- 1Manchester Centre for Genomic Medicine, St Mary’s Hospital, Manchester University Hospitals NHS Foundation Trust, Manchester, United Kingdom
- 2Division of Evolution, Infection and Genomics, School of Biological Sciences, The University of Manchester, Manchester, United Kingdom
- 3Division of Population Health, Manchester Centre for Health Economics, Health Services Research and Primary Care, School of Health Sciences, The University of Manchester, Manchester, United Kingdom
- 4Division of Informatics, Centre for Health Informatics, Imaging and Data Science, School of Health Sciences, The University of Manchester, Manchester, United Kingdom
- 5Division of Population Health, Centre for Primary Care and Health Services Research, Health Services Research and Primary Care, School of Health Sciences, The University of Manchester, Manchester, United Kingdom
Several healthcare organizations have developed pre-emptive pharmacogenetic testing programs, where testing is undertaken prior to the prescription of a medicine. This review characterizes the barriers and facilitators which influenced the development of these programs. A bidirectional citation searching strategy identified relevant publications before a standardized data extraction approach was applied. Publications were grouped by program and data synthesis was undertaken using the Consolidated Framework for Implementation Research (CFIR). 104 publications were identified from 40 programs and 4 multi-center initiatives. 26 (66%) of the programs were based in the United States and 95% in high-income countries. The programs were heterogeneous in their design and scale. The Characteristics of the Intervention, Inner Setting, and Process domains were referenced by 92.5, 80, and 77.5% of programs, respectively. A positive institutional culture, leadership engagement, engaging stakeholders, and the use of clinical champions were frequently described as facilitators to implementation. Clinician self-efficacy, lack of stakeholder knowledge, and the cost of the intervention were commonly cited barriers. Despite variation between the programs, there were several similarities in approach which could be categorized via the CFIR. These form a resource for organizations planning the development of pharmacogenetic programs, highlighting key facilitators which can be leveraged to promote successful implementation.
Introduction
Medicines are the most common therapeutic intervention in healthcare, yet their effectiveness and safety show considerable inter-personal variation (1, 2). Such variation is regularly attributed to the chosen dosing strategy, the accuracy of the initial diagnosis or individual factors, such as co-morbidities, polypharmacy or adherence. In addition, there is an increasing awareness that response to medicine is affected by an individual’s genetic variation, a concept known as pharmacogenetics. This has a significant personal, clinical, and financial impact, leading to poorer individual and population-level outcomes and waste of scarce healthcare resources (3, 4). Using the results of a pharmacogenetic test, embedded in a model of service delivery to inform prescribing decisions (hereafter “pharmacogenetics”) could lead to more accurate medicine selection and dosing, improving patient outcomes and better use of healthcare budgets (5).
Evidence-based guidelines to support pharmacogenetics are available for many commonly prescribed medicines (6–9). Despite a good understanding of these medicine-gene relationships, implementation of pharmacogenetics into clinical practice in many countries is limited to a small number of drug-gene pairs. This is typically carried out where variants in single genes are genotyped at the point of prescription (hereafter “reactive testing”) (10). Given the high population frequency of genetic variation which influences the effectiveness and safety of medicines, an alternative to reactive-testing is pre-emptive panel testing (11). This involves testing individuals for many common pharmacogenetic variants at a set time, irrespective of the medicine they are prescribed (hereafter “pre-emptive testing”). This information can then be stored in medical records and used to inform life-long prescription.
In several countries there is increasing interest in integrating pre-emptive pharmacogenetic testing into routine practice (12, 13). This represents a complex healthcare intervention comprising many interacting components and, as such, several factors influence the likelihood of successful implementation (14). Implementation is defined as the process of integrating evidence-based interventions within a setting (15). Pharmacogenetics represents one such evidence-based intervention, and the “implementation of pharmacogenetics” can be defined as the process by which gene-drug prescribing guidelines (the evidence base) are realized to guide prescribing for patients. Pharmacogenetic programs represent the organizational structures which aim to implement pharmacogenetics in practice. At present, there is no consensus around how pre-emptive pharmacogenetic programs should be designed. It is highly probable that programs designed to deliver pre-emptive pharmacogenetics will differ in their optimal design depending on the clinical and institutional context. Despite this, there are certain design decisions and methodological challenges which are common across programs (Figure 1) (16). The choices made when setting up individual programs, in response to individual organizational requirements, will result in varied approaches to implementation.
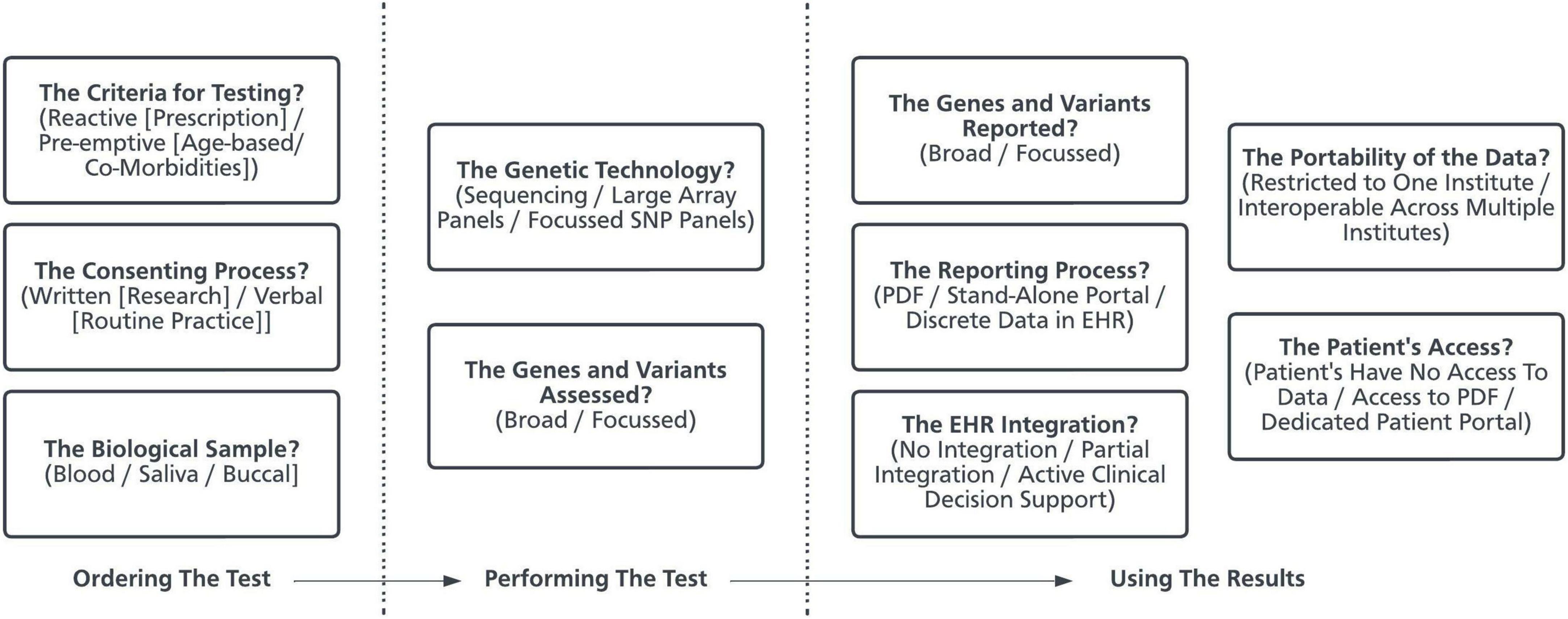
Figure 1. The decisions made when developing a pharmacogenetic program. Using pharmacogenetics in clinical practice is a complex healthcare intervention and is made up of many components (16, 80). This schematic presents some of the key design decisions made by organizations when developing a pharmacogenetic service. PDF, Portable Document Format. EHR, Electronic Health Record. SNP, Single Nucleotide Polymorphisms.
Structured conceptual frameworks provide an approach by which heterogeneous programs can be examined to systematically identify factors which influence implementation (17). Grounded in Diffusion of Innovations theory and widely empirically tested, the Consolidated Framework for Implementation Research (CFIR) is one such framework which can be applied systematically to identify factors that may emerge in various, multi-level contexts to influence implementation (17–19). Characterizing the barriers and facilitators which influenced the development of existing programs to implement pharmacogenetics can support and expedite the formation and development of new and existing initiatives. Common themes which appear to predict success can be included, whilst recurrent barriers can be addressed and overcome. This work identifies and synthesizes the literature describing the application of pre-emptive pharmacogenetics in clinical practice, characterizing the barriers and facilitators to implementation using the CFIR.
