- 1Department of Chemistry “Ugo Schiff,” University of Florence, Florence, Italy
- 2Laboratory of Cell Physiology, Institute of Neuroscience, Université Catholique de Louvain, Louvain-la-Neuve, Belgium
The sarco(endo)plasmic reticulum Ca2+-ATPase (SERCA) is an intracellular membrane transporter that utilizes the free energy provided by ATP hydrolysis for active transport of Ca2+ ions from the cytoplasm to the lumen of sarco(endo)plasmic reticulum. SERCA plays a fundamental role for cell calcium homeostasis and signaling in muscle cells and also in cells of other tissues. Because of its prominent role in many physiological processes, SERCA dysfunction is associated to diseases displaying various degrees of severity. SERCA transport activity can be inhibited by a variety of compounds with different chemical structures. Specific SERCA inhibitors were identified which have been instrumental in studies of the SERCA catalytic and transport mechanism. It has been proposed that SERCA inhibition may represent a novel therapeutic strategy to cure certain diseases by targeting SERCA activity in pathogens, parasites and cancer cells. Recently, novel small molecules have been developed that are able to stimulate SERCA activity. Such SERCA activators may also offer an innovative and promising therapeutic approach to treat diseases, such as heart failure, diabetes and metabolic disorders. In the present review the effects of pharmacologically relevant compounds on SERCA transport activity are presented. In particular, we will discuss the interaction of SERCA with specific inhibitors and activators that are potential therapeutic agents for different diseases.
Introduction
P-type ATPases are membrane transporters that couple the energy provided by ATP hydrolysis to the active transport of various ions or phospholipids. These enzymes generate and maintain crucial electrochemical potential gradients across biological membranes (Kühlbrandt, 2004; Bublitz et al., 2011). During their enzymatic cycle P-type ATPases form a phosphorylated intermediate by interaction of ATP with a conserved aspartate residue at the catalytic domain.
The Ca2+-ATPase from sarco(endo)plasmic reticulum (SERCA), belonging to the PIIA-ATPase subfamily, is an intracellular membrane-associated protein of approximately 110 KDa which is involved in cell calcium signaling and homeostasis (Brini and Carafoli, 2009). In muscle cells this enzyme hydrolyzes one ATP molecule to transport two Ca2+ ions against their electrochemical potential gradient from the cytoplasm to the lumen of sarcoplasmic reticulum (SR) (Inesi and Tadini-Buoninsegni, 2014). SERCA therefore induces muscle relaxation by pumping back cytosolic calcium into the SR lumen. SERCA isoforms are involved in calcium signaling mechanisms for many biological functions, e.g., excitation-contraction coupling, excitation-secretion coupling, gene transcription, and apoptotic mechanisms. Because of its pivotal role, alterations in SERCA expression and impaired pump function have been related to several diseases, such as Brody's disease, Darier's disease, heart failure, cancer, and diabetes (Brini and Carafoli, 2009).
The SERCA enzyme is one of the best investigated membrane transporter. Its structure comprises three distinct cytoplasmic domains, i.e., the A (actuator), N (nucleotide binding), and P (phosphorylation) domains, and a transmembrane region of 10 helical segments (TM1–TM10) including the two Ca2+ binding sites. SERCA transport cycle is described by the E1-E2 scheme (de Meis and Vianna, 1979). If one starts at the E1 state, the ATPase cycle (Figure 1) begins with high affinity binding of two Ca2+ ions derived from the cytosol, followed by phosphorylation of the enzyme by ATP and formation of a high energy E1~P state. During relaxation from the E1~P state to the lower energy E2P state, Ca2+ ions are translocated across the membrane and released into the SR in exchange for luminal protons. Hydrolytic cleavage of the phosphoenzyme (dephosphorylation) is the final reaction step, which allows the enzyme to undergo a new transport cycle. High resolution crystal structures of various conformational states in the SERCA transport cycle were obtained, as described in detailed reviews (Toyoshima, 2008; Møller et al., 2010; Bublitz et al., 2013; Toyoshima and Cornelius, 2013).
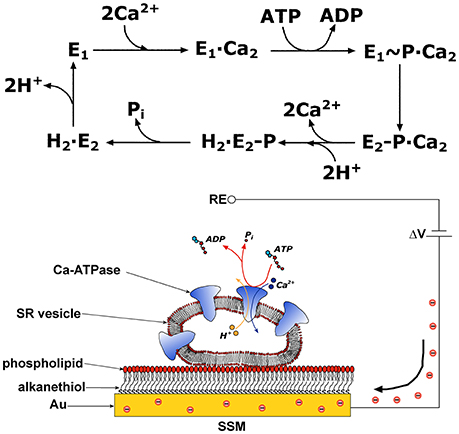
Figure 1. Upper Panel: Schematic diagram of sequential reactions in the transport cycle of SERCA. Lower Panel: SR vesicle adsorbed on a SSM and subjected to an ATP concentration jump (not drawn to scale). If the ATP jump induces net charge displacement, a compensating current flows along the external circuit (the red spheres represent electrons) to keep constant the potential difference ΔV applied across the whole system. RE is the reference electrode. Reprinted from Tadini-Buoninsegni et al. (2008a) with permission from Elsevier.
In this short review we will focus our attention on the interaction of SERCA with specific inhibitors and activators that may represent potential therapeutic agents for different diseases. To investigate the effects of pharmacologically relevant compounds on SERCA transport activity, we employ an electrophysiological technique, which is discussed in the next section.
