Mechanisms and inhibitors of ferroptosis in psoriasis
- 1Health Management Center, Sichuan Academy of Medical Science and Sichuan Provincial People’s Hospital, University of Electronic Science and Technology of China, Chengdu, China
- 2Department of Rheumatology and Immunology, Sichuan Academy of Medical Science and Sichuan Provincial People’s Hospital, University of Electronic Science and Technology of China, Chengdu, China
- 3Clinical Immunology Translational Medicine Key Laboratory of Sichuan Province, Sichuan Provincial People’s Hospital, University of Electronic Science and Technology of China, Chengdu, China
- 4School of Medicine, University of Electronic Science and Technology of China, Chengdu, China
- 5Department of Rheumatology, Wenjiang District People’s Hospital, Chengdu, China
- 6School of Medicine, Faculty of Medicine and Health, The University of Leeds, Leeds, United Kingdom
- 7Department of Critical Care Medicine, Sichuan Academy of Medical Science and Sichuan Provincial People’s Hospital, University of Electronic Science and Technology of China, Chengdu, China
- 8Department of Rheumatology and Immunology, Sichuan Academy of Medical Science and Sichuan Provincial People’s Hospital, Wenjiang District People’s Hospital, Chengdu, China
Psoriasis is a chronic inflammatory skin disease that features localized or widespread erythema, papules, and scaling. It is common worldwide and may be distributed throughout the whole body. The pathogenesis of psoriasis is quite complex and the result of the interplay of genetic, environmental and immune factors. Ferroptosis is an iron-dependent programmed death that is different from cell senescence, apoptosis, pyroptosis and other forms of cell death. Ferroptosis involves three core metabolites, iron, lipids, and reactive oxygen species (ROS), and it is primarily driven by lipid peroxidation. Ferrostatin-1 (Fer-1) is an effective inhibitor of lipid peroxidation that inhibited the changes related to ferroptosis in erastin-treated keratinocytes and blocked inflammatory responses. Therefore, it has a certain effect on the treatment of psoriatic lesions. Although ferroptosis is closely associated with a variety of human diseases, such as inflammatory diseases, no review has focused on ferroptosis in psoriasis. This mini review primarily focused on the pathogenesis of psoriasis, the mechanisms of ferroptosis, the connection between ferroptosis and psoriasis and ferroptosis inhibitors in psoriasis treatment. We discussed recent research advances and perspectives on the relationship between ferroptosis and psoriasis.
Introduction
Psoriasis (PsO) is a chronic, recurrent, and autoimmune skin disorder caused by multiple risk factors, including genetic and environmental factors (Pezzolo and Naldi, 2019; Kanda et al., 2020; Madden et al., 2020; Reid and Griffiths, 2020). The incidence of psoriasis varies greatly worldwide, with a global prevalence of approximately 2%–4% (Liang et al., 2021) or approximately 125 million people (Armstrong and Read, 2020). According to the clinical features, PsO is divided into four types: vulgaris, arthrogryposis, pustular, and erythroderma (Navarini et al., 2017; Carrasquillo et al., 2020; Lo et al., 2020; Nast et al., 2020). Psoriatic lesions initially appear as red papules. With the development of the disease, the red plaques exhibit various morphologies, such as dot-like, map-like, or covered with thick, silvery-white scales (Raychaudhuri et al., 2014). Keratinocytes play an essential role in psoriasis, and their death amplifies the inflammatory effect. A recent study showed that keratinocytes in psoriatic lesions exhibited lipid peroxidation, which is related to a novel type of cell death, ferroptosis (Wen et al., 2019; Shou et al., 2021).
The Brent Stockwell Laboratory formally defined ferroptosis for the first time in 2012, and it is caused by lipid peroxidation due to the accumulation of reactive oxygen species (ROS) (Dixon et al., 2012). Lipids are organic substances that play critical roles in human tissues. Lipids form the cell membrane and participate in a variety of processes, including cell proliferation and apoptosis, inflammatory conditions, and immune responses (van Meer et al., 2008; Nowowiejska et al., 2021). Iron, lipids and ROS play important roles in cell survival. However, these factors lead to fatal damage when metabolic disorders occur (Liu and Gu, 2022). Ferroptosis is closely related to a variety of human diseases (Jiang et al., 2021), including tumors, septicemia, bacterial, and viral diseases, some neurodegenerative diseases and autoimmune diseases, such as systemic lupus erythematosus, inflammatory bowel diseases, and PsO, which we will discuss briefly in this review (Gunther et al., 2013; Li et al., 2021; Shou et al., 2021).
Pathogenesis of psoriasis
The pathogenesis of psoriasis is extremely complicated and is currently believed that the development of the disease is caused by the joint action of genetic and environmental factors, which together result in inflammatory cell infiltration, keratinocyte proliferation, and T cell differentiation, etc. (Ogawa and Okada, 2020; Solmaz et al., 2020). The heritability of psoriasis is approximately 66%–90%, which is one of the highest heritability rates of multifactorial genetic diseases (Traks et al., 2019). Environmental factors, such as infection, obesity, nicotine dependence, etc., also induce and aggravate the progression of psoriasis (Madden et al., 2020; Karpinska-Mirecka et al., 2021; Reolid et al., 2021). For example, drip psoriasis is closely related to acute streptococcal infection, which indicates a link between psoriasis and bacterial infection (Madden et al., 2020; Zhou and Yao, 2022). Although the pathogenic etiology of psoriasis is not clear, psoriasis susceptibility genes are associated with immune mechanisms (Vicic et al., 2021; van de Kerkhof, 2022). The innate and adaptive immune systems play crucial roles (Xu and Zhang, 2017), especially CD4 and CD8 cells and the interleukin-23/T helper 17 pathway (Girolomoni et al., 2017; Ly et al., 2019; Iznardo and Puig, 2021). The abnormal activation and migration of specific T cells to the skin leads to the gradual accumulation of inflammatory cells (Xu and Zhang, 2017). Inflammatory cytokines produced by Th1 and Th22 cells, such as tumor necrosis factor (TNF), interleukin (IL)-17, and IL-22, all contribute to the inflammation. With the induction of IL-23, Th17 cells differentiate, proliferate and secrete IL-17, which disrupts the integrity of the skin barrier and induces keratinocyte hyperproliferation (Rendon and Schakel, 2019).
Mechanisms of ferroptosis
As a new form of programmed cell death (Dixon et al., 2012), ferroptosis differs from other forms of cell death, such as pyroptosis, apoptosis or cellular senescence, and results in membrane damage and cell lysis (Riegman et al., 2020; Ding et al., 2021). Iron, lipids, and ROS maintain a steady state for cell survival (Liu and Gu, 2022). The accumulation of ROS causes lipid peroxidation and further induces ferroptosis (Stockwell, 2022). The detailed mechanisms of ferroptosis are illustrated below and shown in Figure 1.
