Biomarkers for differentiation of coronavirus disease 2019 or extracorporeal membrane oxygenation related inflammation and bacterial/fungal infections in critically ill patients: A prospective observational study
- 1Division of Infectious Diseases and Tropical Medicine, Department of Medicine I, Medical University of Vienna, Vienna, Austria
- 2Department of Clinical Pharmacology, Medical University of Vienna, Vienna, Austria
- 3Ihr Labor, Medical Diagnostics Laboratories, Vienna, Austria
- 4Department of Medicine I, Intensive Care Unit 13i2, Medical University of Vienna, Vienna, Austria
- 5Division of Gastroenterology and Hepatology, Department of Medicine III, Medical University of Vienna, Vienna, Austria
- 6Department of Anaesthesia, Intensive Care Medicine and Pain Medicine, Medical Simulation and Emergency Management Research Group, Medical University of Vienna, Vienna, Austria
Secondary infections in coronavirus disease 2019 (COVID-19) patients are difficult to distinguish from inflammation associated with COVID-19 and/or extracorporeal membrane oxygenation (ECMO). Therefore, highly specific and sensitive biomarkers are needed to identify patients in whom antimicrobial therapy can be safely withheld. In this prospective monocentric study, 66 COVID-19 patients admitted to the intensive care unit (ICU) for ECMO evaluation were included. A total of 46 (70%) patients with secondary infections were identified by using broad microbiological and virological panels and standardized diagnostic criteria. Various laboratory parameters including C-reactive protein (CRP), interleukin (IL)-6, procalcitonin (PCT), and IL-10 were determined at time of study inclusion. The best test performance for differentiating bacterial/fungal secondary infections and COVID-19 and/or ECMO associated inflammation was achieved by IL-10 (ROC-AUC 0.84) and a multivariant step-wise regression model including CRP, IL-6, PCT, and IL-10 (ROC-AUC 0.93). Data obtained in the present study highlights the use of IL-10 to differentiate secondary bacterial/fungal infections from COVID-19 and/or ECMO associated inflammation in severely ill COVID-19 patients.
Introduction
During the first wave in the United States, coronavirus disease 2019 (COVID-19) resulted in high rates of hospitalization (14%), intensive care unit (ICU) admissions (2%), and mortality (5%), leading to an extreme burden on the health care system (1). The very high mortality in European ICUs of about 30% did not change during the first three waves and therefore underscores the extreme burden of the COVID-19 pandemic on global health systems (2). In critically ill patients COVID-19 related pulmonary dysbiosis is a predisposing factor for development of secondary bacterial/fungal infections, occurring in 14–41% of ICU patients (3–5).
Influenced by recent guidelines of the Infectious Disease Society of America on the management of critically ill influenza patients, frequent antimicrobial usage of up to 86.4% has been observed at the beginning of the COVID-19 pandemic (6). This high use of antimicrobials was questioned, based on low rates of secondary infections observed as the pandemic progressed. Nevertheless, secondary bacterial/fungal infections led to increased mortality rates, highlighting the need for rapid and adequate identification of these patients (3).
To date, biomarkers such as white blood cell count (WBC), C-reactive protein (CRP), and procalcitonin (PCT) have been studied to distinguish secondary infections from COVID-19 related inflammation. However, one study could only exclude bacterial co-infections in 46%, while another study lacked sufficient microbiological diagnostics and/or clinical characterization of secondary bacterial/fungal infections (7, 8).
This limited potential of differentiation is further complicated in COVID-19 acute respiratory distress syndrome (ARDS) patients requiring extracorporeal membrane oxygenation (ECMO) support, by non-infectious activation of inflammatory pathways (9). A less studied parameter in this context is interleukin (IL)-10, whose production is stimulated by peptidoglycans of the bacterial cell wall and has been shown in previous studies to be a strong prognostic factor for mortality as well as the bacterial loads in blood of patients with Staphylococcus aureus (S. aureus) bacteremia (10–13).
The aim of the present study was to determine biomarkers that allow safe discontinuation of antimicrobial therapy by correctly identifying secondary bacterial/fungal infections in critically ill COVID-19 patients admitted to a specialized ECMO center for treatment of ARDS.