Methods
A structured literature review was undertaken to summarize the key characteristics of a pre-emptive pharmacogenetic testing program. A bidirectional citation search was used to systematically identify publications of interest followed by evidence-synthesis using the CFIR (20, 21).
Research aim
The study aimed to characterize the barriers and facilitators to the implementation of pre-emptive pharmacogenetics.
Identification of literature pool
Inclusion criteria
Original research published in peer-reviewed journals describing the implementation of a multi-gene (>1 gene) pharmacogenetic panel in clinical practice. For the purposes of this analysis, implementation was considered to have taken place where the results of a pharmacogenetic test were used to influence prescribing behavior. This included both clinical activity and research projects, where testing and prescribing was performed under research ethics.
Exclusion criteria
Systematic reviews or opinion-pieces were excluded. Original studies referenced in identified systematic reviews were identified using hand-searching of the reference lists and included. Conference abstracts and non-English language studies were also excluded.
Search strategy
A Bidirectional Citation Search was used to identify the literature of interest (20). This is a literature searching strategy which provides a systematic approach to maximize the identification of relevant articles in interdisciplinary topics. The starting pool (“initial pearls”) were identified via a pragmatic Boolean search of Medical Subject Heading (MeSH) Terms within Web of Science; MEDLINE (via OVID); Embase (via OVID); and PubMed Central on 1 February 2022. The following search [(((Pharmacogenetics) OR (Pharmacogenomics)) AND (Implementation)) AND (pre-emptive)] was used. No date limits were used. For each article, preliminary relevance was determined by review of the title and abstract. Articles were chosen as the initial pearls based on their breadth and quality, determined in part by pre-existing awareness of the literature and ensuring balance between articles describing research in primary and secondary care. Relevant articles known to the study team which were not identified via the Boolean search were added as further initial pearls. The collation of papers was conducted first by one author (JM) but, to assess the validity of article selection, 25% of the candidate papers was reviewed by a second author (SW). Inter-rater reliability was assessed via Cohen’s Kappa (κ) statistic.
Data extraction
Titles and abstracts of studies identified from the initial Boolean search and at each stage of the bidirectional citation search were screened for eligibility. Those studies deemed suitable for inclusion were assessed using a standardized pro forma (Supplementary Appendix), which included a section dedicated to the CFIR. The CFIR comprises a comprehensive taxonomy across five domains that are likely to influence the implementation of any innovation. These are:
I Intervention characteristics—the features of the innovation that may influence implementation.
II Inner setting—the ways by which organizational leadership, culture and delivery can influence implementation.
III Outer setting—the wider contextual or policy factors that might influence implementation and sustainability.
IV The characteristics, beliefs and attitudes of the individuals involved in implementation.
V How the process of implementation is actually enacted.
These domains are made up of different constructs and sub-constructs, each of which relate to a facet of implementation. Detailed definitions of the CFIR domains and constructs are provided in Supplementary Table 1.
Data extracted included geographic location, clinical setting, participants, ethical framework for undertaking pharmacogenetic testing, chosen testing strategy, integration with the Electronic Health Record (EHR), and mapping to the CFIR. To systematically categorize approaches to return pharmacogenetic test results to prescribers, a pragmatic classification system was developed which included 4 levels, ranging from the most (Level 4) to least (Level 1) disruption to routine prescribing behavior (Figure 2). Each manuscript was reviewed to assess whether a given CFIR domain was referred to. If present, the specific constructs were mapped, and a narrative summary of each domain undertaken. Whether the construct was referenced as a barrier or a facilitator to implementation was also recorded.

Figure 2. Approaches to deliver pharmacogenetic data to prescribers. Approaches to deliver pharmacogenetic data to prescribers were grouped into 4 categories which varied in how much they disrupted normal prescribing behavior. EHR, Electronic Health Record.
Data synthesis
Where several manuscripts described different facets of implementation at the same program, or described the development of a program over time, these were grouped together for analysis to provide an overview of the barriers and facilitators of pharmacogenetics at that specific program. Details of the geography, clinical setting, and participants of each program were summarized. A narrative summary was conducted to review the organizational and ethical frameworks used to undertake testing, specifically focusing on the criteria for testing and whether testing was undertaken on a purely clinical basis (requiring standard clinical consent only) or on a research basis (requiring research consent). Further narrative summaries were also performed to assess the chosen testing strategies, and the approaches to return test results to clinicians. A narrative summary was also constructed for each of the CFIR domains.
Results
The literature pool
Fifty-four unique articles were retrieved via the Boolean search and underwent independent title and abstract review by two authors (JHM and SW). Eight underwent full text review and 3 were chosen as initial pearls (Figure 3). Based on a pre-existing awareness of the literature, 2 further articles were added to the literature pool, resulting in 5 initial pearls entering the bidirectional citation search, agreed upon by all authors (22–26). Four screening rounds were required to complete the bidirectional citation search which involved the review of 8,355 abstracts. 104 relevant publications were identified for analysis (Figure 3). The inter-rater reliability showed 96.7% agreement with a Cohen’s kappa statistic of 0.71, representing substantial agreement.
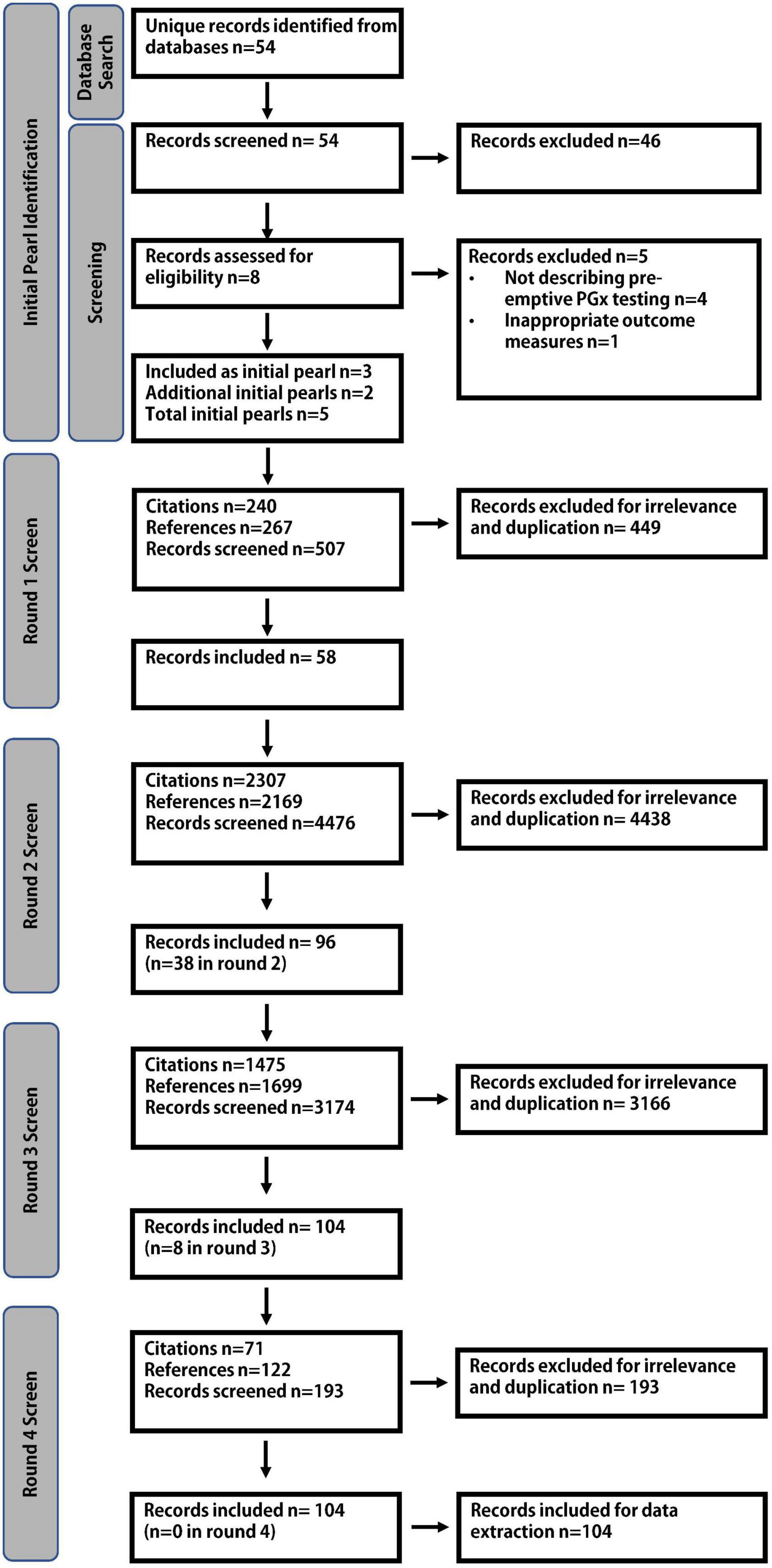
Figure 3. Literature search strategy. An initial Boolean literature search resulted in 5 initial pearls. Four rounds of screening were required to complete the bidirectional citation search, identifying 104 relevant publications.