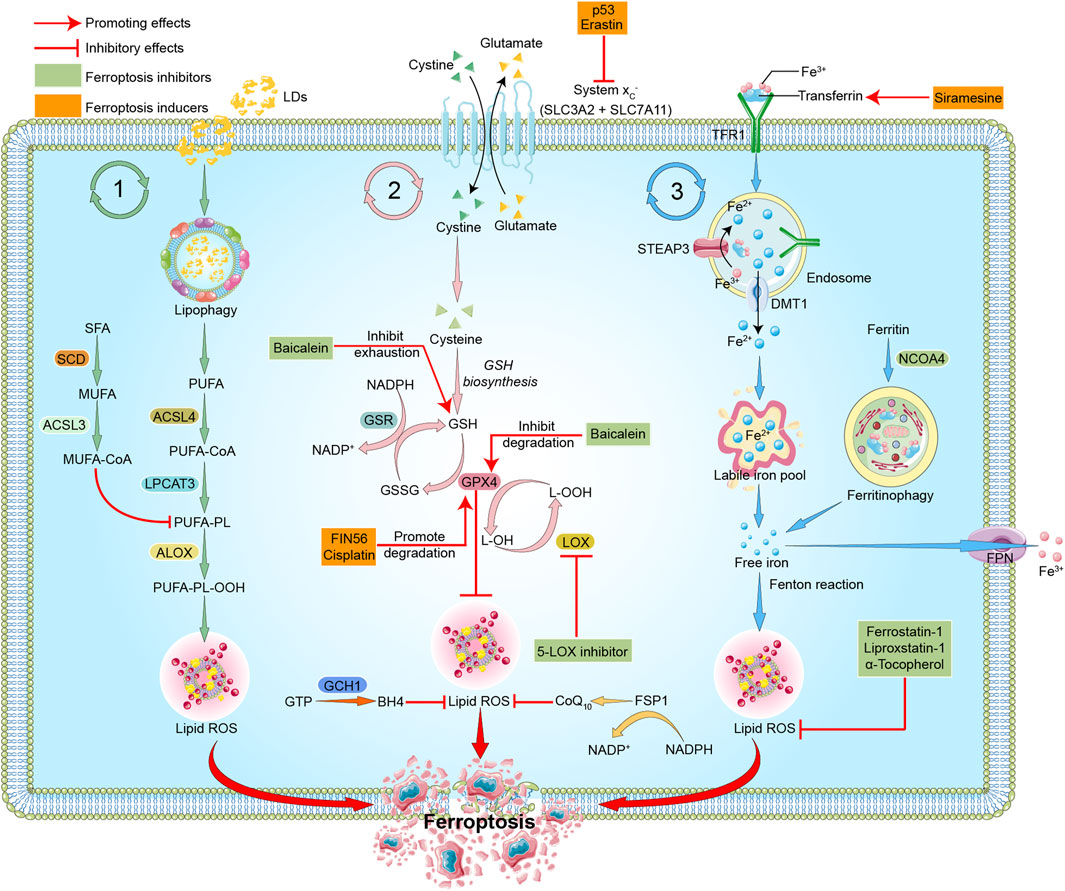
FIGURE 1. Mechanisms of ferroptosis. The three pathways represent lipid metabolism, antioxidant metabolism, and iron metabolism in ferroptosis, respectively. In lipid metabolism, LDs are degraded via lipophagy to release free fatty acids, including PUFAs. Accumulated PUFAs are catalyzed by ACSL4 to generate the key substrate PUFA-CoA, which is finally esterified into PUFA-PLs by LPCAT3. PUFA-PLs can be peroxidized to PUFA-PL-OOH through enzymatic and non-enzymatic lipid peroxidation reactions in the presence of bioactive iron. PLOOH can generate lipid hydroxyl radicals and lipid peroxyl radicals, sensitizing the cell to ferroptosis. MUFAs, likely through MUFA-PLs from MUFA-CoA, can inhibit PUFA-PLs by replacing PUFA from phosphatidylethanolamine, thus reducing the available substrate for lipid peroxidation. In antioxidant metabolism, the two subunits SLC3A2 and SLC7A11 constitute the system Xc-, which is an amino acid antiporter that mediates the exchange of extracellular cystine and intracellular glutamate across the plasma membrane. After entering the cells, cystine is reduced to cysteine and participates in the synthesis of GSH, which serves the substrate of GPX4. GPX4 reduces cytotoxic lipid peroxide (L-OOH) to the corresponding alcohol (L-OH), thus inhibiting the formation of lipid peroxide and ferroptosis. GPX4 can also transform GSH into GSSG, and GSSG can be reduced to GSH under the action of GSR. FSP1-CoQ10 or GCH1-BH4 pathway inhibits ferroptosis independently of GSH. In iron metabolism, ferric iron (Fe3+) is bound to transferrin (TF) to form TF-Fe3+, which is then taken up by the TF receptor (TFR1). In endosomes, STEAP3 reduces Fe3+ to Fe2+, which is then released to cytoplasm through DMT1, and stored in LIP or ferritin. Fe2+ mediates the Fenton reaction, thereby promoting lipid peroxidation and ferroptosis. Excess Fe2+ is oxidized to Fe3+ by FPN. In addition, NCOA4-mediated ferritinophagy can increase LIP, thereby sensitizing the cell to ferroptosis through Fenton reaction. The target of ferroptosis inducers and inhibitors are also indicated. P53 and erastin can interfer with the synthesis of GSH by inhibiting system Xc-. FIN56 and cisplatin promote the degradation of GPX4. Siramesine increases the expression of transferrin in iron metabolism and increases the level of intracellular ferric iron. Baicalein inhibits GSH depletion, GPX4 degradation and lipid peroxidation. 5-LOX inhibitor inhibits the production of ROS. Ferrostatin-1, Liproxstatin-1, and α-Tocopherol scavenge ROS and inhibit lipid peroxidation. Red arrows indicate promoting effects. Short lines with vertical end indicate inhibitory effect. The pink ball represents Fe3+. Blue balls represent Fe2+. Green triangles represent cystine. Yellow triangles represent glutamate. Light green triangles represent cysteine. Green boxes indicate ferroptosis inhibitors. Yellow boxes represent ferroptosis inducers. Abbreviations: ACSL3, acyl-CoA synthetase long chain family member 3; ACSL4, acyl-CoA synthetase long chain family member 4; ALOX, arachidonate lipoxygenase; BH4, tetrahydrobiopterin; CoA, coenzyme A; CoQ10, coenzyme Q10; DMT1, divalent metal transporter 1; FPN, ferroportin; FSP1, ferroptosis suppressor protein 1; GCH1, GTP cyclohydrolase 1; GPX4, glutathione peroxidase 4; GSH, glutathione; GSR, glutathione reductase; GSSG, oxidized glutathione; GTP, guanosine triphosphate; LDs, lipid droplets; LPCAT3, lysophosphatidylcholine acyltransferase 3; LIP, labile iron pool; LOX, lipoxygenase; MUFA, monounsaturated fatty acid; NADP, nicotinamide-adenine-dinucleotide phosphate; NADPH, reduced nicotinamide adenine dinucleotide phosphate; NCOA4, nuclear receptor coactivator 4; OOH, hydroperoxides; PUFA, polyunsaturated fatty acid; PL, phospholipid; ROS, reactive oxygen species; SCD, stearoyl CoA desaturase; SFA, saturated fatty acids; SLC3A2, solute carrier family 3 member 2; SLC7A11, solute carrier family 7 member 11; STEAP3, the six-transmembrane epithelial antigen of prostate 3; TF, transferrin; TFR, transferrin receptor protein.
