- Department of Pediatrics, Shengjing Hospital of China Medical University, Shenyang, China
Necrotizing enterocolitis (NEC) is a life-threatening disease of the digestive system that occurs in the neonatal period. NEC is difficult to diagnose early and the prognosis is poor. Previous studies have reported that abnormalities can be detected before the presentation of clinical symptoms. Based on an analysis of literature related to the early prediction of NEC, we provide a detailed review on the early prediction and diagnosis methods of NEC, including ultrasound, near-infrared spectroscopy, biomarkers, and intestinal microbiota. This review aimed to provide a reference for further research and clinical practice.
Introduction
Necrotizing enterocolitis (NEC) is a life-threatening digestive system disease that occurs in the neonatal period. The incidence of NEC in neonates ranged from 2% to 7%, and the mortality rate was up to 21.9–38% (1). It is one of the leading causes of death in neonates, especially preterm infants. NEC is also associated with long-term complications, such as intestinal adhesion stenosis, short bowel syndrome, and developmental sensory and motor deficits (2, 3).
Abdominal X-ray is the most common diagnostic tool for NEC, but the disease is already in the progression stage by the time when any manifestation can be detected in the X-ray. This, however, seems late. Since the onset of NEC is occult and early clinical manifestations are not specific, early detection of NEC is challenging for clinicians. Since immature intestinal barrier function, hypoxia-ischemia, and flora imbalance are involved in the occurrence and development of NEC (4, 5), it might be feasible to detect NEC from those aspects, by utilizing ultrasound, near-infrared spectroscopy (NIRS), biomarker monitoring, and intestinal microbiota diversity. This article reviews the tools and markers that are potential for predicting or diagnosing NEC at an earlier stage (Figure 1).
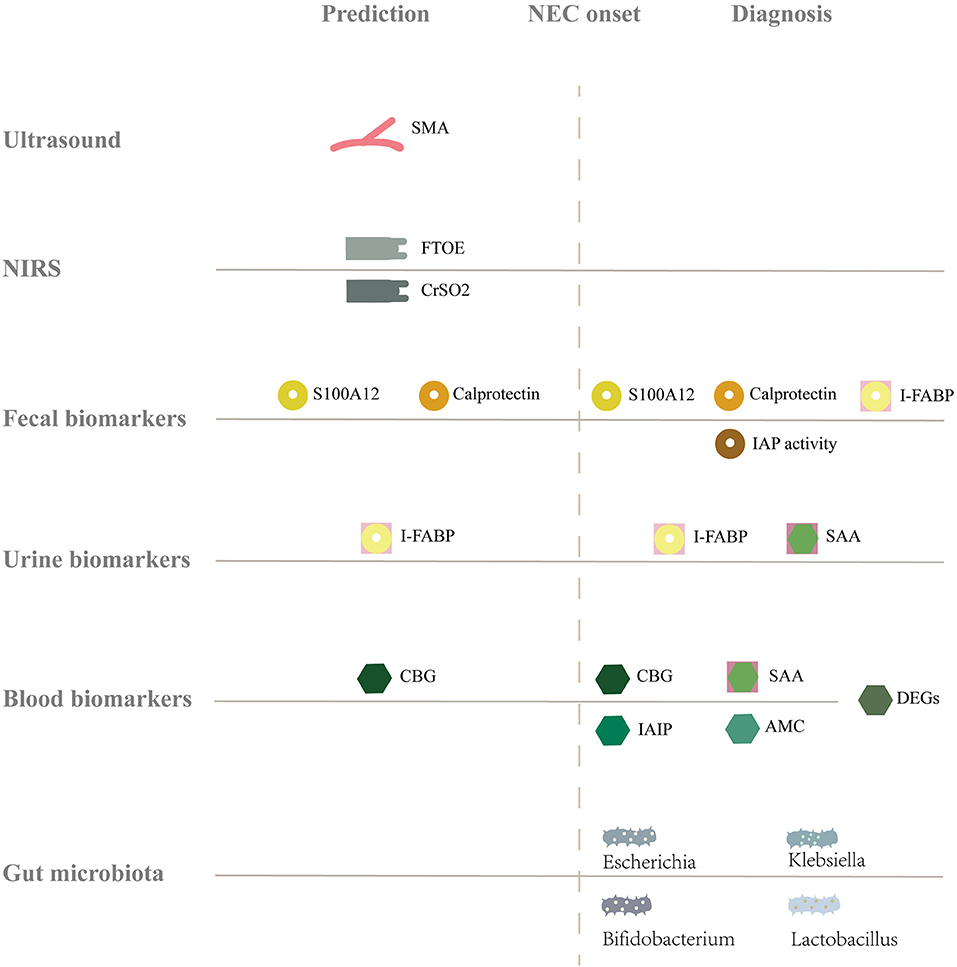
Figure 1. Summary of the full text. The gray solid line represents the baseline level of healthy infants, and above, below, and after the solid line represents the increase, decrease and change of markers, respectively. NEC, Necrotizing enterocolitis; SMA, Superior mesenteric artery; NIRS, Near-infrared spectroscopy; FTOE, Fractional tissue oxygen extraction; CrSO2, Cerebral tissue oxygen saturation; S100A12, S 100 calcium binding protein a 12; I-FABP, Intestinal fatty acid binding proteins; IAP activity, Intestinal alkaline phosphatase activity; SAA, Serum amyloid A; CBG, Cytosolic β- glucosidase; DEGs, Differentially expressed genes; IAIP, Inter-alpha inhibitor proteins; AMC, Absolute monocyte counts.
Ultrasound
In recent years, ultrasound has been widely used in the diagnosis and monitoring of NEC (6). It can detect NEC-related symptoms at the early stage (7), with advantages such as portability, non-invasiveness, and real-time result. NEC patients show portal venous gas, pneumatosis intestinalis, intestinal hypoperfusion, focal fluid collection, and free intraperitoneal gas during ultrasound examination (8–10).
Studies have shown that abdominal ultrasound may predict the occurrence of NEC by monitoring the blood flow of the superior mesenteric artery (SMA). In a prospective study, Guang et al. placed an ultrasonic probe under the xiphoid to monitor the SMA in 104 newborns within 12 h after birth. The results showed that the differential velocity [peak systolic velocity (PSV) minus end-diastolic velocity (EDV)] of the SMA had a high sensitivity (0.875) and specificity (0.604) for predicting NEC with a cut-off value of 34.835 cm/s (11). This study suggests that the increase in differential velocity may be a predictor of NEC. Murdoch et al. found that the risk of NEC was positively correlated with the SMA resistance index (PSV—EDV)/(PSV) on the first day after birth, while the risk of NEC decreased in neonates with an increased SMA end-diastolic blood flow velocity (12). SMA blood flow in a rabbit model of NEC displayed the same changes (13). In a case-control study, 62 newborns were examined by Doppler ultrasound; the results showed that an SMA resistance index >0.75 and a pulsatility index [(PSV—EDV)/mean velocity] >1.85 could distinguish the NEC and control groups (14). Abnormal blood flow in the SMA may induce intestinal ischemic injury, which can then lead to the occurrence of NEC.
