- 1Institut des Sciences Analytiques, Université Claude Bernard Lyon 1, Lyon, France
- 2Bacteriology Department, CHU de Bordeaux, National Reference Center for Campylobacters and Helicobacters, Bordeaux, France
- 3Institut des Agents Infectieux, Hospices Civils de Lyon, Lyon, France
- 4Bordeaux Institute of Oncology, BRIC U1312, INSERM, Université de Bordeaux, Bordeaux, France
- 5Laboratoire Associé CNR de la Résistance aux Antibiotiques, CHU de Clermont-Ferrand, Clermont-Ferrand, France
Introduction: Aminopenicillins resistance among Campylobacter jejuni and Campylobacter coli strains is associated with a single mutation in the promoting region of a chromosomal beta-lactamase blaOXA61, allowing its expression. Clavulanic acid is used to restore aminopenicillins activity in case of blaOXA61 expression and has also an inherent antimicrobial activity over Campylobacter spp. Resistance to amoxicillin-clavulanic acid is therefore extremely rare among these species: only 0.1% of all Campylobacter spp. analyzed in the French National Reference Center these last years (2017–2022).
Material and methods: Whole genome sequencing with bioinformatic resistance identification combined with mass spectrometry (MS) was used to identify amoxicillin-acid clavulanic resistance mechanism in Campylobacters.
Results: A G57T mutation in blaOXA61 promoting region was identified in all C. jejuni and C. coli ampicillin resistant isolates and no mutation in ampicillin susceptible isolates. Interestingly, three C. coli resistant to both ampicillin and amoxicillin-clavulanic acid displayed a supplemental deletion in the promoting region of blaOXA61 beta-lactamase, at position A69. Using MS, a significant difference in the expression of BlaOXA61 was observed between these three isolates and amoxicillin-clavulanic acid susceptible C. coli.
Conclusion: A combined genomics/proteomics approach allowed here to identify a rare putative resistance mechanism associated with amoxicillin-clavulanic acid resistance for C. coli.
Introduction
Campylobacter jejuni and Campylobacter coli are the most common cause of bacterial gastro-enteritis worldwide, before Salmonella (Chlebicz and Śliżewska, 2018). They are foodborne pathogens transmitted via the consumption of contaminated products, especially meat (mainly chicken, beef and pork). In Europe in 2021, campylobacteriosis accounted for more than 120,000 cases of illness (The European Union One Health, 2021) while in the USA, the number of Campylobacter infections is estimated at 1.5 million illnesses each year (Delahoy et al., 2023). Campylobacteriosis can cause symptoms such as abdominal pains, fever and diarrhea, which are significant risks of complications at the extreme ages of life (Fernández-Cruz et al., 2010). Antimicrobial therapy is considered in case of serious infections, but resistance to commonly used antimicrobials is of concern.
In 2022, 6,772 C. jejuni and 1,138 C. coli clinical isolates were tested at the French National Reference Center for Campylobacters and Helicobacters (Lehours et al., 2023) (NRCCH) showing 63.1 and 64% resistance to ciprofloxacin, 46.9 and 79.4% resistance to tetracycline as well as 33.5 and 29.1% resistance for ampicillin, respectively. However, resistance to macrolides and aminoglycosides remained very low, even though C. coli showed a concerning increase in erythromycin resistance through the emergence of new mechanisms (Jehanne et al., 2021). In France, 0.3% of C. jejuni and 7% of C. coli isolates are resistant to erythromycin and 0.4% of C. jejuni and 2.4% of C. coli isolates are resistant to gentamicin. In addition, less than 0.1% of both C. jejuni and C. coli are resistant to amoxicillin-clavulanic acid, a phenomena which is more common for Campylobacter closely related species, for instance Aliarcobacter butzleri (Zacharow et al., 2015).
Due to the excessive use of antimicrobials, especially in animal, specific genetic mechanisms of resistance have been selected among C. jejuni and C. coli (Griggs et al., 2005; Ladely et al., 2009; Qin et al., 2014; Florez-Cuadrado et al., 2017; Chen et al., 2018; Fabre et al., 2018; Elhadidy et al., 2019; Anampa et al., 2020; Greninger et al., 2020; Hormeño et al., 2020; Wallace et al., 2020; Jehanne et al., 2021). The G57T mutation in the promoting region of blaOXA61 (Zeng et al., 2014) is, in particular, associated with ampicillin resistance. However, no amoxicillin-clavulanic acid resistance mechanism has yet been described. Amoxicillin-clavulanic acid can therefore be an antimicrobial of choice against campylobacteriosis, especially in case of bacteremia (Abay et al., 2014; Schiaffino et al., 2019; Tinévez et al., 2022). However, high levels of resistance can be found in some clinical isolates with minimum inhibitory concentration (MIC) reaching 256 mg/L or above. In the present study, 30 clinical isolates from the collection of the NRCCH were analyzed. Amoxicillin-clavulanic acid and ampicillin resistant and susceptible C. jejuni and C. coli were selected (i) to perform whole-genome sequencing (WGS), (ii) to quantify the expression of BlaOXA61 using mass spectrometry (MS) in order to (iii) identify the genetic mechanism associated with amoxicillin-clavulanic acid resistance. It allowed us to link BlaOXA61 expression levels to the sequence of its promoting region and especially amoxicillin-clavulanic acid resistance to the presence of a supplemental deletion at position A69 in C. coli.