Lipid metabolism
Lipid metabolism is closely related to ferroptosis. As one of the basic elements, long-chain polyunsaturated fatty acids (PUFAs) participate in ferroptosis and are quite sensitive to lipid peroxidation (Li et al., 2020). Free PUFAs are esterified and form film phospholipids that transmit ferroptosis signals after oxidation. PUFAs are linked to coenzyme A (CoA) via the catalysis of acyl-CoA synthetase long-chain family member 4 (ACSL4) (Das, 2019). Lysophosphatidylcholine acetyltransferase 3 (LPCAT3) re-esterifies these products into phospholipids and integrates them into membrane phospholipids (Forcina and Dixon, 2019; Li and Li, 2020; Chen et al., 2021b; Liang et al., 2022). Lipid peroxidation leads to the destruction of the lipid layer, which causes cell damage and ferroptosis. Arachidonic acid (AA) is a key phospholipid that induces ferroptosis. ACSL4 and LPCAT3 are involved in the biosynthesis of phosphatidylethanolamine (PE) of adrenaline, a derivative of arachidonic acid, which activates PUFAs and acts as key phospholipids to induce ferroptosis (Stockwell, 2022). ACSL4 and LPCAT3 are also involved in the activation of PUFAs and the binding of them to local membrane lipids, which shows the necessity of PUFAs in the membrane binding environment to have fatal effects on peroxidation (Li et al., 2020; Stockwell, 2022). Because specific carbon atoms in lipids are susceptible to peroxidation, lipid peroxidation depends on the strength of its hydrocarbon bond (Li et al., 2020).
Peroxidation of specific membrane lipids could induce ferroptosis. The latest research shows that cytochrome P450 oxidoreductase is the driver of lipid peroxidation in ferroptosis (Stockwell et al., 2020). Once the production of lipid peroxides far exceeds the antioxidant scavenging capacity, the accumulated lipid peroxides attack the adjacent PUFAs to form new lipid peroxides, which leads to the enhancement of lipid peroxides (Chen et al., 2021a; Stockwell, 2022). Continuous peroxidation of PUFAs leads to changes in the physiological state of cell membranes, such as destruction of the stability and integrity of cell membranes, and ion homeostasis inside and outside of cells (Stockwell, 2022). The decomposition products of lipid peroxide further react with and destroy essential proteins of the human body (Li et al., 2020), which ultimately leads to ferroptosis.
Iron metabolism
Iron ions are critical factors in the production of ROS via enzymatic or non-enzymatic reactions, and it participates in the process of lipid peroxidation and plays a crucial role in ferroptosis (Masaldan et al., 2019; Angelova et al., 2020; Jiang et al., 2021). The abnormal accumulation of free iron in the body often affects normal physiological processes and is a key signal of ferroptosis. Fe2+ is acquired from the intestinal absorption or red blood cells degradation. Under the action of ceruloplasmin, Fe2+ is oxidized to Fe3+, which combines with transferrin (TF) on the cell membrane to form TF-Fe3+ complex via the membrane protein TF receptor 1 (TFR1) (Frazer and Anderson, 2014). The six-transmembrane epithelial antigen of prostate 3 (STEAP3) reduces Fe3+ to Fe2+, and Fe2+ is normally stored in the labile iron pool (LIP) and ferritin, which is mediated by divalent metal transporter 1 (DMT1) or Zinc-Iron regulatory protein family 8/14 (ZIP8/14) (Li et al., 2020). Excess Fe2+ is oxidized to Fe3+ by ferroportin (FPN) (Li et al., 2020). LIP is the source of iron ions in the Fenton reaction (He et al., 2020; Lin et al., 2020). Excessive free iron in the cell mediates the Fenton reaction to produce a large amount of ROS, which further causes a cascade reaction, intensifies the lipid oxidation of the cell membrane, and induces ferroptosis (Shen et al., 2018). Ferrous iron may act as a cofactor of some enzymes that mediate lipid peroxidation and participate in the process of ferroptosis. Therefore, the physiological process of iron ions has an impact on the sensitivity of ferroptosis (Xia et al., 2021).
Silencing the TFR1 gene inhibited erastin-induced ferroptosis (Gao et al., 2015). It was also found that heat shock protein β-1 (HSPB1) inhibited ferroptosis by inhibiting TFR1 expression and reducing the intracellular iron concentration (Sun et al., 2015). In contrast, heme oxygenase-1 (HO-1) supplementation with iron accelerated erastin-induced ferroptosis (Kwon et al., 2015).
Antioxidant metabolism
The antioxidant system is the critical determining factor of the occurrence of iron-induced cell death, and it inhibits the lipid peroxidation chain reaction by reducing lipid peroxides (Seibt et al., 2019). Under physiological conditions, antioxidant enzymes, including glutathione peroxidase 4 (GPX4), inhibit the production of oxidized lipids (Hong et al., 2022). GPX4, which is a glutathione (GSH)- and selenium-dependent glutathione peroxidase, could detoxify lipid hydroperoxides (Miao et al., 2022). GSH is a cysteine-containing tripeptide that exists as an intracellular antioxidant and primarily depends on system Xc-mediated cystine uptake and the concomitant reduction of cystine to cysteine (Ursini and Maiorino, 2020). System Xc- is an amino acid antiporter that is widely distributed in the phospholipid bilayer, and it is a heterodimer composed of two subunits, SLC7A11 and SLC3A2 (Ursini and Maiorino, 2020; Stockwell, 2022). Cysteine and glutamate enter and exit the cell via system Xc-. The absorbed cysteine participates in the synthesis of GSH via a reduction reaction. With the help of GSH as a cofactor, GPX4 converts GSH to oxidized glutathione disulfide (GSSG) and reduces cytotoxic lipid peroxide (L-OOH) to the corresponding alcohols (L-OH), thereby detoxifying peroxide products and preventing the accumulation of lipid ROS, making it an important inhibitor of ferroptosis (Friedmann Angeli et al., 2019; Stockwell, 2019; Li et al., 2020; Ursini and Maiorino, 2020; Miao et al., 2022; Stockwell, 2022).
The NADPH-FSP1 (ferroptosis suppressor protein 1)-CoQ10 and GCH1 (guanosine triphosphate cyclohydrolase 1)-BH4 (tetrahydrobiopterin) pathways are also reported to be involved in inhibiting ferroptosis, with specific roles needed to be further elucidated (Zheng and Conrad, 2020). RAS-selective lethal 3 (RSL3) and the compounds DPI7 and DPI10 may be used as ferroptosis inducers by directly inhibiting the activity of GPX4, thus reduceing the antioxidant capacity of cells and causing the accumulation of ROS to exert ferroptosis (Yang et al., 2014; Magtanong et al., 2019). P53 downregulates the expression of SLC7A11 and inhibits the uptake of cystine by system Xc-, which may also lead to ferroptosis (Jiang et al., 2015; Liu et al., 2019; Liu and Gu, 2021; Liu and Gu, 2022).