Methods
Study design
This prospective observational study included patients with a positive real-time polymerase chain reaction for Severe Acute Respiratory Syndrome Coronavirus type 2 (SARS-CoV-2) from nasopharyngeal swabs and/or bronchial lavage, who had ongoing antimicrobial therapy for at least 48 h and were admitted to an ICU at the University Hospital Vienna. Patients were included in the present study at the time of admission to the participating ICUs, which in most cases was to assess the need for ECMO support for underlying ARDS. Patients below the age of 18 years were excluded from study participation. At study inclusion, a diagnostic panel consisting of inflammatory parameters such as CRP, IL-6, PCT, WBC, microbiological, and radiological diagnostics was performed, and additional blood samples were directly frozen at −80°C until further processing (Supplementary Table 1).
Interleukin-10 assay
Serum IL-10 (R&D Systems, Minneapolis, USA) was determined by sandwich enzyme immunoassays as recommended by the manufacturer, whereas all additional parameters were analyzed as part of routine diagnostics.
Data collection
The Charlson comorbidity index, the Acute Physiology and Chronic Health Evaluation (APACHE) IV, Acute Physiology Score (APS), Sequential Organ Failure Assessment (SOFA), and Simplified Acute Physiology Score (SAPS-II) score were assessed at study inclusion. Disease severity was classified as mild, moderate, severe or critical according to the world health organization (WHO) severity classification.
The site of infection was classified according to the criteria of the European Centre for Disease Prevention and Control (ECDC) Point Prevalence Survey on Healthcare Associated Infections (version 5.3). The clinical, microbiological, and laboratory parameters necessary for this classification, collected within 48 h around admission, were obtained from the patient information system of the general hospital Vienna (14). Each patient was then discussed again in an expert panel consisting of three infectious disease specialists to interpret ambiguous microbiological results and thus select the most appropriate classification.
Statistical analysis
Statistical analysis was performed using R Version 4.0.3 (Vienna, Austria). Categorical data are summarized as count with their percentage. Numeric data are presented as median with 1st and 3rd quartiles. The discriminatory ability of individual parameters was assessed using Wilcoxon rank tests and the area under the Receiver Characteristic Operator (ROC-AUC) curve. Cut-off points were calculated using the Youden Index method (pROC, R Package). Forward stepwise logistic regression models were established minimizing the Akaike information criterion (AIC) using age, sex, WHO-severity score, co-infection, and the laboratory parameters displayed in Supplementary Table 1. Additionally, a model with the two most discriminatory parameters was created. Due to the low number of available observations, cross-validation schemes or other methods for estimating the robustness of the predictive ability were not performed. Where appropriate, an accumulation of an alpha error related to multiple testing was controlled by the Bonferroni-Holm method.
Statistical significance was defined as p-values less than 0.05.
Results
Patients’ demographics and disease related characteristics are shown in Table 1. A total of 66 patients with a median age of 54 years and ongoing antimicrobial therapy were included in this study. Overall 92% of patients were defined as critical according to WHO severity classification, with 55% receiving ECMO support, which was started on average 1 day (SD ± 1.2 days) before study inclusion.
Secondary infections were observed in 46 patients at time of study inclusion. Out of these, 26 patients had monomicrobial (21 bacterial, 5 fungal) and 11 patients polymicrobial infections. Nine patients were classified as systemic infections (SYS) according to the ECDC criteria as no causative pathogen could be detected, clinical sings of an acute infection were present and the physician instituted treatment for sepsis. The most common infection sources were pulmonary (n = 26, 57%), catheter related (n = 5, 11%) and urinary tract infections (n = 2, 4%). Additionally, blood stream infections of unknown origin and SYS without detected causative pathogens were found in 2 (4%) and 9 (20%) patients, respectively. The overall mortality was 38% (n = 25) with a 28-day mortality of 15% (n = 10) and a median hospital stay of 47 days (Supplementary Table 1).
When comparing patients with and without ECMO support, no apparent differences in levels of CRP, IL-6, IL-10, PCT, lactate dehydrogenase (LDH), D-dimer, leukocytes, fibrinogen, ferritin, the neutrophils/lymphocyte ratio, the IL-6/IL-10 ratio, and the SOFA score were observed (Supplementary Figure 1).
