- 1Hunan Provincial Key Laboratory for Biology and Control of Plant Pests, College of Plant Protection, Hunan Agricultural University, Changsha, China
- 2College of Life Science and Technology, Beijing University of Chemical Technology, Beijing, China
The Asian citrus psyllid (ACP), Diaphorina citri (Kuwayama) (Hemiptera: Liviidae), is a notorious Rutaceae plant pest. Frequent and extensive use of pesticides has resulted in severe insecticide resistance in ACP populations. Fully understanding the mechanism of ACP resistance to pesticides is vital for us to control or delay the development of resistance. Therefore, we compared the difference in resistance to imidacloprid and thiamethoxam between Hunan (Yongzhou, Chenzhou) and Guangdong (Guangzhou) ACP populations and analyzed the correlations between the resistance level and genes and symbiotic fungi. The results showed that the resistance of the Guangdong ACP population to imidacloprid and thiamethoxam was lower than that of Hunan ACP population, and the relative expression of genes associated with P450 mono-oxygenase and acetylcholinesterase was significantly lower in the Guangdong ACP population than in Hunan ACP population. The differences of mean relative abundances of four symbiotic bacteria among three populations were marginally significant; however, the mean relative abundance of 16 fungi among three populations was significantly different, and positive linear correlations were observed between the resistance level and two fungi (Aspergillus niger and Aureobasidium pullulans) and two genes (CYP4C70 and CYP4DB1). Negative correlations were only observed between the resistance level and two fungi (Golubevia pallescens and Acremonium sclerotigenum). Moreover, four fungi were unique to the Chenzhou population which was the highest resistance to imidacloprid and thiamethoxam. These findings suggested the P450 mono-oxygenase and symbiotic fungi together affected ACP resistance to imidacloprid and thiamethoxam. In the future, we may use environmental G. pallescens and A. sclerotigenum to control or delay ACP resistance.
Introduction
Chemical control is the most important and widely used measure in controlling most agricultural pests (Dang et al., 2017). The resistance level of pests is increasing with the intensive and frequent use of insecticides, reducing the efficiency of pesticides, increasing costs in the agricultural industry, and causing serious environmental pollution and potential risks to human health. Fully understanding the mechanisms of pesticide resistance is vital for us to control or delay the development of resistance. Therefore, the analysis of metabolic enzyme activity, application of insecticide synergists, and expression of genes have been used to explore the mechanisms of pesticide resistance. Wu et al. (2018) found that the CYP6ER1 gene in Drosophila melanogaster conferred resistance to imidacloprid, thiamethoxam, and buprofezin. Garrood et al. (2016) found that the CYP6ER1 gene provided the brown planthopper (BPH) (Nilaparvata lugens) resistance to imidacloprid and ethiprole. Increased activity and reduced sensitivity of acetylcholinesterase affected oriental migratory locust resistance to the organophosphate insecticide malathion (Yang et al., 2009). Liu et al. (2005) confirmed that nicotinic acetylcholine receptor (nAChR) genes, as targets of neonicotinoid insecticides, affected BPH resistance to imidacloprid. Overall, most studies on insecticide resistance mechanisms have focused on evolutionary changes in pest insect genomes, such as the alteration of pesticide target sites, increase in pesticide excretion rates, and upregulation of ATP binding protein and metabolic enzymes, including general esterase, glutathione S-transferase, and cytochrome P450 mono-oxygenase (Cao et al., 2008; Kikuchi et al., 2012; Wei et al., 2015; Safi et al., 2017; Tian et al., 2018; Wang et al., 2019).
In fact, insects are multiorganismal symbionts and harbor many microbes that also mediate insecticide resistance (Douglas, 2015). An early study found that Pseudomonas melophthora, an obligate symbiont, helped an insect host (apple maggot, Rhagoletis pomonella) degrade 6 insecticides (Boush and Matsumura, 1967). The densities of symbiotic bacteria Arsenophonus, Rickettsia, and Wolbachia from the Q biotype whitefly associated with the host’s susceptibility to thiamethoxam, imidacloprid, pyriproxyfen, and spiromesifen (Ghanim and Kontsedalov, 2009). The symbiotic bacteria Burkholderia mediated the resistance of its insect host, Riptortus pedestris, to the organophosphate pesticide fenitrothion by degrading the pesticide (Kikuchi et al., 2012). Cheng et al. (2017) found that the gut symbiont Citrobacter sp. helps the host insect fruit fly Bactrocera dorsalis degrade the organophosphate insecticide trichlorphon. Pang et al. (2018) found that Arsenophonus strains (S-type Arsenophonus) in the BPH negatively affected the insecticide resistance of the host by downregulating xenobiotic metabolism and increasing amino acid accumulation.
In addition to the many studies focused on bacteria, the degradation of pesticides by fungi has also been explored. Marecik et al. (2008) found that Volutella ciliata degraded atrazine in soil. Cladosporium cladosporioides displayed maximum degradation of chlorpyrifos in soil (Bisht et al., 2019). The symbiotic fungus (Candida lipolytica) of the BPH (N. lugens) participated in resistance to imidacloprid (Li et al., 2010). Symbiotic yeast (Symbiotaphrina kochii) helps host cigarette beetles (Lasioderma serricorne) resist toxins (Dowd and Shen, 2011). Aspergillus fumigatus plays an important role in azole resistance (Howard et al., 2006). Aspergillus flavus can produce terreulactone with antiacetylcholinesterase activity and phosphohydrolases to help pest host detoxification to pesticides (Rudramurthy et al., 2019). Moreover, a previous study showed that Thysanophora penicillioides and Dothideomycetes affected the sensitivity of the Q biotype whitefly to Beauveria bassiana (a biological pesticide) (Hong et al., 2016). Therefore, microbes can influence host insect resistance to pesticides.
The Asian citrus psyllid (ACP), Diaphorina citri (Kuwayama) (Hemiptera: Liviidae), is a notorious Rutaceae plant pest. The ACP, a phloem-sap feeding pest, directly causes nutrient depletion within the plant, affecting the growth and death of young foliage at high population densities (Gottwald, 2010). Furthermore, ACP secretes honeydew, reducing photosynthesis and resulting in sooty mold (Grafton-Cardwell et al., 2013). Moreover, ACP is a natural vector of Candidatus Liberibacter asiaticus (CLas) and Candidatus Liberibacter americanus (CLam), which result in one of the most devastating citrus diseases worldwide, Huanglongbing (HLB) (Duan et al., 2009). HLB causes small and bitter fruits, blotchy mottling, twig dieback, poor root growth, and, ultimately, plant death within 5 years. This disease has resulted in severe economic damage to the Florida citrus industry, with up to $8.9 billion/year in damage since 2006 (Eason et al., 2018). Currently, chemical control is the most effective measure to manage the ACP and HLB because there is a lack of HLB-resistant cultivars. However, the frequent and extensive use of pesticides has also resulted in insecticide resistance in ACP populations. Tiwari et al. (2011) reported that ACP populations from Florida have a high level of resistance to imidacloprid. However, Kanga et al. (2015) showed that ACP populations from Florida in the United States have no resistance to imidacloprid. Pardo et al. (2018) showed that the insecticide resistance of adults and nymphs from ACP populations was significantly different. Tian et al. (2018) found that ACP populations from Guangdong in China have a high level of resistance to imidacloprid. This result indicated that ACP populations from different locations had different resistance levels to the same insecticide, which may result from different resistance mechanisms. Wang et al. (2019) found that the ABC (ATP-binding cassette) transporters of the ACP may affect resistance to imidacloprid. Whether there is a difference in resistance to imidacloprid and thiamethoxam between different ACP populations is unclear. Whether genes associated with acetylcholinesterase and cytochrome P450 mono-oxygenase affected the resistance of ACP from populations in different geographical areas is also unclear.
The ACP harbors the primary endosymbionts Candidatus Carsonella ruddii and Candidatus Profftella armature, located on the surface and inside of the bacteriome, respectively (Nakabachi et al., 2013). The ACP also harbors secondary symbionts (Rickettsia, Wolbachia, and Arsenophonus), which are located in organs other than the bacteriome (Subandiyah et al., 2000; Saha et al., 2012; Nakabachi et al., 2020). Studies have suggested that Profftella and Wolbachia affect the acquisition and transmission of Candidatus Liberibacter spp. through the toll-signaling pathway, which regulates immunity and resistance (Ramsey et al., 2015; Zhang et al., 2016; Ren et al., 2018). Gandarilla-Pacheco et al. (2013) suggested B. bassiana and Isaria fumosorosea could be used to biocontrol to ACP in México. However, whether symbiotic bacteria and fungi affect the resistance of the ACP to insecticides is unclear.
In this study, we hypothesized that there are differences in the insecticide resistance level of ACP populations from Hunan and Guangdong to imidacloprid and thiamethoxam and that the resistance differences were affected by the expression of genes associated with cytochrome P450 mono-oxygenase or acetylcholinesterase or the microbial community, either separately or together. To test this hypothesis, we determined (1) the resistance level of A from Hunan and Guangdong to imidacloprid and thiamethoxam, (2) the expression of genes associated with cytochrome P450 mono-oxygenase (CYP4C67, CYP4C68, CYP4G70, CYP4DA1, and CYP4DB1) and acetylcholine esterase (AChE -1–like and ChE-2–like, ACH1 and ACH2), and (3) the composition and abundance of the symbiotic bacteria and fungi associated with the ACP.
Materials and Methods
Insecticides and Insect Collections
In this study, three geographically distinct populations were collected using aspirators. Two populations were collected from commercial citrus orchards located in Jiangyong County of Yongzhou city (111.33E-25.28N) and Yongxing County of Chenzhou city (113.10E-26.13N) in Hunan Province. The third ACP population came from a commercial Murraya paniculata Jack grove located in the Xinfang village of the Liwan district, Guangzhou city (113.23E, 23.16N), Guangdong Province (Figure 1). To enlarge the populations and ensure that all the ACPs were maintained under the same conditions, field ACP populations were cultured for one generation on M. paniculata Jack in an air-conditioned glasshouse maintained at 28°C ± 2°C and 70% ± 10% RH under natural light. Technical-grade samples (>98% purity) of imidacloprid and thiamethoxam were purchased from Shanghai Focus Trade Co., Ltd. (Shanghai) and Zhejiang Haizheng Chemical Co., Ltd. (Taizhou), respectively.
