- Department of Ophthalmology, Xinhua Hospital Affiliated to Shanghai Jiao Tong University School of Medicine, Shanghai, China
Familial exudative vitreoretinopathy (FEVR) is an important cause of childhood blindness and is clinically characterized by phenotypic heterogeneity. FEVR patients harboring the same genetic mutation vary widely in disease severity. The purpose of this study was to explore non-genetic factors that regulate FEVR phenotypic heterogeneity. We detected methylation levels of 21 CpG sites located at the FZD4 exon 1 region of 11 probands, 12 asymptomatic/paucisymptomatic carriers and 11 non-carriers from 10 unrelated FZD4-associated FEVR families using bisulfite amplicon sequencing (BSAS). Our results showed reduced methylation level of FZD4 exon 1 in probands, suggesting that FZD4 exon 1 methylation level may be negatively linked with FEVR disease severity. It provided a new research direction for follow-up research, helping us better understand the complexity of the FEVR-causing mechanism.
Introduction
Familial exudative vitreoretinopathy (FEVR) is a clinically and genetically heterogeneous ophthalmic disorder characterized by incomplete retinal vascular development. The disease was first described in 1969 by Criswick and Schepens (1), and it is a major cause of vision loss in juveniles. Several sight-threatening complications are considered secondary to retinal avascularity, including retinal neovascularization and exudates, retinal fold and detachments, vitreous hemorrhage, and macular ectopia, ultimately leading to total blindness. The clinical appearance of FEVR varies considerably. The mildly affected patients may have only a small avascular area at the peripheral retina, which is visible only by fundus fluorescein angiography (FFA), while severely affected patients often registered as blind during infancy. With extensive fundus screening, we now realize that the prevalence of FEVR is much higher than we thought before (2). Therefore, it is imperative to study the mechanism underlying FEVR in order to seek for advanced therapy.
In the past decades, research on FEVR has mainly focused on genomics. At least 9 genes have been reported to cause FEVR: norrin cystine knot growth factor NDP (NDP; MIM: 300658) (3); low-density lipoprotein receptor-related protein 5 (LRP5; MIM: 603506) (4), tetraspanin 12 (TSPAN12; MIM: 613138) (5), frizzled class receptor 4 (FZD4; MIM: 604579) (6, 7), kinesin family member 11 (KIF11; MIM:148760) (8, 9), zinc finger protein 408 (ZNF408; MIM: 616454) (10), catenin, β-1 (CTNNB1; MIM: 116806) (11, 12), jagged 1 (JAG1; MIN: 601920) (13), and catenin, α-1 (CTNNA1; MIM:116805) (14). These gene mutations explain approximately 50% of FEVR cases. Mutations in six of the nine genes (FZD4, TSPAN12, NDP, LRP5, CTNNB1, and CTNNA1) are related to the Norrin/ Wnt β-catenin signaling pathway, which coordinates retinal development and angiogenesis by regulating cell survival, differentiation, proliferation, and migration (15, 16). The NDP gene encodes the extracellular ligand Norrin, which binds specifically to the receptor/coreceptor (FZD4, LRP5 and TSPAN12) and forms a ligand-receptor complex. Upon activation of Norrin signaling, β-catenin translocates to the nucleus, binds to TCF/LEF (T cell factor/lymphoid-enhancing factor) and subsequently modulates gene expression (17–19). Mutations in CTNNA1 induce overactivation of Norrin/β-catenin signaling and interrupt cell junctions (20, 21). The inheritance patterns of FEVR include autosomal dominant (AD), autosomal recessive (AR), and X-linked recessive (XL), with AD being the most common mode. Despite clear definition of causative genes, mutations of the same gene often manifest with drastic phenotypic heterogeneity in carriers (2, 22, 23). First-degree relatives of index patients with severe FEVR are often asymptomatic despite carrying the same mutation (24). This suggests that other risk factors beyond genetic mutation may contribute to FEVR pathogenesis.
Gene expression variability is one of the important causes for phenotypic variation. Epigenetic modification affects gene expression. One of the best understood epigenetic modification is DNA methylation. DNA methylation usually occurs in the cytosine guanine (CpG) dinucleotide. It affects gene expression by changing chromatin structure, DNA conformation, DNA stability and protein accessibility to DNA (25, 26). CpG islands are regions in the genome where CpG levels are significantly higher than the average CpG levels of the whole gene. CpG islands usually are found in the promoter or exon 1 region. Although few studies addressed DNA methylation change in FEVR, methylation changes have been demonstrated in other ocular vitreoretinopathy diseases such as age-related macular degeneration (AMD), proliferative vitreoretinopathy (PVR), and diabetic retinopathy (DR) (27–30). Therefore, we hypothesized that DNA methylation changes exist in FEVR causative genes, and such changes could affect gene expression and leading to FEVR phenotypic heterogeneity.
In this study, 10 separate FZD4-associated FEVR families were recruited to investigate the FZD4 methylation status of FEVR probands and their asymptomatic/paucisymptomatic family members carrying the same mutation. Our results showed reduced FZD4 exon 1 methylation in probands, suggesting that FZD4 exon 1 methylation level may be negatively linked with FEVR disease severity. To the best of our knowledge, this is the first paper that attempts to investigate the association between DNA methylation and heterogeneous presentation of FEVR.
