- 1Key Laboratory of Sugarcane Biotechnology and Genetic Improvement (Guangxi), Ministry of Agriculture, Sugarcane Research Center, Chinese Academy of Agricultural Sciences, Guangxi Key Laboratory of Sugarcane Genetic Improvement, Sugarcane Research Institute, Guangxi Academy of Agricultural Sciences, Nanning, China
- 2Guangxi Key Laboratory of Crop Genetic Improvement and Biotechnology, Nanning, China
- 3School of Marine Sciences and Biotechnology, Guangxi University for Nationalities, Nanning, China
- 4State Key Laboratory of Conservation and Utilization of Subtropical Agro-Bio Resources, College of Agriculture, Guangxi University, Nanning, China
- 5Microbiology Institute, Guangxi Academy of Agricultural Sciences, Nanning, China
- 6Interdisciplinary Center for Agriculture Green Development in Yangtze River Basin, College of Resources and Environment, Southwest University, Chongqing, China
- 7Queensland Alliance for Agriculture and Food Innovation, The University of Queensland, St Lucia, QLD, Australia
Excessive, long-term application of chemical fertilizers in sugarcane crops disrupts soil microbial flora and causes environmental pollution and yield decline. The role of endophytic bacteria in improving crop production is now well-documented. In this study, we have isolated and identified several endophytic bacterial strains from the root tissues of five sugarcane species. Among them, eleven Gram-negative isolates were selected and screened for plant growth-promoting characteristics, i.e., production of siderophores, indole-3-acetic acid (IAA), ammonia, hydrogen cyanide (HCN), and hydrolytic enzymes, phosphorus solubilization, antifungal activity against plant pathogens, nitrogen-fixation, 1-aminocyclopropane-1-carboxylic acid deaminase activity, and improving tolerance to different abiotic stresses. These isolates had nifH (11 isolates), acdS (8 isolates), and HCN (11 isolates) genes involved in N-fixation, stress tolerance, and pathogen biocontrol, respectively. Two isolates Pantoea cypripedii AF1and Kosakonia arachidis EF1 were the most potent strains and they colonized and grew in sugarcane plants. Both strains readily colonized the leading Chinese sugarcane variety GT42 and significantly increased the activity of nitrogen assimilation enzymes (glutamine synthetase, NADH glutamate dehydrogenase, and nitrate reductase), chitinase, and endo-glucanase and the content of phytohormones gibberellic acid, indole-3-acetic acid, and abscisic acid. The gene expression analysis of GT42 inoculated with isolates of P. cypripedii AF1 or K. arachidis EF1 showed increased activity of nifH and nitrogen assimilation genes. Also, the inoculated diazotrophs significantly increased plant nitrogen content, which was corroborated by the 15N isotope dilution analysis. Collectively, these findings suggest that P. cypripedii and K. arachidis are beneficial endophytes that could be used as a biofertilizer to improve plant nitrogen nutrition and growth of sugarcane. To the best of our knowledge, this is the first report of sugarcane growth enhancement and nitrogen fixation by Gram-negative sugarcane root-associated endophytic bacteria P. cypripedii and K. arachidis. These strains have the potential to be utilized as sugarcane biofertilizers, thus reducing nitrogen fertilizer use and improving disease management.
Introduction
Global food security is a major sustainable development goal of United Nations. This is also a major challenge for developing countries with limited resources and scientific capacity. Agriculture is a major consumer of energy and cause of environmental damages, mainly due to the large input of fertilizers, land use practices, and use of fossil fuel (Fraser and Campbell, 2019; Yang et al., 2021). Sustainable intensification of agriculture is a well-recognized concept and is being practiced in the developed world (Tilman et al., 2002; Vanlauwe et al., 2019). It improves resource use efficiency, reduces agricultural inputs, especially fertilizers and other agri-chemicals, and expansion of mechanized farm operations. In this context, considerable research to understand and exploit soil and rhizosphere microbiomes and plant endophytes to reduce fertilizer input and suppress pathogens are now underway globally. This is particularly relevant when 60–90% of the applied chemical fertilizers are wasted depending on the crop and region, and the manufacturing of agri-chemicals like nitrogen (N) fertilizers is a highly energy-intensive process (Bhardwaj et al., 2014). Biofertilizers, which are live cells of microbes, are a potential alternative to chemical fertilizers since they provide nutrients to plants, reduce soil-borne diseases, and improve the health and quality of soil (Bhardwaj et al., 2014; Kour et al., 2020).
Sugarcane (Saccharum spp. interspecific hybrids), a member of Poaceae, is a major commercial crop grown in the tropical and subtropical areas of the world. Due to its large contribution to sugar production, manufacture of ethanol, and a source of environmentally sustainable green energy, it is an economically important crop worldwide (Vital et al., 2017). It accounts for more than 80% of global sugar production, with Brazil, India, China, and Thailand accounting for 60% of total output (FAO, 2020a). Global sugar output was around 166.18 million metric tons during the 2019–2020 (United States Department of Agriculture [USDA], 2020). China is the world’s third largest producer of sugar, with an annual output of over 13 million tons. Sugarcane accounts for more than 90% of sugar production in China, with Guangxi province accounting for over 65% of total production (Li et al., 2016). Chinese sugarcane crop productivity, however, is lower than the world average. This is largely caused by sub-optimal crop production management and widespread occurrence of diseases and pests (Li et al., 2016). Endophytic bacteria, which survive and grow inside plant tissue, have been extensively studied for control of diseases and amelioration of stresses in a variety of plants (Kumar et al., 2017). The use of plant growth-promoting endophytic bacteria (PGPEB), can therefore be an effective tool for improving crop growth and productivity under non-stresses as well as challenging environmental conditions, including poor soil fertility.
Nitrogen fixation is an important source of N for many crops and both plant growth-promoting bacteria (PGPB) growing on the root surface and PGPEB facilitate plant N availability (Sur et al., 2010; Leghari et al., 2016; Oleńska et al., 2020). The crop productivity depends on N, and use of inorganic N for crop production is still increasing at a global scale (FAO, 2020b) and it undermines efforts to mitigate climate change. The utilization of biological N fixation (BNF) to minimize the input of N fertilizers in sugarcane has been reported, though the BNF microbes are not well characterized. Different bacterial genera such as Azotobacter, Azospirillum, Bacillus, Pseudomonas, and Enterobacter, have been shown to be associated with BNF (Martins et al., 2020). The use of PGPEB to improve crop production may have advantages over epiphytic and rhizosphere bacteria as they are more vulnerable to soil and other external conditions (James, 2000). These bacteria produce several phytohormones (auxin, cytokinin, ethylene, and gibberellins) and growth-enhancing compounds (siderophore, hydrogen cyanide, fixed N, and hydrolyzing enzymes) and can be used as biofertilizers (Stefan et al., 2013; Mihalache et al., 2015; Li et al., 2017; Raklami et al., 2019). Our previous research showed BNF in certain varieties of commercially grown sugarcane, and we have identified some rhizobacteria involved in BNF from those varieties (Li et al., 2017; Singh et al., 2020b,c). This research is now extended to study the endophytic bacteria from sugarcane roots, to assess their plant growth-promoting (PGP) potential, including their ability for BNF.
Here, we report the isolation and characterization of non-pathogenic endophytic Pantoea and Kosakonia diazotrophic bacteria colonized in sugarcane roots. The genera Pantoea and Kosakonia, belong to Enterobacteriaceae family, are non-spore-forming, rod-shaped, and Gram-negative bacteria (Tambong, 2019). Members of Pantoea and Kosakonia genera are known to interact and elicit beneficial effects on plant growth (Brock et al., 2018; Chen and Liu, 2019; Romano et al., 2020; Singh et al., 2020b, 2021b; Suman et al., 2020), but little is known about their potential for disease control and N-fixation in sugarcane. Considering the widespread occurrence of diseases and the large reduction in N input required in sugarcane crops in China, we studied the disease control and BNF properties of members of Pantoea and Kosakonia genera, and the results are presented here.
Materials and Methods
Sampling and Endophytic Bacteria Isolation
Five sugarcane species, Saccharum officinarum L. cv Badila, Saccharum barberi Jesw. cv Pansahi, Saccharum robustum, Saccharum spontaneum, and Saccharum sinense Roxb. cv Uba were used for this study. All plants were obtained from the experimental farm of Sugarcane Research Institute, Guangxi Academy of Agricultural Sciences, Nanning, Guangxi (latitude 22° 50′ N, longitude 108° 14′ E, and elevation 70 m), China. The climate in the study region was humid subtropical, with an annual mean temperature of 21.83°C and 1,290 mm of rainfall. At the plant elongation stage, root samples were taken for the isolation of endophytic bacteria according to Dobereiner et al. (1993) method. The soil adhering to the roots was cleaned by thoroughly washing them with tap water, and then rinsed with sterile distilled water, 75% ethanol (5 min), and sodium hypochlorite solution (3% for 5 min). Five root samples were chosen from each sugarcane species. The root samples with white tips, which indicated continued growth, were used to isolate bacteria. Root pieces (1 gm per clone) were transferred to cold, sterilized mortal and pestles, and crushed with 1 mL of sterile 5% sucrose solution. An aliquot of 100 μL from each sample was spread on the different medium (Supplementary Table 1) and incubated for 3–5 days at 30 ± 2°C. After incubation, morphologically different bacterial colonies were chosen. The isolated strains were kept at −20°C in a 25% glycerol solution.
Identification of Endophytic Isolates and Analysis of nifH, acdS, and Hydrogen Cyanide Biosynthetic Genes
The strains were identified by analyzing their 16S rRNA gene sequences amplified from genomic DNA using universal primers pA and pH (Supplementary Table 2) (Edwards et al., 1989), and conditions as described by Singh et al. (2021b). Briefly, pure bacterial cultures were grown in Luria-Bertani (LB) broth for 48 h on a shaker incubator and maintained at 32 ± 2°C for 160 rpm. Genomic DNA was isolated from 1.5 mL broth culture using a DNA extraction kit (CWBIO, Beijing-China) following the manufacturer’s instructions. The PCR amplification of the 16S rRNA gene was completed and the amplified PCR products were purified by using a BioFlux kit (Hangzhou, China) and then sequenced by Sangon Biotech (Shanghai, China). Phylogenetic analysis was performed to verify identities and determine the evolutionary relationship of the isolates with reference strains from the GenBank public database and aligned by ClustalW. Phylogenetic trees were created by MEGAX for the 16S rRNA gene (Kumar et al., 2018) via Neighbor-Joining method (Saitou and Nei, 1987). The evolutionary distances were calculated by using the neighbor-joining method (Nei and Kumar, 2000). The bootstrap study (1,000 replicates) was carried out as described earlier (Felsenstein, 1985).
The primer sequences shown in Supplementary Table 2 were used for the amplification of nifH (Poly et al., 2001), acdS (Li et al., 2011), and HCN (Ramette et al., 2003) for all selected strains.
Acetylene Reduction Assay and 1-Aminocyclopropane-1-Carboxylate Deaminase Activity
In vivo nitrogenase activity of all bacterial isolates was determined by acetylene reduction assay (ARA) by inoculating pure culture in 10 mL of McCartney vial comprising semi-solid N-free medium and incubated at 30 ± 2°C for 48 h (Hardy et al., 1968).
1-aminocyclopropane-1-carboxylate deaminase (ACCD) activity of bacterial isolates was measured based on their capability to use ACC (3 mM) as a sole N source in the Dworkin and Foster (DF) salt minimal medium (Penrose and Glick, 2003). All strains were spot inoculated on (i) Petri plates comprising DF salts minimal medium containing ACC, (ii) DF minimal medium without of ACC (negative control), and (iii) DF minimal medium with (NH4)2SO4 (2 g L–1) (positive control). And, growth was compared with controls after incubation at 32 ± 2°C for 5–7 days. Strains that showed good growth on ACC plates were chosen for quantitative analysis, following Honma and Shimomura (1978) protocol.
Qualitative and Quantitative Evaluation of Plant Growth-Promoting Characteristics of Bacterial Isolates
Phosphate Solubilization
An aliquot of ∼10 μL of freshly produced bacteria was spotted on Pikovskayas agar medium to study the phosphate (P) solubilization capability (Hi-Media). The spotted plates were placed at 30 ± 2°C for 3–5 days and detected for the development of a clear zone around the bacterial colony.
For quantitative P-solubilization, each bacterial isolate was inoculated in 100 mL Erlenmeyer flasks containing 25 mL of Pikovskaya’s medium (≈108 CFU mL–1) and incubated in a shaker (180 rpm) at 30 ± 2°C for 72 h. Autoclaved medium (uninoculated) served as control. 20 mL of each culture was collected after 72 h of growth and centrifuged for 10 min at 13,000 g to obtain cell-free supernatants. The amount of phosphorus in the culture supernatant was measured using Fiske and Subbarow (1925). The pH of the bacterial broth was also measured with a digital pH meter.
Hydrogen Cyanide Production
The Hydrogen cyanide (HCN) produced by each endophytic bacterial isolate was determined by Lorck (1948) process. Briefly, pure bacterial isolate in LB medium with 4.4 g glycine L–1 was inoculated in 15 mL broth in a test tube. A sterile Whatman filter paper no. 1 soaked in picric acid (1%) solution was hung in the test tubes once dried. The test tubes were then closed with parafilm and kept for 5–10 days at 30 ± 2°C. After incubation, the change in the color of the filter paper, i.e., yellow to orange-brown, or reddish-brown shows the production of cyanide from bacterial strains.
Siderophore Production
To measure the siderophore production by bacterial isolates, both qualitative and quantitative methods were used. The capacity of bacterial isolates to produce siderophores was evaluated using the universal Chrome azurol S (CAS) agar medium (Schwyn and Neilands, 1987). The pure freshly produced all bacterial isolates were dotted on Petri plates with CAS medium and cultured for 4–5 days at 30 ± 2°C. The formation of an orange zone (hydroxamate-type siderophore) or a purple zone (catechol-type siderophore) surrounding the bacterial colonies on the plates was used to determine siderophore production by endophytic bacteria.
Quantitative estimation (hydroxamate-type siderophore) was completed by taking the supernatant of bacterial cultures grown in a broth of LB medium (Hu and Xu, 2011). Screwcap tubes (20 mL) containing 5 mL of LB broth were autoclaved. Afterward, 10 μL of freshly grown bacterial suspension (≈108 CFU mL–1) was inoculated, and the uninoculated broth was maintained as the control. After incubation at 30 ± 2°C for 3 days, bacterial cultures were pelleted by centrifuging at 12,000 rpm for 10 min. at 5°C, and the clear supernatant was used to determine the production of siderophore. 0.5 mL supernatant of each bacterial isolate was mixed with 0.5 mL CAS solution, kept in dark condition for 20 min, and measured its optical density at 630 nm by SPARK® multimode microplate reader (Model- SW Sparkctl. Magellan V2.2 STD 2PC, Austria). Payne (1993) method was used to determine siderophore production in percent siderophore unit (PSU).
Test for Colorimetric Indole-3-Acetic Acid (IAA) Detection
Indole-3-acetic acid synthesis capacity of all isolates were measured the colorimetric method of IAA quantitation as described by Gordon and Weber (1951). Overnight bacterial strains were cultured in LB broth medium at 32 ± 2°C with shaking at 180 rpm after inoculum was prepared (≈108 CFU mL–1). The inoculum was added in LB broth medium supplemented with L-tryptophan (0.5 and 1.0 g L–1) as the IAA precursor and incubated for 7 days at 30 ± 2°C. Subsequently, bacterial cells were separated by centrifugation, and the supernatant was used for quantitative measurement of IAA-producing bacteria by using Salkowski’s reagent.
Ammonia Production
Freshly cultured bacterial strains were grown in peptone water broth for 5 days at 30 ± 2°C and supernatant was used to measure ammonia production using Nessler’s reagent following the method described previously (Goswami et al., 2014). The concentration of ammonia was calculated using the ammonium sulfate standard curve, which ranged from 0.1 to 1 μ moL mL–1.