Assessment of ACP Resistance to Insecticides
To compare the difference in resistance between the Hunan and Guangdong ACP populations, the previously described glass vial bioassay technique was used to detect the resistance of ACP to imidacloprid and thiamethoxam (Kanga et al., 2015). Different concentrations of insecticides dissolved in acetone were used to treat the glass scintillation vials (10 mL), and young leaves were soaked for 30 s and dried naturally. Control vials were treated with acetone only. The concentration of imidacloprid was diluted from 80 mg/L to 1.25 mg/L (and 0.625–40 mg/L used in Guangzhou ACP), and the concentration of thiamethoxam was diluted from 80 mg/L to 1.25 mg/L according to 1:2 ratio (twofold). The concentrations were based on preparative experiment. Ten ACPs were placed in each treatment and control vial with fresh young leaves with and without insecticides, respectively, and held at room temperature (28°C ± 1°C and 70% RH; a vial served as a replicate for each concentration tested). Three biological replicates and three technical replicates were performed for each concentration of each insecticide in the study. In total, 1,440 (3 × 3 × 10 × 8 × 2) ACPs were used in the bioassays. Mortality was determined and recorded 24 h after exposure. ACPs unable to walk (>5 mm) after probing with a fine brush were recorded as dead. The resistance difference among the populations from different geographic locations was determined by the resistance ratio (RR) based on the median lethal concentrations (LC50) of the highly resistant population divided by the LC50 of the low-resistance population. The virulence regression equation, LC50, were estimated and the χ2 test was performed using DPS software.
Relative Gene Expression Associated With Resistance to Insecticides
To explore whether changes in gene expression altered ACP resistance to insecticides, we determined the ACP mRNA levels of the genes associated with cytochrome P450 mono-oxygenase (CYP4C67, CYP4C68, CYP4DA1, CYP4DB1, and CYP4G70) and acetylcholine esterase [AChE-1–like (ACH1) and ChE-2-like (ACH2)]. Approximately 100 live ACP individuals/population were used to extract total RNA and to synthesize cDNA at the same time as the bioassay, and resistance was assayed. To further verify the functions of P450 mono-oxygenase in ACP resistance to insecticides, total RNA was extracted, from ACP treated with imidacloprid (LC50) after 12, 24, and 48 h. Extraction was performed using Trizol according to the manufacturer’s protocols. The RNA was quantified by a NanoDrop 2000 spectrophotometer (ND 2000) (NanoDrop Technologies Inc., Wilmington, DE, United States). Fifty nanograms of total RNA in a 20-μL volume was used for cDNA synthesis with a transcriptor first-strand cDNA synthesis kit (Trans Gen Biotech, Beijing, China) according to the manufacturer’s protocols. To obtain reliable normalization of the reverse transcriptase–quantitative polymerase chain reaction (RT-qPCR) data, threefold diluted cDNA templates were used to verify each of the primer pairs to find the optimal concentration range for the RT-qPCRs (Hong et al., 2017, 2019). The optimal cDNA concentration was used in each of the RT-qPCR mixtures (10 μL), and three randomly selected optimal cDNA concentrations were used to validate the stability of the housekeeping gene (actin). RT-qPCRs were performed using SYBR Green I (Trans Gen Biotech, Beijing, China) and Bio-Rad software (Bio-Rad, United States). The thermal cycling conditions were as follows: 95°C for 2 min followed by 40 cycles of 95°C for 15 s and Tm temperature for 30 s; this was followed by a dissociation curve analysis, with a ramp-up from 65°C to 95°C and a read every 0.5°C. Ten biological replicates with three technical replicates were performed. The relative quantification of RNA was performed using the Livak method (2–ΔCT) (Livak and Schmittgen, 2001), and the values obtained for each mRNA were normalized to the ACP Actin. The linear relationship between RR50 values and the relative expression of the genes was analyzed.
The Composition and Abundance of the Microbial Communities
To explore whether changes in the microbial communities affected ACP resistance to insecticides, we determined the composition and abundance of the bacterial and fungal communities from Hunan and Guangdong provinces by 16S and ITS sequencing. Approximately 30 live ACPs/population/time were frozen for 3–5 min (0°C), soaked in 75% (vol:vol) ethanol for 2–3 min, washed three to five times, and used to extract the genomic DNA of the microbe using an insect DNA extraction kit (Mobio, Carlsbad, CA, United States). Sterile water was used as negative controls in the extracted DNA. The quality and concentration of the purified DNA were assessed by an ND 2000 spectrophotometer. The bacterial universal primers 338F (5′-ACTCCTACGGGAGGCAGCA-3′) and 806R (5′-GGACTACHVG GGTWTCTAAT-3′) were used to amplify the V3–V4 region of the bacterial 16S rRNA (Caporaso et al., 2011). The fungal universal primers ITS3-2024 (5′-GCATCGATGA AGAACGCAGC-3′) and ITS4-2409 (5′-TCCTCCGCTTATTGATATGC-3′) were used to amplify the ITS 3/4 region of the fungal ribosomal locus (White et al., 1990). The PCR amplifications were conducted in a 20-μL mixture containing 4 μL of 5 × Fast-Pfu buffer, 2 μL of 2.5 mM dNTPs, 0.8 μL of each primer (5 μM), 0.4 μL of Fast-Pfu polymerase, and 10 ng of template DNA, for which the barcode is an eight-base sequence unique to each sample. The PCR reactions were performed in triplicate (95°C for 2 min followed by 25 cycles at 95°C for 30 s, 55°C for 30 s, and 72°C for 30 s with a final extension at 72°C for 5 min). Amplicons were extracted from 2% agarose gels and purified using an AxyPrep DNA Gel Extraction Kit (Axygen Biosciences, Union City, CA, United States) according to the manufacturer’s instructions and quantified using QuantiFluorTM-ST (Promega, United States). The purified amplicons were pooled in equimolar and paired-end sequenced on an Illumina MiSeq PE300 platform according to standard protocols. Raw Fastq files were demultiplexed and quality-filtered using QIIME (version 1.17). The sequencing reads were assigned to each sample according to the unique barcode of each sample, and pairs of reads from the original DNA fragments were merged using FLASH (Martin, 2011). Reads that could not be assembled were discarded, and the number of sequences in each sample was greater than 10,000. Operational taxonomic units (OTUs) were clustered with a 97% similarity cutoff using UPARSE (version 7.1), and chimeric sequences were identified and removed using UCHIME. The taxonomy of each 16S rRNA gene sequence was analyzed by RDP Classifier against the SILVA (SSU115) 16S rRNA database using a confidence threshold that sequences with ≥97% similarity are assigned to the same species, whereas those with ≥95% similarity are assigned to the same genus (Breglia et al., 2010). For each representative sequence from ITS, the Unite Database1 was used basing on BLAST algorithm, which was calculated by QIIME software (version 1.9.1)2 to annotate taxonomic information using a confidence that sequences with ≥97% similarity are assigned to the same species, whereas those with ≥95% similarity are assigned to the same genus (Breglia et al., 2010). OTU abundance information was normalized using a standard of sequence number corresponding to the sample with the least sequences. The relative abundance was the number of species sequences divided by the total sequences in a sample. The demultiplexed sequence data have been deposited in the National Center for Biotechnology Information3 (16s accession PRJNA646487 and ITS accession: PRJNA646485). In this study, 15 microbial samples from three geographical populations (five samples/population) were used to analyze the composition and abundance of the bacterial and fungal communities. We evaluated the differences in the relative abundances of the different geographic populations based on OTUs and species and genus after removing some microbiota that appeared only once and in only one sample. The linear relationship between the RR50 value and the mean relative abundance of fungi and the linear relationship between the mean relative abundance of fungi and the mean relative expression of genes were analyzed. To further verify fungi function, the species classification tree based on the top 20 species with the largest relative abundance was constructed. Finally, in the discussion, we inferred the ecological function of the significant fungi based on Fun-Guild and published literatures on fungi detoxification (Luan et al., 2012, 2017; Selvam et al., 2013; Fattahi et al., 2014; Nguyen et al., 2016).
Statistical Analyses
The percentage of mortality in the treatments was corrected for control mortality by using Abbott’s formula (Abbott, 1925). The virulence regression equation and median lethal concentrations (LC50) were estimated, and the χ2 test was performed using DPS software. The RR was determined as the LC50 of the high-resistance population (Chenzhou or Yongzhou) divided by that of the population with the lowest resistance (Guangzhou). Multivariate analysis and, specifically, a general linear model were used to analyze the relative expression of genes and the relative abundances of the microbial communities; the level of significance was set at P < 0.05. The model fit was based on the mean value and the standard deviation (SD) of the corresponding element. The statistical analyses were conducted using SPSS 21.0 (IBM, United States). To further analyze the link between the ACP population resistance (RR50) to imidacloprid and the microbiota abundance and gene expression, we established several linear regression equations and determined their correlation coefficients.
Results
Assessment of ACP Resistance to Insecticides
The resistance of the ACP to thiamethoxam and imidacloprid appeared to be significantly different among the three geographical populations based on LC50 and LC95. Regardless of insecticide type (i.e., either thiamethoxam or imidacloprid), at a given concentration, the ACP population from Guangdong Province has the highest probability of mortality and the lowest resistance to thiamethoxam or imidacloprid. The value of the RR for thiamethoxam between the Chenzhou ACP population and Guangzhou ACP population was up to 3.85 for the RR50. The value of the RR for thiamethoxam between the Yongzhou ACP population and Guangdong ACP population was 2.18 for the RR50. Similarly, the level of resistance of the Hunan ACP populations (Chenzhou and Yongzhou) to imidacloprid was significantly higher than that of the Guangdong ACP population (Table 1 and Figure 2A). And the levels of resistance of Yongzhou and Chenzhou ACP populations to thiamethoxam at LC50 were higher than that to imidacloprid (Table 1 and Figure 2).