Characteristics of the pharmacogenetic literature
The 104 publications mapped to 40 distinct programs and 4 multi-center initiatives, namely the US based Implementing GeNomics In pracTticE (IGNITE) and Electronic Medical Records and Genomics (eMERGE) programs, the international Pharmacogenomics Global Research Network (PGRN) initiative and the European U-PGX project (Supplementary Table 2). 26 (66%) of the programs were based in the United States, whilst Spain (3) and the Netherlands (2) had the greatest number of independent programs in Europe (Supplementary Figure 1 and Supplementary Table 2). Only 2 (5%) of programs were based in middle-income countries. The remaining 38 (95%) were located in high-income countries. Programs varied considerably in their scale and complexity. Some programs were referenced by multiple manuscripts, whereas others were described by a single manuscript only (range 1–10).
The eligibility for pharmacogenetic testing
Several organizations implemented pharmacogenetics within the context of a research trial, requiring formal written consent approved under research ethics. The structure and governance of these trials varied and, in part, was prescribed by the prevailing regulatory landscape for conducting clinical research (27, 28). Some of these studies were focused on specific populations or disease states as part of the research design. The Toronto based IMPACT study undertook testing in individuals treated with psychotropic medication, whilst the University of Chicago’s ImPreSS Trial aims to explore the usefulness of pre-emptive pharmacogenetic testing in the peri-operative setting (29, 30). Some initiatives recruited patients in certain age groups, the PHARM-GENOME-PACE study recruiting participants over the age of 55 years, and the University of Utah exploring benefits in those aged over 65 years of age (31, 32).
Several programs leveraged existing biobanks to implement pharmacogenetics. Mount Sinai Hospital’s CLIPMERGE PGx initiative enrolled patients to BioMe, an existing electronic health record (EHR) linked biobank (33). Similarly, The Mayo Clinic recruited participants from the Mayo Clinic Biobank for the Right Drug, Right Dose, Right Time (RIGHT) Trial (34). The University of Colorado also adopted a comparable approach, using the Colorado Center for Personalized Medicine Biobank to support its pharmacogenetic service (35).
Although some institutes implemented pharmacogenetics through research protocols with strict eligibility criteria, others developed a strategy where widely available pharmacogenetic programs were iteratively assessed via research methodologies. This is best exemplified at St Jude Children’s Hospitals, where pharmacogenetic testing is available for patients without restrictive eligibility criteria, though this still operates under research ethics (36). The University of Florida have utilized a similar model, although with some key differences. The institute has developed a pre-emptive pharmacogenetic gene panel which does not require research ethics, but the institute also engages in more targeted pharmacogenetic testing for specific indications such as selective serotonin reuptake inhibitors (SSRIs), opioid and Proton Pump Inhibitor (PPI) prescribing (37, 38). This more targeted testing has been undertaken under the auspices of research ethics, as part of pragmatic randomized trials.
There were several examples of pharmacogenetic programs which were undertaken on a clinical basis with no research consent required, rather than requiring distinct ethical approval. Most notably, the PREDICT project at Vanderbilt University Medical Center (VUMC), which has tested well over 10,000 patients, was designed as a quality improvement project, with the aim of better implementing FDA guidance for prescribing (39). During the initial design phase the PREDICT steering group, recognizing the seminal nature of the program, asked the local ethics committee to review the proposal to assess for any ethical concerns. As such, there is no formal informed consent process, beyond what would be expected as part of standard clinical practice, for pharmacogenetic testing at VUMC. Importantly, this approach has not impacted the PREDICT’s team’s ability to make major contributions to the pharmacogenetic literature (39, 40).
The genetic technology
An important component of any pharmacogenetic program is the choice of genetic technology—specifically the laboratory test which is able to identify whether the patient carries clinically relevant variants. There were differences between programs both in relation to the genes and variants tested (hereafter “targets”), and also with regard to the genetic technology used (Supplementary Table 2). However, the majority of programs adopted array-based platforms (26, 36, 41–46). These ranged from extremely broad systems, with the capacity to flexibly test for many thousands of variants across hundreds of genes, to more targeted gene panels (35). Where a service was designed around a specific indication, such as mental health or hypertension, panels were occasionally restricted to include genes and variants associated with medicines related to those diseases only (47–49). Array platforms from several commercial vendors were used by pharmacogenetic programs, and most systems allowed for an element of customization. These results demonstrate a lack of standardization in both panel design and platform.
Despite variability in the choice of test provider and target selection, almost all centers made use of genotyping rather than sequencing approaches, though there were two exceptions. The Hospital for Sick Children in Toronto, as part of a clinical trial, compared pre-emptive against reactive-testing (50). Pre-emptive pharmacogenetic testing involved the reanalysis of whole genome sequencing (WGS) data undertaken as part of separate investigations for congenital cardiac malformations. The Mayo Clinic developed PGRNseq for the RIGHT Study which is a Targeted Capture Sequencing Panel for over 250 genes (34, 41, 51).
Electronic health record integration
There were several strategies for how pharmacogenetic data was returned to be used at the point of prescribing to enable informed treatment choices. These were categorized into four main approaches which vary in their complexity and impact on typical prescribing behavior (Figure 2). The most disruptive approach (Level 4) was to produce a standalone report with no integration into the patient’s EHR. These reports were typically sent to the requesting clinician as PDF documents via e-mail. A variant of this approach was to develop a standalone pharmacogenetic interface which clinicians could interact with externally to their main EHR. Academics at the University of British Colombia developed a medication decision support system (MDDS) which via a series of logic trees would consider several patient variables, including pharmacogenetic variation, and recommend optimal therapeutic approaches for specific conditions (35). Critically, this was a distinct piece of software and not built into existing electronic prescribing tools. Most centers developed at least some level of integration with their EHR. The least complex approach was to upload a copy of the pharmacogenetics report to the EHR (Level 3), so it was available to view by the prescriber. The reports were predominantly uploaded as Portable Document Format (PDF) documents, though some centers were able to upload the results as discrete data.
Several programs have achieved more sophisticated levels of integration within their EHRs, and a number of manuscripts are dedicated to describing the technical methodologies these centers have utilized (42, 52–55). The general approach was to make use of EHR clinical decision support (CDS) functionality, specifically alert based decision support tools. In the EPIC® EHR, these are known as best practice advisories (BPAs). These would trigger under certain conditions and could be used to facilitate pharmacogenetic guided prescribing. The Cincinnati Children’s Hospital was initially unable to store pharmacogenetic data as a discrete variable within the EHR, meaning that drug-variant BPAs could not be developed (48). Instead, they developed an advisory alert indicating pharmacogenetic results were available within the EHR when certain medicines were prescribed (Level 2). This would then require the prescriber to search for the stored results if they wished to make use of them. Where organizations were able to store pharmacogenetic results as a discrete variable within their EHR, they were able to make use of active BPAs (Level 1) (36, 40, 42, 56–61). These would trigger when a drug was prescribed where there was a clinically actionable pharmacogenetic prescribing recommendation available.
Characterizing pharmacogenetic programs via the consolidated framework for implementation research
Not all programs were described in the same level of detail. The implementation efforts at some organizations were clearly described, with multiple manuscripts detailing their development over time (Supplementary Figure 2). Others meanwhile were described by single manuscripts with less detail. Comparing reporting within and between each CFIR domain meant that data from all programs delivering pharmacogenetics could be extracted and synthesized, highlighting overall barriers and facilitators in the identified literature. Certain domains and constructs were referenced significantly more frequently than others (Table 1 and Figure 4).