Drug Interactions Investigated by Electrophysiology Based on Solid Supported Membranes
The ion transport mechanism of P-type ATPases, e.g., Na+,K+-ATPase, SERCA, and Cu+-ATPases (ATP7A and ATP7B) (Pintschovius et al., 1999; Tadini-Buoninsegni et al., 2008a; Lewis et al., 2012; Inesi et al., 2014; Tadini-Buoninsegni and Smeazzetto, 2017), was investigated by an electrophysiological technique based on a solid supported membrane (SSM). In particular, SSM-based electrophysiology was useful to identify electrogenic steps and to assign rate constants to partial reactions in the transport cycle of P-type ATPases. In the case of Na+,K+-ATPase SSM-based electrophysiology provided a direct proof for the electrogenicity of Na+ binding to the cytoplasmic side of the protein (Pintschovius et al., 1999). Also in the case of SERCA SSM-based electrophysiology was employed for a detailed characterization of the enzyme's transport cycle, especially as concerns Ca2+ binding and Ca2+/H+ exchange (Tadini-Buoninsegni et al., 2006; Liu et al., 2009).
This technique makes use of a hybrid alkanethiol/phospholipid bilayer supported by a gold electrode (SSM, Figure 1; Pintschovius and Fendler, 1999). The SSM is formed in two sequential self-assembly steps. First, an octadecanethiol monolayer is obtained which is covalently bound to the gold electrode via the sulfur atom. Then, a second phosphatidylcholine monolayer is formed on top of the thiol layer. Proteoliposomes, membrane fragments, or vesicles containing the ATPase are adsorbed on the SSM surface (Figure 1). Once adsorbed, the ATPase molecules are activated by a concentration jump of a specific substrate through fast solution exchange. By rapidly changing from a solution containing no substrate for the protein to one that contains a substrate, the protein is activated and a current transient is detected, which is related to charge displacement across the ATPase. The transient nature of the current signal is a consequence of the capacitively coupled system formed by the SSM and the membrane entities adsorbed on it (Schulz et al., 2008; Tadini-Buoninsegni and Bartolommei, 2016). In the case of SERCA, an ATP concentration jump on SERCA-containing vesicles adsorbed on the SSM generates a current signal, that is related to an electrogenic event corresponding to translocation and release of bound Ca2+ upon phosphorylation by ATP within the first enzyme cycle (Tadini-Buoninsegni et al., 2006). Therefore, the SSM technique allows pre-steady state measurements of charge displacements within the first transport cycle of the ATPase, while steady-state currents are not measured.
SSM-based electrophysiology was successfully employed to investigate drug interactions with P-type ATPases. In this respect, the effects of various compounds of pharmacological interest on SERCA pumping activity were characterized by SSM-based current measurements (Tadini-Buoninsegni et al., 2008b, 2017; Bartolommei et al., 2011; Ferrandi et al., 2013; Sadafi et al., 2014). A molecular mechanism was proposed to explain the effect of each compound, and the reaction step and/or intermediate of the pump cycle affected by the drug was identified.
We point out that the SSM electrode combined with robotized instrumentation is an attractive tool for drug screening and development (Kelety et al., 2006). In this respect, high-throughput devices capable of performing automated measurements have been developed. For example, the SURFE2R 96SE device (Nanion Technologies, Munich, Germany) is able to analyze 96 SSM sensors in a fully parallel mode allowing determination of the dose dependence of 100 compounds in <30 min (Bazzone et al., 2017).
Pharmacological Inhibitors of SERCA Activity
Various SERCA inhibitors with a variety of chemical structures are known (Michelangeli and East, 2011). These compounds represent a very useful tool in studies of the SERCA catalytic and transport mechanism. Using X-ray crystallography of SERCA-inhibitor complexes (Toyoshima and Nomura, 2002; Olesen et al., 2004; Obara et al., 2005; Moncoq et al., 2007; Laursen et al., 2009), distinct SERCA conformational states were determined at atomic resolution.
A very potent and highly selective inhibitor is thapsigargin (TG), a sesquiterpene lactone derived from the plant Thapsia garganica (Rasmussen et al., 1978). TG is the most widely employed SERCA inhibitor (Michelangeli and East, 2011) and can inhibit SERCA activity with an IC50 in the sub-nanomolar range (Sagara and Inesi, 1991). Other specific SERCA inhibitors are cyclopiazonic acid (CPA) (Seidler et al., 1989), a secondary metabolite from certain fungi, and the synthetic compound 2,5-di(tert-butyl)hydroquinone (DBHQ) (Moore et al., 1987). Mutational analysis and crystallographic data have shown that CPA and DBHQ occupy the same binding pocket at the cytoplasmic ends of the transmembrane helices TM1–TM4, while TG binds in a groove delimited by TM3, TM5, and TM7 (for a review see Yatime et al., 2009), where the residue Phe256 plays a fundamental role for both binding and inhibitory effect of TG (Xu et al., 2004). TG, CPA, and DBHQ (Table 1) affect SERCA transport activity in a similar way. These inhibitors bind to SERCA in a calcium-free E2 conformation and stabilize a compact ATPase conformational state (dead-end state), preventing cytoplasmic calcium binding and catalytic activation (Inesi et al., 2005; Yatime et al., 2009; Michelangeli and East, 2011).
The inhibitory effects of TG, CPA, DBHQ, and1,3-dibromo-2,4,6-tris (methyl-isothio-uronium) benzene (Br2-TITU), another SERCA inhibitor (Berman and Karlish, 2003), on Ca2+-ATPase transport activity were also characterized by electrophysiological measurements on a SSM (Tadini-Buoninsegni et al., 2008b). In this study it was shown that Br2-TITU displays an inhibitory mechanism different from that of TG, CPA, and DBHQ. In particular, it was demonstrated that the inhibitory effect of Br2-TITU is related to kinetic interference with a conformational transition of the phosphorylated intermediate (E1P-Ca2 to E2P transition).