Biocontrol Assay of Endophytic Bacteria
Using the dual-culture method established by Singh et al. (2014), the chosen bacterial isolates were evaluated for in vitro antifungal activity against Fusarium verticillioides (FV), and Fusarium oxysporum f. sp. cubense (FOC). A fungal disk (5 mm) was placed in the center of a potato dextrose agar (PDA) and nutritional agar (NA) (1:1) plates and bacterial isolates suspended in LB broth (∼6 × 108 cell mL–1) were spotted 3 cm away from the fungal disk. Plates with fungal disks (without bacterial isolates) were applied as a control. The plates were maintained at 28 ± 2°C for 5–7 days. By comparing the development of fungal mycelia with and without the tested bacterial isolates, the antifungal activity was determined.
To examine the cell-free crude extract of chosen (dual-culture method) bacteria against the pathogens, strains were cultured in LB broth medium for 5–7 days at 120 rpm and 32 ± 2°C on an orbital shaker incubator. The bacterial cells were separated from the broth medium by centrifugation (14,000 rpm for 15 min at 4°C) and filtered using a sterilized membrane (0.22 m pore size; Merck Millipore Ltd.), and the filtrate was stored at −20°C until further use. The pathogen spores were scraped and suspended in sterile distilled water (10 mL) and diluted the spore suspension (106 CFU mL–1; colony-forming units), then distributed on Petri dishes comprising PDA. A 5-mm diameter hole was made into the medium with a cork borer, and the well was sealed with sterilized agarose (0.2%). Once the agarose was set and dried, 100 μL of cell-free culture filtrate and LB broth medium (control) was applied. The plates were incubated at 26 ± 2°C for 3–5 days.
Bacterial Production of Cell Wall Degrading Enzymes
Use of hydrolytic enzymes is a biocontrol strategy utilized by many microorganisms to restrict the development of fungal pathogens. In this study, the production of hydrolytic enzymes such as chitinase (MM1062O1), protease (MM1206O1), β- 1,3 glucanase (MM91504O1), and cellulase (MM91502O1) by selected strains was studied (Guo et al., 2020). A single strain of freshly grown bacteria was inoculated into 20 mL of LB medium and maintained at 32 ± 2°C for 36–48 h in an incubator shaker, after which the supernatant was centrifuged at 12,000 rpm for 10 min at 4°C and utilized for different enzyme activity measurement using enzyme-linked immunosorbent assays (ELISA) (Wuhan Colorful Gene Biological Technology Co. Ltd., China).
Scanning Electron Microscopy
Biocontrol interaction studied of the fungal plant pathogens and selected diazotrophs were done by scanning electron microscopy (SEM). A small piece of hyphae (∼3 mm) was cut at an interaction point into the plate and control plate only fungal pathogen, fixed in 2% glutaraldehyde for 4 h at room temperature, then rinsed three times with phosphate buffer (0.1 M, pH 7.4) for 15 min and after transferred into blocks with 1% OsO4 in 0.1 M PB (pH 7.4) for 1–2 h at 20°C. Then, blocks were washed with 0.1 M phosphate buffer (0.1 M, pH 7.4) for 15 min. The samples were desiccated with different concentrations of ethanol, i.e., 30, 50, 70, 80, 90, 95, and 100% for 15 min and at last with isoamyl acetate for 15 min. Samples were dried in a critical point dryer (model K850 Quorum) by a carbon sticker sputter-coated with gold-palladium for the 30 s. Samples were observed with SEM (HITACHI, SU8100, Japan). This technique is also used to study the colony morphology of selected diazotrophs as well as to verify the colonization in different tissues (root and leaf) of sugarcane plants.
Stress Resistance Test of Endophytic Bacteria
In vitro screening of endophytic isolates for abiotic stress tolerance was analyzed with a broad range of temperatures (20–45°C), pH (5–10), and different NaCl concentrations (7–12%) (Sharma et al., 2019, 2021). 100 μL (≈108 CFU mL–1) of fresh cultures were transferred in 5 mL LB broth medium and incubated in a gyratory shaker set at 120 rpm for 36 h at 32 ± 2°C, and growth was measured at 600 nm using SPARK® multimode microplate reader (Model- SW Sparkctl. Magellan V2.2 STD 2PC, Austria).
Colonization Pattern of Selected Diazotrophs in the Sugarcane Plant
Plasmid Transformation
For this experiment, we selected strains AF1 and EF1, the most potential isolates based on the above studies. Both isolates were resistant to ampicillin and taken as recipients with GFP-pPROBE-pTetr-OT tagging sensitive to kanamycin. Plasmid pPROBE-pTetr-OT comprising the green fluorescent protein (GFP) gene expressed under the Tetr promoter was inserted by biparental mating with donor strain Escherichia coli TG1 (Lin et al., 2012). The plasmid has a wide host range and could be quantified in both Gram-positive and Gram-negative bacteria. In an incubator shaker, the recipient and donor isolates were combined in a 1:2 ratio and maintained at 30 ± 2°C for 48–72 h. A 100 μL aliquot of the above mixture was spread onto LB agar plate and kept overnight at 30 ± 2°C and strains displaying green fluorescence under UV illumination were used for further study.
Inoculation of Micro-Propagated Sugarcane Plantlets
Micro-propagated sugarcane plantlets (variety GT42) were procured from Sugarcane Research Institute, Guangxi Academy of Agriculture Sciences (GXAAS), Nanning, China. Five separate plantlets were moved into a glass bottle containing 50–75 mL of MS liquid medium with sucrose and basal salt mixture. After, 3 days sugarcane plantlets were shifted in other autoclave bottles comprising GFP-tagged bacterial suspension (2.0 × 106 mL–1), and without suspension have been prepared for utilizing as a control. All plantlets were grown in a growth chamber at 30°C with a 14 h photoperiod at 60 μmoL m–2 s–1 photon flux density.
Laser Scanning Confocal Microscopy
Afterward, 4–5 days sugarcane plantlets both inoculated and un-inoculated were carefully removed from the bottles and washed with autoclaved distilled water, then dried at room temperature. Sugarcane plantlets tissues (root, leaf, and stem) were cut into small parts and mounted on a clean glass slide under a coverslip. Different tissues of sugarcane plantlets were viewed with a Leica DMI 6000 microscope (Mannheim, Germany) using a confocal scanning laser microscope (Olympus SXZ16) at different emission lengths.
Plant Inoculation Studies of Potential Diazotrophic Isolates, i.e., a Pot Experiment
Experimental Design and Treatments
The pot experiment was performed in January, 2020, at the Sugarcane Research Institute, GXAAS, Nanning. Sugarcane variety GT42 was used to establish the interactions between diazotrophic isolates and the host plant. The trial was completed in a greenhouse and the seed canes of test variety were obtained from Sugarcane Research Institute. A disease-free seed cane was used for this experiment. Hot-water treatments of sugarcane stalks (45–50°C for 2 h) were used to disinfect seed cane. Soil was collected from the top 2–20 cm soil depth from the experimental field sites of Sugarcane Research Institute and air-dried, crushed, and its physicochemical properties were analyzed. A plastic pot (30 cm diameter and 40 cm deep) holding 20 kg of soil and sand mixture (3:1 w/w) were used. Approximately similar size and shape of sugarcane plantlets at a three-leaf stage were carefully removed from nursery plants. And finally, the roots were washed slowly with flowing tap water then put in a tub and rinsed carefully until the root was cleaned. The experiment was accomplished in a complete randomized block design with five biological repeats and each pot contained two treated plantlets. The three treatments comprised of: (1) without inoculation-control (WI), (2) inoculation with P. cypripedii (AF1), and (3) Inoculation with K. arachidis (EF1). The diazotrophic bacteria were cultured in LB medium for 24–36 h (200 rpm, 32 ± 2°C) and centrifuged. Pellet was dissolved with autoclaved distilled water and prepared a bacterial cell suspension were adjusted at 108 CFU mL–1, and mixed with 1% autoclaved carboxymethyl cellulose (CMC) solution. All plantlets of sugarcane roots were immersed in CMC solution for 1 h. Following these treatments, plantlets were plotted into plastic pots and kept under controlled environmental conditions at 14 h day/10 h night; 60–70% relative humidity, and 26°C/20°C day/night temperature.
Plant’s Harvest, RNA Extraction, Purification, and cDNA Synthesis
Isolation of total RNA of root (100 mg) was done with Trizol reagent (Tiangen, China), and purified by RNeasy Plant Mini Kit (Qiagen), following the manufacturer’s commands. Extracted RNA samples were processed with DNase I (Promega, United States) to eliminate contaminating DNA and quantified using a Nano photometer (Pearl, Implen-3780, United States). The Prime-Script™ RT Reagent Kit (TaKaRa, Dalian, China) was used to synthesize single-stranded cDNA from 1 g of total RNA, consistent with the manufacturer’s guidelines.
cDNA Amplification
Amplification of the cDNA sequences employed the expression of aminomethyl transferase- AMT, nitrate transporter- NRT, Nitrate reductases- NR, Glutamate synthase- GS, Glutamine synthetase- GOGAT, N fixation-nifH, endo-glucanase- β-1, 4-GA, and chitinase- CHI, genes in the root tissues of sugarcane during plant-microbes interaction at tillering phase were analyzed in greenhouse condition after treatment with strains (AF1 and EF1) in GT42 sugarcane varieties with control plants. The primer sequences used in this study are presented in Supplementary Table 2. The qRT-PCR reaction mixture consisted of SYBR Premix Ex Tap™ II (TaKaRa, Japan), 10 μL of SYBR Premix, 1 μL of each primer (10 μM), 2 μL of RNA template (10 × diluted cDNA), and 6 μL of ddH2O in a total volume of 20 μL with five repeats in Real-Time PCR Detection System (Bio-Rad, United States). Amplification was started with a denaturation step of 94°C for 2 min, followed by 40 cycles of 94°C for 30 s, 60°C for 20 s, and 72°C for 30 s, and the last extension at 72°C for 2 min. To standardize qRT-PCR data, the housekeeping gene glyceraldehyde 3-phosphate dehydrogenase (GAPDH) was utilized as the reference gene, and the relative expression of all genes was quantified using the 2–ΔΔCt technique (Livak and Schmittgen, 2001).
15N Abundance Plant Analysis
The 15N isotope dilution method quantifies the biological N fixation in the GT42 sugarcane variety (Singh et al., 2020a). This method involves the testing of N-fixing crops with the inoculation of AF1 and EF1 isolates. Soil and sand were mixed with a ratio of 1:3 (w/w) and sterilized two times for 45 min at 121°C. After cooled at room temperature 10 mg ammonium sulfate-15N (10.12 percent atom 15N excess) per kg of soil was added and homogenized for proper distribution of 15N. Twenty kg of 15N soil-sand mixture was filled in the pots and each pot contains two treated sugarcane plantlets for GT42. This experiment was performed in a completely randomized block design with five biological repetitions, and comprised three treatments: (1) without bacterial inoculation (control) (2) inoculation with P. cypripedii (AF1), and (3) inoculation with K. arachidis (EF1) of each variety. At the tillering phase, plants were harvested and washed with distilled water to eliminate the soil attached to the roots and plants. Roots, leaves, and stems were separated and ground to a fine powder. Five milligrams of powdered root, leaf, and stem materials for all samples were analyzed for 15N isotope content using K05 automatic Kjeldahl N determination equipment (Shanghai Sonnen automated research instrument co. Ltd.), and elementary analysis was done by isotope ratio mass spectrometers (Thermo Fisher Delta V Advantage IRMS). The contribution of N derived from the air (Ndfa) in different tissues of all sugarcane varieties was calculated by Urquiaga et al. (1992).
Determination of Physiological Parameters and Enzymes Associated With Nitrogen Metabolism, and Biocontrol, and Phytohormone Analysis
The experimental plants were collected at the tillering stage. The growth parameters of sugarcane plants including plant height, fresh weight (root and shoot), leaf area (Cl 203 Handheld laser leaf area meter, Bio-Science), chlorophyll content (Chlorophyll meter; SPAD-502 Plus; Konica Minolta Inc.; Japan), net photosynthetic rate, transpiration rate, and stomatal conductance (LI-6,800 compact portable photosynthesis system) were recorded.
Also, the activity of different N-metabolism enzymes (glutamine synthetase-GS, NADH glutamate dehydrogenase, and nitrate reductase-NR), biocontrol-related enzymes (β-1,4 and β-1,3 glucanase-GLU, and chitinase-CHI) (Singh et al., 2021c), and hormones (Gibberellins- GA3, Indole-3-acetic acid- IAA, and Abscisic acid- ABA) were extracted (Singh et al., 2018) and analyzed by plant ELISA kit (Colorful Gene Biological Technology Co. Ltd., Wuhan, China) according to the manufacturer’s guidelines.
Statistical Analysis
All tests were carried out in three repetitions. The standard error was computed using mean values, and the statistical significance threshold was set at p ≤ 0.05. MS Excel 2016 was utilized for basic statistical analysis of data, i.e., means standard deviation, and bar graphs. Analysis of variance was used to evaluate the statistical significance of the experimental data, followed by multiple comparisons using Tukey’s HSD test. A heatmap was also prepared following the method described by to Babicki et al. (2016), a heat map was created.
Results
Isolation of Endophytic Bacteria
A total of 175 endophytic bacterial strains were isolated from the roots of five sugarcane species Among these, only 11 Gram-negative strains of two genera Pantoea and Kosakonia were selected based on partial sequencing of the 16S rRNA gene. Further, the increase in interest in both genera for their potential PGP and nitrogenase activities was also prompted us to select these strains for the study.
Molecular Identification of Endophytic Diazotrophic Bacteria
Sequencing of 16S rRNA Gene
In this present study, molecular identification of all diazotrophic isolates was accomplished through the amplification of 16S rRNA gene sequencing (∼1.4–1.5 kb) with the primer’s pA and pH. Using BLAST-N searches with type strain database, we found that all the strains belong to the genus Pantoea and Kosakonia with rRNA sequences similarity values at ≈97.77–99.58%. All sequences of the isolates were deposited to NCBI GenBank under MZ497007 - MZ497017 accessions numbers (Table 1).
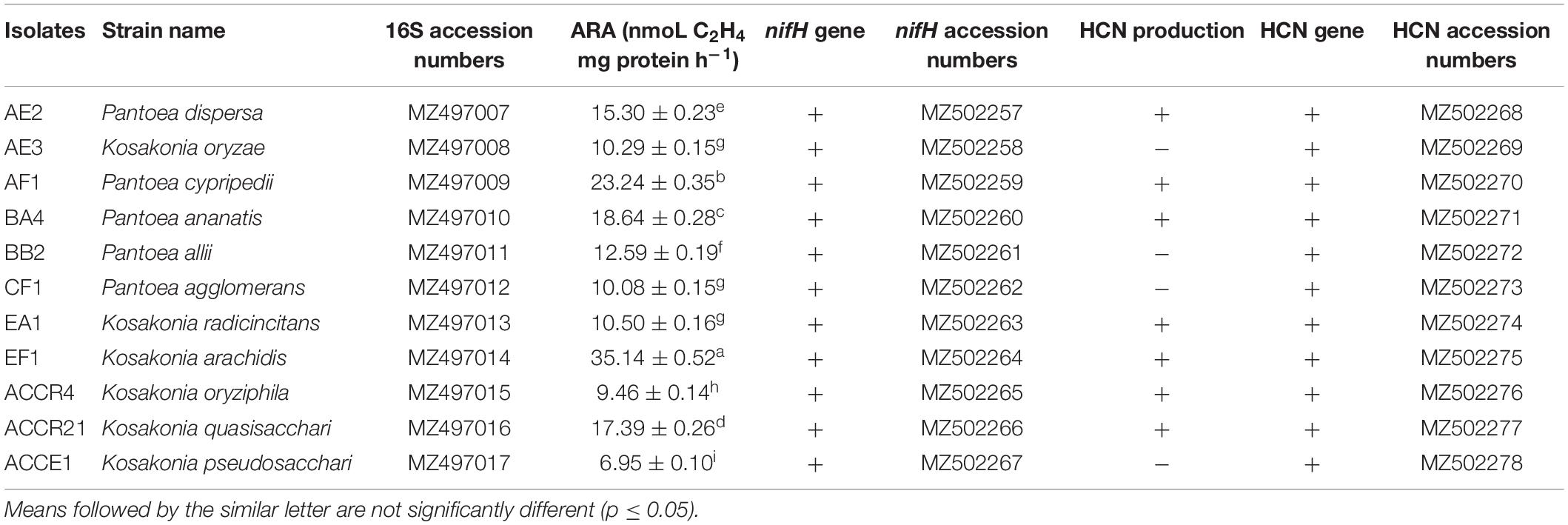
Table 1. Identification of selected isolates through 16S rRNA gene and their nitrogenase activity via acetylene reduction assay, hydrogen cyanide production, and the presence of gene amplification with an accession number.
