Evaluation of bacterial safety approaches of platelet blood concentrates: bacterial screening and pathogen reduction
- 1Department of Hematology, Faculty of Allied Medicine, Iran University of Medical Sciences, Tehran, Iran
- 2BioClinicum, Department of Oncology-Pathology, Karolinska Institute, Stockholm, Sweden
- 3Pediatrics Growth and Development Research Center, Institute of Endocrinology and Metabolism, Iran University of Medical Sciences, Tehran, Iran
- 4Autoimmune Diseases Research Center, Shahid Beheshti Hospital, Kashan University of Medical Sciences, Kashan, Iran
- 5Physiology Research Center, Institute for Basic Sciences, Kashan University of Medical Sciences, Kashan, Iran
- 6Department of Laboratory Sciences, Sirjan School of Medical Sciences, Sirjan, Iran
This mini-review analyzed two approaches to screening bacterial contamination and utilizing pathogen reduction technology (PRT) for Platelet concentrates (PCs). While the culture-based method is still considered the gold standard for detecting bacterial contamination in PCs, efforts in the past two decades to minimize transfusion-transmitted bacterial infections (TTBIs) have been insufficient to eliminate this infectious threat. PRTs have emerged as a crucial tool to enhance safety and mitigate these risks. The evidence suggests that the screening strategy for bacterial contamination is more successful in ensuring PC quality, decreasing the necessity for frequent transfusions, and improving resistance to platelet transfusion. Alternatively, the PRT approach is superior regarding PC safety. However, both methods are equally effective in managing bleeding. In conclusion, PRT can become a more prevalent means of safety for PCs compared to culture-based approaches and will soon comprehensively surpass culture-based bacterial contamination detection methods.
1 Introduction
More than 100 million blood units are transfused annually, making blood transfusion one of the most common hospital procedures (1). Among these, platelet concentrate (PC) transfusion is vital in various hematological and oncological diseases. Studies show 9–30% of intensive care unit (ICU) patients receive platelet transfusions (2, 3).
Infections caused by bacteria entering the patient’s body, transfusion-transmitted bacterial infections (TTBIs), remain a significant cause of mortality and morbidity. According to the US Food and Drug Administration (US FDA) report, infections caused by bacterial contamination between 2012 and 2016 are the third cause of mortality caused by blood transfusion (4). Compared to viral infection, bacterial infection occurs 100 to 1,000 times more.
The testing for bacterial contamination in PCs is typically done through platelet fraction sampling, as PCs are more susceptible to bacterial contamination than other products (4, 5). Bacterial contamination can range from a very low titer to as high as 1010 colony-forming units (CFUs) in each bag (6, 7).
While culture-based methods are still considered the gold standard in detecting bacterial contamination in PCs, they do not eliminate the risk of transmission of TTBIs (5, 8). There are two ways to increase the safety of PCs: pathogen reduction (PR) and bacterial screening (7, 9). Presently, three commonly used technologies—INTERCEPT (confirmed by the US FDA), Mirasol, and THERAFLEX—employ exposure to ultraviolet (UV) light to reduce the risk of various pathogens (10).
In a previous review, we evaluated screening methods for bacterial contamination in PCs (5). This mini-review is based on the PubMed database to analyze the advantages and disadvantages of various PRTs and bacterial screening. All articles were collected until October 2023. This review focuses on identifying bacterial contamination in PCs, as traditional tests involve sampling the platelet portion.
2 Bacterial contaminations
Platelet concentrates (PCs) were first introduced as a therapeutic product in the 1960s and have since become a crucial component in treating thrombocytopenia, particularly in cases caused by chemotherapy (11). PCs include single donor platelets (SDPs) and whole blood platelets (WBPs). There are two methods for preparing whole blood platelets (WBPs): Buffy coat (BC) and platelet-rich plasma (PRP).
The primary contamination source is bacteria from the skin’s normal flora due to inadequate sterilization of equipment and surfaces. PCs may contain Gram-positive and Gram-negative bacteria (5, 12). Notably, Staphylococcus epidermidis, a Gram-positive bacterium, is the most frequently isolated organism in PCs (5).
PCs now have a 5-day shelf life, instead of 7, to comply with US FDA recommendations and prevent bacterial contamination (12). Some countries, such as Japan, have further reduced the lifespan of PCs from 5 days to 3 days to reduce bacterial contamination (13). However, it is essential to note that bacterial contamination is only sometimes detectable before PCs are injected. PCs are the most susceptible to infection and sepsis of all blood products, primarily because they are stored at room temperature (RT). However, PRT is an effective method for extending the shelf life of PCs by up to 7 days (2).
Storage PCs at a cold temperature (1–6°C) can also be effective in reducing the growth of bacteria, maintaining platelet function (14), and, at the same time, increasing their lifespan (11). Most studies in the last two decades found platelet concentrates cold storage (CS) is beneficial (15). Some studies have shown that CS can have harmful and even irreversible effects on their morphology and function (16, 17). There is also some concern about whether PRT can be used on CS platelets without negatively affecting their therapeutic effectiveness (18). More research is needed to determine the effectiveness of CS platelets in treating acute bleeding despite their superiority over RT platelets (19, 20). The US FDA recently approved a 14-day shelf life of PCs to treat active bleeding only when RT platelets are unavailable (21).
The first prevention strategy involves screening and selecting donors based on their medical condition and asking about any recent antibiotic treatments (22). Immunological procedures to prevent transfusion-transmitted bacterial infections (TTBIs) begin with careful donor screening and blood testing for known pathogens (23). However, this approach cannot detect asymptomatic bacteremia (22, 24). Another effective method is to remove a small amount of blood (around 10–20 mL) at the beginning of the collection process, which can significantly reduce the risk of bacterial contamination, especially from gram-positive bacteria, although it cannot eliminate it. The diversion method is a standard for preventing bacterial contamination (24).
Two other significant strategies include bacterial screening methods and pathogen reduction technologies (PRTs) (2). Table 1 provides a detailed comparison of these strategies.
