- 1International Centre for Advanced Mediterranean Agronomic Studies (CIHEAM of Bari), Bari, Italy
- 2National Research Council of Italy (CNR), Institute for Sustainable Plant Protection (IPSP), University of Bari, Bari, Italy
- 3Department of Soil, Plant and Food Science, University of Bari, Bari, Italy
- 4Department of Chemistry, University of Bari, Bari, Italy
- 5National Research Council of Italy (CNR), Institute for Sustainable Plant Protection (IPSP), Naples, Italy
The lack of sustainable strategies for combating Xylella fastidiosa (Xf) highlights the pressing need for novel practical antibacterial tools. In this study, Lactococcus lactis subsp. lactis strain ATCC 11454 (L. lactis), known for its production of nisin A, was in vitro tested against Xf subsp. pauca. Preliminary investigations showed that nisin A was involved in a strong antagonistic activity exhibited by L. lactis against Xf. Thus, the efficacy of nisin A was comprehensively assessed through a combination of in vitro and in planta experiments. In vitro investigations employing viable-quantitative PCR, spot assay, turbidity reduction assay, fluorescence microscopy, and transmission electron microscopy demonstrated nisin’s robust bactericidal effect on Xf at a minimal lethal concentration of 0.6 mg/mL. Moreover, results from fluorescence and transmission electron microscopies indicated that nisin directly and rapidly interacts with the membranes of Xf cells, leading to the destruction of bacterial cells in few minutes. In in planta tests, nisin also demonstrated the ability to tackle Xf infections within Nicotiana benthamiana plants that remained asymptomatic 74 days post inoculation. Furthermore, RPLC-ESI-MS/MS analyses showed that nisin translocated to all parts of the plants and remains intact for up to 9 days. For the first time, this study underscores the nisin-based strategy as a realistic and eco-friendly approach to be further investigated against Xf infections in the field.
1 Introduction
Xylella fastidiosa (Xf) is a Gram-negative bacterium that continues to inflict substantial losses to various important crops throughout the world, including coffee, citrus, grapevine, olive, and almond. In Italy, following the initial detection of Xf subsp. pauca in Apulia region in 2013, it has emerged as the predominant threat to olive trees in the country (Velasco-Amo et al., 2023). Xylella fastidiosa infections have led to a significant decline in olive crop yields, with approximately 5 million trees infected or dead, representing losses equivalent to around 10% of the Italian olive production (White et al., 2020). Beyond the direct economic implications, Xf diseases have also exerted noteworthy societal impacts (Ali et al., 2021). The challenges arise from the scarcity of effective bactericides and the inherent difficulty in accessing the vascular system of the plant, where the pathogen establishes itself (Ali et al., 2021). Although, in rare cases, some treatments promise to reduce the population level of the bacteria in infected plants, the challenge persists due to the lack of sustainability of these treatments (Scortichini et al., 2021). This underscores the pressing need for novel biocontrol strategies in Xf disease management. In response to these challenges, environmentally friendly options and sustainable strategies that conform to European natural trends are needed. Bacteriocins, which are polypeptides or precursor polypeptides with bacteriostatic or bactericidal activity produced by certain bacteria in the metabolic process through ribosome synthesis mechanism, have become a research hotspot for new antibacterial agents due to their low cost, complex and diverse bactericidal mechanisms of action (Simons et al., 2020). Among bacteriocins, nisin A (hereafter named nisin) represents the most prominent and widely applied natural bacteriocin with high antibacterial activity against a wide range of Gram-positive bacteria (Field et al., 2023).
Nisin, a member of the class I bacteriocins referred to as lantibiotics, is produced by certain Lactococcus lactis subsp. lactis strains (L. lactis) (Field et al., 2023). It is distinguished by a cationic, polycyclic, thermostable peptide structure with a molecular weight of 3.4 kDa, and a broad spectrum of antibacterial activity primarily targeting Gram-positive bacteria (Małaczewska and Kaczorek-Łukowska, 2021). The antibacterial activity of nisin against Gram-positive and -negative bacteria stems from its triple mode of action, which includes binding to lipid II, a crucial precursor in peptidoglycan biosynthesis, formation of pores within the cell membrane made up of lipid II, and directly degrading target cell DNA (Field et al., 2023). By binding to lipid II and forming pores in the membrane, nisin can cause the efflux of cellular constituents and inhibit cell wall biosynthesis. However, the activity of nisin against Gram-negative bacteria could in some cases be much lower than that against Gram-positive bacteria, mainly because lipid II is located at the inner membrane, and the rather impermeable outer membrane in Gram-negative bacteria prevents nisin from reaching lipid II (Li et al., 2018). Leveraging its potent antimicrobial properties, minimal propensity to induce bacterial resistance, and low cellular cytotoxicity at antimicrobial concentrations, nisin has proven highly effective against drug-resistant bacteria (Barbosa et al., 2021) and has found successful application in the field of food preservation (Ibarra-Sánchez et al., 2020). These characteristics in conjunction with its generally regarded as safe (GRAS) status and its stability under various conditions, have contributed to the worldwide success of nisin as a natural food preservative (E234) (Field et al., 2023). These advantages collectively make nisin a potential antibacterial agent for the control of bacterial plant diseases, potentially complementing existing biocontrol agents in the pursuit of integrative, sustainable, and environmentally friendly agriculture.
Despite the recognized importance of nisin, it has not been yet examined for its efficacy against plant pathogenic bacteria. This study addresses this gap by presenting, for the first time, an in-depth investigation into the antagonistic effect of L. lactis and its well-established bacteriocin, nisin, against Xf subsp. pauca both in vitro and in planta.
2 Materials and methods
2.1 Bacterial and growth conditions
Xylella fastidiosa subsp. pauca strain A0PT1, isolated from olive trees affected by the olive quick decline syndrome (OQDS) in southern Italy, Apulia region, was used in all experiments. The strain was stored in Pierce disease broth (PD2) (Davis et al., 1980) supplemented with glycerol (50%) and maintained at −80°C. When needed, aliquots were cultured in buffered charcoal yeast extract (BCYE) (Wells et al., 1981) agar plates and grown at 28°C for 10 to15 days. Cell suspensions were prepared using phosphate buffered saline (PBS, pH 7.4, 0.01 M) and adjusted to 108 CFU/mL (OD600 ≅ 0.32) (El Handi et al., 2022). L. lactis subsp. lactis ATCC 11454 was grown on YPGA plates (5.0 g/L yeast extract, 5.0 g/L peptone, 10.0 g/L glucose, and 15.0 g/L agar) for 2 days at 28°C.
Nisin (ITSISLCTPGCKTGALMGCNMKTATCHCSIHVSK), extracted and purified from L. lactis, was purchased from Sigma-Aldrich (Merck KGaA, Darmstadt, Germany). According to the manufacturer, the formulation contains 2.5% (w/w) pure nisin with potency ≥900 IU/mg. Lyophilized nisin was solubilized in sterile Milli-Q water to a stock concentration of 12.5 mg/mL and filter sterilized through a 0.22 μm nylon Acrodisc® syringe filter (Merck, Rome, Italy).
2.2 In-vitro investigations for antagonism between Lactococcus lactis subsp. lactis and Xylella fastidiosa
The antagonistic effect of L. lactis subsp. lactis ATCC 11454 against Xf subsp. pauca was investigated as follows: four drops of Xf suspension (108 CFU/mL), each containing 30 μL, were positioned at the upper portion of the BCYE agar plates, with approximately 1.5 cm between them. These drops were allowed to slowly descend to the opposite side, forming four parallel rows of Xf cultures. Subsequently, two drops (10 μL each) of Lactococcus lactis suspension (108 CFU/mL), prepared in PBS, were administered to the center of the rows of Xf cultures after drying under the laminar flow hood. PBS served as the negative control. Following an incubation period of 10 days at 28°C, the antagonistic activity of Lactococcus lactis was perceived as an inhibition zone of Xf growth, which was measured using a digital caliper. This experiment was conducted in triplicate for reliability.
2.3 Characterization of proteins secreted by Lactococcus lactis challenged by Xylella fastidiosa
Reversed-phase liquid chromatography (RPLC) coupled with electrospray (ESI) and tandem mass spectrometry (MS/MS) was used to explore the proteins involved in the inhibitory effect induced by L. lactis against Xf. For inhibition effect examination, two pieces of culture medium from the inhibition zone were cut and subjected to extraction with 200 μL of 10 mM Tri-HCl for an easy protein/peptide recovery. In addition, 100 μL of L. lactis suspension (108 CFU/mL) was challenged by 100 μL of Xf suspension (108 CFU/mL) in 2 mL of YPG liquid medium for 24 h at 28°C. After incubation, the mixture was passed through a 0.22 μm pore filter and subjected to RPLC-ESI-MS/MS.
For analysis, water, acetonitrile, and formic acid LC-MS grade were purchased from Merck (Milan, Italy). Five microliters of the extract or standard solutions were injected in the UHPLC Ultimate 3000 system (Dionex Thermo Fisher Scientific, Italy) coupled to HESI (heated electrospray ionization) interface and VelosPro mass spectrometer (Thermo Scientific, Waltham, MA, United States) equipped with a double linear trap mass analyzer. The reversed-phase (RP) chromatographic separations were accomplished at 40°C using a Phenomenex Aeris WIDEPORE 200 Å C18 column (250 × 2.1 mm, 3.6 μm) equipped with Phenomenex AJO 8783 WIDEPORE C18 (2 × 2.1 mm ID) security guard cartridge and a mobile phase based on H2O (solvent A) and ACN (solvent B) both containing 0.1% of formic acid. Specifically, the gradient used during each chromatographic run, at a flow rate of 0.200 mL/min, was the following: 0–3 min linear from 5 to 20% solvent B; 3–5 min 20% solvent B; 5–12 min linear from 20 to 85% (v/v) solvent B; 12–16 min isocratic at 85% of solvent B; 16–17 min back to the initial composition, followed by 5 min equilibration time. Mass spectrometry analyses were carried out in full scan and in selected reaction monitored (SRM) transitions mode in positive polarity or in targeted MS/MS mode.