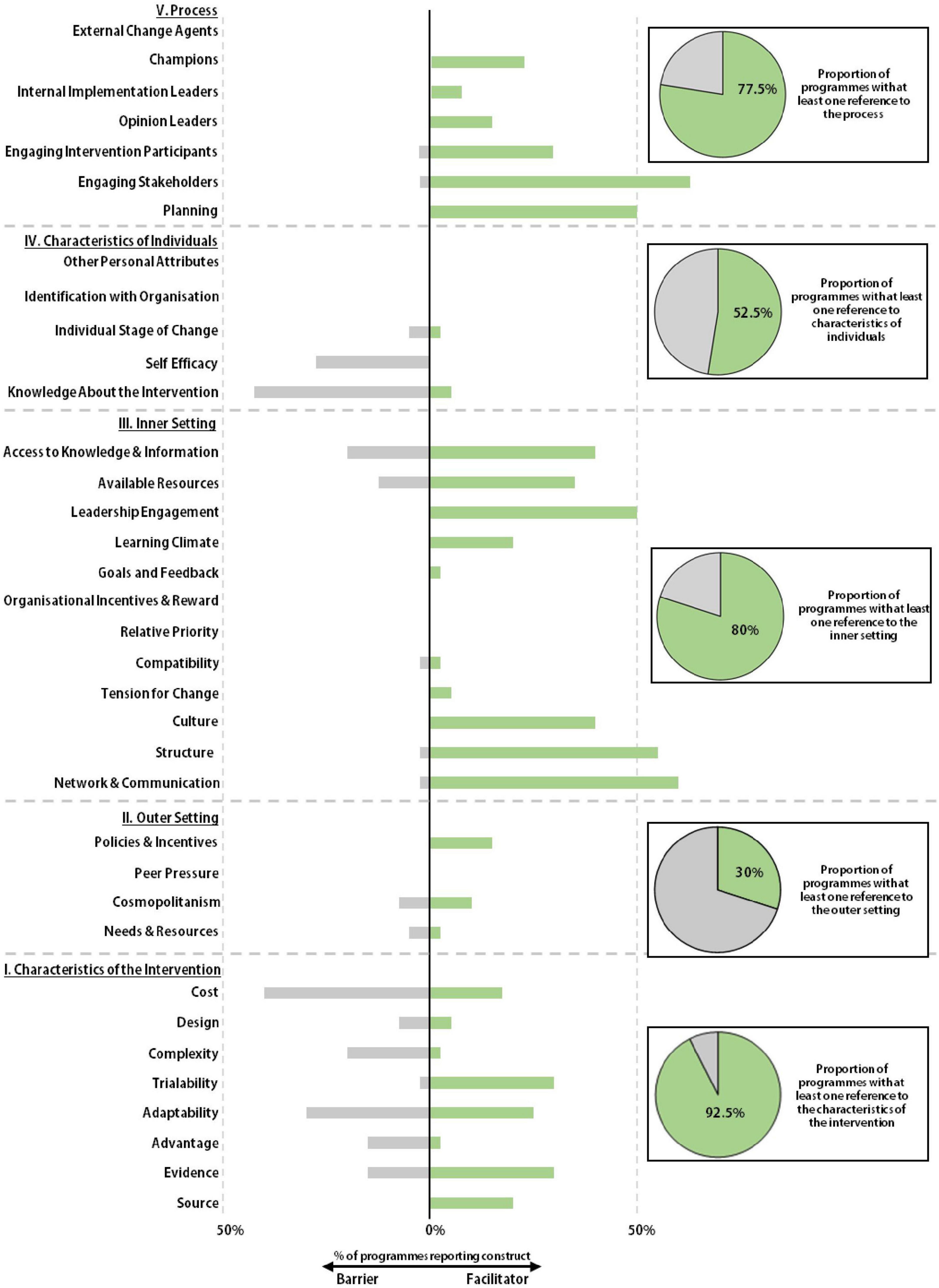
Figure 4. Facilitators and barriers to implementation. The Consolidated Framework for Implementation Research (CFIR) was used as a framework to identify the barriers and facilitators to implementation at each pharmacogenetic program. Whether a program referenced any construct within a given domain was recorded and displayed as a circle graph. Referenced CFIR constructs were recorded as being described as either a barrier or facilitator to implementation and displayed onto the stacked bar chart.
Domain I: Characteristics of the intervention
The Characteristics of the Intervention domain is comprised of eight constructs (Figure 4 and Supplementary Figure 3). Each intervention construct was cited by at least one of the identified programs and 92.5% of all programs made at least one reference to a construct within the domain. Adaptability was one of the most frequently mapped CFIR domains, discussed by 55% of all programs, 30% describing the construct as a barrier and 25% referencing it as a facilitator. In the context of a pharmacogenetic service, adaptability relates to how pharmacogenetic guided prescribing can be implemented without disrupting normal clinical practice. Authors from several institutes noted that tailoring their service to existing clinical practice resulted in higher levels of satisfaction amongst stakeholders.
The Eskenazi Health System, as part of the INGENIOUS trial, developed a pharmacogenetic consult service, analogous to existing pathology consultations in their institution (62). Where required, physicians could request support with pharmacogenetic guided prescribing, making use of established clinical pathways. Several programs were able to integrate pharmacogenetic data directly into their local EHRs, thus facilitating implementation. Centers which were unable to integrate results into their local EHRs via clinical decision support tools were more likely to discuss adaptability as a challenge or barrier to integration (63). One study which did not have BPA functionality noted that “developing CDS tools is critical for the clinical utility to parallel the longevity of PGx results,” whilst another concluded that “current electronic and paper laboratory test information stores relegate historical test findings to an ‘out of mind’ position for most clinicians” (47).
Cost was the most frequently discussed construct within the intervention domain and was overwhelmingly cited as a barrier to implementation, with 46% of programs discussing cost as a barrier. This barrier is related to the absolute dollar price of the test but also, predominantly in the US market, is related to the complexities of navigating the health insurance system where there is considerable variation in coverage and a lack of transparency around which tests can be ordered (64). 32% of programs discussed the strong evidence base which exists to support the implementation of pharmacogenetics as a facilitator, whilst 22% of programs noted stakeholders responded positively to the system being developed by their own institution. 31 (77.5%) of the programs made reference to CPIC in at least one publication, demonstrating the importance of these guidelines. However, it was not possible to directly assess how these guidelines impacted the design of the pharmacogenetic programs or the uptake from clinical stakeholders.
Domain II: Outer setting
The Outer Setting relates to the wider contextual or policy factors that might influence implementation and sustainability. This was one of the least referenced CFIR domains in the literature pool (Figure 4). Only 30% of programs referred to this domain, in contrast to the Inner Setting which nearly 80% of programs referred to at least once. The most frequent reference to the Outer Setting was in relation to the Policies and Incentives domain which was referenced by 15% of programs. This was typically in relation to US national collaboratives such as the IGNITE network and e-MERGE. Some organizations described their involvement in these groups and noted how they stimulated implementation activity and supported the sharing of best practice (53, 65). The concept of cosmopolitanism, the degree to which a pharmacogenetic program is networked with external organizations, was referred to by 18% of programs, meaning the scalability of pharmacogenetic data across organizations was not a commonly reported design attribute.
Domain III: Inner setting
The Inner Setting Domain within the CFIR considers whether an institute has the structure, institutional climate and the networks and communication to support implementation. It also considers an institution’s readiness for implementation, which corresponds to leadership engagement, the availability of resources to support implementation, and stakeholder access to relevant educational material. Many centers established educational programs and developed dedicated programs or departments to support implementation (26, 66, 67). A positive learning climate was described by 35% of programs and ready access to information was positively referenced by 40% of institutes. Where stakeholders were not provided with sufficient access to knowledge and information (20% of programs), or resources were not readily available (13% of programs), this was viewed as a barrier to implementation as clinicians struggled to interpret and therefore, implement the pharmacogenetic data.
Support from the host institution, whether fiscal, organizational, or simply prioritization of the initiative, was seen as a facilitator to the development of a successful implementation program. The Mayo Clinic, Vanderbilt University Hospitals and St. Jude Children’s Hospitals are three leading centers for pharmacogenetics. These programs are by no means identical, but there are notable similarities in their development. All three organizations formed internal program committees, with stakeholders from different specialities to oversee the growth and implementation of their service (36, 40, 68). Early leadership engagement was seen as one of the most important facilitators to the development and ongoing operation of a pharmacogenetic service, referenced by 50% of all programs.
Many pharmacogenetic services have been developed at large academic hospitals. These organizations are typically fertile environments for the implementation of new services, and this type of innovation is embedded into their institutional culture with 40% of programs referencing a supportive institutional environment as a facilitator. 60% of programs referenced the value of effective communication networks, whilst 55% of programs made note of the intrinsic structural characteristics of their institute (maturity, academic expertise, size etc.) as being important to successful implementation.