The most discriminatory parameters for secondary bacterial/fungal infections (p-value, ROC-AUC, 95% CI) were CRP (p = 0.0005, 0.77, 0.65–0.88), IL-10 (p < 0.0001, 0.88, 0.73–0.94), and PCT (p = 0.0008, 0.76, 0.65–0.88) (Supplementary Table 2). A forward step-wise logistic regression model was calculated resulting in a ROC-AUC of 0.93 (95% CI: 0.87–0.99). The final model included CRP, IL-6, IL-10, and PCT (model 1). A model solely based on CRP and IL-10 (model 2) resulted in a ROC-AUC of 0.91 (95% CI: 0.87–0.98) (Figure 1). However, both logistic regression models were not superior at predicting the presence of superinfections compared to IL-10 alone (p = 0.154, p = 0.263). IL-10 showed the best sensitivity (96%) and specificity (100%) at a cut-off value of 15.4 pg/ml compared to CRP, IL-6 and PCT (Supplementary Table 2). When a correlation matrix of major biomarkers and clinical scores was calculated, apart from the APS with the APACHE-IV score, there was no strong correlation, even between commonly used inflammation parameters (Supplementary Figure 2).
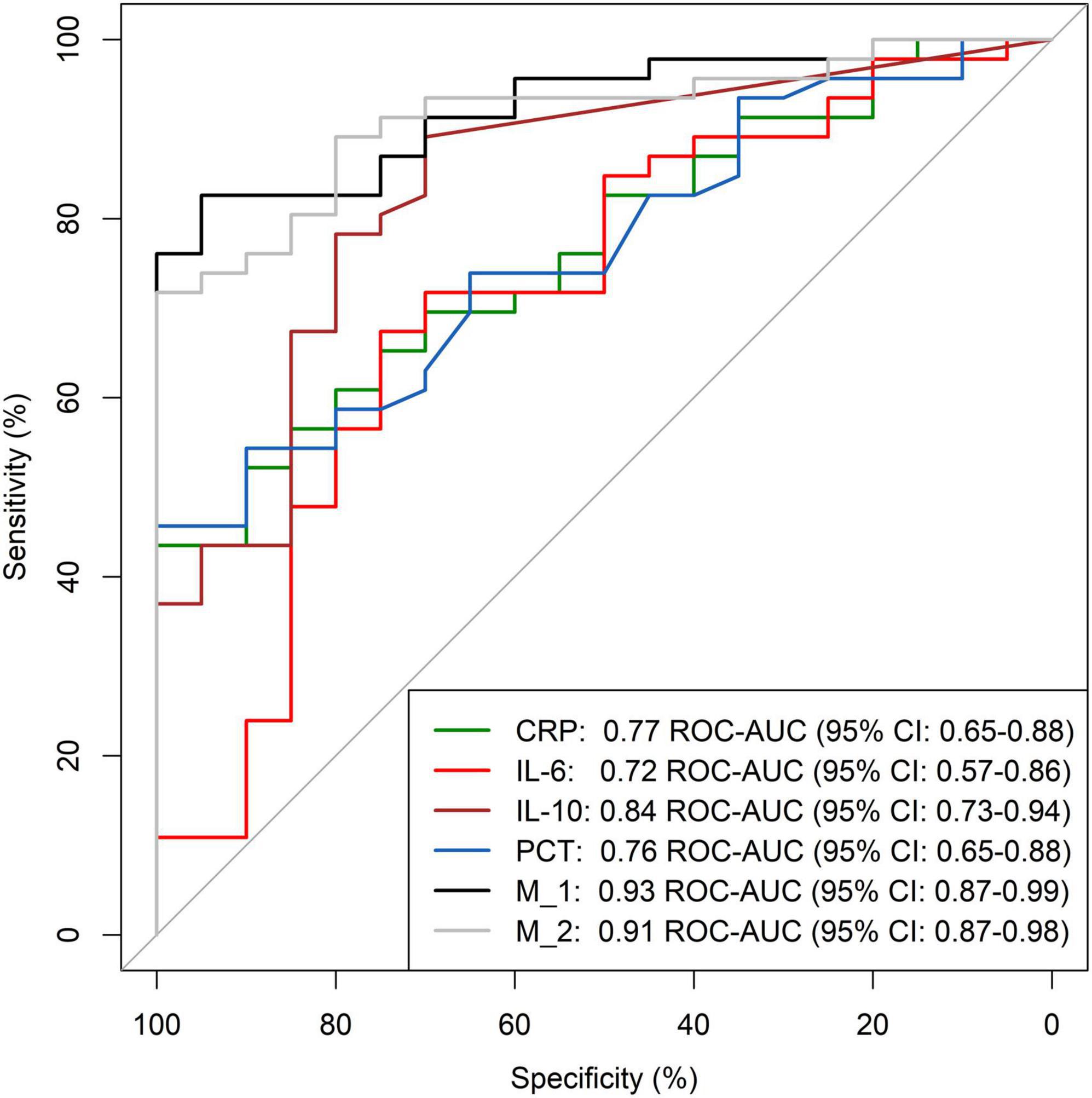
Figure 1. ROC analysis for detection of additional infections in COVID-19 ICU patients. ROC-AUC, area under the receiver operating characteristics curve; 95% CI, 95% Confidence interval; CRP, C-reactive Protein; IL-6, Interleukin-6; IL-10, Interleukin-10; PCT, Procalcitonin; M_1 (model 1) and M_2 (model 2), multivariate step-wise regression models. M_1 included CRP, IL-6, IL-10 and procalcitonin. M_2 included CRP and IL-10.