Ferroptosis in psoriasis
There is an intricate relationship between ferroptosis and inflammation in psoriatic lesions. Abnormal lipid expression and metabolism are frequently observed in patients with psoriasis, especially in keratinocytes from the psoriatic lesions (Benhadou et al., 2019; Shou et al., 2021). At the single-cell level, the lipid oxidation pathway was significantly upregulated in keratinocyte groups of psoriasis, and lipid peroxidation was enhanced during psoriasis (Shou et al., 2021). Compared to other cells, such as fibroblasts, macrophages, dendritic cells, endothelial cells, and T cells, the lipid oxidation activity in keratinocytes highly correlated with the Th22/Th17 pathway and had a time- and concentration-dependent effect on the stimulation of erastin-dependent ferroptosis (Orsmond et al., 2021; Shou et al., 2021).
Cell death related to ferroptosis was also reported to be activated in psoriatic lesions. For example, GPX4 was highly expressed in all layers of the epidermis in normal samples while under-expressed in psoriatic skin, and ACSL4 was highly expressed in the basal layer of the epidermis in psoriasis compared to the normal skin (Shou et al., 2021). The expression of prostaglandin-endoperoxide synthase 2 (PTGS2) and transferrin receptor (TFRC) also increased significantly in psoriatic samples, while the expression of ferritin heavy chain 1 (FTH1) and ferritin light chain (FTL) decreased (Shou et al., 2021). PTGS2 is a potential biomarker for cells undergoing ferroptosis (Wu et al., 2022), and FTH1 and FTL is involved in the storage, entry and homeostasis of ion (Park and Chung, 2019). As a derivative of lipid peroxidation, 4-hydroxynonenol (4-HNE) is also increased in psoriatic lesions and enhances ferroptosis (Liu et al., 2020; Shou et al., 2021). GPX4 is a selenoprotein (Proneth and Conrad, 2019; Zhang et al., 2021b), and the exact reason for its reduced expression in psoriatic lesions is unknown. It is observed that selenium was decreased and related to the severity of psoriasis in patients with long disease duration, and selenium deficiency affects the biosynthesis of GPX4, which might explain the decreased antioxidant activity and susceptibility towards ferroptosis in psoriatic patients (Serwin et al., 2003; Ingold et al., 2018).
Ferroptosis not only promotes cell death, but also triggers inflammation in psoriatic keratinocytes. Several studies showed that ferroptosis triggers and amplifies a variety of inflammatory responses (Tsurusaki et al., 2019; Bebber et al., 2020; Ren et al., 2020). It enhances inflammatory responses via the release of damage-associated molecular patterns (DAMPs) and alarmins (Chen et al., 2021a), which could further activate the immune cells and significantly stimulate the expression of inflammatory cytokines, making a complex link between the inflammatory response and ferroptosis in psoriatic lesions.
Ferroptosis inhibitors in psoriasis treatment
Ferroptosis is involved in several pathophysiological processes and the development of miscellaneous conditions, including iron overload disease and myocardial diseases (Yang et al., 2020; Liu and Gu, 2021; Weber et al., 2021; Lai et al., 2022; Liu and Gu, 2022). Reasonable induction or inhibition of ferroptosis contributes to the treatment of these diseases. For example, the ferroptosis inducer erastin selectively kills tumor cells by regulating oxidative stress (Kose et al., 2019; Zhao et al., 2020). FIN56, siramesine, cisplatin and other inducers also improve the treatment of some diseases (Table 1) (Ma et al., 2016; Guo et al., 2018; Zhao et al., 2020; Zhang et al., 2021a).
However, ferroptosis inhibitors play an essential role, especially in psoriasis. There are many inhibitors, such as ferrostatin-1 (fer-1), liproxstatin-1 (lip-1) and α-Tocopherol (α-TOH) (Xie et al., 2016; Yuan et al., 2016; Zilka et al., 2017; Guerrero-Hue et al., 2019). Fer-1 and lip-1 are more effective compared toα-TOH, which is a relatively weak ferroptosis inhibitor (Stockwell et al., 2017; Shah et al., 2018; Yao et al., 2019; Miotto et al., 2020).
Fer-1 was obtained from the high-throughput screening of a small molecule library. As an aromatic amine antioxidant, Fer-1 prevented erastin-induced lipid ROS production and inhibited ferroptosis in HT-1080 cells, but it did not inhibit the cell death induced by other lethal oxidative compounds, such as H2O2 or apoptosis inducers (Armstrong and Read, 2020). Fer-1 reduces lipid peroxidation by inhibiting oxidative stress through downregulating prostaglandin endoperoxide synthase 2 and upregulating GPX4 and Nuclear factor E2 related factor 2 (NRF2) (Asano et al., 2017). In psoriatic keratinocytes, Fer-1 blocks the inflammatory responses and alleviates the skin lesions by inhibiting the lipid peroxidation (Kajarabille and Latunde-Dada, 2019; Miotto et al., 2020). Fer-1 was also demonstrated to eliminate erastin-induced death in keratinocytes and alleviate imiquimod-induced psoriasiform dermatitis in mice (Shou et al., 2021). The complex effect of Fer-1 against psoriasis-like inflammatory responses suggests that lipid peroxidation in psoriatic lesions also amplifies inflammatory responses, and the two act together to contribute to ferroptosis (Gong et al., 2019). Further studies need to identify key pathogenic mediators of this process and provide more specific and accurate therapeutic targets.
The level of mammalian targets of rapamycin (mTOR) signaling protein and the expression of mTOR complex 1 (mTORC1) was elevated in psoriatic skin (Raychaudhuri and Raychaudhuri, 2014). Through activating mTORC1, cystine and cysteine promotes the biosynthesis of not only GSH, but also GPX4, while inhibition of mTORC1 sensitizes cells to ferroptosis by decreasing the synthesis of GPX4, demonstrating a link between mTORC1 and ferroptosis (Zhang et al., 2021b; Conlon et al., 2021).
Conclusion and perspective
Ferroptosis primarily consists of three components: 1) oxidation of PUFAs, 2) excess active iron, and 3) inactivation of GPX4 (Battaglia et al., 2020). Studies have shown that ferroptosis is closely associated with the development of several human diseases, including autoimmune diseases, tumors, infectious diseases, and neurodegenerative diseases, such as Alzheimer’s disease (AD), Parkinson’s disease (PD), and Huntington’s disease (Qiu et al., 2020; Reichert et al., 2020; Lai et al., 2022). The current mini review focused on the mechanisms and treatment of psoriasis associated with ferroptosis and the therapeutic advances that contribute to the effective improvement of psoriatic skin manifestations, including skin thickness and scales, in the role of the inhibition of ferroptosis. Although studies on the role of ferroptosis in psoriasis are scarce, the limited available literature shows that ferroptosis inhibitors have good effects in the treatment of this disease.
However, as a novel cell death mechanism, many issues must be solved urgently. Current research results of ferroptosis in psoriasis are limited, and the specific role of ferroptosis in the occurrence and development of psoriasis is not known. The medical field faces challenges in psoriasis treatment, such as the side effects and adherence of medications. To promote the quality of life of psoriatic patients, we need more effective treatment strategies. Determining the specific connection of ferroptosis and PsO will facilitate targeted therapies and personalized treatment for psoriasis, provide guidance for precision medicine and achieve better therapeutic effects.