Domain IV: Characteristics of the individual
A total of 52.5% of all programs referred to a construct within the Characteristics of The Individual domain at least once (Figure 4). When referenced, constructs within this domain were mostly described as barriers to implementation. Knowledge and Beliefs about the Intervention, was a frequently considered construct within the pharmacogenetic literature, referred to by 48% of all programs. The majority of these programs highlighted this construct as a potential barrier to implementation. In an institutional profile of the Colorado Center for Personalized Medicine, the authors state that clinical stakeholders questioned the rationale for their testing approach and noted that “conversations about the pharmacogenomic initiative were side-tracked by concerns about genetic testing, which were largely a result of stakeholders’ experiences with genetic testing (for rare disease)” (35). The authors of several manuscripts across multiple organizations noted that real world evidence to support the widespread implementation of pharmacogenetics is somewhat variable, which could also impact beliefs about the utility of the intervention. A number of more recent manuscripts have begun to address this lack of evidence (51).
To improve clinical stakeholders’ knowledge and beliefs about the use of pharmacogenetics in clinical practice, several centers established educational programs. In 2017, the e-MERGE network published an overview of the educational programs operated by its 10 member organizations (69). All of the sites recognized the need to educate clinicians about pharmacogenetics either within the context of specific studies or as part of deploying CDS tools within the EHR. The educational strategies deployed by different programs varied in their scale, content and methodological approach (66, 67). Several sites made use of online resources which clinicians could access in their own time to support asynchronous learning. Some sites, such as the Mayo and Sanford Health, also offered more structured certificates to educate the workforce.
One major aim of these educational programs was to improve a clinician’s self-efficacy which, in the context of pharmacogenetics, refers to an individual’s confidence to perform pharmacogenetic guided prescribing via their institutional program. Self-efficacy, specifically clinician awareness or understanding of how to apply the pharmacogenetic data, was highlighted as barrier by 28% of programs (26, 70–73). Whether self-efficacy was improved by the educational initiatives can be inferred, to an extent, by the longevity and success of many initiatives, though this was not formally measured. Indeed, there are few studies which directly assess the impact of these programs on individual behavior. Rather, they focus on the global change in practice within an institution. An Individual’s Identification with the Organization and the Individual’s Stage of Change are two of the least mapped CFIR constructs within the pharmacogenetic literature, with no references identified during this study.
Domain V: Process
The Process domain can be summarized as how change has been enacted at a given institution. 77.5% of programs referenced at least one construct from within this domain. At many centers, steering committees were established to oversee development of pharmacogenetics programs. These groups were tasked with designing and implementing a program within an organization, meaning their effective functioning could be a powerful facilitator to successful implementation. This planning period appears to have been important in the development of several programs and was referred to by 50% of all institutes. When the Cincinnati Children’s Hospital Medical Center launched their pharmacogenetics program, they established a multi-disciplinary committee with representation from a broad range of specialities (48). The group would meet regularly to discuss all aspects of the program’s development and were able to cascade information back to their own clinical teams, promoting stakeholder engagement, the most consistently mapped construct within the pharmacogenetic literature, referenced as a facilitator by 63% of programs.
Engaging stakeholders, both clinical and non-clinical, is an important part of any program and impacts how an organization promotes adoption of the intervention. This can take the form of clinical representation on steering groups, as discussed above, but also includes the establishment of educational programs or the appointment of clinical champions. These individuals, typically chosen from within the organization, contribute to the implementation of an intervention through early adoption, promotion, and marketing. 23% of all programs referenced their use of clinical champions as a facilitator to implementation. A retrospective review of the Mission Health Personalized Medicine Program found that the use of physician champions, and ensuring they were supportive of the program in each speciality, was one of the most significant factors in the success of the program (74).
Discussion
This study has identified several key barriers and facilitators important to the development and implementation of pharmacogenetics. Using the CFIR, these constructs have been categorized into domains and constructs which highlight similarities between programs. The findings from this study may be beneficial to individual organizations or health care systems when developing programs in the future.
This review has identified a number of constructs which existing programs consistently cite, or fail to cite, which could highlight potential areas for focus within other healthcare systems (Table 1). Many programs had strong institutional support, early leadership engagement and learning cultures suited toward implementation. This will be important within any new program. However, whereas individual centers can manage their institutional eco-systems closely and foster support for implementation, this is more challenging across larger and more complex healthcare systems, such as in the United Kingdom. In nationally co-ordinated healthcare systems, there is no mechanism for individual hospitals to develop their own discrete pharmacogenetic program, as is the case in the US. This provides an argument to suggest that, within such healthcare systems, there should be support for early-adopter centers, or regional hubs, which can develop exemplar services followed by iterative expansion across the wider system. This would facilitate the trialability of pharmacogenetics, a frequently identified construct throughout the pharmacogenetic literature.
The Outer Setting was one of the least referenced domains within the literature. Identified manuscripts overwhelmingly focused on the development of a pharmacogenetic service at a single institution, rather than across a state-wide or national healthcare system. As such, the portability of pharmacogenetic data between different settings, or different EHRs, was not an essential consideration. This has obvious disadvantages where a patient’s care might be managed across various organizations, resulting in a need to share data across institutional boundaries. This is especially the case in healthcare systems where patients routinely transition between hospitals and primary care providers for their management. Even in the US, where many patients might receive a large proportion of their care from a single provider, there remains notable fragmentation for some users, disproportionally affecting vulnerable populations (75, 76). As such, in many healthcare settings the concept of Cosmopolitanism should be an essential design consideration. Designing an IT solution which can support pharmacogenetic guided prescribing for clinicians in an effective and equitable way across a healthcare system is a tractable problem, but one which will require considerable collaboration and effort. The data presented here suggests that launching programs to deliver pharmacogenetics without such a solution would be a significant barrier to uptake and reduce the potential long-term benefits, failing patients and clinicians.
It should be noted that domains and constructs within the CFIR do not exist in isolation of each other. Theoretical frameworks such as the CFIR are useful tools to catalog barriers and facilitators within implementation programs, but careful interpretation of these mapping exercises is required to make informed connections between the mapped domains. For example, the design of a pharmacogenetic prescribing system and the strength of evidence supporting the intervention will both impact on stakeholder engagement. Although these are separate constructs within the Characteristics of the Implementation domain, their impact in combination is arguably greater than their individual relevance. For example, the evidence collated within this review suggests that a well evidenced gene-drug interaction has little chance of successful implementation without a properly designed EHR system to deliver the pharmacogenetic data to prescribers in a usable format at the point of use. Equally, a well-designed EHR has limited usefulness if there is only poor evidence for a specific gene-drug pair. Furthermore many constructs will be positively correlated. For example, a positive institutional culture, which promotes the adoption of new technologies, is very likely to be correlated with an effective organizational structure with strong networks of communication as was the case for several leading pharmacogenetic programs.
The IGNITE network has previously surveyed their member organizations and used the CFIR as a tool to categorize and assess their responses (77–79). When IGNITE project teams were asked to rate the CFIR constructs in order of perceived value for genomic medicine, the findings were consistent with those identified in this study (77). No high-priority constructs were identified in the Outer Setting, whilst engaging stakeholders, a positive institutional culture and individual knowledge and beliefs were recognized as important constructs. A similar exercise, undertaken with IGNITE members who had implemented pharmacogenetic guided prescribing for antidepressants, asked stakeholders to choose the CFIR constructs considered “most important” in each domain. There is a good correlation between these “most important” constructs, and the recurrent constructs identified in this work (Table 1). Where inconsistencies exist, they are most likely explained by the different methodologies used to identify key constructs. The IGNITE studies made use of survey-based methods, whereas this work utilized a retrospective analysis of the published literature. Each approach has its advantages and limitations, but the consistency in constructs considered importance in these manuscripts suggests the findings can be considered reliable.
This study has limitations which are important to consider when interpreting the extracted data. Firstly, the identified manuscripts are subject to survivor bias. Some organizations may have chosen not to publish manuscripts detailing the development of their programs and the ones that have may skew toward larger academic health centers which may not be entirely representative of the pharmacogenetic implementation initiatives. Furthermore, there may be a reporting bias in how institutes describe their programs. Programs may not have disclosed some of the barriers they faced or may have made the decision to focus on certain aspects of implementation over others. For example, although we have interpreted the lack of reference to the Outer Setting as a sign that this was less frequently considered by programs, it is possible that this it is routinely considered though less frequently reported in the literature. As such, other research methodologies such as semi-structured interviews should be used to supplement and corroborate these data in the future. Finally, the majority of identified programs were US based and are unlikely to be entirely comparable to how pharmacogenetic services might be developed in other healthcare systems.