Methods
Subjects
760 probands were recruited based on a clinical diagnosis of FEVR at the ophthalmology department of Xinhua hospital from May 2010 to August 2019. Next Generation Sequencing (NGS) revealed that 112 probands carried only FZD4 gene mutations, without other known FEVR-associated gene mutation. We further collected family history information of these 112 FZD4 Gene mutation probands. Finally, 10 FZD4-associated FEVR families met the following inclusion criteria were recruited. each enrolled family contained at least one FEVR proband (Staged 3–5), one asymptomatic/ paucisymptomatic carrier of the mutant allele (clinically healthy or staged 1) and one non-carrier. Stage 2 individuals were excluded to maximize the phenotypic heterogeneity feature in our study design. Clinical staging was based on Trese's 5-stage system (31) and the severity of patients was determined by the highest stage of FEVR in either eye. Subjects with histories of oxygen inhalation and preterm birth were also excluded. all enrolled participants were diagnosed by ophthalmologist with expertise in FEVR Based on clinical manifestations, genotyping, fundus examination and FFA. This study was approved by the Institutional Review Board of Xin Hua Hospital Affiliated to Shanghai Jiao Tong University School of Medicine. All work was performed in accordance with the approved study protocol. written informed content was obtained from all the participants or their parents.
Analysis of FZD4 Rs10128621 single nucleotide polymorphisms
Cis-regulatory variants might also modify mutation penetrance and contribute to clinical heterogeneity (32). FZD4 rs10128621 SNP is the only known SNP which could affect FZD4 gene expression (33). We performed Sanger sequencing to analyze FZD4 rs10128621 SNP in our cohort. The primer sequences were as follows: 5'-ACCTTGGCTTCCTAAAGTGTTG-3' (forward primer) and 5'-CTGCCTGAGAGACAGAGTAAGA-3' (reverse primer). The expected amplicon is 297 bp in length.
Bisulfite amplicon sequencing for methylation analysis
DNA methylation was analyzed by Bisulfite amplicon sequencing (BSAS). About 5 mL of peripheral blood samples were collected from each participant in a standard EDTA tube. Genomic DNA was extracted and purified using QIAamp® DNA Mini kit. One hundred thirty microliter of CT Conversion Reagent was added to 300 ng purified DNA samples as per the manufacturer's instructions. This process converted unmethylated cytosines (C) in CpG sites to uracil (U), whereas methylated C remained C. The primer sequences were as follows: 5′-TTTAGTTTGGGGTTGTTTTTGTAGT-3′ (forward primer) and 5′-CTTTTCCAAAAAAACTATCTCCTTC-3′ (reverse primer). PCR library was constructed using VAHTS Turbo DNA Library Prep Kit for Illumina and sequenced on a Miseq system (Illumina). Quality control was performed using FastQC v 0.11.2. Mapping and calling of the methylation levels were performed using bismark software and methylKit (R package 4.1.0), respectively.
Statistical analysis
Independent Samples T-test was used to pairwise compare the methylation differences among probands, carriers and non-carriers. Significant differences were determined as p < 0.05. Data analysis was performed using Graphpad 9.0.
Results
Characteristics of subjects
Thirty-four members of 10 FZD4-associated FEVR families were recruited in our study, including 11 FEVR probands, 12 asymptomatic/paucisymptomatic carriers of the same mutation, and 11 non-carriers of the mutant alleles. FEVR probands and family members were studied as a whole to best ensure similar genetic and environmental backgrounds. While FEVR probands had the most advanced ocular manifestations early in life, most of the family members carrying the same gene mutation were asymptomatic, only 4 carriers presented with Stage 1 FEVR. The mean age of probands, carriers and non-carriers were 35.1 ± 48.5 months (average ± standard deviation), 32.2 ± 12.2 years and 32.5 ± 14.8 years, respectively. Other than mutations in FZD4, no additional known FEVR-associated mutation was found in these subjects. Family No. 2, 5, 8 (chr11-86665923 c.205C>T) and family No. 7, 10 (chr11: 86665894-86665911 c.217_234del) shared the same mutation, respectively. The clinical details and genetic test results were summarized in Table 1. Fundus photographs and FFA of family No. 8 were presented in Figure 1.