Contrast-enhanced ultrasound (CEUS) can be used to assess intestinal perfusion and motor degradation. CEUS enhances blood flow and tissue echo through contrast microbubbles. When CEUS is performed to evaluate intestinal wall and SMA perfusion, contrast agents are usually injected intravenously, but there is no dose guideline at present (15). Studies have shown that CEUS may be applied to many gastrointestinal diseases (16). Since high-frequency oscillator impedes the detection of intestinal perfusion by Doppler ultrasound, a case report used CEUS to evaluate intestinal perfusion in children undergoing high-frequency oscillator ventilation, which confirmed the absence of intestinal perfusion. Subsequently, laparotomy exploration revealed total intestinal necrosis, which indicated that CEUS could be used when Doppler ultrasound failed to evaluate blood perfusion (17).
NIRS
NIRS is a non-invasive tool to monitor tissue blood oxygen (18). Animal studies have found that NIRS can detect instant and persistent gastrointestinal hypoperfusion (19) and that monitoring tissue oxygen saturation (rSO2) in infants by NIRS is feasible (20). Therefore, NIRS may be able to predict NEC through the continuous monitoring of intestinal and cerebral oxygenation.
Studies have demonstrated impaired visceral oxygenation before the onset of symptoms of NEC (21, 22), by placing the sensors below the umbilicus or over the right lower abdomen. The average splanchnic oxygen saturation of patients with NEC during the first postnatal week was significantly lower than that of non-NEC controls (23). Abdominal oxygen saturation ≤ 56% has been suggested to be predictive of NEC, with a sensitivity of 86% and specificity of 64% (24).
Fractional tissue oxygen extraction (FTOE) reflects the relationship between tissue oxygen supply and oxygen consumption. FTOE is calculated based on the data measured by NIRS: FTOE = (oxygen saturation—rSO2)/ oxygen saturation. A study found that the intestinal FTOE of children with NEC would increase 2 days before the onset of symptoms, suggesting that FTOE may also be predictive of NEC (22). Increased FTOE may be a compensatory response to hypoxia to maintain aerobic metabolism (22, 25). Interestingly, Schat et al. predicted abdominal diseases by monitoring brain oxygenation and found that the prevalence of NEC in infants with cerebral rSO2 <70% within 48 h after birth was significantly higher than that in infants with cerebral rSO2 ≥70% [odds ratio (OR): 9.00] (22). Cerebral rSO2 is a marker of systemic perfusion, and hypoxia-ischemia is closely related to the occurrence of NEC (22, 26).
Currently, NIRS is mainly used to monitor brain oxygen saturation in children, so NIRS algorithms are mostly based on the brain and are not fully applicable to the abdomen (27). Since there are differences in oxygenation in different parts of the viscera, it is difficult to obtain accurate data (27, 28). Abdominal oxygen saturation has more variation than that of the brain and kidney (29), which also limits the clinical application of NIRS.
Biomarkers
Many studies have proposed diagnostic and predictive biomarkers for NEC (Tables 1–3). Biomarkers are typically obtained from serum, fecal, or urine samples. Therefore, they are not affected by the preference or skills of the performers. Besides, the tests will be very easy and non-invasive when fecal or urine samples are used. However, most of them are not as specific as ultrasound presentations. Different methods or essays may result in diverse reference values that are impossible to integrate into a universal reference. Therefore, more studies are needed to investigate the markers for the prediction and early diagnosis of NEC.
Calprotectin
Calprotectin belongs to the S100 family and is mainly produced by neutrophils (61). It is involved in the innate immune response by activating Toll-like receptors (62), which participate in the pathogenesis of NEC. Therefore, some scholars believe that calprotectin is a biomarker for the early prediction of NEC (30, 31).
Elevated fecal calprotectin in the early life of newborns may be related to NEC (32). For preterm infants with a gestational age ≤ 26 + 1 weeks, the sensitivity and specificity of fecal calprotectin at 24 h before the onset of clinical symptoms were 0.89 and 0.87, respectively (30). The fecal calprotectin concentration of patients with NEC was significantly increased by 2.1 times (63). A meta-analysis of ten studies showed that the sensitivity, specificity, diagnostic odds ratio (DOR), and area under the curve (AUC) for the early diagnosis by fecal calprotectin were 0.86, 0.79, 34.78, and 0.92, respectively (33). In addition, Thibault et al. reported that the combined regimen of calprotectin and lipocalin-2 was more favorable for the prediction of NEC within the first 10 days before the onset of NEC than either marker independently (31).
There is some controversy on this. Some studies suggest that fecal calprotectin has a large variation between individuals (64). A prospective case-control study reported that there was no statistical difference in calprotectin concentration between the NEC group and the control group at the 6–8 days (P = 0.80), the 3–5 days (P = 0.80), and within 48 h (P = 0.80) before NEC was suspected (64). Additionally, Goold et al. analyzed two cut-off values of calprotectin for diagnosing NEC (226 and 247 μg/g), both with low diagnostic efficiency (34). According to the research above, fecal calprotectin remains to be further explored to guide the early prediction and diagnosis of NEC.
S100A12
Human S100A12 up-regulates the expression of inflammatory genes by interacting with Toll-like receptor 4 (65). It is also involved in ischemia-reperfusion injury through the activation of ERK signaling (66), which is considered to be involved in the pathogenesis of transfusion-related NEC. A study found that the level of S100A12 in the feces of infants with suspected NEC was higher than that of infants without gastrointestinal diseases; the sensitivity, specificity, and positive and negative predictive values of fecal S100A12 for NEC detection were 70%, 68%, 37%, and 89%, respectively (35).
Intestinal alkaline phosphatase
IAP is expressed in the gastrointestinal tract and plays a role in maintaining the homeostasis of the intestinal environment (67). Animal experiments have shown that the IAP activity of NEC pups was 0.18 U/mg, which was significantly lower than that of healthy controls (0.57 U/mg). Furthermore, IAP activity increases after the removal of NEC stressors (36). Heath et al. observed similar results in human NEC patients and believed that IAP could not only diagnose NEC but also predict the severity of the disease (37). In this study, the fecal protein IAP activity of children with severe NEC (characterized by radiologic evidence of pneumatosis intestinalis and/or portal venous gas) and suspected NEC (abnormal clinical and laboratory findings without evidence of pneumatosis intestinalis or portal venous gas) was 183(56-507) μmol/min/g and 355 (172–608) μmol/min/g, respectively, and the accuracy of assessing severe NEC by IAP activity was 0.76 (95% CI: 0.64–0.86; P < 0.001) (37).
Intestinal fatty acid binding proteins
Fatty acid binding proteins (FABPs) have the pleiotropic function to maintain healthy tissue homeostasis and participate in disease pathogenesis (68). I-FABP is a 15-kDa cytoplasmic protein expressed mainly by intestinal cells located at the top of the intestinal villi. This protein is released when the intestinal tissue is ischemic or damaged. The I-FABP response to intestinal hypoperfusion is related to the pathogenesis of NEC (69). Studies suggest that I-FABP levels in the urine and blood can be used in the prediction and early diagnosis of NEC (38–41).