Phylogenetic Analysis
The sequences of the 16S rRNA gene were aligned and used to build a phylogenetic tree using the neighbor-joining method. We found that all isolates were distributed into four major clusters using a total of 1,000 bootstrap samples of representative isolates compared to type strains of related taxa. According to this, all endophytic diazotroph bacterial isolates were grouped into different clusters with cluster I formed with two isolates (BA4- Pantoea ananatis and BB2- Pantoea allii), cluster II also formed with two isolates (AE2- Pantoea dispersa and AF1- P. cypripedii), cluster III formed with one isolate (CF1- Pantoea agglomerans), and cluster IV formed with six isolates (AE3- Kosakonia oryzae, EA1- Kosakonia radicincitans, EF1- K. arachidis, ACCR4- K. oryziphila, ACCE1- K. pseudosacchari, and ACCR21- Kosakonia quasisacchari) as shown in Figure 1.
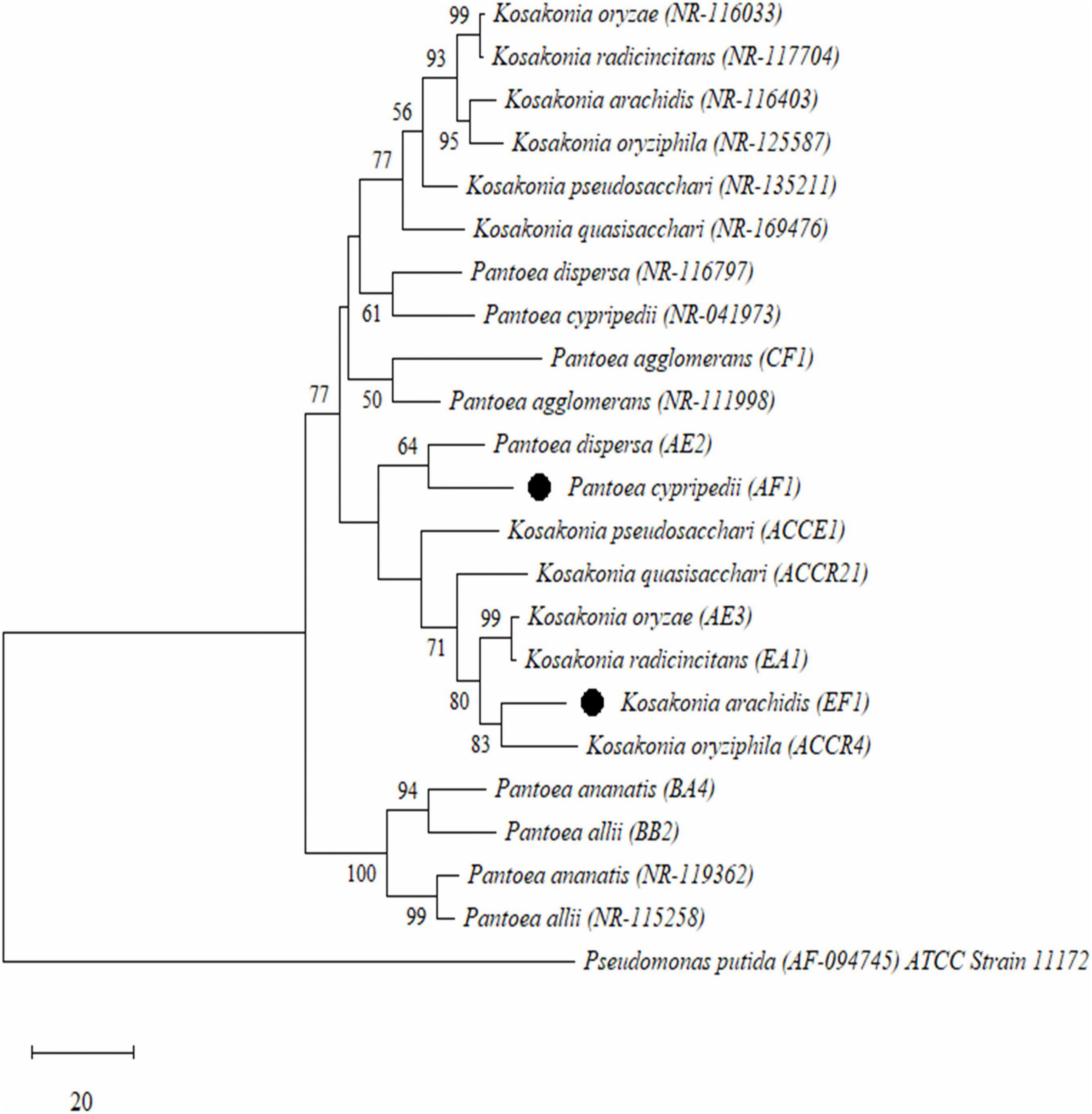
Figure 1. Phylogenetic tree analysis based on the partial 16S rRNA gene sequences of all selected Gram-negative endophytic nitrogen-fixing bacterial isolates isolated from sugarcane root. Scale bar means the number of changes per base location and Pseudomonas putida was used as an outgroup.
Detection of nifH, acdS, and HCN Genes
The PCR-based molecular detection of nifH, acdS, and HCN genes that promote plant development directly or indirectly was studied. Results showed that all strains had positive nifH gene amplification (Supplementary Figure 1A), and the evolutionary relationship of the nifH phylogenetic tree is presented (Supplementary Figure 1D). After sequencing, all sequences were matched with nifH gene sequences obtained from NCBI by BLASTN search. The nifH sequences of test isolates were submitted to NCBI GenBank with accession numbers MZ502257 – MZ502267 (Table 1).
Amplification of the acdS gene revealed that nine strains had ACC deaminase gene (Supplementary Figure 1B). The HCN gene was present in all 11 strains at ∼587 bp of amplification (Supplementary Figure 1C). All sequences showed 95–100% similarity with other HCN genes present in the NCBI database. Accession numbers of HCN genes of seven isolates are MZ502268 – MZ502278 (Table 1).
Nitrogenase Activity
The nitrogenase activity obtained from isolates varied from 6.95 ± 0.10 to 35.14 ± 0.52 C2H4 mg protein h–1 nmoL (Table 1). The maximum nitrogenase activity was obtained in EF1 followed by AF1, BA4, ACCR21, and AE2 (which is ≥15 nmoL C2H4 mg protein h–1, respectively). Isolate ACCE1 exhibited ≤10 nmol C2H4 mg protein h–1 level of nitrogenase activity as compared with other isolates (Table 1).
1-Aminocyclopropane-1-Carboxylate Deaminase Activity
1-aminocyclopropane-1-carboxylate deaminase activity was measured to determine isolates’ ability to utilize ACC as a nitrogen source to grow. After 4–5 days of incubation at 32 ± 2°C, all of the endophytic isolates were able to grow on medium supplemented with 3 mMoL L–1 of ACC (Table 2). Based on this result, the ACCD enzyme activity was determined quantitatively and the amount of α-ketobutyrate breakdown during ACC by the ACCD enzyme. It was observed that AF1, EF1, and AE2 isolates used maximum ACC and produced 1325.62 ± 19.67, 824.33 ± 12.23, and 676.70 ± 10.04 α-ketobutyrate μmoL mg–1 h–1, respectively (Table 2).
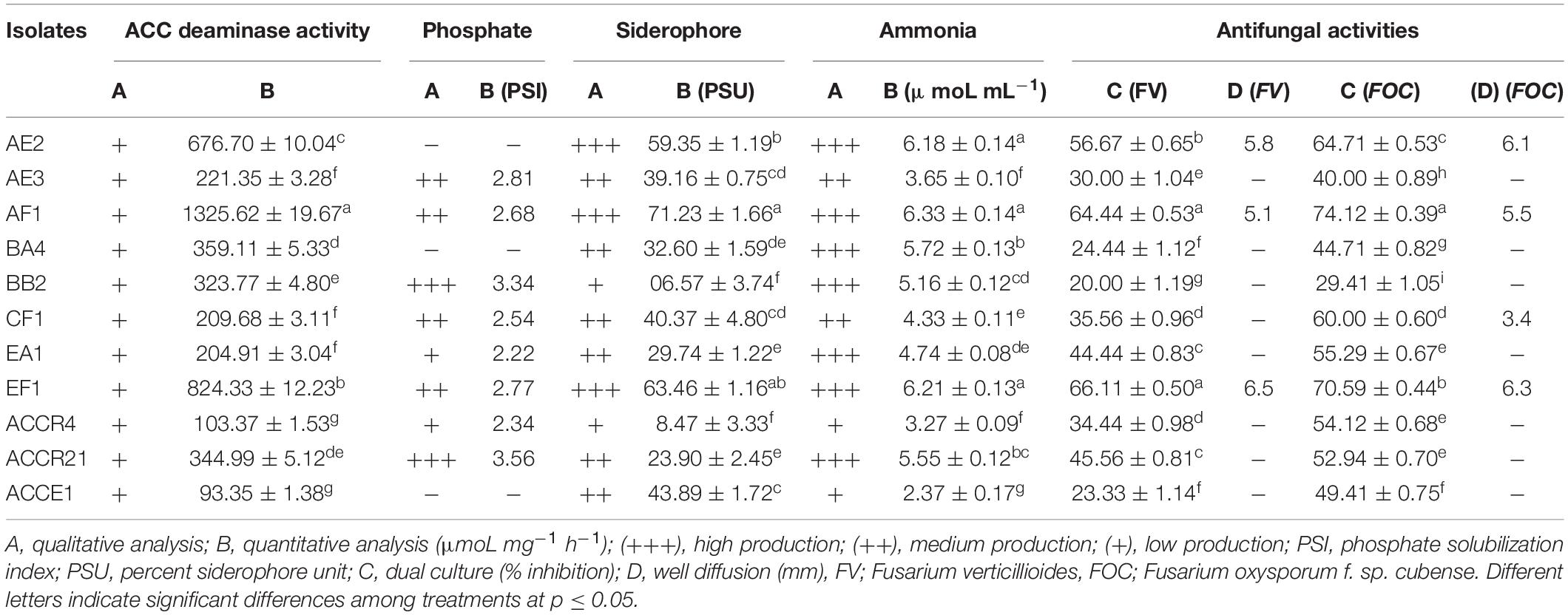
Table 2. In vitro screening for assessing the endophytic plant growth-promoting, 1-Aminocyclopropane-1-carboxylate deaminase activity and antifungal activities for selected bacterial isolates obtained from sugarcane root.
Plant Growth Promoting Traits of Endophytic Isolates
A total of 11 selected endophytic bacterial isolates (from AE2 to ACCE1) were tested for their potential PGP activities, such as the production of siderophores, phosphate, HCN, and ammonia (Tables 1, 2 and Supplementary Figure 2).
After 5–7 days of incubation, eight (73%) endophytic isolates displayed positive phosphate solubilization on the Pikovskaya agar medium plate containing Ca3(PO4)2, with a clear zone around the colony (Table 2). Isolates BB2 and ACCR21 showed phosphate solubilization index ≥3 PSI; while the other six (AE3, AF1, CF1, EA1, EF1, and ACCR4) isolates showed from 2.81 to 2.34 PSI (Table 2).
The HCN-producing ability of all endophytic isolates was tested. Seven (64%) isolates exhibited an orange-brown color of Whatman filter paper no. 1 (soaked in 2% sodium carbonate in 0.1% picric acid solution) that confirmed a positive result and four isolates (AE3, BB2, CF1, and ACCE1) could not produce HCN (Table 1).
In CAS agar plate media, an orange-colored halo zone formed around the colonies, indicating that bacterial strains were producing siderophores. All the bacterial isolates selected in the study produced siderophores, and strains AE2, AF1, and EF1 had the greatest capacity for siderophore production (Table 2). The concentration of siderophore generated by bacterial strains ranged from 06.57 ± 1.59 to 71.23 ± 1.66 PSU. The AF1, EF1, and AE2 isolates displayed the greatest production of siderophores (71.23 ± 1.66, 63.46 ± 1.16, and 59.35 ± 1.19 PSU) among the tested isolates (Table 2).
The quantitative estimation of IAA production of all endophytic isolates is shown in Figure 2. In the presence of L-tryptophan (0.5 and 1%), IAA production was higher but it showed considerable variation depending on the amount of tryptophan (100.35 ± 0.75–523.65 ± 9.50 and 71.42 ± 1.30–429.39 ± 7.79 μg mL–1). AF1, BA4, CF1, EF1, EA1, and ACCR4 strains displayed significantly higher amounts of IAA production with an increase in tryptophan concentration up to ≥100–432 μg mL–1 without addition of L-tryptophan. The maximum IAA production without supplementation of L-tryptophan in the medium (432.94 ± 7.85 μg mL–1) was found in CF1 isolate (Figure 2).
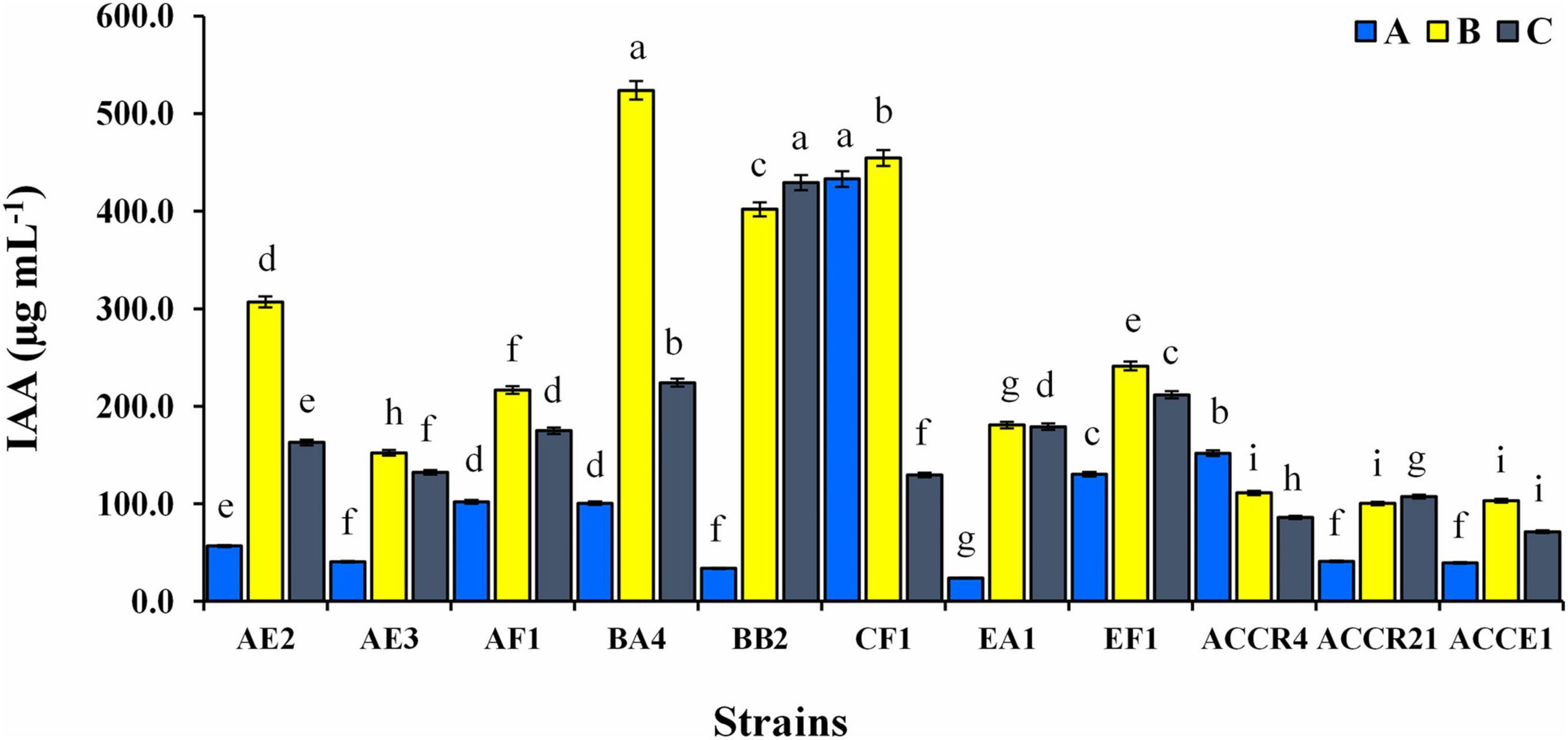
Figure 2. Indole-3-acetic acid production of endophytic bacterial isolates at different concentrations of L-tryptophan (A) absence of L-tryptophan and (B,C) presence of L-tryptophan (0.5 and 1.0 g L–1). Dissimilar lowercase letters show a significant difference at p ≤ 0.05.