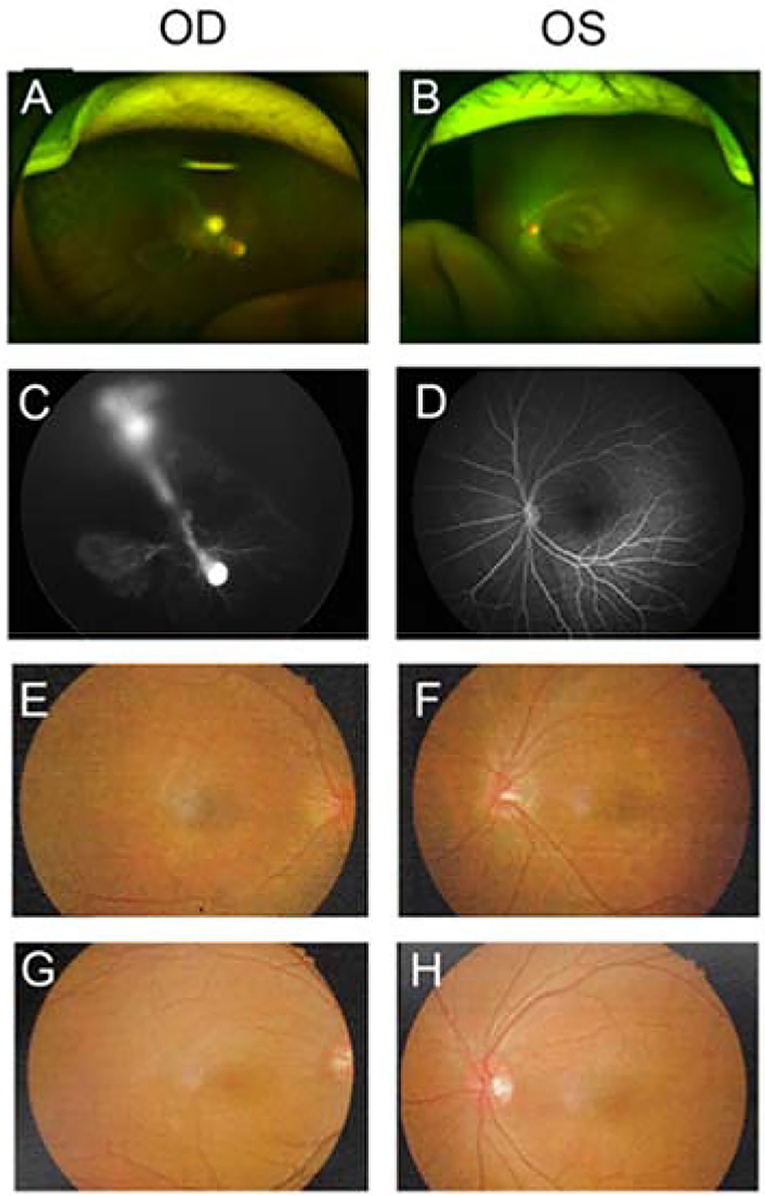
Figure 1. Fundus photographs and fundus fluorescein angiography (FFA) of subjects from family No. 8. In this family, the c.205C>T mutation was detected in a 4-year-old proband boy and it was inherited from his father. OD and OS represented right eye and left eye, respectively. (A–D) Fundus and FFA photographs of FEVR proband. The proband suffered from monocular FEVR disease in the right eye and was staged 4A. Severe fibrous proliferation membrane connected the optic disc to the lens in the right eye, with subtotal retinal detachment and peripheral avascular zone. The left eye exhibited normal retinal appearance without prolonged filling time or leakage. (E,F) Fundus photographs of father. Fundus examination didn't reveal any FEVR presentation although the father carried the c.205C>T mutation. (G,H) Fundus photographs of mother. The mother was a non-carrier without any fundus abnormality.
Map of CpG island in the FZD4 exon 1 region
We confirmed the methylation of CpG islands in FZD4 using a genomic map of FZD4 (NM_012193.4). FZD4 is located on the negative strand of chromosome 11. Most of the CpG island (CpG:69) exists in the exon 1 region. Twenty-one CpG sites were identified from a fragment in the exon 1 region at 86,665,722–86,666,073 (Figure 2).
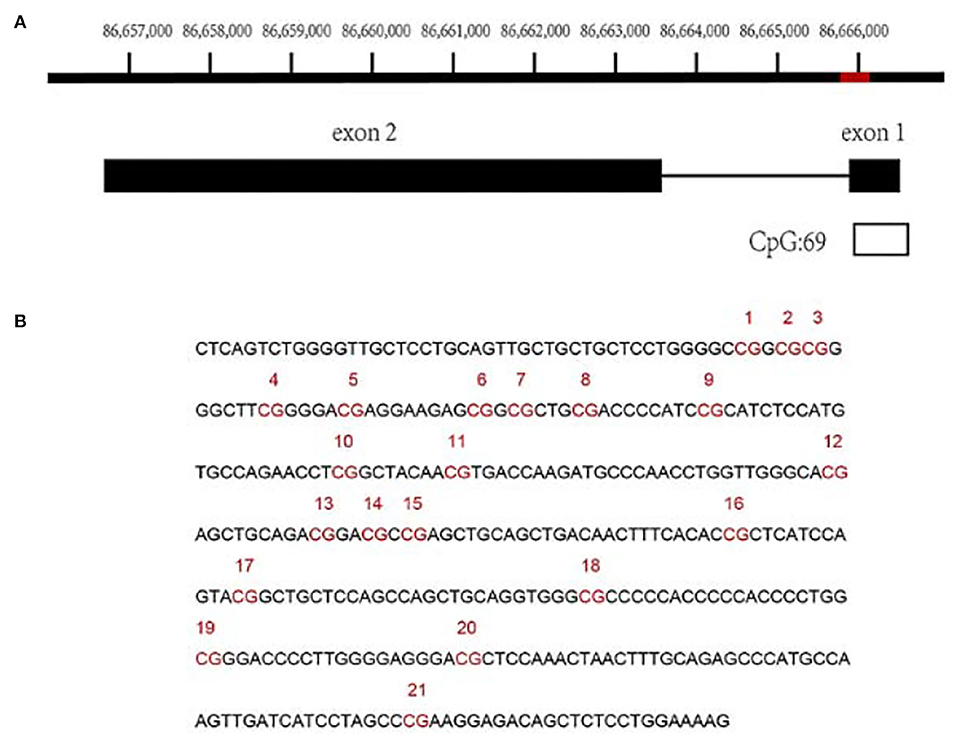
Figure 2. Analysis of methylation of CpG sites in the FZD4 exon 1 region. (A) The red region showed the location of tested sequence. The black box showed FZD4, black line indicated intergenic region and the white box indicated the CpG island region (CpG: 69) located in the exon 1 region of FZD4. (B) The sequence and CpG sites analyzed in this study were presented. The CpG sites were numbered and indicated as red.