It is noteworthy that TG-related prodrugs are being evaluated as anticancer drugs. Since TG will inhibit SERCA proteins regardless of the cell type thereby damaging intracellular calcium homeostasis not only in cancer cells but also in normal cells, its high cytotoxicity prevents direct use of TG as a general antitumor agent. However, a TG-based prodrug strategy was developed to overcome the above-mentioned limitation (Denmeade et al., 2012; Andersen et al., 2015; Doan et al., 2015; Cui et al., 2017). In particular, a prodrug, named mipsagargin, was obtained by conjugating a TG analog to a peptide that is targeted by prostate-specific membrane antigen (PSMA) (Denmeade et al., 2012; Andersen et al., 2015; Doan et al., 2015), which is overexpressed in prostate cancer cells and most tumor endothelial cells. This inactive and non-toxic prodrug becomes activated once it reaches tumor cells and the specific peptide sequence is cleaved by PSMA, thereby releasing the active cytotoxic TG analog. The prodrug mipsagargin can therefore be considered as a potential therapeutic agent for the treatment of various types of cancer, including prostate, breast and bladder cancers.
In the context of antitumor agents, SSM-based electrophysiology was employed to analyze the interaction of metal-based anticancer drugs with P-type ATPases, i.e., SERCA, Na+,K+-ATPase and Cu+-ATPases (Sadafi et al., 2014; Tadini-Buoninsegni et al., 2014, 2017). In particular, the inhibitory effect of cisplatin (Table 1) on the transport activity of SERCA and Na+,K+-ATPase was very recently investigated (Figure 2) (Tadini-Buoninsegni et al., 2017). Cisplatin is a platinum-containing anticancer drug, which is widely employed as a chemotherapeutic agent against several tumors (Wang and Lippard, 2005). However, cisplatin administration causes inevitable adverse effects, which include nephrotoxicity, ototoxicity and neurotoxicity. We have shown that cisplatin is able to inhibit ATP-dependent cation translocation by SERCA and Na+,K+-ATPase with different degrees of potency (Figure 2). In particular, cisplatin was found to be a much stronger inhibitor of SERCA (IC50 of 1.3 μM) than of Na+,K+-ATPase (IC50 of 11.1 μM). We propose that cisplatin inhibition of SERCA and Na+,K+-ATPase activities may be relevant to the molecular mechanisms that underlie the various adverse effects of cisplatin and other platinum-containing anticancer drugs.
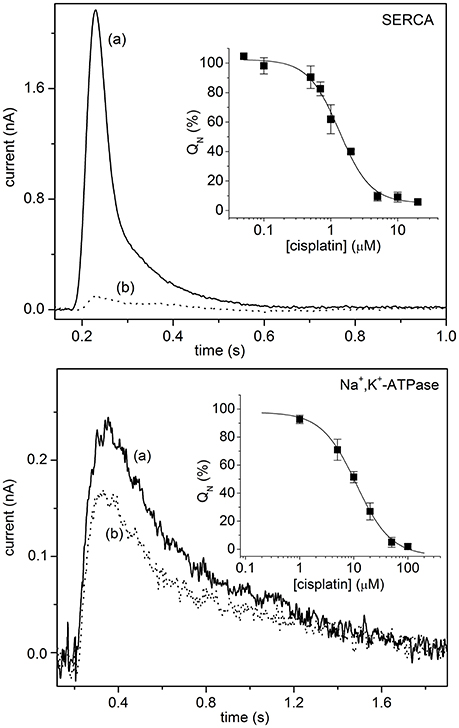
Figure 2. Upper Panel: SERCA current signals induced by 100 μM ATP concentration jumps in the presence of 10 μM free Ca2+ and in the absence (control measurement, solid line, a) or in the presence of 5 μM cisplatin (dotted line, b). (Inset) Normalized charges (QN) related to ATP concentration jumps in the presence of Ca2+ ions as a function of cisplatin concentration. The charges are normalized with reference to the maximum charge attained in the absence of cisplatin (control measurement). The solid line represents the fitting curve to the ATP-induced charges (IC50 = 1.3 ± 0.1 μM). The error bars represent S.E. of three independent measurements. Lower Panel: Na+,K+-ATPase current signals induced by 100 μM ATP concentration jumps in the presence of 80 mM NaCl and 50 mM KCl, and in the absence (control measurement, solid line, a) or in the presence of 5 μM cisplatin (dotted line, b). (Inset) Normalized charges (QN) related to ATP concentration jumps in the presence of Na+ and K+ ions as a function of cisplatin concentration. The charges are normalized with respect to the maximum charge measured in the absence of cisplatin (control measurement). The solid line represents the fitting curve to the ATP-induced charges (IC50 = 11.1 ± 0.8 μM). The error bars represent S.E. of three independent measurements. Tadini-Buoninsegni et al. (2017)—Reproduced by permission of The Royal Society of Chemistry.
As concerns other specific inhibitors of SERCA activity, CPA has been proposed to have therapeutic properties. In a study of the isolated rabbit heart CPA was found to have a cardioprotective effect on myocardial ischemia (Avellanal et al., 1998). The mechanism involved in CPA cardioprotection is not fully understood and could be partly attributed to a decreased SR calcium contribution to the calcium overload induced by ischemia.
It is noteworthy that CPA has been found to be a potent inhibitor of the SERCA ortholog PfATP6 of Plasmodium falciparum (Cardi et al., 2010; Arnou et al., 2011), the protozoan parasite causing malaria which is responsible for most malaria-related deaths globally. Considering that the parasite P. falciparum is becoming increasingly resistant to some of the most commonly used antimalarial drugs, PfATP6 has been validated as a potential and promising target for the development of new and effective antimalarials. PfATP6 and mammalian SERCA are characterized by a different pharmacological profile: compared to rabbit SERCA1a isoform from the skeletal muscle, PfATP6 is less sensitive to TG and DBHQ and exhibits a much higher affinity for CPA (Cardi et al., 2010; Arnou et al., 2011). Moreover, it was shown that PfATP6 is not inhibited by the widely employed antimalarial drug artemisinin (Cardi et al., 2010; Arnou et al., 2011), which was proposed to target PfATP6 (Eckstein-Ludwig et al., 2003). In a recent study, the interaction of CPA with PfATP6 and SERCA was characterized by molecular dynamics simulations (Di Marino et al., 2015). This study points to significant differences in the mode of CPA binding to the plasmodial and mammalian SERCA. These are useful information that can assist in the design and development of CPA derivatives, which are selective toward PfATP6 and with a reduced activity against mammalian SERCA. Besides PfATP6, the P. falciparum protein PfATP4, a P-type Na+-ATPase in the plasma membrane of the parasite, has emerged as a potential antimalarial drug target and inhibition of this pump is also being considered as a possible treatment against malaria (Turner, 2016).