2.4 Nisin minimal lethal concentration
Given the unique characteristics of Xf (slow growing propriety), an initial in vitro screening was performed to determine an approximate initial concentration of nisin suitable for subsequent challenges to Xf cells. This preliminary screening was assessed by a contact test coupled with viable-quantitative PCR (v-qPCR) using the PMAxx™ (Biotium, Rome, Italy) (Baró et al., 2020). PMAxx™ is a photo-reactive dye that forms a covalent bond with disrupted DNA, rendering it un-amplifiable by PCR. This unique feature makes PMAxx™ extremely useful in selective detection of bacterial cells with intact plasma membranes by qPCR. Briefly, 50 μL of Xf cells with OD at 600 nm of 0.32 measured by NanoDrop™ One/OneC Microvolume UV–Vis Spectrophotometer (Thermo Fisher Scientific), were treated with 50 μL of nisin at 6, 3, 1.5, 1, 0.8, 0.6, 0.4, 0.2, 0.1, and 0.05 mg/mL. Consequently, series of dilutions of Xf ranging from OD = 0.32 to OD = 0.02 were employed as controls, and samples were incubated for 3 h at 28°C. After incubation, samples were treated with PMAxx™ at a final concentration of 7.5 μM, incubated in the dark at room temperature for 8 min, and followed by a 15 min photoactivation step using the PMA-Lite™ LED Photolysis Device. Genomic DNA of all samples was extracted following the CTAB protocol (Burbank and Ortega, 2018). For analysis, a TaqMan-based qPCR assay was carried out in a thermocycler apparatus (Bio-Rad CFX96, BioRad, Milan, Italy), utilizing specific primers Xf-F: 5′-CACGGCTGGTAACGGAAGA-3′ and Xf-R: 5′-GGGTTGCGTGGTGAAATCAAG-3′, along with the probe Xf-Prb: 5′-6FAM-TCGCATCCCGTGGCTCAGTCC-BHQ-1-3′ (Burbank and Ortega, 2018).
2.5 Spot assay
To corroborate the findings derived from the v-qPCR assay pertaining to the lethal concentrations of nisin against Xf, a spot assay was conducted as follows: Xf subsp. pauca was cultured at 28°C on BCYE agar plates for up to 10 days. Then, the cultures were suspended in PBS and 30 μL of bacterial suspension (OD600 = 0.32) were mixed with 30 μL of nisin at 3, 1.5, 1, 0.8, and 0.6 mg/mL. Subsequently, the mixtures were spotted onto the surface of the BCYE agar plates and allowed to air-dry within the laminar flow hood. Negative controls consisted of PBS, while positive controls involved 30 μL of Xf mixed with 30 μL of PBS (Mourou et al., 2022). Growth inhibition was assessed 10 days post-incubation at 28°C, checked by the level of Xf growth. The experiment was conducted with three independent replicates.
2.6 Turbidity reduction assay
The effect of the minimal lethal concentration (MLC) of nisin on Xf cells in liquid medium was assessed by quantifying the degree of turbidity at OD600 in both nisin-treated and untreated Xf suspensions over 3 h. Briefly, a 50 μL aliquot of Xf suspension in PD2 (Pierce disease 2 media) was mixed with 50 μL of nisin at 0.6 mg/mL, resulting in a final turbidity of Xf with an OD600 of 0.41. Untreated control involved 50 μL of Xf mixed with 50 μL of PBS with a final turbidity of 0.41 at OD600. Bacterial turbidity was assessed by tracking the OD600 using the NanoDrop™ One/OneC Microvolume UV–Vis Spectrophotometer at 0 min, 1 h, 2 h, and 3 h of incubation at 28°C. The experiment was conducted twice, with three replicates for each treatment.
2.7 Fluorescence microscopy
Fluorescence microscopy (FM) provides an accurate assessment methodology for the bacterial lysis process by enumerating the populations of intact and permeable cells at various time intervals. In this context, 100 μL Xf suspension (OD600 = 0.32) was mixed with 100 μL of nisin at its MLC (0.6 mg/mL) and incubated at room temperature for 3 h. Untreated control consisted of bacterial suspension treated with sterile distilled water. To assess the viability of Xf cells exposed to nisin, the LIVE/DEAD® BacLight™ viability kit (provided by Molecular Probes) was employed. This kit contains two nucleic acid dyes, SYTO 9 and propidium iodide (PI), which enable the differentiation of live cells with intact plasma membranes (visualized in the green channel) from bacteria that have succumbed to membrane compromise due to nisin activity (visible in the red channel). Photomicrographs were captured at 15 min, 30 min, 1 h, 2 h and 3 h post-incubation, using a Nikon E800 microscope equipped with fluorescein isothiocyanate (480/30 excitation filter, DM505 dichroic mirror, 535/40 emission filter) and tetramethyl rhodamine isothiocyanate (546/10 excitation filter, DM575 dichroic mirror, 590 emission filter) fluorescence filter sets.
2.8 Transmission electron microscopy
To scrutinize the antibacterial properties of nisin against Xf cells, a suspension of Xf (OD600 = 0.32) was exposed to nisin at 0.6 mg/mL for a duration of 3 h at room temperature. Representative images were taken after 3 h via transmission electron microscopy (TEM) (FEI MORGAGNI 282D, United States) using the dip method. Briefly, carbon-coated copper/rhodium grids were immersed in both the nisin-treated and untreated bacterial suspensions for 5 min, followed by a rinse with 200 μL of distilled water. To achieve negative staining, the grids were floated on 200 μL of a 0.5% w/v UA-zero EM stain solution (Agar-Scientific Ltd., Stansted, United Kingdom), and observed under an accelerating voltage of 80 kV.
2.9 Spectrum of action
The nisin’s spectrum of action at MLC (0.6 mg/mL) was assessed by targeting various pathogenic and beneficial plant bacteria using the spot assay. Therefore, 16 phytopathogenic bacterial isolates, including three different isolates of Xf subsp. pauca, together with one epiphytic (Pantoea agglomerans) and three endophytic bacteria (Bacillus subtilis, Paenibacillus rigui, and Bacillus pumilus) isolated from olive trees, which are reported in the literature to exhibit antibacterial activity against Xf subsp. pauca (Mourou et al., 2022), were examined (Table 1). For Xf isolates, spot tests were carried out on BCYE agar plates as described earlier. For other bacteria, spot tests were performed on YPGA plates as follows: bacterial isolates were cultured at 28°C on YPGA plates for up to 2 days. Then, the cultures were suspended in sterile distilled water and 200 μL of bacterial suspension (OD600 = 0.2) were mixed with 6 mL of YPG soft agar (i.e., YPG supplemented with 0.7% agar), poured into YPGA plates, and allowed to dry. Subsequently, drops of 10 μL of nisin were spotted onto the surface of the plates. Spots were dried at room temperature and the plates cultured for up to 2 days at 28°C. The presence of a clear zone in the spot area was indicative of bacterial susceptibility to nisin. The experiment was replicated three times for validation.
2.10 In planta antagonistic activity of nisin against Xylella fastidiosa
To explore the antagonistic effect of nisin on Xf within plant tissues, an in-planta assay was conducted. This involved the Xf infection and the application of nisin to the stems of Nicotiana benthamiana (N. benthamiana), which was validated as a model plant host for Xf (Baró et al., 2022). Briefly, one-month-old N. benthamiana plants were inoculated using a 0.1 mL insulin syringe, with the needle penetrating approximately half the plant stem diameter to access the vascular system. Two inoculations (25 μL each) of Xf suspension (OD600 = 0.32), prepared in PBS, were administered on the same side of the stem in a section of 4 cm at around 10 cm above the soil level. Two applications of 50 μL each of the nisin at 6 mg/mL (100 μL of nisin/plant, corresponding to 0.6 mg of nisin per plant) were performed at the same side of the stem in section of 8 cm at around 8 cm above the soil level (Figure 1). Preventive treatment with nisin was performed 24 h prior to Xf inoculation, whereas curative treatment was carried out 24 h after Xf inoculation. Each treatment consisted of 10 plants, including both positive and negative control groups, and inoculated plants were maintained in a controlled quarantine laboratory environment at 25°C. Two separate experiments were conducted to ensure the reliability of our findings.
Over a period of 74 days post inoculation (dpi), inoculated plants were visually inspected for the development of characteristic symptoms of Xf (i.e., leaf scorch), and movement of Xf within the plant was ascertained through TaqMan qPCR assay applied on genomic DNA extracted from leaves above the inoculation points (Figure 1). At the end of the experiment, leaves exhibiting suspected symptoms were analyzed for the presence of Xf using TaqMan qPCR assay as described earlier. Briefly, 1 g of leaf tissue was grinded with 2 mL of CTAB buffer, followed by heating at 65°C for 30 min. The plant extract was then centrifuged at 13,000 g for 15 min, and the resulting supernatant was washed with chloroform and precipitated in cold isopropyl alcohol. The resulting pellet, which contained the total DNA, was diluted in 100 μL of sterile water. Subsequently, 50 ng of this DNA was used for the TaqMan qPCR.
