas A, et al. Anti-SARS-CoV-2 antibodies in the CSF, blood-brain barrier dysfunction, and neurological outcome: studies in 8 stuporous and comatose patients. Neurol Neuroimmunol Neuroinflamm. (2020) 7:e893. doi: 10.1212/NXI.0000000000000893
33. Du M, Cai G, Chen F, Christiani DC, Zhang Z, Wang M. Multiomics evaluation of gastrointestinal and other clinical characteristics of COVID-19. Gastroenterology. (2020) 158:2298–301.e7. doi: 10.1053/j.gastro.2020.03.045
34. Grasselli G, Zangrillo A, Zanella A, Antonelli M, Cabrini L, Castelli A, et al. Baseline characteristics and outcomes of 1591 patients infected with SARS-CoV-2 admitted to ICUs of the Lombardy Region, Italy. JAMA. (2020) 323:1574–81. doi: 10.1001/jama.2020.5394
35. Giacomelli A, Pezzati L, Conti F, Bernacchia D, Siano M, Oreni L, et al. Self-reported olfactory and taste disorders in patients with severe acute respiratory Coronavirus 2 infection: a cross-sectional study. Clin Infect Dis. (2020) 71:889–90. doi: 10.1093/cid/ciaa330
36. Zhang Y, Zheng L, Liu L, Zhao M, Xiao J, Zhao Q. Liver impairment in COVID-19 patients: a retrospective analysis of 115 cases from a single centre in Wuhan city, China. Liver Int. (2020) 40:2095–103. doi: 10.1111/liv.14455
37. D’Ardes D, Boccatonda A, Cocco G, Fabiani S, Rossi I, Bucci M, et al. Impaired coagulation, liver dysfunction and COVID-19: discovering an intriguing relationship. World J Gastroenterol. (2022) 28:1102–12. doi: 10.3748/wjg.v28.i11.1102
38. Kaklamanos A, Belogiannis K, Skendros P, Gorgoulis VG, Vlachoyiannopoulos PG, Tzioufas AG. COVID-19 immunobiology: lessons learned, new questions arise. Front Immunol. (2021) 12:719023. doi: 10.3389/fimmu.2021.719023
39. Jothimani D, Venugopal R, Abedin MF, Kaliamoorthy I, Rela M. COVID-19 and the liver. J Hepatol. (2020) 73:1231–40. doi: 10.1016/j.jhep.2020.06.006
40. Flor M, Valga F, Alexander M, Miguel Rodeles del P. Hyponatremia in COVID-19 infection - possible causal factors and management. J Clin Nephrol Ren Care. (2020) 6:53–6. doi: 10.23937/2572-3286.1510057
41. Aggarwal S, Garcia-Telles N, Aggarwal G, Lavie C, Lippi G, Henry BM. Clinical features, laboratory characteristics, and outcomes of patients hospitalized with coronavirus disease 2019 (COVID-19): early report from the United States. Diagnosis. (2020) 7:91–6. doi: 10.1515/dx-2020-0046
42. Lippi G, South AM, Henry BM. Electrolyte imbalances in patients with severe coronavirus disease 2019 (COVID-19). Ann Clin Biochem. (2020) 57:262–5. doi: 10.1177/0004563220922255
43. Berni A, Malandrino D, Parenti G, Maggi M, Poggesi L, Peri A. Hyponatremia, IL-6, and SARS-CoV-2 (COVID-19) infection: may all fit together? J Endocrinol Invest. (2020) 43:1137–9. doi: 10.1007/s40618-020-01301-w
44. Swart RM, Hoorn EJ, Betjes MG, Zietse R. Hyponatremia and inflammation: the emerging role of interleukin-6 in osmoregulation. Nephron Physiol. (2011) 118:45–51. doi: 10.1159/000322238
45. Khalangot M. COVID-19 and SIADH relations: impact of the positive pressure ventilation. Am J Physiol Endocrinol Metab. (2020) 319:E196. doi: 10.1152/ajpendo.00288.2020