Materials and methods
Isolates selection
A total of 30 isolates (12 C. coli and 18 C. jejuni), listed in Table 1 with their corresponding ENA accession number, were analyzed in the present study as well as two references (C. jejuni CCUG 11284 and C. coli CCUG 11283). The mean age and sex ratio (m/f) of the dataset were 38.4 years and 1.7, respectively. It is composed of clinical strains that have been isolated between 2017 and 2020 from stools and send to the French National Reference Center for Campylobacters and Helicobacters (NRCCH) (Bordeaux, France) by clinical laboratories participating to its surveillance network. Among the C. jejuni isolates, nine were ampicillin (AMP)-susceptible (S), and nine were AMP-resistant (R). They were all amoxicillin-clavulanic acid (AMC)-susceptible. Four of the 12 C. coli were AMP-S and eight were AMP-R. Three AMP-R C. coli, from 2017, 2018 and 2020, were resistant to amoxicillin-clavulanic acid (AMC). Species were identified by matrix-assisted laser desorption ionization-time of flight-mass spectrometry as already described (Bessède et al., 2011). Antimicrobial susceptibility to ampicillin, amoxicillin-clavulanic acid, ciprofloxacin, erythromycin, tetracycline, and gentamicin were performed by diffusion using EUCAST guidelines.1 Ampicillin and amoxicillin-clavulanic acid MICs were determined using Etest (bioMérieux, Marcy l’Etoile, France) and interpreted according to the cut-off values proposed by the CASFM: S ≤ 4 mg/L, R > 16 mg/L.2
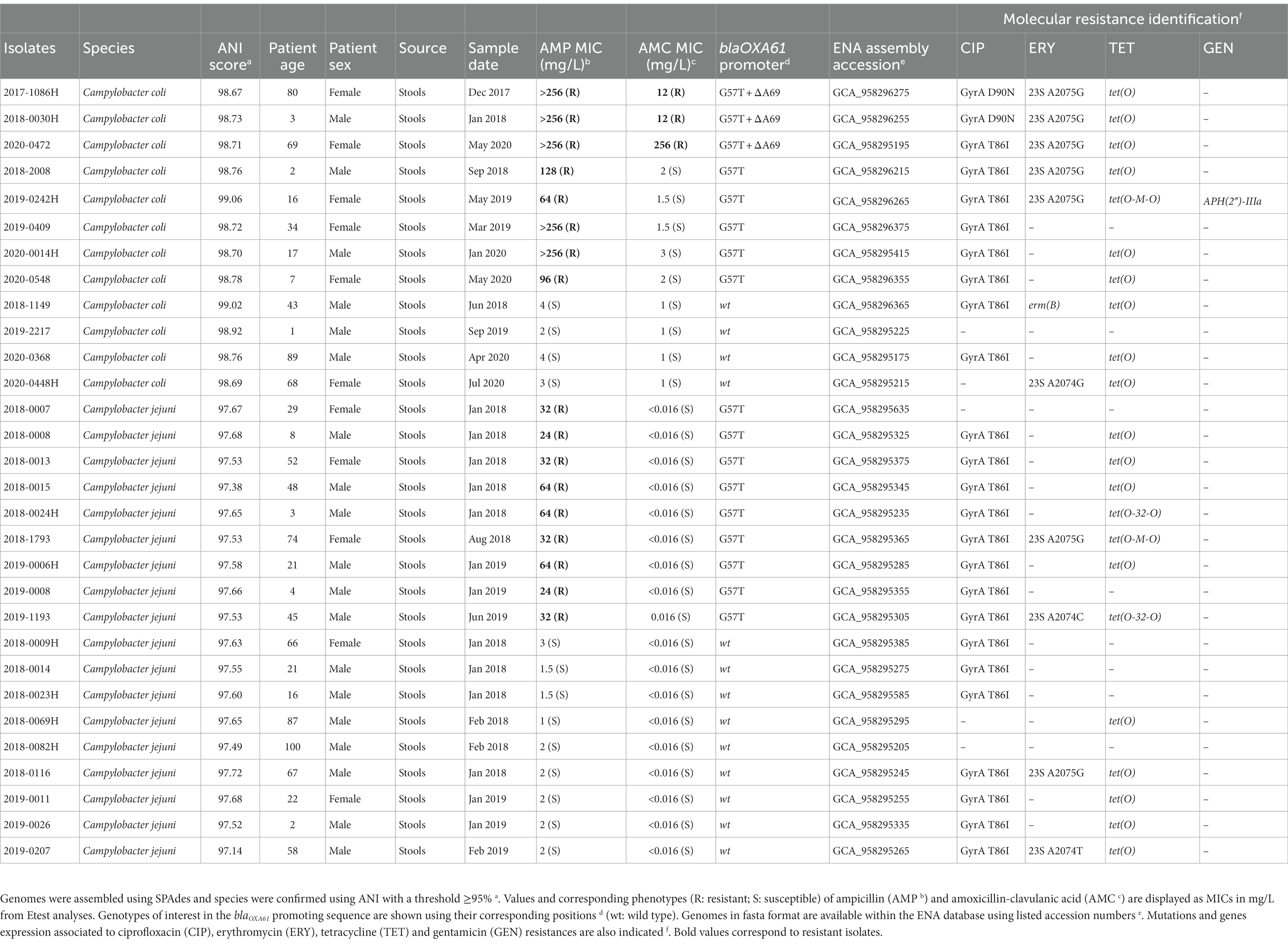
Table 1. C. coli and C. jejuni French clinical isolates from 2017 to 2020 analyzed in the present study.
Whole genome sequencing and genomes analyses
Each clinical isolate was grown on Columbia blood agar (CBA) plate with 5% sheep’s blood (Thermo Fisher Scientific, MA) and incubated at 37°C in a jar. An Anoxomat microprocessor (Mart Microbiology BV, Lichtenvoorde, The Netherlands) created an microaerobic atmosphere of 79.7% N2, 7.1% CO2, and 7.1% H2 and 6% O2. DNA was then extracted from pure bacterial colonies using the MagNA Pure 6 DNA and viral NA SV kit, and DNA purification was performed by bacterial lysis on a MagNA Pure 96 system (Roche Applied Science, Manheim, Germany). Whole genome sequencing (WGS) was performed either on Illumina ISeq 100 (locally at the NRCCH) or Nova Seq 6000 sequencer (Integragen, Evry, France). Sequencing data was analyzed using an in-house pipeline. Specifically, raw reads were cleaned using Sickle v1.33 (Joshi and Fass, 2011) and genomes were assembled using SPAdes v3.15.5 (Bankevich et al., 2012). Generated contigs were filtered depending on their depth (minimum five) and length (minimum 200). Bacterial species were confirmed from in vitro Average Nucleotide Identity method using FastANI v1.1 (Jain et al., 2018) and potential sources of contamination were identified using STRUCTURE tool v2.3.4 (Pritchard et al., 2000) combined with host-segregating genomic markers for C. jejuni (Thépault et al., 2017) and C. coli (Jehanne et al., 2020). Finally, resistance markers (genes and mutations) were isolated from each assembled genomes using Nucleotide-Nucleotide/Protein–Protein BLAST 2.12.0+ (Altschul et al., 1997) command line tool combined with multiple databases: ncbi, card, resfinder, plasmidfinder and an in-house database for Campylobacter sp. based on various previous publications (Griggs et al., 2005; Ladely et al., 2009; Qin et al., 2014; Zeng et al., 2014; Fabre et al., 2018; Hormeño et al., 2020; Jehanne et al., 2021).
Sample preparation for LC–MS/MS analysis
Primary cultures of C. coli and C. jejuni strains were performed in triplicates on Columbia agar +5% sheep blood plates (bioMérieux, Marcy L’étoile, France) at 37°C for 48 h in microaerobic atmosphere. Sub-cultures were performed under the same conditions and bacterial suspensions were prepared in LC–MS grade water to reach a minimum density of four McFarland. Two hundred microliters of bacterial suspension were transferred into 1.5 mL tubes containing approximately 70 mg of 150–212 μm glass beads (Sigma-Aldrich) and 50 μL of 1 mg/ml recombinant trypsin (Roche) in 150 mM NH4HCO3 (Sigma-Aldrich) were added to each tube. Bacterial lysis and protein digestion were performed in a thermostated (50°C) Bioruptor ultrasonicator (Diagenode, Lièges, Belgium) for 10 min with ultrasounds being applied for 30 s every minute. Trypsin digestion was stopped by adding 5 μL of formic acid (Sigma-Aldrich). Tubes were then centrifuged at 9600 × g for 5 min and 100 μL of supernatant were transferred to a final 2 mL glass vial for LC–MS/MS analysis.