Discussion
In critically ill COVID-19 patients predominantly admitted for ECMO evaluation, IL-10 supported the discrimination of bacterial/fungal secondary infections from inflammation caused by COVID-19 and/or ECMO. IL-10 alone could rule out secondary bacterial/fungal infections with an negative predictive value (NPV) of 89% (cut-off: 15.4 ng/mL), providing intensivists with a much needed tool to avoid unnecessary antibiotic therapy and to treat actual secondary infections as soon as possible.
The present study investigated a well-defined patient population with high rates of standardized and tailored microbiological/virological diagnostics obtained upon study inclusion (Supplementary Table 3). Comparable studies either focused on specific infections like pneumonia, showed lower rates of microbiological diagnostics (17–64%) due to a retrospective study design or have not stated their microbiological workup (15–17). Based on frequent microbiological diagnostics and the specific study population, including high rates of patients on ECMO (55%), high rates of secondary infections (70%), characterized using well defined ECDC criteria, were observed in the present study.
Previous reports in different ICU cohorts demonstrating lower morbidity severity indices (e.g., APACHE IV and SOFA score) with no or low rates (9.5%) of patients on ECMO observed secondary infections in 21–69% (16, 18–20). Although the study by De Bruyn et al. included less severely ill COVID-19 patients and markedly reduced microbiological diagnostics, it also showed high rates of secondary infections (69%), with pneumonia (57%), bloodstream infections of unknown origin (30%), and catheter-related sepsis (15%) being the most common sites of infection.
Acute COVID-19 infection is associated with inflammatory states and may be followed by a prolonged immune system dysregulation including consistently elevated inflammatory markers like IL-6, ferritin or CRP, for several weeks (21). ECMO therapy, a corner stone in the management of severe COVID-19 ARDS, increases inflammatory markers like WBC, IL-6, IL-10, and tumor necrosis factor (TNF)-alpha due to activation of inflammatory and coagulation pathways caused by the constant contact of cellular and humoral blood components on the large extracorporeal surface (9).
COVID-19 and/or ECMO induced inflammation might therefore complicate diagnosis of secondary bacterial/fungal infections, frequently supported by well-established laboratory markers. Various biomarkers were investigated to distinguish COVID-19 from bacterial infections such as CRP and/or WBC. A combination of WBC at admission and trajectories of CRP over 72 h allowed to exclude 46% of bacterial co-infections in COVID-19 patients (8). Another study investigated the outcome of COVID-19 patients newly admitted to the hospital with a PCT < 0.25 ng/mL, resulting in lower rates of antimicrobial prescription, ICU admission and 28-day mortality (7).
Previous studies investigated the usage of CRP and PCT to guide antimicrobial therapy (15). In a collective of 66 ICU patients secondary infections occurred in 50%, PCT demonstrated a positive predictive value (PPV) of 93% and a NPV of 81% with a cutoff of > 1 and < 0.25 μg/L, respectively. The present study showed a NPV of 77% and a PPV of 100% using a PCT cut-off of 0.6 ng/mL (Supplementary Table 2). Although van Berkel et al. investigated a less severely ill patient population, indicated by the lack of ECMO support, CRP and PCT showed comparable ROC-AUC values of 0.76 and 0.8, respectively, when compared to the present study (15).
In addition, Pink et al. showed higher ROC-AUC values for CRP (0.86) and PCT (0.88) for discrimination of secondary infections in a mixed patients collective consisting of 52 ICU and 47 non-ICU COVID-19 patients (22). However, the inclusion of non-ICU patients, the lack of ECMO support and the low rates of vasopressor use limit comparability with the present study.