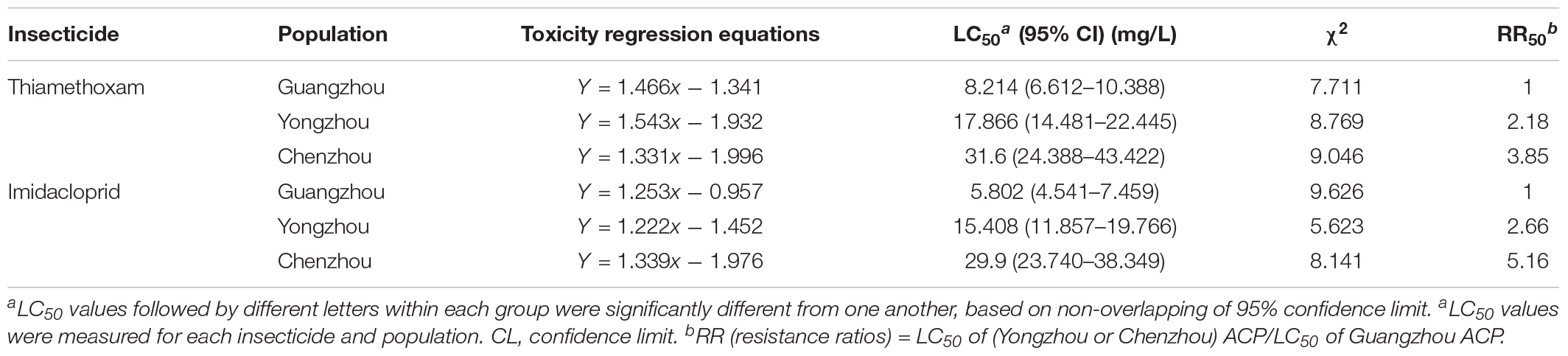
Table 1. Toxicity regression equations and χ2 test and relative parameter of the logarithm of pesticides concentration to probability of death of different ACP populations exposed to different insecticides.
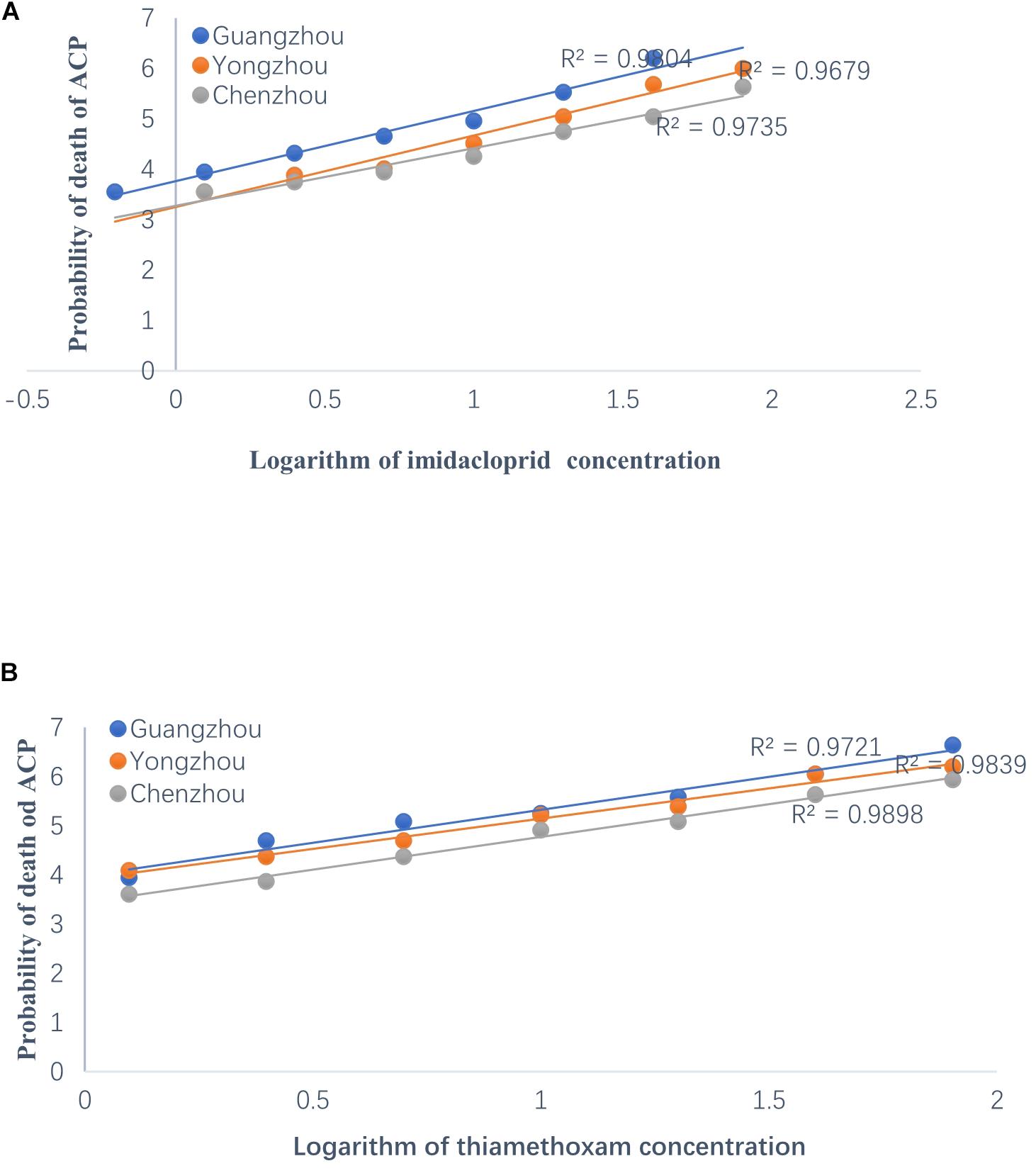
Figure 2. Regression analysis on the logarithm of concentration of insecticides to the probability of death of ACP from Guangzhou, Yongzhou, and Chenzhou. Y-axis was the probability of death, which was subjected to probit transformation based on mortality (according to conversion table of biostatistics probability value). The median lethal concentrations (LC50) were estimated using DPS software. (A) Regression analysis on the logarithm of imidacloprid concentration to the probability of death of ACP. The dilution concentration of imidacloprid was from 40 mg/L to 0.625 mg/L according to 1:2 ratio and the X-axis was –0.204, 0.097, 0.398, 0.699, 1, 1.301, 1.602, and 1.903 (logarithmic of imidacloprid concentration). (B) Regression analysis on the logarithm of thiamethoxam concentration to the probability of death value of ACP. The dilution concentration of thiamethoxam was from 80 mg/L to 1.25 mg/L according to 1:2 ratio, and the X-axis was 0.097, 0.398, 0.699, 1, 1.301, 1.602, and 1.903 (logarithmic of thiamethoxam concentration). Blue dot was for Guangzhou ACP population, orange dot was for Yongzhou ACP population, and gray dot was for Chenzhou ACP population.
Relative Gene Expression Associated With Resistance to Insecticides
The relative expression of all the genes except ACH2 was significantly different among the three populations, and the relative expression of all the genes was the lowest in the Guangzhou population. In fact, among the three populations, extremely significant differences were found for CYP4C67 [F(2,27) = 21.056, P < 0.001], CYP4C68 [F(2,27) = 14.979, P < 0.001], CYP4C70 [F(2,27) = 28.084, P < 0.001], and CYP4DB1 [F(2,27) = 19.673, P < 0.001]. CYP4DA1 [F(2,27) = 6.991, P = 0.004] was moderately significantly different (Figure 3A and Supplementary Table S1). To further verify the effect of P450 on ACP resistance to insecticides, we determined the relative expression of P450 in the Guangzhou population during treatment with semilethal doses
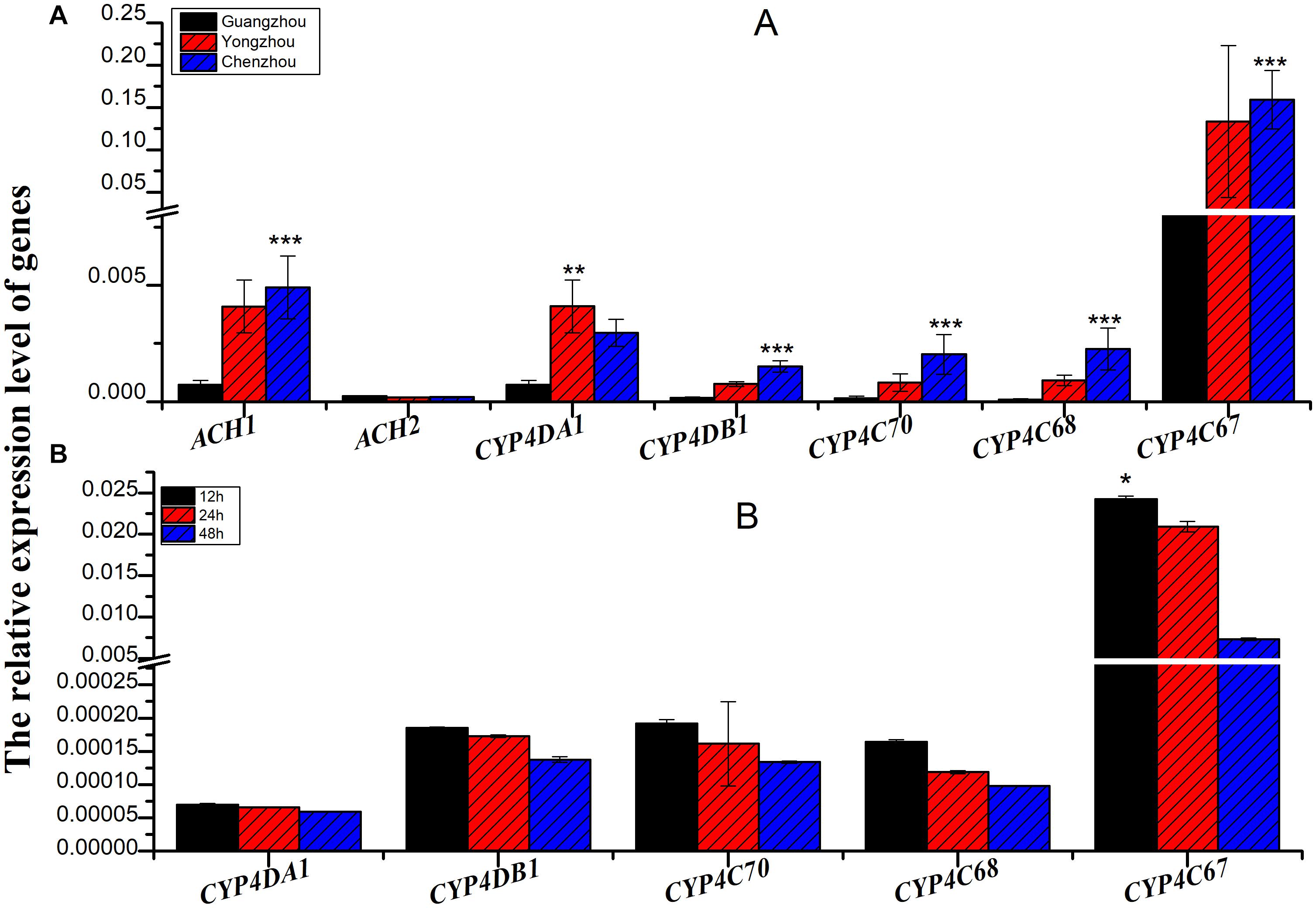
Figure 3. The relative expression of genes associated with cytochrome P450 mono-oxygenase (CYP4C67, CYP4C68, CYP4DA1, CYP4DB1, and CYP4G70) and acetylcholine esterase [AChE-1-like (ACH1) and ChE-2-like (ACH2)]. (A) The relative expression of genes associated with cytochrome P450 mono-oxygenase and acetylcholine esterase from different ACP populations. (B) The relative expression of genes associated with cytochrome P450 mono-oxygenase from Guangzhou ACP at different times after LC50 imidacloprid treatment. Black bar was from Guangzhou ACP population, red bar was from Yongzhou ACP population, and blue bar was from Chenzhou ACP population *P ≤ 0.05, **P ≤ 0.01, ***P ≤ 0.001.