2.11 Stability and translocation of nisin in plants
To explore the stability and translocation of nisin throughout the plant parts, one-month-old N. benthamiana plants (3 replicates) were inoculated as described in in planta section with 100 μL of nisin at 6 mg/mL. The plants were divided into five sections, with nisin inoculation carried out in the stem of section 3 (Figure 2). After 24 h, 48 h, 6 days, and 9 days, stems and leaves of each section were collected separately, ground in a mortar with 1 mL of sterile Milli-Q water, and then transferred to a centrifuge tube. After centrifugation at 3,000 g for 5 min at 5°C, the supernatant was filtered through a 0.22 μm nylon filter for RPLC-ESI-MS/MS analysis, using the same instrumentation as described above, with the following modifications. The gradient used for nisin quantification was: 0–2 min at 20% solvent B; 2–6 min linear from 20 to 85% (v/v) of solvent B; 6–9 min isocratic at 85% of solvent B; 9–10 min back to the initial composition, followed by 5 min equilibration time. For MS acquisitions, parameters were set as follows: sheath gas flow rate 35 arbitrary units (a.u.); auxiliary gas flow rate 5 a.u.; spray voltage 3.5 kV; capillary temperature 320°C; S-Lens radio frequency level 60 a.u. Acquisitions were performed in positive ion mode within the m/z interval 200–2000 and collisionally induced ionization (CID) energy was set as 35% a.u. for MS/MS ions fragmentation. Nisin was detected as tetra-charged [M + 4H]4+ or tri-charged [M + 3H]3+ ion, respectively, at m/z 839.3 and m/z 1118.7. Data were acquired in positive ionization with two transitions: 839.3 → 1080.7 and 1118.7 → 1451.7. The nisin standard A from Lactococcus lactis was used for calibration directly in plant sap to compensate the matrix effect exploring concentration from 6 μg/mL down to 0.06 ng/mL. The linearity of the method was determined by analyzing the standard plots associated with an 8-point standard calibration curve and the method was found to be linear in 3 orders of magnitude in the range 60–0.06 ng/mL. The LOD was calculated as 3 × the SD of the intercept or slope of the calibration curve, and LOQ was calculated as 10 × the SD of the intercept or slope of the calibration curve. The software Xcalibur 2.2 SP1.48 (Thermo Scientific) was used for raw RPLC-MS data.
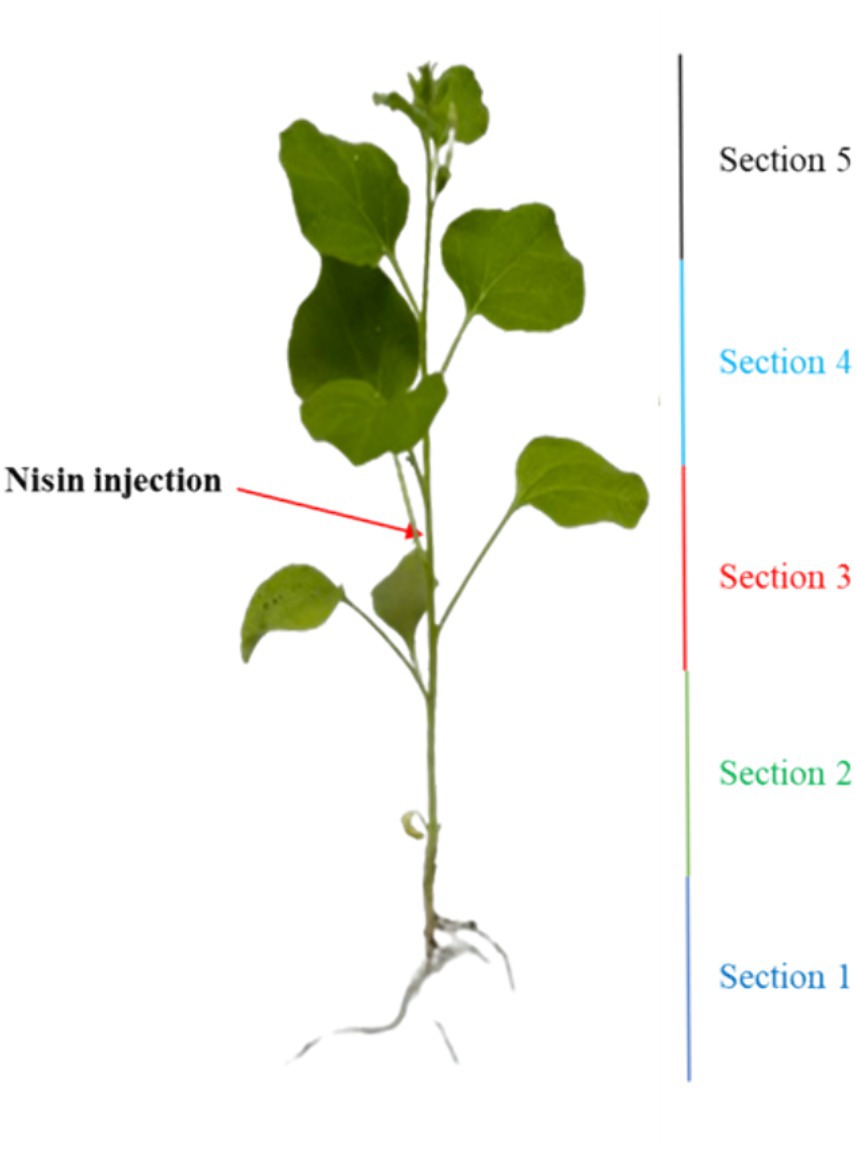
Figure 2. Illustration outlining the nisin injection and the segmentation of the plant into five distinct sections.
3 Results
3.1 Antagonistic activity of Lactococcus lactis against Xylella fastidiosa
The inhibitory activity of L. lactis against Xf growth was strong with an inhibition zone of 33.2 mm, highlighting the efficacy of L. lactis in restraining the Xf growth (Figure 3). In addition, L. lactis exhibited a lateral inhibition expansion, hindering the growth of Xf in the adjacent PBS-treated row C (Figure 3), demonstrating a high antagonism between L. lactis and Xf.
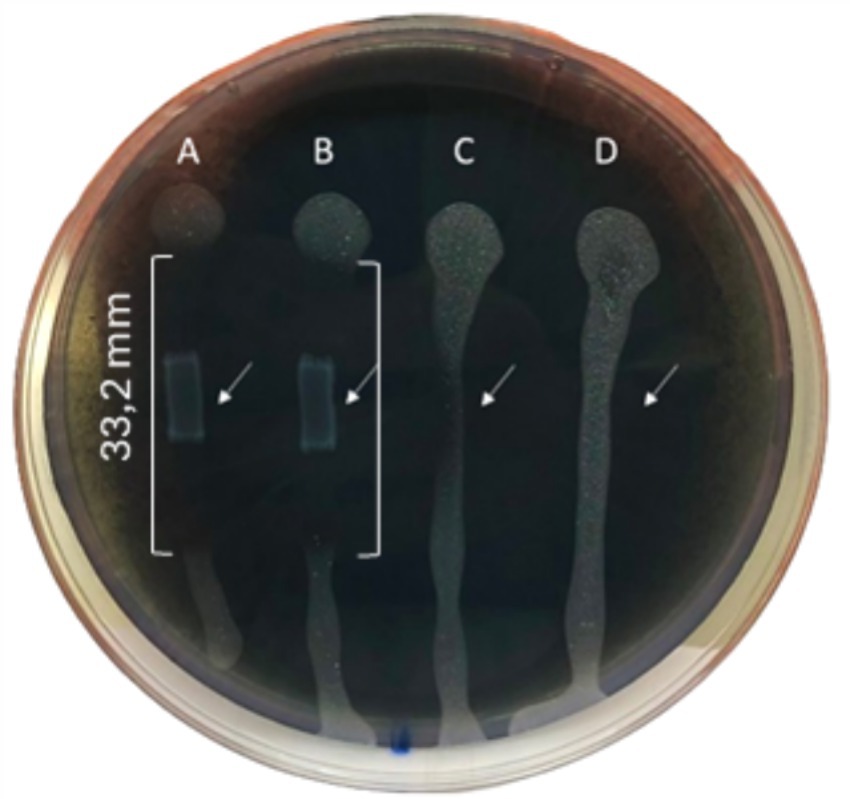
Figure 3. BYCE plate showing the antagonistic activity of Lactococcus lactis subsp. lactis against Xylella fastidiosa subsp. pauca. (A,B) Xylella fastidiosa challenged with Lactococcus lactis subsp. lactis. (C,D) Xylella fastidiosa treated with PBS. Brackets indicate the inhibition zones. Arrows indicate the sites of treatments.
3.2 Characterization of Lactococcus lactis-secreted proteins
The RPLC-ESI-MS/MS analyses conducted on the inhibition zone, to investigate about the nature of compounds involved in the inhibitory activity of L. lactis against Xf, revealed the existence of nine peptides reported in Table 2. These peptides derive from different protein sources associated to plasma membrane or trans-membrane proteins of L. lactis. Some of these proteins are related to ATP-binding or hydrolysis activity, other to sugar, amino-sugar, and hexosamine metabolism (Table 2). The analyses could not reveal the presence of nisin in the inhibition zones, but the amino acid sequence of the transmembrane nisin-resistance protein was detected, which is a normally overexpressed by L. lactis to protect itself from the inhibitory effect of nisin. However, the RPLC-ESI-MS/MS has detected the presence of nisin, in a concentration of 345 ng/mL in the liquid medium after 24 h of incubation. This finding was taken as empirical evidence on the involvement of nisin in the inhibition of Xf growth. Based on this, we proceeded forward in the experimental design using pure nisin A in our research endeavors.