LC–MS/MS analysis
Samples were analyzed with an Agilent 1290 Infinity liquid chromatography coupled to a SCIEX QTRAP6500+ Triple-quadrupole mass spectrometer equipped with an ESI Turbo V ion source. Ten microliters of samples were injected on the system. Mobile phases were H2O + 0.1% formic acid (Buffer A) and ACN + 0.1% formic acid (Buffer B). LC separation was carried out on a Waters Xbridge Peptide BEH C18 (1 mm × 100 mm, particle size 3.5 μm) column heated at 60°C. The analytical gradient was set as follow: 2 to 10% B from 0 to 0.1 min, 10 to 30.5% B from 0.1 to 13.1 min, and 30.5 to 50% B from 13.1 to 15.95 min. Flow rate was set at 100 μL/min. The mass spectrometer ion source temperature was 550°C and the ion spray voltage set at 5500 V. Curtain gas, nebulizer gas (GS1) and heating gas (GS2) were, respectively, set at 50, 70, and 60 psi.
MRM assay development
The amino-acid sequence of BlaOXA61 beta-lactamase was digested in silico with trypsin using Skyline software (version 22.2.0.351) to generate every potential surrogate peptide. An initial MRM method was built monitoring three y ion-type fragments for each doubly and triply charged putative peptides to identify the ones detectable in strains known to express high amounts of BlaOXA61. All peptides selected during the first screening step were included in a second MRM method to monitor every y and b ions of the most intense charge state. Signal specificity was confirmed when at least eight transitions were detected at the same retention time in strains known to express blaOXA61 and when no signal was observed for strains known to have intrinsic repression of the beta-lactamase expression. A final MRM assay was built with the three most intense fragment ions of each BlaOXA61 peptide as well as three y ions for five peptides derived from Campylobacter ribosomal proteins (Supplementary Table 1).
Peak detection and relative quantification
Raw chromatograms were analyzed using Skyline software. For each peptide, peak integration was manually checked and curated when necessary to ensure correct quantification. Label-free quantification strategies have now been widely applied and demonstrated their robustness for quantification of proteins in biological samples (Cecchini et al., 2018; Fournier et al., 2021; Pivard et al., 2023). Those approaches rely on the assumption that signal of the most intense transitions of the best flying peptide correlate with protein abundance and can therefore be used as an indicator of protein amounts (Ludwig et al., 2012). Here, the area of the three transitions of three BlaOXA61 peptides (EQAILLFR, YLDELVK and IDTFWLDNSLK) were summed to compensate for potential slight variations in fragmentation and trypsin digestion repeatability across runs. Moreover, to take into account bacterial load variations across samples, the sum of BlaOXA61 peptides is expressed relative to signals of five housekeeping peptides derived from ribosomal proteins used as indicators of bacterial density due to their quantotypic properties (Cecchini et al., 2018; Pivard et al., 2023). In other words, quantification values are obtained using Equation 1:
Results
Genomic analyses
C. jejuni and C. coli genomes were properly assembled and species were confirmed with average scores of 97.57% (±0.14) and 98.79% (±0.13) for C. jejuni and C. coli isolates, respectively (Table 1). The average genomes size was 1.72 Mbp (±67 kbp) with an average contigs number of 80 (±196; isolate fasta “2018–0015” is comprised of more than 1000 contigs which significantly increases the data) and an average contig length of 42 kbp (±15.8 kbp). Moreover, genomes GC % was about 32% (±2.2%) and the average number of coding sequences (CDS) was 1,766 (±66), in accordance with previously published data (Pearson et al., 2013; He et al., 2020). Additionally, most of the selected clinical isolates were attributed to the chicken reservoir using source attribution models (data not shown), which represents 63.3% of the dataset. Genomic antimicrobial resistance (AMR) analysis allowed to display the G57T mutation in the blaOXA61 promoting region among every AMP-R C. jejuni and C. coli isolates, highlighting the importance of this marker in ampicillin resistance for Campylobacters (Zeng et al., 2014). A supplemental deletion in position A69 of that promoting sequence was also exclusively identified among all three AMC-R C. coli isolates (two from chicken and one from pig according to the source attribution markers), as shown in Figure 1. The use of mass-spectrometry was used to estimate the impact of such deletion on the expression of BlaOXA61.
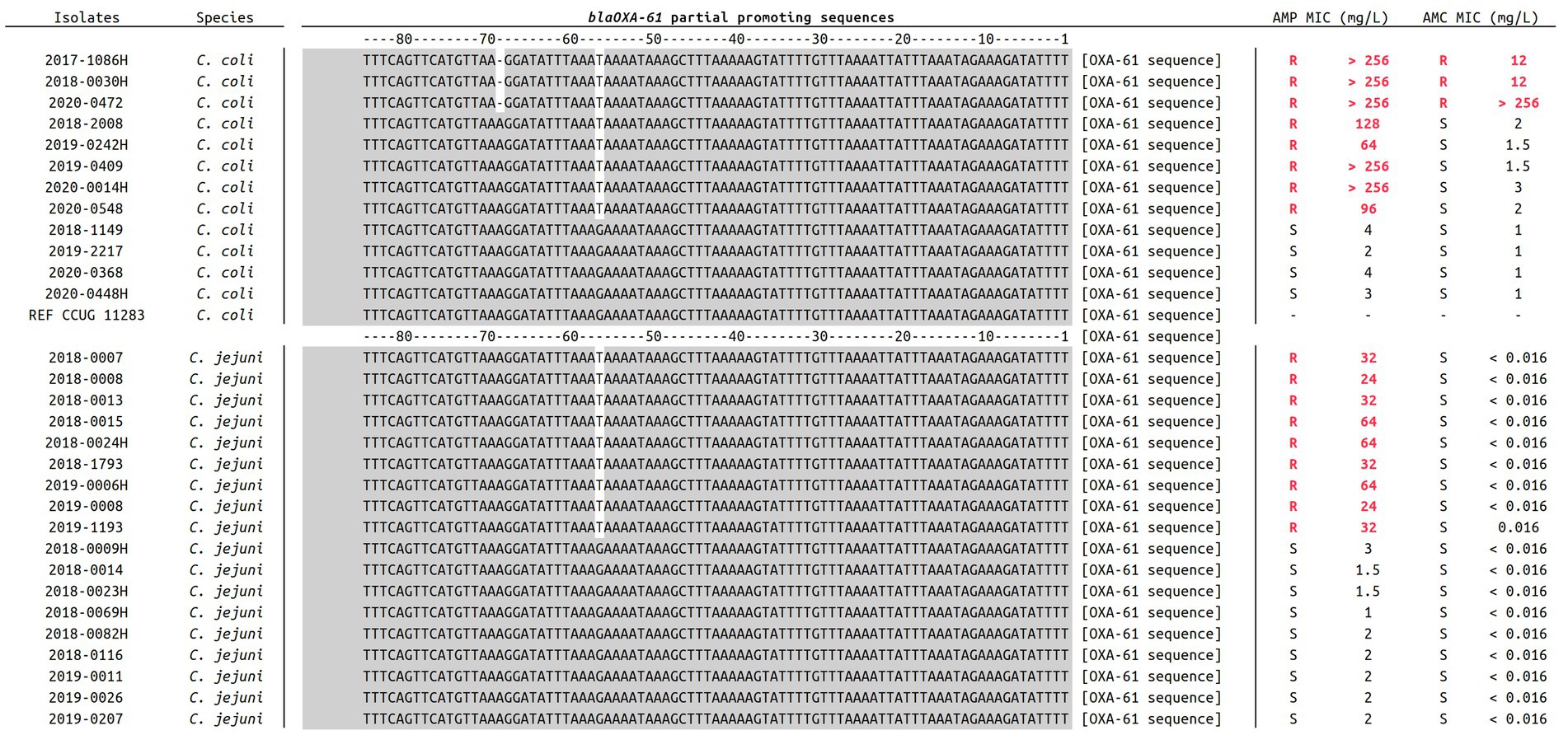
Figure 1. Alignment of blaOXA61 promoting sequences in 18 C. jejuni and 12 C. coli included in the present study. For each isolate, ampicillin and amoxicillin-clavulanic acid MICs are indicated. Promoting regions were extracted from every assembled genome using Nucleotide-Nucleotide BLAST 2.12.0+ (Altschul et al., 1997), and sequences were aligned using Muscle v3.8.1551 (Edgar, 2004). Relevant genotypes are here highlighted in white at positions −57 and −69.