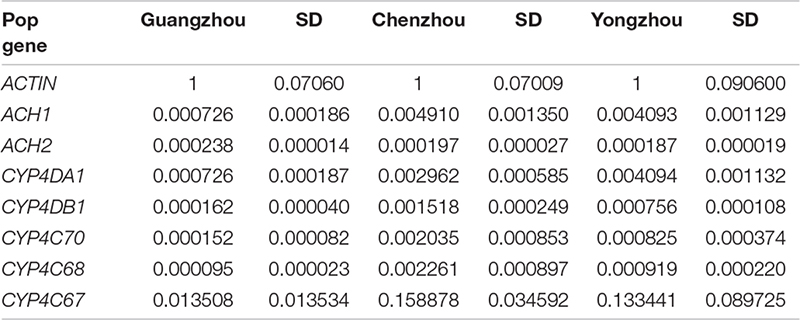
(LC50) of imidacloprid for 12–48 h. The relative expression of all the genes was up-regulated, and the highest relative expression occurred after 12 h. However, the differences among four of the genes (excluding CYP4C67) were not significant (Figures 3B, Supplementary Table S2). Moreover, positive linear relationships between the RR50 value and relative expression of CYP4DB1 and CYP4C70 were observed, and positive linear relationships between the relative abundance of Aspergillus niger and Aureobasidium pullulans and relative expression of CYP4DB1 and CYP4C70 were observed (Figures 4C–H).
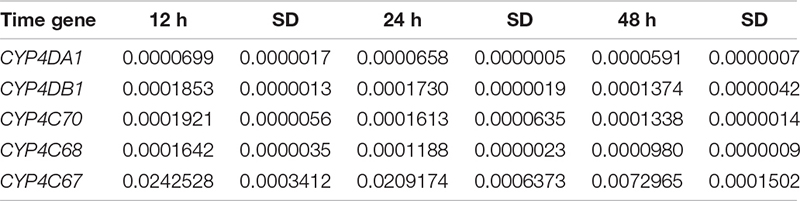
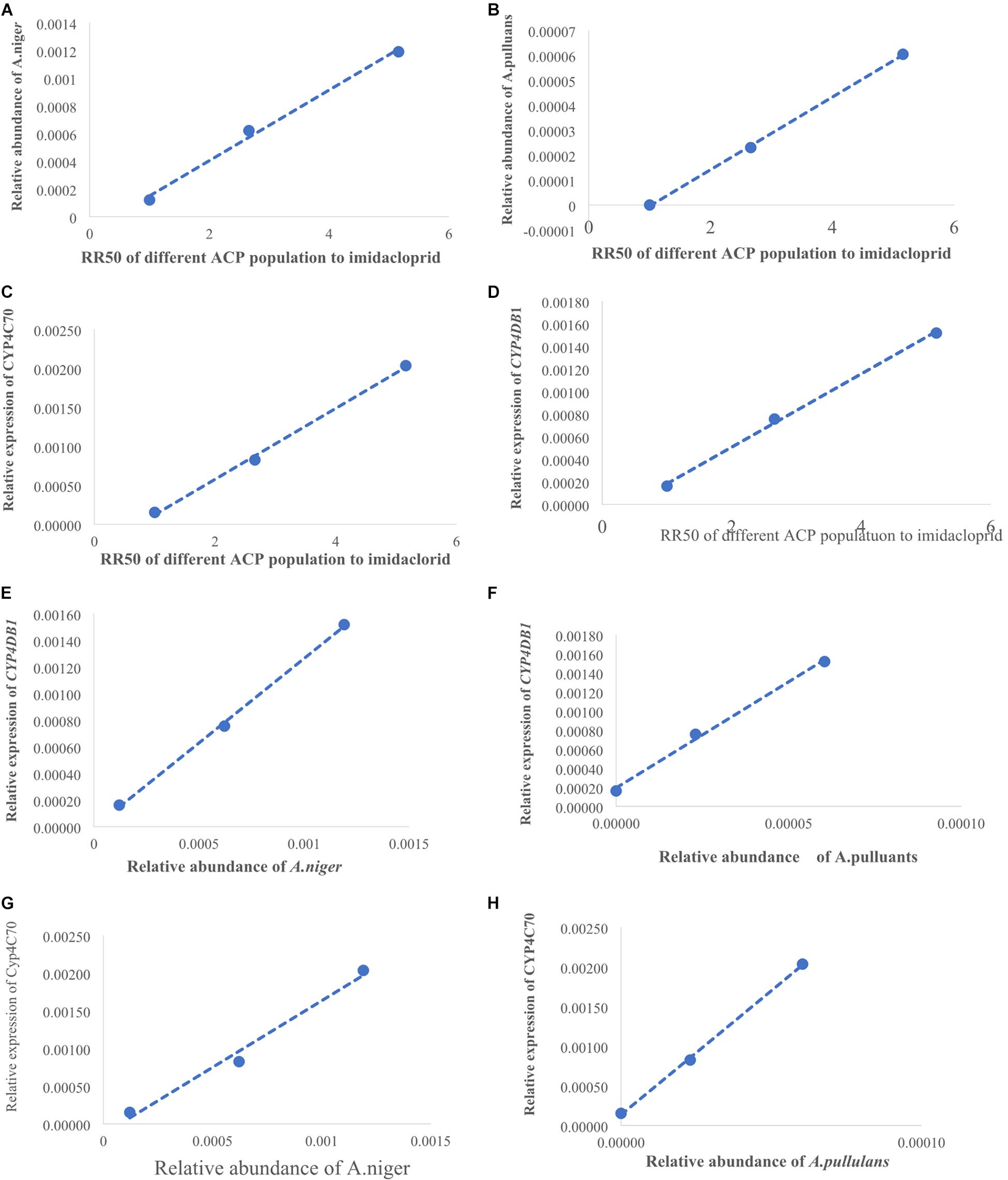
Figure 4. The positive linear correlations picture. (A) Linear relationship between RR50 of imidacloprid and the relative abundance of A. niger. (B) Linear relationship between RR50 of imidacloprid and the relative abundance of A. pullulans. (D) Linear relationship between RR50 of imidacloprid and relative expression of CYP4DB1. (C) Linear relationship between RR50 of imidacloprid and relative expression of CYP4C70. (E) Linear relationship between A. niger and CYP4DB1. (G) Linear relationship between A. niger and CYP4C70. (F) Linear relationship between A. pullulans and CYP4DB1. (H) Linear relationship between A. pullulans and CYP4C70. X-axis of panel (A–D) shows the RR50 value; the first dot was for Guangzhou ACP resistance (=1), the second dot was for Yongzhou ACP RR50, and the third dot was for Chenzhou ACP RR50. X-axis of panel (E–H) was relative abundance of fungi; the first dot was for Guangzhou ACP, the second dot was for Yongzhou ACP, and the third dot was for Chenzhou ACP. Y-axis of panels (A,B) shows the relative abundance of fungi, and Y-axis of panels (D,H) shows the relative expression of genes.
The Composition and Abundance of the Microbial Communities
A total of 528 OTUs were generated, and 315 genus and 152 species were annotated in all samples by 16S sequencing. The known primary symbiont (Candidatus Profftella) and secondary symbionts (Wolbachia) of ACP were assigned by our analytical procedure for the 16S amplicon sequence. And the relative mean abundance of primary symbiont was more than 0.8 (≥80%), the relative mean abundance of secondary symbiont was more than 0.1(≥10%). One hundred sixteen OTUs were unique to Guangzhou ACP population, 134 OTUs were unique to Yongzhou ACP population, and 39 OTUs were unique to Chenzhou ACP population (Venn diagram Supplementary Figure S1).