3.3 Determination of MLC of nisin
During this investigation, the antimicrobial efficacy of nisin against Xf was systematically assessed across different concentrations (6, 3, 1.5, 1, 0.8, 0.6, 0.4, 0.2, 0.1, 0.05 mg/mL). Employing v-qPCR as an initial in vitro screening method (Figure 4), the study aimed to determine the MLC of nisin for Xf. The obtained results were subsequently validated through a spot assay (Figure 5). According to the v-qPCR, nisin exhibited exceptional potency, effectively lysing Xf at different concentrations, with 0.6 mg/mL as the MLC. The spot assay results also supported this finding, showing the absence of Xf growth at concentration of nisin above 0.6 mg/mL.
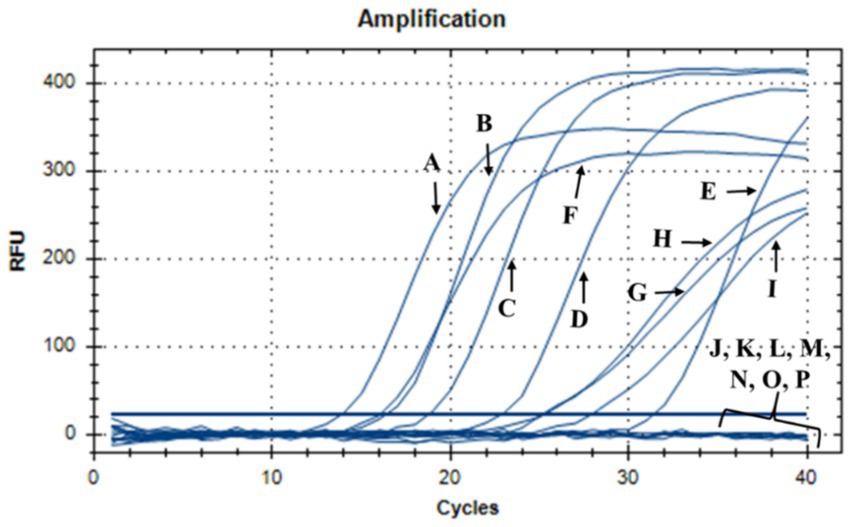
Figure 4. v-qPCR assay showing DNA amplification curves obtained from untreated Xf: (A) Xf (OD600 = 0.32), (B) Xf (OD600 = 0.16), (C) Xf (OD600 = 0.08), (D) Xf (OD600 = 0.04), (E) Xf (OD600 = 0.02), and from nisin-treated Xf: (F) nisin 0.05 mg/mL, (G) nisin 0.1 mg/mL, (H) nisin 0.2 mg/mL, (I) nisin 0.4 mg/mL, (J) nisin 0.6 mg/mL, (K) nisin 0.8 mg/mL, (L) nisin 1 mg/mL, (M) nisin 1.5 mg/mL, (N) nisin 3 mg/mL, (O) nisin 6 mg/mL. (P) Sterile distilled water used as negative control.
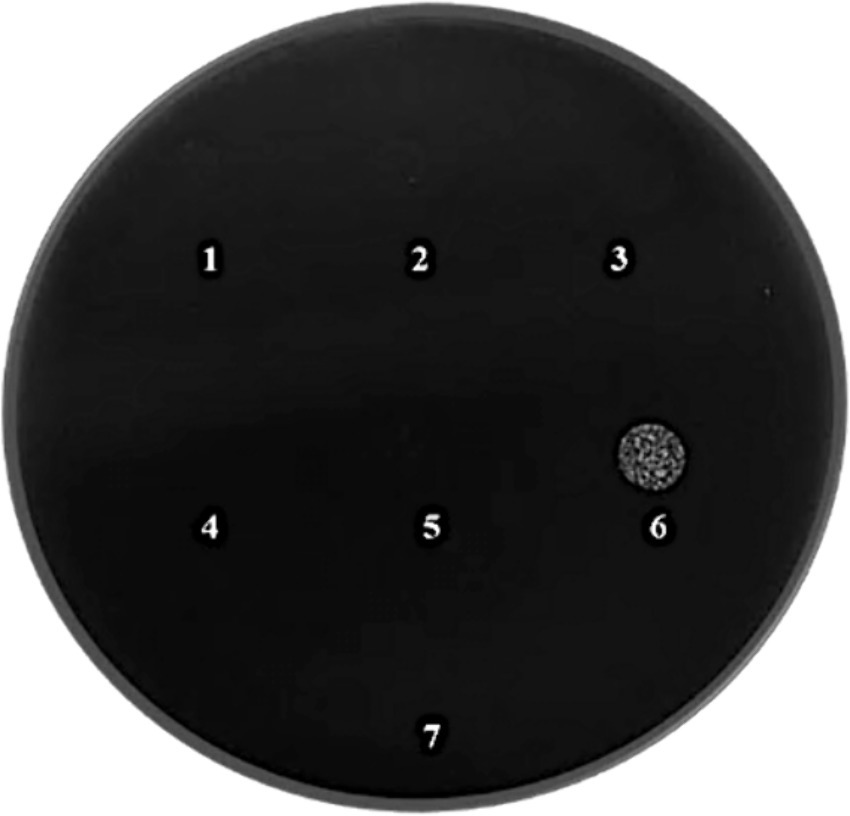
Figure 5. BCYE agar plate showing the spot assay of nisin against Xf; (1) nisin 3 mg/mL-treated Xf; (2) nisin 1.5 mg/mL-treated Xf; (3) nisin 1 mg/mL-treated Xf; (4) nisin 0.8 mg/mL-treated Xf; (5) nisin 0.6 mg/mL-treated Xf; (6) Untreated Xf used as a positive control; (7) PBS used as a negative control.
3.4 Bactericidal activity of nisin against Xylella fastidiosa
The response of Xf to nisin at 0.6 mg/mL was assessed during a 3 h incubation period. In summary, nisin showed almost complete reductive activity against Xf cells within the first hour of exposure, with a reduction of up to 90% (Figure 6). Whereas, this reductive activity endured for the entire 3 h post-contact period, resulting in the complete elimination of Xf cells (Figure 6). The efficacy of nisin against Xf cells was also investigated and confirmed by fluorescence microscopy (FM) using two dyes capable of distinguishing between live cells with intact plasma membranes (green channel) and dead bacteria with membranes compromised by nisin (red channel). The micrographs obtained corroborate the results of the turbidity assay, confirming once again that nisin exhibits significant and rapid destructive activity on Xf, as evidenced by the intense red channels, indicative of substantial lysing of Xf cells (Figure 7).
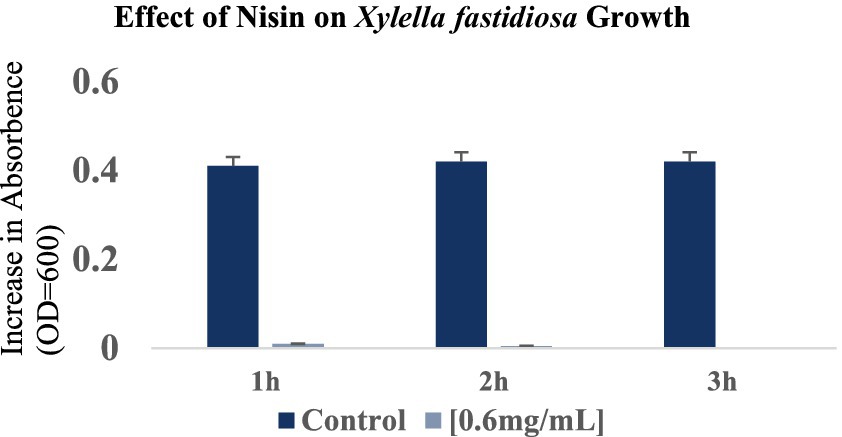
Figure 6. Optical density values at 600 nm showing the reduction in Xylella fastidiosa cell growth at 1 h, 2 h and 3 h after the addition of nisin at 0.6 mg/mL. The histograms show the mean values of three experimental repetitions with standard deviation.