Increased urinary I-FABP in infants is associated with subsequent NEC (38). A cohort study showed that I-FABP >13.3 ng/mL could predict NEC with 60% sensitivity and 78% specificity seven days before the onset of symptoms, and I-FABP >13.9 ng/mL was found to have a 65% sensitivity and 84% specificity within 3 days before NEC onset (41). Urinary I-FABP/creatinine >10.2 pg/nmol had a sensitivity of 100% and specificity of 95.6% 1 day before NEC onset (42).
The urinary and plasma level of I-FABP in patients with NEC was reported to be significantly higher than that in other infants (40, 43). Schurink et al. determined that the cut-off values for NEC diagnosis within 8 h after onset were 9 ng/mL (plasma I-FABP) and 218 ng/mL (urinary I-FABP), and the corresponding likelihood ratios were 5.6 and 5.1, respectively (40). FABP can also help to predict the need for surgical treatment (39, 70, 71). I-FABP distinguishes patients who need conservative treatment from those that need surgical treatment in the early stage of NEC with the cut-off values of 19 ng/mL (plasma I-FABP) and 232 ng/mL (urinary I-FABP) (40).
I-FABP is also a potential marker for distinguishing NEC from sepsis. In a study of 42 infants, the I-FABP concentration in patients with NEC is significantly higher than that in patients with sepsis (72). Another study combines plasma I-FABP, liver-type FABP (L-FABP), and intestinal trefoil factor as LIT score (0–9) to differentiate NEC from sepsis. The LIT score of children with NEC is significantly higher than that of children with septicemia (P = 0.001) (73).
Although there have been many studies on I-FABP as an early diagnostic and predictive marker of NEC, the cut-off value for the diagnosis and staging of NEC has not been determined due to the inconsistency of the studies. Therefore, multicenter studies with larger sample sizes are needed to aid clinicians in decision making.
Inter-alpha inhibitor proteins
IAIP has extensive anti-inflammatory activity and participates in neutralizing extracellular histone cytotoxicity (74). A prospective observational study of 34 newborns shows that the plasma IAIP level in patients with NEC decreases (P < 0.0001) (46). Shah et al. suggests that IAIP <207 mg/L has a high diagnostic value for NEC (AUC: 0.98, sensitivity: 100%, specificity: 88.2%). On this basis, IAIP can help differentiate NEC from spontaneous intestinal perforation (47). However, IAIP is not specific for predicting NEC as it also decreases in the plasma of patients with sepsis (75). Stober et al. have shown that IAIP can relieve endothelial inflammation in sepsis and protect endothelial cells from damage caused by activation of C5a (76), but there is no research on its effect against NEC.
Differentially expressed genes
Changes in gene expression of the whole blood are mainly related to severe NEC, and their expression in mild NEC changes slightly (48). Compared to normal controls, 53 circular RNAs have been found to change in the NEC group (49). In recent years, studies have identified some DEGs in infants with NEC. The expression of micro RNA (miR)-223 and miR-451a is up-regulated in patients with NEC at disease onset (50, 63) and miR-223/nuclear factor I-A axis may play an important role in the pathophysiology of NEC by aggravating inflammation and tissue damage (77). A meta-analysis shows that miR-429/200a/b and miR-141/200c clusters are poorly expressed in patients with NEC. They can down-regulate target genes related to NEC, and be used as potential biomarkers for early detection (50). Furthermore, a prospective cohort study found that miR1290 might be used as a specific marker for the early diagnosis of NEC (sensitivity: 0.83, specificity: 0.96, DOR: 127.50). It has high diagnostic efficiency and can effectively distinguish NEC from sepsis (51).
Serum amyloid A
SAA is mainly derived from hepatocytes. Its expression increases rapidly in case of inflammatory response. Serum SAA level increases significantly after infection, trauma, and other stimulation (78).
In a study including 144 infants, the SAA level in blood samples of NEC patients is significantly higher than that of healthy controls (43.2 ± 47.5 mg/dl vs. 3.2 ± 3.4 mg/dl, P < 0.05) at the time of NEC diagnosis (52). SAA is more common in predicting NEC together with other markers. A prospective cohort study differentiates NEC from sepsis using apoSAA score, which is calculated from plasma apolipoprotein C2 (apoC2) and SAA; the results show that the apoSAA score is helpful in the early diagnosis of NEC and the differentiation of NEC from sepsis (53). A combination of liver FABP, I-FABP, and SAA may indicate portal venous gas in NEC (38). However, Reisinger et al. find that the combination of urinary SAA and I-FABP can not improve the diagnostic accuracy of NEC compared with I-FABP alone (44). Urinary SAA in the NEC surgery group is significantly higher than that in the conservative group before surgery (38). The cut-off value was 34.4 ng/mL (sensitivity: 83%; specificity: 83%) (45). Therefore, SAA combined with other markers is expected to promote the early diagnosis of NEC.
Cytosolic β-glucosidase
CBG is a member of the cellulase family, which is widely distributed in nature and has also been detected in the human body. CBG is a hydrolytic enzyme distributed in intestinal epithelial cells. Animal experiments find that serum CBG is significantly elevated in ischemic injury caused by arterial occlusion or closed intestinal obstruction (79). Dimmitt et al. measured the serum CBG activity in an NEC rat model and found that serum CBG activity increased before the onset of transmural injury, suggesting that CBG was meaningful for early monitoring of intestinal ischemic injury (54). Subsequently, an increase in serum CBG in neonates with NEC is also confirmed (55). Serum CBG activity of NEC neonates is higher than that of non-NEC neonates, and the cut-off value of 15.6 mU/mg may distinguish neonates with NEC from healthy neonates at the early stage (56). Therefore, CBG can provide potential evidence for the early diagnosis of NEC.
Surrogate markers of NETosis
Neutrophils participate in the NEC inflammatory response by phagocytosis, degranulation, or neutrophil extracellular traps (NETs) (80). NETs occur in both human and mouse NEC tissue (57). The formation of NETs is accompanied by the death of neutrophils, known as NETosis (81). In recent years, studies have shown that NETs play a role in the intestinal inflammatory response containing NEC (58, 82–84). Vincent et al. report that serum substitute markers, such as cfDNA and DNase of NETosis may predict the occurrence of NEC in mouse models; cfDNA and DNase increase significantly with the induction time of the NEC model, and receiver operating curve analysis shows that the cut-off value of cfDNA is 1,250 ng/mL, which has a sensitivity and specificity of 100% for NEC (58). Serum substitutes of NETosis are expected to become biomarkers for the early diagnosis of NEC; however, the evidence is limited. More studies establishing a relationship between NEC and NETosis are still needed.
Absolute monocyte counts
Compared with the baseline value, the AMC of children with NEC significantly decreased. In infants with feeding intolerance, AMC decreases by more than 20%, and the sensitivity and specificity for predicting NEC are 0.70 and 0.71, respectively (59). AMC also differs at different stages of NEC. The AMC of patients with NEC stage III decreases by 81.9%, which is significantly lower than that of II-NEC (44.5%) (60). Thus, AMC may be a potential biomarker for the early diagnosis and severity assessment of NEC.