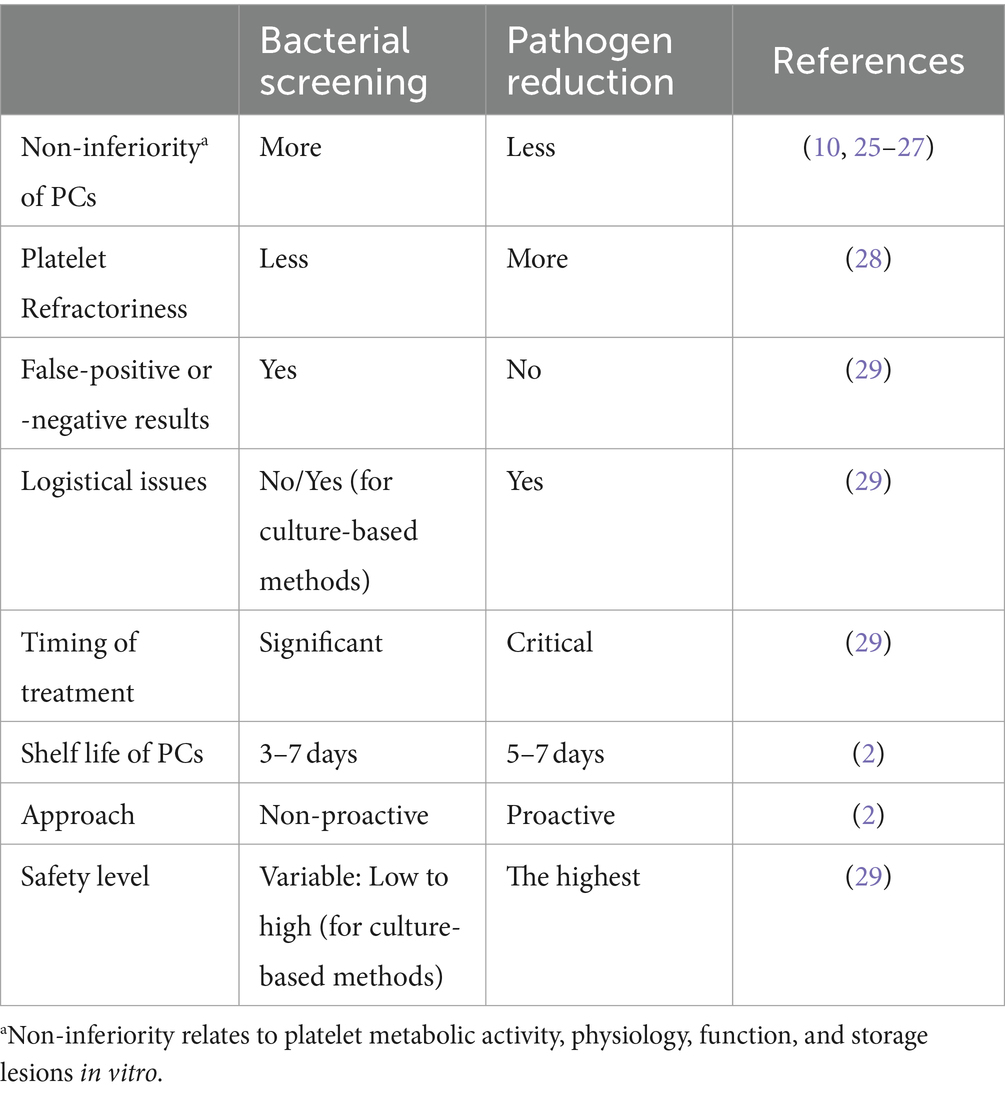
Table 1. Comparison of advantages and disadvantages of two strategies of bacterial screening and pathogen reduction (PR) of platelet concentrates (PCs).
3 Discussion
3.1 Bacterial screening methods of platelet concentrates
While the reported frequency of bacterial contamination ranges from 1 in 750 to 1 in 2,500 blood units, both transfusion-transmitted bacterial infections (TTBIs) and sepsis transfusion reactions (STRs) are often underreported and can seriously threaten patients (2). One factor contributing to this issue is that many centers only perform aerobic blood cultures, which cannot detect anaerobic bacterial contamination (30). Additionally, some hospitals fail to quarantine PCs for the recommended 12 to 24 h, which increases the risk of STRs. However, even a 24-h quarantine may not eliminate slow-growing or non-multiplying bacteria in storage conditions.
The second critical strategy in preventing the occurrence of bacterial, PC screening methods, can be divided into two categories: culture-based and non-culture-based.
First, non-culture-based methods offer the crucial advantage of being time-effective. Although culture-based methods will remain the gold standard, the significance of rapid methods for screening for bacterial contamination has increased. Rapid new methods provide powerful tools for improving the bacterial safety of blood components (6). The analytical sensitivity between the different detection methods ranged between 50 and 100,000 CFU/mL. The sample volume these testing systems use varies between 0.5 and 1.0 mL of PCs (31). Generally, non-culture-based methods are classified into low-sensitivity and high-sensitivity subgroups.
The high-sensitivity subset is composed of nuclear polymerase reaction (PCR) with a sensitivity of 10–103 colony forming unit (CFU) per milliliter of platelet concentrate (ml PC) and flows cytometry with a sensitivity of 10–103 CFU (mL PC) (32). Nonetheless, implementing non-culture techniques is complicated and costly regardless of the challenges of detecting bacterial contamination during the first few days of PC storage life.
There are several low-sensitivity methods to detect bacterial contamination in PCs. These methods include gram staining, acridine orange, glucose consumption, pH measurement, pan genera detection (PGD), and bio-responsive polymers. Gram staining and acridine orange have similar sensitivity levels (105–106 CFU/mL PC) but require significant time and expertise to perform accurately. Moreover, their favorable detection rates are low (33). Glucose consumption and pH measurement methods are not commonly used in clinical settings due to their high rate of false positives (34). However, using these methods shortly before injection can enhance PCs’ safety (35). The PGD method is a qualitative immunological technique that detects lipopolysaccharide (LPS) in gram-negative bacteria and lipoteichoic acid in Gram-positive bacteria. Bio-responsive polymers use enzyme reactions to detect bacterial contamination (36). Of all the methods listed, only the PGD approach is approved by the US FDA. It is used to ensure the safety of blood distribution points and for quality control measurements. This test extends the lifespan of PCs from 5 days to 7 days by measuring them within 24 h of injection on day 6 or 7 (or both) (2, 6).