A total of 1,008 OTUs were generated, and 239 genus and 265 species were annotated in all samples by ITS sequencing. One hundred thirteen OTUs were unique to Guangzhou ACP population, 147 OTUs were unique to Yongzhou ACP population, and 117 OTUs were unique to Chenzhou ACP population (Venn diagram Supplementary Figure S2).
The numbers of unique OTUs in bacteria and fungi from Yongzhou ACP populations was the biggest, and that from Chenzhou ACP populations was the smallest in bacteria, and that from Guangzhou ACP population was the smallest in fungi (Supplementary Figures S1, S2). At the same time, 59.5% OTUs were unnamed species in fungi, and 90.2% OTUs were unnamed species in bacteria. Although the numbers of OTUs from 16S sequencing among three ACP populations were different, but the difference level of composition and abundance of bacteria at species or genus level was not significant. For example, the high difference of four bacteria from three ACP populations, Staphylococcus sciuri [F(2,12) = 4.638, P = 0.073], Brevibacterium epidermidis [F(2,12) = 4.937, P = 0.066], Ileibacterium valens [F(2,12) = 4.597, P = 0.074], and Bacteroides acidifaciens [F(2,12) = 5.031, P = 0.063], were marginally significantly different (0.05 < P < 0.1). However, the mean abundances of sixteen fungi were significantly different among the three ACP populations. Among them, four fungi were unique to the Chenzhou ACP population, and only one fungus was unique to the Guangzhou and Yongzhou ACP populations (Table 2 and Figure 5). Moreover, positive linear correlations were also observed between the mean abundances of two fungi and ACP resistance to pesticides (Figures 4A,B), and negative correlations were observed between only the mean abundances of two fungi and ACP resistance to pesticides, and positive linear correlations were also observed between the mean abundances of two fungi and the mean expression of two genes (Figures 4E–H). The species classification tree (Figure 6) showed Aspergillus, Acremonium, Penicillium, and Cladosporium were of high abundance and may be associated with ACP resistance. Recently, we assessed A. niger and A. pullulans resistance to pesticides by plate confrontation in vitro. The result showed A. niger and A. pullulans could grow in PDA with up to 160 mg/L imidacloprid and up to 96 mg/L thiamethoxam, and the concentration of pesticides negatively affected the diameter of fungi disk in 48 h, and the diameters of fungi disk grown on treatment and control PDA were not different after 72 h (Supplementary Figure S3).
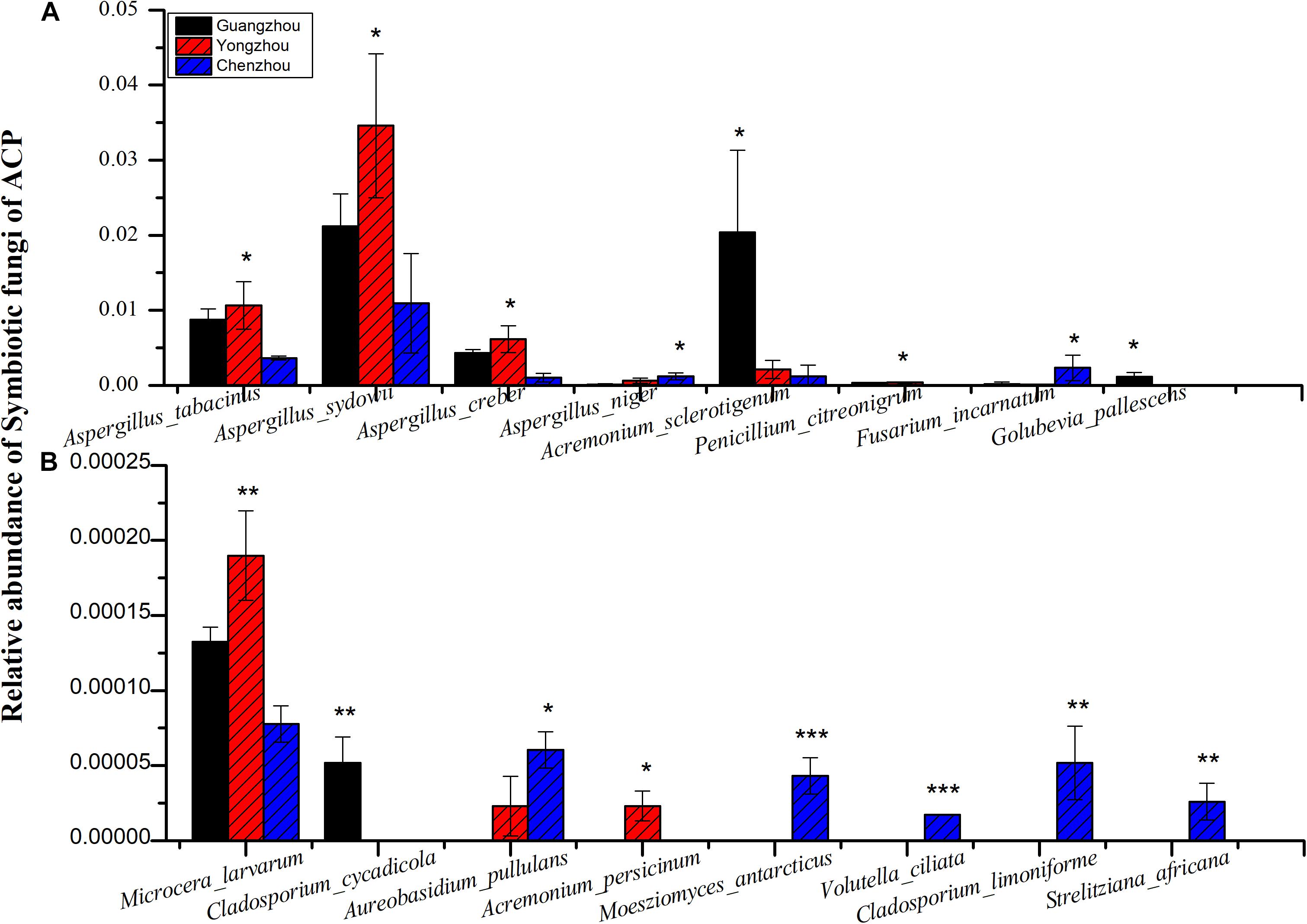
Figure 5. Comparing the relative abundance of the fungi from different ACP populations. Black bar was from Guangzhou ACP population, red bar was from Yongzhou ACP population, blue bar was from Chenzhou ACP population. (A) Relative high abundance fungi. (B) Relative low abundance fungi. Y-axis shows the relative abundance (0–1) of symbiotic fungi of ACP; that is, the OTUs of every fungus were divided by total OTUs. X-axis shows the name of the fungi. *P ≤ 0.05, **P ≤ 0.01, ***P ≤ 0.001.
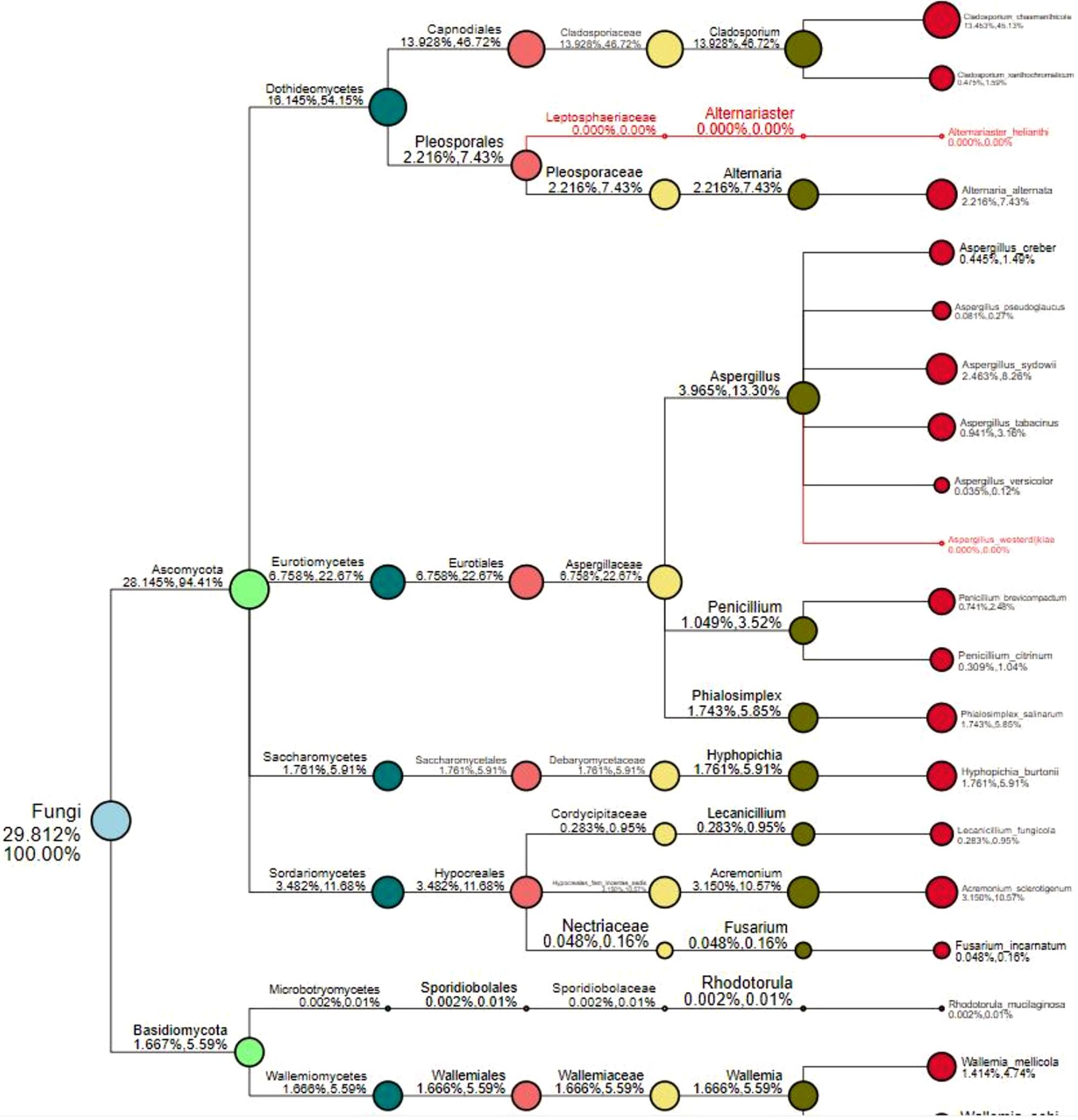
Figure 6. Species classification tree. The circles of different colors indicate different classification levels, corresponding to the legend on the left; the size of the circle represents the relative abundance of the category; the two numbers below the category name both indicate the relative abundance percentage; the former indicates that the category occupies the sample. The percentage of all classified species in the sample; the latter indicates the percentage of the classification in the classified species selected in the sample. The classification in red font indicates that the classification annotation does not exist in the sample, but it exists in other analyzed samples.