Among SERCA inhibitors of pharmacological interest, it is worth mentioning the polyphenolic compound curcumin (Table 1). Curcumin, which is obtained from the spice turmeric, is known for its antioxidant, anti-inflammatory and anticancer effects (Schaffer et al., 2015). It was reported that curcumin inhibits SERCA activity with an IC50 in the micromolar range (Bilmen et al., 2001; Dao et al., 2016) by stabilizing the E1 conformational state of SERCA and preventing ATP binding and ATP-dependent phosphoenzyme formation (Bilmen et al., 2001). In the search for new antimalarial agents, curcumin was shown to possess a remarkable antiplasmodial activity, as demonstrated by the inhibitory effects of curcumin on a chloroquine-resistant P. falciparum strain (Reddy et al., 2005). It was proposed that the PfATP6 protein could be a possible target for curcumin antimalarial action (Reddy et al., 2005). In a very recent study, selected curcumin analogs were synthesized and their antimalarial activity against different P. falciparum strains was evaluated (Dohutia et al., 2017). In particular, molecular docking was performed to investigate the interaction of these curcumin analogs with PfATP6. The results of this study may provide useful information for the development of curcumin derivatives which could serve as promising drug candidates against malaria.
Pharmacological Stimulation of SERCA Activity
Molecules that are able to stimulate SERCA activity have been recently identified (Table 1). A remarkable example of SERCA activators is the drug istaroxime. Istaroxime is an innovative cardiac drug which combines inotropic (cardiomyocyte contraction) and lusotropic (cardiomyocyte relaxation) properties (Hasenfuss and Teerlink, 2011). Istaroxime has a double mechanism of action, i.e., it inhibits Na+,K+-ATPase activity and exerts a stimulatory effect on SERCA2a (Micheletti et al., 2002, 2007; Rocchetti et al., 2005, 2008), the SERCA isoform in the heart which is central to cardiac electrophysiological and mechanical function. Preclinical studies and clinical trials indicate that combining SERCA2a stimulation and Na+,K+-ATPase inhibition may increase contractility and facilitate active relaxation, improving both systolic and diastolic heart function (Gheorghiade et al., 2011). The stimulatory effect of istaroxime on SERCA activity was investigated by combining different experimental methods, including electrophysiological measurements on dog cardiac SR vesicles adsorbed to a SSM (Ferrandi et al., 2013). This study shows that istaroxime enhances SERCA2a activity, Ca2+ uptake, and Ca2+-dependent charge movements into SR vesicles from healthy or failing dog hearts. It was proposed that istaroxime acts by displacing the regulatory protein phospholamban (PLN) from the SERCA2a/PLN complex, thereby removing the inhibitory effect of PLN on this complex. Displacement of PLN from SERCA2a may favor the SERCA2a conformational transition E2 to E1, thus accelerating Ca2+ cycling. Istaroxime with its unique mode of action may provide a new small-molecule therapeutics for the treatment of both acute and chronic heart failure.
Very recently, a pyridone derivative was reported to activate the SERCA2a isoform by attenuating the inhibitory effect of PLN (Kaneko et al., 2017). In vitro and in vivo experiments have demonstrated that the pyridone derivative stimulates the Ca2+-dependent ATPase activity of cardiac SR vesicles, increases Ca2+ transients of isolated adult rat cardiomyocytes and accelerates contraction and relaxation of isolated perfused rat hearts. It was concluded that the pyridone derivative behaves like a SERCA2a activator, which binds and inhibits PLN, and enhances systolic and diastolic functions of the heart. The pyridone derivative is thus proposed as a novel lead compound for therapeutic applications in heart failure.
Impaired SERCA function leads to elevation of intracellular calcium concentration and alterations in calcium homeostasis, which trigger endoplasmic reticulum (ER) stress. ER stress is associated with a variety of common diseases (Oyadomari and Mori, 2004), including the metabolic syndrome and type 2 diabetes (Back and Kaufman, 2012). Pharmacological activation of SERCA can reduce ER stress and may therefore represent a novel strategy for the treatment of diabetes and metabolic disorders. A recent study (Kang et al., 2016) evaluated the metabolic effects of the quinoline-amide compound CDN1163, which is a novel SERCA activator (Cornea et al., 2013; Gruber et al., 2014). CDN1163 directly binds to the SERCA enzyme to activate Ca2+-ATPase activity, probably via an allosteric mechanism (Cornea et al., 2013; Gruber et al., 2014). Kang et al. (2016) demonstrated that CDN1163 activation of the SERCA2b isoform from mouse liver reduces ER stress and improves mitochondrial efficiency and metabolic parameters in an animal model of insulin resistance and type 2 diabetes (ob/ob mice), suggesting that SERCA activators may represent promising pharmacological agents to treat diabetes and metabolic dysfunction. Finally, since defective SERCA is a significant cause of ER stress and neuron loss in Parkinson's disease, direct activation of SERCA by small-molecule drugs is currently explored as a viable strategy to develop a novel therapeutic approach to cure Parkinson's disease (Dahl, 2017).