Methylation analysis of 21 CpG sites
Before DNA methylation, we performed Sanger sequencing to analyze FZD4 rs10128621 SNP in our cohort. Sanger sequencing results showed a complete consistency of G base. Our results did not support the role of FZD4 rs10128621 SNP in the phenotypic heterogeneity of our FEVR patients. (Supplementary Figure S1)
DNA methylation levels of 21 CpG sites identified above in probands and their family members were presented in Figure 3, except for the non-carrier of family No. 8, whose blood sample was not obtained due to data missing. At almost all tested CpG sites (19 out of 21), FEVR probands held the lowest average methylation level compared to carriers and non-carriers, except for CpG4 and CpG5, where probands had slightly higher methylation levels than carriers. At more than half of the CpG sites (12 out of 21), carriers held lower methylation levels than non-carriers. DNA methylation levels of probands were significantly lower than symptomatic/paucisymptomatic carriers in CpG14 (p = 0.0009), CpG15 (p = 0.0489) and CpG16 (p = 0.0320). DNA methylation levels of probands were significantly lower than non-carriers at CpG1 (p = 0.0009), CpG9 (p = 0.0371) and CpG13 (p = 0.0021). Overall, the results suggested probands had lower DNA methylation compared with carriers and non-carriers in FZD4 exon 1 region.
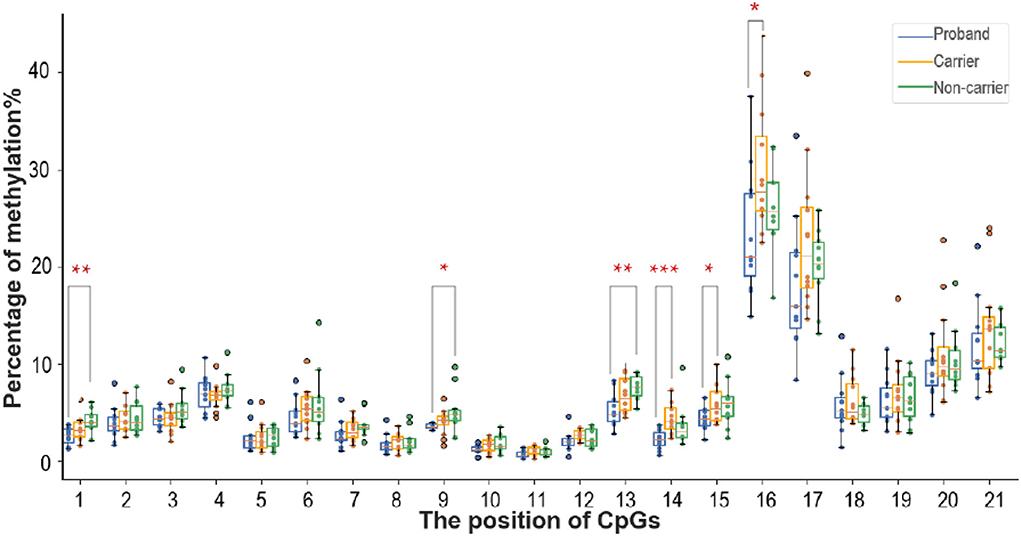
Figure 3. The graph showed methylation levels of 21 tested CpG sites. The horizontal axis represented the position of CpG sites and the vertical axis represented percentage of methylation. Blue, orange and green points respectively indicated to 11 FEVR probands, 12 carriers and 11 non-carriers. Box plot data represented range (error bars), median (horizontal line), quartile values (box) and outliers (dots). Median methylation levels of probands, carriers and non-carriers were compared in pair using student's t-test. DNA methylation levels of CpG1, CpG9 and CpG13 were significantly different between probands and non-carriers. DNA methylation levels of CpG14, CpG15 and CpG16 were significantly different between probands and carriers. *, p < 0.05; **, p < 0.01; ***, p < 0.001.
The methylation levels of these six significantly different CpG sites in each of the 10 FEVR families were shown in Figure 4. It showed that FEVR probands had lower methylation levels nearly in almost all cases. For example, at CpG14, probands in all 10 families had lower methylation levels. In addition, we noticed a negative correlation at CpG14 between methylation levels and disease severity within three families (No. 2, 5 and 8) that happened to carry the same mutation (chr11-86665923 c.205C>T). Proband from family No. 2 had the most advanced FEVR and the lowest methylation level at CpG14 compared to the rest. Similar negative correlations also existed for CpG15 and CpG16.
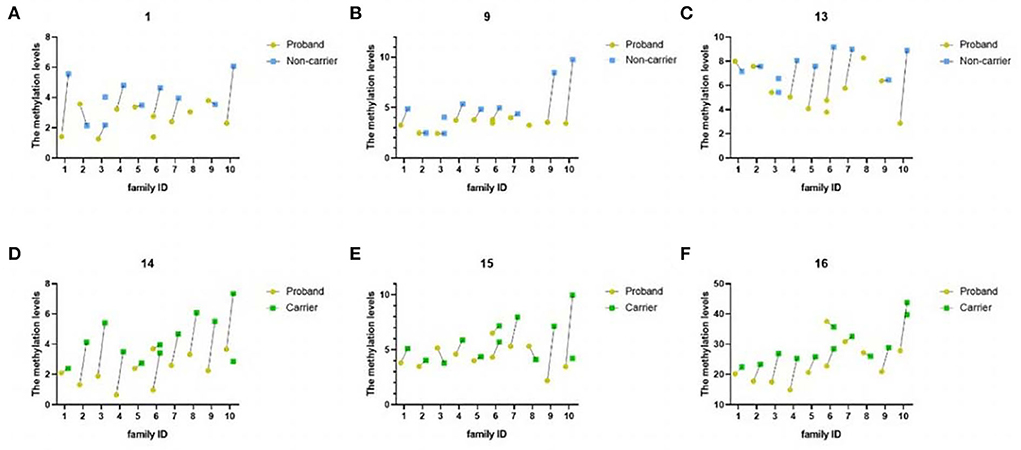
Figure 4. The figure above showed methylation levels at six significantly different CpG sites of 10 FEVR families. The horizontal axis represented family ID and the vertical axis represented the methylation level of specific CpG site. (A–C) DNA methylation levels of CpG1, CpG9 and CpG13 were significantly different between probands and non-carriers. Yellow and blue points indicated to probands and non-carriers, respectively. (D–F) DNA methylation levels of CpG14, CpG15 and CpG16 were significantly different between probands and carriers. Yellow and green points indicated to probands and carriers, respectively.