Ammonia production is another important PGP feature of PGPEB. This feature was seen in all chosen endophytic isolates that used peptone as a substrate to produce ammonia (Table 2). AE2, AF1, and EF1 isolates produced maximum ammonia, which was 6.18 ± 0.14, 6.33 ± 0.14, and 6.24 ± 0.13 μmoL mL–1. Isolates BA4, ACCR21 and BB2, showed in descending rank depending on ammonia production capacity ≥5 moL mL–1 (Table 2).
Biocontrol Activity and Hydrolytic Enzymes Production of Endophytic Bacterial Isolates
The antagonistic potential of 11 identified endophytic strains (Pantoea dispersa, Kosakonia oryzae- 2, P. cypripedii, P. ananatis, P. agglomerans- 2, K. pseudosacchari, K. oryziphila, K. arachidis, and K. radicincitans) was examined against two fungal pathogens (FV and FOC). Results showed that reduction of FV mycelia by strains AE2, AF1, and EF1 was more than 55%, whereas AE2, AF1, CF1, EA1, and EF1 strains were able to reduce the growth of FOC pathogen. Among all, AF1 and EF1 strains showed (≥ 60%) maximum inhibition of both pathogens (Figure 3).
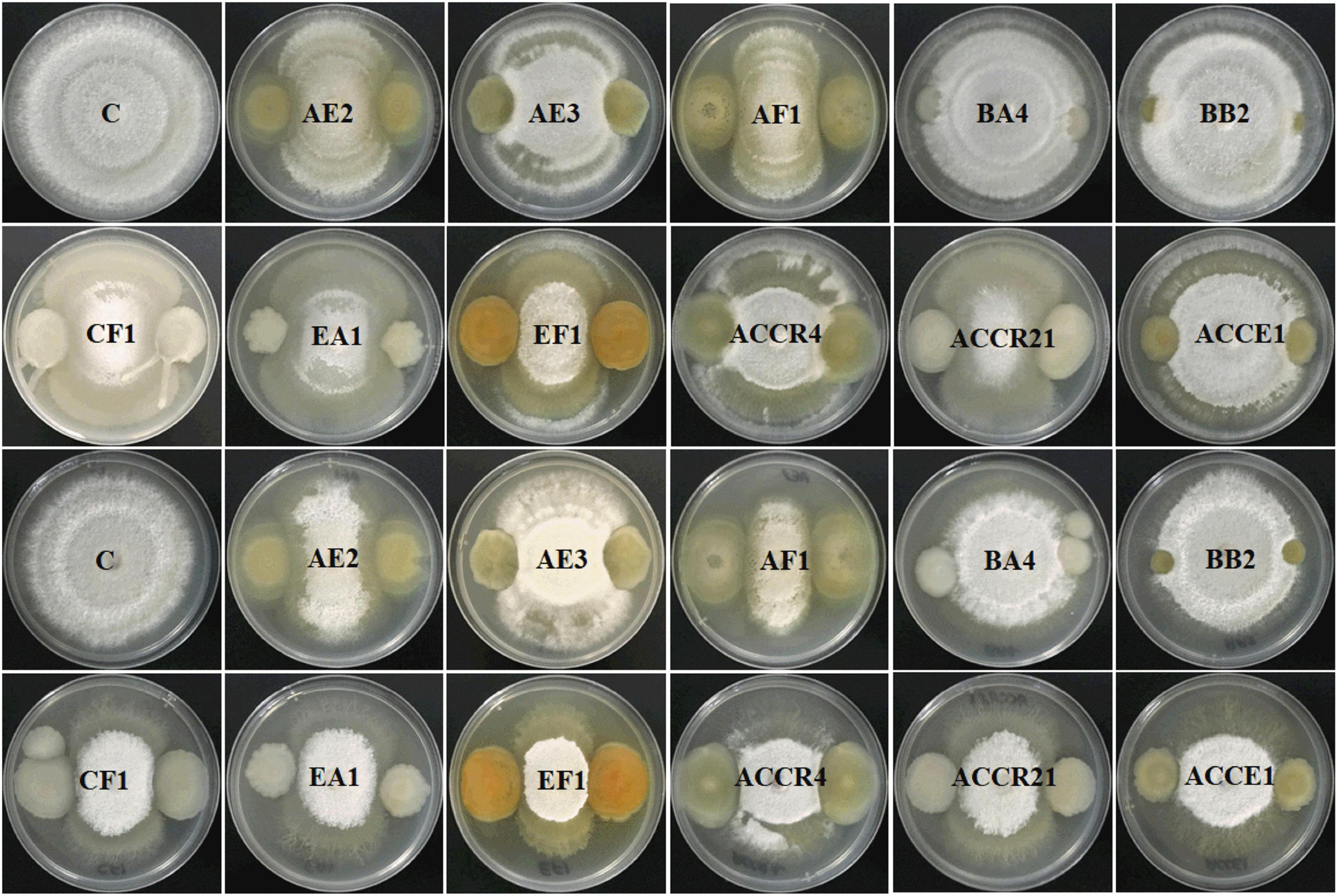
Figure 3. The activity of all selected Gram-negative endophytic antagonistic bacteria against Fusarium verticillioides and Fusarium oxysporum f. sp. cubense pathogens in dual culture and inhibition percentage by culture filtrate method.
Figure 4, showing the quantitative estimation of enzymes, i.e., chitinase, protease, cellulase, and endoglucanase of all selected endophytic strains. Endophytic isolates of sugarcane roots were able to generate a large quantity of hydrolytic enzyme activities, which ranged from 386.87–711.37, 137.39–239.85, 741.56–1363.02, and 1530.26–2388.28 IU mL–1, respectively. The strain AF1 showed the maximum enzymatic activities of all enzymes as compared to EF1 and other potential strains (Figure 4).
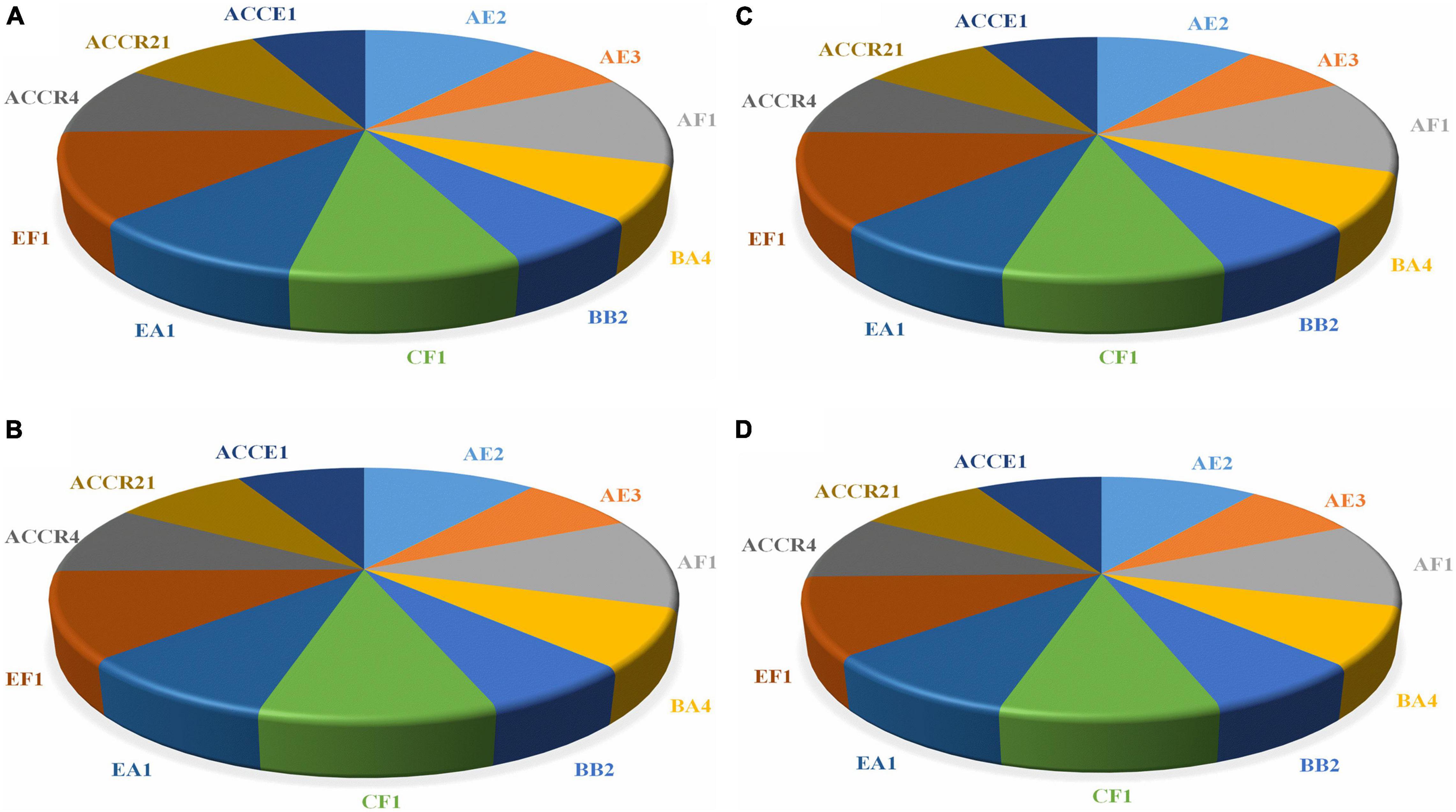
Figure 4. The endophytic isolates displaying different concentrations of hydrolytic enzymes activity and proficient to degrade the cell wall of fungal pathogens. (A) Chitinase, (B) Protease, (C) Cellulase, and (D) Endoglucanase.
Scanning Electron Microscopy of Bacteria-Fungal Pathogens Interaction
Inhibition of both pathogens’ growth by AF1 and EF1 isolates was further validated in vitro by SEM investigations (Figure 5). Control plates- without strains AF1 and EF1 had healthy mycelia of FV and FOC pathogens, i.e., with regular in shape and cylindrical (Figures 5A,D), whereas, morphological changes were observed in the mycelia of FV and FOC inoculated with pathogens AF1 and EF1 isolates had broken mycelial surface and fragmentation of mycelia (Figures 5B,C,E,F).
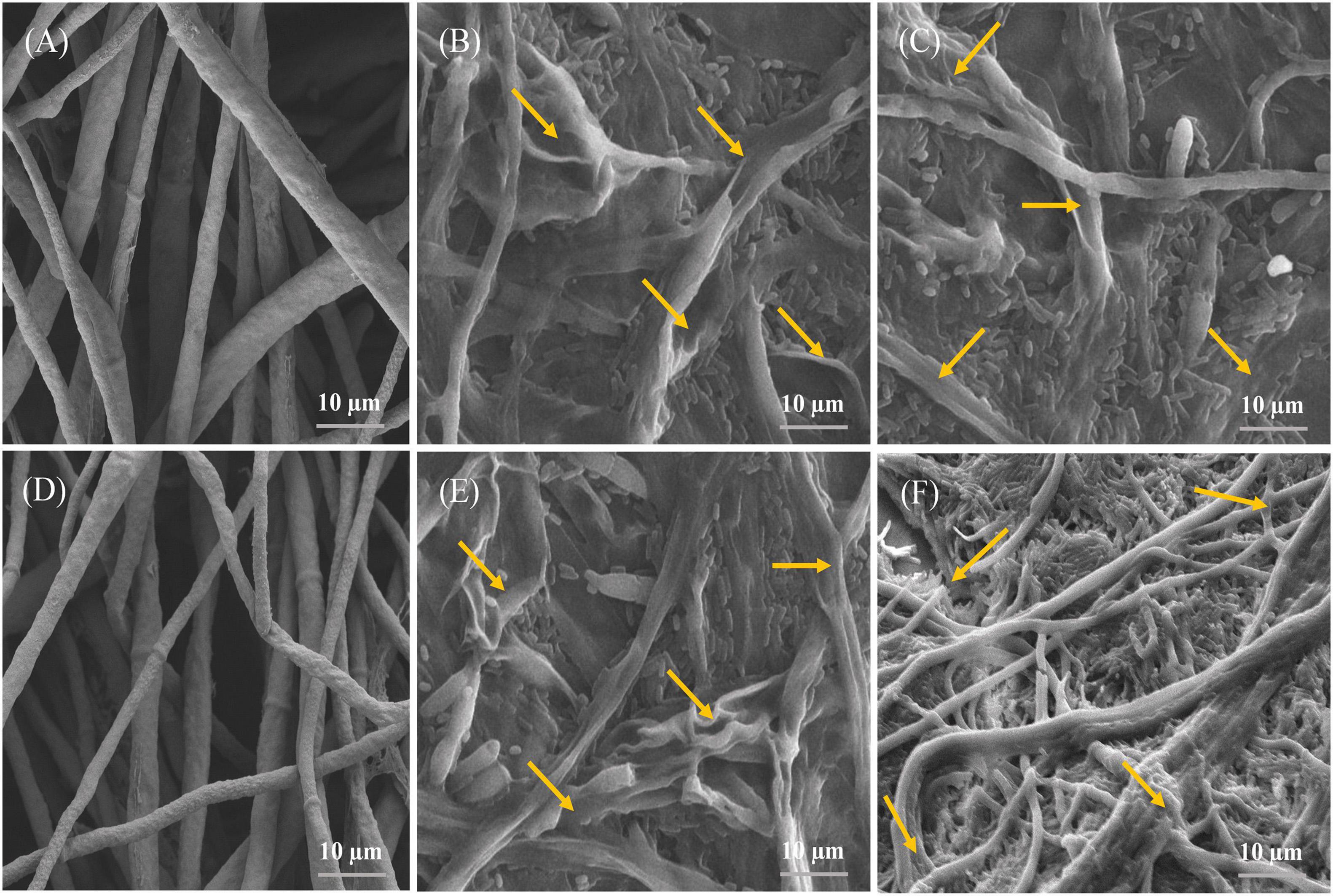
Figure 5. Images obtained by scanning electron microscopy of the antagonistic bacteria interacting with hyphae of selected fungal pathogens on NA: PDA medium after 5 days incubation. (A,D) indicating a normal hyphae of F. verticillioides and F. oxysporum f. sp. cubense, whereas (B,C,E,F), indicating abnormal hyphae of selected fungal pathogens.
Effect of Abiotic Stress Factors
In this study, growth was measured for all isolates in various abiotic stress environments, i.e., temperature (20–45°C), pH (5–10), and NaCl (7–12%) and the results are presented using a heatmap (Figure 6).