IL-10 is mainly known as an anti-inflammatory cytokine and was shown to be a prognostic marker for poor outcome in COVID-19 patients. While the exact mechanisms are still unclear, evidence points in either a pro-inflammatory effect of high IL-10 levels causing T-cell exhaustion and/or an IL-10 “resistance” which is entangled with hyperglycemia (23). IL-10 concentrations observed in the present study (median: 13 pg/mL) were comparable to published data in patients with S. aureus bacteremia (median: 10–20 pg/mL) (24).
This association of IL-10 with poor outcomes in COVID-19 patients might be at least partly explained by secondary infections. IL-10 was the single parameter with the highest discriminatory power compared with CRP, IL-6 and PCT, which was exceeded only by using combinations of four markers (Model 1: CRP, IL-6, IL10, PCT; ROC-AUC 0.93) or CRP and IL-10 (Model 2: ROC-AUC 0.91) in two logistic regression models. In previous studies, elevated IL-10 at time of hospital admission or up to 72 h after presentation was shown to be predictive for mortality in patients with S. aureus bacteremia (13, 25). Although the exact reason for the association between increased IL-10 and increased mortality is not known, it has been demonstrated that IL-10 production is stimulated by components of the bacterial cell wall, particularly peptidoglycans, and is dependent on antigen levels and thus bacterial load (11, 12). Therefore, the increased mortality rate could be due to delayed or inadequate treatment of bacterial infections, so that the bacterial load in the blood, and consequently IL-10, remained significantly elevated. In conjunction with the data obtained in this study, IL-10 may thus be a valuable marker for a variety of infections, not only S. aureus bacteremia, and may potentially aid in clinical diagnosis finding. In addition, previous data investigating IL-10 serum concentrations in patients with S. aureus bacteremia demonstrated no significant change within the first 72 h, even with targeted antimicrobial treatment (24).
Interestingly, in this study, no differences in inflammatory parameters were detected between patients with and without ECMO support, although previous literature has described activation of inflammatory pathways by the extracorporeal surface during ECMO therapy. This lack of differences could be explained by the inflammation already triggered by COVID-19. However, further studies examining patients on ECMO support with and without COVID-19 are needed for a definitive explanation.
One of the main limitations of this study is the variability regarding the time of symptom onset to ICU admission, study inclusion and ECMO therapy start. This may lead to variations in the initial inflammatory stimulus, which, in conjunction with different production times and half-lives of the biomarkers studied, may explain the lack of correlation of frequently used inflammatory parameters with each other and with IL-10 (11, 26–28). Nevertheless, this study represents a real life scenario of a specialized center experienced in ARDS treatment and ECMO support, providing care of patients directly admitted from the emergency department, but also from external ICUs due to worsening respiratory failure.
This data emphasizes the use of IL-10 alone or in combination with CRP, IL-6 and PCT, to distinguish secondary infections from COVID-19 associated inflammation severely and critically ill COVID-19 patients. This biomarker may allow clinicians to omit unnecessary antimicrobial therapy. This hypothesis generating study warrants further interventional trials to evaluate the feasibility and safety of biomarker based use of antiinfectives.
Data availability statement
The original contributions presented in the study are included in this article/Supplementary material, further inquiries can be directed to the corresponding author/s.
Ethics statement
The studies involving human participants were reviewed and approved by the Ethics Commitee of the Medical University of Vienna, Vienna, Austria (No. 1404/2020). The patients or legal representatives provided their written informed consent to participate in this study if possible. If this was not feasible at the time of enrollment, informed consent was obtained from patients or their legal representatives at a later time if possible.
Author contributions
MWT, LT, and MKu wrote the manuscript and participated in the infectious disease panel. MWT gathered the patient data and performed the experiments. LT and MKu designed the study. HB, BJ, MO, FR, and OR critically read and revised the manuscript. MO performed additional laboratory analysis. FR performed the statistical analysis. TS, OR, MS, BR, and LT treated and recruited the patients. All authors read and approved the final manuscript.
Funding
This work was part of the ACOVACT study of the Medical University of Vienna and was financially supported by the Austrian Federal Ministry of Education, Science and Research.
Conflict of interest
The authors declare that the research was conducted in the absence of any commercial or financial relationships that could be construed as a potential conflict of interest.
Publisher’s note
All claims expressed in this article are solely those of the authors and do not necessarily represent those of their affiliated organizations, or those of the publisher, the editors and the reviewers. Any product that may be evaluated in this article, or claim that may be made by its manufacturer, is not guaranteed or endorsed by the publisher.