Microbiota
The normal intestinal flora resists pathogens and participates in the intestinal immune response (85). Intestinal microflora disorders are present before and after NEC (86–88), indicating that microbial communities are related to the occurrence and development of NEC.
A study analyzes the fecal samples of children with NEC using 16 s rRNA and metagenomic sequencing technology and finds that the intestinal microbial diversity of children with NEC decreases (87, 89). Hosfield et al. also demonstrate the association between intestinal microbial diversity and NEC in an animal model (86). In contrast, no difference in intestinal microbial diversity is found in a case-control study of 32 newborns, possibly due to factors such as a small sample size (90). Children with NEC not only have changes in microbial diversity, but they also have significant changes in the composition of the flora. The intestinal flora of healthy infants consists mainly of Bifidobacterium (91). The relative abundance of Bifidobacterium and Lactobacillus in patients with NEC decreases (86, 87, 90, 92), whereas the abundance of Escherichia and Klebsiella increases (86). Similar changes also occur before the onset of NEC (88, 89, 92). Therefore, monitoring neonatal intestinal microbiota may be a potential method for the prediction of NEC. However, its application is limited due to the significant differences in intestinal flora composition among different regions (93).
Maternal milk affects the establishment of the early neonatal intestinal microbiota (94). Human milk oligosaccharides are important components that participate in the regulation of intestinal microbiota by inhibiting pathogen adhesion, growth, and reproduction in the intestinal tract (95). Masi et al. analyzed 19 oligosaccharides of mother's breast milk and found that the concentration of disialyllacto-N-tetraose in oligosaccharides of mother's breast milk of children with NEC decreased (P < 0.001). Univariate analysis shows that the concentration of disialyllacto-N-tetraose is 241 nmol/mL, which can distinguish NEC from non-NEC infants with an accuracy of 91% (92). Another case-control study also supports this conclusion and indicates that oligosaccharides are protective factors against NEC.
Volatile organic compounds (VOCs) in the feces reflect the composition of intestinal microflora and the interaction between microbiota and the host. Fecal VOCs assessed by electronic nose can be used to predict microbial composition (96). Fecal VOC profiles change significantly in inflammatory bowel disease (97) and colitis (98). In NEC experimental animals, fecal VOC profiles are different from those of the control group (86). Moreover, a multicentre prospective study reports that children with NEC can be distinguished from the control group by fecal VOC profiles at 2–3 days (AUC: 0.77 ± 0.21; sensitivity: 83%; specificity: 75%) and 0–1 days (AUC: 0.99 ± 0.04; sensitivity: 89%; specificity: 89%) before the onset of clinical symptoms of NEC (99). Wright et al. also suggest that VOCs can be detected early before NEC occurs (100). Fecal VOC may be a promising marker for the prediction of NEC.
Limitation
We only reviewed the prediction and early diagnosis of NEC with a single tool, and did not discuss the combination of tools. In addition, we have not conducted much analysis on the applicability of each tool.
Summary and conclusion
Although there have been numerous attempts in the literature to develop new techniques or summarize existing regimens to predict the incidence of NEC or diagnose NEC at a very early stage, there have been no established regimen that can be universally accepted. Ultrasound and NIRS mainly detect changes in blood flow and oxygenation, which are helpful in the early prediction of NEC. Novel biomarkers, including calprotectin and I-FABP show great potential to become an independent or part of a complex regimen. However, some other biomarkers are still facing a long way from experimental studies to clinical practice. The intestinal microbiota has been profoundly investigated, but our current achievements still cannot guarantee its wide application. Since the prediction and timely diagnosis of early-stage NEC may significantly benefit NEC victims, future investigations and co-operations are still invaluable.
Method
Data for this review were identified by searches of PubMed, we first searched through “diagnosis” and “necrotizing enterocolitis” and screened out the literature related to the early diagnosis of NEC through abstracts and rough reading of the literature. Then we searched through “prediction” and “necrotizing enterocolitis ” and screened out the literature predicting NEC. Finally, we searched through “ultrasound,” “near infrared spectroscopy,” “biomarkers,” “microbiota,” “diagnosis,” “prediction” and “necrotizing enterocolitis” and other related words were searched to avoid missing relevant literature.
Author contributions
YS and TL designed the overall study. SW drafted the manuscript of the article. SW and SD performed the systematic literature search. YS revised the structure and logic of the article. All authors reviewed the manuscript critically and approved the final version of manuscript.
Funding
This work was supported by National Natural Science Foundation of China (82171709 and 81801500), the 345 Talent Project of Shengjing Hospital (M0275 and M0279), and Key R&D Guidance Plan Projects in Liaoning Province (2020JH1/10300001).
Conflict of interest
The authors declare that the research was conducted in the absence of any commercial or financial relationships that could be construed as a potential conflict of interest.
Publisher's note
All claims expressed in this article are solely those of the authors and do not necessarily represent those of their affiliated organizations, or those of the publisher, the editors and the reviewers. Any product that may be evaluated in this article, or claim that may be made by its manufacturer, is not guaranteed or endorsed by the publisher.