MRM analyses
To quantitatively assess the expression levels of BlaOXA61, each strain was grown in triplicates and trypsin-digested bacterial lysates were analyzed by targeted mass spectrometry for the detection of BlaOXA61. Due to variations observed in the relative intensities of ribosomal peptides (used as housekeeping standards for cell number and expression level normalization, as described in material and methods) between C. jejuni and C. coli, BlaOXA61 expression levels were only compared within the same species.
In comparison to the wild type promoter, the addition of the G57T mutation in blaOXA61 promoting region leads to an increase of the beta-lactamase expression and confers resistance to ampicillin for both Campylobacter species (Figures 2A, 3A). Relative quantification revealed a significant 15-fold and 16-fold increase in overexpression of BlaOXA61 compared to the ampicillin susceptible (wild type promoter) in C. jejuni and C. coli isolates, respectively (Figures 2B, 3B).
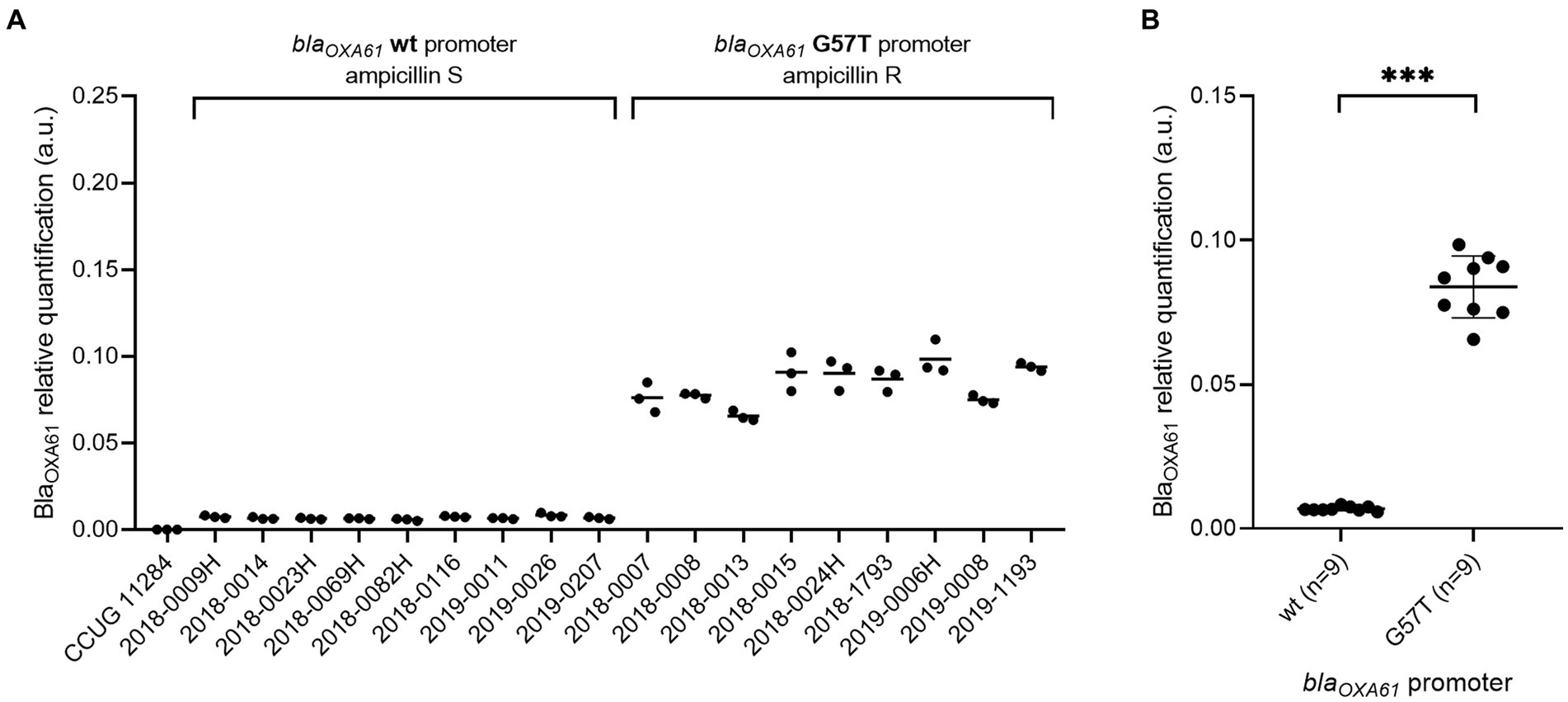
Figure 2. BlaOXA61 relative quantification in ampicillin-susceptible and resistant C. jejuni isolates. (A) Each strain individually. (B) Strains grouped according to the blaOXA61-promoting sequences. Data are expressed in arbitrary units. In panel (B), the mean of triplicates was used to perform a Mann–Whitney U test. ***p value <0.001. wt, wild type; S, susceptible; R, resistant. No signal was observed for C. jejuni reference CCUG 11284.