Conclusions
SERCA plays an essential role in the maintenance of cellular calcium homeostasis and calcium pump malfunction is associated to severe disorders and several pathophysiological conditions. Therefore, the SERCA enzyme represents an important target for the development of new drugs. SERCA inhibitors have been identified as potential drug candidates against various diseases. As discussed above, inhibition of SERCA activity in cancer cells provides an alternative therapeutic approach to cure different types of cancer. Moreover, specific inhibitors targeting the SERCA ortholog PfATP6 in the parasite P. falciparum may serve as innovative and effective antimalarial agents. In addition, studies on novel SERCA activators indicate that pharmacological activation of SERCA may constitute a promising strategy for the treatment of heart failure and diabetes. Research effort is devoted to the synthesis of highly selective and potent drugs, that can target SERCA in a tissue-specific manner. As most tissue/cell types can express more than one SERCA isoform, it would be highly desirable, although very challenging, to develop isoform-specific drugs that can interact selectively with one SERCA isoform, leaving the other protein molecules intact (Yatime et al., 2009; Michelangeli and East, 2011).
Author Contributions
FT-B: Wrote the manuscript and revised it critically for important intellectual content; SS, RG, and MM: Edited the manuscript and revised it critically for important intellectual content; SS: Helped FT-B in preparing the figures. All authors have read and agreed with the final version of the manuscript.
Conflict of Interest Statement
The authors declare that the research was conducted in the absence of any commercial or financial relationships that could be construed as a potential conflict of interest.
Acknowledgments
The Italian Ministry of Education, University and Research (MIUR) and Ente Cassa di Risparmio di Firenze are gratefully acknowledged for support.
Abbreviations
Br2-TITU, 1,3-dibromo-2,4,6-tris(methyl-isothio-uronium) benzene; DBHQ, 2,5-di(tert-butyl)hydroquinone; CPA, cyclopiazonic acid; ER, endoplasmic reticulum; PLN, phospholamban; PSMA, prostate-specific membrane antigen; SR, sarcoplasmic reticulum; SERCA, sarco(endo)plasmic reticulum Ca2+-ATPase; SSM, solid supported membrane; TG, thapsigargin.
References
Andersen, T. B., López, C. Q., Manczak, T., Martinez, K., and Simonsen, H. T. (2015). Thapsigargin–from Thapsia L. to mipsagargin. Molecules 20, 6113–6127. doi: 10.3390/molecules20046113
Arnou, B., Montigny, C., Morth, J. P., Nissen, P., Jaxel, C., Møller, J. V., et al. (2011). The Plasmodium falciparum Ca2+-ATPase PfATP6: insensitive to artemisinin, but a potential drug target. Biochem. Soc. Trans. 39, 823–831. doi: 10.1042/BST0390823
Avellanal, M., Rodriguez, P., and Barrigon, S. (1998). Protective effects of cyclopiazonic acid on ischemia-reperfusion injury in rabbit hearts. J. Cardiovasc. Pharmacol. 32, 845–851. doi: 10.1097/00005344-199811000-00022
Back, S. H., and Kaufman, R. J. (2012). Endoplasmic reticulum stress and type 2 diabetes. Annu. Rev. Biochem. 81, 767–793. doi: 10.1146/annurev-biochem-072909-095555
Bartolommei, G., Tadini-Buoninsegni, F., Moncelli, M. R., Gemma, S., Camodeca, C., Butini, S., et al. (2011). The Ca2+-ATPase (SERCA1) is inhibited by 4-aminoquinoline derivatives through interference with catalytic activation by Ca2+, whereas the ATPase E2 state remains functional. J. Biol. Chem. 286, 38383–38389. doi: 10.1074/jbc.M111.287276
Bazzone, A., Barthmes, M., and Fendler, K. (2017). SSM-based electrophysiology for transporter research. Methods Enzymol. 594, 31–83. doi: 10.1016/bs.mie.2017.05.008
Berman, M. C., and Karlish, S. J. (2003). Interaction of an aromatic dibromoisothiouronium derivative with the Ca2+-ATPase of skeletal muscle sarcoplasmic reticulum. Biochemistry 42, 3556–3566. doi: 10.1021/bi026071n
Bilmen, J. G., Khan, S. Z., Javed, M. H., and Michelangeli, F. (2001). Inhibition of the SERCA Ca2+ pumps by curcumin. Curcumin putatively stabilizes the interaction between the nucleotide-binding and phosphorylation domains in the absence of ATP. Eur. J. Biochem. 268, 6318–6327. doi: 10.1046/j.0014-2956.2001.02589.x
Brini, M., and Carafoli, E. (2009). Calcium pumps in health and disease. Physiol. Rev. 89, 1341–1378. doi: 10.1152/physrev.00032.2008
Bublitz, M., Morth, J. P., and Nissen, P. (2011). P-type ATPases at a glance. J. Cell Sci. 124, 2515–2519. doi: 10.1242/jcs.088716
Bublitz, M., Musgaard, M., Poulsen, H., Thøgersen, L., Olesen, C., Schiøtt, B., et al. (2013). Ion pathways in the sarcoplasmic reticulum Ca2+-ATPase. J. Biol. Chem. 288, 10759–10765. doi: 10.1074/jbc.R112.436550