Non-culture-based methods are generally known for having high false positive results and needing to be more laborious and costly (2). The visual inspection method can effectively identify bacterial contamination in its place (37). Sample collection should occur in the final 48 h of PC storage or just before product injection due to their lower sensitivity for non-culture-based methods. The key benefit of these methods is their ability to produce rapid results before PC injection (31).
Second, culture-based methods are currently considered the most reliable way to detect bacterial contamination. These methods can be used to check for contamination during the storage period of PCs or shortly before they are given to patients. However, waiting until before injection may be too late to prevent contamination. It is recommended to check for contamination on the third or fourth day of the platelet product’s storage period (38). However, these methods require a large sample and an incubation time of more than 24 h (39). The BacT/ALERT and eBDS systems have been approved by the FDA.
Culture-based systems generally have a high sensitivity and can detect 1–10 CFU per ml. However, their sensitivity may need to be revised and can vary depending on the age of the product being tested (8). Most culture-based systems may not be effective in detecting biofilm-producing bacteria (6, 13). Bacterial culture quality control in PCs is primarily carried out through culture-based methods. However, these methods are time-consuming and are not considered rapid screening methods. Research has shown that sepsis caused by PCs cannot be entirely prevented, regardless of the age of the product (37).
3.2 Pathogen reduction technologies
Pathogen reduction (PR) is a highly effective third strategy that has proven particularly useful in reducing bacterial contamination in PCs. PR technologies (PRTs) that employ ultraviolet (UV) rays are commonly used in blood products to eliminate contamination and prevent TTBIs. The irreversible prevention of DNA replication and RNA transcription by UV rays is highly effective in targeting viruses, bacteria, parasites, protozoa, and other nucleated cells, such as leukocytes (2). In addition, PRT is a proactive approach compared to donor screening strategies and PC screening (9).
PRT can effectively prolong the shelf life of PCs (to 7 days). Platelet activation and storage lesions (PSLs) are inherent to PR technologies. Further studies are necessary to improve the safety and efficacy of platelet recovery treatments, as these lesions can be large enough to affect post-transfusion platelet survival. A platelet additive solution (PAS) has not entirely solved these problems (40). Other studies have revealed that PR’s effect on platelet function during storage of PCs is minimal depending on time, particularly in the last days (5th and later) and technology employed. However, PRT treatment may require more injections at shorter intervals and may be associated with increased platelet resistance and alloimmunization (28). On the other hand, most studies indicate that PRT has the necessary safety standards for injection to patients (41–46).
Despite the challenge posed by the cost of these technologies (47), data have shown that they can be cost-effective compared to other blood safety interventions (48). Results of a review study revealed that PCs have the highest safety standards in countries such as Belgium, Switzerland, and France, where PR is performed. However, there are logistical challenges (centralized vs. peripherally localized platelet product preparation sites, hospital-based vs. regionally or nationally organized blood services, etc.), potential risks of pyrogenic compounds, insufficient killing of sporulating and biofilm-producing bacteria, time of treatment, as well as additional costs that need to be considered. Although some of these challenges, such as additional cost and logistical issues, may also be seen in other strategies, especially culture-based methods (29). Finally, the anticipated benefits of PRT should be carefully evaluated before making a decision (49).
Various factors, including the timing of treatment (50) and the entity of bacteria present, such as growth speed (fast-growing vs. slow-growing) (51) or sporulation, can directly affect the effectiveness of PRT. If PR is delayed after blood collection, bacteria may grow and reach high levels, potentially breaking through the system function (52). For example, A study showed that the delay of treatment with Mirasol from 26 to 32 h post-WB collection affects the efficiency of PR for Klebsiella pneumoniae strains (53). Following PR, endospore bacteria such as Bacillus subtilis and Bacillus cereus showed PC growth and could be detected using RT-PCR and automated culture (54). Furthermore, post-manufacturing contamination of pathogen-reduced PLT may have been caused by environmental sources or inherent/acquired bag defects (55). In contrast to late bacterial culture, treating PC with PR methods early is essential to avoid high bacterial loads (29, 56). It has been suggested that in cases where PR cannot be performed immediately after preparation, a combination of PR technology with a rapid bacterial screen test on the 4th or 5th day after donation may offer a potential solution further to mitigate the risk of bacterial transmission by transfusion (56).
Since 1990, several techniques have been developed to reduce pathogens that may be chemically present in PCs. Table 2 provides an overview of the characteristics of three common PRTs that are compatible with platelets (2, 55). These technologies differ regarding the UV wavelengths, doses, energy levels, and photosensitive compounds employed (Figure 1). Typically, a lower wavelength accompanied by a higher energy level is more effective in causing damage to the pathogen cells. The three technologies are INTERCEPT, Mirasol, and THERAFLEX. In the case of the first two technologies, a photosensitive compound is added to the PCs before UV exposure. For THERAFLEX, short-wavelength UV is employed (23).