Conclusion
This study identified a large number of programs to deliver pharmacogenetics with varying designs across a wide geography and timespan. Despite heterogeneity, there were a number of similarities identified which could be categorized using a structured implementation framework. These form a useful resource for organizations approaching the development of pharmacogenetic services. Early engagement of stakeholders, developing a fertile implementation climate, the development of adaptable IT solutions and an iterative approach to implementation are all fundamental constructs which should be considered when implementing pharmacogenetics.
Data availability statement
The original contributions presented in this study are included in the article/Supplementary material, further inquiries can be directed to the corresponding author/s.
Author contributions
JM and PW developed the concept for the manuscript. JM and SW identified and reviewed the literature. JM undertook the analysis with support of SW, WN, and PW. JM drafted the manuscript with critical review from all authors. All authors read and approved the final version of this manuscript and contributed to the development of the methodology.
Funding
JM was supported by the National Institute for Health and Care Research (NIHR) Doctoral Fellowship Award (DRF) (NIHR 301748). WN and JM were supported by the Manchester NIHR Biomedical Research Center BRC grant code: (IS-BRC-1215-20007).
Conflict of interest
The authors declare that the research was conducted in the absence of any commercial or financial relationships that could be construed as a potential conflict of interest.
Publisher’s note
All claims expressed in this article are solely those of the authors and do not necessarily represent those of their affiliated organizations, or those of the publisher, the editors and the reviewers. Any product that may be evaluated in this article, or claim that may be made by its manufacturer, is not guaranteed or endorsed by the publisher.
Author disclaimer
The views expressed in this publication are those of the authors and not necessarily those of the NHS, the National Institute for Health Research or the Department of Health and Social Care.
Supplementary material
The Supplementary Material for this article can be found online at: https://www.frontiersin.org/articles/10.3389/fmed.2022.945352/full#supplementary-material
References
1. Royal Pharmaceutical Society. Medicines Optimisation: Helping Patients To Make The Most Of Medicines. London: Royal Pharmaceutical Society (2013).
2. Scholes S, Faulding S, Mindell J. The Health Survey For England: Use Of Prescribed Medicines. London: Office for National Statistics (2013).
3. Davies EC, Green CF, Taylor S, Williamson PR, Mottram DR, Pirmohamed M. Adverse drug reactions in hospital in-patients: a prospective analysis of 3695 patient-episodes. PLoS One. (2009) 4:e4439. doi: 10.1371/journal.pone.0004439
4. Pirmohamed M, James S, Meakin S, Green C, Scott AK, Walley TJ, et al. Adverse drug reactions as cause of admission to hospital: prospective analysis of 18 820 patients. BMJ. (2004) 329:15–9. doi: 10.1136/bmj.329.7456.15
5. Shabaruddin FH, Fleeman ND, Payne K. Economic evaluations of personalized medicine: existing challenges and current developments. Pharmgenomics Pers Med. (2015) 8:115–26.
6. Amstutz U, Henricks LM, Offer SM, Barbarino J, Schellens JHM, Swen JJ, et al. Clinical Pharmacogenetics Implementation Consortium (CPIC) Guideline for Dihydropyrimidine Dehydrogenase Genotype and Fluoropyrimidine Dosing: 2017 Update. Clin pharmacol Therapeut. (2018) 103:210. doi: 10.1002/cpt.911
7. Crews KR, Gaedigk A, Dunnenberger HM, Klein TE, Shen DD, Callaghan JT, et al. Clinical Pharmacogenetics Implementation Consortium (CPIC) guidelines for codeine therapy in the context of cytochrome P450 2D6 (CYP2D6) genotype. Clin Pharmacol Ther. (2012) 91:321–6. doi: 10.1038/clpt.2011.287
8. Hicks J, Bishop J, Sangkuhl K, Müller D, Ji Y, Leckband S, et al. Clinical pharmacogenetics implementation consortium (CPIC) Guideline for CYP2D6 and CYP2C19 genotypes and dosing of selective serotonin reuptake inhibitors. Clin Pharmacol Ther. (2015) 98:127–34.
9. McDermott JH, Wolf J, Hoshitsuki K, Huddart R, Caudle KE, Whirl-Carrillo M, et al. Clinical Pharmacogenetics Implementation Consortium (CPIC) guideline for the use of aminoglycosides based on MT-RNR1 genotype. Clin Pharmacol Ther. (2021) 25:366–72. doi: 10.1002/cpt.2309
10. Krebs K, Milani L. Translating pharmacogenomics into clinical decisions: do not let the perfect be the enemy of the good. Hum Genomics. (2019) 13:39.
11. McInnes G, Lavertu A, Sangkuhl K, Klein TE, Whirl−Carrillo M, Altman RB. Pharmacogenetics at Scale: an analysis of the UK Biobank. Clin Pharmacol Therapeut. (2021) 109:1528–37.
12. van der Wouden CH, Cambon-Thomsen A, Cecchin E, Cheung KC, Dávila-Fajardo CL, Deneer VH, et al. Implementing Pharmacogenomics in Europe: design and implementation strategy of the ubiquitous pharmacogenomics consortium. Clin Pharmacol Therapeut. (2017) 101:341–58.
13. Keeling NJ, Rosenthal MM, West-Strum D, Patel AS, Haidar CE, Hoffman JM. Preemptive pharmacogenetic testing: exploring the knowledge and perspectives of US payers. Genet Med. (2019) 21:1224–32. doi: 10.1038/gim.2017.181
14. Skivington K, Matthews L, Simpson SA, Craig P, Baird J, Blazeby JM, et al. A new framework for developing and evaluating complex interventions: update of Medical Research Council guidance. BMJ. (2021) 374:n2061.
15. Rabin BA, Brownson RC, Haire-Joshu D, Kreuter MW, Weaver NLA. Glossary for Dissemination and Implementation Research in Health. J Public Health Manag Pract. (2008) 14:117–23. doi: 10.1097/01.PHH.0000311888.06252.bb
16. Turner RM, Newman WG, Bramon E, McNamee CJ, Wong WL, Misbah S, et al. Pharmacogenomics in the UK National Health Service: opportunities and challenges. Pharmacogenomics. (2020) 21:1237–46.
17. Keith RE, Crosson JC, O’Malley AS, Cromp D, Taylor EF. Using the consolidated framework for implementation research (CFIR) to produce actionable findings: a rapid-cycle evaluation approach to improving implementation. Implement Sci. (2017) 12:15. doi: 10.1186/s13012-017-0550-7
18. Kirk MA, Kelley C, Yankey N, Birken SA, Abadie B, Damschroder L. A systematic review of the use of the Consolidated Framework for Implementation Research. Implement Sci. (2016) 11:72.
19. Rogers EM. A prospective and retrospective look at the diffusion model. J Health Commun. (2004) 9, Suppl 1:13–9. doi: 10.1080/10810730490271449
20. Hinde S, Spackman E. Bidirectional citation searching to completion: an exploration of literature searching methods. Pharmacoeconomics. (2015) 33:5–11.
21. Damschroder LJ, Aron DC, Keith RE, Kirsh SR, Alexander JA, Lowery JC. Fostering implementation of health services research findings into practice: a consolidated framework for advancing implementation science. Implement Sci.? (2009) 4:50. doi: 10.1186/1748-5908-4-50
22. David V, Fylan B, Bryant E, Smith H, Sagoo GS, Rattray M. An analysis of pharmacogenomic-guided pathways and their effect on medication changes and hospital admissions: a systematic review and meta-analysis. Front Genet. (2021) 12:698148. doi: 10.3389/fgene.2021.698148
23. Duarte JD, Dalton R, Elchynski AL, Smith DM, Cicali EJ, Lee JC, et al. Multisite investigation of strategies for the clinical implementation of pre-emptive pharmacogenetic testing. Genet Med. (2021) 23:2335–41. doi: 10.1038/s41436-021-01269-9
24. Hayward J, McDermott J, Qureshi N, Newman W. Pharmacogenomic testing to support prescribing in primary care: a structured review of implementation models. Pharmacogenomics. (2021) 22:761–76. doi: 10.2217/pgs-2021-0032
25. Jameson A, Fylan B, Bristow GC, Sagoo GS, Dalton C, Cardno A, et al. What are the barriers and enablers to the implementation of pharmacogenetic testing in mental health care settings? Front Genet. (2021) 12:740216. doi: 10.3389/fgene.2021.740216
26. Thornley T, Esquivel B, Wright DJ, Dop HVD, Kirkdale CL, Youssef E. Implementation of a pharmacogenomic testing service through community pharmacy in the netherlands: results from an early service evaluation. Pharmacy (Basel). (2021) 9:38. doi: 10.3390/pharmacy9010038
27. Lukaseviciene V, Hasford J, Lanzerath D, Gefenas E. Implementation of the EU clinical trial regulation transforms the ethics committee systems and endangers ethical standards. J Med Ethics. (2021) 47:e82.