References
1. Battersby C, Santhalingam T, Costeloe K, Modi N. Incidence of neonatal necrotising enterocolitis in high-income countries: a systematic review. Arch Dis Child Fetal Neonatal Ed. (2018) 103:F182–9. doi: 10.1136/archdischild-2017-313880
2. Matei A, Montalva L, Goodbaum A, Lauriti G, Zani A. Neurodevelopmental impairment in necrotising enterocolitis survivors: systematic review and meta-analysis. Arch Dis Child Fetal Neonatal Ed. (2020) 105:432–9. doi: 10.1136/archdischild-2019-317830
3. Bazacliu C, Neu J. Necrotizing enterocolitis: long term complications. Curr Pediatr Rev. (2019) 15:115–24. doi: 10.2174/1573396315666190312093119
4. Fundora JB, Guha P, Shores DR, Pammi M, Maheshwari A. Intestinal dysbiosis and necrotizing enterocolitis: assessment for causality using bradford hill criteria. Pediatr Res. (2020) 87:235–48. doi: 10.1038/s41390-019-0482-9
5. Neu J. Necrotizing enterocolitis: the future. Neonatology. (2020) 117:240–4. doi: 10.1159/000506866
6. Ahle M, Ringertz HG, Rubesova E. The role of imaging in the management of necrotising enterocolitis: a multispecialist survey and a review of the literature. Eur Radiol. (2018) 28:3621–31. doi: 10.1007/s00330-018-5362-x
7. De Bernardo G, Sordino D, De Chiara C, Riccitelli M, Esposito F, Giordano M, et al. Management of nec: surgical treatment and role of traditional X-ray versus ultrasound imaging, experience of a single centre. Curr Pediatr Rev. (2019) 15:125–30. doi: 10.2174/1573396314666181102122626
8. Cuna AC, Reddy N, Robinson AL, Chan SS. Bowel ultrasound for predicting surgical management of necrotizing enterocolitis: a systematic review and meta-analysis. Pediatr Radiol. (2018) 48:658–66. doi: 10.1007/s00247-017-4056-x
9. Tracy SA, Lazow SP, Castro-Aragon IM, Fujii AM, Estroff JA, Parad RB, et al. Is abdominal sonography a useful adjunct to abdominal radiography in evaluating neonates with suspected necrotizing enterocolitis?J Am Coll Surg. (2020) 230:903–11.e2. doi: 10.1016/j.jamcollsurg.2020.01.027
10. Gao HX Yi B, Mao BH Li WY, Bai X, Zhang Y, et al. Efficacy of abdominal ultrasound inspection in the diagnosis and prognosis of neonatal necrotizing enterocolitis. Clinics (São Paulo, Brazil). (2021) 76:e1816. doi: 10.6061/clinics/2021/e1816
11. Guang Y, Ying D, Sheng Y, Yiyong F, Jun W, Shuqiang G, et al. Early doppler ultrasound in the superior mesenteric artery and the prediction of necrotizing enterocolitis in preterm neonates. J Ultrasound Med. (2019) 38:3283–9. doi: 10.1002/jum.15064
12. Murdoch EM, Sinha AK, Shanmugalingam ST, Smith GC, Kempley ST. Doppler flow velocimetry in the superior mesenteric artery on the first day of life in preterm infants and the risk of neonatal necrotizing enterocolitis. Pediatrics. (2006) 118:1999–2003. doi: 10.1542/peds.2006-0272
13. Kim HY, Kim IO, Kim WS, Kang GH. Bowel sonography in sepsis with pathological correlation: an experimental study. Pediatr Radiol. (2011) 41:237–43. doi: 10.1007/s00247-010-1806-4
14. Urboniene A, Palepsaitis A, Uktveris R, Barauskas V. Doppler flowmetry of the superior mesenteric artery and portal vein: impact for the early prediction of necrotizing enterocolitis in neonates. Pediatr Surg Int. (2015) 31:1061–6. doi: 10.1007/s00383-015-3792-y
15. Gokli A, Dillman JR, Humphries PD, Ključevšek D, Mentzel HJ, Rubesova E, et al. Contrast-enhanced ultrasound of the pediatric bowel. Pediatr Radiol. (2021) 51:2214–28. doi: 10.1007/s00247-020-04868-x
16. Braden B, Ignee A, Hocke M, Palmer RM, Dietrich C. Diagnostic value and clinical utility of contrast enhanced ultrasound in intestinal diseases. Dig Liver Dis. (2010) 42:667–74. doi: 10.1016/j.dld.2010.05.018
17. Benjamin JL, Dennis R, White Jr S, Munson D, Anupindi SA, Piskunowicz M, et al. Improved diagnostic sensitivity of bowel disease of prematurity on contrast-enhanced ultrasound. J Ultrasound Med. (2020) 39:1031–6. doi: 10.1002/jum.15168
18. Gumulak R, Lucanova LC, Zibolen M. Use of near-infrared spectroscopy (Nirs) in cerebral tissue oxygenation monitoring in neonates. Biomed Pap Med Fac Univ Palacky Olomouc Czech Repub. (2017) 161:128–33. doi: 10.5507/bp.2017.012
19. Chen MW, Reyes M, Kulikowicz E, Martin L, Hackam DJ, Koehler RC, et al. Abdominal near-infrared spectroscopy in a piglet model of gastrointestinal hypoxia produced by graded hypoxia or superior mesenteric artery ligation. Pediatr Res. (2018) 83:1172–81. doi: 10.1038/pr.2018.37
20. Cortez J, Gupta M, Amaram A, Pizzino J, Sawhney M, Sood BG. Noninvasive evaluation of splanchnic tissue oxygenation using near-infrared spectroscopy in preterm neonates. J Matern Fetal Neonatal Med. (2011) 24:574–82. doi: 10.3109/14767058.2010.511335
21. Kimak KS, de Castro Antunes MM, Braga TD, Brandt KG, de Carvalho Lima M. Influence of enteral nutrition on occurrences of necrotizing enterocolitis in very-low-birth-weight infants. J Pediatr Gastroenterol Nutr. (2015) 61:445–50. doi: 10.1097/MPG.0000000000000835
22. Schat TE, van Zoonen A, van der Laan ME, Mebius MJ, Bos AF, Hulzebos CV, et al. Early cerebral and intestinal oxygenation in the risk assessment of necrotizing enterocolitis in preterm infants. Early Hum Dev. (2019) 131:75–80. doi: 10.1016/j.earlhumdev.2019.03.001
23. Palleri E, Wackernagel D, Wester T, Bartocci M. Low splanchnic oxygenation and risk for necrotizing enterocolitis in extremely preterm newborns. J Pediatr Gastroenterol Nutr. (2020) 71:401–6. doi: 10.1097/MPG.0000000000002761
24. Patel AK, Lazar DA, Burrin DG, Smith EO, Magliaro TJ, Stark AR, et al. Abdominal near-infrared spectroscopy measurements are lower in preterm infants at risk for necrotizing enterocolitis. Pediatr Crit Care Med. (2014) 15:735–41. doi: 10.1097/PCC.0000000000000211
25. Balegar VK, Low GK, Nanan RK. Regional tissue oxygenation and conventional indicators of red blood cell transfusion in anaemic preterm infants. EClin Med. (2022) 46:101365. doi: 10.1016/j.eclinm.2022.101365
26. Berken JA, Chang J. Neurologic consequences of neonatal necrotizing enterocolitis. Dev Neurosci. (2022). doi: 10.1159/000525378
27. Bailey SM, Mally PV. Review of splanchnic oximetry in clinical medicine. J Biomed Opt. (2016) 21:091306. doi: 10.1117/1.JBO.21.9.091306
28. Isler H, Schenk D, Bernhard J, Kleiser S, Scholkmann F, Ostojic D, et al. Absorption spectra of early stool from preterm infants need to be considered in abdominal nirs oximetry. Biomed Opt Express. (2019) 10:2784–94. doi: 10.1364/BOE.10.002784