46. Machado RRG, Glaser T, Araujo DB, Petiz LL, Oliveira DBL, Durigon GS, et al. Inhibition of severe acute respiratory syndrome coronavirus 2 replication by hypertonic saline solution in lung and kidney epithelial cells. ACS Pharmacol Transl Sci. (2021) 4:1514–27. doi: 10.1021/acsptsci.1c00080
47. Nicolson CHH, Stirling RG, Borg BM, Button BM, Wilson JW, Holland AE. The long term effect of inhaled hypertonic saline 6% in non-cystic fibrosis bronchiectasis. Respir Med. (2012) 106:661–7. doi: 10.1016/j.rmed.2011.12.021
48. Kellett F, Robert NM. Nebulised 7% hypertonic saline improves lung function and quality of life in bronchiectasis. Respir Med. (2011) 105:1831–5. doi: 10.1016/j.rmed.2011.07.019
49. Dellon EP, Donaldson SH, Johnson R, Davis SD. Safety and tolerability of inhaled hypertonic saline in young children with cystic fibrosis. Pediatr Pulmonol. (2008) 43:1100–6. doi: 10.1002/ppul.20909
50. Ho KS, Narasimhan B, Kumar A, Flynn E, Salonia J, El-Hachem K, et al. Syndrome of inappropriate antidiuretic hormone as the initial presentation of COVID-19: a novel case report. Nefrologia. (2021) 41:219–20. doi: 10.1016/j.nefro.2020.05.004
51. Ramalingam S, Graham C, Dove J, Morrice L, Sheikh A. A pilot, open labelled, randomised controlled trial of hypertonic saline nasal irrigation and gargling for the common cold. Sci Rep. (2019) 9:1015. doi: 10.1038/s41598-018-37703-3
52. Ramalingam S, Graham C, Dove J, Morrice L, Sheikh A. Hypertonic saline nasal irrigation and gargling should be considered as a treatment option for COVID-19. J Glob Health. (2020) 10:10332. doi: 10.7189/jogh.10.010332
53. Singh S, Sharma N, Singh U, Singh T, Mangal DK, Singh V. Nasopharyngeal wash in preventing and treating upper respiratory tract infections: Could it prevent COVID-19?. Lung India. (2020) 37:246–51. doi: 10.4103/lungindia.lungindia_241_20
54. Ye Q, Wang B, Mao J. The pathogenesis and treatment of the ‘Cytokine Storm’ in COVID-19. J Infect. (2020) 80:607–13. doi: 10.1016/j.jinf.2020.03.037
55. Mehta P, McAuley DF, Brown M, Sanchez E, Tattersall RS, Manson JJ. COVID-19: consider cytokine storm syndromes and immunosuppression. Lancet. (2020) 395:1033–4. doi: 10.1016/S0140-6736(20)30628-0
56. Zhang H, Du F, Cao X-J, Feng X-L, Zhang H-P, Wu Z-X, et al. Clinical characteristics of coronavirus disease 2019 (COVID-19) in patients out of Wuhan from China: a case control study. BMC Infect Dis. (2021) 21:207. doi: 10.1186/s12879-021-05897-z
57. Qin C, Zhou L, Hu Z, Zhang S, Yang S, Tao Y, et al. Dysregulation of immune response in patients with COVID-19 in Wuhan, China. Clin Infect Dis. (2020) 71:762–8. doi: 10.1093/cid/ciaa248
58. Huang C, Wang Y, Li X, Ren L, Zhao J, Hu Y, et al. Clinical features of patients infected with 2019 novel coronavirus in Wuhan, China. Lancet. (2020) 395:497–506. doi: 10.1016/S0140-6736(20)30183-5
59. Tzoulis P, Grossman AB, Baldeweg SE, Bouloux P, Kaltsas G. MANAGEMENT OF ENDOCRINE DISEASE. Dysnatraemia in COVID-19: prevalence, prognostic impact, pathophysiology, and management. Eur J Endocrinol. (2021) 185:R103–11. doi: 10.1530/EJE-21-0281
60. Thiel M, Buessecker F, Eberhardt K, Chouker A, Setzer F, Kreimeier U, et al. Effects of hypertonic saline on expression of human polymorphonuclear leukocyte adhesion molecules. J Leukoc Biol. (2001) 70:261–73.