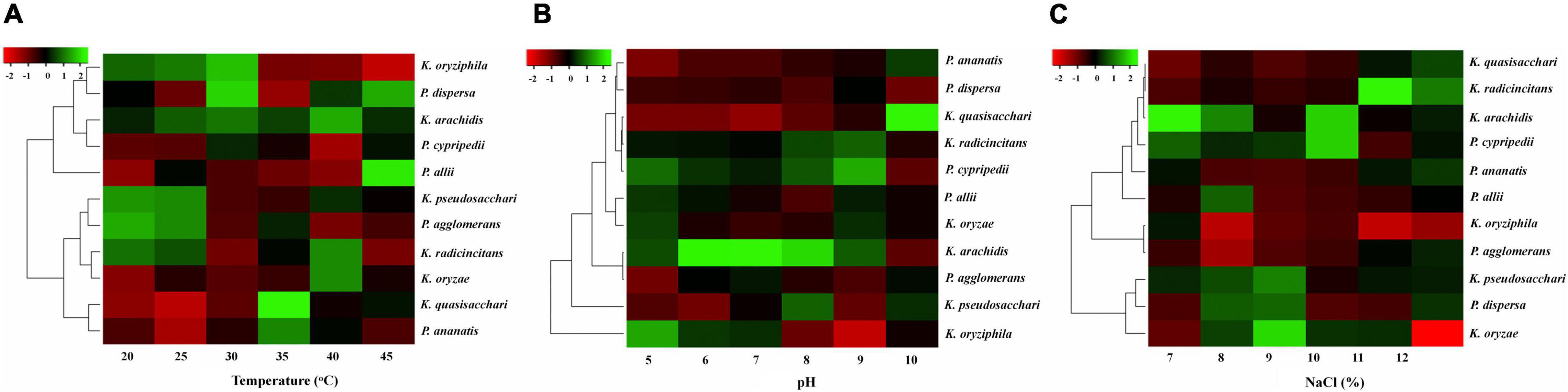
Figure 6. Effects of different abiotic stresses on selected isolates. (A) Temperature, (B) pH level, and (C) Different concentrations of salt.
All isolates showed maximum favorable growth at 25–35°C. However, at 40°C the growth of the isolates was decreased, and at 45°C growth was observed but decreased remarkably with only a few isolates showed little growth, as shown in Figure 6A. The result of diverse pH values (5–10) on the growth of all diazotrophs is shown in Figure 6B. All diazotrophs showed little growth at pH 5 but grew well from pH 6 to 9. Also, it was observed that all isolates showed optimum growth up to 7–8% NaCl concentration, but increasing NaCl concentration beyond that level (9–12% NaCl) decreased growth, and only a few strains showed very limited growth at 12% NaCl (Figure 6C).
Localization of Diazotrophs in the Sugarcane Plant
Scanning electron microscopy was also used to confirm the colony morphology and colonization of AF1 and EF1 in the root and stem of sugarcane plants (Figures 7A–H). In general, inoculated plants showed the colonization of bacterial isolates on the root and stem with high density and it was dispersed throughout the plant body. This result suggests that both diazotrophic isolates are aggressive endophytic colonizers of the sugarcane cultivated regions.
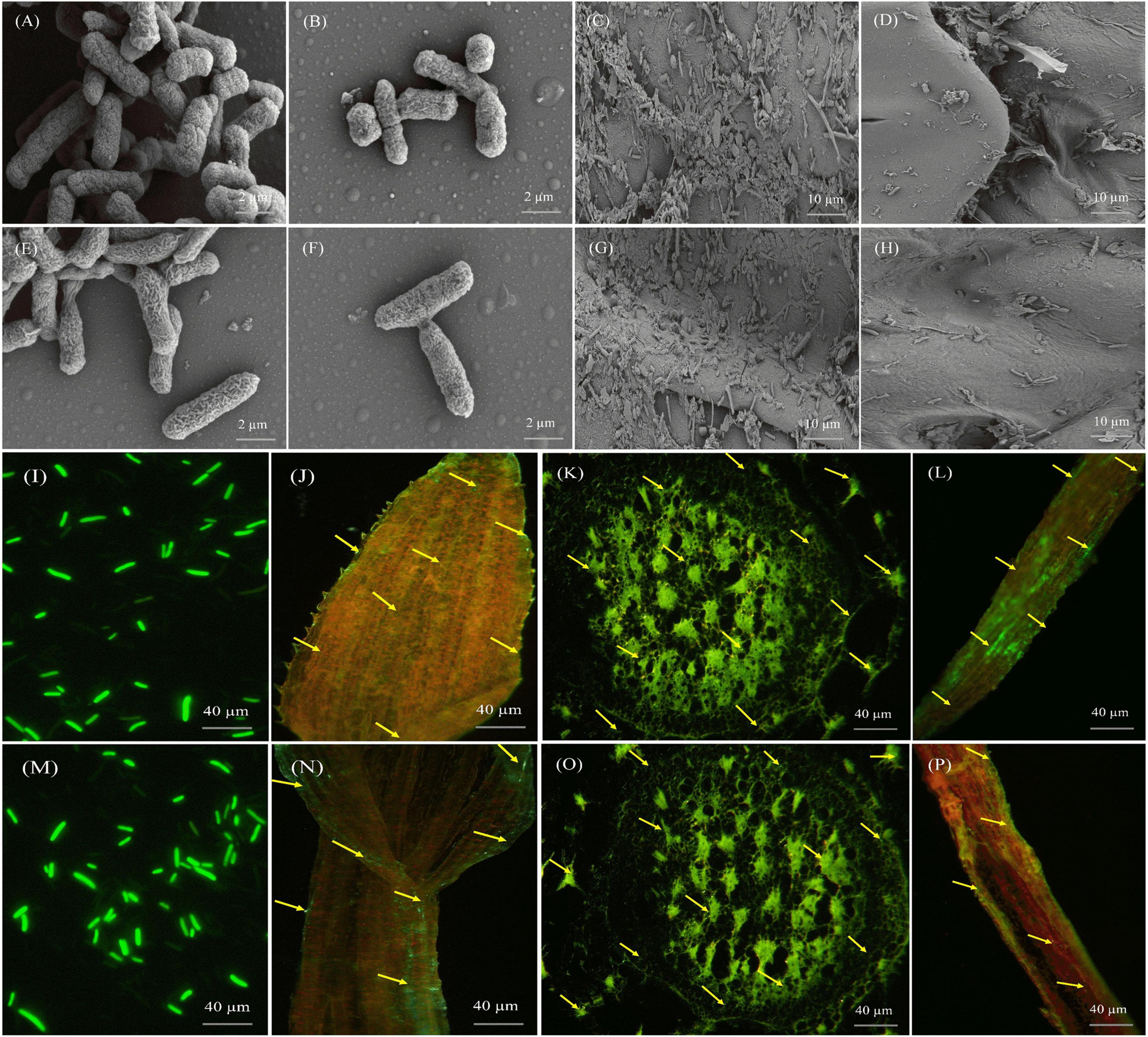
Figure 7. Microscopic pictures presenting the morphology and colonization of Pantoea cypripedii AF1 and Kosakonia arachidis EF1 in sugarcane (GT42). (A,B,E,F) Scanning electron micrographs (SEM) displaying morphology (rod-shaped) of AF1 and EF1 bacteria. (C,D,G,H) Colonization of AF1 and EF1 in root and stem tissues of sugarcane plant. (I,M) Confocal laser scanning micrographs (CLSM) displaying morphology of GFP tagged AF1 and EF1 strains. (J–L,N–P) Colonization of AF1 and EF1 strains as green dots in leaf, stem, and root tissues of sugarcane.
We also examined the colonization of AF1 and EF1isolates by CLSM in sugarcane plant tissues (root, stem, and leaf). Figure 7 clearly shows the colonies of bacterial isolates inside the root, stem, and leaf tissues. Both isolates colonized root hairs and showed their presence in root epidermal cells when inoculated separately. Green fluorescence of GFP-tagged bacterial isolates was detected in numerous cells in different tissues throughout the plant (Figure 7). At 40× resolution, GFP-tagged cells were localized in inter-cellular regions, within the vascular tissues, and in fissures at the points of lateral root emergence (Figures 7J–L,N–P).
Studies on N Metabolism and Pathogen-Control Related Genes
RT-qPCR was used to quantify the expression level of N metabolism (AMT, NRT, NR, GS, GOGAT, and NifH) and biocontrol (β-1,4-GA, and CHI) related genes in root tissues of sugarcane plant (Variety- GT42) after AE1 and EF1 inoculation (Figure 8). The results showed significant changes in the expression of all the analyzed genes in sugarcane after AE1 and EF1 inoculation as compared to control. Whereas AF1-inoculated plants showed higher expression levels of NRT, NR, GOGAT, NifH, β-1,4-GA, and CHI genes, and expression of AMT and GS was maximum in EF1inoculated plants (Figure 8).
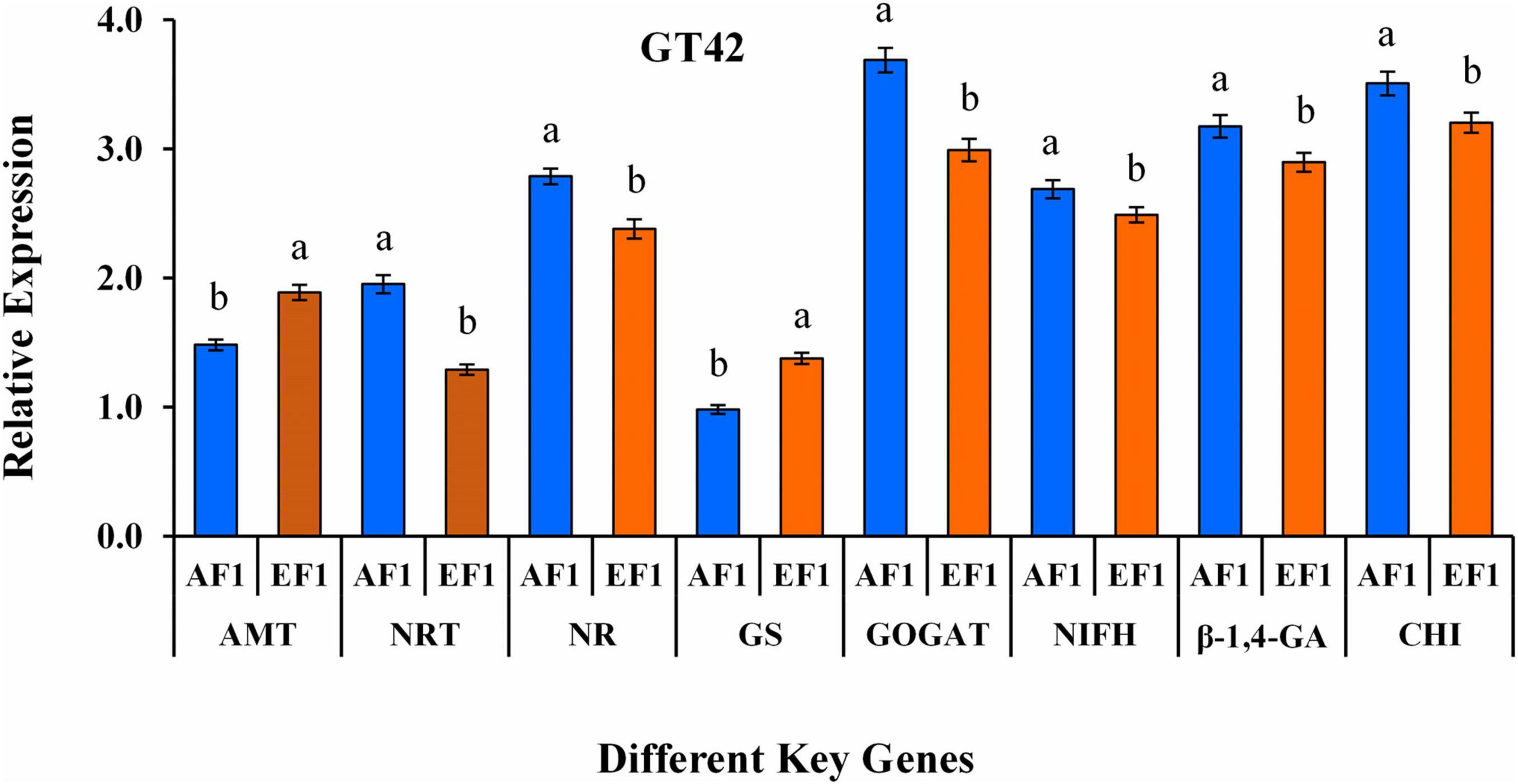
Figure 8. qRT-PCR study of aminomethyl transferase- AMT, nitrate transporter- NRT, nitrate reductases- NR, glutamate synthase- GS, glutamine synthetase- GOGAT, nitrogen fixation- nifH, endo-glucanase- β-1,4-GA, and chitinase- CHI genes in root tissue of sugarcane variety (GT42) inoculated with P. cypripedii AF1 and K. arachidis EF1 strains. The GAPDH expression level was used to standardize the data. All data points are viewed as the mean ± SE (n = 3). Different letters specify significant differences among the treatments at p ≤ 0.05.
Biological Nitrogen Fixation Contribution by the 15N Isotopic Dilution Method
15N isotope dilution method showed that N content was higher in sugarcane plants (GT42) after inoculation of AE1 and EF1 strains as compared to control plants (Figure 9). The maximum tissue concentration of N was found in roots than leaf and stem tissues, respectively (Figure 9A). Whereas, % 15N atom excess of sugarcane leaf was higher than the root and stem tissues after bacterial inoculation (Figure 9B). Therefore, biological N fixation in sugarcane by selected isolates was confirmed.
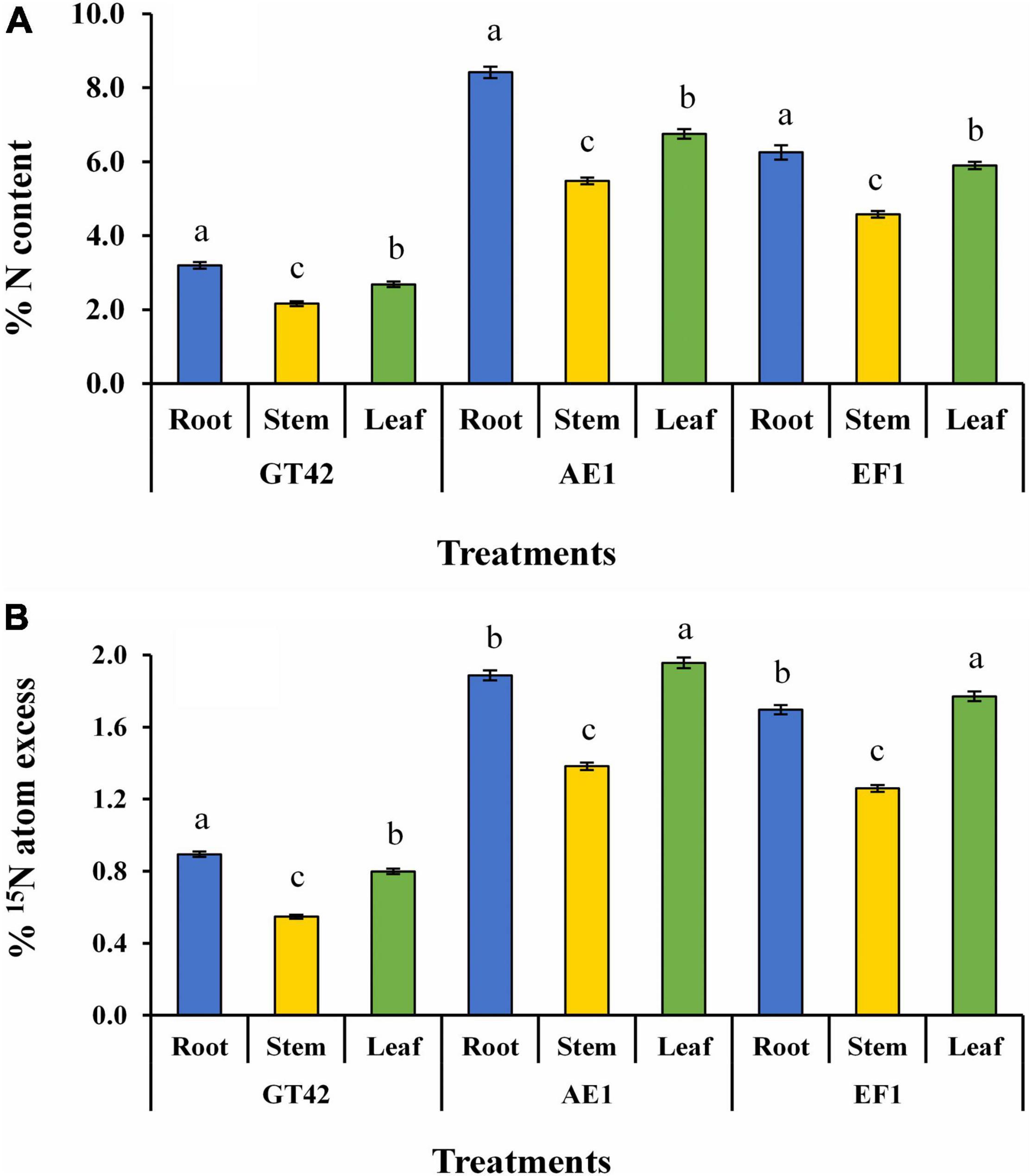
Figure 9. Estimation of biological nitrogen fixation in sugarcane tissues (dry matter yield of root, stem, and leaf) by P. cypripedii AF1 and K. arachidis EF1 cultivated in 15N-labeled soil (values are the average of three repeats) (A) % N content and (B) % 15N atom excess. Significant changes among treatments at p ≤ 0.05 are showed by dissimilar letters.