Supplementary material
The Supplementary Material for this article can be found online at: https://www.frontiersin.org/articles/10.3389/fmed.2022.917606/full#supplementary-material
Supplementary Figure 1 | Comparison of different biomarkers between patient with and without ECMO support. Figures consist of individual data points, the median and 95% confidence interval. Solitary data points outside of figure scales were set as maximum scale values and are shown as (X). For IL-10, procalcitonin and IL6/IL10 ratio, samples below the limit of detection were set as 0.1 for graphical representation. CRP, C-reactive protein; IL-6, Interleukin-6; IL-10, Interluekin-10; LDH, Lactate Dehydrogenase.
Supplementary Figure 2 | Heat map of correlation matrix between different biomarkers and clinical scores. The correlation is indicated via a color scale proportional to its strength of association, ranging from blue (negative correlation; negative values) to red (positive correlation; positive values). Each cell contains the pair-wise spearman correlation coefficient between the respective biomarkers from the overall population analyzed. PTZ, prothrombin time; INR, international normalized ratio; APTT, activated partial thromboplastin time; ASAT, aspartate transaminase; ALAT, alanine transaminase; LDH, lactate dehydrogenase; CRP, C-reactive protein; IL-6, interleukin 6; IL-10, interleukin 10.
Supplementary Table 1 | Table of raw patient data.
Supplementary Table 2 | Laboratory parameters for detection of secondary infections in COVID-19 ICU patients.
Supplementary Table 3 | Table of microbiological tests performed within 48 h of study inclusion.
References
1. Stokes EK. Coronavirus Disease 2019 case surveillance — United States, January 22–May 30, 2020. MMWR Morb Mortal Wkly Rep. (2020) 69:759–65. doi: 10.15585/mmwr.mm6924e2
2. Carbonell R, Urgelés S, Rodríguez A, Bodí M, Martín-Loeches I, Solé-Violán J, et al. Mortality comparison between the first and second/third waves among 3,795 critical COVID-19 patients with pneumonia admitted to the ICU: a multicentre retrospective cohort study. Lancet Reg Health Eur. (2021) 11:100243. doi: 10.1016/j.lanepe.2021.100243
3. Lansbury L, Lim B, Baskaran V, Lim WS. Co-infections in people with COVID-19: a systematic review and meta-analysis. J Infect. (2020) 81:266–75. doi: 10.1016/j.jinf.2020.05.046
4. Musuuza JS, Watson L, Parmasad V, Putman-Buehler N, Christensen L, Safdar N. Prevalence and outcomes of co-infection and superinfection with SARS-CoV-2 and other pathogens: a systematic review and meta-analysis. PLoS One. (2021) 16:e0251170. doi: 10.1371/journal.pone.0251170
5. Hernández-Terán A, Mejía-Nepomuceno F, Herrera MT, Barreto O, García E, Castillejos M, et al. Dysbiosis and structural disruption of the respiratory microbiota in COVID-19 patients with severe and fatal outcomes. Sci Rep. (2021) 11:21297. doi: 10.1038/s41598-021-00851-0
6. Uyeki TM, Bernstein HH, Bradley JS, Englund JA, File TM, Fry AM, et al. Clinical practice guidelines by the infectious diseases society of America: 2018 update on diagnosis, treatment, chemoprophylaxis, and institutional outbreak management of seasonal influenza. Clin Infect Dis. (2019) 68:e1–47. doi: 10.1093/cid/ciy866
7. Williams EJ, Mair L, de Silva TI, Green DJ, House P, Cawthron K, et al. Evaluation of procalcitonin as a contribution to antimicrobial stewardship in SARS-CoV-2 infection: a retrospective cohort study. J Hosp Infect. (2021) 110:103–7. doi: 10.1016/j.jhin.2021.01.006
8. Mason CY, Kanitkar T, Richardson CJ, Lanzman M, Stone Z, Mahungu T, et al. Exclusion of bacterial co-infection in COVID-19 using baseline inflammatory markers and their response to antibiotics. J Antimicrob Chemother. (2021) 76:1323–31. doi: 10.1093/jac/dkaa563