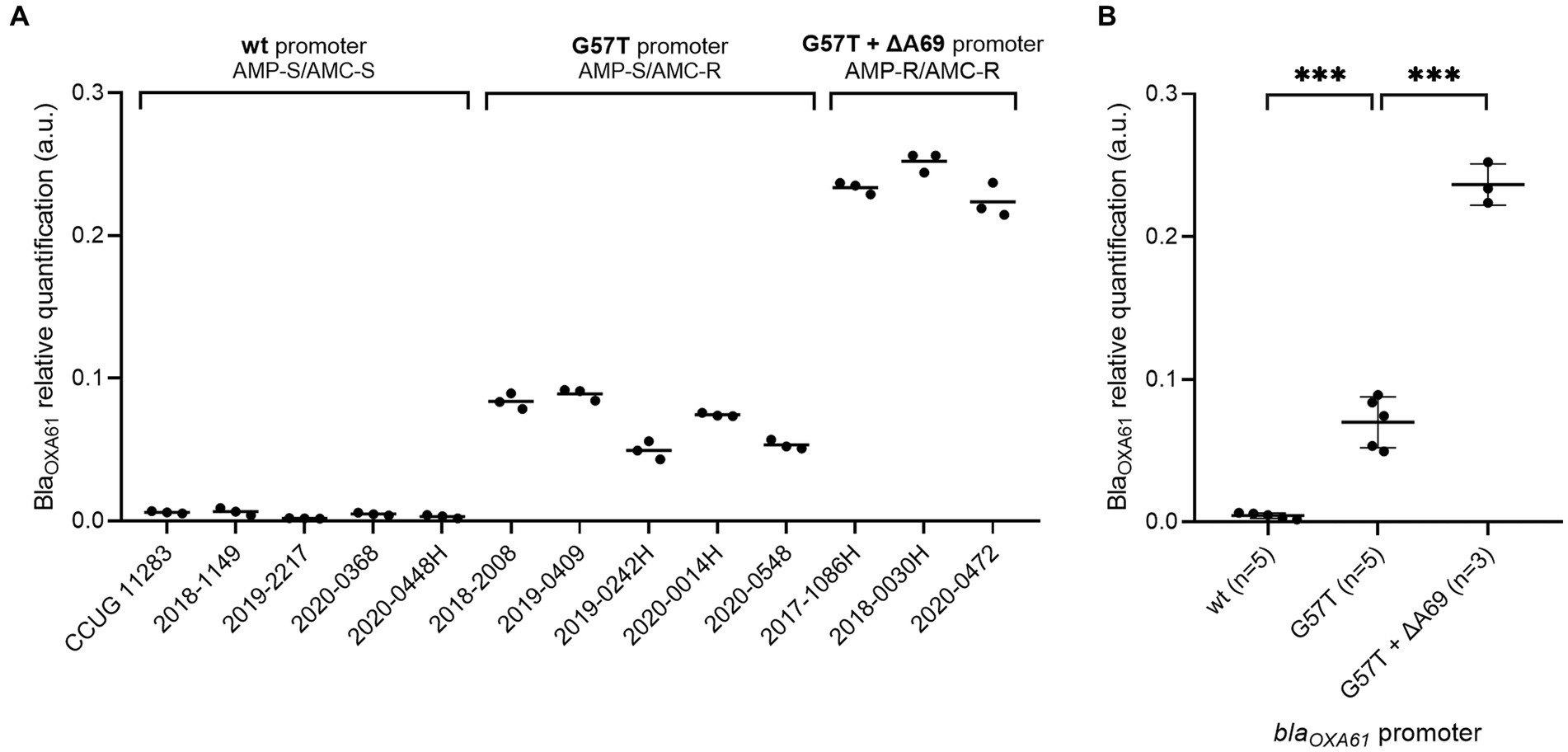
Figure 3. Expression levels of BlaOXA61 in C. coli isolates with wild type, G57T or G57T + ∆A69 promoters. (A) Each strain individually. (B) Strains grouped according to the blaOXA61-promoting sequences. Data are expressed in arbitrary units. In panel (B), the mean of triplicates was used to perform one-way ANOVA. ***p-value <0.001. wt, wild type; AMP, ampicillin; AMC, amoxicillin + clavulanic acid; S, susceptible; R, resistant.
The combination of the G57T mutation with a supplemental deletion in position A69 was associated with a significant overexpression of BlaOXA61 and resistance to amoxicillin-clavulanic acid in the three C. coli strains included in the present study. Indeed, a significant 54 and 16-fold increase was observed compared with strains harboring a WT or a G57T blaOXA61 promoter (Figure 3B), respectively.
Data availability
Corresponding genome accession numbers of each clinical isolates are available in Table 1 and under ENA Study number PRJEB63218. MRM raw data and transition list are available via PASSEL with the accession number PASS05834.3
Discussion
Campylobacter resistance to antimicrobials is concerning (Wieczorek and Osek, 2013). However, resistance to amoxicillin combined to clavulanic acid beta lactamase-inhibitor among human Campylobacter isolates remains sparse (Deckert et al., 2013; Post et al., 2017; Wallace et al., 2021), even though ampicillin resistance rates in poultry reservoirs are high (Casagrande Proietti et al., 2020; Béjaoui et al., 2022; Gharbi et al., 2023) (up to 73%). This current study proposes a combined genomic and proteomic approach to characterize the mechanism responsible for amoxicillin-clavulanic acid (AMC) resistance in Campylobacter clinical isolates, specifically C. coli. This experimental strategy used both molecular and mass spectrometry analyzes and highlighted a deletion at position A69 in the blaOXA61 promoting region contributing to a significant increase of the beta-lactamase expression, which is associated with amoxicillin-clavulanic acid resistance.
The existence of beta-lactamases among C. jejuni and C. coli isolates is now well described, such as blaOXA61, blaOXA489 or blaOXA193, with blaOXA61 being the most frequently observed (Cobo-Díaz et al., 2021). Even though their expression has been widely associated with aminopenicillin resistance, many previous studies are based uniquely on the presence or absence of beta-lactamase genes without taking into account the importance of the promoting region (Gharbi et al., 2023). As a matter of fact, a single nucleotide at position 57 (G → T) in this specific sequence is associated with ampicillin resistance (Zeng et al., 2014). Activity of beta-lactamase inhibitors such as clavulanic acid used in combination to amoxicillin may be significantly impaired in case of blaOXA61 overexpression, as shown in a previous study using PCR and qPCR amplification (Casagrande Proietti et al., 2020).
In the present study, LC–MS/MS allowed to confirm that the G57T mutation in the blaOXA61 promoting region is responsible for an increased expression of the beta-lactamase, thus conferring ampicillin resistance. However, every C. jejuni and C. coli clinical isolates which only displayed that single mutation remain highly susceptible to the amoxicillin-clavulanic acid activity. Nevertheless, we showed that three AMC-resistant C. coli isolates displayed a supplemental A69 deletion responsible for an overexpression of BlaOXA61. Those high levels of BlaOXA61 production seems to fully encounter clavulanic acid inhibitor activity. Additional C. coli isolates with both G57T + ∆A69 genotypes but also isolates showing ∆A69 only would need to be collected to ensure more robust statistical analysis and therefore better assess the correlation between their resistance phenotype and the expression level of BlaOXA61.