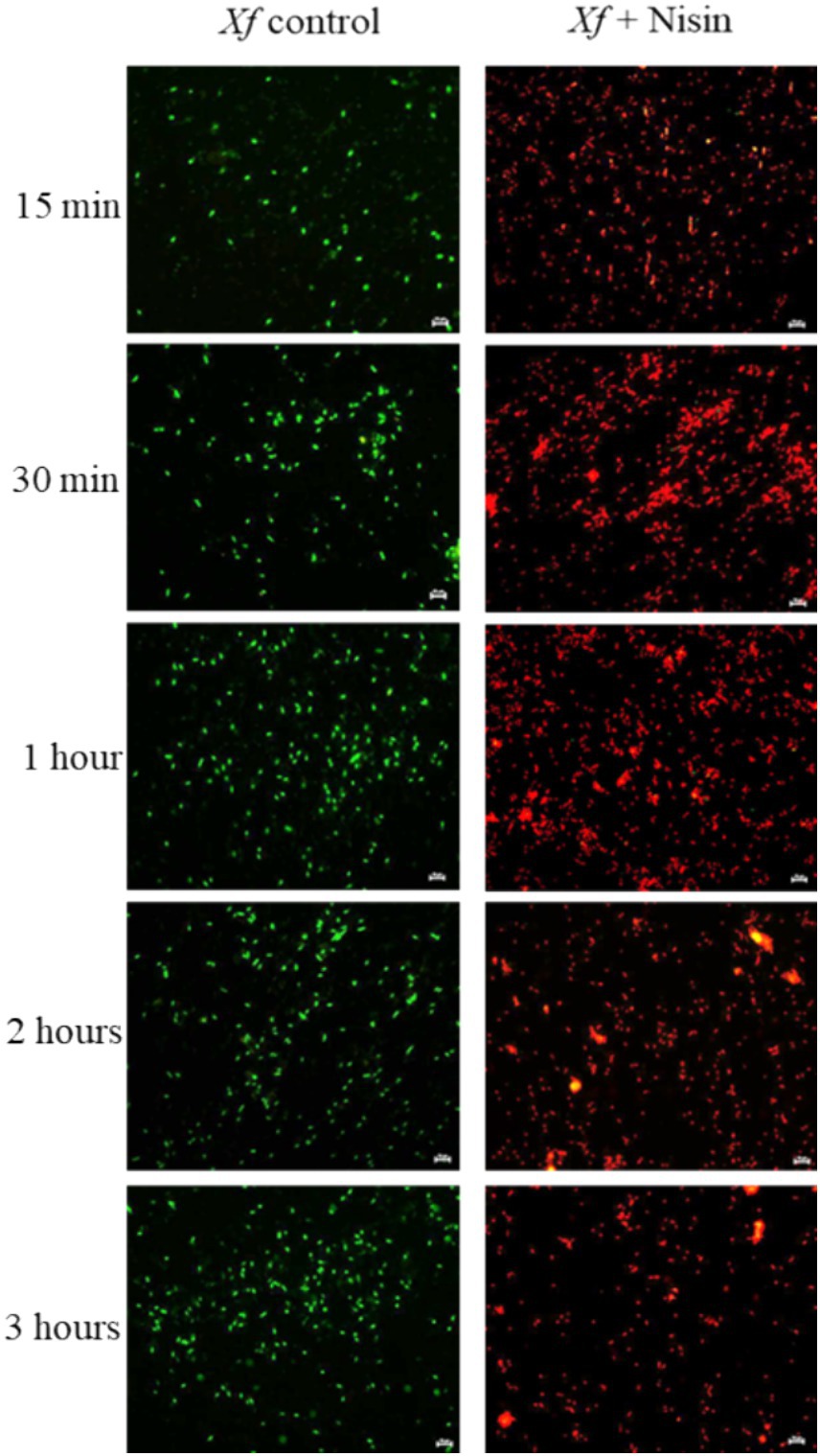
Figure 7. Fluorescent micrographs showing nisin-treated Xylella fastidiosa cells at 0.6 mg/mL. Green and red fluorescence represent viable and dead cells, respectively. Bar: 10 μm.
3.5 Effect of nisin on structural integrity of Xylella fastidiosa
Analyzing the impact of nisin on Xf cells using TEM, micrographs disclosed remarkable alterations at the structural level, leading to fragmentation of the cell wall membrane (Figure 8G). Specifically, the nisin-treated Xf cells presented cytoplasmic condensation, fragmented cell walls, disrupted internal morphology, and alterations in the outer membrane structure; all resulting in destruction of both inner and outer bacterial membranes (Figure 8). Moreover, membranes of Xf-nisin treated cells showed serial pores and protrusions, accompanied by condensed cytoplasmic outflow from the outer membrane in the form of vesicles (Figure 8H).
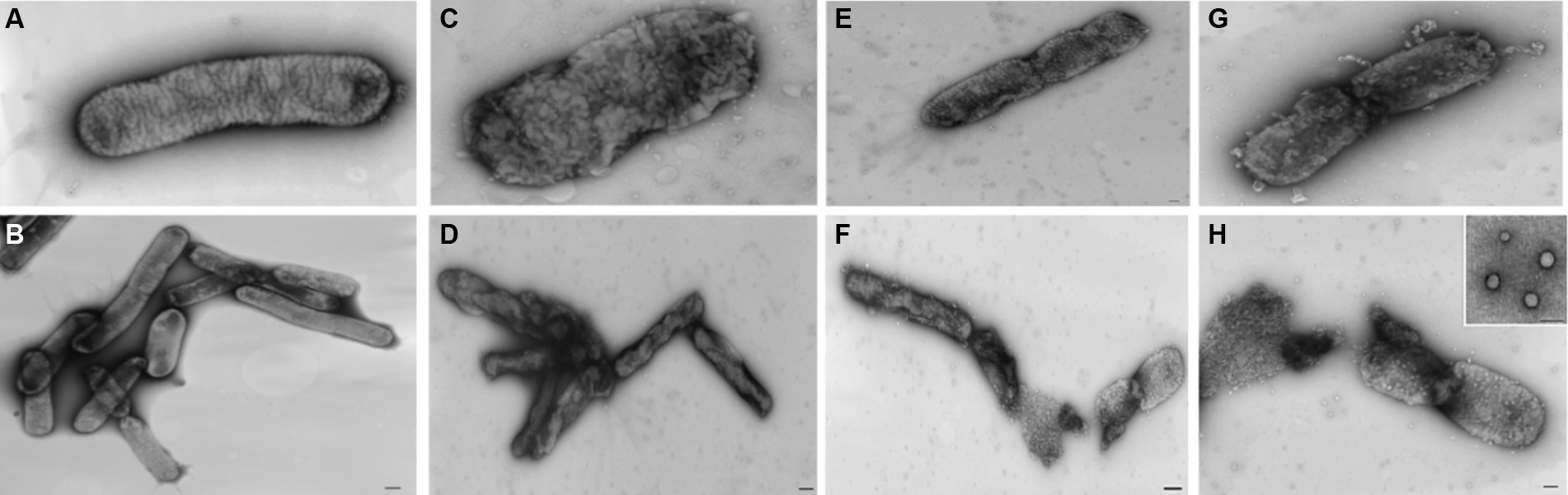
Figure 8. Transmission electron micrographs showing the impact of nisin on Xf cells. (A,B) Untreated Xf cells, used as control. (C–H) Nisin-treated Xf cells showing structural, cell wall, and cytoplasm alterations. Bar: 100 nm.
3.6 Spectrum of action
For a sustainable use of nisin in an integrated pest management strategy, the antibacterial activity of nisin was tested against four Xf antagonistic bacteria (Table 1). The results showed that nisin had no antibacterial effect on them, suggesting its potential use, in combination with these antagonistic bacteria as part of integrated biocontrol approach against Xf. Similarly, nisin did not showed any effect on the 16 phytopathogenic bacteria tested, except for strains of Xf subsp. pauca (Table 1). This emphasizes the specific antibacterial activity of nisin at its MLC against Xf subsp. pauca among the tested bacteria.
3.7 Nisin effectiveness in controlling Xylella fastidiosa in planta
The antibacterial effect of nisin against Xf in plant was evaluated in N. benthamiana plants treated with nisin before Xf infection (preventive) and after Xf infection (curative). Results of TaqMan-based qPCR performed 20 dpi on leaves collected above the inoculation sites, showed that Xf translocated only within the nisin-untreated infected plants (positive controls) (data not shown). By 40 dpi, the positive controls displayed typical Xf symptoms, i.e., leaf scorch (Figure 9B). In contrast, infected plants treated preventively and curatively with nisin demonstrated no typical symptoms of Xf (Figures 9C,D), indicating that nisin effectively countered the pathogen and prevented its impact on tobacco plants. At the end of the experiment (74 dpi), leaves with suspicious symptoms were analyzed for the presence of Xf using TaqMan-based qPCR assays, which showed the absence of the bacterium in all treatments; except leaves of positive controls (Figure 10).