9. Kowalewski M, Fina D, Słomka A, Raffa GM, Martucci G, Lo Coco V, et al. COVID-19 and ECMO: the interplay between coagulation and inflammation—a narrative review. Crit Care. (2020) 24:205. doi: 10.1186/s13054-020-02925-3
10. Matsui K, Ikeda R. Peptidoglycan in combination with muramyldipeptide synergistically induces an interleukin-10-dependent T helper 2-dominant immune response. Microbiol Immunol. (2014) 58:260–5. doi: 10.1111/1348-0421.12139
11. Frodermann V, Chau TA, Sayedyahossein S, Toth JM, Heinrichs DE, Madrenas JA. Modulatory interleukin-10 response to staphylococcal peptidoglycan prevents Th1/Th17 adaptive immunity to Staphylococcus aureus. J Infect Dis. (2011) 204:253–62. doi: 10.1093/infdis/jir276
12. Rose WE, Shukla SK, Berti AD, Hayney MS, Henriquez KM, Ranzoni A, et al. Increased endovascular Staphylococcus aureus inoculum is the link between elevated serum interleukin 10 concentrations and mortality in patients with bacteremia. Clin Infect Dis. (2017) 64:1406–12. doi: 10.1093/cid/cix157
13. Rose WE, Eickhoff JC, Shukla SK, Pantrangi M, Rooijakkers S, Cosgrove SE, et al. Elevated serum interleukin-10 at time of hospital admission is predictive of mortality in patients with Staphylococcus aureus bacteremia. J Infect Dis. (2012) 206:1604–11. doi: 10.1093/infdis/jis552
14. European Centre for Disease Prevention and Control. Point prevalence survey of healthcare-associated infections and antimicrobial use in European acute care hospitals: protocol version 5.3: ECDC PPS 2016–2017. (2016). Available online at: https://data.europa.eu/doi/10.2900/374985 (Accessed June 18, 2021).
15. van Berkel M, Kox M, Frenzel T, Pickkers P, Schouten J, RCI-COVID-19 Study Group, et al. Biomarkers for antimicrobial stewardship: a reappraisal in COVID-19 times? Crit Care. (2020) 24:600. doi: 10.1186/s13054-020-03291-w
16. Pickens CO, Gao CA, Cuttica MJ, Smith SB, Pesce LL, Grant RA, et al. Bacterial superinfection pneumonia in patients mechanically ventilated for COVID-19 pneumonia. Am J Respir Crit Care Med. (2021) 204:921–32. doi: 10.1164/rccm.202106-1354OC
17. Russell CD, Fairfield CJ, Drake TM, Turtle L, Seaton RA, Wootton DG, et al. Co-infections, secondary infections, and antimicrobial use in patients hospitalised with COVID-19 during the first pandemic wave from the ISARIC WHO CCP-UK study: a multicentre, prospective cohort study. Lancet Microbe. (2021) 2:e354-65. doi: 10.1016/S2666-5247(21)00090-2
18. Bardi T, Pintado V, Gomez-Rojo M, Escudero-Sanchez R, Azzam Lopez A, Diez-Remesal Y, et al. Nosocomial infections associated to COVID-19 in the intensive care unit: clinical characteristics and outcome. Eur J Clin Microbiol Infect Dis. (2021) 40:495–502. doi: 10.1007/s10096-020-04142-w
19. De Bruyn A, Verellen S, Bruckers L, Geebelen L, Callebaut I, De Pauw I, et al. Secondary infection in COVID-19 critically ill patients: a retrospective single-center evaluation. BMC Infect Dis. (2022) 22:207. doi: 10.1186/s12879-022-07192-x
20. Zhang H, Zhang Y, Wu J, Li Y, Zhou X, Li X, et al. Risks and features of secondary infections in severe and critical ill COVID-19 patients. Emerg Microbes Infect. (2020) 9:1958–64. doi: 10.1080/22221751.2020.1812437
21. Patel P, DeCuir J, Abrams J, Campbell AP, Godfred-Cato S, Belay ED. Clinical characteristics of multisystem inflammatory syndrome in adults: a systematic review. JAMA Netw Open. (2021) 4:e2126456. doi: 10.1001/jamanetworkopen.2021.26456
22. Pink I, Raupach D, Fuge J, Vonberg R-P, Hoeper MM, Welte T, et al. C-reactive protein and procalcitonin for antimicrobial stewardship in COVID-19. Infection. (2021) 49:935–43. doi: 10.1007/s15010-021-01615-8
23. Islam H, Chamberlain TC, Mui AL, Little JP. Elevated interleukin-10 levels in COVID-19: potentiation of pro-inflammatory responses or impaired anti-inflammatory action? Front Immunol. (2021) 12:677008. doi: 10.3389/fimmu.2021.677008