61. Oreopoulos GD, Hamilton J, Rizoli SB, Fan J, Lu Z, Li YH, et al. In vivo and in vitro modulation of intercellular adhesion molecule (ICAM)-1 expression by hypertonicity. Shock. (2000) 14:405–9. doi: 10.1097/00024382-200014030-00029
62. Oreopoulos GD, Bradwell S, Lu Z, Fan J, Khadaroo R, Marshall JC, et al. Synergistic induction of IL-10 by hypertonic saline solution and lipopolysaccharides in murine peritoneal macrophages. Surgery. (2001) 130:157–65. doi: 10.1067/msy.2001.115829
63. Tabary O, Escotte S, Couetil JP, Hubert D, Dusser D, Puchelle E, et al. High susceptibility for cystic fibrosis human airway gland cells to produce IL-8 through the I kappa B kinase alpha pathway in response to extracellular NaCl content. J Immunol. (2000) 164:3377–84. doi: 10.4049/jimmunol.164.6.3377
64. Hashimoto S, Matsumoto K, Gon Y, Nakayama T, Takeshita I, Horie T. Hyperosmolarity-induced interleukin-8 expression in human bronchial epithelial cells through p38 mitogen-activated protein kinase. Am J Respir Crit Care Med. (1999) 159:634–40. doi: 10.1164/ajrccm.159.2.9712090
65. Schaeffer V, Arbabi S, Garcia IA, Knoll ML, Cuschieri J, Bulger EM, et al. Role of the mTOR pathway in LPS-activated monocytes: influence of hypertonic saline. J Surg Res. (2011) 171:769–76. doi: 10.1016/j.jss.2010.05.035
66. Lee L, Kelher MR, Moore EE, Banerjee A, Silliman CC. Hypertonic saline inhibits arachidonic acid priming of the human neutrophil oxidase. J Surg Res. (2012) 174:24–8. doi: 10.1016/j.jss.2011.06.022
67. Paff T, Daniels JMA, Weersink EJ, Lutter R, Vonk Noordegraaf A, Haarman EG. A randomised controlled trial on the effect of inhaled hypertonic saline on quality of life in primary ciliary dyskinesia. Eur Respir J. (2017) 49:1601770. doi: 10.1183/13993003.01770-2016
68. Aitken ML, Greene KE, Tonelli MR, Burns JL, Emerson JC, Goss CH, et al. Analysis of sequential aliquots of hypertonic saline solution-induced sputum from clinically stable patients with cystic fibrosis. Chest. (2003) 123:792–9. doi: 10.1378/chest.123.3.792
69. Elkins MR, Robinson M, Rose BR, Harbour C, Moriarty CP, Marks GB, et al. A controlled trial of long-term inhaled hypertonic saline in patients with cystic fibrosis. N Engl J Med. (2006) 354:229–40. doi: 10.1056/NEJMoa043900
70. Borges L, Passos MEP, Silva MBB, Santos VC, Momesso CM, Pithon-Curi TC, et al. Dance training improves cytokine secretion and viability of neutrophils in diabetic patients. Mediators Inflamm. (2019) 2019:2924818. doi: 10.1155/2019/2924818
71. Passos MEP, Borges L, Dos Santos-Oliveira LC, Alecrim-Zeza AL, Lobato TB, de Oliveira HH, et al. Recreational dance practice modulates lymphocyte profile and function in diabetic women. Int J Sports Med. (2020) 42:749–59. doi: 10.1055/a-1309-2037
72. Lamichhane PP, Samarasinghe AE. The role of innate leukocytes during influenza virus infection. J Immunol Res. (2019) 2019:8028725. doi: 10.1155/2019/8028725
73. Rosales C. Neutrophils at the crossroads of innate and adaptive immunity. J Leukoc Biol. (2020) 108:377–96. doi: 10.1002/JLB.4MIR0220-574RR