According to Tajada et al. (1996) the great activity of clavulanic acid in C. jejuni and C. coli is due to its ability to bind Penicillin Binding Proteins with lower MICs in C. jejuni compared to C. coli. It is therefore maybe not surprising to observe amoxicillin-clavulanic acid resistance only in C. coli. Amoxicillin-clavulanic acid resistance remains however very rare among C. jejuni and C. coli clinical isolates. Nevertheless, such broadened resistance spectrum conferred by overexpression of a beta-lactamases due to point mutations in the promoter region is not unusual, as it has been well described in other species especially E. coli (Corvec et al., 2002; Tracz et al., 2005; Singh et al., 2019). Site-directed mutagenesis to induce changes in the blaOXA61 promoting sequence of amoxicillin-clavulanic acid and ampicillin susceptible isolates could however be performed in order to fully observe the impact on the gene expression.
In conclusion, combined genomic-proteomic approach here allowed us to identify a new blaOXA61 promoting region displaying both G57T mutation and an uncharacterized A69 deletion which leads to a significative overexpression of this beta-lactamase and may be responsible for amoxicillin-clavulanic acid resistance. These results are even more valuable as we lack consensus data on such phenotypes. Indeed, C. jejuni and C. coli breakpoints for amoxicillin-clavulanic acid today are based on that for other species, such as the use of EUCAST Enterobacteriaceae recommendations. Although this mechanism is rare among C. coli clinical isolates, it needs to be seriously considered since C. coli species isolates can easily adapt to their environment.
Data availability statement
The datasets presented in this study can be found in online repositories. The names of the repository/repositories and accession number(s) can be found in the article/Supplementary material.
Ethics statement
Ethical review and approval was not required for the study in accordance with the local legislation and institutional requirements. Written informed consent for participation was not required for this study in accordance with the national legislation and the institutional requirements. Written informed consent was not obtained from the individual(s) for the publication of any potentially identifiable images or data included in this article.
Author contributions
FD: Formal analysis, Methodology, Validation, Writing – original draft, Writing – review & editing. QJ: Formal analysis, Writing review & editing, Software. LB: Methodology, Writing – review & editing. JA: Methodology, Writing – review & editing. RP: Methodology, Writing – review & editing. CD: Methodology, Writing – review & editing. AD: Methodology, Writing – review & editing. MJ: Writing – review & editing. RB: Writing – review & editing, Methodology. FV: Writing – review & editing. JL: Writing – review & editing. PL: Writing – review & editing, Data curation, Formal analysis, Funding acquisition, Methodology, Project administration, Supervision, Validation, Writing – original draft.
Funding
The author(s) declare financial support was received for the research, authorship, and/or publication of this article. Open access funding provided by the French National Reference Center for Campylobacters and Helicobacters, Bordeaux, France. Financially supported by Santé Publique France (www.santepubliquefrance.fr).
Acknowledgments
The authors want to thank all the laboratories that sent Campylobacter isolates to our reference center, particularly the Centre Hospitalier de Cannes (Cannes, France) and the laboratory SYNLAB Beaumont (Beaumont, France), which sent the 3 amoxicillin-clavulanic-acid-resistant C. coli isolates included in the present study. The current manuscript was edited for proper English language using American Journal Experts services (verification code 4A53-3591-EE3C-F0A7-37A0).
Conflict of interest
The authors declare that the research was conducted in the absence of any commercial or financial relationships that could be construed as a potential conflict of interest.
Publisher’s note
All claims expressed in this article are solely those of the authors and do not necessarily represent those of their affiliated organizations, or those of the publisher, the editors and the reviewers. Any product that may be evaluated in this article, or claim that may be made by its manufacturer, is not guaranteed or endorsed by the publisher.
Supplementary material
The Supplementary material for this article can be found online at: https://www.frontiersin.org/articles/10.3389/fmicb.2023.1285236/full#supplementary-material
Footnotes
2. ^https://www.sfm-microbiologie.org/boutique/comite-de-lantibiograme-de-la-sfm-casfm/
3. ^https://db.systemsbiology.net/sbeams/cgi/PeptideAtlas/PASS_View
References
Abay, S., Kayman, T., Otlu, B., Hizlisoy, H., Aydin, F., and Ertas, N. (2014). Genetic diversity and antibiotic resistance profiles of Campylobacter jejuni isolates from poultry and humans in Turkey. Int. J. Food Microbiol. 178, 29–38. doi: 10.1016/j.ijfoodmicro.2014.03.003
Altschul, S. F., Madden, T. L., Schäffer, A. A., Zhang, J., Zhang, Z., Miller, W., et al. (1997). Gapped BLAST and PSI-BLAST: a new generation of protein database search programs. Nucleic Acids Res. 25, 3389–3402. doi: 10.1093/nar/25.17.3389
Anampa, D., Benites, C., Lázaro, C., Espinoza, J., Angulo, P., Díaz, D., et al. (2020). Detection of the ermB gene associated with macrolide resistance in Campylobacter strains isolated from chickens marketed in Lima, Peru. Rev. Panam. Salud Publica Pan Am. J. Public Health 44:e60. doi: 10.26633/rpsp.2020.60
Bankevich, A., Nurk, S., Antipov, D., Gurevich, A. A., Dvorkin, M., Kulikov, A. S., et al. (2012). SPAdes: a new genome assembly algorithm and its applications to single-cell sequencing. J. Comput. Biol. 19, 455–477. doi: 10.1089/cmb.2012.0021
Béjaoui, A., Gharbi, M., Bitri, S., Nasraoui, D., Ben Aziza, W., Ghedira, K., et al. (2022). Virulence profiling, multidrug resistance and molecular mechanisms of Campylobacter strains from chicken carcasses in Tunisia. Antibiotics 11:830. doi: 10.3390/antibiotics11070830
Bessède, E., Solecki, O., Sifré, E., Labadi, L., and Mégraud, F. (2011). Identification of Campylobacter species and related organisms by matrix assisted laser desorption ionization-time of flight (MALDI-TOF) mass spectrometry. Clin. Microbiol. Infect. Off. Publ. Eur. Soc. Clin. Microbiol. Infect. Dis. 17, 1735–1739. doi: 10.1111/j.1469-0691.2011.03468.x