28. Giannuzzi V, Devlieger H, Margari L, Odlind VL, Ragab L, Bellettato CM, et al. The ethical framework for performing research with rare inherited neurometabolic disease patients. Eur J Pediatr. (2017) 176:395–405.
29. Truong TM, Apfelbaum J, Shahul S, Anitescu M, Danahey K, Knoebel RW, et al. The impress trial: implementation of point-of-care pharmacogenomic decision support in perioperative care. Clin Pharmacol Ther. (2019) 106:1179–83. doi: 10.1002/cpt.1567
30. Herbert D, Neves-Pereira M, Baidya R, Cheema S, Groleau S, Shahmirian A, et al. Genetic testing as a supporting tool in prescribing psychiatric medication: design and protocol of the IMPACT study. J Psychiatr Res. (2018) 96:265–72. doi: 10.1016/j.jpsychires.2017.09.002
31. Bain KT, Schwartz EJ, Knowlton OV, Knowlton CH, Turgeon J. Implementation of a pharmacist-led pharmacogenomics service for the Program of All-Inclusive Care for the Elderly (PHARM-GENOME-PACE). J Am Pharm Assoc. (2018) 58:281–9. doi: 10.1016/j.japh.2018.02.011
32. Brixner D, Biltaji E, Bress A, Unni S, Ye X, Mamiya T, et al. The effect of pharmacogenetic profiling with a clinical decision support tool on healthcare resource utilization and estimated costs in the elderly exposed to polypharmacy. J Med Econ. (2016) 19:213–28. doi: 10.3111/13696998.2015.1110160
33. Gottesman O, Scott SA, Ellis SB, Overby CL, Ludtke A, Hulot JS, et al. The CLIPMERGE PGx Program: clinical implementation of personalized medicine through electronic health records and genomics-pharmacogenomics. Clin Pharmacol Ther. (2013) 94:214–7. doi: 10.1038/clpt.2013.72
34. Bielinski SJ, St Sauver JL, Olson JE, Larson NB, Black JL, Scherer SE, et al. Cohort profile: the right drug, right dose, right time: using genomic data to individualize treatment protocol (RIGHT Protocol). Int J Epidemiol. (2020) 49:23–4. doi: 10.1093/ije/dyz123
35. Aquilante CL, Kao DP, Trinkley KE, Lin CT, Crooks KR, Hearst EC, et al. Clinical implementation of pharmacogenomics via a health system-wide research biobank: the University of Colorado experience. Pharmacogenomics. (2020) 21:375–86. doi: 10.2217/pgs-2020-0007
36. Hoffman JM, Haidar CE, Wilkinson MR, Crews KR, Baker DK, Kornegay NM, et al. PG4KDS: A Model for the Clinical Implementation of Pre-emptive Pharmacogenetics. Am J Med Genet C Semin Med Genet. (2014) 166c:45–55. doi: 10.1002/ajmg.c.31391
37. Cicali EJ, Weitzel KW, Elsey AR, Orlando FA, Vinson M, Mosley S, et al. Challenges and lessons learned from clinical pharmacogenetic implementation of multiple gene–drug pairs across ambulatory care settings. Genet Med. (2019) 21:2264–74. doi: 10.1038/s41436-019-0500-7
38. Marrero RJ, Cicali EJ, Arwood MJ, Eddy E, DeRemer D, Ramnaraign BH, et al. How to transition from single gene pharmacogenetic testing to preemptive panel-based testing: a tutorial. Clin Pharmacol Ther. (2020) 108:557–65. doi: 10.1002/cpt.1912
39. Driest SLV, Shi Y, Bowton EA, Schildcrout JS, Peterson JF, Pulley J, et al. Clinically actionable genotypes among 10,000 patients with preemptive pharmacogenomic testing. Clin Pharmacol Therapeut. (2014) 95:423. doi: 10.1038/clpt.2013.229
40. Pulley JM, Denny JC, Peterson JF, Bernard GR, Vnencak-Jones CL, Ramirez AH, et al. Operational implementation of prospective genotyping for personalized medicine: the design of the Vanderbilt PREDICT project. Clin Pharmacol Ther. (2012) 92:87–95. doi: 10.1038/clpt.2011.371
41. Gordon AS, Fulton RS, Qin X, Mardis ER, Nickerson DA, Scherer S. PGRNseq: a targeted capture sequencing panel for pharmacogenetic research and implementation. Pharmacogenet Genomics. (2016) 26:161–8. doi: 10.1097/FPC.0000000000000202
42. Manzi SF, Fusaro VA, Chadwick L, Brownstein C, Clinton C, Mandl KD, et al. Creating a scalable clinical pharmacogenomics service with automated interpretation and medical record result integration - experience from a pediatric tertiary care facility. J Am Med Inform Assoc. (2017) 24:74–80. doi: 10.1093/jamia/ocw052
43. Keller MA, Gordon ES, Stack CB, Gharani N, Sill CJ, Schmidlen TJ, et al. Coriell Personalized Medicine Collaborative§: a prospective study of the utility of personalized medicine. Per Med. (2010) 7:301–17.
44. Kitzmiller JP, Embi PJ, Manickam K, Sweet KM, Phelps MA, Jackson RD, et al. Program in pharmacogenomics at the Ohio State University Medical Center. Pharmacogenomics. (2012) 13:751–6.
45. Niedrig DF, Rahmany A, Heib K, Hatz KD, Ludin K, Burden AM, et al. Clinical relevance of a 16-gene pharmacogenetic panel test for medication management in a cohort of 135 patients. J Clin Med. (2021) 10:3200. doi: 10.3390/jcm10153200
46. Patel PD, Vimalathas P, Niu X, Shannon CN, Denny JC, Peterson JF, et al. CYP2C19 loss-of-function is associated with increased risk of ischemic stroke after transient ischemic attack in intracranial atherosclerotic disease. J Stroke Cerebrovasc Dis. (2021) 30:105464. doi: 10.1016/j.jstrokecerebrovasdis.2020.105464
47. Dunbar L, Butler R, Wheeler A, Pulford J, Miles W, Sheridan J. Clinician experiences of employing the AmpliChip§CYP450 test in routine psychiatric practice. J Psychopharmacol. (2012) 26:390–7. doi: 10.1177/0269881109106957
48. Ramsey LB, Prows CA, Zhang K, Saldaña SN, Sorter MT, Pestian JP, et al. Implementation of Pharmacogenetics at Cincinnati Children’s Hospital Medical Center: Lessons learned over 14 years of personalizing medicine. Clin Pharmacol Therapeut. (2019) 105:49. doi: 10.1002/cpt.1165
49. Wang Y, Xiao F, Chen Y, Xiao LD, Wang LY, Zhan Y, et al. Analytics of the clinical implementation of pharmacogenomics testing in 12 758 individuals. Clin Transl Med. (2021) 11:e586. doi: 10.1002/ctm2.586
50. Cohn I, Manshaei R, Liston E, Okello JBA, Khan R, Curtis MR, et al. Assessment of the implementation of pharmacogenomic testing in a pediatric tertiary care setting. JAMA Netw Open. (2021) 4:e2110446. doi: 10.1001/jamanetworkopen.2021.10446
51. Wang L, Scherer SE, Bielinski SJ, Muzny DM, Jones LA, Black JL, et al. Implementation of preemptive DNA sequence–based pharmacogenomics testing across a large academic medical center: The Mayo-Baylor RIGHT 10K Study. Genet Med. (2022) 24:1062–72. doi: 10.1016/j.gim.2022.01.022
52. Hicks JK, Dunnenberger HM, Gumpper KF, Haidar CE, Hoffman JM. Integrating pharmacogenomics into electronic health records with clinical decision support. Am J Health Syst Pharm. (2016) 73:1967–76.