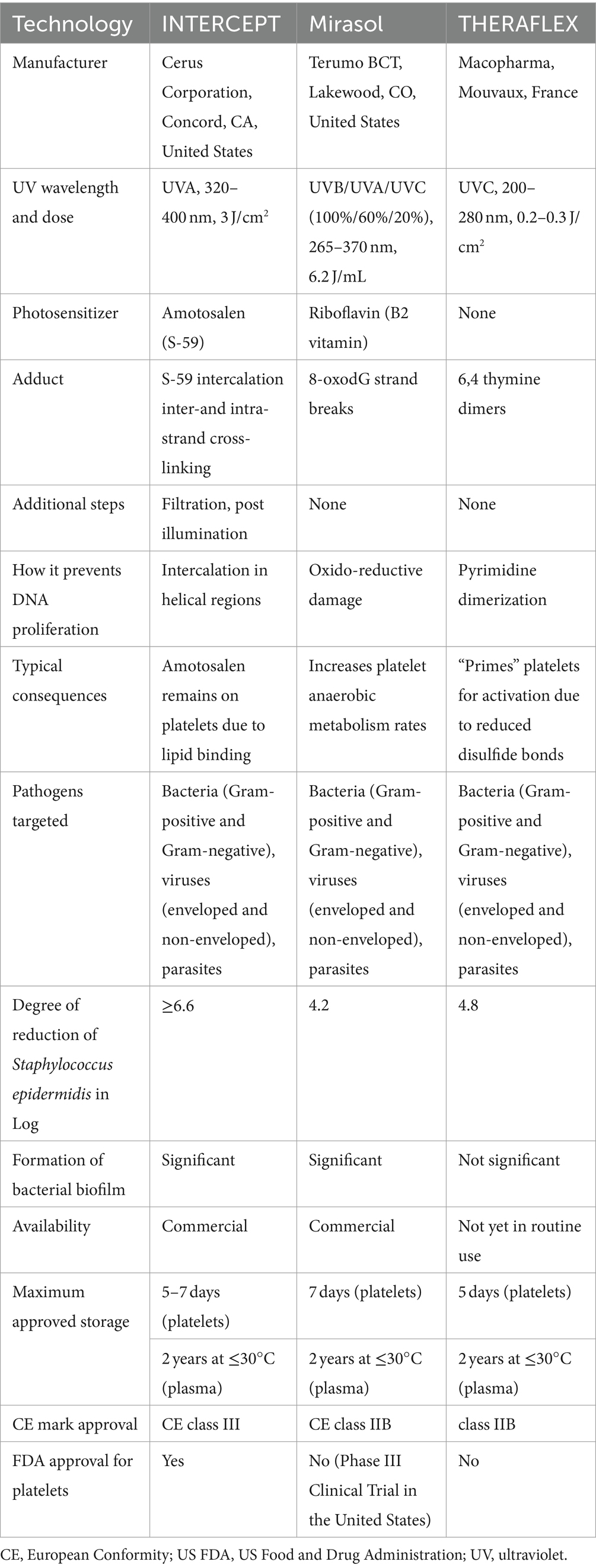
Table 2. An overview of the comparison of the features of three leading pathogen reduction technologies (PRTs) (2, 55, 57).
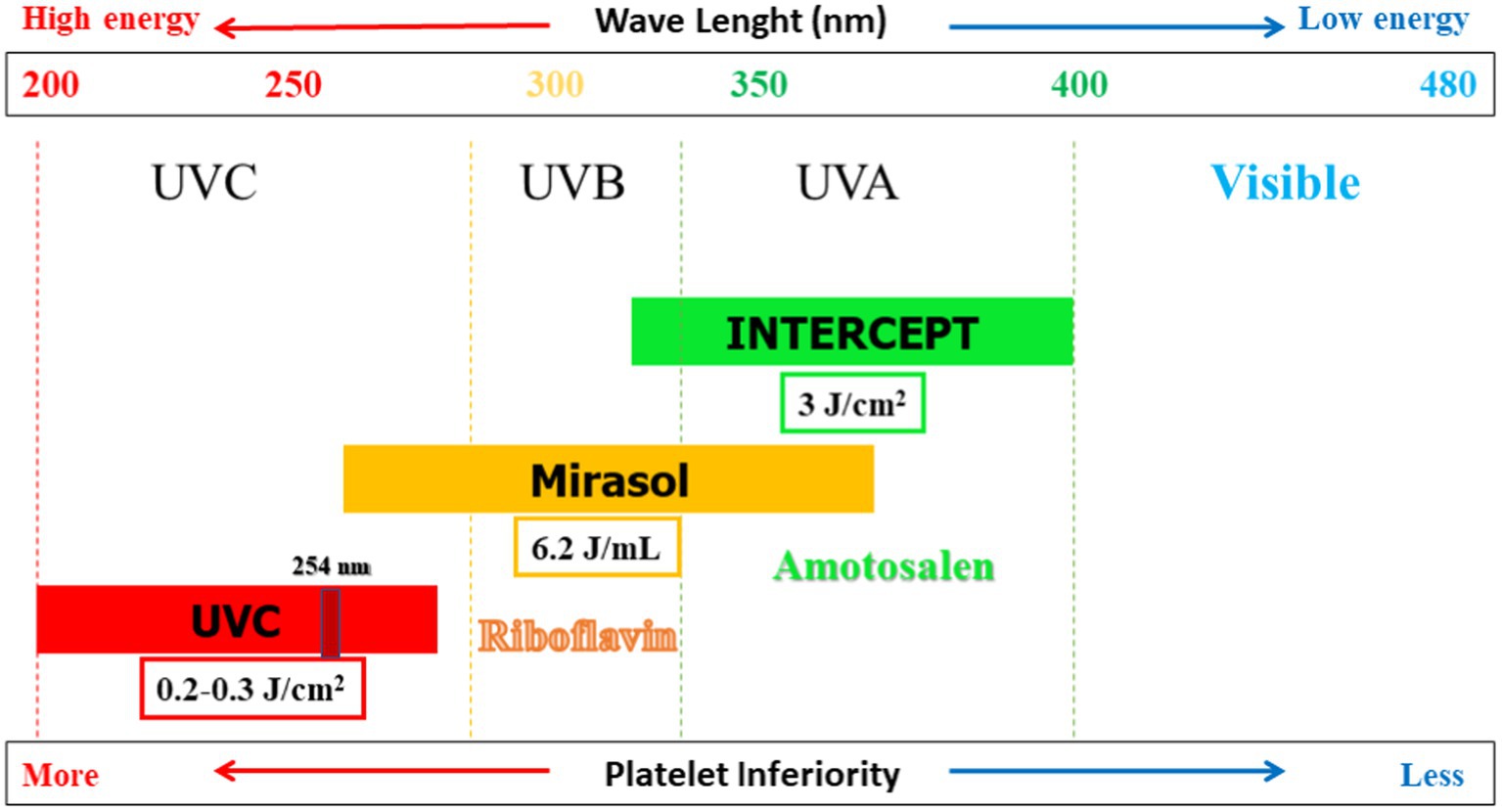
Figure 1. Pathogen reduction technologies (PRTs) compatible with platelet concentrates (PCs): Dose, wavelength, energy level, and photosensitizer are shown.