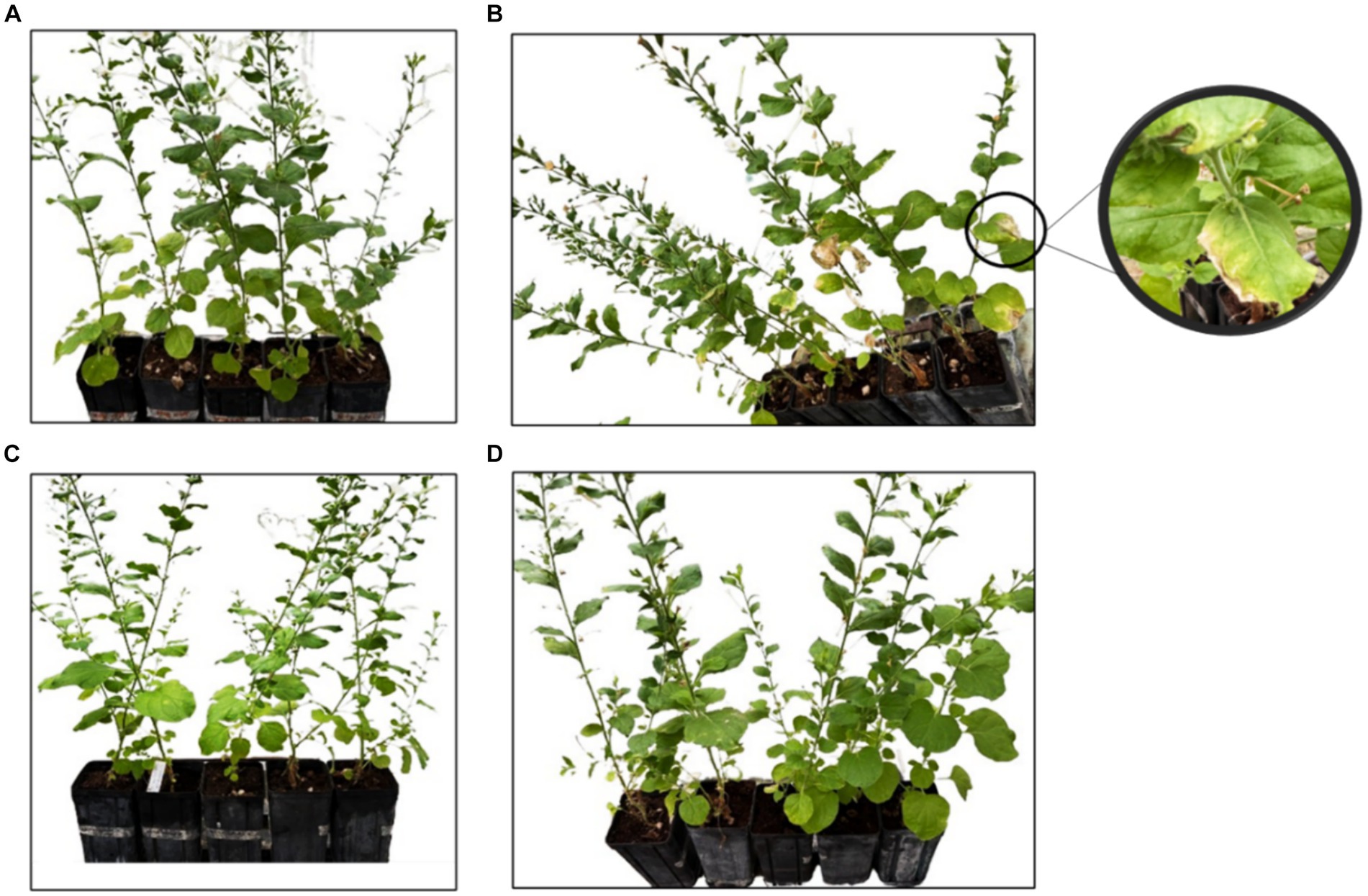
Figure 9. In planta assay showing the effect of nisin on Xylella fastidiosa subsp. pauca in Nicotiana benthamiana plants 74 dpi. (A) Healthy plants with no symptoms; (B) Xf-infected plants showing leaf scorch symptoms; (C) preventive nisin-treated Xf-infected plants with no symptoms; (D) curative nisin-treated Xf-infected plants with no symptoms.
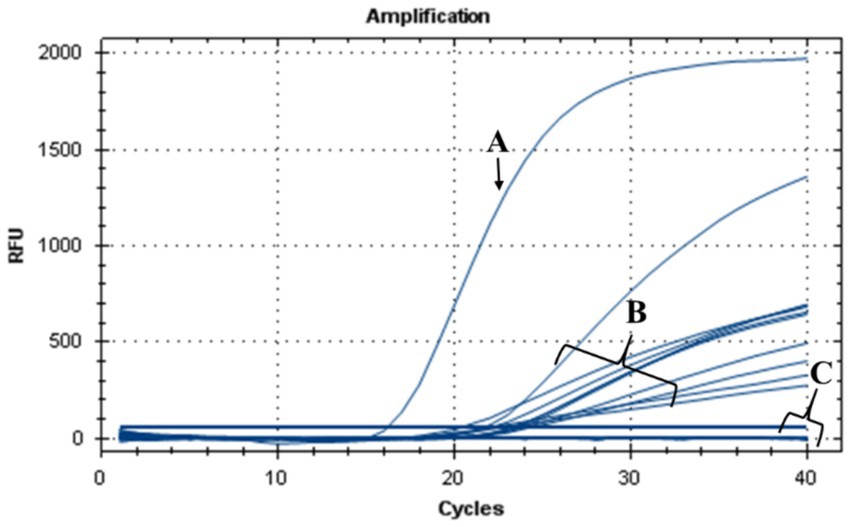
Figure 10. TaqMan-based qPCR assay showing DNA amplification curves obtained from (A) genomic DNA of Xf used as positive control, (B) genomic DNA extracted from leaves of nisin-untreated Xf-infected Nicotiana benthamiana plants (positive controls), (C) genomic DNA extracted from leaves of Xf-infected Nicotiana benthamiana plants treated preventively and curatively with nisin and from healthy plants used as a negative control.
3.8 In planta nisin detection using RPLC-ESI-MS/MS
To assess the stability and the translocation of nisin within N. benthamiana, RPLC-ESI-MS/MS analyses were carried out on sap recovered from different plant sections of three plants, as described above. Typically, low collisional energy and selected reaction monitoring (SRM) acquisition mode are employed in proteomic studies to enhance sensitivity and were thus also applied in the present case. When multi-charged molecules are analyzed, the transitions to a higher m/z ratio are considered highly. Therefore, the two most intense fragment ions with a higher m/z ratio than the precursor ions were chosen to develop the SRM method for nisin A. The highly specific transitions from the precursor ions [M + 4H]4+ and [M + 3H]3+ to higher m/z ratios were identified; the most intense one 839.3 → 1080.7 was used as quantifier while the other 1118.7 → 1451.7 was used as a confirmatory transition. As shown in Figure 11, a peak in the chromatogram appeared at a retention time of 7.8 min both in the standard spiked solution (A), and in a sample derived from stem (section 4) after 24 h (B). We also found that the tandem mass spectrum obtained in the stem of section 4 after 24 h (Figure 11C) had product ions identical to literature (Lavanant et al., 1998) and to those retrieved for standard solution (data not shown).
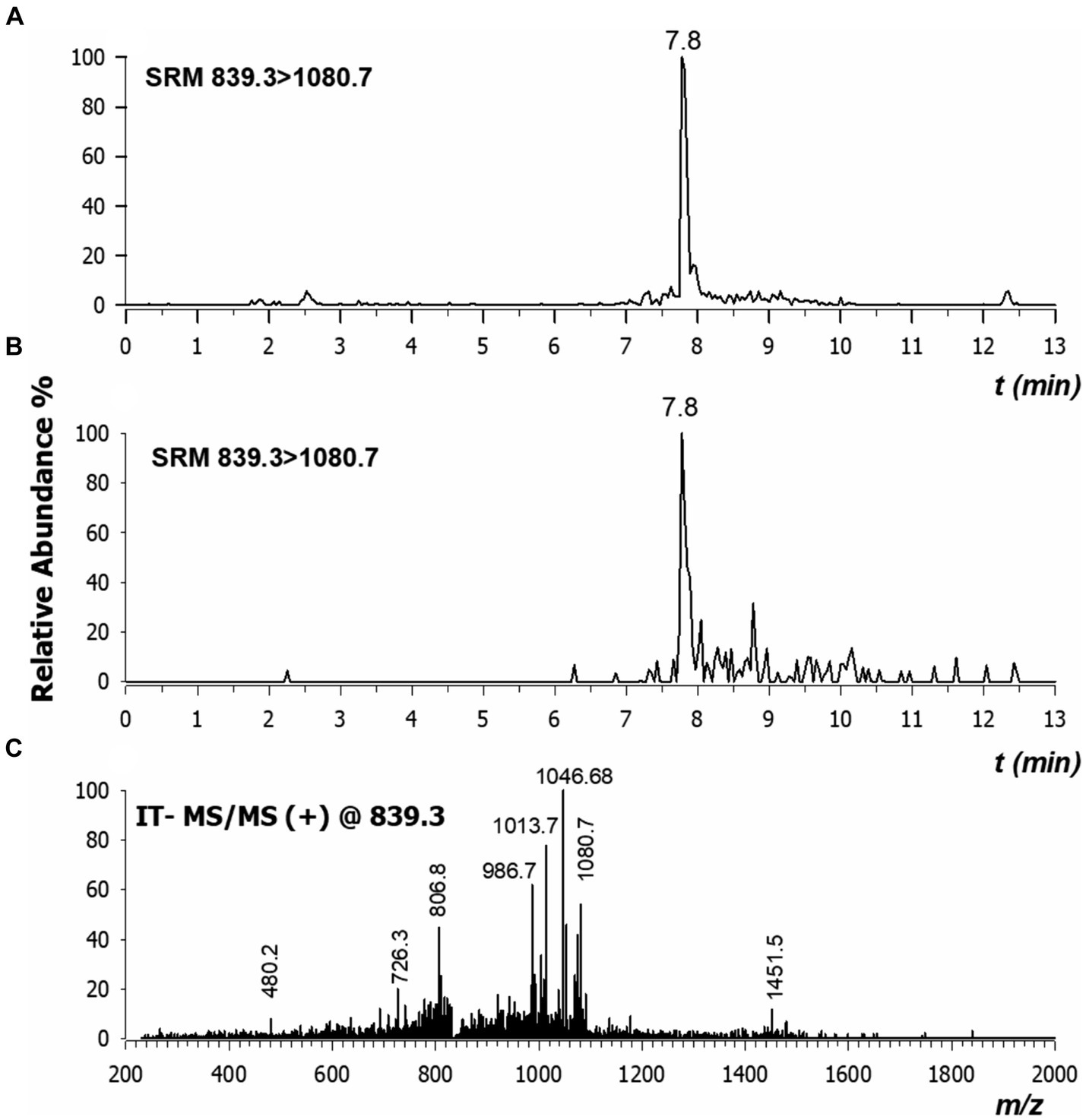
Figure 11. Chromatograms by RPLC-ESI-MS/MS in selected reaction monitoring (SRM) of nisin A in plant sap spiked at 30 ng/mL concentration (A) and in the stem of section 4 extracted after 24 h (B). Major product ions detected in CID-MS/MS spectrum of tetra-charged ion at m/z 839.3 (C). Values here reported are the means of three replicates.