Discussion
As a notorious Rutaceae plant pest, ACP not only affects the growth of young foliage by direct feeding but also transmits C. Liberibacter asiaticus (Las) and C. Liberibacter americanus (Lam), resulting in one of the most devastating citrus diseases worldwide, HLB (Duan et al., 2009; Gottwald, 2010; Grafton-Cardwell et al., 2013; Shimwela et al., 2016). Organophosphates, carbamates, pyrethroids, phenylpyrazoles, and neonicotinoids have been used extensively to control all kinds of insect pests, resulting in different resistance levels to major pesticides (Wang et al., 2009; Wen et al., 2009). In this study, we found that the ACP population in China had resistance to imidacloprid and thiamethoxam and that the Hunan ACP populations had higher resistance levels than the Guangzhou ACP population. The results were consistent with previous studies showing that ACP populations from different geographic locations had different levels of resistance to imidacloprid (Tiwari et al., 2011; Kanga et al., 2015; Tian et al., 2018).
Many studies have also shown that increased activities of general esterase and P450 mono-oxygenase are common insecticide resistance mechanisms against organophosphate, pyrethroid, and neonicotinoid insecticides in some insect pests (Cao et al., 2008; Zhang et al., 2014; Garrood et al., 2016). Studies have shown that enhanced expression of genes associated with cytochrome P450 mono-oxygenase contributes to neonicotinoid resistance in BPHs (Puinean et al., 2010; Bass et al., 2011; Tian et al., 2018). In this study, the positive linear relationship between the relative expression of two genes (CYP4C70 and CYP4DB1) associated with P450 mono-oxygenase and the ACP RR50 to imidacloprid and the positive linear relationship between the relative expression of CYP4C70 and CYP4DB1 and the relative abundance of A. niger and A. pullulans were observed, suggesting A. niger and A. pullulans and P450 together affected ACP resistance to imidacloprid and thiamethoxam. Moreover, the relative expression of all the genes from the Guangzhou population, which is associated with cytochrome P450, increased after insecticide treatment; the expression level was highest 12 h after treatment and then declined. The results were similar with literature that cytochrome P450 mono-oxygenase was mainly involved in ACP resistance to imidacloprid and thiamethoxam (Guitard et al., 2014; Tian et al., 2018). The literature shows that CYP6ER1 affects the resistance of N. lugens to imidacloprid, thiamethoxam, buprofezin, and ethiprole (Wu et al., 2018), whereas in this study, CYP4C70 and CYP4DB1 affected ACP resistance to imidacloprid and thiamethoxam; these findings may be due to the same enzyme being regulated by different microbiology in different species.
The literature also shows that bacteria such as Stenotrophomonas maltophilia, Bacillus licheniformis, Bacillus megaterium, Rahnella aquatilis, and fungi such as Umbelopsis isabellina, V. ciliata, and Botrytis cinerea can degrade atrazine, which is the most commonly detected pesticide in food and drinking water (Marecik et al., 2008; Singh et al., 2018). In this study, the relative abundance of all the bacteria among the three ACP populations was not significant. This result suggested that the symbiotic bacteria of the ACP were not similar to those of Wolbachia in the whitefly and the mosquito Culex pipiens L. (Diptera: Culicidae), which may be involved in host’s resistance to imidacloprid and thiamethoxam (Ghanim and Kontsedalov, 2009). Furthermore, there were significant differences in the resistance mechanisms of different species to the same pesticides (Kanga et al., 2015; Tian et al., 2018; Wang et al., 2019). Moreover, that there was not significantly different bacterial community among ACP populations at species level may be due to 90.2% OTUs being unnamed species in bacteria.
FunGuild is a fungal environmental function database. Based on the support of existing literature, the ecological function of fungi is classified, and the FunGuild database is constructed. Based on species information obtained from amplicon analysis, the ecological functions of existing species in the literature can be queried in the environment. Because FunGuild was mainly used in analysis on environmental microbiology and limited to existing literature, the fungi from ACP were associated plant pathogen and animal pathogen (Gandarilla-Pacheco). Although FunGuild analysis did not find significantly different fungi that were associated with ACP resistance to pesticides, yet the species classification tree (Figure 6) showed Aspergillus, Acremonium, Penicillium, and Cladosporium were dominant strains.
A. pullulans and A. niger can exist in different agroecological niches, and they produce a neutral polysaccharide, antimycotic aureobasidin, antibacterial compounds, melanin, liamocins, siderophore, and extracellular enzymes such as P450 (Franken et al., 2014; Molnárová et al., 2014; Prasongsuk et al., 2018). Polysaccharides, antimycotic aureobasidins, antibacterial compounds, and melanin play important roles in insect immune and defense systems (Luan et al., 2012, 2017; Selvam et al., 2013). P450 was involved in insecticide resistance of pest (Li et al., 2010; Puinean et al., 2010; Safi et al., 2017; Tian et al., 2018; Wang et al., 2019). Although the role of symbiotic fungi in the degradation of insecticides has not been studied extensively, roles of A. niger in the immunity and growth of insect and animal have been reported (Medeiros et al., 2009; Fattahi et al., 2014). In this study, four Aspergillus species, A. tabacinus, A. sydowii, A. creber, and A. niger, were significantly different among the three populations. Positive linear correlations were observed between the relative mean abundances of A. niger and A. pullulans and ACP resistance to imidacloprid, and positive linear correlations were also observed between the relative mean abundances of A. niger and A. pullulans and the relative mean expression of CYP4DB1 and CYP4C70. Recently, we assessed A. niger and A. pullulans resistance to pesticides by plate confrontation in vitro. The result showed A. niger and A. pullulans could grow in PDA with 160 mg/L imidacloprid and 96 mg/L thiamethoxam, and the concentration of pesticides negatively affected the diameter of fungi disk in 48 h, and the diameters of fungi disk grown on treatment and control PDA were not different after 72 h (Supplementary Figure S3). These findings suggested that A. niger and A. pullulans may be positively affect ACP resistance, and G. pallescens and A. sclerotigenum may be negatively affect ACP resistance. Whether ACP symbiotic fungi affect insect host resistance to pesticides by producing compounds associated with ACP immunity, such as polysaccharide, antimycotic aureobasidin, antibacterial compounds, melanin, liamocins, siderophore, or producing P450 to degrade pesticides, remains to be elucidated, which will be our work in the future. Moreover, four fungi, Moesziomyces antarcticus, V. ciliata, Cladosporium limoniforme, and Strelitziana africana, were unique to the Chenzhou population, which had the highest resistance level among the ACP populations. Whether they were associated with ACP resistance needs further study.
Data Availability Statement
The demultiplexed sequence data have been deposited in National Center for Biotechnology Information (https://www.ncbi.nlm.nih.gov/sra, 16s accession PRJNA646487 and ITS accession PRJNA646485).
Author Contributions
TY and YH designed the experiment and wrote the manuscript. LL, LH, and JY performed the experiment. LL and LD analyzed data and drew dragram. All authors contributed to the article and approved the submitted version.
Conflict of Interest
The authors declare that the research was conducted in the absence of any commercial or financial relationships that could be construed as a potential conflict of interest.
Funding
The project was supported by the National Key Research and Development Project of China: “Research and demonstration of prevention and control technology in central China citrus HLB interdiction belt and low epidemic areas (2018YFD0201505),” Innovation Platform Open Fund Project of Hunan Province Education Department: “The abundance and composition of bacteria and fungi inside of the body and on the surface of the ACP affected the ‘Candidatus Liberibacter sp.’ propagation and transmission (18K044),” China Scholarship Council Project: “Program of Study Abroad for Young Scholar (201809175003),” Double First-Class Construction Project of Hunan Agricultural University (SYL2019027), and Hunan Province Key Research and Development Project: “Research and application of key technologies for green control of citrus pests and diseases (2018NK 2012).”
Acknowledgments
We thank Jiefu Dun, Liudeicai Wang, and Jia Jiang from the Hunan Agricultural University for their assistance in experiment.