Discussion
FEVR is a rare disease featured by peripheral retinal vascular abnormalities (19, 34). It is clinically characterized by phenotypic heterogeneity, suggesting that non-genetic factors are involved in disease etiology (2, 22, 23). Comparing the epigenetic features among FEVR family members with or without mutation would provide important information regarding epigenetic factors which could contribute to phenotypic heterogeneity. DNA methylation is a widely studied epigenetic modification type. Increasing number of evidence supported the role of DNA methylation in modulating the phenotypic expression of diseases (35–37). For example, Coppede et al. found that the increase of global DNA methylation might contribute to disease manifestation in amyotrophic lateral sclerosis (ALS) carriers of not fully penetrant SOD1 mutations (38). DNA methylation at promoter CpG islands (CGI) of Wnt pathway genes showed a significant association with progression-free survival in ovarian tumor patients (39). Although little is known about FEVR disease, altered DNA methylation has been demonstrated in many other vitreoretinopathy diseases such as age-related macular degeneration (AMD), proliferative vitreoretinopathy (PVR), and diabetic retinopathy (DR). Therefore, we hypothesized that DNA methylation changes might play a role in FEVR phenotypic heterogeneity.
Up to date, at least 9 genes have been reported to cause FEVR. In previous studies, we and others showed that with FZD4 mutations accounting for the greatest proportion of FEVR in Chinese population (23, 40, 41). The FZD4 gene encodes a 537–amino acid protein, which functions as a receptor of the Wnt signaling pathway (42). According to our previous work, more than half (51.43%) in the FZD4 group were identified asymmetry, suggesting FZD4 mutations might initiate the most diverse and asymmetric phenotypes (22). Previous study suggested epigenetic modifications might involve in the developing fetal retina which caused high asymmetric FZD4 gene expression (43). Therefore, it is important to study non-genetic factors underlying FZD4-associated FEVR phenotypic heterogeneity.
Bisulfite sequencing was first reported by Frommer et al. in (44). The bisulfite treated-DNA is amplified by PCR, and then the cloning and sequencing results of PCR products are used to determine whether the CpG site was methylated. This method has been recognized as one of the most powerful approaches to investigate DNA methylation patterns due to its reliable results, high accuracy and high sensitivity. It allows the determination of methylation at a single-cytosine resolution and has become the “gold standard” for DNA methylation detection (45, 46).
The current study aimed to access evidence of a link between FEVR phenotypic heterogeneity and FZD4 methylation status. We focused on 10 unrelated clinically heterogeneous FEVR families carrying only FZD4 gene mutation. In this study, our goal was to investigate whether there was a difference in methylation levels between probands at severe FEVR stage and parents with mild phenotype. Stage 1 FEVR has only peripheral avascular zone seen by fluorescein fundus angiography. Patients with stage 1 FEVR may be stable for decades without any progression. Therefore, we grouped asymptomatic and stage 1 carriers together. Our data indicated a negative correlation between DNA methylation in FZD4 exon 1 region and FEVR severity, which requires further validation. Among the six significantly different CpG sites, four sites (CpG13, CpG14, CpG15 and CpG16) were adjacent, suggesting that this region might play an important and unique role in the regulation of FZD4 expression. Although the CpG9 of Family No. 9 and No.10 are obviously different, the probands still had the lowest average methylation level of the three groups and non-carriers had the highest average methylation level when these two families were eliminated. Our study suggests that changes in DNA methylation might contribute to the severity in FZD4-associated FEVR patients. The function of intragenic DNA methylation hasn't been well recognized. Brenet et al. found that DNA methylation at the first exon is tightly linked to transcriptional silencing (47), while others believed that the methylation in the body of genes can actually activate gene transcription (48, 49). Although it remains controversial, given that altered methylation of a specific CpG site might be enough to change gene expression [50], FEVR probands with reduced DNA methylation are highly likely to undergo altered gene expression, thus causing different degrees of disease severity.
This study is limited by the number of FEVR families enrolled. For 5 mutations analyzed here, only one family was found for each mutation. Alternatively, we took the approach of comparing the average overall methylation level in probands, carriers and non-carriers, which could have masked the effect of specific mutation on DNA methylation. In addition, age may be an independent factor in the methylation level. However, in this study, after excluding the “grandmother” and “grandfather” subjects in family 6, the probands still had the lowest average methylation level among the three groups, which is in line with our conclusion. What's more, we do not have RNA samples from our patients to validate the expression of FZD4. Future study with larger sample size is needed to validate our findings. Nevertheless, our results provided a new mechanism potentially responsible for phenotypic heterogeneity amongst FEVR patients.
Data availability statement
The raw data supporting the conclusions of this article will be made available by the authors, without undue reservation.
Ethics statement
The studies involving human participants were reviewed and approved by the Institutional Review Board of Xin Hua Hospital Affiliated to Shanghai Jiao Tong University School of Medicine. Written informed consent to participate in this study was provided by the participants' legal guardian/next of kin.