Greenhouse Assay for Diazotrophic Isolates
We measured the different physiological growth parameters such as plant height, root and shoot weight, leaf area, chlorophyll content, photosynthesis, transpiration rate, stomatal conductance, and total protein content of sugarcane (GT42) inoculated with (AF1 and EF1) strains under greenhouse conditions. As compared to control, isolates AF1 and EF1 showed increased plant height, root weight, and shoot weight, i.e., 46, 32, and 72% and 31, 169, and 123%, respectively (Table 3). Whereas, no more significant difference was observed in leaf area and total protein content of sugarcane (Table 3). Table 3 shows significantly higher chlorophyll content (SPAD values) in inoculated sugarcane leaves than in control plants. In general, the SPAD values were lower in EF1 than AF1. The photosynthesis rate was marginally higher in plants-inoculated with AF1 (19.82 ± 0.19 μ mol CO2 m–2 s–1) than those with EF1 (18.55 ± 0.18 μ mol CO2 m–2 s–1). And, it was also found that the transpiration rate in the sugarcane plant was significantly lower in EF1 than AF1 inoculated bacteria but as compared to the control plant both isolates increased up to 131 and 120% (Table 3). When comparing both strain’s stomatal conductance of sugarcane plants, there were no more significant differences observed, but over control, the observed percent increase values were 107 and 105 for AF1 and EF1 strains (Table 3). Overall, these results suggest that both selected diazotrophic isolates are effective in sugarcane growth enhancement, and the application of strain AF1 showed more significant improvement as compared to EF1 in GT42.
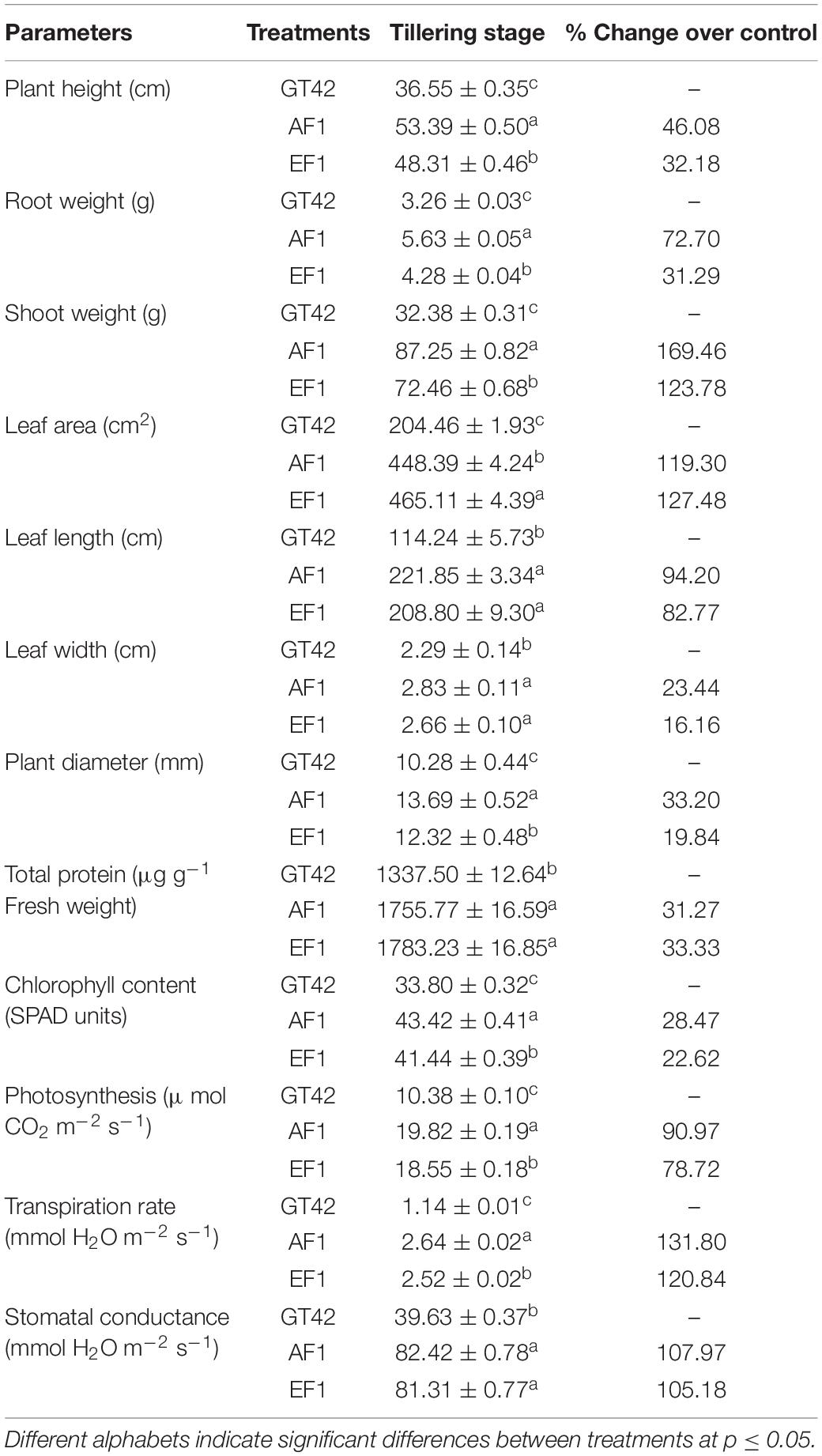
Table 3. The assessing of diazotrophic endophytes Pantoea cypripedii AF1 and Kosakonia arachidis EF1 inoculum on the sugarcane (variety GT42) in the greenhouse experiment.
Measurement of N Metabolism and Biocontrol Enzymes Activities
An increased level of selected N metabolism and biocontrol-related enzymes was observed in sugarcane plants (GT42) treated with diazotrophs (AF1 and EF1) (Figure 10). The plants inoculated with AF1 and EF1 showed 61 and 68% greater GS activity than control plants (Figure 10A), whereas, increase in NADH-GDH content was higher in AF1 (43%) inoculated plants than EF1 (36%) as compared to their controls (Figure 10B). Following inoculation with isolates, NR activity was higher in EF1 and AF1 inoculated plants (66.56 ± 2.11 and 62.78 ± 1.33 n mol–1 h–1mg proteins) than control (52.39 ± 0.98 n mol–1 h–1 mg proteins) (Figure 10C). Inoculation with endophytic diazotroph EF1 and AF1 significantly enhanced 19 and 16% β-1,4-glucanase activity in the root as compared to non-inoculated sugarcane plants (Figure 10D). A similar pattern of β-1,3-glucanase activity was observed in sugarcane inoculated with both isolates (AF1 and EF1) (Figure 10E). A significant increase in chitinase activity (28 and 38%) was observed in roots of GT42 inoculated with AF1and EF1 as compared to non-inoculated sugarcane plants (Figure 10F).
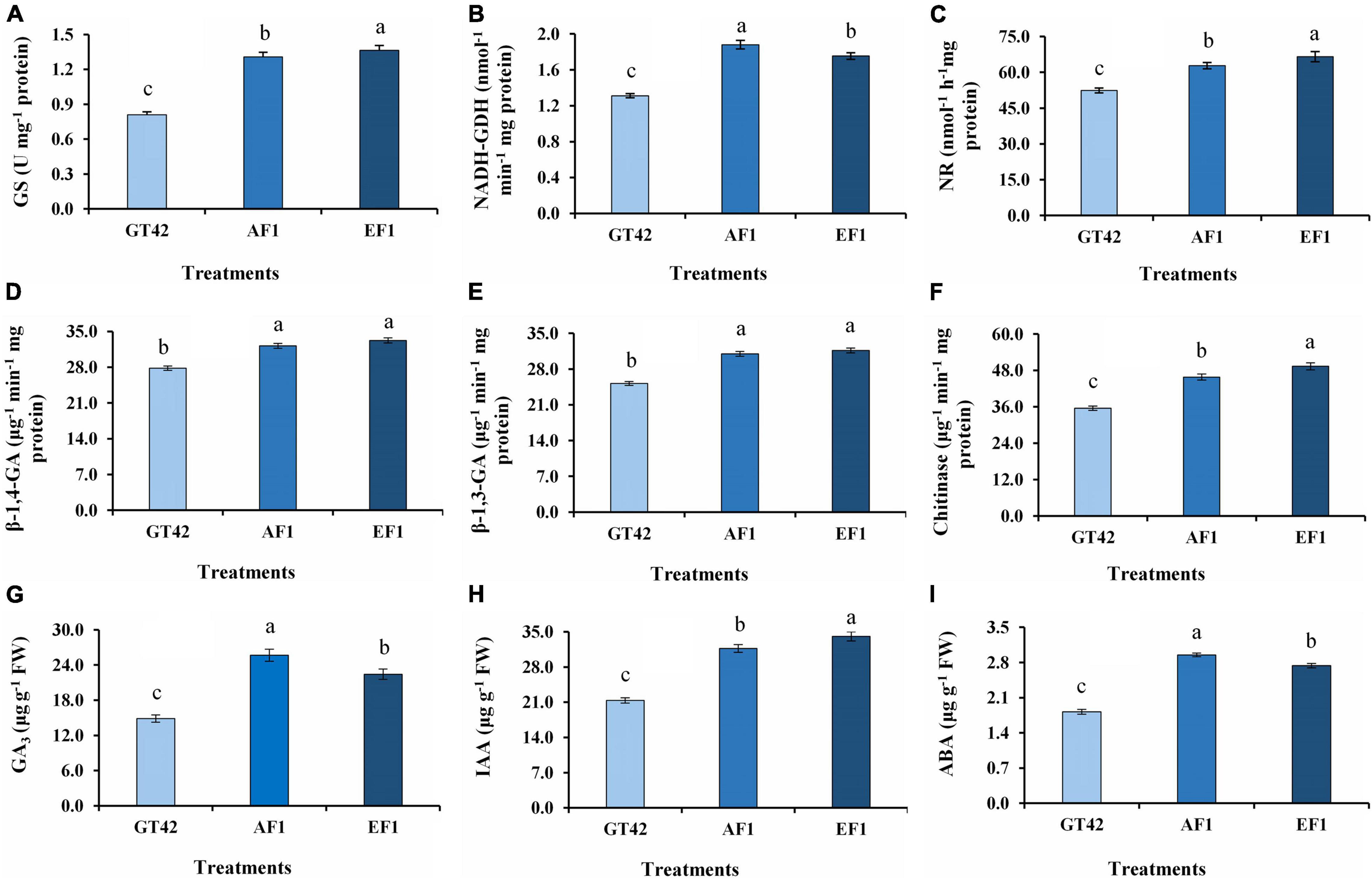
Figure 10. Analysis of N-metabolism enzymes, hydrolytic enzymes, and hormone activities in root tissues of sugarcane (GT42) inoculated with P. cypripedii AF1 and K. arachidis EF1. (A) Glutamine synthetase, (B) glutamate dehydrogenase, (C) nitrate reductase, (D,E) β-1,4 and β-1,3 glucanase, (F) chitinase, (G) gibberellins, (H) indole-3-acetic acid, and (I) abscisic acid. Different lowercase letters display a significant difference at p ≤ 0.05.
In this study, the significant difference in GA3, IAA, and ABA level was observed between the roots of bacteria-inoculated and uninoculated sugarcane plants (Figure 10). The treatment of plants with AF1 and EF1 increased GA production in root by 72 and 51%, respectively, as compared to control (Figure 10G). The IAA production in the root was increased more with the EF1 inoculation (Figure 10H). EF1 and AF1 inoculation increased IAA content by 59 and 48%, respectively, in inoculated plants, as compared to the non-inoculated plants. ABA production by sugarcane plants inoculated with AF1 and EF1 strains in 62 and 50%, respectively, higher compared with the non-inoculated plants.
Discussion
Diazotrophs, known for their BNF properties, also produce antibiotics, HCN, and siderophores, as well as build systemic resistance in plants, to inhibit a wide spectrum of plant fungal pathogens (Das et al., 2017; Ali et al., 2020). Hence, the development of diazotrophic bacteria as a biofertilizer with multi-functional traits might help boost plant growth and crop production. In a previous survey of sugarcane-producing regions in Guangxi, China, Lin et al. (2012) found that Klebsiella is the most abundant plant-associated N-fixing bacteria. Thus, the screening and selection of different diazotrophic bacteria with PGP activities will be valuable for sustainable sugarcane agriculture, especially in low fertile soils. In this research, a culture-independent approach was used to discover the potential Gram-negative endophytic diazotrophic bacteria along with PGP features for their potential application as biofertilizers in sugarcane. A total of 175 endophytic isolates were isolated and after 16S rRNA gene sequencing, we selected 11 strains of genus Kosakonia (K. oryzae, K. radicincitans, K. arachidis, K. oryziphila, K. quasisacchari, and K. pseudosacchari) and Pantoea (P. dispersa, P. cypripedii, P. ananatis, P. allii, and P. agglomerans). A number of different diazotrophic bacterial species isolated from sugarcane can also be opportunistic pathogens, but these microbes usually do not cause diseases in a healthy host (Fishman, 2013; Berg et al., 2014). The strains selected in this study also showed abiotic stress tolerance properties as they grew on a wide range of temperatures (20–45°C), pH (5–10), and salinity (7–12% NaCl), suggesting that these isolates may tolerate stressful crop production environments. Two strains P. cypripedii AF1 and K. arachidis EF1, which exhibited multiple PGP traits, were selected for detailed study.
The use of N-fixing beneficial microbes in agriculture might reduce the usage of chemical N fertilizers in agriculture, and reducing their negative environmental impacts (Noar and Bruno-Bárcena, 2018). Both selected strains showed the presence of nifH gene, which encodes the nitrogenase reductase enzyme. We observed the enhanced expression of N metabolism (AMT, NRT, NR, GS, and GOGAT) and BNF-related genes (nifH) in sugarcane after inoculation with AF1 or EF1 strains. AMT and NRT facilitate nitrate-N and ammonium-N uptake in the roots (Singh et al., 2016). The expression of NRT, NR, and GOGAT was higher in EF1-inoculated GT42 roots compared with AF1-inoculated ones with, indicating the strain variability for plant N metabolism regulation. Nitrate is taken up by roots and converted into glutamine and glutamate, which are used by plants to make other amino acids and nitrogenous compounds (Pratelli and Pilot, 2014). The primary assimilation of nitrate-N and ammonium-N in plants is mediated by two important N-assimilation enzymes, NR and GS (Funayama et al., 2013). The activities of N metabolism-related enzymes (GS, NADH-GDH, and NR) in AF1- and EF1-inoculated plants show that these bacteria had considerably increased N use efficiency in sugarcane (Figure 10). Glutamate synthase is a vital enzyme in N assimilation, metabolism, and remobilization, and its activity is influenced by environmental factors (Miflin and Habash, 2002; Stitt et al., 2002). It was observed that the activity of GS was higher in EF1 than that observed for AF1 (Figure 10). The high concentration of GS1 transcripts in sugarcane leaves implies that this isoform plays an important role in C4 plants for N metabolism (Nogueira et al., 2005). Ammonia production by PGP diazotrophic isolates contributes N to the host plants and supports biomass production (Marques et al., 2010). Our results showed that all Gram-negative endophytic bacteria produced ammonia, with P. cypripedii AF1 and K. arachidis EF1 being more efficient than the other isolates.