Cardi, D., Pozza, A., Arnou, B., Marchal, E., Clausen, J. D., Andersen, J. P., et al. (2010). Purified E255L mutant SERCA1a and purified PfATP6 are sensitive to SERCA-type inhibitors but insensitive to artemisinins. J. Biol. Chem. 285, 26406–26416. doi: 10.1074/jbc.M109.090340
Cornea, R. L., Gruber, S. J., Lockamy, E. L., Muretta, J. M., Jin, D., Chen, J., et al. (2013). High-throughput FRET assay yields allosteric SERCA activators. J. Biomol. Screen. 18, 97–107. doi: 10.1177/1087057112456878
Cui, C., Merritt, R., Fu, L., and Pan, Z. (2017). Targeting calcium signaling in cancer therapy. Acta Pharm. Sin. B 7, 3–17. doi: 10.1016/j.apsb.2016.11.001
Dahl, R. (2017). A new target for Parkinson's disease: small molecule SERCA activator CDN1163 ameliorates dyskinesia in 6-OHDA-lesioned rats. Bioorg. Med. Chem. 25, 53–57. doi: 10.1016/j.bmc.2016.10.008
Dao, T. T., Sehgal, P., Tung, T. T., Møller, J. V., Nielsen, J., Palmgren, M., et al. (2016). Demethoxycurcumin is a potent inhibitor of P-Type ATPases from diverse kingdoms of life. PLoS ONE 11:e0163260. doi: 10.1371/journal.pone.0163260
de Meis, L., and Vianna, A. L. (1979). Energy interconversion by the Ca2+-dependent ATPase of the sarcoplasmic reticulum. Annu. Rev. Biochem. 48, 275–292. doi: 10.1146/annurev.bi.48.070179.001423
Denmeade, S. R., Mhaka, A. M., Rosen, D. M., Brennen, W. N., Dalrymple, S., Dach, I., et al. (2012). Engineering a prostate-specific membrane antigen-activated tumor endothelial cell prodrug for cancer therapy. Sci. Transl. Med. 4:140ra86. doi: 10.1126/scitranslmed.3003886
Di Marino, D., D'Annessa, I., Coletta, A., Via, A., and Tramontano, A. (2015). Characterization of the differences in the cyclopiazonic acid binding mode to mammalian and P. falciparum Ca2+ pumps: a computational study. Proteins 83, 564–574. doi: 10.1002/prot.24734
Doan, N. T., Paulsen, E. S., Sehgal, P., Møller, J. V., Nissen, P., Denmeade, S. R., et al. (2015). Targeting thapsigargin towards tumors. Steroids 97, 2–7. doi: 10.1016/j.steroids.2014.07.009
Dohutia, C., Chetia, D., Gogoi, K., and Sarma, K. (2017). Design, in silico and in vitro evaluation of curcumin analogues against Plasmodium falciparum. Exp. Parasitol. 175, 51–58. doi: 10.1016/j.exppara.2017.02.006
Eckstein-Ludwig, U., Webb, R. J., Van Goethem, I. D., East, J. M., Lee, A. G., Kimura, M., et al. (2003). Artemisinins target the SERCA of Plasmodium falciparum. Nature 424, 957–961. doi: 10.1038/nature01813
Ferrandi, M., Barassi, P., Tadini-Buoninsegni, F., Bartolommei, G., Molinari, I., Tripodi, M. G., et al. (2013). Istaroxime stimulates SERCA2a and accelerates calcium cycling in heart failure by relieving phospholamban inhibition. Br. J. Pharmacol. 169, 1849–1861. doi: 10.1111/bph.12278
Gheorghiade, M., Ambrosy, A. P., Ferrandi, M., and Ferrari, P. (2011). Combining SERCA2a activation and Na-K ATPase inhibition: a promising new approach to managing acute heart failure syndromes with low cardiac output. Discov. Med. 12, 141–151.
Gruber, S. J., Cornea, R. L., Li, J., Peterson, K. C., Schaaf, T. M., Gillispie, G. D., et al. (2014). Discovery of enzyme modulators via high-throughput time-resolved FRET in living cells. J. Biomol. Screen. 19, 215–222. doi: 10.1177/1087057113510740
Hasenfuss, G., and Teerlink, J. R. (2011). Cardiac inotropes: current agents and future directions. Eur. Heart J. 32, 1838–1845. doi: 10.1093/eurheartj/ehr026
Inesi, G., Hua, S., Xu, C., Ma, H., Seth, M., Prasad, A. M., et al. (2005). Studies of Ca2+ ATPase (SERCA) inhibition. J. Bioenerg. Biomembr. 37, 365–368. doi: 10.1007/s10863-005-9472-1
Inesi, G., Pilankatta, R., and Tadini-Buoninsegni, F. (2014). Biochemical characterization of P-type copper ATPases. Biochem. J. 463, 167–176. doi: 10.1042/BJ20140741
Inesi, G., and Tadini-Buoninsegni, F. (2014). Ca2+/H+ exchange, lumenal Ca2+ release and Ca2+/ATP coupling ratios in the sarcoplasmic reticulum ATPase. J. Cell Commun. Signal. 8, 5–11. doi: 10.1007/s12079-013-0213-7
Kaneko, M., Yamamoto, H., Sakai, H., Kamada, Y., Tanaka, T., Fujiwara, S., et al. (2017). A pyridone derivative activates SERCA2a by attenuating the inhibitory effect of phospholamban. Eur. J. Pharmacol. 814, 1–8. doi: 10.1016/j.ejphar.2017.07.035
Kang, S., Dahl, R., Hsieh, W., Shin, A., Zsebo, K. M., Buettner, C., et al. (2016). Small molecular allosteric activator of the sarco/endoplasmic reticulum Ca2+-ATPase (SERCA) attenuates diabetes and metabolic disorders. J. Biol. Chem. 291, 5185–5198. doi: 10.1074/jbc.M115.705012
Kelety, B., Diekert, K., Tobien, J., Watzke, N., Dörner, W., Obrdlik, P., et al. (2006). Transporter assays using solid supported membranes: a novel screening platform for drug discovery. Assay Drug. Dev. Technol. 4, 575–582. doi: 10.1089/adt.2006.4.575
Kühlbrandt, W. (2004). Biology, structure and mechanism of P-type ATPases. Nat. Rev. Mol. Cell Biol. 5, 282–295. doi: 10.1038/nrm1354