Author contributions
ML and JLu are responsible for conceptualization, methodology, software, investigation, and writing original draft. HF is responsible for clinical data collection and writing. JLi and XZ are responsible for software and writing review. PF and PZ are responsible for supervision, writing review and funding acquisition. All authors contributed to the article and approved the submitted version.
Funding
This research was supported by Grant 81770963 from National Natural Science Foundation of China (to FP) and Grant 2018YFA0800801 from National Key Research and Development Program of China (to LJ).
Acknowledgments
The authors thank all the patients and their family members for their participation.
Conflict of interest
The authors declare that the research was conducted in the absence of any commercial or financial relationships that could be construed as a potential conflict of interest.
Publisher's note
All claims expressed in this article are solely those of the authors and do not necessarily represent those of their affiliated organizations, or those of the publisher, the editors and the reviewers. Any product that may be evaluated in this article, or claim that may be made by its manufacturer, is not guaranteed or endorsed by the publisher.
Supplementary material
The Supplementary Material for this article can be found online at: https://www.frontiersin.org/articles/10.3389/fmed.2022.976520/full#supplementary-material
References
1. Criswick VG, Schepens CL. Familial exudative vitreoretinopathy. Am J Ophthalmol. (1969) 68:578–94. doi: 10.1016/0002-9394(69)91237-9
2. Kashani AH, Learned D, Nudleman E, Drenser KA, Capone A, Trese MT. High prevalence of peripheral retinal vascular anomalies in family members of patients with familial exudative vitreoretinopathy. Ophthalmology. (2014) 121:262–8. doi: 10.1016/j.ophtha.2013.08.010
3. Chen ZY, Battinelli EM, Fielder A, Bundey S, Sims K, Breakefield XO, et al. A mutation in the Norrie disease gene (NDP) associated with X-linked familial exudative vitreoretinopathy. Nat Genet. (1993) 5:180–3. doi: 10.1038/ng1093-180
4. Fei P, Zhang Q, Huang L, Xu Y, Zhu X, Tai Z, et al. Identification of two novel LRP5 mutations in families with familial exudative vitreoretinopathy. Mol Vis. (2014) 20:395–409.
5. Nikopoulos K, Gilissen C, Hoischen A, van Nouhuys CE, Boonstra FN, Blokland EA, et al. Next-generation sequencing of a 40 Mb linkage interval reveals TSPAN12 mutations in patients with familial exudative vitreoretinopathy. Am J Hum Genet. (2010) 86:240–7. doi: 10.1016/j.ajhg.2009.12.016
6. Fei P, Zhu X, Jiang Z, Ma S, Li J, Zhang Q, et al. Identification and functional analysis of novel FZD4 mutations in Han Chinese with familial exudative vitreoretinopathy. Sci Rep. (2015) 5:16120. doi: 10.1038/srep16120
7. Robitaille J, MacDonald ML, Kaykas A, Sheldahl LC, Zeisler J, Dube MP, et al. Mutant frizzled-4 disrupts retinal angiogenesis in familial exudative vitreoretinopathy. Nat Genet. (2002) 32:326–30. doi: 10.1038/ng957
8. Robitaille JM, Gillett RM, LeBlanc MA, Gaston D, Nightingale M, Mackley MP, et al. Phenotypic overlap between familial exudative vitreoretinopathy and microcephaly, lymphedema, and chorioretinal dysplasia caused by KIF11 mutations. JAMA Ophthalmol. (2014) 132:1393–9. doi: 10.1001/jamaophthalmol.2014.2814
9. Li JK, Fei P, Li Y, Huang QJ, Zhang Q, Zhang X, et al. Identification of novel KIF11 mutations in patients with familial exudative vitreoretinopathy and a phenotypic analysis. Sci Rep. (2016) 6:26564. doi: 10.1038/srep26564
10. Collin RW, Nikopoulos K, Dona M, Gilissen C, Hoischen A, Boonstra FN, et al. ZNF408 is mutated in familial exudative vitreoretinopathy and is crucial for the development of zebrafish retinal vasculature. Proc Natl Acad Sci U S A. (2013) 110:9856–61. doi: 10.1073/pnas.1220864110
11. Dixon MW, Stem MS, Schuette JL, Keegan CE, Besirli CG. CTNNB1 mutation associated with familial exudative vitreoretinopathy (FEVR) phenotype. Ophthalmic Genet. (2016) 37:468–470. doi: 10.3109/13816810.2015.1120318
12. Panagiotou ES, Sanjurjo Soriano C, Poulter JA, Lord EC, Dzulova D, Kondo H, et al. Defects in the cell signaling mediator β-catenin cause the retinal vascular condition FEVR. Am J Hum Genet. (2017) 100:960–8. doi: 10.1016/j.ajhg.2017.05.001
13. Zhang L, Zhang X, Xu H, Huang L, Zhang S, Liu W, et al. Exome sequencing revealed Notch ligand JAG1 as a novel candidate gene for familial exudative vitreoretinopathy. Genet Med. (2020) 22:77–84. doi: 10.1038/s41436-019-0571-5