Taken together, ferroptosis has a close connection with psoriasis, and there should be more experimental data to support further investigations. Psoriasis treatment strategies based on ferroptosis research will provide great advances in the future and benefit psoriatic patients.
Author contributions
YW, JS, and XH contributed to the conception and design of the work. QZ, LY, and TL drafted the manuscript. KW prepared the figure. YW, JS, and XH substantively revised the manuscript. All authors read and approved the final manuscript.
Funding
This research was supported by the National Natural Science Foundation of China (81802504, 81872207), the Sichuan Science and Technology Bureau (2019YFS0439, 2020JDJQ0067, 2020JDRC0118, 2021YJ0564, 2022YFH0005), the Science and Technology Innovation Project of Chengdu, China (No. 2021-YF05-00225-SN), and a Sichuan Medical Association grant (No. Q19037).
Conflict of interest
The authors declare that the research was conducted in the absence of any commercial or financial relationships that could be construed as a potential conflict of interest.
Publisher’s note
All claims expressed in this article are solely those of the authors and do not necessarily represent those of their affiliated organizations, or those of the publisher, the editors and the reviewers. Any product that may be evaluated in this article, or claim that may be made by its manufacturer, is not guaranteed or endorsed by the publisher.
References
Angelova, P. R., Choi, M. L., Berezhnov, A. V., Horrocks, M. H., Hughes, C. D., De, S., et al. (2020). Alpha synuclein aggregation drives ferroptosis: An interplay of iron, calcium and lipid peroxidation. Cell Death Differ. 27, 2781–2796. doi:10.1038/s41418-020-0542-z
Armstrong, A. W., and Read, C. (2020). Pathophysiology, clinical presentation, and treatment of psoriasis: A review. Jama 323, 1945–1960. doi:10.1001/jama.2020.4006
Asano, M., Yamasaki, K., Yamauchi, T., Terui, T., and Aiba, S. (2017). Epidermal iron metabolism for iron salvage. J. Dermatol. Sci. 87, 101–109. doi:10.1016/j.jdermsci.2017.04.003
Battaglia, A. M., Chirillo, R., Aversa, I., Sacco, A., Costanzo, F., and Biamonte, F. (2020). Ferroptosis and cancer: Mitochondria meet the "iron maiden" cell death. Cells 9, 1505. doi:10.3390/cells9061505
Bebber, C. M., Muller, F., Prieto Clemente, L., Weber, J., and Von Karstedt, S. (2020). Ferroptosis in cancer cell biology. Cancers (Basel) 12, E164. doi:10.3390/cancers12010164
Benhadou, F., Mintoff, D., and Del Marmol, V. (2019). Psoriasis: Keratinocytes or immune cells - which is the trigger? Dermatology 235, 91–100. doi:10.1159/000495291
Carrasquillo, O. Y., Pabon-Cartagena, G., Falto-Aizpurua, L. A., Santiago-Vazquez, M., Cancel-Artau, K. J., Arias-Berrios, G., et al. (2020). Treatment of erythrodermic psoriasis with biologics: A systematic review. J. Am. Acad. Dermatol. 83, 151–158. doi:10.1016/j.jaad.2020.03.073
Chen, X., Kang, R., Kroemer, G., and Tang, D. (2021a). Ferroptosis in infection, inflammation, and immunity. J. Exp. Med. 218, e20210518. doi:10.1084/jem.20210518
Chen, X., Li, J., Kang, R., Klionsky, D. J., and Tang, D. (2021b). Ferroptosis: Machinery and regulation. Autophagy 17, 2054–2081. doi:10.1080/15548627.2020.1810918
Conlon, M., Poltorack, C. D., Forcina, G. C., Armenta, D. A., Mallais, M., Perez, M. A., et al. (2021). A compendium of kinetic modulatory profiles identifies ferroptosis regulators. Nat. Chem. Biol. 17, 665–674. doi:10.1038/s41589-021-00751-4
Das, U. N. (2019). Saturated fatty acids, mufas and pufas regulate ferroptosis. Cell Chem. Biol. 26, 309–311. doi:10.1016/j.chembiol.2019.03.001
Ding, Y., Chen, X., Liu, C., Ge, W., Wang, Q., Hao, X., et al. (2021). Identification of A small molecule as inducer of ferroptosis and apoptosis through ubiquitination of Gpx4 in triple negative breast cancer cells. J. Hematol. Oncol. 14, 19. doi:10.1186/s13045-020-01016-8
Dixon, S. J., Lemberg, K. M., Lamprecht, M. R., Skouta, R., Zaitsev, E. M., Gleason, C. E., et al. (2012). Ferroptosis: An iron-dependent form of nonapoptotic cell death. Cell 149, 1060–1072. doi:10.1016/j.cell.2012.03.042
Forcina, G. C., and Dixon, S. J. (2019). Gpx4 at the crossroads of lipid homeostasis and ferroptosis. Proteomics 19, E1800311. doi:10.1002/pmic.201800311
Frazer, D. M., and Anderson, G. J. (2014). The regulation of iron transport. Biofactors 40, 206–214. doi:10.1002/biof.1148
Friedmann Angeli, J. P., Miyamoto, S., and Schulze, A. (2019). Ferroptosis: The greasy side of cell death. Chem. Res. Toxicol. 32, 362–369. doi:10.1021/acs.chemrestox.8b00349
Gao, M., Monian, P., Quadri, N., Ramasamy, R., and Jiang, X. (2015). Glutaminolysis and transferrin regulate ferroptosis. Mol. Cell 59, 298–308. doi:10.1016/j.molcel.2015.06.011
Girolomoni, G., Strohal, R., Puig, L., Bachelez, H., Barker, J., Boehncke, W. H., et al. (2017). The role of il-23 and the il-23/Th 17 immune Axis in the pathogenesis and treatment of psoriasis. J. Eur. Acad. Dermatol. Venereol. 31, 1616–1626. doi:10.1111/jdv.14433
Gong, Y., Wang, N., Liu, N., and Dong, H. (2019). Lipid peroxidation and Gpx4 inhibition are common causes for myofibroblast differentiation and ferroptosis. DNA Cell Biol. 38, 725–733. doi:10.1089/dna.2018.4541
Guerrero-Hue, M., Garcia-Caballero, C., Palomino-Antolin, A., Rubio-Navarro, A., Vazquez-Carballo, C., Herencia, C., et al. (2019). Curcumin reduces renal damage associated with rhabdomyolysis by decreasing ferroptosis-mediated cell death. Faseb J. 33, 8961–8975. doi:10.1096/fj.201900077R
Gunther, C., Neumann, H., Neurath, M. F., and Becker, C. (2013). Apoptosis, necrosis and necroptosis: Cell death regulation in the intestinal epithelium. Gut 62, 1062–1071. doi:10.1136/gutjnl-2011-301364