74. Bordon J, Aliberti S, Fernandez-Botran R, Uriarte SM, Rane MJ, Duvvuri P, et al. Understanding the roles of cytokines and neutrophil activity and neutrophil apoptosis in the protective versus deleterious inflammatory response in pneumonia. Int J Infect Dis. (2013) 17:e76–83. doi: 10.1016/j.ijid.2012.06.006
75. Barnes BJ, Adrover JM, Baxter-Stoltzfus A, Borczuk A, Cools-Lartigue J, Crawford JM, et al. Targeting potential drivers of COVID-19: neutrophil extracellular traps. J Exp Med. (2020) 217:e20200652. doi: 10.1084/jem.20200652
76. Lagunas-Rangel FA. Neutrophil-to-lymphocyte ratio and lymphocyte-to-C-reactive protein ratio in patients with severe coronavirus disease 2019 (COVID-19): a meta-analysis. J Med Virol. (2020) 92:1733–4. doi: 10.1002/jmv.25819
77. Deitch EA, Shi HP, Feketeova E, Hauser CJ, Xu D-Z. Hypertonic saline resuscitation limits neutrophil activation after trauma-hemorrhagic shock. Shock. (2003) 19:328–33. doi: 10.1097/00024382-200304000-00006
78. Chen Y, Bao Y, Zhang J, Woehrle T, Sumi Y, Ledderose S, et al. Inhibition of neutrophils by hypertonic saline involves pannexin-1, CD39, CD73, and other ectonucleotidases. Shock. (2015) 44:221–7. doi: 10.1097/SHK.0000000000000402
79. Rizoli SB, Kapus A, Fan J, Li YH, Marshall JC, Rotstein OD. Immunomodulatory effects of hypertonic resuscitation on the development of lung inflammation following hemorrhagic shock. J Immunol. (1998) 161:6288–96.
80. Angle N, Hoyt DB, Cabello-Passini R, Herdon-Remelius C, Loomis W, Junger WG. Hypertonic saline resuscitation reduces neutrophil margination by suppressing neutrophil L selectin expression. J Trauma. (1998) 45:3–7. doi: 10.1097/00005373-199807000-00002
81. Zallen G, Moore EE, Tamura DY, Johnson JL, Biffl WL, Silliman CC. Hypertonic saline resuscitation abrogates neutrophil priming by mesenteric lymph. J Trauma. (2000) 48:45–8. doi: 10.1097/00005373-200001000-00008
82. Tillinger W, McCole DF, Keely SJ, Bertelsen LS, Wolf PL, Junger WG, et al. Hypertonic saline reduces neutrophil-epithelial interactions in vitro and gut tissue damage in a mouse model of colitis. Am J Physiol Regul Integr Comp Physiol. (2008) 295:R1839–45. doi: 10.1152/ajpregu.00412.2007
83. Hatanaka E, Shimomi FM, Curi R, Campa A. Sodium chloride inhibits cytokine production by lipopolysaccharide-stimulated human neutrophils and mononuclear cells. Shock. (2007) 27:32–5. doi: 10.1097/01.shk.0000238061.69579.a5
84. Rizoli SB, Kapus A, Parodo J, Rotstein OD. Hypertonicity prevents lipopolysaccharide-stimulated CD11b/CD18 expression in human neutrophils in vitro: role for p38 inhibition. J Trauma. (1999) 46:794–9. doi: 10.1097/00005373-199905000-00006
85. Matsumoto T, Takahashi K, Kubo S, Haraoka M, Mizunoe Y, Tanaka M, et al. Suppression of chemotactic activity of neutrophils in hyperosmotic conditions comparable to the renal medulla: partial preservation by phosphoenolpyruvate. Urol Int. (1993) 50:1–5. doi: 10.1159/000282438
86. Rizoli SB, Rotstein OD, Parodo J, Phillips MJ, Kapus A. Hypertonic inhibition of exocytosis in neutrophils: central role for osmotic actin skeleton remodeling. Am J Physiol Cell Physiol. (2000) 279:C619–33. doi: 10.1152/ajpcell.2000.279.3.C619