INTERCEPT technology, developed by Cerus Corporation based in Concord, CA, United States, has received FDA approval for its use of Amotosalen as a photosensitizing compound in the process of exposing PCs to ultraviolet A (UV A) light ranging from 320 to 400 nm. To minimize the potentially toxic effects, we have a process to remove residual Amotosalen safely. Any remaining Amotosalen (synthetic psoralen) is then safely removed through absorption. This process is typically completed within 24 h of platelet donation within the blood collection department (2, 55). The INTERCEPT system was not 100% effective for high concentrations of certain Klebsiella pneumoniae strains or spore-forming Bacillus cereus. Like other PR systems, this technology has limitations for fast-growing and spore-forming bacteria (56). Data has demonstrated that the use of INTERCEPT technology does not harm the metabolism or function of CS platelets (30). Using PCs containing triple sugar (TS) and platelet additive solution III or IIIM (PAS-III or PAS-IIIM) is relatively safe (58). INTERCEPT technology is more effective than Mirasol technology in maintaining the quality of platelets in vitro, and 7 days of storage are achieved based on quality criteria using INTERCEPT technology (59). However, there is also the possibility of microbial resistance (60).
In Mirasol technology (Terumo BCT, Lakewood, CO, United States), riboflavin (vitamin B2) is used as a light-sensitive compound, followed by ultraviolet B/A/C spectrum (265–370 nm). Unlike Amotosalen, there is no need to remove residual riboflavin. Its action mechanism strongly depends on reactive oxygen species, and guanine bases are selectively targeted. This technology, which received European conformity Class IIB (CE class IIB) in 2007, is used in over 20 countries (2, 55, 61). It is currently in a phase III clinical trial in the United States (47). In this technology, riboflavin is mixed with PC and then exposed to UV light for less than 10 min (62). Studies show the impact of this technology on different bacterial species (41). Based on plate count, the PR capability of the Intercept method is greater than that of the Mirasol method (63). Platelet loss level is significantly higher in the INTERCEPT units than in the Mirasol units. Additionally, the harmful impact and level of platelet loss are more elevated in WBPs than in single SDPs (59). However, this technology is only partially effective in deactivating biofilm-producing species such as Staphylococcus epidermidis (64).
The THERAFLEX technology, developed by Macopharma in Mouvaux, France, does not require a photosensitive compound for PCs. Instead, it uses short wavelength UV C light (254 nm) in PCs with agitation. This light gets absorbed by nucleic acids, forming pyrimidine dimers that stop nucleic acid transcripts’ elongation. 2009, this technology was awarded the European Conformity of class IIB (CE class IIB). Using a wavelength of 265 nm effectively inactivated bacteria, especially Staphylococcus aureus and Bacillus cereus (8). Several studies have assessed the impact and effectiveness of THERAFLEX technology in PCs (25). According to the available data, bacterial biofilm formation is not a significant concern in the context of the THERAFLEX UV-Platelets procedure. Proper pre-treatment with PI is crucial in this process, followed by the transfer of platelets to the illumination bag. Biofilms can provide a protective layer to encapsulated bacteria, making them less susceptible to inactivation by reducing the penetration of UV light or photochemical (64).
In conclusion, PRTs can be highly efficient in eliminating various pathogens. However, they may need to be more practical, completely inactivating pathogens in PCs. PRTs can impact platelets’ activation, function, and survival and may encounter Microbic resistance. The platelet additive solution (PAS) is a partial approach to solving them. Through extensive research, identifying and resolving preclinical and clinical shortcomings, and optimizing their advantages, PRTs will soon comprehensively surpass culture-based bacterial contamination detection methods. An important reason is that the maximum safety of PCs in using PRT has been shown in countries such as France, Switzerland, and Belgium compared to Germany, Italy, Bulgaria, and Poland without mandatory use (29). To promote widespread adoption, reinforcing preclinical and clinical safety standards of PRTs is imperative.
Author contributions
MR: Writing – original draft, Validation. AM: Writing – original draft. AS-N: Writing – original draft. ME: Writing – original draft, Supervision, Writing – review & editing.
Funding
The author(s) declare that no financial support was received for the research, authorship, and/or publication of this article.
Conflict of interest
The authors declare that the research was conducted in the absence of any commercial or financial relationships that could be construed as a potential conflict of interest.
Publisher’s note
All claims expressed in this article are solely those of the authors and do not necessarily represent those of their affiliated organizations, or those of the publisher, the editors and the reviewers. Any product that may be evaluated in this article, or claim that may be made by its manufacturer, is not guaranteed or endorsed by the publisher.
References
1. World Health Organization . Global status report on Blood safety and availability 2021. (2022). Available at: https://www.who.int/publications/i/item/9789240051683
2. Levy, JH, Neal, MD, and Herman, JH. Bacterial contamination of platelets for transfusion: strategies for prevention. Crit Care. (2018) 22:271–8. doi: 10.1186/s13054-018-2212-9
3. Jahromi, M, Yari, F, and Esmaeili, MA. Effect of platelet-derived microparticles on the production of igg antibody from human peripheral Blood B-lymphocytes. J Mazandaran Univ Med Sci. (2016) 25:267–76.
4. Yamket, W, Sathianpitayakul, P, Santanirand, P, and Ratthawongjirakul, P. Implementation of helicase-dependent amplification with Sybr Green I for prompt naked-eye detection of bacterial contaminants in platelet products. Sci Rep. (2023) 13:3238. doi: 10.1038/s41598-023-30410-8
5. Esmaili, MA, Razjou, F, Nazel Khosroshahi, B, Amini, A, Namjoo, S, and Rezvani, MR. Evaluations of detection methods of bacterial contamination in platelet components. Int J Med Lab. (2017) 4:232–45.