We were able to qualitatively detect nisin A in stems, roots, and leaves; but to quantify the residual intact of nisin, we constructed an appropriate calibration curve spiking nisin A in blank plant sap. The linearity of the method was assessed at concentrations ranging from 0.06 to 60 ng/mL (5 levels) with a correlation coefficient of 0.9983 (Figure 12). The estimated LOD and LOQ values for nisin A were 2.1 and 7.1 ng/mL, respectively. Most of the values were below the LOD, some residual values above the LOD (2–4 ng/mL) were recovered in leaves (sections 3, 4 and 5) up to 9 days while the highest reliable concentration was found after 24 h in stem just above the injection point (section 4, 23.1 ng/mL). This experiment provides preliminary results on the stability of nisin in the plant, which can remain intact for up to 9 days after application, the period studied in our experiment.
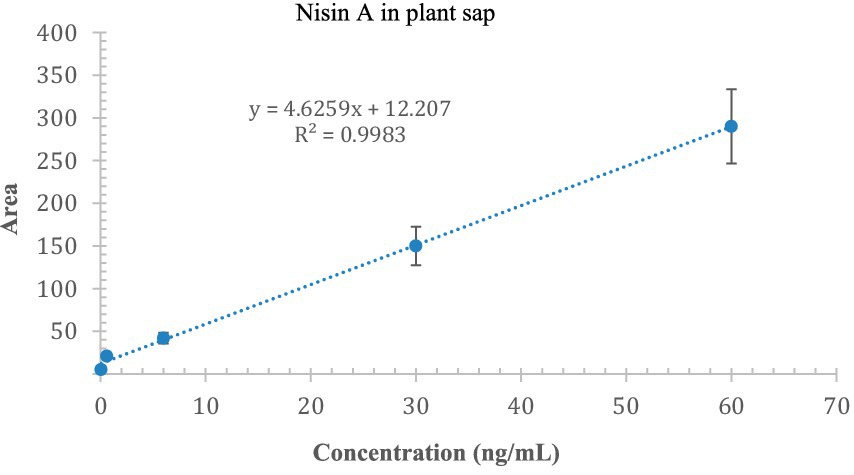
Figure 12. Calibration curve of nisin A in plant sap; the x-axis indicates the concentration of nisin, and the y-axis indicates the area of the SRM chromatogram.
4 Discussion
The emergence of Xf poses significant challenges to the agricultural sector worldwide, affecting economically significant crops and resulting in economic losses, changes in farming practices, and potential enduring damage to affected industries (Occhibove et al., 2020). To address the pressing need for effective solutions to combat Xf infections in many hosts, this study considered the use of lactic acid bacteria (LAB), which are recognized for their safety for human and animal consumption by the Food and Drug Administration (Ayivi et al., 2020), versatility across food, agriculture, and medicine. In particular, the use of nisin, a natural antimicrobial peptide (AMP) secreted by L. lactis subsp. lactis, to address Xf infections specifically highlights the potential of LAB in sustainable and environmentally friendly control strategies (Jaffar et al., 2023).
Our research has delimited the MLC of nisin, which at 0.6 mg/mL showed conspicuous antimicrobial potency across all in vitro assays, including spot assay, turbidity reduction assay, v-qPCR, FM, and TEM. Most importantly, FM and TEM results showed that nisin promptly reacts with Xf within the first 15 min of contact, leading to an immediate and complete lysis of the cellular membrane and with high potency. This observed effect aligns with the well-documented attributes of nisin, characterized by cationic and hydrophobic peptides (Musiejuk and Kafarski, 2023). The rapid and robust impact of nisin on Xf cells over a brief timeframe is most probably due to the high binding affinity of nisin to lipid II, disrupting the lipid bilayer. This causes the formation of pores, thereby enhancing membrane permeability and subsequent leakage of cellular contents, ultimately impeding bacterial growth (Meade et al., 2020). This action is most likely accentuated by the nature of Xf in forming biofilm-like aggregates, for which the transition from the planktonic to the biofilm-associated state involves profound physiological changes including changes in the protein composition of the bacterial cell envelope. Indeed, changes in outer membrane protein composition are known to have a profound effect on the sensitivity of Gram-negative bacteria to detergents, antibiotics, and bacteriophages (Nikaido, 1996). However, the composition and structure of the outer membrane of Xf is still not entirely understood; and more studies are needed to depict the mechanism of nisin in affecting the Xf cells.
To extend the knowledge on nisin’s antibacterial activity and its stability in a complex environmental context, serial experiments were performed in Xf-host plant model, i.e., N. benthamiana. The results consistently showed that nisin was able to prevent the development of Xf symptoms over a period of 74 days and to exert antibacterial activity despite the presence of plant compounds capable of degrading nisin, i.e., proteases. Indeed, nisin remained intact within the plant up to 9 days after injection, justifying the nisin efficacy in planta against Xf in both preventive and curative applications. This attribute, coupled with its strong and rapid antibacterial activity, its selectivity for Xf cells, as well as its effortless translocation, makes it an indispensable option in tackling the Xf infections.
Top of form being a naturally occurring antimicrobial peptide, nisin has several advantages over synthetic antimicrobial peptides, which may be produced at a considerable expense and require certain conditions for synthesis and purification. It is produced by L. lactis in a straightforward laboratory setting involving common media and incubation conditions (Khelissa et al., 2021). In our laboratory conditions, nisin was secreted by L. lactis subsp. lactis strain ATCC 11454 at a concentration of 345 ng/mL in YPG liquid medium after 24 h of incubation. This strain is also reported in the literature to produce 0.2 g/L of nisin in 25% milk and 25% MRS medium (Penna et al., 2005). However, the presence of the nisin in the inhibition zone was not discernable, most likely due to the difficulty of tracking nisin on solid media, contrarily to the liquid culture media. The ease of producing large quantities of nisin in the laboratory and at low cost makes this compound a sustainable approach for agricultural application in the field. Unlike in the medical and food preservation domains, substantial nisin purification which might lead to increased costs, is not required in agriculture. On a practical level, the application of nisin in the field can be done by trunk injection methods; as in our case Xf is a xylem-limited pathogen difficult to reach, the spraying method is ineffective and inapplicable. Indeed, the trunk injection approach has been used to control fire blight bacterial disease of apple trees and huanglongbing of citrus caused by the systemic pathogen Candidatus liberibacter Garnier (Aćimović et al., 2014; Puttamuk et al., 2014). Furthermore, the same bacteria that produce nisin are also capable of creating a complex of substances that might support soil enrichment and plant development, in addition to its antibacterial qualities (Naskar and Kim, 2021; Raman et al., 2022). This dual functionality could present an integrated strategy for both treating and fortifying plants in their supposed susceptibility to Xf.
In conclusion, we are confident that nisin represents a noteworthy sustainable and practical tool to treat challenging Xf subsp. pauca infections in plants. This aligns with the principles of greener agriculture, in accordance with European Union regulations on quarantine pests, particularly for Xf. Indeed, bacteriocin-based biobactericides are gaining more attention for their compatibility with eco-friendly and sustainable agricultural practices. They present a realistic approach for controlling plant pathogenic bacteria, replacing, or complementing the existing agrochemicals within comprehensive control programs. Lastly, further studies will be important to elucidate the ability of nisin and nisin-producing bacteria to enhance plant resistance to Xf subsp. pauca and to promote better growth and development of Xf subsp. pauca host plants in agricultural production. Further research is needed to explore nisin potential applications in field conditions against different Xf-host plant species.
Data availability statement
The raw data supporting the conclusions of this article will be made available by the authors, without undue reservation.
Author contributions
MS: Conceptualization, Data curation, Formal analysis, Investigation, Methodology, Software, Validation, Visualization, Writing – original draft, Writing – review & editing. KH: Conceptualization, Data curation, Formal analysis, Investigation, Methodology, Software, Validation, Visualization, Writing – original draft, Writing – review & editing. FV: Data curation, Investigation, Validation, Visualization, Writing – review & editing. AS: Data curation, Investigation, Validation, Visualization, Writing – review & editing, Formal analysis, Methodology, Software. OC: Data curation, Formal analysis, Investigation, Software, Visualization, Writing – review & editing. CC: Data curation, Formal analysis, Investigation, Visualization, Writing – review & editing, Methodology, Validation. MB: Formal analysis, Visualization, Writing – review & editing, Software. AT: Formal analysis, Software, Visualization, Writing – review & editing. TE: Formal analysis, Software, Visualization, Writing – review & editing, Conceptualization, Data curation, Funding acquisition, Investigation, Methodology, Project administration, Resources, Supervision, Validation, Writing – original draft.
Funding
The author(s) declare that financial support was received for the research, authorship, and/or publication of this article. This research was financially supported by the Italian Ministry of Agriculture, Food Sovereignty and Forestry (MASAF), in the frame of project “Approcci Nanotecnologici per un Controllo Sostenibile e Innovativo di Xylella fastidiosa—“ANCOSIX” CUP n. J83C22001990005.
Conflict of interest
The authors declare that the research was conducted in the absence of any commercial or financial relationships that could be construed as a potential conflict of interest.
Publisher’s note
All claims expressed in this article are solely those of the authors and do not necessarily represent those of their affiliated organizations, or those of the publisher, the editors and the reviewers. Any product that may be evaluated in this article, or claim that may be made by its manufacturer, is not guaranteed or endorsed by the publisher.