87. Papayannopoulos V, Zychlinsky A. NETs: a new strategy for using old weapons. Trends Immunol. (2009) 30:513–21. doi: 10.1016/j.it.2009.07.011
88. Brinkmann V, Reichard U, Goosmann C, Fauler B, Uhlemann Y, Weiss DS, et al. Neutrophil extracellular traps kill bacteria. Science. (2004) 303:1532–5. doi: 10.1126/science.1092385
89. Soy M, Keser G, Atagündüz P, Tabak F, Atagündüz I, Kayhan S. Cytokine storm in COVID-19: pathogenesis and overview of anti-inflammatory agents used in treatment. Clin Rheumatol. (2020) 39:2085–94. doi: 10.1007/s10067-020-05190-5
90. Sørensen OE, Borregaard N. Neutrophil extracellular traps - the dark side of neutrophils. J Clin Invest. (2016) 126:1612–20. doi: 10.1172/JCI84538
91. Borges L, Pithon-Curi TC, Curi R, Hatanaka E. COVID-19 and neutrophils: the relationship between hyperinflammation and neutrophil extracellular traps. Mediators Inflamm. (2020) 2020:8829674. doi: 10.1155/2020/8829674
92. Bartoszewski R, Matalon S, Collawn JF. Ion channels of the lung and their role in disease pathogenesis. Am J Physiol Lung Cell Mol Physiol. (2017) 313:L859–72. doi: 10.1152/ajplung.00285.2017
93. Nadesalingam A, Chen JHK, Farahvash A, Khan MA. Hypertonic saline suppresses NADPH oxidase-dependent neutrophil extracellular trap formation and promotes apoptosis. Front Immunol. (2018) 9:359. doi: 10.3389/fimmu.2018.00359
94. Huijghebaert S, Hoste L, Vanham G. Essentials in saline pharmacology for nasal or respiratory hygiene in times of COVID-19. Eur J Clin Pharmacol. (2021) 77:1275–93. doi: 10.1007/s00228-021-03102-3
95. Delgado-Enciso I, Paz-Garcia J, Barajas-Saucedo CE, Mokay-Ramírez KA, Meza-Robles C, Lopez-Flores R, et al. Patient-reported health outcomes after treatment of COVID-19 with nebulized and/or intravenous neutral electrolyzed saline combined with usual medical care versus usual medical care alone: a randomized, open-label, controlled trial. Res Sq. [Preprint]. (2020). doi: 10.21203/rs.3.rs-68403/v1
Keywords: coronavirus, leukocytes, neutrophils, immune system, NETs, NETosis, NaCl
Citation: Gennari-Felipe M, Borges L, Dermargos A, Weimann E, Curi R, Pithon-Curi TC and Hatanaka E (2022) Hypertonic Solution in Severe COVID-19 Patient: A Potential Adjuvant Therapy. Front. Med. 9:917008. doi: 10.3389/fmed.2022.917008
Received: 10 April 2022; Accepted: 06 June 2022;
Published: 21 June 2022.
Edited by:
Ahmed Yaqinuddin, Alfaisal University, Saudi ArabiaReviewed by:
Philipp Buehler, University Hospital Zürich, SwitzerlandCopyright © 2022 Gennari-Felipe, Borges, Dermargos, Weimann, Curi, Pithon-Curi and Hatanaka. This is an open-access article distributed under the terms of the Creative Commons Attribution License (CC BY). The use, distribution or reproduction in other forums is permitted, provided the original author(s) and the copyright owner(s) are credited and that the original publication in this journal is cited, in accordance with accepted academic practice. No use, distribution or reproduction is permitted which does not comply with these terms.
*Correspondence: Elaine Hatanaka, ZWxhaW5laGF0YW5ha2FAZ21haWwuY29t
†These authors have contributed equally to this work