6. Störmer, M, and Vollmer, T. Diagnostic methods for platelet Bacteria screening: current status and developments. Transfus Med Hemother. (2014) 41:19–27. doi: 10.1159/000357651
7. Mellmann, A, Spindler-Raffel, E, Bletz, S, Prax, M, and Bekeredjian-Ding, I. Genome sequences of the first who repository of platelet transfusion-relevant bacterial reference strains. Genome Announc. (2017) 5:00001–17. doi: 10.1128/genomeA.00001-17
8. Hayashi, T, Oguma, K, Fujimura, Y, Furuta, RA, Tanaka, M, Masaki, M, et al. Uv light-emitting diode (Uv-led) at 265 nm as a potential light source for disinfecting human platelet concentrates. PLoS One. (2021) 16:e0251650. doi: 10.1371/journal.pone.0251650. eCollection 2021
9. Montag, T . Strategies of Bacteria screening in cellular Blood components. Clin Chem Lab Med. (2008) 46:926–32. doi: 10.1515/CCLM.2008.176
10. Escolar, G, Diaz-Ricart, M, and McCullough, J. Impact of different pathogen reduction technologies on the biochemistry, function, and clinical effectiveness of platelet concentrates: an updated view during a pandemic. Transfusion. (2022) 62:227–46. doi: 10.1111/trf.16747
11. George, CE, Saunders, CV, Morrison, A, Scorer, T, Jones, S, and Dempsey, NC. Cold stored platelets in the Management of Bleeding: is it about bioenergetics? Platelets. (2023) 34:2188969. doi: 10.1080/09537104.2023.2188969
12. Brecher, ME, and Hay, SN. Bacterial contamination of Blood components. Clin Microbiol Rev. (2005) 18:195–204. doi: 10.1128/CMR.18.1.195-204.2005
13. Amano, M, Matsumoto, M, Sano, S, Oyama, M, Nagumo, H, Watanabe-Okochi, N, et al. Characteristics of false-positive alarms in the Bact/Alert 3d system. Microbiol Spectr. (2022) 10:e00055–22. doi: 10.1128/spectrum.00055-22
14. Johnson, L, Vekariya, S, Tan, S, Padula, MP, and Marks, DC. Extended storage of thawed platelets: refrigeration supports Postthaw quality for 10 days. Transfusion. (2020) 60:2969–81. doi: 10.1111/trf.16127
15. Jimenez-Marco, T, Castrillo, A, Hierro-Riu, F, Vicente, V, and Rivera, J. Frozen and cold-stored platelets: reconsidered platelet products. Platelets. (2022) 33:27–34. doi: 10.1080/09537104.2021.1967917
16. Nair, PM, Pandya, SG, Dallo, SF, Reddoch, KM, Montgomery, RK, Pidcoke, HF, et al. Platelets stored at 4°C contribute to superior clot properties compared to current standard-of-care through fibrin-crosslinking. Br J Haematol. (2017) 178:119–29. doi: 10.1111/bjh.14751
17. van der Wal, DE, Du, VX, Lo, KS, Rasmussen, JT, Verhoef, S, and Akkerman, JW. Platelet apoptosis by cold-induced glycoprotein Ibα clustering. J Thromb Haemost. (2010) 8:2554–62. doi: 10.1111/j.1538-7836.2010.04043.x
18. Zhao, H, and Devine, DV. The missing pieces to the cold-stored platelet puzzle. Int J Mol Sci. (2022) 23:1100. doi: 10.3390/ijms23031100
19. Lu, J, Karkouti, K, Peer, M, Englesakis, M, Spinella, PC, Apelseth, TO, et al. Cold-stored platelets for acute bleeding in cardiac surgical patients: a narrative review. Can J Anaesth. (2023) 70:1682–700. doi: 10.1007/s12630-023-02561-9
20. Mack, JP, Miles, J, and Stolla, M. Cold-stored platelets: review of studies in humans. Transfus Med Rev. (2020) 34:221–6. doi: 10.1016/j.tmrv.2020.08.003
21. Blood, ST . Fda Grants South Texas Blood and Tissue Center First License for new process that triples shelf life of critically needed platelets. (2020). Available at: https://www.globenewswire.com/news-release/2020/02/28/1992715/0/en/FDA-grants-South-Texas-Blood-Tissue-Center-first-license-for-new-process-that-triples-shelf-life-of-critically-needed-platelets.html
22. Grossman, B, Kollins, P, Lau, P, Perreten, J, Bowman, R, Malcolm, S, et al. Screening Blood donors for gastrointestinal illness: a strategy to eliminate carriers of Yersinia Enterocolitica. Transfusion. (1991) 31:500–1. doi: 10.1046/j.1537-2995.1991.31691306245.x
23. Atreya, C, Glynn, S, Busch, M, Kleinman, S, Snyder, E, Rutter, S, et al. Proceedings of the Food and Drug Administration public workshop on pathogen reduction Technologies for Blood Safety 2018 (commentary, P. 3026). Transfusion. (2019) 59:3002. doi: 10.1111/trf.15344
24. de Korte, D, and Marcelis, JH. Platelet concentrates: reducing the risk of transfusion-transmitted bacterial infections. Int J Clin Transfus Med. (2014) 2:29–37. doi: 10.2147/IJCTM.S40037
25. Tynngård, N, Trinks, M, and Berlin, G. In vitro function of platelets treated with ultraviolet C light for pathogen inactivation: a comparative study with nonirradiated and gamma-irradiated platelets. Transfusion. (2015) 55:1169–77. doi: 10.1111/trf.12963
26. Bashir, S, Cookson, P, Wiltshire, M, Hawkins, L, Sonoda, L, Thomas, S, et al. Pathogen inactivation of platelets using ultraviolet C light: effect on in vitro function and recovery and survival of platelets. Transfusion. (2013) 53:990–1000. doi: 10.1111/j.1537-2995.2012.03854.x
27. Brixner, V, Bug, G, Pohler, P, Krämer, D, Metzner, B, Voss, A, et al. Efficacy of Uvc-treated, pathogen-reduced platelets versus untreated platelets: a randomized controlled non-inferiority trial. Haematologica. (2021) 106:1086–96. doi: 10.3324/haematol.2020.260430