24. Volk CF, Burgdorf S, Edwardson G, Nizet V, Sakoulas G, Rose WE. Interleukin (IL)-1β and IL-10 host responses in patients with Staphylococcus aureus bacteremia determined by antimicrobial therapy. Clin Infect Dis. (2020) 70:2634–40. doi: 10.1093/cid/ciz686
25. Minejima E, Bensman J, She RC, Mack WJ, Tran MT, Ny P, et al. A dysregulated balance of pro- and anti-inflammatory host cytokine response early during therapy predicts persistence and mortality in Staphylococcus aureus bacteremia. Crit Care Med. (2016) 44:671–9. doi: 10.1097/CCM.0000000000001465
26. Weidhase L, Wellhöfer D, Schulze G, Kaiser T, Drogies T, Wurst U, et al. Is interleukin-6 a better predictor of successful antibiotic therapy than procalcitonin and C-reactive protein? A single center study in critically ill adults. BMC Infect Dis. (2019) 19:150. doi: 10.1186/s12879-019-3800-2
27. Schuetz P, Albrich W, Mueller B. Procalcitonin for diagnosis of infection and guide to antibiotic decisions: past, present and future. BMC Med. (2011) 9:107. doi: 10.1186/1741-7015-9-107
28. Schmit X, Vincent JL. The time course of blood c-reactive protein concentrations in relation to the response to initial antimicrobial therapy in patients with sepsis. Infection. (2008) 36:213–9. doi: 10.1007/s15010-007-7077-9
29. Vincent JL, Moreno R, Takala J, Willatts S, De Mendonça A, Bruining H, et al. The SOFA (Sepsis-related Organ Failure Assessment) score to describe organ dysfunction/failure. On behalf of the working group on sepsis-related problems of the european society of intensive care medicine. Intensive Care Med. (1996) 22:707-710. doi: 10.1007/BF01709751
30. Le Gall JR, Lemeshow S, Saulnier F. A new simplified acute physiology score (SAPS II) based on a European/North American multicenter study. JAMA. (1993) 270:2957-2963. doi: 10.1001/jama.270.24.2957
31. Zimmerman JE, Kramer AA, McNair DS, Malila FM. Acute physiology and chronic health evaluation (APACHE) IV: hospital mortality assessment for today’s critically ill patients. Crit Care Med. (2006) 34:1297-1310. doi: 10.1097/01.CCM.0000215112.84523.F0
32. World Health Organization [WHO]. COVID-19 clinical management: living guidance, 25 January 2021. Document No: WHO/2019-nCoV/clinical/2021.1. Geneva: World Health Organization (2021).
Keywords: diagnosis differential, specificity and sensitivity, co-infection, interleukin-10, intensive care unit, extracorporeal membrane oxygenation (ECMO)
Citation: Weiss-Tessbach M, Ratzinger F, Obermueller M, Burgmann H, Staudinger T, Robak O, Schmid M, Roessler B, Jilma B, Kussmann M and Traby L (2022) Biomarkers for differentiation of coronavirus disease 2019 or extracorporeal membrane oxygenation related inflammation and bacterial/fungal infections in critically ill patients: A prospective observational study. Front. Med. 9:917606. doi: 10.3389/fmed.2022.917606
Received: 11 April 2022; Accepted: 14 September 2022;
Published: 06 October 2022.
Edited by:
Denise Battaglini, Unit of Anaesthesia and Intensive Care, San Martino Hospital (IRCCS), ItalyReviewed by:
Manabu Kinoshita, National Defense Medical College, JapanVasiliki Tsolaki, University Hospital of Larissa, Greece
Copyright © 2022 Weiss-Tessbach, Ratzinger, Obermueller, Burgmann, Staudinger, Robak, Schmid, Roessler, Jilma, Kussmann and Traby. This is an open-access article distributed under the terms of the Creative Commons Attribution License (CC BY). The use, distribution or reproduction in other forums is permitted, provided the original author(s) and the copyright owner(s) are credited and that the original publication in this journal is cited, in accordance with accepted academic practice. No use, distribution or reproduction is permitted which does not comply with these terms.
*Correspondence: Manuel Kussmann, manuel.kussmann@meduniwien.ac.at; Ludwig Traby, ludwig.traby@meduniwien.ac.at
†These authors have contributed equally to this work and share last authorship