Guo, J., Xu, B., Han, Q., Zhou, H., Xia, Y., Gong, C., et al. (2018). Ferroptosis: A novel anti-tumor action for cisplatin. Cancer Res. Treat. 50, 445–460. doi:10.4143/crt.2016.572
He, Y. J., Liu, X. Y., Xing, L., Wan, X., Chang, X., and Jiang, H. L. (2020). Fenton reaction-independent ferroptosis therapy via glutathione and iron redox couple sequentially triggered lipid peroxide generator. Biomaterials 241, 119911. doi:10.1016/j.biomaterials.2020.119911
Hong, M., Rong, J., Tao, X., and Xu, Y. (2022). The emerging role of ferroptosis in cardiovascular diseases. Front. Pharmacol. 13, 822083. doi:10.3389/fphar.2022.822083
Ingold, I., Berndt, C., Schmitt, S., Doll, S., Poschmann, G., Buday, K., et al. (2018). Selenium utilization by Gpx4 is required to prevent hydroperoxide-induced ferroptosis. Cell 172, 409–422. doi:10.1016/j.cell.2017.11.048
Iznardo, H., and Puig, L. (2021). Exploring the role of il-36 cytokines as A new target in psoriatic disease. Int. J. Mol. Sci. 22, 4344. doi:10.3390/ijms22094344
Jiang, L., Kon, N., Li, T., Wang, S. J., Su, T., Hibshoosh, H., et al. (2015). Ferroptosis as A P53-mediated activity during tumour suppression. Nature 520, 57–62. doi:10.1038/nature14344
Jiang, X., Stockwell, B. R., and Conrad, M. (2021). Ferroptosis: Mechanisms, biology and role in disease. Nat. Rev. Mol. Cell Biol. 22, 266–282. doi:10.1038/s41580-020-00324-8
Kajarabille, N., and Latunde-Dada, G. O. (2019). Programmed cell-death by ferroptosis: Antioxidants as mitigators. Int. J. Mol. Sci. 20, 4968. doi:10.3390/ijms20194968
Kanda, N., Hoashi, T., and Saeki, H. (2020). Nutrition and psoriasis. Int. J. Mol. Sci. 21, 5405. doi:10.3390/ijms21155405
Karpinska-Mirecka, A., Bartosinska, J., and Krasowska, D. (2021). The impact of hypertension, diabetes, lipid disorders, overweight/obesity and nicotine dependence on health-related quality of life and psoriasis severity in psoriatic patients receiving systemic conventional and biological treatment. Int. J. Environ. Res. Public Health 18, 13167. doi:10.3390/ijerph182413167
Kose, T., Vera-Aviles, M., Sharp, P. A., and Latunde-Dada, G. O. (2019). Curcumin and (-)- epigallocatechin-3-gallate protect murine Min6 pancreatic beta-cells against iron toxicity and erastin-induced ferroptosis. Pharm. (Basel) 12, 26. doi:10.3390/ph12010026
Kwon, M. Y., Park, E., Lee, S. J., and Chung, S. W. (2015). Heme oxygenase-1 accelerates erastin-induced ferroptotic cell death. Oncotarget 6, 24393–24403. doi:10.18632/oncotarget.5162
Lai, B., Wu, C. H., Wu, C. Y., Luo, S. F., and Lai, J. H. (2022). Ferroptosis and autoimmune diseases. Front. Immunol. 13, 916664. doi:10.3389/fimmu.2022.916664
Li, D., and Li, Y. (2020). The interaction between ferroptosis and lipid metabolism in cancer. Signal Transduct. Target. Ther. 5, 108. doi:10.1038/s41392-020-00216-5
Li, J., Cao, F., Yin, H. L., Huang, Z. J., Lin, Z. T., Mao, N., et al. (2020). Ferroptosis: Past, present and future. Cell Death Dis. 11, 88. doi:10.1038/s41419-020-2298-2
Li, P., Jiang, M., Li, K., Li, H., Zhou, Y., Xiao, X., et al. (2021). Glutathione peroxidase 4-regulated neutrophil ferroptosis induces systemic autoimmunity. Nat. Immunol. 22, 1107–1117. doi:10.1038/s41590-021-00993-3
Liang, D., Minikes, A. M., and Jiang, X. (2022). Ferroptosis at the intersection of lipid metabolism and cellular signaling. Mol. Cell 82, 2215–2227. doi:10.1016/j.molcel.2022.03.022
Liang, X., Ou, C., Zhuang, J., Li, J., Zhang, F., Zhong, Y., et al. (2021). Interplay between skin microbiota dysbiosis and the host immune system in psoriasis: Potential pathogenesis. Front. Immunol. 12, 764384. doi:10.3389/fimmu.2021.764384
Lin, L., Wang, S., Deng, H., Yang, W., Rao, L., Tian, R., et al. (2020). Endogenous labile iron pool-mediated free radical generation for cancer chemodynamic therapy. J. Am. Chem. Soc. 142, 15320–15330. doi:10.1021/jacs.0c05604
Liu, P., Feng, Y., Li, H., Chen, X., Wang, G., Xu, S., et al. (2020). Ferrostatin-1 alleviates lipopolysaccharide-induced acute lung injury via inhibiting ferroptosis. Cell. Mol. Biol. Lett. 25, 10. doi:10.1186/s11658-020-00205-0
Liu, Y., and Gu, W. (2022). P53 in ferroptosis regulation: The new weapon for the old guardian. Cell Death Differ. 29, 895–910. doi:10.1038/s41418-022-00943-y
Liu, Y., and Gu, W. (2021). The complexity of P53-mediated metabolic regulation in tumor suppression. Semin. Cancer Biol.. doi:10.1016/j.semcancer.2021.03.010
Liu, Y., Tavana, O., and Gu, W. (2019). P53 modifications: Exquisite decorations of the powerful guardian. J. Mol. Cell Biol. 11, 564–577. doi:10.1093/jmcb/mjz060
Lo, Y., Chiu, H. Y., and Tsai, T. F. (2020). Clinical features and genetic polymorphism in Chinese patients with erythrodermic psoriasis in A single dermatologic clinic. Mol. Diagn. Ther. 24, 85–93. doi:10.1007/s40291-019-00441-x
Ly, K., Smith, M. P., Thibodeaux, Q., Reddy, V., Liao, W., and Bhutani, T. (2019). Anti il-17 in psoriasis. Expert Rev. Clin. Immunol. 15, 1185–1194. doi:10.1080/1744666X.2020.1679625
Ma, S., Henson, E. S., Chen, Y., and Gibson, S. B. (2016). Ferroptosis is induced following siramesine and lapatinib treatment of breast cancer cells. Cell Death Dis. 7, E2307. doi:10.1038/cddis.2016.208
Madden, S. K., Flanagan, K. L., and Jones, G. (2020). How lifestyle factors and their associated pathogenetic mechanisms impact psoriasis. Clin. Nutr. 39, 1026–1040. doi:10.1016/j.clnu.2019.05.006
Magtanong, L., Ko, P. J., To, M., Cao, J. Y., Forcina, G. C., Tarangelo, A., et al. (2019). Exogenous monounsaturated fatty acids promote A ferroptosis-resistant cell state. Cell Chem. Biol. 26, 420–432. doi:10.1016/j.chembiol.2018.11.016