28. Pati, I, Masiello, F, Pupella, S, Cruciani, M, and De Angelis, V. Efficacy and safety of pathogen-reduced platelets compared with standard apheresis platelets: a systematic review of Rcts. Pathogens. (2022) 11:639. doi: 10.3390/pathogens11060639
29. Prax, M, Bekeredjian-Ding, I, and Krut, O. Microbiological screening of platelet concentrates in Europe. Transfus Med Hemother. (2019) 46:76–86. doi: 10.1159/000499349
30. Ramirez-Arcos, S, DiFranco, C, McIntyre, T, and Goldman, M. Residual risk of bacterial contamination of platelets: six years of experience with sterility testing. Transfusion. (2017) 57:2174–81. doi: 10.1111/trf.14202
31. Schmidt, M, Ramirez-Arcos, S, Stiller, L, and McDonald, C. ISBT transfusion-transmitted infectious diseases working party SoB. Current status of rapid bacterial detection methods for platelet components: a 20-year review by the Isbt transfusion-transmitted infectious diseases working party subgroup on Bacteria. Vox Sang. (2022) 117:983–8. doi: 10.1111/vox.13283
32. Vollmer, T, Kleesiek, K, and Dreier, J. Detection of bacterial contamination in platelet concentrates using flow cytometry and real-time Pcr methods. PCR Detect Microb Pathog. (2013) 28:91–103. doi: 10.1007/978-1-60327-353-4_5
33. Yomtovian, R, Lazarus, H, Goodnough, L, Hirschler, N, Morrissey, A, and Jacobs, M. A prospective microbiologic surveillance program to detect and prevent the transfusion of bacterially contaminated platelets. Transfusion. (1993) 33:902–9. doi: 10.1046/j.1537-2995.1993.331194082380.x
34. Yomtovian, RA, Palavecino, EL, Dysktra, AH, Downes, KA, Morrissey, AM, Bajaksouzian, S, et al. Evolution of surveillance methods for detection of bacterial contamination of platelets in a university hospital, 1991 through 2004. Transfusion. (2006) 46:719–30. doi: 10.1111/j.1537-2995.2006.00790.x
35. Razjou, F, Naghadeh, HT, Ferdowsi, S, and Dabirmoghadam, A. Evaluation of the sensitivity and specificity of use of glucose and Ph for bacterial screening of platelet concentrates compared to the Bact/Alert. Indian J Hematol Blood Transfus. (2017) 33:116–20. doi: 10.1007/s12288-016-0660-4
36. Gamerith, C, Heinzle, A, Schneider, KP, Hulla-Gumbsch, E, Gewessler, U, Ducoroy, L, et al. Bioresponsive polymers for the detection of bacterial contaminations in platelet concentrates. New Biotechnol. (2014) 31:150–5. doi: 10.1016/j.nbt.2013.11.001. Epub 2013 Nov 17
37. Satake, M, Kozakai, M, Matsumoto, M, Matsubayashi, K, Taira, R, and Goto, N. Platelet safety strategies in Japan: impact of short shelf life on the incidence of septic reactions. Transfusion. (2020) 60:731–8. doi: 10.1111/trf.15733
38. Murphy, W, Foley, M, Doherty, C, Tierney, G, Kinsella, A, Salami, A, et al. Screening platelet concentrates for bacterial contamination: low numbers of Bacteria and slow growth in contaminated units mandate an alternative approach to product safety. Vox Sang. (2008) 95:13–9. doi: 10.1111/j.1423-0410.2008.01051.x
39. Ezuki, S, Kawabata, K, Kanno, T, and Ohto, H. Culture-based bacterial detection Systems for Platelets: the effect of time prior to sampling and duration of incubation required for detection with aerobic culture. Transfusion. (2007) 47:2044–9. doi: 10.1111/j.1537-2995.2007.01428.x
40. Hosseini, E, Kianinodeh, F, and Ghasemzadeh, M. Irradiation of platelets in transfusion medicine: risk and benefit judgments. Platelets. (2022) 33:666–78. doi: 10.1080/09537104.2021.1990250
41. Marschner, S, and Goodrich, R. Pathogen reduction technology treatment of platelets, plasma and whole Blood using riboflavin and Uv light. Transfus Med Hemother. (2011) 38:8–18. doi: 10.1159/000324160
42. Picker, SM . Current methods for the reduction of Blood-borne pathogens: a comprehensive literature review. Blood Transfus. (2013) 11:343–8. doi: 10.2450/2013.0218-12
43. van der Meer, PF, Ypma, PF, van Geloven, N, van Hilten, JA, van Wordragen-Vlaswinkel, RJ, Eissen, O, et al. Hemostatic efficacy of pathogen-inactivated vs untreated platelets: a randomized controlled trial. Blood. (2018) 132:223–31. doi: 10.1182/blood-2018-02-831289
44. Cid, J, Escolar, G, and Lozano, M. Therapeutic efficacy of platelet components treated with Amotosalen and ultraviolet a pathogen inactivation method: results of a Meta-analysis of randomized controlled trials. Vox Sang. (2012) 103:322–30. doi: 10.1111/j.1423-0410.2012.01614.x
45. Vamvakas, EC . Meta-analysis of the studies of bleeding complications of platelets pathogen-reduced with the Intercept system. Vox Sang. (2012) 102:302–16. doi: 10.1111/j.1423-0410.2011.01555.x
46. Infanti, L, Holbro, A, Passweg, J, Bolliger, D, Tsakiris, DA, Merki, R, et al. Clinical impact of Amotosalen-ultraviolet a pathogen-inactivated platelets stored for up to 7 days. Transfusion. (2019) 59:3350–61. doi: 10.1111/trf.15511
47. Lu, M, Dai, T, Hu, S, Zhang, Q, Bhayana, B, Wang, L, et al. Antimicrobial blue light for decontamination of platelets during storage. J Biophotonics. (2020) 13:e201960021. doi: 10.1002/jbio.201960021
48. Moeremans, K, Warie, H, and Annemans, L. Assessment of the economic value of the Intercept Blood system in Belgium. Transfus Med. (2006) 16:17–30. doi: 10.1111/j.1365-3148.2006.00644.x