Laursen, M., Bublitz, M., Moncoq, K., Olesen, C., Møller, J. V., Young, H. S., et al. (2009). Cyclopiazonic acid is complexed to a divalent metal ion when bound to the sarcoplasmic reticulum Ca2+-ATPase. J. Biol. Chem. 284, 13513–13518. doi: 10.1074/jbc.C900031200
Lewis, D., Pilankatta, R., Inesi, G., Bartolommei, G., Moncelli, M. R., and Tadini-Buoninsegni, F. (2012). Distinctive features of catalytic and transport mechanisms in mammalian sarco-endoplasmic reticulum Ca2+ ATPase (SERCA) and Cu+ (ATP7A/B) ATPases. J. Biol. Chem. 287, 32717–32727. doi: 10.1074/jbc.M112.373472
Liu, Y., Pilankatta, R., Lewis, D., Inesi, G., Tadini-Buoninsegni, F., Bartolommei, G., et al. (2009). High-yield heterologous expression of wild type and mutant Ca2+ ATPase: characterization of Ca2+ binding sites by charge transfer. J. Mol. Biol. 391, 858–871. doi: 10.1016/j.jmb.2009.06.044
Michelangeli, F., and East, J. M. (2011). A diversity of SERCA Ca2+ pump inhibitors. Biochem. Soc. Trans. 39, 789–797. doi: 10.1042/BST0390789
Micheletti, R., Mattera, G. G., Rocchetti, M., Schiavone, A., Loi, M. F., Zaza, A., et al. (2002). Pharmacological profile of the novel inotropic agent (E,Z)-3-((2-aminoethoxy)imino)androstane-6,17-dione hydrochloride (PST2744). J. Pharmacol. Exp. Ther. 303, 592–600. doi: 10.1124/jpet.102.038331
Micheletti, R., Palazzo, F., Barassi, P., Giacalone, G., Ferrandi, M., Schiavone, A., et al. (2007). Istaroxime, a stimulator of sarcoplasmic reticulum calcium adenosine triphosphatase isoform 2a activity, as a novel therapeutic approach to heart failure. Am. J. Cardiol. 99, 24A−32A. doi: 10.1016/j.amjcard.2006.09.003
Møller, J. V., Olesen, C., Winther, A. M., and Nissen, P. (2010). The sarcoplasmic Ca2+-ATPase: design of a perfect chemi-osmotic pump. Q. Rev. Biophys. 43, 501–566. doi: 10.1017/S003358351000017X
Moncoq, K., Trieber, C. A., and Young, H. S. (2007). The molecular basis for cyclopiazonic acid inhibition of the sarcoplasmic reticulum calcium pump. J. Biol. Chem. 282, 9748–9757. doi: 10.1074/jbc.M611653200
Moore, G. A., McConkey, D. J., Kass, G. E., O'Brien, P. J., and Orrenius, S. (1987). 2,5-Di(tert-butyl)-1,4-benzohydroquinone–a novel inhibitor of liver microsomal Ca2+ sequestration. FEBS Lett. 224, 331–336. doi: 10.1016/0014-5793(87)80479-9
Obara, K., Miyashita, N., Xu, C., Toyoshima, I., Sugita, Y., Inesi, G., et al. (2005). Structural role of countertransport revealed in Ca2+ pump crystal structure in the absence of Ca2+. Proc. Natl. Acad. Sci. U.S.A. 102, 14489–14496. doi: 10.1073/pnas.0506222102
Olesen, C., Sørensen, T. L., Nielsen, R. C., Møller, J. V., and Nissen, P. (2004). Dephosphorylation of the calcium pump coupled to counterion occlusion. Science 306, 2251–2255. doi: 10.1126/science.1106289
Oyadomari, S., and Mori, M. (2004). Roles of CHOP/GADD153 in endoplasmic reticulum stress. Cell Death Differ. 11, 381–389. doi: 10.1038/sj.cdd.4401373
Pintschovius, J., and Fendler, K. (1999). Charge translocation by the Na+/K+-ATPase investigated on solid supported membranes: rapid solution exchange with a new technique. Biophys. J. 76, 814–826. doi: 10.1016/S0006-3495(99)77245-0
Pintschovius, J., Fendler, K., and Bamberg, E. (1999). Charge translocation by the Na+/K+-ATPase investigated on solid supported membranes: cytoplasmic cation binding and release. Biophys. J. 76, 827–836. doi: 10.1016/S0006-3495(99)77246-2
Rasmussen, U., Brøogger Christensen, S., and Sandberg, F. (1978). Thapsigargine and thapsigargicine, two new histamine liberators from Thapsia garganica L. Acta Pharm. Suec. 15, 133–140.
Reddy, R. C., Vatsala, P. G., Keshamouni, V. G., Padmanaban, G., and Rangarajan, P. N. (2005). Curcumin for malaria therapy. Biochem. Biophys. Res. Commun. 326, 472–474. doi: 10.1016/j.bbrc.2004.11.051
Rocchetti, M., Alemanni, M., Mostacciuolo, G., Barassi, P., Altomare, C., Chisci, R., et al. (2008). Modulation of sarcoplasmic reticulum function by PST2744 [istaroxime; (E,Z)-3-((2-aminoethoxy)imino) androstane-6,17-dione hydrochloride)] in a pressure-overload heart failure model. J. Pharmacol. Exp. Ther. 326, 957–965. doi: 10.1124/jpet.108.138701
Rocchetti, M., Besana, A., Mostacciuolo, G., Micheletti, R., Ferrari, P., Sarkozi, S., et al. (2005). Modulation of sarcoplasmic reticulum function by Na+/K+ pump inhibitors with different toxicity: digoxin and PST2744 [(E,Z)-3-((2-aminoethoxy)imino)androstane-6,17-dione hydrochloride]. J. Pharmacol. Exp. Ther. 313, 207–215. doi: 10.1124/jpet.104.077933
Sadafi, F. Z., Massai, L., Bartolommei, G., Moncelli, M. R., Messori, L., and Tadini-Buoninsegni, F. (2014). Anticancer ruthenium(III) complex KP1019 interferes with ATP-dependent Ca2+ translocation by sarco-endoplasmic reticulum Ca2+-ATPase (SERCA). ChemMedChem 9, 1660–1664. doi: 10.1002/cmdc.201402128
Sagara, Y., and Inesi, G. (1991). Inhibition of the sarcoplasmic reticulum Ca2+ transport ATPase by thapsigargin at subnanomolar concentrations. J. Biol. Chem. 266, 13503–13506.