Masaldan, S., Bush, A. I., Devos, D., Rolland, A. S., and Moreau, C. (2019). Striking while the iron is hot: Iron metabolism and ferroptosis in neurodegeneration. Free Radic. Biol. Med. 133, 221–233. doi:10.1016/j.freeradbiomed.2018.09.033
Miao, Y., Chen, Y., Xue, F., Liu, K., Zhu, B., Gao, J., et al. (2022). Contribution of ferroptosis and Gpx4's dual functions to osteoarthritis progression. Ebiomedicine 76, 103847. doi:10.1016/j.ebiom.2022.103847
Miotto, G., Rossetto, M., Di Paolo, M. L., Orian, L., Venerando, R., Roveri, A., et al. (2020). Insight into the mechanism of ferroptosis inhibition by ferrostatin-1. Redox Biol. 28, 101328. doi:10.1016/j.redox.2019.101328
Nast, A., Smith, C., Spuls, P. I., Avila Valle, G., Bata-Csorgo, Z., Boonen, H., et al. (2020). Euroguiderm guideline on the systemic treatment of psoriasis vulgaris - Part 1: Treatment and monitoring recommendations. J. Eur. Acad. Dermatol. Venereol. 34, 2461–2498. doi:10.1111/jdv.16915
Navarini, A. A., Burden, A. D., Capon, F., Mrowietz, U., Puig, L., Koks, S., et al. (2017). European consensus statement on phenotypes of pustular psoriasis. J. Eur. Acad. Dermatol. Venereol. 31, 1792–1799. doi:10.1111/jdv.14386
Nowowiejska, J., Baran, A., and Flisiak, I. (2021). Aberrations in lipid expression and metabolism in psoriasis. Int. J. Mol. Sci. 22, 6561. doi:10.3390/ijms22126561
Ogawa, K., and Okada, Y. (2020). The current landscape of psoriasis genetics in 2020. J. Dermatol. Sci. 99, 2–8. doi:10.1016/j.jdermsci.2020.05.008
Orsmond, A., Bereza-Malcolm, L., Lynch, T., March, L., and Xue, M. (2021). Skin barrier dysregulation in psoriasis. Int. J. Mol. Sci. 22, 10841. doi:10.3390/ijms221910841
Park, E., and Chung, S. W. (2019). Ros-mediated autophagy increases intracellular iron levels and ferroptosis by ferritin and transferrin receptor regulation. Cell Death Dis. 10, 822. doi:10.1038/s41419-019-2064-5
Pezzolo, E., and Naldi, L. (2019). The relationship between smoking, psoriasis and psoriatic arthritis. Expert Rev. Clin. Immunol. 15, 41–48. doi:10.1080/1744666X.2019.1543591
Proneth, B., and Conrad, M. (2019). Ferroptosis and necroinflammation, A yet poorly explored link. Cell Death Differ. 26, 14–24. doi:10.1038/s41418-018-0173-9
Qiu, Y., Cao, Y., Cao, W., Jia, Y., and Lu, N. (2020). The application of ferroptosis in diseases. Pharmacol. Res. 159, 104919. doi:10.1016/j.phrs.2020.104919
Raychaudhuri, S. K., Maverakis, E., and Raychaudhuri, S. P. (2014). Diagnosis and classification of psoriasis. Autoimmun. Rev. 13, 490–495. doi:10.1016/j.autrev.2014.01.008
Raychaudhuri, S. K., and Raychaudhuri, S. P. (2014). Mtor signaling cascade in psoriatic disease: Double kinase mtor inhibitor A novel therapeutic target. Indian J. dermatol. 59, 67–70. doi:10.4103/0019-5154.123499
Reichert, C. O., De Freitas, F. A., Sampaio-Silva, J., Rokita-Rosa, L., Barros, P. L., Levy, D., et al. (2020). Ferroptosis mechanisms involved in neurodegenerative diseases. Int. J. Mol. Sci. 21, 8765. doi:10.3390/ijms21228765
Reid, C., and Griffiths, C. E. M. (2020). Psoriasis and treatment: Past, present and future aspects. Acta Derm. Venereol. 100, Adv00032. doi:10.2340/00015555-3386
Ren, J. X., Sun, X., Yan, X. L., Guo, Z. N., and Yang, Y. (2020). Ferroptosis in neurological diseases. Front. Cell. Neurosci. 14, 218. doi:10.3389/fncel.2020.00218
Rendon, A., and Schakel, K. (2019). Psoriasis pathogenesis and treatment. Int. J. Mol. Sci. 20, 1475. doi:10.3390/ijms20061475
Reolid, A., Munoz-Aceituno, E., Abad-Santos, F., Ovejero-Benito, M. C., and Dauden, E. (2021). Epigenetics in non-tumor immune-mediated skin diseases. Mol. Diagn. Ther. 25, 137–161. doi:10.1007/s40291-020-00507-1
Riegman, M., Sagie, L., Galed, C., Levin, T., Steinberg, N., Dixon, S. J., et al. (2020). Ferroptosis occurs through an osmotic mechanism and propagates independently of cell rupture. Nat. Cell Biol. 22, 1042–1048. doi:10.1038/s41556-020-0565-1
Seibt, T. M., Proneth, B., and Conrad, M. (2019). Role of Gpx4 in ferroptosis and its pharmacological implication. Free Radic. Biol. Med. 133, 144–152. doi:10.1016/j.freeradbiomed.2018.09.014
Serwin, A. B., Wasowicz, W., Gromadzinska, J., and Chodynicka, B. O. (2003). Selenium status in psoriasis and its relations to the duration and severity of the disease. Nutrition 19, 301–304. doi:10.1016/s0899-9007(02)01081-x
Shah, R., Shchepinov, M. S., and Pratt, D. A. (2018). Resolving the role of lipoxygenases in the initiation and execution of ferroptosis. ACS Cent. Sci. 4, 387–396. doi:10.1021/acscentsci.7b00589
Shen, Z., Liu, T., Li, Y., Lau, J., Yang, Z., Fan, W., et al. (2018). Fenton-reaction-acceleratable magnetic nanoparticles for ferroptosis therapy of orthotopic brain tumors. Acs Nano 12, 11355–11365. doi:10.1021/acsnano.8b06201
Shou, Y., Yang, L., Yang, Y., and Xu, J. (2021). Inhibition of keratinocyte ferroptosis suppresses psoriatic inflammation. Cell Death Dis. 12, 1009. doi:10.1038/s41419-021-04284-5
Solmaz, D., Bakirci, S., Kimyon, G., Gunal, E. K., Dogru, A., Bayindir, O., et al. (2020). Impact of having family history of psoriasis or psoriatic arthritis on psoriatic disease. Arthritis Care Res. 72, 63–68. doi:10.1002/acr.23836
Stockwell, B. R. (2019). A powerful cell-protection system prevents cell death by ferroptosis. Nature 575, 597–598. doi:10.1038/d41586-019-03145-8
Stockwell, B. R. (2022). Ferroptosis turns 10: Emerging mechanisms, physiological functions, and therapeutic applications. Cell 185, 2401–2421. doi:10.1016/j.cell.2022.06.003
Stockwell, B. R., Friedmann Angeli, J. P., Bayir, H., Bush, A. I., Conrad, M., Dixon, S. J., et al. (2017). Ferroptosis: A regulated cell death nexus linking metabolism, redox biology, and disease. Cell 171, 273–285. doi:10.1016/j.cell.2017.09.021