Casagrande Proietti, P., Guelfi, G., Bellucci, S., de Luca, S., di Gregorio, S., Pieramati, C., et al. (2020). Beta-lactam resistance in Campylobacter coli and Campylobacter jejuni chicken isolates and the association between blaOXA-61 gene expression and the action of β-lactamase inhibitors. Vet. Microbiol. 241:108553. doi: 10.1016/j.vetmic.2019.108553
Cecchini, T., Yoon, E. J., Charretier, Y., Bardet, C., Beaulieu, C., Lacoux, X., et al. (2018). Deciphering multifactorial resistance phenotypes in Acinetobacter baumannii by genomics and targeted label-free proteomics. Mol. Cell. Proteomics 17, 442–456. doi: 10.1074/mcp.RA117.000107
Chen, J. C., Tagg, K. A., Joung, Y. J., Bennett, C., Francois Watkins, L., Eikmeier, D., et al. (2018). Report of erm(B)+ Campylobacter jejuni in the United States. Antimicrob. Agents Chemother. 62:e02615-17. doi: 10.1128/AAC.02615-17
Chlebicz, A., and Śliżewska, K. (2018). Campylobacteriosis, salmonellosis, yersiniosis, and listeriosis as zoonotic foodborne diseases: a review. Int. J. Environ. Res. Public Health 15:863. doi: 10.3390/ijerph15050863
Cobo-Díaz, J. F., González del Río, P., and Álvarez-Ordóñez, A. (2021). Whole resistome analysis in Campylobacter jejuni and C. coli genomes available in public repositories. Front. Microbiol. 12:662144. doi: 10.3389/fmicb.2021.662144
Corvec, S., Caroff, N., Espaze, E., Marraillac, J., and Reynaud, A. (2002). −11 mutation in the ampC promoter increasing resistance to β-lactams in a clinical Escherichia coli strain. Antimicrob. Agents Chemother. 46, 3265–3267. doi: 10.1128/AAC.46.10.3265-3267.2002
Deckert, A. E., Reid-Smith, R. J., Tamblyn, S. E., Morrell, L., Seliske, P., Jamieson, F. B., et al. (2013). Antimicrobial resistance and antimicrobial use associated with laboratory-confirmed cases of Campylobacter infection in two health units in Ontario. Can. J. Infect. Dis. Med. Microbiol. 24:e16–21. doi: 10.1155/2013/176494
Delahoy, M. J., Shah, H. J., Weller, D. L., Ray, L. C., Smith, K., McGuire, S., et al. (2023). Preliminary incidence and trends of infections caused by pathogens transmitted commonly through food — foodborne diseases active surveillance network, 10 U.S. Sites, 2022. MMWR Morb. Mortal. Wkly Rep. 72, 701–706. doi: 10.15585/mmwr.mm7226a1
Edgar, R. C. (2004). MUSCLE: multiple sequence alignment with high accuracy and high throughput. Nucleic Acids Res. 32, 1792–1797. doi: 10.1093/nar/gkh340
Elhadidy, M., Miller, W. G., Arguello, H., Álvarez-Ordóñez, A., Dierick, K., and Botteldoorn, N. (2019). Molecular epidemiology and antimicrobial resistance mechanisms of Campylobacter coli from diarrhoeal patients and broiler carcasses in Belgium. Transbound. Emerg. Dis. 66, 463–475. doi: 10.1111/tbed.13046
Fabre, A., Oleastro, M., Nunes, A., Santos, A., Sifré, E., Ducournau, A., et al. (2018). Whole-genome sequence analysis of multidrug-resistant Campylobacter isolates: a focus on aminoglycoside resistance determinants. J. Clin. Microbiol. 56:e00390-18. doi: 10.1128/JCM.00390-18
Fernández-Cruz, A., Muñoz, P., Mohedano, R., Valerio, M., Marín, M., Alcalá, L., et al. (2010). Campylobacter bacteremia: clinical characteristics, incidence, and outcome over 23 years. Medicine 89, 319–330. doi: 10.1097/MD.0b013e3181f2638d
Florez-Cuadrado, D., Ugarte-Ruiz, M., Meric, G., Quesada, A., Porrero, M. C., Pascoe, B., et al. (2017). Genome comparison of erythromycin resistant Campylobacter from turkeys identifies hosts and pathways for horizontal spread of erm(B) genes. Front. Microbiol. 8:2240. doi: 10.3389/fmicb.2017.02240
Fournier, D., Carrière, R., Bour, M., Grisot, E., Triponney, P., Muller, C., et al. (2021). Mechanisms of resistance to ceftolozane/tazobactam in Pseudomonas aeruginosa: results of the GERPA multicenter study. Antimicrob. Agents Chemother. 65:e01117-20. doi: 10.1128/AAC.01117-20
Gharbi, M., Béjaoui, A., Hamrouni, S., Arfaoui, A., and Maaroufi, A. (2023). Persistence of Campylobacter spp. in poultry flocks after disinfection, virulence, and antimicrobial resistance traits of recovered isolates. Antibiot. Basel Switz. 12:890. doi: 10.3390/antibiotics12050890
Greninger, A. L., Addetia, A., Starr, K., Cybulski, R. J., Stewart, M. K., Salipante, S. J., et al. (2020). International spread of multidrug-resistant Campylobacter coli in men who have sex with men in Washington state and Québec, 2015–2018. Clin. Infect. Dis. 71, 1896–1904. doi: 10.1093/cid/ciz1060
Griggs, D. J., Johnson, M. M., Frost, J. A., Humphrey, T., Jørgensen, F., and Piddock, L. J. V. (2005). Incidence and mechanism of ciprofloxacin resistance in Campylobacter spp. isolated from commercial poultry flocks in the United Kingdom before, during, and after fluoroquinolone treatment. Antimicrob. Agents Chemother. 49, 699–707. doi: 10.1128/AAC.49.2.699-707.2005
He, Y., Reed, S., and Strobaugh, T. P. (2020). Complete genome sequence and annotation of Campylobacter jejuni YH003, isolated from retail chicken. Microbiol. Resour. Announc. 9:e01307-19. doi: 10.1128/MRA.01307-19
Hormeño, L., Campos, M. J., Vadillo, S., and Quesada, A. (2020). Occurrence of tet(O/M/O) mosaic gene in tetracycline-resistant Campylobacter. Microorganisms 8:1710. doi: 10.3390/microorganisms8111710
Jain, C., Rodriguez-R, L. M., Phillippy, A. M., Konstantinidis, K. T., and Aluru, S. (2018). High throughput ANI analysis of 90K prokaryotic genomes reveals clear species boundaries. Nat. Commun. 9:5114. doi: 10.1038/s41467-018-07641-9
Jehanne, Q., Bénéjat, L., Ducournau, A., Domingues-Martins, C., Cousinou, T., Bessède, E., et al. (2021). Emergence of erythromycin resistance methyltransferases in Campylobacter coli strains in France. Antimicrob. Agents Chemother. 65:e0112421. doi: 10.1128/AAC.01124-21
Jehanne, Q., Pascoe, B., Bénéjat, L., Ducournau, A., Buissonnière, A., Mourkas, E., et al. (2020). Genome-wide identification of host-segregating single-nucleotide polymorphisms for source attribution of clinical Campylobacter coli isolates. Appl. Environ. Microbiol. 86:e01787-20. doi: 10.1128/AEM.01787-20
Joshi, N., and Fass, J. Sickle: a sliding-window, adaptive, quality-based trimming tool for FastQ files (Version 1.33) [software]. (2011).
Ladely, S. R., Meinersmann, R. J., Englen, M. D., Fedorka-Cray, P. J., and Harrison, M. A. (2009). 23S rRNA gene mutations contributing to macrolide resistance in Campylobacter jejuni and Campylobacter coli. Foodborne Pathog. Dis. 6, 91–98. doi: 10.1089/fpd.2008.0098
Lehours, P, Bessède, E., Jauvain, M., Bénéjat, L., Jehanne, Q., Ducournau, A., et al. French National Reference Center for Campylobacters & Helicobacters (Bordeaux Hospital University Center). 2022 campylobacters surveillance report. French National Reference Center for Campylobacters & Helicobacters, Bordeaux, France. (2023).