Schaffer, M., Schaffer, P. M., and Bar-Sela, G. (2015). An update on Curcuma as a functional food in the control of cancer and inflammation. Curr. Opin. Clin. Nutr. Metab. Care. 18, 605–611. doi: 10.1097/MCO.0000000000000227
Schulz, P., Garcia-Celma, J. J., and Fendler, K. (2008). SSM-based electrophysiology. Methods 46, 97–103. doi: 10.1016/j.ymeth.2008.07.002
Seidler, N. W., Jona, I., Vegh, M., and Martonosi, A. (1989). Cyclopiazonic acid is a specific inhibitor of the Ca2+-ATPase of sarcoplasmic reticulum. J. Biol. Chem. 264, 17816–17823.
Tadini-Buoninsegni, F., and Bartolommei, G. (2016). Electrophysiological measurements on solid supported membranes. Methods Mol. Biol. 1377, 293–303. doi: 10.1007/978-1-4939-3179-8_26
Tadini-Buoninsegni, F., Bartolommei, G., Moncelli, M. R., and Fendler, K. (2008a). Charge transfer in P-type ATPases investigated on planar membranes. Arch. Biochem. Biophys. 476, 75–86. doi: 10.1016/j.abb.2008.02.031
Tadini-Buoninsegni, F., Bartolommei, G., Moncelli, M. R., Guidelli, R., and Inesi, G. (2006). Pre-steady state electrogenic events of Ca2+/H+ exchange and transport by the Ca2+-ATPase. J. Biol. Chem. 281, 37720–37727. doi: 10.1074/jbc.M606040200
Tadini-Buoninsegni, F., Bartolommei, G., Moncelli, M. R., Inesi, G., Galliani, A., Sinisi, M., et al. (2014). Translocation of platinum anticancer drugs by human copper ATPases ATP7A and ATP7B. Angew. Chem. Int. Ed. Engl. 53, 1297–1301. doi: 10.1002/anie.201307718
Tadini-Buoninsegni, F., Bartolommei, G., Moncelli, M. R., Tal, D. M., Lewis, D., and Inesi, G. (2008b). Effects of high-affinity inhibitors on partial reactions, charge movements, and conformational states of the Ca2+ transport ATPase (sarco-endoplasmic reticulum Ca2+ ATPase). Mol. Pharmacol. 73, 1134–1140. doi: 10.1124/mol.107.043745
Tadini-Buoninsegni, F., and Smeazzetto, S. (2017). Mechanisms of charge transfer in human copper ATPases ATP7A and ATP7B. IUBMB Life 69, 218–225. doi: 10.1002/iub.1603
Tadini-Buoninsegni, F., Sordi, G., Smeazzetto, S., Natile, G., and Arnesano, F. (2017). Effect of cisplatin on the transport activity of PII-type ATPases. Metallomics 9, 960–968. doi: 10.1039/C7MT00100B
Toyoshima, C. (2008). Structural aspects of ion pumping by Ca2+-ATPase of sarcoplasmic reticulum. Arch. Biochem. Biophys. 476, 3–11. doi: 10.1016/j.abb.2008.04.017
Toyoshima, C., and Cornelius, F. (2013). New crystal structures of PII-type ATPases: excitement continues. Curr. Opin. Struct. Biol. 23, 507–514. doi: 10.1016/j.sbi.2013.06.005
Toyoshima, C., and Nomura, H. (2002). Structural changes in the calcium pump accompanying the dissociation of calcium. Nature 418, 605–611. doi: 10.1038/nature00944
Turner, H. (2016). Spiroindolone NITD609 is a novel antimalarial drug that targets the P-type ATPase PfATP4. Future Med. Chem. 8, 227–238. doi: 10.4155/fmc.15.177
Wang, D., and Lippard, S. J. (2005). Cellular processing of platinum anticancer drugs. Nat. Rev. Drug Discov. 4, 307–320. doi: 10.1038/nrd1691
Xu, C., Ma, H., Inesi, G., Al-Shawi, M. K., and Toyoshima, C. (2004). Specific structural requirements for the inhibitory effect of thapsigargin on the Ca2+ ATPase SERCA. J. Biol. Chem. 279, 17973–17979. doi: 10.1074/jbc.M313263200
Keywords: anticancer drug, antimalarial agent, drug-protein interaction, sarco(endo)plasmic reticulum Ca2+-ATPase, SERCA activator, SERCA inhibitor, solid supported membrane
Citation: Tadini-Buoninsegni F, Smeazzetto S, Gualdani R and Moncelli M (2018) Drug Interactions With the Ca2+-ATPase From Sarco(Endo)Plasmic Reticulum (SERCA). Front. Mol. Biosci. 5:36. doi: 10.3389/fmolb.2018.00036
Received: 07 February 2018; Accepted: 26 March 2018;
Published: 11 April 2018.
Edited by:
Cesare Indiveri, University of Calabria, ItalyReviewed by:
Annamaria Tonazzi, Consiglio Nazionale delle Ricerche (CNR), ItalyRonald Clarke, University of Sydney, Australia
Copyright © 2018 Tadini-Buoninsegni, Smeazzetto, Gualdani and Moncelli. This is an open-access article distributed under the terms of the Creative Commons Attribution License (CC BY). The use, distribution or reproduction in other forums is permitted, provided the original author(s) and the copyright owner are credited and that the original publication in this journal is cited, in accordance with accepted academic practice. No use, distribution or reproduction is permitted which does not comply with these terms.
*Correspondence: Francesco Tadini-Buoninsegni, ZnJhbmNlc2NvLnRhZGluaUB1bmlmaS5pdA==