Supplementary Material
The Supplementary Material for this article can be found online at: https://www.frontiersin.org/articles/10.3389/fmicb.2020.522164/full#supplementary-material
Footnotes
- ^ https://unite.ut.ee
- ^ http://qiime.org/scripts/assigntaxonomy.html
- ^ https://www.ncbi.nlm.nih.gov/sra
References
Abbott, W. S. (1925). A method of computing the effectiveness of an insecticide. J. Econ. Entomol. 18, 265–267. doi: 10.1093/jee/18.2.265a
Bass, C., Carvalho, R. A., Oliphant, L., Puinean, A. M., Field, L. M., and Nauen, R. (2011). Overexpression of a cytochrome P450 monooxygenase, CYP6ER1, is associated with resistance to imidacloprid in the brown planthopper, Nilaparvata lugens. Insect. Mol. Biol. 20, 763–773. doi: 10.1111/j.1365-2583.2011.01105.x
Bisht, J., Harsh, N. S. K., Palni, L. M. S., Agnihotri, V., and Kumar, A. (2019). Biodegradation of chlorinated organic pesticides endosulfan and chlorpyrifos in soil extract broth using fungi. Remediat. J. 29, 63–77. doi: 10.1002/rem.21599
Boush, M. G., and Matsumura, F. (1967). Insecticidal degradation by Pseudomonas melophthora, the bacterial symbiote of the apple maggot. J. Econ. Entomol. 60, 918–920. doi: 10.1093/jee/60.4.918
Breglia, S. A., Yubuki, N., Hoppenrath, M., and Leander, B. S. (2010). Ultrastructure and molecular phylogenetic position of a novel euglenozoan with extrusive episymbiotic bacteria: bihospites bacati n. gen. et sp. (Symbiontida). BMC Microbiol. 10:145. doi: 10.1186/1471-2180-10-145
Cao, C. W., Zhang, J., Gao, X. W., Liang, P., and Guo, H. L. (2008). Overexpression of carboxylesterase gene associated with organophosphorous insecticide resistance in cotton aphids, Aphis gossypii (Glover). Pestic. Biochem. Physiol 90, 175–180. doi: 10.1016/j.pestbp.2007.11.004
Caporaso, J. G., Lauber, C. L., Walters, W. A., Berg-Lyons, D., Lozupone, C. A., and Turnbaugh, P. J. (2011). Global patterns of 16S rRNA diversity at a depth of millions of sequences per sample. Proc. Natl. Acad. Sci. U.S.A. 108(Suppl. 1), 4516–4522. doi: 10.1073/pnas.1000080107
Cheng, D., Guo, Z., Riegler, M., Xi, Z., Liang, G., and Xu, Y. (2017). Gut symbiont enhance insecticide resistance in a significant pest, the oriental fruit fly Bactrocera dorsalis (Hendel). Microbiome 5:13. doi: 10.1186/s40168-017-0236-z
Dang, K., Doggett, S. L., Singham, G. V., and Lee, C. Y. (2017). Insecticide resistance and resistance mechanisms in bed bugs cimex spp. (hemiptera: cimicidae). Parasit. Vect. 10:318. doi: 10.1186/s13071-017-2232-3
Douglas, A. E. (2015). Multiorganismal insects: diversity and function of resident microorganisms. Annu. Rev. Entomol. 7, 17–34. doi: 10.1146/annurev-ento-010814-020822
Dowd, P. F., and Shen, S. K. (2011). The contribution of symbiotic yeast to toxin resistance of the cigarette beetle (Lasioderma serricorne). Entomol. Exp. Appl. 56, 241–248. doi: 10.1111/j.1570-7458.1990.tb01402.x
Duan, Y., Zhou, L., Hall, D. G., Li, W., Doddapaneni, H., Lin, H., et al. (2009). Complete genome sequence of citrus huanglongbing bacterium, ‘Candidatus Liberibacter asiaticus’ obtained through metagenomics. Mol. Plant Microbe. Interact. 22, 1011–1020. doi: 10.1094/mpmi-22-8-1011
Eason, J., Kanga, L. H., Haseeb, M., Quershi, J. A., and Legaspi, J. (2018). Mechanisms of resistance to organophosphorus and pyrethroid insecticides in Asian Citrus Psyllid Diaphorina citri, populations in Florida. Curr. Inves. Agri. Curr. Res. 1:111. doi: 10.32474/CIACR.2018.01.000111
Fattahi, H., Jafaryan, H., Khosravi, A. R., and Arpanahi, D. A. (2014). The probiotic effects of dietary saccharomyces cerevisiae and aspergillus niger on the growth and some immunity factors of cultured juvenile beluga sturgeon (huso huso). Iran. J. Fish. Sci. 23, 47–61.
Franken, A. C. W., Lechner, B. E., Werner, E. R., Haas, H., Lokman, B. C., and Ram, A. F. J. (2014). Genome mining and functional genomics for siderophore production in Aspergillus niger. Briefings Funct. Genom. 13, 482–492. doi: 10.1093/bfgp/elu026
Gandarilla-Pacheco, F. L., Gálan-Wong, L. J., Arroyo, J. I., Rodríguez-Guerra, R., and Quintero-Zapata, I. (2013). Optimization of pathogenicity tests for selection of native isolates of entomopathogenic fungi isolated from citrus growing areas of méxico on adults of diaphorina citri kuwayama (Hemiptera: Liviidae). Flo. Entomol. 96, 187–195. doi: 10.1653/024.096.0125
Garrood, W. T., Zimmer, C. T., Gorman, K. J., Nauen, R., Bass, C., and Davies, T. G. (2016). Field−evolved resistance to imidacloprid and ethiprole in populations of brown planthopper nilaparvata lugens collected from across south and east Asia. Pest Manag. Sci. 72, 140–149. doi: 10.1002/ps.3980
Ghanim, M., and Kontsedalov, S. (2009). Susceptibility to insecticides in the Q biotype of Bemisia tabaci is correlated with bacterial symbiont densities. Pest Manag. Sci. 65, 939–942. doi: 10.1002/ps.1795
Gottwald, T. R. (2010). Current epidemiological understanding of citrus Huanglongbing. Ann. Rev. Phytopathol. 48, 119–139. doi: 10.1146/annurev-phyto-073009-114418
Grafton-Cardwell, E. E., Stelinski, L. L., and Stansly, P. A. (2013). Biology and management of Asian citrus psyllid, vector of the huanglongbing pathogens. Annu. Rev. Entomol. 58, 413–432.
Guitard, J., Degulys, A., Buot, G., Aline-Fardin, A., Dannaoui, E., Rio, B., et al. (2014). Acremonium sclerotigenum-Acremonium egyptiacum: a multi-resistant fungal pathogen complicating the course of aplastic anaemia. Clin. Microbiol. Infect. 20, 30–32.
Hong, Y. Y., Luo, Y. Y., Yi, J. L., He, L., Dai, L. Y., and Yi, T. Y. (2019). Screening nested-PCR primer for‘Candidatus Liberibacter asiaticus’ associated with citrus Huanglongbing and application in Hunan,China. PLoS One 14:e0212020. doi: 10.1371/journal.pone.0212020
Hong, Y. Y., Yi, T. Y., Tan, X. L., Su, J. W., and Ge, F. (2017). High ozone (O3) affects the transmition of TYLCCNV by Q biotype Bemisia tabaci. Sci. Rep. 7:14412. doi: 10.1038/s41598-017-14023-6
Hong, Y. Y., Yi, T. Y., Tan, X. L., Zhao, Z. H., and Ge, F. (2016). High ozone (O3) affects the fitness associated with the microbial composition and abundance of Q biotype Bemisia tabaci. Front. Microbiol. 7:1593. doi: 10.3389/fmicb.01593
Howard, S. J., Webster, I., Moore, C. B., Gardiner, R. E., Park, S., Perlin, D. S., et al. (2006). Multi-azole resistance in Aspergillus fumigatus. Int. J. Antimicrob. Agents 28, 450–453.
Kanga, L. H. B., Eason, J., Haseeb, M., Qureshi, J., and Stansly, P. (2015). Monitoring for insecticide resistance in asian citrus psyllid (Hemiptera: Psyllidae) populations in Florida. J. Econ. Entomol. 15, 1–5.
Kikuchi, Y., Hayatsu, M., Hosokawa, T., Nagayama, A., Tago, K., and Fukatsu, T. (2012). Symbiont-mediated insecticide resistance. Proc. Natl. Acad. Sci. U.S.A. 109, 8618–8622. doi: 10.1073/pnas.1200231109
Li, J., Shao, Y., Ding, Z. P., Bao, H. B., Liu, Z. W., Han, Z. J., et al. (2010). Native subunit composition of two insect nicotinic receptor subtypes with differing affinities for the insecticide imidacloprid. Insect Biochem. Mol. Biol. 40, 17–22. doi: 10.1016/j.ibmb.2009.12.003
Liu, Z., Williamson, M. S., Lansdell, S. J., Denholm, I., Han, Z., and Millar, N. S. (2005). A nicotinic acetylcholine receptor mutation conferring target-site resistance to imidacloprid in Nilaparvata lugens (brown planthopper). Proc. Natl. Acad. Sci. U.S.A. 102, 8420–8425. doi: 10.1073/pnas.0502901102
Livak, K. J., and Schmittgen, T. D. (2001). Analysis of relative gene expression data using real-time quantitative PCR and the 2-ΔCT method. Methods 25, 402–408. doi: 10.1006/meth.2001.1262
Luan, G. D., Guo, Z., Riegler, M., Xi, Z., Liang, G., and Xu, Y. (2017). Gut symbiont enhances insecticide resistance in a significant pest, the oriental fruit fly bactrocera dorsalis (hendel). Microbiome 5, 13–21.
Luan, Z. Y., Chen, X., Yi, S., and Zhen, C. (2012). Bacterial degradation of chloropyrifos by Bacillus cereus. Adv. Mater. Res. 35, 676–680. doi: 10.4028/www.scientific.net/amr.356-360.676
Marecik, R., Króliczak, P., Czaczyk, K., Białas, W., Olejnik, A., and Cyplik, P. (2008). Atrazine degradation by aerobic microorganisms isolated from the rhizosphere of sweet flag (Acorus calamus L.). Biodegradation 19, 293–301. doi: 10.1007/s10532-007-9135-5
Martin, M. (2011). Cut adapt removes adapter sequences from high-throughput sequencing reads. Emb. J. 11:17.