Stockwell, B. R., Jiang, X., and Gu, W. (2020). Emerging mechanisms and disease relevance of ferroptosis. Trends Cell Biol. 30, 478–490. doi:10.1016/j.tcb.2020.02.009
Sun, X., Ou, Z., Xie, M., Kang, R., Fan, Y., Niu, X., et al. (2015). Hspb1 as A novel regulator of ferroptotic cancer cell death. Oncogene 34, 5617–5625. doi:10.1038/onc.2015.32
Traks, T., Keermann, M., Prans, E., Karelson, M., Loite, U., Koks, G., et al. (2019). Polymorphisms in Il36g gene are associated with plaque psoriasis. BMC Med. Genet. 20, 10. doi:10.1186/s12881-018-0742-2
Tsurusaki, S., Tsuchiya, Y., Koumura, T., Nakasone, M., Sakamoto, T., Matsuoka, M., et al. (2019). Hepatic ferroptosis plays an important role as the trigger for initiating inflammation in nonalcoholic steatohepatitis. Cell Death Dis. 10, 449. doi:10.1038/s41419-019-1678-y
Ursini, F., and Maiorino, M. (2020). Lipid peroxidation and ferroptosis: The role of gsh and Gpx4. Free Radic. Biol. Med. 152, 175–185. doi:10.1016/j.freeradbiomed.2020.02.027
Van De Kerkhof, P. C. (2022). From empirical to pathogenesis-based treatments for psoriasis. J. Invest.. Dermatol. 142, 1778–1785. doi:10.1016/j.jid.2022.01.014
Van Meer, G., Voelker, D. R., and Feigenson, G. W. (2008). Membrane lipids: Where they are and how they behave. Nat. Rev. Mol. Cell Biol. 9, 112–124. doi:10.1038/nrm2330
Vicic, M., Kastelan, M., Brajac, I., Sotosek, V., and Massari, L. P. (2021). Current concepts of psoriasis immunopathogenesis. Int. J. Mol. Sci. 22, 11574. doi:10.3390/ijms222111574
Weber, B., Merola, J. F., Husni, M. E., Di Carli, M., Berger, J. S., and Garshick, M. S. (2021). Psoriasis and cardiovascular disease: Novel mechanisms and evolving therapeutics. Curr. Atheroscler. Rep. 23, 67. doi:10.1007/s11883-021-00963-y
Wen, Q., Liu, J., Kang, R., Zhou, B., and Tang, D. (2019). The release and activity of Hmgb1 in ferroptosis. Biochem. Biophys. Res. Commun. 510, 278–283. doi:10.1016/j.bbrc.2019.01.090
Wu, J., Xue, R., Wu, M., Yin, X., Xie, B., and Meng, Q. (2022). Nrf2-Mediated ferroptosis inhibition exerts A protective effect on acute-on-chronic liver failure. Oxid. Med. Cell. Longev. 2022, 4505513. doi:10.1155/2022/4505513
Xia, J., Si, H., Yao, W., Li, C., Yang, G., Tian, Y., et al. (2021). Research progress on the mechanism of ferroptosis and its clinical application. Exp. Cell Res. 409, 112932. doi:10.1016/j.yexcr.2021.112932
Xie, Y., Song, X., Sun, X., Huang, J., Zhong, M., Lotze, M. T., et al. (2016). Identification of baicalein as A ferroptosis inhibitor by natural product library screening. Biochem. Biophys. Res. Commun. 473, 775–780. doi:10.1016/j.bbrc.2016.03.052
Xu, X., and Zhang, H. Y. (2017). The immunogenetics of psoriasis and implications for drug repositioning. Int. J. Mol. Sci. 18, 2650. doi:10.3390/ijms18122650
Yang, L., Wang, H., Yang, X., Wu, Q., An, P., Jin, X., et al. (2020). Auranofin mitigates systemic iron overload and induces ferroptosis via distinct mechanisms. Signal Transduct. Target. Ther. 5, 138. doi:10.1038/s41392-020-00253-0
Yang, W. S., Sriramaratnam, R., Welsch, M. E., Shimada, K., Skouta, R., Viswanathan, V. S., et al. (2014). Regulation of ferroptotic cancer cell death by Gpx4. Cell 156, 317–331. doi:10.1016/j.cell.2013.12.010
Yao, X., Zhang, Y., Hao, J., Duan, H. Q., Zhao, C. X., Sun, C., et al. (2019). Deferoxamine promotes recovery of traumatic spinal cord injury by inhibiting ferroptosis. Neural Regen. Res. 14, 532–541. doi:10.4103/1673-5374.245480
Yuan, H., Li, X., Zhang, X., Kang, R., and Tang, D. (2016). Identification of Acsl4 as A biomarker and contributor of ferroptosis. Biochem. Biophys. Res. Commun. 478, 1338–1343. doi:10.1016/j.bbrc.2016.08.124
Zhang, X., Guo, Y., Li, H., and Han, L. (2021a). Fin56, A novel ferroptosis inducer, triggers lysosomal membrane permeabilization in A tfeb-dependent manner in glioblastoma. J. Cancer 12, 6610–6619. doi:10.7150/jca.58500
Zhang, Y., Swanda, R. V., Nie, L., Liu, X., Wang, C., Lee, H., et al. (2021b). Mtorc1 couples cyst(E)ine availability with Gpx4 protein synthesis and ferroptosis regulation. Nat. Commun. 12, 1589. doi:10.1038/s41467-021-21841-w
Zhao, Y., Li, Y., Zhang, R., Wang, F., Wang, T., and Jiao, Y. (2020). The role of erastin in ferroptosis and its prospects in cancer therapy. Onco. Targets. Ther. 13, 5429–5441. doi:10.2147/OTT.S254995
Zheng, J., and Conrad, M. (2020). The metabolic underpinnings of ferroptosis. Cell Metab. 32, 920–937. doi:10.1016/j.cmet.2020.10.011
Zhou, S., and Yao, Z. (2022). Roles of infection in psoriasis. Int. J. Mol. Sci. 23, 6955. doi:10.3390/ijms23136955
Keywords: psoriasis, ferroptosis, lipid metabolism, ferrostatin-1, treatment
Citation: Zhou Q, Yang L, Li T, Wang K, Huang X, Shi J and Wang Y (2022) Mechanisms and inhibitors of ferroptosis in psoriasis. Front. Mol. Biosci. 9:1019447. doi: 10.3389/fmolb.2022.1019447
Received: 15 August 2022; Accepted: 02 September 2022;
Published: 15 September 2022.
Edited by:
Xin Wang, National Institutes of Health (NIH), United StatesReviewed by:
Jingquan He, Shenzhen Traditional Chinese Medicine Hospital, ChinaSi-Yuan Song, Baylor College of Medicine, United States
Copyright © 2022 Zhou, Yang, Li, Wang, Huang, Shi and Wang. This is an open-access article distributed under the terms of the Creative Commons Attribution License (CC BY). The use, distribution or reproduction in other forums is permitted, provided the original author(s) and the copyright owner(s) are credited and that the original publication in this journal is cited, in accordance with accepted academic practice. No use, distribution or reproduction is permitted which does not comply with these terms.
*Correspondence: Xiaobo Huang, drhuangxb@163.com; Jingfen Shi, 963725462@qq.com; Yi Wang, w_yi2022@163.com
†These authors share first authorship