29. McNeill S, Gatenby JC, McElroy S, Engelhardt B. Normal cerebral, renal and abdominal regional oxygen saturations using near-infrared spectroscopy in preterm infants. J Perinatol. (2011) 31:51–7. doi: 10.1038/jp.2010.71
30. Zoppelli L, Guttel C, Bittrich HJ, Andree C, Wirth S, Jenke A. Fecal calprotectin concentrations in premature infants have a lower limit and show postnatal and gestational age dependence. Neonatology. (2012) 102:68–74. doi: 10.1159/000337841
31. Thibault MP, Tremblay E, Horth C, Fournier-Morin A, Grynspan D, Babakissa C, et al. Lipocalin-2 and calprotectin as stool biomarkers for predicting necrotizing enterocolitis in premature neonates. Pediatr Res. (2022) 91:129–36. doi: 10.1038/s41390-021-01680-7
32. MacQueen BC, Christensen RD, Yost CC, Gordon PV, Baer VL, Schlaberg R, et al. Reference intervals for stool calprotectin in preterm neonates and their utility for the diagnosis of necrotizing enterocolitis. J Perinatol. (2018) 38:1379–85. doi: 10.1038/s41372-018-0108-9
33. Qu Y, Xu W, Han J, Zhou W, Wu H. Diagnostic value of fecal calprotectin in necrotizing enterocolitis: a meta-analysis. Early Hum Dev. (2020) 151:105170. doi: 10.1016/j.earlhumdev.2020.105170
34. Goold E, Pearson L, Johnson LM. Can fecal calprotectin serve as a screen for necrotizing enterocolitis in infants? Clin Biochem. (2020) 84:51–4. doi: 10.1016/j.clinbiochem.2020.06.015
35. Dabritz J, Jenke A, Wirth S, Foell D. Fecal phagocyte-specific S100a12 for diagnosing necrotizing enterocolitis. J Pediatr. (2012) 161:1059–64. doi: 10.1016/j.jpeds.2012.06.003
36. Biesterveld BE, Koehler SM, Heinzerling NP, Rentea RM, Fredrich K, Welak SR, et al. Intestinal alkaline phosphatase to treat necrotizing enterocolitis. J Surg Res. (2015) 196:235–40. doi: 10.1016/j.jss.2015.02.030
37. Heath M, Buckley R, Gerber Z, Davis P, Linneman L, Gong Q, et al. Association of intestinal alkaline phosphatase with necrotizing enterocolitis among premature infants. JAMA Netw Open. (2019) 2:e1914996. doi: 10.1001/jamanetworkopen.2019.14996
38. Coufal S, Kokesova A, Tlaskalova-Hogenova H, Frybova B, Snajdauf J, Rygl M, et al. Urinary I-Fabp, L-Fabp, Tff-3, and Saa can diagnose and predict the disease course in necrotizing enterocolitis at the early stage of disease. J Immunol Res. (2020) 2020:3074313. doi: 10.1155/2020/3074313
39. El-Abd Ahmed A, Hassan MH, Abo-Halawa N, Abdel-Razik GM, Moubarak FA, Sakhr HM. Lactate and intestinal fatty acid binding protein as essential biomarkers in neonates with necrotizing enterocolitis: ultrasonographic and surgical considerations. Pediatr Neonatol. (2020) 61:481–9. doi: 10.1016/j.pedneo.2020.03.015
40. Schurink M, Kooi EM, Hulzebos CV, Kox RG, Groen H, Heineman E, et al. Intestinal fatty acid-binding protein as a diagnostic marker for complicated and uncomplicated necrotizing enterocolitis: a prospective cohort study. PLoS ONE. (2015) 10:e0121336. doi: 10.1371/journal.pone.0121336
41. Gregory KE, Winston AB, Yamamoto HS, Dawood HY, Fashemi T, Fichorova RN, et al. Urinary intestinal fatty acid binding protein predicts necrotizing enterocolitis. J Pediatr. (2014) 164:1486–8. doi: 10.1016/j.jpeds.2014.01.057
42. Gollin G, Stadie D, Mayhew J, Slater L, Asmerom Y, Boskovic D, et al. Early detection of impending necrotizing enterocolitis with urinary intestinal fatty acid-binding protein. Neonatology. (2014) 106:195–200. doi: 10.1159/000362497
43. Thuijls G, Derikx JP, van Wijck K, Zimmermann LJ, Degraeuwe PL, Mulder TL, et al. Non-invasive markers for early diagnosis and determination of the severity of necrotizing enterocolitis. Ann Surg. (2010) 251:1174–80. doi: 10.1097/SLA.0b013e3181d778c4
44. Reisinger KW, Van der Zee DC, Brouwers HA, Kramer BW, van Heurn LW, Buurman WA, et al. Noninvasive measurement of fecal calprotectin and serum amyloid a combined with intestinal fatty acid-binding protein in necrotizing enterocolitis. J Pediatr Surg. (2012) 47:1640–5. doi: 10.1016/j.jpedsurg.2012.02.027
45. Reisinger KW, Kramer BW, Van der Zee DC, Brouwers HA, Buurman WA, van Heurn E, et al. Non-invasive serum amyloid a (Saa) measurement and plasma platelets for accurate prediction of surgical intervention in severe necrotizing enterocolitis (Nec). PLoS ONE. (2014) 9:e90834. doi: 10.1371/journal.pone.0090834
46. Chaaban H, Shin M, Sirya E, Lim YP, Caplan M, Padbury JF. Inter-alpha inhibitor protein level in neonates predicts necrotizing enterocolitis. J Pediatr. (2010) 157:757–61. doi: 10.1016/j.jpeds.2010.04.075
47. Shah BA, Migliori A, Kurihara I, Sharma S, Lim YP, Padbury J. Blood level of inter-alpha inhibitor proteins distinguishes necrotizing enterocolitis from spontaneous intestinal perforation. J Pediatr. (2017) 180:135–40 e1. doi: 10.1016/j.jpeds.2016.09.016
48. Pan X, Muk T, Ren S, Nguyen DN, Shen RL, Gao F, et al. Blood transcriptomic markers of necrotizing enterocolitis in preterm pigs. Pediatr Res. (2022) 91:1113–20. doi: 10.1038/s41390-021-01605-4
49. Pan Y, Chen W, Yan X, Yu B, Yao S, Chen X, et al. Differential expression profiles and functional prediction of circrnas in necrotizing enterocolitis. Biomed Res Int. (2021) 2021:9862066. doi: 10.1155/2021/9862066
50. Liu H, Wang YB. Systematic large-scale meta-analysis identifies mirna-429/200a/B and mirna-141/200c clusters as biomarkers for necrotizing enterocolitis in newborn. Biosci Rep. (2019) 39:BSR20191503. doi: 10.1042/BSR20191503
51. Ng PC, Chan KYY, Yuen TP, Sit T, Lam HS, Leung KT, et al. Plasma Mir-1290 is a novel and specific biomarker for early diagnosis of necrotizing enterocolitis-biomarker discovery with prospective cohort evaluation. J Pediatr. (2019) 205:83–90 e10. doi: 10.1016/j.jpeds.2018.09.031
52. Cetinkaya M, Ozkan H, Köksal N, Akaci O, Ozgür T. The efficacy of serial serum amyloid a measurements for diagnosis and follow-up of necrotizing enterocolitis in premature infants. Pediatr Surg Int. (2010) 26:835–41. doi: 10.1007/s00383-010-2635-0
53. Ng PC, Ang IL, Chiu RW Li K, Lam HS, Wong RP, et al. Host-response biomarkers for diagnosis of late-onset septicemia and necrotizing enterocolitis in preterm infants. J Clin Invest. (2010) 120:2989–3000. doi: 10.1172/JCI40196
54. Dimmitt RA, Glew R, Colby C, Brindle M, Skarsgard E, Moss RL. Serum cytosolic beta-glucosidase activity in a rat model of necrotizing enterocolitis. Pediatr Res. (2003) 54:462–5. doi: 10.1203/01.PDR.0000081310.47579.49
55. Dongmei C, Guofeng L, Weilin P. Serum cytosolic B-glucosidase levels in neonatal necrotizing enterocolitis. Iran J Pediatr. (2012) 22:452–6.