Medeiros, M. N. D., Belmonte, R., Soares, B. C. C., Medeiros, L. N. D., Canetti, C., and Freire-De-Lima, C. G. (2009). Arrest of oogenesis in the bug rhodnius prolixus challenged with the fungus aspergillus niger is mediated by immune response-derived pge2. J. Insect Physiol. 55, 151–158. doi: 10.1016/j.jinsphys.2008.10.019
Molnárová, J., Vadkertiová, R., and Stratilová, E. (2014). Extracellular enzymatic activities and physiological profiles of yeasts colonizing fruit trees. J. Basic Microbiol. 54, S74–S84.
Nakabachi, A., Piel, J., Malenovský, I., and Hirose, Y. (2020). Comparative genomics underlines multiple roles of profftella, an obligate symbiont of psyllids: providing toxins, vitamins, and carotenoids. Genome Biol. Evol. 12, 1975–1987. doi: 10.1093/gbe/evaa175
Nakabachi, A., Ueoka, R., Oshima, K., Teta, R., Mangoni, A., Gurgui, M., et al. (2013). Defensive bacteriome symbiont with a drastically reduced genome. Curr. Biol. 23, 1478–1484. doi: 10.1016/j.cub.2013.06.027
Nguyen, N. H., Song, Z., Bates, S. T., Branco, S., Tedersoo, L., Menke, J., et al. (2016). FUNGuild: an open annotation tool for parsing fungal community datasets by ecological guild[J]. Fun. Ecol. 20, 241–248. doi: 10.1016/j.funeco.2015.06.006
Pang, R., Chen, M., Yue, L., Xing, K., Li, T., Kang, K., et al. (2018). A distinct strain of arsenophonus symbiont decreases insecticide resistance in its insect host. PLoS Genet. 14:e1007725. doi: 10.1371/journal.pgen.1007725
Pardo, S., Martinez, A. M., Figueroa, J. I., Chavarrieta, J. M., Viñuela, E., and Rebollar-Alviter, Á, et al. (2018). Insecticide resistance of adults and nymphs of Asian citrus psyllid populations from Apatzingán Valley, Mexico. Pest Manag. Sci. 74, 135–140. doi: 10.1002/ps.4669
Prasongsuk, S., Lotrakul, P., Ali, I., Bankeeree, W., and Punnapayak, H. (2018). The current status of Aureobasidium pullulans in biotechnology. Folia Microbiol. (Praha) 63, 129–140. doi: 10.1007/s12223-017-0561-4
Puinean, A. M., Denholm, I., Millar, N. S., Nauen, R., and Williamson, M. S. (2010). Characterisation of imidacloprid resistance mechanisms in the brown planthopper, Nilaparvata lugens St (Hemiptera: Delphacidae). Pestic Biochem. Physiol. 97, 129–132. doi: 10.1016/j.pestbp.2009.06.008
Ramsey, J. S., Johnson, R. S., Hoki, J. S., Kruse, A., Mahoney, J., Hilf, M. E., et al. (2015). Metabolic interplay between the asian citrus psyllid and its profftella symbiont: an achilles’ heel of the citrus greening insect vector. PLoS One 10:e0140826. doi: 10.1371/journal.pone.0140826
Ren, S. L., Li, Y. H., Ou, D., Guo, Y. J., Qureshi, J. A., Stansly, P. A., et al. (2018). Localization and dynamics of wolbachiainfection in asian citrus psylliddiaphorina citri, the insect vector of the causal pathogens of huanglongbing. Microbiologyopen 7:e00561. doi: 10.1002/mbo3.561
Rudramurthy, S. M., Paul, R. A., Chakrabart, A., Mouton, J. W., and Meis, J. F. (2019). Invasive aspergillosis by Aspergillus flavus: epidemiology, diagnosis, antifungal, resistance, and management. J. Fungi 5:55. doi: 10.3390/jof5030055
Safi, N. H., Ahmadi, A. A., Nahzat, S., Ziapour, S. P., Nikookar, S. H., Fazeli-Dinan, M., et al. (2017). Evidence of metabolic mechanisms playing a role in multiple insecticides resistance in Anopheles stephensi populations from Afghanistan. Malaria J. 16:100. doi: 10.1186/s12936-017-1744-9
Saha, S., Hunter, W. B., Reese, J., Morgan, J. K., Marutani-Hert, M., Huang, H., et al. (2012). Survey of endosymbionts in the diaphorina citri metagenome and assembly of a wolbachia wdi draft genome. PLoS One 7:e50067. doi: 10.1371/journal.pone.0050067
Selvam, A., Gnana, D., Thatheyu, A. J., and Vidhya, R. (2013). Biodegradation of the synthetic pyrethroid, fenvalerate by Bacillus cereusMtcc 1305. World J. Environ. Eng. 2, 21–26.
Shimwela, M. M., Narouei-Khandan, H., Halbert, S. E., Keremane, M. L., Minsavage, G. V., Timilsina, S., et al. (2016). First occurrence of diaphorina citriin east africa, characterization of theca. liberibacter species causing huanglongbing (HLB) in tanzania, and potential further spread ofd. citriand hlb in africa and europe. Eur. J. Plant Pathol. 146, 349–368. doi: 10.1007/s10658-016-0921-y
Singh, S., Kumar, V., Chauhan, A., Datta, S., Wani, A. B., Singh, N., et al. (2018). Toxicity, degradation and analysis of the herbicide atrazine. Environ. Chem. Lett. 16, 211–237. doi: 10.1007/s10311-017-0665-8
Subandiyah, S., Nikoh, N., Tsuyumu, S., Somowiyarjo, S., and Fukatsu, T. (2000). Complex endosymbiotic microbiota of the citrus psyllid diaphorina citri (homoptera: psylloidea). Zool. Sci. 17, 983–989. doi: 10.2108/zsj.17.983
Tian, F. J., Mo, X. F., Rizvi, S. A. H., Li, C. F., and Zeng, X. N. (2018). Detection and biochemical characterization of insecticide resistance in field populations of asian citrus psyllid in guangdong of china. Sci. Rep. 8:12587. doi: 10.1038/s41598-018-30674-5
Tiwari, S. S., Mann, R. S., Rogers, M. E., and Stelinski, L. (2011). Insecticide resistance in field populations of Asian citrus psyllid in Florida. Pest Manag. Sci. 67, 1258–1268. doi: 10.1002/ps.2181
Wang, Z., Yao, M., and Wu, Y. (2009). Cross-resistance, inheritance and biochemical mechanisms of imidacloprid resistance in B-biotype Bemisia tabaci. Pest Manag. Sci. 65, 1189–1194. doi: 10.1002/ps.1808
Wang, Z. B., Tian, F. J., Cai, L. J., Zhang, J., Liu, J. L., and Zeng, X. N. (2019). Identifcation of candidate ATPbinding cassette transporter gene family members in Diaphorina citri (Hemiptera: Psyllidae) via adult tissues transcriptome analysis. Sci. Rep. 9:15842. doi: 10.1038/s41598-019-52402-3
Wei, Q. B., Lei, Z. R., Nauen, R., Cai, D. C., and Gao, Y. L. (2015). Abamectin resistance in strains of vegetable leafminer, Liriomyza sativae(Diptera: Agromyzidae) is linked to elevated glutathione S-transferase activity. Insect. Sci. 22, 243–250. doi: 10.1111/1744-7917.12080
Wen, Y., Liu, Z., Bao, H., and Han, Z. (2009). Imidacloprid resistance and its mechanisms in field populations of brown planthopper,Nilaparvata lugens in China. Pestic. Biochem. Physiol. 94, 36–48. doi: 10.1016/j.pestbp.2009.02.009
White, T. J., Bruns, T. D., Lee, S., and Taylor, J. (1990). “Amplification and direct sequencing of fungal ribosomal RNA genes for phylogenetics,” in PCR Protocols: a Guide to Method and Applications, eds M. A. Innis, D. H. Gefland, J. J. Sninsky, and T. J. White (San Diego, CA: Academic Press.), 315–322. doi: 10.1016/b978-0-12-372180-8.50042-1
Wu, S. F., Zeng, B., Zheng, C., Mu, X. C., Zhang, Y., Hu, J., et al. (2018). The evolution of insecticide resistance in the brown planthopper (Nilaparvata lugens Stål) of China in the period 2012–2016. Sci. Rep. 8:4586.
Yang, M. L., Zhang, J. Z., Zhu, K. Y., Xuan, T., Liu, X. J., and Guo, Y. P. (2009). Mechanism of organophosphate resistance in a field population of oriental migratory locust, Locusta migratoria manilensis (Meyen). Arch Insect. Biochem. Physiol. 71, 3–15. doi: 10.1002/arch.20254
Zhang, K., Zhang, W., Zhang, S., Wu, S. F., Ban, L. F., Su, J. Y., et al. (2014). Susceptibility of Sogatella furcifera and Laodelphax striatellus (Hemiptera: Delphacidae) to six insecticides in China. J. Econ. Entomol. 107, 1916–1922. doi: 10.1603/ec14156
Keywords: Asian citrus psyllid (ACP), symbiotic fungus, resistance, imidacloprid, thiamethoxam
Citation: Yi T, Lei L, He L, Yi J, Li L, Dai L and Hong Y (2020) Symbiotic Fungus Affected the Asian Citrus Psyllid (ACP) Resistance to Imidacloprid and Thiamethoxam. Front. Microbiol. 11:522164. doi: 10.3389/fmicb.2020.522164
Received: 21 December 2019; Accepted: 09 November 2020;
Published: 16 December 2020.
Edited by:
Takema Fukatsu, National Institute of Advanced Industrial Science and Technology (AIST), JapanReviewed by:
Jason E. Stajich, University of California, Riverside, United StatesMinoru Moriyama, National Institute of Advanced Industrial Science and Technology (AIST), Japan
Copyright © 2020 Yi, Lei, He, Yi, Li, Dai and Hong. This is an open-access article distributed under the terms of the Creative Commons Attribution License (CC BY). The use, distribution or reproduction in other forums is permitted, provided the original author(s) and the copyright owner(s) are credited and that the original publication in this journal is cited, in accordance with accepted academic practice. No use, distribution or reproduction is permitted which does not comply with these terms.
*Correspondence: Yanyun Hong, hongyanyun@126.com