53. Rasmussen-Torvik LJ, Stallings SC, Gordon AS, Almoguera B, Basford MA, Bielinski SJ, et al. Design and anticipated outcomes of the eMERGE-PGx Project: a multi-center pilot for pre-emptive pharmacogenomics in electronic health record systems. Clin Pharmacol Therapeut. (2014) 96:482. doi: 10.1038/clpt.2014.137
54. Bell GC, Crews KR, Wilkinson MR, Haidar CE, Hicks JK, Baker DK, et al. Development and use of active clinical decision support for preemptive pharmacogenomics. J Am Med Inform Assoc. (2014) 21:e93–9. doi: 10.1136/amiajnl-2013-001993
55. Haidar CE, Relling MV, Hoffman JM. Preemptively precise: returning and updating pharmacogenetic test results to realize the benefits of preemptive testing. Clin Pharmacol Ther. (2019) 106:942–4. doi: 10.1002/cpt.1613
56. Gill PS, Yu FB Jr., Porter-Gill PA, Boyanton BL Jr., Allen JC, Farrar JE, et al. Implementing pharmacogenomics testing: single center experience at arkansas children’s hospital. J Pers Med. (2021) 11:394. doi: 10.3390/jpm11050394
57. Pasternak AL, Ward KM, Ateya MB, Choe HM, Thompson AN, Clark JS, et al. Establishment of a pharmacogenetics service focused on optimizing existing pharmacogenetic testing at a large academic health center. J Pers Med. (2020) 10:E154. doi: 10.3390/jpm10040154
58. Bank PCD, Swen JJ, Schaap RD, Klootwijk DB, Pablo RB, Guchelaar HJ. A pilot study of the implementation of pharmacogenomic pharmacist initiated pre-emptive testing in primary care. Eur J Hum Genet. (2019) 27:1532–41. doi: 10.1038/s41431-019-0454-x
59. Sukasem C, Jantararoungtong T, Koomdee N. Pharmacogenomics research and its clinical implementation in Thailand: lessons learned from the resource-limited settings. Drug Metab Pharmacokinet. (2021) 39:100399. doi: 10.1016/j.dmpk.2021.100399
60. Schneider TM, Eadon MT, Cooper-DeHoff RM, Cavanaugh KL, Nguyen KA, Arwood MJ, et al. Multi-Institutional Implementation of Clinical Decision Support for APOL1, NAT2, and YEATS4 Genotyping in Antihypertensive Management. J Pers Med. (2021) 11:480. doi: 10.3390/jpm11060480
61. Scott SA, Owusu Obeng A, Botton MR, Yang Y, Scott ER, Ellis SB, et al. Institutional profile: translational pharmacogenomics at the Icahn School of Medicine at Mount Sinai. Pharmacogenomics. (2017) 18:1381–6. doi: 10.2217/pgs-2017-0137
62. Eadon MT, Desta Z, Levy KD, Decker BS, Pierson RC, Pratt VM, et al. Implementation of a Pharmacogenomics Consult Service to Support the INGENIOUS Trial. Clin Pharmacol Therapeut. (2016) 100:63. doi: 10.1002/cpt.347
63. Brown JT, MacDonald D, Yapel A, Luczak T, Hanson A, Stenehjem DD. Integrating pharmacogenetic testing via medication therapy management in an outpatient family medicine clinic. Pharmacogenomics. (2021) 22:203–12. doi: 10.2217/pgs-2020-0178
64. Park SK, Thigpen J, Lee IJ. Coverage of pharmacogenetic tests by private health insurance companies. J Am Pharm Assoc. (2020) 60:352–6. doi: 10.1016/j.japh.2019.10.003
65. Cavallari LH, Beitelshees AL, Blake KV, Dressler LG, Duarte JD, Elsey A, et al. The IGNITE Pharmacogenetics Working Group: an opportunity for building evidence with pharmacogenetic implementation in a real−world setting. Clin Transl Sci. (2017) 10:143. doi: 10.1111/cts.12456
66. Formea CM, Nicholson WT, Vitek CR. An inter-professional approach to personalized medicine education: one institution’s experience. Pers Med. (2015) 12:129. doi: 10.2217/pme.14.63
67. Bishop JR, Huang RS, Brown JT, Mroz P, Johnson SG, Allen JD, et al. Pharmacogenomics education, research and clinical implementation in the state of Minnesota. Pharmacogenomics. (2021) 22:681–91.
68. Farrugia G, Weinshilboum RM. Challenges in Implementing Genomic Medicine: The Mayo Clinic Center for Individualized Medicine. Clin Pharmacol Therapeut. (2013) 94:204. doi: 10.1038/clpt.2013.52
69. Vitek CRR, Abul-Husn NS, Connolly JJ, Hartzler AL, Kitchner T, Peterson JF, et al. Healthcare provider education to support integration of pharmacogenomics in practice: the eMERGE Network experience. Pharmacogenomics. (2017) 18:1013. doi: 10.2217/pgs-2017-0038
70. Dunnenberger HM, Biszewski M, Bell GC, Sereika A, May H, Johnson SG, et al. Implementation of a multidisciplinary pharmacogenomics clinic in a community health system. Am J Health System Pharmacy. (2016) 73:1956–66. doi: 10.2146/ajhp160072
71. Borden BA, Galecki P, Wellmann R, Danahey K, Lee SM, Patrick-Miller L, et al. Assessment of provider-perceived barriers to clinical use of pharmacogenomics during participation in an institutional implementation study. Pharmacogenet Genomics. (2019) 29:31–8. doi: 10.1097/FPC.0000000000000362
72. O’Donnell PH, Bush A, Spitz J, Danahey K, Saner D, Das S, et al. The 1200 patients project: creating a new medical model system for clinical implementation of pharmacogenomics. Clin Pharmacol Therapeut. (2012) 92:446. doi: 10.1038/clpt.2012.117
73. St Sauver JL, Bielinski SJ, Olson JE, Bell EJ, Mc Gree ME, Jacobson DJ, et al. Integrating Pharmacogenomics into Clinical Practice: Promise vs Reality. Am J Med. (2016) 129:1093–9. doi: 10.1016/j.amjmed.2016.04.009
74. Dressler LG, Bell GC, Ruch KD, Retamal JD, Krug PB, Paulus RA. Implementing a personalized medicine program in a community health system. Pharmacogenomics. (2018) 19:1345–56. doi: 10.2217/pgs-2018-0130
75. Kaltenborn Z, Paul K, Kirsch JD, Aylward M, Rogers EA, Rhodes MT, et al. Super fragmented: a nationally representative cross-sectional study exploring the fragmentation of inpatient care among super-utilizers. BMC Health Serv Res. (2021) 21:338. doi: 10.1186/s12913-021-06323-5
76. Kern LM, Seirup JK, Rajan M, Jawahar R, Stuard SS. Fragmented ambulatory care and subsequent healthcare utilization among Medicare beneficiaries. Am J Manag Care. (2018) 24:e278–84.
77. Orlando LA, Sperber NR, Voils C, Nichols M, Myers RA, Wu RR, et al. Developing a common framework for evaluating the implementation of genomic medicine interventions in clinical care: the ignite network’s common measures working group. Genet Med. (2018) 20: 655–63.
78. Sperber NR, Carpenter JS, Cavallari LH, J Damschroder L, Cooper-DeHoff RM, Denny JC, et al. Challenges and strategies for implementing genomic services in diverse settings: experiences from the Implementing GeNomics In pracTicE (IGNITE) network. BMC Med Genomics. (2017) 10:35. doi: 10.1186/s12920-017-0273-2
79. Tuteja S, Salloum RG, Elchynski AL, Smith DM, Rowe E, Blake KV, et al. Multisite evaluation of institutional processes and implementation determinants for pharmacogenetic testing to guide antidepressant therapy. Clin Transl Sci. (2022) 15:371–83. doi: 10.1111/cts.13154
Keywords: pharmacogenetics, precision medicine, implementation science, medical informatics, pharmacogenomics
Citation: McDermott JH, Wright S, Sharma V, Newman WG, Payne K and Wilson P (2022) Characterizing pharmacogenetic programs using the consolidated framework for implementation research: A structured scoping review. Front. Med. 9:945352. doi: 10.3389/fmed.2022.945352
Received: 17 May 2022; Accepted: 29 July 2022;
Published: 18 August 2022.
Edited by:
Viviana Giannuzzi, Fondazione per la Ricerca Farmacologica Gianni Benzi Onlus, ItalyReviewed by:
Sony Tuteja, University of Pennsylvania, United StatesJulio Duarte, University of Florida, United States
Copyright © 2022 McDermott, Wright, Sharma, Newman, Payne and Wilson. This is an open-access article distributed under the terms of the Creative Commons Attribution License (CC BY). The use, distribution or reproduction in other forums is permitted, provided the original author(s) and the copyright owner(s) are credited and that the original publication in this journal is cited, in accordance with accepted academic practice. No use, distribution or reproduction is permitted which does not comply with these terms.
*Correspondence: John H. McDermott, am9obi5tY2Rlcm1vdHRAbWZ0Lm5ocy51aw==