49. Fachini, RM, Fontão-Wendel, R, Achkar, R, Scuracchio, P, Brito, M, Amaral, M, et al. The 4-year experience with implementation and routine use of pathogen reduction in a Brazilian hospital. Pathogens. (2021) 10:1499. doi: 10.3390/pathogens10111499
50. Taha, M, Kalab, M, Yi, QL, Maurer, E, Jenkins, C, Schubert, P, et al. Bacterial survival and distribution during Buffy coat platelet production. Vox Sang. (2016) 111:333–40. doi: 10.1111/vox.12427
51. Brecher, ME, Holland, PV, Pineda, AA, Tegtmeier, GE, and Yomtovian, R. Growth of Bacteria in inoculated platelets: implications for Bacteria detection and the extension of platelet storage. Transfusion. (2000) 40:1308–12. doi: 10.1046/j.1537-2995.2000.40111308.x
52. Benjamin, RJ, and Wagner, SJ. Bacterial pathogen reduction requires validation under conditions of intended use. Transfusion. (2015) 55:2060–3. doi: 10.1111/trf.13232
53. Alabdullatif, M, Osman, IE, Alrasheed, M, Ramirez-Arcos, S, Alyousef, M, Althawadi, S, et al. Evaluation of riboflavin and ultraviolet light treatment against Klebsiella Pneumoniae in whole Blood-derived platelets: a pilot study. Transfusion. (2021) 61:1562–9. doi: 10.1111/trf.16347
54. Störmer, M, Vollmer, T, Kleesiek, K, and Dreier, J. Spore-forming organisms in platelet concentrates: a challenge in transfusion bacterial safety. Transfus Med. (2008) 18:371–6. doi: 10.1111/j.1365-3148.2008.00895.x
55. Feys, HB, Van Aelst, B, and Compernolle, V. Biomolecular consequences of platelet pathogen inactivation methods. Transfus Med Rev. (2019) 33:29–34. doi: 10.1016/j.tmrv.2018.06.002
56. Schmidt, M, Hourfar, MK, Sireis, W, Pfeiffer, U, Göttig, S, Kempf, VA, et al. Evaluation of the effectiveness of a pathogen inactivation technology against clinically relevant transfusion-transmitted bacterial strains. Transfusion. (2015) 55:2104–12. doi: 10.1111/trf.13171
57. Gravemann, U, Handke, W, Müller, TH, and Seltsam, A. Bacterial inactivation of platelet concentrates with the Theraflex Uv-platelets pathogen inactivation system. Transfusion. (2019) 59:1324–32. doi: 10.1111/trf.15119
58. Infanti, L, Pehlic, V, Mitrovic, S, Holbro, A, Andresen, S, Payrat, JM, et al. Pathogen inactivation treatment of triple-dose apheresis platelets with Amotosalen and ultraviolet a light. Transfus Med. (2022) 32:505–11. doi: 10.1111/tme.12913
59. Malvaux, N, Defraigne, F, Bartziali, S, Bellora, C, Mommaerts, K, Betsou, F, et al. In vitro comparative study of platelets treated with two pathogen-inactivation methods to extend shelf life to 7 days. Pathogens. (2022) 11:343. doi: 10.3390/pathogens11030343
60. Green, AB, Chiaraviglio, L, Truelson, KA, Zulauf, KE, Cui, M, Zhang, Z, et al. Rnd pump-mediated efflux of Amotosalen, a compound used in pathogen inactivation technology to enhance safety of Blood transfusion products, may compromise its gram-negative anti-bacterial activity. Msphere. (2023) 8:e00673–22. doi: 10.1128/msphere.00673-22
61. Goodrich, R . The use of riboflavin for the inactivation of pathogens in Blood products. Vox Sang. (2000) 78:211–5.
62. Valsami, S, Grouzi, E, Mochandreou, D, Pouliakis, A, Piroula-Godoy, M, Kokori, S, et al. Effect of Mirasol pathogen reduction technology system on immunomodulatory molecules of apheresis platelets. Transfus Apher Sci. (2023) 62:103523. doi: 10.1016/j.transci.2022.103523
63. McDonald, CP, Bearne, J, Aplin, K, and Sawicka, D. Assessing the inactivation capabilities of two commercially available platelet component pathogen inactivation systems: effectiveness at end of shelf life. Vox Sang. (2021) 116:416–24. doi: 10.1111/vox.13040
64. Taha, M, Culibrk, B, Kalab, M, Schubert, P, Yi, QL, Goodrich, R, et al. Efficiency of riboflavin and ultraviolet light treatment against high levels of biofilm-derived Staphylococcus Epidermidis in Buffy coat platelet concentrates. Vox Sang. (2017) 112:408–16. doi: 10.1111/vox.12519. Epub 2017 Apr 5
Keywords: platelet transfusion, the safety of platelet products, prevention strategies, pathogen reduction systems, bacterial contamination screening
Citation: Rezvany MR, Moradi Hasan-Abad A, Sobhani-Nasab A and Esmaili MA (2024) Evaluation of bacterial safety approaches of platelet blood concentrates: bacterial screening and pathogen reduction. Front. Med. 11:1325602. doi: 10.3389/fmed.2024.1325602
Edited by:
Mehran Ghasemzadeh, Blood Transfusion Research Center, High Institute for Research and Education in Transfusion Medicine, IranReviewed by:
Ehteramolsadat Hosseini, Blood Transfusion Research Center, High Institute for Research and Education in Transfusion Medicine, IranSilvano Wendel, Hospital Sirio Libanes, Brazil
Copyright © 2024 Rezvany, Moradi Hasan-Abad, Sobhani-Nasab and Esmaili. This is an open-access article distributed under the terms of the Creative Commons Attribution License (CC BY). The use, distribution or reproduction in other forums is permitted, provided the original author(s) and the copyright owner(s) are credited and that the original publication in this journal is cited, in accordance with accepted academic practice. No use, distribution or reproduction is permitted which does not comply with these terms.
*Correspondence: Mohammad Ali Esmaili, esmailionline@gmail.com; esmailimohammadali@yahoo.com
†ORCID: Mohammad Ali Esmaili, https://orcid.org/0000-0001-6851-7872