14. Zhu X, Yang M, Zhao P, Li S, Zhang L, Huang L, et al. Catenin α 1 mutations cause familial exudative vitreoretinopathy by overactivating Norrin/β-catenin signaling. J Clin Invest. (2021) 131:9869. doi: 10.1172/JCI139869
15. Warden SM, Andreoli CM, Mukai S. The Wnt signaling pathway in familial exudative vitreoretinopathy and Norrie disease. Semin Ophthalmol. (2007) 22:211–7. doi: 10.1080/08820530701745124
16. Wang Z, Liu CH, Huang S, Chen J. Wnt Signaling in vascular eye diseases. Prog Retin Eye Res. (2019) 70:110–33. doi: 10.1016/j.preteyeres.2018.11.008
17. Dan H, Wang D, Huang Z, Shi Q, Zheng M, Xiao Y, et al. Whole exome sequencing revealed 14 variants in NDP, FZD4, LRP5, and TSPAN12 genes for 20 families with familial exudative vitreoretinopathy. BMC Med Genomics. (2022) 15:54. doi: 10.1186/s12920-022-01204-0
18. Xiao H, Tong Y, Zhu Y, Peng M. Familial exudative vitreoretinopathy-related disease-causing genes and norrin/β-catenin signal pathway: structure, function, and mutation spectrums. J Ophthalmol. (2019) 2019:5782536. doi: 10.1155/2019/5782536
19. Tauqeer Z, Yonekawa Y. Familial exudative vitreoretinopathy: pathophysiology, diagnosis, and management. Asia Pac J Ophthalmol (Phila). (2018) 7:176–82. doi: 10.22608/201855
20. He Y, Yang M, Zhao R, Peng L, Dai E, Huang L, et al. Novel truncating variants in CTNNB1 cause familial exudative vitreoretinopathy. J Med Genet. (2022). doi: 10.1136/jmedgenet-2021-108259
21. Wang S, Zhang X, Hu Y, Fei P, Xu Y, Peng J, et al. Clinical and genetical features of probands and affected family members with familial exudative vitreoretinopathy in a large Chinese cohort. Br J Ophthalmol. (2021) 105:83–6. doi: 10.1136/bjophthalmol-2019-315598
22. Lu J, Huang L, Sun L, Li S, Zhang Z, Jiang Z, et al. FZD4 in a large Chinese population with familial exudative vitreoretinopathy: molecular characteristics and clinical manifestations. Invest Ophthalmol Vis Sci. (2022) 63:7. doi: 10.1167/iovs.63.4.7
23. Toomes C, Bottomley HM, Scott S, Mackey DA, Craig JE, Appukuttan B, et al. Spectrum and frequency of FZD4 mutations in familial exudative vitreoretinopathy. Invest Ophthalmol Vis Sci. (2004) 45:2083–90. doi: 10.1167/iovs.03-1044
24. Lorincz MC, Dickerson DR, Schmitt M, Groudine M. Intragenic DNA methylation alters chromatin structure and elongation efficiency in mammalian cells. Nat Struct Mol Biol. (2004) 11:1068–75. doi: 10.1038/nsmb840
25. Schübeler D, Lorincz MC, Cimbora DM, Telling A, Feng YQ, Bouhassira EE, et al. Genomic targeting of methylated DNA: influence of methylation on transcription, replication, chromatin structure, and histone acetylation. Mol Cell Biol. (2000) 20:9103–12. doi: 10.1128/MCB.20.24.9103-9112.2000
26. Hunter A, Spechler PA, Cwanger A, Song Y, Zhang Z, Ying GS, et al. DNA methylation is associated with altered gene expression in AMD. Invest Ophthalmol Vis Sci. (2012) 53:2089–105. doi: 10.1167/iovs.11-8449
27. Corso-Díaz X, Jaeger C, Chaitankar V, Swaroop A. Epigenetic control of gene regulation during development and disease: a view from the retina. Prog Retin Eye Res. (2018) 65:1–27. doi: 10.1016/j.preteyeres.2018.03.002
28. Shafabakhsh R, Aghadavod E, Ghayour-Mobarhan M, Ferns G, Asemi Z. Role of histone modification and DNA methylation in signaling pathways involved in diabetic retinopathy. J Cell Physiol. (2019) 234:7839–46. doi: 10.1002/jcp.27844
29. Wei L, Liu B, Tuo J, Shen D, Chen P, Li Z, et al. Hypomethylation of the IL17RC promoter associates with age-related macular degeneration. Cell Rep. (2012) 2:1151–8. doi: 10.1016/j.celrep.2012.10.013
30. Pendergast SD, Trese MT. Familial exudative vitreoretinopathy Results of surgical management. Ophthalmology. (1998) 105:1015–23. doi: 10.1016/S0161-6420(98)96002-X
31. Wang F, Zhang S, Wen Y, Wei Y, Yan H, Liu H, et al. Revealing the architecture of genetic and epigenetic regulation: a maximum likelihood model. Brief Bioinform. (2014) 15:1028–43. doi: 10.1093/bib/bbt076
32. Castel SE, Cervera A, Mohammadi P, Aguet F, Reverter F, Wolman A, et al. Modified penetrance of coding variants by cis-regulatory variation contributes to disease risk. Nat Genet. (2018) 50:1327–34. doi: 10.1038/s41588-018-0192-y
33. Gilmour DF. Familial exudative vitreoretinopathy and related retinopathies. Eye (Lond). (2015) 29:1–14. doi: 10.1038/eye.2014.70
34. Quiñonez-Silva G, Dávalos-Salas M, Recillas-Targa F, Ostrosky-Wegman P, Aranda DA, Benítez-Bribiesca L. Monoallelic germline methylation and sequence variant in the promoter of the RB1 gene: a possible constitutive epimutation in hereditary retinoblastoma. Clin Epigenetics. (2016) 8:1. doi: 10.1186/s13148-015-0167-0