References
Aćimović, S. G., VanWoerkom, A. H., Reeb, P. D., Vandervoort, C., Garavaglia, T., Cregg, B. M., et al. (2014). Spatial and temporal distribution of trunk-injected imidacloprid in apple tree canopies. Pest Manag. Sci. 70, 1751–1760. doi: 10.1002/ps.3747
Ali, B. M., van der Werf, W., and Oude Lansink, A. (2021). Assessment of the environmental impacts of Xylella fastidiosa subsp. pauca in Puglia. Crop Prot. 142:105519. doi: 10.1016/j.cropro.2020.105519
Ayivi, R. D., Gyawali, R., Krastanov, A., Aljaloud, S. O., Worku, M., Tahergorabi, R., et al. (2020). Lactic acid bacteria: food safety and human health applications. Dairy 1, 202–232. doi: 10.3390/dairy1030015
Barbosa, A. A. T., de Melo, M. R., da Silva, C. M. R., Jain, S., and Dolabella, S. S. (2021). Nisin resistance in Gram-positive bacteria and approaches to circumvent resistance for successful therapeutic use. Crit. Rev. Microbiol. 47, 376–385. doi: 10.1080/1040841X.2021.1893264
Baró, A., Badosa, E., Montesinos, L., Feliu, L., Planas, M., Montesinos, E., et al. (2020). Screening and identification of BP100 peptide conjugates active against Xylella fastidiosa using a viability-qPCR method. BMC Microbiol. 20, 1–14. doi: 10.1186/s12866-020-01915-3
Baró, A., Saldarelli, P., Saponari, M., Montesinos, E., and Montesinos, L. (2022). Nicotiana benthamiana as a model plant host for Xylella fastidiosa: control of infections by transient expression and endotherapy with a bifunctional peptide. Front. Plant Sci. 13:1061463. doi: 10.3389/fpls.2022.1061463
Burbank, L. P., and Ortega, B. C. (2018). Novel amplification targets for rapid detection and differentiation of Xylella fastidiosa subspecies fastidiosa and multiplex in plant and insect tissues. J. Microbiol. Methods 155, 8–18. doi: 10.1016/j.mimet.2018.11.002
Davis, M. J., Thomson, S. V., and Purcell, A. H. (1980). Etiological role of a xylem-limited bacterium causing Pierce’s disease in almond leaf scorch. Phytopathology 70:472. doi: 10.1094/Phyto-70-472
El Handi, K., Sabri, M., Valentini, F., De Stradis, A., Achbani, E. H., Hafidi, M., et al. (2022). Exploring active peptides with antimicrobial activity in planta against Xylella fastidiosa. Biology 11:1685. doi: 10.3390/biology11111685
Field, D., Fernandez de Ullivarri, M., Ross, R. P., and Hill, C. (2023). After a century of nisin research—where are we now? FEMS Microbiol. Rev. 47:fuad023. doi: 10.1093/femsre/fuad023
Ibarra-Sánchez, L. A., El-Haddad, N., Mahmoud, D., Miller, M. J., and Karam, L. (2020). Invited review: Advances in nisin use for preservation of dairy products. J. Dairy Sci. 103, 2041–2052. doi: 10.3168/jds.2019-17498
Jaffar, N. S., Jawan, R., and Chong, K. P. (2023). The potential of lactic acid bacteria in mediating the control of plant diseases and plant growth stimulation in crop production—a mini review. Front. Plant Sci. 13:1047945. doi: 10.3389/fpls.2022.1047945
Khelissa, S., Chihib, N.-E., and Gharsallaoui, A. (2021). Conditions of nisin production by Lactococcus lactis subsp. lactis and its main uses as a food preservative. Arch. Microbiol. 203, 465–480. doi: 10.1007/s00203-020-02054-z
Lavanant, H., Derrick, P. J., Heck, A. J. R., and Mellon, F. A. (1998). Analysis of nisin A and some of its variants using fourier transform ion cyclotron resonance mass spectrometry. Anal. Biochem. 255, 74–89.
Li, Q., Montalban-Lopez, M., and Kuipers, O. P. (2018). Increasing the antimicrobial activity of nisin based lantibiotics against Gram-negative pathogens. Appl. Environ. Microbiol. 84:e00052. doi: 10.1128/AEM.00052-18
Małaczewska, J., and Kaczorek-Łukowska, E. (2021). Nisin a lantibiotic with immunomodulatory properties: a review. Peptides 137:170479. doi: 10.1016/j.peptides.2020.170479
Meade, E., Slattery, M. A., and Garvey, M. (2020). Bacteriocins, potent antimicrobial peptides and the fight against multi drug resistant species: resistance is futile? Antibiotics 9:32. doi: 10.3390/antibiotics9010032
Mourou, M., Hanani, A., D’onghia, A. M., Davino, S. W., Balestra, G. M., and Valentini, F. (2022). Antagonism and antimicrobial capacity of epiphytic and endophytic bacteria against the phytopathogen Xylella fastidiosa. Agronomy 12:1266. doi: 10.3390/agronomy12061266
Musiejuk, M., and Kafarski, P. (2023). Engineering of nisin as a means for improvement of its pharmacological properties: a review. Pharmaceuticals 16:1058. doi: 10.3390/ph16081058
Naskar, A., and Kim, K. (2021). Potential novel food-related and biomedical applications of nanomaterials combined with bacteriocins. Pharmaceutics 13:86. doi: 10.3390/pharmaceutics13010086
Nikaido, H. (1996). “Outer Membrane” in Escherichia coli and Salmonella: cellular and molecular biology. eds. F. C. Neidhardt, J. Ingraham, E. C. C. Lin, K. B. Low, B. Magasanik, and W. Reznikoff, et al. (Washington, DC: American Society for Microbiology).
Occhibove, F., Chapman, D. S., Mastin, A. J., Parnell, S. S., Agstner, B., Mato-Amboage, R., et al. (2020). Eco-epidemiological uncertainties of emerging plant diseases: the challenge of predicting Xylella fastidiosa dynamics in novel environments. Phytopathology 110, 1740–1750. doi: 10.1094/PHYTO-03-20-0098-RVW
Penna, T. C. V., Jozala, A. F., De Lencastre Novaes, L. C., Pessoa, A., and Cholewa, O. (2005). Production of nisin by Lactococcus lactis in media with skimmed milk. Appl. Biochem. Biotechnol. 122, 619–637. doi: 10.1385/ABAB:122:1-3:0619
Puttamuk, T., Zhang, S., Duan, Y., Jantasorn, A., and Thaveechai, N. (2014). Effect of chemical treatments on ‘Candidatus Liberibacter asiaticus’ infected pomelo (Citrus maxima). Crop Prot. 65, 114–121. doi: 10.1016/j.cropro.2014.07.018
Raman, J., Kim, J.-S., Choi, K. R., Eun, H., Yang, D., Ko, Y.-J., et al. (2022). Application of lactic acid bacteria (LAB) in sustainable agriculture: advantages and limitations. Int. J. Mol. Sci. 23:7784. doi: 10.3390/ijms23147784
Scortichini, M., Loreti, S., Pucci, N., Scala, V., Tatulli, G., Verweire, D., et al. (2021). Progress towards sustainable control of Xylella fastidiosa subsp. pauca in olive groves of Salento (Apulia, Italy). Pathogens 10:668. doi: 10.3390/pathogens10060668
Simons, A., Alhanout, K., and Duval, R. E. (2020). Bacteriocins, antimicrobial peptides from bacterial origin: overview of their biology and their impact against multidrug-resistant bacteria. Microorganisms 8:639. doi: 10.3390/microorganisms8050639
Velasco-Amo, M. P., Arias-Giraldo, L. F., Román-Écija, M., Fuente, L. D. L., Marco-Noales, E., Moralejo, E., et al. (2023). Complete circularized genome resources of seven strains of Xylella fastidiosa subsp. fastidiosa Using hybrid assembly reveals unknown plasmids. Phytopathology 113, 1128–1132. doi: 10.1094/PHYTO-10-22-0396-A
Wells, J. M., Raju, B. C., Nyland, G., and Lowe, S. K. (1981). Medium for isolation and growth of bacteria associated with plum leaf scald and phony peach diseases. Appl. Environ. Microbiol. 42, 357–363. doi: 10.1128/aem.42.2.357-363.1981
Keywords: bacteria, antimicrobial peptide, v-qPCR, electron microscopy, RPLC-ESI-MS/MS, biocontrol
Citation: Sabri M, El Handi K, Valentini F, De Stradis A, Cara O, Calvano CD, Bianco M, Trani A and Elbeaino T (2024) Nisin-based therapy: a realistic and eco-friendly biocontrol strategy to contrast Xylella fastidiosa subsp. pauca infections in planta. Front. Microbiol. 15:1406672. doi: 10.3389/fmicb.2024.1406672
Edited by:
Jørgen J. Leisner, University of Copenhagen, DenmarkCopyright © 2024 Sabri, El Handi, Valentini, De Stradis, Cara, Calvano, Bianco, Trani and Elbeaino. This is an open-access article distributed under the terms of the Creative Commons Attribution License (CC BY). The use, distribution or reproduction in other forums is permitted, provided the original author(s) and the copyright owner(s) are credited and that the original publication in this journal is cited, in accordance with accepted academic practice. No use, distribution or reproduction is permitted which does not comply with these terms.
*Correspondence: Toufic Elbeaino, elbeaino@iamb.it
†These authors share first authorship