56. Gomez-Chaparro Moreno JL, Rodriguez Torronteras A, Ruiz Gonzalez MD, Izquierdo Palomares L, Bonilla Valverde D, Ruiz Laguna J, et al. The beta-glucosidase assay: a new diagnostic tool for necrotizing enterocolitis. Sensitivity, specificity, and predictive values. Eur J Pediatr. (2016) 175:931–41. doi: 10.1007/s00431-016-2724-8
57. Chaaban H, Burge K, Eckert J, Keshari RS, Silasi R, Lupu C, et al. Neutrophil extracellular trap inhibition increases inflammation, bacteraemia and mortality in murine necrotizing enterocolitis. J Cell Mol Med. (2021) 25:10814–24. doi: 10.1111/jcmm.15338
58. Vincent D, Klinke M, Eschenburg G, Trochimiuk M, Appl B, Tiemann B, et al. Nec is likely a nets dependent process and markers of netosis are predictive of nec in mice and humans. Sci Rep. (2018) 8:12612. doi: 10.1038/s41598-018-31087-0
59. Remon J, Kampanatkosol R, Kaul RR, Muraskas JK, Christensen RD, Maheshwari A. Acute drop in blood monocyte count differentiates nec from other causes of feeding intolerance. J Perinatol. (2014) 34:549–54. doi: 10.1038/jp.2014.52
60. Desiraju S, Bensadoun J, Bateman D, Kashyap S. The role of absolute monocyte counts in predicting severity of necrotizing enterocolitis. J Perinatol. (2020) 40:922–7. doi: 10.1038/s41372-020-0596-2
61. Ayling RM, Kok K. Fecal calprotectin. Adv Clin Chem. (2018) 87:161–90. doi: 10.1016/bs.acc.2018.07.005
62. Vogl T, Tenbrock K, Ludwig S, Leukert N, Ehrhardt C, van Zoelen MAD, et al. Mrp8 and Mrp14 are endogenous activators of toll-like receptor 4, promoting lethal, endotoxin-induced shock. Nat Med. (2007) 13:1042–9. doi: 10.1038/nm1638
63. Ng PC, Chan KYY, Lam HS, Wong RPO, Ma TPY, Sit T, et al. A prospective cohort study of fecal Mir-223 and Mir-451a as noninvasive and specific biomarkers for diagnosis of necrotizing enterocolitis in preterm infants. Neonatology. (2020) 117:555–61. doi: 10.1159/000511655
64. van Zoonen A, Hulzebos CV, Muller Kobold AC, Kooi EMW, Bos AF, Hulscher JBF. Serial fecal calprotectin in the prediction of necrotizing enterocolitis in preterm neonates. J Pediatr Surg. (2019) 54:455–9. doi: 10.1016/j.jpedsurg.2018.04.034
65. Foell D, Wittkowski H, Kessel C, Lüken A, Weinhage T, Varga G, et al. Proinflammatory S100a12 can activate human monocytes via toll-like receptor 4. Am J Respir Crit Care Med. (2013) 187:1324–34. doi: 10.1164/rccm.201209-1602OC
66. Zhang X, Shen R, Shu Z, Zhang Q, Chen Z. S100a12 promotes inflammation and apoptosis in ischemia/reperfusion injury via erk signaling in vitro study using Pc12 cells. Pathol Int. (2020) 70:403–12. doi: 10.1111/pin.12924
67. Bilski J, Mazur-Bialy A, Wojcik D, Zahradnik-Bilska J, Brzozowski B, Magierowski M, et al. The role of intestinal alkaline phosphatase in inflammatory disorders of gastrointestinal tract. Med Inflamm. (2017) 2017:9074601. doi: 10.1155/2017/9074601
68. Li B, Hao J, Zeng J, Sauter ER. Snapshot: fabp functions. Cell. (2020) 182:1066-.e1. doi: 10.1016/j.cell.2020.07.027
69. Voth M, Lustenberger T, Relja B, Marzi I. Is I-fabp not only a marker for the detection abdominal injury but also of hemorrhagic shock in severely injured trauma patients? World J Emerg Surg. (2019) 14:49. doi: 10.1186/s13017-019-0267-9
70. Abdel-Haie OM, Behiry EG, Abd Almonaem ER, Ahmad ES, Assar EH. Predictive and diagnostic value of serum intestinal fatty acid binding protein in neonatal necrotizing enterocolitis (case series). Ann Med Surg. (2017) 21:9–13. doi: 10.1016/j.amsu.2017.05.010
71. Evennett NJ, Hall NJ, Pierro A, Eaton S. Urinary intestinal fatty acid-binding protein concentration predicts extent of disease in necrotizing enterocolitis. J Pediatr Surg. (2010) 45:735–40. doi: 10.1016/j.jpedsurg.2009.09.024
72. Coufal S, Kokesova A, Tlaskalova-Hogenova H, Snajdauf J, Rygl M, Kverka M. Urinary intestinal fatty acid-binding protein can distinguish necrotizing enterocolitis from sepsis in early stage of the disease. J Immunol Res. (2016) 2016:5727312. doi: 10.1155/2016/5727312
73. Ng EW, Poon TC, Lam HS, Cheung HM, Ma TP, Chan KY, et al. Gut-associated biomarkers L-Fabp, I-Fabp, and Tff3 and lit score for diagnosis of surgical necrotizing enterocolitis in preterm infants. Ann Surg. (2013) 258:1111–8. doi: 10.1097/SLA.0b013e318288ea96
74. Chaaban H, Keshari RS, Silasi-Mansat R, Popescu NI. Mehta-D'Souza P, Lim YP, et al. Inter-A inhibitor protein and its associated glycosaminoglycans protect against histone-induced. Injury Blood. (2015) 125:2286–96. doi: 10.1182/blood-2014-06-582759
75. Baek YW, Brokat S, Padbury JF, Pinar H, Hixson DC, Lim YP. Inter-alpha inhibitor proteins in infants and decreased levels in neonatal sepsis. J Pediatr. (2003) 143:11–5. doi: 10.1016/S0022-3476(03)00190-2
76. Stober VP, Lim YP, Opal S, Zhuo L, Kimata K, Garantziotis S. Inter-A-inhibitor ameliorates endothelial inflammation in sepsis. Lung. (2019) 197:361–9. doi: 10.1007/s00408-019-00228-1
77. Wu YZ, Chan KYY, Leung KT, Lam HS, Tam YH, Lee KH, et al. The mir-223/nuclear factor I-a axis regulates inflammation and cellular functions in intestinal tissues with necrotizing enterocolitis. FEBS Open Bio. (2021) 11:1907–20. doi: 10.1002/2211-5463.13164
78. Sack Jr GH. Serum amyoid a (Saa) proteins. Sub Cell Biochem. (2020) 94:421–36. doi: 10.1007/978-3-030-41769-7_17
79. Morris S, Hays W, Enomoto M, Glew R, Feddersen R, Fry D, et al. Serum cytosolic beta-glucosidase elevation and early ischemic injury to guinea pig small intestine. Surgery. (1999) 125:202–10. doi: 10.1016/S0039-6060(99)70266-7
80. Papayannopoulos V. Neutrophil extracellular traps in immunity and disease. Nat Rev Immunol. (2018) 18:134–47. doi: 10.1038/nri.2017.105
81. Thiam HR, Wong SL, Wagner DD, Waterman CM. Cellular mechanisms of netosis. Annu Rev Cell Dev Biol. (2020) 36:191–218. doi: 10.1146/annurev-cellbio-020520-111016