Ludwig, C., Claassen, M., Schmidt, A., and Aebersold, R. (2012). Estimation of absolute protein quantities of unlabeled samples by selected reaction monitoring mass spectrometry. Mol. Cell. Proteomics 11:M111.013987. doi: 10.1074/mcp.M111.013987
Pearson, B. M., Rokney, A., Crossman, L. C., Miller, W. G., Wain, J., and van Vliet, A. H. M. (2013). Complete genome sequence of the Campylobacter coli clinical isolate 15-537360. Genome Announc. 1:e01056-13. doi: 10.1128/genomeA.01056-13
Pivard, M., Bastien, S., Macavei, I., Mouton, N., Rasigade, J. P., Couzon, F., et al. (2023). Targeted proteomics links virulence factor expression with clinical severity in staphylococcal pneumonia. Front. Cell. Infect. Microbiol. 13:1162617. doi: 10.3389/fcimb.2023.1162617
Post, A., Martiny, D., van Waterschoot, N., Hallin, M., Maniewski, U., Bottieau, E., et al. (2017). Antibiotic susceptibility profiles among Campylobacter isolates obtained from international travelers between 2007 and 2014. Eur. J. Clin. Microbiol. Infect. Dis. 36, 2101–2107. doi: 10.1007/s10096-017-3032-6
Pritchard, J. K., Stephens, M., and Donnelly, P. (2000). Inference of population structure using multilocus genotype data. Genetics 155, 945–959. doi: 10.1093/genetics/155.2.945
Qin, S., Wang, Y., Zhang, Q., Zhang, M., Deng, F., Shen, Z., et al. (2014). Report of ribosomal RNA methylase gene erm(B) in multidrug-resistant Campylobacter coli. J. Antimicrob. Chemother. 69, 964–968. doi: 10.1093/jac/dkt492
Schiaffino, F., Colston, J. M., Paredes-Olortegui, M., François, R., Pisanic, N., Burga, R., et al. (2019). Antibiotic resistance of Campylobacter species in a pediatric cohort study. Antimicrob. Agents Chemother. 63:e01911-18. doi: 10.1128/aac.01911-18
Singh, T., Singh, P. K., das, S., Wani, S., Jawed, A., and Dar, S. A. (2019). Transcriptome analysis of beta-lactamase genes in diarrheagenic Escherichia coli. Sci. Rep. 9:3626. doi: 10.1038/s41598-019-40279-1
Tajada, P., Gomez-Graces, J. L., Alós, J. I., Balas, D., and Cogollos, R. (1996). Antimicrobial susceptibilities of Campylobacter jejuni and Campylobacter coli to 12 beta-lactam agents and combinations with beta-lactamase inhibitors. Antimicrob. Agents Chemother. 40, 1924–1925. doi: 10.1128/AAC.40.8.1924
The European Union One Health (2021) Zoonoses Report | EFSA. Available at: https://www.efsa.europa.eu/en/efsajournal/pub/7666 (2022).
Thépault, A., Méric, G., Rivoal, K., Pascoe, B., Mageiros, L., Touzain, F., et al. (2017). Genome-wide identification of host-segregating epidemiological markers for source attribution in Campylobacter jejuni. Appl. Environ. Microbiol. 83:e03085-16. doi: 10.1128/AEM.03085-16
Tinévez, C., Velardo, F., Ranc, A. G., Dubois, D., Pailhoriès, H., Codde, C., et al. (2022). Retrospective multicentric study on Campylobacter spp. bacteremia in France: the campylobacteremia study. Rev. Infect. Dis. 75, 702–709. doi: 10.1093/cid/ciab983
Tracz, D. M., Boyd, D. A., Bryden, L., Hizon, R., Giercke, S., van Caeseele, P., et al. (2005). Increase in ampC promoter strength due to mutations and deletion of the attenuator in a clinical isolate of cefoxitin-resistant Escherichia coli as determined by RT–PCR. J. Antimicrob. Chemother. 55, 768–772. doi: 10.1093/jac/dki074
Wallace, R., Bulach, D., McLure, A., Varrone, L., Jennison, A. V., Valcanis, M., et al. (2021). Antimicrobial resistance of Campylobacter spp. causing human infection in Australia: an international comparison. Microb. Drug Resist. 27, 518–528. doi: 10.1089/mdr.2020.0082
Wallace, R. L., Bulach, D., Valcanis, M., Polkinghorne, B. G., Pingault, N., Stylianopoulos, A., et al. (2020). Identification of the first erm(B)-positive Campylobacter jejuni and Campylobacter coli associated with novel multidrug resistance genomic islands in Australia. J. Glob. Antimicrob. Resist. 23, 311–314. doi: 10.1016/j.jgar.2020.09.009
Wieczorek, K., and Osek, J. (2013). Antimicrobial resistance mechanisms among Campylobacter. Biomed. Res. Int. 2013:340605. doi: 10.1155/2013/340605
Zacharow, I., Bystroń, J., Wałecka-Zacharska, E., Podkowik, M., and Bania, J. (2015). Prevalence and antimicrobial resistance of Arcobacter butzleri and Arcobacter cryaerophilus isolates from retail meat in lower Silesia region, Poland. Pol. J. Vet. Sci. 18, 63–69. doi: 10.1515/pjvs-2015-0008
Keywords: AMR, amoxicillin-clavulanic acid, Campylobacter, beta-lactamase, gene expression
Citation: Deforet F, Jehanne Q, Bénéjat L, Aptel J, Prat R, Desbiolles C, Ducournau A, Jauvain M, Bonnet R, Vandenesch F, Lemoine J and Lehours P (2023) Combined genomic-proteomic approach in the identification of Campylobacter coli amoxicillin-clavulanic acid resistance mechanism in clinical isolates. Front. Microbiol. 14:1285236. doi: 10.3389/fmicb.2023.1285236
Edited by:
Ozan Gundogdu, University of London, United KingdomReviewed by:
Marja-Liisa Hänninen, University of Helsinki, FinlandMohamed K. Fakhr, University of Tulsa, United States
Copyright © 2023 Deforet, Jehanne, Bénéjat, Aptel, Prat, Desbiolles, Ducournau, Jauvain, Bonnet, Vandenesch, Lemoine and Lehours. This is an open-access article distributed under the terms of the Creative Commons Attribution License (CC BY). The use, distribution or reproduction in other forums is permitted, provided the original author(s) and the copyright owner(s) are credited and that the original publication in this journal is cited, in accordance with accepted academic practice. No use, distribution or reproduction is permitted which does not comply with these terms.
*Correspondence: Philippe Lehours, philippe.lehours@u-bordeaux.fr
†ORCID: Philippe Lehours https://orcid.org/0000-0001-6077-1825