The technique of 15N isotope dilution has been frequently utilized to measure the contribution of associative N-fixing systems to graminaceous crops like sugarcane (Urquiaga et al., 1992; Asis et al., 2002; Montañez et al., 2009; Thaweenut et al., 2011; Lin et al., 2012; Li et al., 2017; Singh et al., 2020c,2021b). This study was performed in pots that used total N balance and enriched 15N fertilizer isotope-dilution methods, and the outcome was impressive. The findings of the 15N enrichment indicated that there was a significant amount of non-labeled N present, which could only have originated from BNF (Martins et al., 2020). Previous, investigations have found that Brazilian sugarcane cultivars were able to acquire 40–100 kg N ha–1 yr–1 from BNF utilizing plants without any treatment. A significant development of plant growth and N accumulation using diazotrophic bacteria was also observed in different sugarcane varieties (Li et al., 2017; Singh et al., 2020b). However, even after three decades of research, our understanding as to how the diazotrophs work, fix N, and transfer the fixed N to the crop remains limited (Martins et al., 2020).
Plant growth and crop productivity can be improved by siderophore-producing diazotrophic bacteria by improving Fe accessibility and boosting plant growth (Ahmed and Holmström, 2014). As expected, all chosen strains were capable of generating iron-chelating siderophores at various levels, and this trait is usually present in Pantoea and Kosakonia genera (Chen et al., 2017; Lambrese et al., 2018; Romano et al., 2020). Also, in this study, only eight diazotrophic isolates (73%) were able to solubilize phosphate. In the soil, Phosphate (P) is present abundantly in most a crop land but mostly unavailable to the plants, hence this is one of the main growth-limiting nutrients for plants in agricultural systems. It’s also critical for root formation, early shoot growth and tillering, early productivity, and plant stem elongation. Previously, a few strains of genus Kosakonia with P solubilizing ability were reported (Chakdar et al., 2018; Singh et al., 2020b). And, Chen and Liu (2019), studies found the genus Pantoea a highly effective P-solubilizing bacterium.
Hydrogen cyanide is a volatile secondary metabolite generated by several endophytic bacterial isolates involved in disease suppression and protection of the plants, particularly from fungal pathogens (Olanrewaju et al., 2017). The results showed that only 63% of endophytic isolates produced HCN. Strains that tested positive for HCN production were shown to be effective against sugarcane pathogens in this study. In many respects, these PGP results are similar to previous studies (Li et al., 2017; Guo et al., 2020; Singh et al., 2021a).
The sugarcane plant is affected by several fungal pathogens, therefore we screened 11 endophytic isolates for the antifungal property. Among them two selected strains showed good antagonistic activity against fungal pathogens, which was further corroborated by the degradation of fungal mycelia seen during SEM analysis. This finding supports earlier observations that, some diazotrophic bacterial strains isolated from sugarcane can suppress the plant fungal pathogens (Li et al., 2017; Guo et al., 2020). Additionally, all isolates were also tested for an important role in the production of hydrolytic enzymes such as chitinase, protease, cellulase, and endoglucanase. The role of these enzymes in biological control of various fungal pathogens has been previously reported (Huang et al., 2005; Singh et al., 2013; Passari et al., 2017, 2018). Pot experiment also showed the interaction of P. cypripedii AF1 and K. arachidis EF1 strains enhanced the production of CHI and GLU in root tissues of sugarcane. Similarly, CHI and GLU produced by different strains of genera Pantoea and Kosakonia improved sugarcane growth (Quecine et al., 2012; Singh et al., 2021b). The colonization of endophytic bacteria on plant tissues is also playing a crucial role in disease management and plant growth enhancement (Li et al., 2017; Guo et al., 2020). We examined the colonization of selected endophytic strains individually with genetically tagged GFP and found that both strains effectively colonized all plant tissues.
Our findings revealed a substantial influence of diazotrophic bacterial inoculation of sugarcane plant (GT42) with GA3, IAA, and ABA content. Sugarcane inoculated with selected isolates had considerably greater levels of all phytohormones than GT42 control. These hormones promote plant growth, root development, fertilizer absorption and assimilation, water uptake and a variety of metabolic processes in response to abiotic and biotic stresses (Graham, 2003; Stepanova et al., 2007; Singh et al., 2019). 1-aminocyclopropane-1-carboxylate deaminase activity is one of the principal mechanisms of endophytic bacteria that can assist plant growth in the presence of various biotic and abiotic stresses. All endophytic isolates selected in this study synthesized ACC deaminase, and the presence of acdS gene was confirmed in eight isolates. The reason for the inability to detect this gene in three isolates remains unclear. Endophytic bacteria protect host plants from a range of stress conditions by generating chemicals and enzymes such as ACC deaminase to decrease ethylene production (Glick, 2014; Mercado-Blanco and Lugtenberg, 2014). Apart from conferring stress tolerance, ACC deaminase also promotes root growth, which will have a positive effect on healthy shoot development (Belimov et al., 2002; Glick et al., 2007). Bacterial isolates with ACC deaminase activity have been reported, but it did not show the presence of acdS gene (Nascimento et al., 2014).
Conclusion
Despite the variety of bacterial species identified in sugarcane-producing habitats, there is little information regarding the diversity of Gram-negative bacteria, their ecology, and biotechnological potential in commercial sugarcane production. The significance of this study is highlighted by the promotion of plant growth and development by the Gram-negative endophytic diazotrophs P. cypripedii AF1 and K. arachidis EF1, and this is the first report to show that these two diazotrophic bacteria reside in sugarcane roots and potentially contributing to sugarcane growth, development, and disease suppression. These isolates exhibited a variety of PGP characteristics, including antifungal action against plant pathogens, N fixation, and the synthesis of enzymes and phytohormones. Further research into these diazotrophic isolates is needed to evaluate their commercial value as bio-fertilizers for improved sugarcane agricultural productivity.
Data Availability Statement
The datasets presented in this study can be found in online repositories. The names of the repository/repositories and accession number(s) can be found in the article/Supplementary Material.
Author Contributions
RS, PS, and Y-RL designed the experiments. RS, PS, and D-JG conducted the majority of the experiments. RS and PS wrote the article. PL, L-TY, and Y-RL reviewed and editing the article. The other authors assisted in experiments and discussed the results. All authors read and approved this manuscript.
Funding
This research was funded by the Guangxi Innovation Teams of Modern Agriculture Technology (nytxgxcxtd-2021-03-01) and Fund of Guangxi Academy of Agricultural Sciences (2021YT011).
Conflict of Interest
The authors declare that the research was conducted in the absence of any commercial or financial relationships that could be construed as a potential conflict of interest.
Publisher’s Note
All claims expressed in this article are solely those of the authors and do not necessarily represent those of their affiliated organizations, or those of the publisher, the editors and the reviewers. Any product that may be evaluated in this article, or claim that may be made by its manufacturer, is not guaranteed or endorsed by the publisher.
Supplementary Material
The Supplementary Material for this article can be found online at: https://www.frontiersin.org/articles/10.3389/fmicb.2021.774707/full#supplementary-material
References
Ahmed, E., and Holmström, S. J. (2014). Siderophores in environmental research: roles and applications. Microbial Biotechnol. 7, 196–208. doi: 10.1111/1751-7915.12117
Ali, J. G., Casteel, C., Mauck, K., and Trase, O. (2020). Chemical ecology of multitrophic microbial interactions: plants, insects, microbes and the metabolites that connect them. J. Chem. Ecol. 46, 645–648. doi: 10.1007/s10886-020-01209-y
Asis, C. A. Jr., Kubota, M., Ohta, H., Arima, Y., Ohwaki, Y., Yoneyama, T., et al. (2002). Estimation of the nitrogen fixation by sugarcane cultivars NiF-8 using 15N dilution and natural 15N abundance techniques. Soil Sci. Plant Nutr. 48, 283–285.
Babicki, S., Arndt, D., Marcu, A., Liang, Y., Grant, J. R., Maciejewski, A., et al. (2016). Heatmapper: web-enabled heat mapping for all. Nucleic Acids Res. 44, W147–W153. doi: 10.1093/nar/gkw419
Belimov, A. A., Safronova, V. I., and Mimura, T. (2002). Response of spring rape (Brassica napus L. var. Oleifera) to inoculation with plant growth promoting rhizobacteria containing 1-aminocyclopropane-1-carboxylate deaminase depends on nutrient status of the plant. Can. J. Microbiol. 48, 189–199. doi: 10.1139/w02-007
Berg, G., Erlacher, A., Smalla, K., and Krause, R. (2014). Vegetable microbiomes: is there a connection among opportunistic infections, human health and our “gut feeling”? Microb. Biotechnol. 7, 487–495. doi: 10.1111/1751-7915.12159
Bhardwaj, D., Ansari, M. W., Sahoo, R. K., and Tuteja, N. (2014). Biofertilizers function as key player in sustainable agriculture by improving soil fertility, plant tolerance and crop productivity. Microbial Cell Factories 13:66. doi: 10.1186/1475-2859-13-66
Brock, A. K., Berger, B., Schreiner, M., Ruppel, S., and Mewis, I. (2018). Plant growth-promoting bacteria Kosakonia radicincitans mediate anti-herbivore defense in Arabidopsis thaliana. Planta 248, 1383–1392. doi: 10.1007/s00425-018-2964-0
Chakdar, H., Dastager, S. G., Khire, J. M., Rane, D., and Dharne, M. S. (2018). Characterization of mineral phosphate solubilizing and plant growth promoting bacteria from termite soil of arid region. 3 Biotech 8:463. doi: 10.1007/s13205-018-1488-4
Chen, C., Xin, K., Liu, H., Cheng, J., Shen, X., Wang, Y., et al. (2017). Pantoea alhagi, a novel endophytic bacterium with ability to improve growth and drought tolerance in wheat. Sci. Rep. 7:41564.
Chen, Q., and Liu, S. (2019). Identification and characterization of the phosphate-solubilizing bacterium Pantoea sp. S32 in reclamation Soil in Shanxi, China. Front. Microbiol. 10:2171. doi: 10.3389/fmicb.2019.02171
Das, K., Prasanna, R., and Saxena, A. K. (2017). Rhizobia: a potential biocontrol agent for soilborne fungal pathogens. Folia Microbiol. 62, 425–435. doi: 10.1007/s12223-017-0513-z
Dobereiner, J., Reis, V. M., Paula, M. A., and Olivares, F. L. (1993). “Endophytic diazotrophs in sugar cane, cereals and tuber plants,” in New Horizons in Nitrogen Fixation, eds R. Palacios, J. Mora, and W. E. Newton (Dordrecht: Kluwer Academic), 671–676. doi: 10.1007/978-94-017-2416-6_55
Edwards, U., Rogall, T., Blöcker, H., Emde, M., and Böttger, E. C. (1989). Isolation and direct complete nucleotide determination of entire genes. characterization of a gene coding for 16S ribosomal RNA. Nucleic Acids Res. 17, 7843–7853. doi: 10.1093/nar/17.19.7843
Felsenstein, J. (1985). Confidence limits on phylogenies, an approach using the bootstrap. Evolution 39, 783–791. doi: 10.1111/j.1558-5646.1985.tb00420.x
Fishman, J. A. (2013). Opportunistic infections–coming to the limits of immune suppression? Cold Spring Harb. Perspect. Med. 3:a015669. doi: 10.1101/cshperspect.a015669
Fiske, C. H., and Subbarow, Y. (1925). A colorimetric determination of phosphorus. J. Biol. Chem. 66, 375–400.
Fraser, E. D. G., and Campbell, M. (2019). Agriculture 5.0: reconciling production with planetary health. One Earth 1, 278–280.
Funayama, K., Kojima, S., Tabuchi-Kobayashi, M., Sawa, Y., Nakayama, Y., Hayakawa, T., et al. (2013). Cytosolic glutamine synthetase1;2 is responsible for the primary assimilation of ammonium in rice roots. Plant Cell Physiol. 54, 934–943. doi: 10.1093/pcp/pct046
Glick, B. R. (2014). Bacteria with ACC deaminase can promote plant growth and help to feed the world. Microbiol. Res. 169, 30–39. doi: 10.1016/j.micres.2013.09.009
Glick, B. R., Todorovic, B., Czarny, J., Cheng, Z., Duan, J., and McConkey, B. (2007). Promotion of plant growth by bacterial ACC deaminase. Crit. Rev. Plant Sci. 26, 227–242.
Gordon, S. A., and Weber, R. P. (1951). Colorimetric estimation of indole acetic acid. Plant Physiol. 26, 192–195.
Goswami, D., Dhandhukia, P., Patel, P., and Thakker, J. N. (2014). Screening of PGPR from saline desert of Kutch: growth promotion in Arachis hypogea by Bacillus licheniformis A2. Microbiol. Res. 169, 66–75. doi: 10.1016/j.micres.2013.07.004
Guo, D. J., Singh, R. K., Singh, P., Li, D. P., Sharma, A., Xing, Y. X., et al. (2020). Complete genome sequence of Enterobacter roggenkampii ED5, a nitrogen fixing plant growth promoting endophytic bacterium with biocontrol and stress tolerance properties, isolated from sugarcane root. Front. Microbiol. 11:580081. doi: 10.3389/fmicb.2020.580081
Hardy, R. W. F., Holsten, R. D., Jackson, E. K., and Burns, R. C. (1968). The acetylene ethylene assay for N fixation, laboratory and field evaluation. Plant Physiol. 43, 1185–1207. doi: 10.1104/pp.43.8.1185
Honma, M., and Shimomura, T. (1978). Metabolism of 1-aminocyclopropane-1- carboxylic acid. Agric. Biol. Chem. 42, 1825–1831.
Hu, Q. P., and Xu, J. G. (2011). A simple double-layered chrome azurol S agar (SDCASA) plate assay to optimize the production of siderophores by a potential biocontrol agent Bacillus. Afr. J. Microbiol. Res. 5, 4321–4327.
Huang, C. J., Wang, T. K., Chung, S. C., and Chen, C. Y. (2005). Identification of an antifungal chitinase from a potential biocontrol agent, Bacillus cereus 28-9. J. Biochem. Mol. Biol. 38, 82–88.
James, E. K. (2000). Nitrogen fixation in endophytic and associative symbiosis. Food Crop Res. 65, 197–209.
Kour, D., Rana, K. L., Yadav, A. N., Yadav, N., Kumar, M., Kumar, V., et al. (2020). Microbial biofertilizers: bioresources and eco-friendly technologies for agricultural and environmental sustainability. Biocatal. Agric. Biotechnol. 23:101487. doi: 10.1016/j.bcab.2019.101487
Kumar, M., Grader, G., Sessitsch, A., Maki, A., van Elsas, J. D., and Nissien, R. (2017). Plants assemble species specific bacterial communities from common core taxa in three arcto-alpine climate zones. Front. Microbiol. 8:12. doi: 10.3389/fmicb.2017.00012
Kumar, S., Stecher, G., Li, M., Knyaz, C., and Tamura, K. (2018). MEGA X, molecular evolutionary genetics analysis across computing platforms. Mol. Biol. Evol. 35, 1547–1549. doi: 10.1093/molbev/msy096
Lambrese, Y., Guiñez, M., Calvente, V., Sansone, G., Cerutti, S., Raba, J., et al. (2018). Production of siderophores by the bacterium Kosakonia radicincitans and its application to control of phytopathogenic fungi. Bioresour. Technol. Rep. 3, 82–87.
Leghari, S. J., Wahocho, N. A., Laghari, G. M., Laghari, A. H., Bhabhan, G. M., Talpur, K. H., et al. (2016). Role of nitrogen for plant growth and development: a review. Adv. Environ. Biol. 10, 209–218.