82. Klinke M, Vincent D, Trochimiuk M, Appl B, Tiemann B, Bergholz R, et al. Degradation of extracellular dna significantly ameliorates necrotizing enterocolitis severity in mice. J Surg Res. (2019) 235:513–20. doi: 10.1016/j.jss.2018.10.041
83. Klinke M, Vincent D, Trochimiuk M, Appl B, Tiemann B, Reinshagen K, et al. Development of an improved murine model of necrotizing enterocolitis shows the importance of neutrophils in nec pathogenesis. Sci Rep. (2020) 10:8049. doi: 10.1038/s41598-020-65120-y
84. Klinke M, Wiskemann H, Bay B, Schäfer HJ, Pagerols Raluy L, Reinshagen K, et al. Cardiac and inflammatory necrotizing enterocolitis in newborns are not the same entity. Front Pediatr. (2020) 8:593926. doi: 10.3389/fped.2020.593926
85. Ronan V, Yeasin R, Claud EC. Childhood development and the microbiome-the intestinal microbiota in maintenance of health and development of disease during childhood development. Gastroenterology. (2021) 160:495–506. doi: 10.1053/j.gastro.2020.08.065
86. Hosfield BD, Drucker NA, Pecoraro AR, Shelley WC Li H, Baxter NT, et al. The assessment of microbiome changes and fecal volatile organic compounds during experimental necrotizing enterocolitis. J Pediatr Surg. (2021) 56:1220–5. doi: 10.1016/j.jpedsurg.2021.02.043
87. Tarracchini C, Milani C, Longhi G, Fontana F, Mancabelli L, Pintus R, et al. Unraveling the microbiome of necrotizing enterocolitis: insights in novel microbial and metabolomic biomarkers. Microbiol Spectr. (2021) 9:e0117621. doi: 10.1128/Spectrum.01176-21
88. Olm MR, Bhattacharya N, Crits-Christoph A, Firek BA, Baker R, Song YS, et al. Necrotizing enterocolitis is preceded by increased gut bacterial replication, klebsiella, and fimbriae-encoding bacteria. Sci Adv. (2019) 5:eaax5727. doi: 10.1126/sciadv.aax5727
89. Dobbler PT, Procianoy RS, Mai V, Silveira RC, Corso AL, Rojas BS, et al. Low microbial diversity and abnormal microbial succession is associated with necrotizing enterocolitis in preterm infants. Front Microbiol. (2017) 8:2243. doi: 10.3389/fmicb.2017.02243
90. Feng J, He Y, Liu D, Li L, Chen J, Yu J. The constitution and functional prediction of the microbiota in necrotizing enterocolitis with a gestational age of over 28 weeks. Medicine. (2019) 98:e17206. doi: 10.1097/MD.0000000000017206
91. Saturio S, Nogacka AM, Suárez M, Fernández N, Mantecón L, Mancabelli L, et al. Early-life development of the bifidobacterial community in the infant gut. Int J Mol Sci. (2021) 22:3382. doi: 10.3390/ijms22073382
92. Masi AC, Embleton ND, Lamb CA, Young G, Granger CL, Najera J, et al. Human milk oligosaccharide dslnt and gut microbiome in preterm infants predicts necrotising enterocolitis. Gut. (2021) 70:2273–82. doi: 10.1136/gutjnl-2020-322771
93. Ravi A, Estensmo ELF, Abee-Lund TML, Foley SL, Allgaier B, Martin CR, et al. Association of the gut microbiota mobilome with hospital location and birth weight in preterm infants. Pediatr Res. (2017) 82:829–38. doi: 10.1038/pr.2017.146
94. Milani C, Duranti S, Bottacini F, Casey E, Turroni F, Mahony J, et al. The first microbial colonizers of the human gut: composition, activities, and health implications of the infant gut microbiota. Microbiol Mol Biol Rev. (2017) 81:e00036–17. doi: 10.1128/MMBR.00036-17
95. Plaza-Díaz J, Fontana L, Gil A. Human milk oligosaccharides and immune system development. Nutrients. (2018) 10:1038. doi: 10.3390/nu10081038
96. Hosfield BD, Pecoraro AR, Baxter NT, Hawkins TB, Markel TA. The assessment of fecal volatile organic compounds in healthy infants: electronic nose device predicts patient demographics and microbial enterotype. J Surg Res. (2020) 254:340–7. doi: 10.1016/j.jss.2020.05.010
97. El Manouni El Hassani S, Bosch S, Lemmen JPM, Brizzio Brentar M, Ayada I, Wicaksono AN, et al. Simultaneous assessment of urinary and fecal volatile organic compound analysis in de novo pediatric Ibd. Sensors. (2019) 19:4496. doi: 10.3390/s19204496
98. Reade S, Williams JM, Aggio R, Duckworth CA, Mahalhal A, Hough R, et al. Potential role of fecal volatile organic compounds as biomarkers of chemically induced intestinal inflammation in mice. FASEB J. (2019) 33:3129–36. doi: 10.1096/fj.201800076RR
99. de Meij TG, van der Schee MP, Berkhout DJ, van de Velde ME, Jansen AE, Kramer BW, et al. Early detection of necrotizing enterocolitis by fecal volatile organic compounds analysis. J Pediatr. (2015) 167:562–7 e1. doi: 10.1016/j.jpeds.2015.05.044
Keywords: necrotizing enterocolitis, early prediction, ultrasound, near-infrared spectroscopy, biomarkers, microbiota
Citation: Wu S, Di S, Liu T and Shi Y (2022) Emerging prediction methods for early diagnosis of necrotizing enterocolitis. Front. Med. 9:985219. doi: 10.3389/fmed.2022.985219
Received: 03 July 2022; Accepted: 31 August 2022;
Published: 16 September 2022.
Edited by:
Maria Pierro, Azienda Unità Sanitaria Locale (AUSL) della Romagna, ItalyReviewed by:
Alain Cuna, Children's Mercy Hospital, United StatesMehdi Oualha, Unité de soins pédiatriques intensifs Hôpital Necker, hôpital Necker-Enfants Malades, France
Copyright © 2022 Wu, Di, Liu and Shi. This is an open-access article distributed under the terms of the Creative Commons Attribution License (CC BY). The use, distribution or reproduction in other forums is permitted, provided the original author(s) and the copyright owner(s) are credited and that the original publication in this journal is cited, in accordance with accepted academic practice. No use, distribution or reproduction is permitted which does not comply with these terms.
*Correspondence: Yongyan Shi, eXlzaGlAY211LmVkdS5jbg==; Tianjing Liu, bGl1dGpAc2otaG9zcGl0YWwub3Jn