35. Eloy P, Dehainault C, Sefta M, Aerts I, Doz F, Cassoux N, et al. A parent-of-origin effect impacts the phenotype in low penetrance retinoblastoma families segregating the c.1981C>T/p.Arg661Trp Mutation of RB1. PLoS Genet. (2016) 12:e1005888. doi: 10.1371/journal.pgen.1005888
36. Pizzolo F, Friso S, Morandini F, Antoniazzi F, Zaltron C, Udali S, et al. Apparent mineralocorticoid excess by a novel mutation and epigenetic modulation by HSD11B2 promoter methylation. J Clin Endocrinol Metab. (2015) 100:E1234–41. doi: 10.1210/jc.2015-1760
37. Coppedè F, Stoccoro A, Mosca L, Gallo R, Tarlarini C, Lunetta C, et al. Increase in DNA methylation in patients with amyotrophic lateral sclerosis carriers of not fully penetrant SOD1 mutations. Amyotroph Lateral Scler Frontotemporal Degener. (2018) 19:93–101. doi: 10.1080/21678421.2017.1367401
38. Dai W, Teodoridis JM, Zeller C, Graham J, Hersey J, Flanagan JM, et al. Systematic CpG islands methylation profiling of genes in the wnt pathway in epithelial ovarian cancer identifies biomarkers of progression-free survival. Clin Cancer Res. (2011) 17:4052–62. doi: 10.1158/1078-0432.CCR-10-3021
39. Li Y, Peng J, Li J, Zhang Q, Li J, Zhang X, et al. The characteristics of digenic familial exudative vitreoretinopathy. Graefes Arch Clin Exp Ophthalmol. (2018) 256:2149–56. doi: 10.1007/s00417-018-4076-8
40. Seo SH, Yu YS, Park SW, Kim JH, Kim HK, Cho SI, et al. Molecular Characterization of FZD4, LRP5, and TSPAN12 in Familial Exudative Vitreoretinopathy. Invest Ophthalmol Vis Sci. (2015) 56:5143–51. doi: 10.1167/iovs.14-15680
41. Zhang K, Harada Y, Wei X, Shukla D, Rajendran A, Tawansy K, et al. An essential role of the cysteine-rich domain of FZD4 in Norrin/Wnt signaling and familial exudative vitreoretinopathy. J Biol Chem. (2011) 286:10210–5. doi: 10.1074/jbc.M110.194399
42. Aldiri I, Xu B, Wang L, Chen X, Hiler D, Griffiths L, et al. The dynamic epigenetic landscape of the retina during development, reprogramming, and tumorigenesis. Neuron. (2017) 94:550–68.e10. doi: 10.1016/j.neuron.2017.04.022
43. Frommer M, McDonald LE, Millar DS, Collis CM, Watt F, Grigg GW, et al. A genomic sequencing protocol that yields a positive display of 5-methylcytosine residues in individual DNA strands. Proc Natl Acad Sci U S A. (1992) 89:1827–31. doi: 10.1073/pnas.89.5.1827
44. Sibbritt T, Schumann U, Shafik A, Guarnacci M, Clark SJ, Preiss T. Target-specific profiling of RNA m(5)C methylation level using amplicon sequencing methods. Mol Biol. (2022) 2404:375–92. doi: 10.1007/978-1-0716-1851-6_21
45. Masser DR, Stanford DR, Freeman WM. Targeted DNA methylation analysis by next-generation sequencing. J Vis Exp. (2015) 96:52488. doi: 10.3791/52488
46. Brenet F, Moh M, Funk P, Feierstein E, Viale AJ, Socci ND, et al. DNA methylation of the first exon is tightly linked to transcriptional silencing. PLoS ONE. (2011) 6:e14524. doi: 10.1371/journal.pone.0014524
47. Baylin SB, Jones PA. A decade of exploring the cancer epigenome - biological and translational implications. Nat Rev Cancer. (2011) 11:726–34. doi: 10.1038/nrc3130
48. Rivera RM, Bennett LB. Epigenetics in humans: an overview. Curr Opin Endocrinol Diabetes Obes. (2010) 17:493–9. doi: 10.1097/MED.0b013e3283404f4b
Keywords: familial exudative vitreoretinopathy (FEVR), the receptor frizzled-4 (FZD4), phenotypic heterogeneity, DNA methylation, epigenetics
Citation: Liu M, Luo J, Feng H, Li J, Zhang X, Zhao P and Fei P (2022) Decrease of FZD4 exon 1 methylation in probands from FZD4-associated FEVR family of phenotypic heterogeneity. Front. Med. 9:976520. doi: 10.3389/fmed.2022.976520
Received: 23 June 2022; Accepted: 27 September 2022;
Published: 24 October 2022.
Edited by:
Xiaoyan Ding, Sun Yat-sen University, ChinaReviewed by:
Santasree Banerjee, Beijing Genomics Institute (BGI), ChinaZongyi Zhan, Shenzhen Eye Hospital, China
Copyright © 2022 Liu, Luo, Feng, Li, Zhang, Zhao and Fei. This is an open-access article distributed under the terms of the Creative Commons Attribution License (CC BY). The use, distribution or reproduction in other forums is permitted, provided the original author(s) and the copyright owner(s) are credited and that the original publication in this journal is cited, in accordance with accepted academic practice. No use, distribution or reproduction is permitted which does not comply with these terms.
*Correspondence: Ping Fei, ZmVpcGluZ0B4aW5odWFtZWQuY29tLmNu; Peiquan Zhao, emhhb3BlaXF1YW5AeGluaHVhbWVkLmNvbS5jbg==
†These authors have contributed equally to this work and share first authorship