Li, H. B., Singh, R. K., Singh, P., Song, Q. Q., Xing, Y. X., Yang, L. T., et al. (2017). Genetic diversity of nitrogen-fixing and plant growth promoting Pseudomonas species isolated from sugarcane rhizosphere. Front. Microbiol. 8:1268. doi: 10.3389/fmicb.2017.01268
Li, Y. R., Song, X. P., Wu, J. M., Li, C. N., Liang, Q., Liu, X. H., et al. (2016). Sugar industry and improved sugarcane farming technologies in China. Sugar Tech. 18, 603–611. doi: 10.1007/s12355-016-0480-488
Li, Z., Chang, S., Lin, L., Li, Y., and An, Q. (2011). A colorimetric assay of 1- aminocyclopropane-1-carboxylate (ACC) based on ninhydrin reaction for rapid screening of bacteria containing ACC deaminase. Lett. Appl. Microbiol. 53, 178–185. doi: 10.1111/j.1472-765X.2011.03088.x
Lin, L., Li, Z., Hu, C., Zhang, X., Chang, S., Yang, L., et al. (2012). Plant growth-promoting nitrogen-fixing enterobacteria are in association with sugarcane plants growing in Guangxi. China. Microbes Environ. 27, 391–398. doi: 10.1264/jsme2.me11275
Livak, K. J., and Schmittgen, T. D. (2001). Analysis of relative gene expression data using real-time quantitative PCR and the 2-11Ct method. Methods 25, 402–408.
Marques, A. P. G. C., Pires, C., Moreira, H., Rangel, A. O. S. S., and Castro, P. M. L. (2010). Assessment of the plant growth promotion abilities of six bacterial isolates using Zea mays as indicator plant. Soil Biol. Biochem. 42, 1229–1235.
Martins, D. S., Reis, V. M., Schultz, N., Alves, B. J. R., Urquiaga, S., Pereira, W., et al. (2020). Both the contribution of soil nitrogen and of biological N2 fixation to sugarcane can increase with the inoculation of diazotrophic bacteria. Plant Soil. 454, 155–169.
Mercado-Blanco, J., and Lugtenberg, B. (2014). Biotechnological applications of bacterial endophytes. Curr. Biotechnol. 3, 60–75.
Miflin, B. J., and Habash, D. Z. (2002). The role of glutamine synthetase and glutamate dehydrogenase in nitrogen assimilation and possibilities for improvement in the nitrogen utilization of crops. J. Exp. Bot. 53, 979–987. doi: 10.1093/jexbot/53.370.979
Mihalache, G., Zamfirache, M. M., and Stefan, M. (2015). Root associated bacteria—friends or enemies? a review. Mem. Sci. Sect. Rom. Acad. 38, 27–54.
Montañez, A., Abreu, C., Gill, P. R., Hardarson, G., and Sicardi, M. (2009). Biological nitrogen fixation in maize (Zea mays L.) by 15N isotope-dilution and identification of associated culturable diazotrophs. Biol Fert Soils. 45, 253–263.
Nascimento, F. X., Rossi, M. J., Soares, C. R. F. S., McConkey, B. J., and Glick, B. R. (2014). New insights into 1-Aminocyclopropane1-carboxylate (ACC) deaminase phylogeny. evolution and ecological significance. PLoS One 9:e99168. doi: 10.1371/journal.pone.0099168
Nei, M., and Kumar, S. (2000). Molecular Evolution and Phylogenetics. New York, NY: Oxford University Press.
Noar, J. D., and Bruno-Bárcena, J. M. (2018). Azotobacter vinelandii: the source of 100 years of discoveries and many more to come. Microbiology 164, 421–436. doi: 10.1099/mic.0.000643
Nogueira, E. D. M., Olivares, F. L., Japiassu, J. C., Vilar, C., Vinagre, F., Baldani, J. I., et al. (2005). Characterization of glutamine synthetase genes in sugarcane genotypes with different rates of biological nitrogen fixation. Plant Sci. 169, 819–832.
Olanrewaju, O. S., Glick, B. R., and Babalola, O. O. (2017). Mechanisms of action of plant growth promoting bacteria. World J. Microbiol. Biotechnol. 33: 197.
Oleńska, E., Małek, W., Wójcik, M., Swiecicka, I., Thijs, S., and Vangronsveld, J. (2020). Beneficial features of plant growth-promoting rhizobacteria for improving plant growth and health in challenging conditions: a methodical review. Sci. Total Enviorn. 743:140682. doi: 10.1016/j.scitotenv.2020.140682
Passari, A. K., Lalsiamthari, P. C., Zothanpuia, Leo, V. V., Mishra, V. K., Yadav, M. K., et al. (2018). Biocontrol of Fusarium wilt of Capsicum annuum by rhizospheric bacteria isolated from turmeric endowed with plant growth promotion and disease suppression potential. Eur. J. Plant Pathol. 150, 831–846.
Passari, A. K., Mishra, V. K., Singh, G., Singh, P., Kumar, B., Gupta, V. K., et al. (2017). Insights into the functionality of endophytic actinobacteria with a focus on their biosynthetic potential and secondary metabolites production. Sci. Rep. 7:11809.
Payne, S. M. (1993). Iron acquisition in microbial pathogenesis. Trends Microbiol. 1, 66–69. doi: 10.1016/0966-842x(93)90036-q
Penrose, D. M., and Glick, B. R. (2003). Methods for isolating and characterizing ACC deaminase-containing plant growth-promoting rhizobacteria. Physiol. Plant. 118, 10–15. doi: 10.1034/j.1399-3054.2003.00086.x
Poly, F., Monrozier, L. J., and Bally, R. (2001). Improvement in the RFLP procedure for studying the diversity of nifH genes in communities of nitrogen fixers in soil. Res. Microbiol. 152, 95–103. doi: 10.1016/s0923-2508(00)01172-4
Pratelli, R., and Pilot, G. (2014). Regulation of amino acid metabolic enzymes and transporters in plants. J. Exp. Bot. 65, 5535–5556. doi: 10.1093/jxb/eru320
Quecine, M. C., Araújo, W. L., Rossetto, P. B., Ferreira, A., Tsui, S., Lacava, P. T., et al. (2012). Sugarcane growth promotion by the endophytic bacterium Pantoea agglomerans 33.1. Appl. Environ. Microbiol. 78, 7511–7518. doi: 10.1128/AEM.00836-12
Raklami, A., Bechtaoui, N., Tahiri, A. I., Anli, M., Meddich, A., and Oufdou, K. (2019). Use of rhizobacteria and mycorrhizae consortium in the open field as a strategy for improving crop nutrition, productivity and soil fertility. Front. Microbiol. 10:1106. doi: 10.3389/fmicb.2019.01106
Ramette, A., Frapolli, M., Defago, G., and Moenne-Loccoz, Y. (2003). Phylogeny of HCN synthase-encoding hcnBC genes in biocontrol fluorescent pseudomonads and its relationship with host plant species and HCN synthesis ability. Mol. Plant Microb. Interact. 16, 525–535. doi: 10.1094/MPMI.2003.16.6.525
Romano, I., Ventorino, V., Ambrosino, P., Testa, A., Chouyia, F. E., and Pepe, O. (2020). Development and application of low-cost and eco-sustainable bio-stimulant containing a new plant growth-promoting strain Kosakonia pseudosacchari TL13. Front. Microbiol. 11:2044. doi: 10.3389/fmicb.2020.02044
Saitou, N., and Nei, M. (1987). The neighbor-joining method, a new method for reconstructing phylogenetic trees. Mol. Biol. Evol. 4, 406–425.
Schwyn, B., and Neilands, J. B. (1987). Universal chemical assay for the detection and determination of siderophores. Anal. Biochem. 160, 47–56. doi: 10.1016/0003-2697(87)90612-9
Sharma, A., Kashyap, P. L., Srivastava, A. K., Bansal, Y. K., and Kaushik, R. (2019). Isolation and characterization of salt-tolerant bacilli from chickpea (Cicer arietinum L.) rhizosphere for plant growth promotion and biocontrol traits. Eur. J. Plant Pathol. 153, 787–800.
Sharma, A., Singh, R. K., Singh, P., Vaishnav, A., Guo, D.-J., Verma, K. K., et al. (2021). Insights into the bacterial and nitric oxide-induced salt tolerance in sugarcane and their growth promoting abilities. Microorganisms 9: 2203.
Singh, A., Kumar, P., Gautam, V., Rengasamy, B., Adhikari, B., Udayakumar, M., et al. (2016). Root transcriptome of two contrasting indica rice cultivars uncovers regulators of root development and physiological responses. Sci. Rep. 6:39266. doi: 10.1038/srep39266
Singh, P., Singh, R. K., Li, H. B., Guo, D. J., Sharma, A., Lakshmanan, P., et al. (2021b). Diazotrophic bacteria pantoea dispersa and Enterobacter asburiae promote sugarcane growth by inducing nitrogen uptake and defense-related gene expression. Front. Microbiol. 11:600417. doi: 10.3389/fmicb.2020.600417
Singh, P., Singh, R. K., Song, Q. Q., Li, H. B., Guo, D. J., Malviya, M. K., et al. (2021c). Comparative analysis of protein and differential responses of defense related gene and enzyme activity reveals the long-term molecular responses of sugarcane inoculated with Sporisorium scitamineum. J. Plant Interact. 16, 12–29.
Singh, P., Singh, R. K., Guo, D.-J., Sharma, A., Singh, R. N., Li, D.-P., et al. (2021a). Whole genome analysis of sugarcane root-associated endophyte Pseudomonas aeruginosa B18—A plant growth-promoting bacterium with antagonistic potential against Sporisorium scitamineum. Front. Microbiol. 1:628376. doi: 10.3389/fmicb.2021.628376
Singh, R. K., Singh, P., Li, H. B., Guo, D. J., Song, Q. Q., Yang, L. T., et al. (2020b). Plant-PGPR interaction study of plant growth-promoting diazotrophs Kosakonia radicincitans BA1 and Stenotrophomonas maltophilia COA2 to enhance growth and stress-related gene expression in Saccharum spp. J. Plant Interact. 15, 427–445.
Singh, R. K., Singh, P., Li, H. B., Song, Q. Q., Guo, D. J., Solanki, M. K., et al. (2020c). Diversity of nitrogen-fixing rhizobacteria associated with sugarcane, a comprehensive study of plant-microbe interactions for growth enhancement in Saccharum spp. BMC Plant Biol. 20:220. doi: 10.1186/s12870-020-02400-9
Singh, P., Singh, R. K., Song, Q. Q., Li, H. B., Yang, L. T., and Li, Y. R. (2020a). Methods for estimation of nitrogen components in plants and microorganisms. Methods Mol. Biol. 2057, 103–112. doi: 10.1007/978-1-4939-9790-9_10
Singh, P., Song, Q. Q., Singh, R. K., Li, H. B., Solanki, M. K., Malviya, M. K., et al. (2019). Proteomic analysis of the resistance mechanisms in sugarcane during Sporisorium scitamineum infection. Int. J. Mol. Sci. 20:569. doi: 10.3390/ijms20030569
Singh, P., Song, Q. Q., Singh, R. K., Li, H. B., Solanki, M. K., Yang, L. T., et al. (2018). Physiological and molecular analysis of sugarcane (varieties- F134 and NCo310) during Sporisorium scitamineum interaction. Sugar Tech. 21, 631–644.
Singh, R. K., Kumar, D. P., Singh, P., Solanki, M. K., Srivastava, S., Srivastva, et al. (2014). Multifarious plant growth promoting characteristics of chickpea rhizosphere associated Bacilli help to suppress soil-borne pathogens. Plant Growth Regul. 73, 91–101.
Singh, R. K., Kumar, D. P., Solanki, M. K., Singh, P., Srivastva, A. K., Kumar, S., et al. (2013). Optimization of media components for chitinase production by chickpea rhizosphere associated Lysinibacillus fusiformis B-CM18. J. Basic Microbiol. 53, 451–460. doi: 10.1002/jobm.201100590
Stefan, M., Munteanu, N., Stoleru, V., and Mihasan, M. (2013). Effects of inoculation with plant growth promoting rhizobacteria on photosynthesis, antioxidant status and yield of runner bean. Rom. Biotechnol. Lett. 18, 8132–8143.
Stepanova, A. N., Yun, J., Likhacheva, A. V., and Alonso, J. M. (2007). Multilevel interactions between ethylene and auxin in Arabidopsis roots. Plant Cell 19, 2169–2185. doi: 10.1105/tpc.107.052068
Stitt, M., Müller, C., Matt, P., Gibon, Y., Carillo, P., Morcuende, R., et al. (2002). Steps towards an integrated view of nitrogen metabolism. J. Exp. Bot. 53, 959–970. doi: 10.1093/jexbot/53.370.959
Suman, A., Shukla, L., Marag, P. S., Verma, P., Gond, S., and Prasad, J. S. (2020). Potential use of plant colonizing Pantoeaas generic plant growth promoting bacteria for cereal crops. J. Environ. Biol. 41, 987–994. doi: 10.1128/AEM.00582-21
Sur, S., Bothra, A. K., and Sen, A. (2010). Symbiotic nitrogen fixation-a bioinformatics perspective. Biotech 9, 257–273.
Tambong, J. T. (2019). Taxogenomics and systematics of the genus pantoea. Front. Microbiol. 10:2463. doi: 10.3389/fmicb.2019.02463
Thaweenut, N., Hachisuka, Y., Ando, S., Yanagisawa, S., and Tadakatsu, Y. (2011). Two seasons’ study on nifH gene expression and nitrogen fixation by diazotrophic endophytes in sugarcane (Saccharum spp. hybrids): expression of nifH genes similar to those of rhizobia. Plant Soil. 338, 435–449.
Tilman, D., Cassman, K. G., Matson, P. A., Naylor, R., and Polasky, S. (2002). Agricultural sustainability and intensive production practices. Nature 418, 671–677. doi: 10.1038/nature01014
United States Department of Agriculture [USDA] (2020). Sugar: World Markets and Trade. Washington, D.C: USDA.
Urquiaga, S., Cruz, K. H. S., and Boddey, R. M. (1992). Contribution of nitrogen fixation to sugarcane: nitrogen-15 and nitrogen-balance estimates. Soil Sci. Soc. Am. J. 56, 105–114.
Vanlauwe, B., Hungria, M., Kanampiu, F., and Giller, K. E. (2019). The role of legumes in the sustainable intensification of African smallholder agriculture: lessons learnt and challenges for the future. Agric. Ecosyst. Environ. 284:106583. doi: 10.1016/j.agee.2019.106583
Vital, C. E., Giordano, A., de Almeida Soares, E., Rhys Williams, T. C., Mesquita, R. O., Vidigal, P., et al. (2017). An integrative overview of the molecular and physiological responses of sugarcane under drought conditions. Plant Mol. Biol. 94, 577–594. doi: 10.1007/s11103-017-0611-y
Keywords: antifungal activity, endophytes, nitrogen fixation, Pantoea cypripedii, Kosakonia arachidis, PGP, colonization, sugarcane
Citation: Singh RK, Singh P, Guo D-J, Sharma A, Li D-P, Li X, Verma KK, Malviya MK, Song X-P, Lakshmanan P, Yang L-T and Li Y-R (2021) Root-Derived Endophytic Diazotrophic Bacteria Pantoea cypripedii AF1 and Kosakonia arachidis EF1 Promote Nitrogen Assimilation and Growth in Sugarcane. Front. Microbiol. 12:774707. doi: 10.3389/fmicb.2021.774707
Received: 12 September 2021; Accepted: 12 November 2021;
Published: 15 December 2021.
Edited by:
Prem Lal Kashyap, Indian Institute of Wheat and Barley Research (ICAR), IndiaReviewed by:
Sajid Ali, Yeungnam University, South KoreaShyam Lal Kandel, University of Washington, United States
Copyright © 2021 Singh, Singh, Guo, Sharma, Li, Li, Verma, Malviya, Song, Lakshmanan, Yang and Li. This is an open-access article distributed under the terms of the Creative Commons Attribution License (CC BY). The use, distribution or reproduction in other forums is permitted, provided the original author(s) and the copyright owner(s) are credited and that the original publication in this journal is cited, in accordance with accepted academic practice. No use, distribution or reproduction is permitted which does not comply with these terms.
*Correspondence: Yang-Rui Li, liyr@gxaas.net