- 1Centre for Experimental Pathogen Host Research, University College Dublin, Dublin, Ireland
- 2Department of Infectious Diseases, St Vincent’s University Hospital, Dublin, Ireland
The emergence of persistent ill-health in the aftermath of SARS-CoV-2 infection has presented significant challenges to patients, healthcare workers and researchers. Termed long COVID, or post-acute sequelae of COVID-19 (PASC), the symptoms of this condition are highly variable and span multiple body systems. The underlying pathophysiology remains poorly understood, with no therapeutic agents proven to be effective. This narrative review describes predominant clinical features and phenotypes of long COVID alongside the data supporting potential pathogenesis of these phenotypes including ongoing immune dysregulation, viral persistence, endotheliopathy, gastrointestinal microbiome disturbance, autoimmunity, and dysautonomia. Finally, we describe current potential therapies under investigation, as well as future potential therapeutic options based on the proposed pathogenesis research.
Introduction
Identification of long COVID as clinical syndrome
The clinical spectrum of COVID-19, the disease caused by severe acute respiratory syndrome coronavirus 2 (SARS-CoV-2) infection, has been extensively characterised (Huang et al., 2020a; Xu et al., 2020). The majority of affected individuals experience a mild or moderate disease phenotype, with fewer than 5% developing critical illness. In the aftermath of the first wave of infection, there were increasing reports of persistent ill-health beyond resolution of acute infection. These ongoing symptoms were protean, with fatigue, cognitive dysfunction, and respiratory symptoms most commonly reported (Carfì et al., 2020; Townsend et al., 2020). Up to one-fifth of those who have ever had COVID report symptoms of long COVID (USA DoHaHS, 2022). Many experience disabling symptoms, with 44% of those with long COVID in one survey unable to return to work (Cutler, 2022) Post-viral conditions following other infections have been previously described. Indeed, the assessment of the long-term consequences of the severe acute respiratory syndrome (SARS) epidemic (caused by the novel coronavirus SARS-CoV) found that a subset (51%) of those affected in Toronto experienced persistent fatigue, diffuse myalgias, weakness and depression 1 year after their acute illness and could not return to work (Moldofsky and Patcai, 2011). In a similar follow-up study amongst 233 SARS survivors in Hong Kong, over 40% of respondents reported ongoing fatigue 40 months after infection (Lam et al., 2009). In those affected by the subsequent Middle-Eastern Respiratory Syndrome Coronavirus (MERS-CoV) outbreak in Korea, prolonged symptoms and fatigue were reported up to 18 months after acute infection (Lee et al., 2019). However, no pathological mechanism underlying these symptoms has been described.
Post-infectious fatigue syndromes have been reported following a myriad of infections other than coronaviruses, including Epstein-Barr Virus (EBV), Q-Fever and Ross River Virus (RRV) infections, as well as rickettsiosis (Hickie et al., 2006). Similar to post-coronavirus illnesses, no definite mechanism has been identified for the development of these syndromes. An area of prior focus in these conditions has been the autonomic system, with post-infectious dysautonomia proposed as an underlying mechanism to explain commonly reported post-infectious symptoms such as abnormal heart rate and blood pressure responses to physiological stressors, most notably postural orthostatic tachycardia syndrome (POTS). Interestingly, prior studies in the area of chronic fatigue syndrome/myalgic encephalomyelitis (CFS/ME) have also shown a variety of changes in autonomic function, but a clear unifying pathological basis to explain the emergence of dysautonomia post infection is lacking (Newton et al., 2007).
A common stumbling block for mechanistic studies into post-infectious fatigue and reduced exercise tolerance is an absence of clear timing of onset from the purported triggering infection. The COVID-19 pandemic has provided an opportunity to study physical sequalae and their underlying mechanisms following acute viral infection in a setting seldom found: a large number of individuals with confirmed infection by a known virus at a known timepoint. Another challenge lies in how to appropriately characterise long COVID, given its heterogenous nature and the wide number of symptoms reported. There are various terms and clinical definitions from different guidance bodies used to classify the constellation of symptoms and incident conditions that occur following acute COVID-19, including ‘post COVID-19 syndrome’, ‘post COVID-19 condition’ and ‘post-acute sequelae of COVID-19’ (PASC) (Venkatesan, 2021; WHO, 2021; Centers for Disease Control and Prevention, 2022). Long COVID is the term coined by those who are experiencing this condition, and more recently the CDC have incorporated this label as the working definition in its National Research Action Plan. This working definition defines long COVID as signs, symptoms or conditions that develop after SARS-CoV-2 infection and are present four or weeks after the initial infection (USA DoHaHS, 2022). However there is heterogeneity in definitions used in studies, with some requiring symptoms to persist for eight or 12 weeks (Sudre et al., 2021; Subramanian et al., 2022). In this review we outline the range of symptoms encompassed by this condition, the leading hypotheses of the pathophysiologic basis for this condition, research gaps and future directions.
Clinical spectrum of long COVID
Long COVID phenotypes
Symptoms of long COVID span multiple physiological domains, with more than 100 different symptoms reported (Subramanian et al., 2022), examples of which are shown in Figure 1. Given the heterogeneity in post COVID sequelae and the breadth of symptoms reported, a major challenge in studying long COVID is characterising distinct phenotypes that can then assist with the selection of appropriate cohorts of individuals and controls to include in translational studies or therapeutic trials of specific agents. This is particularly challenging in the large cohort of individuals who, despite debilitating symptoms, have normal routine clinical investigations (Sneller et al., 2022). Further complicating the matter, older individuals who were hospitalised during the acute phase of the COVID-19 illness are overrepresented in many studies to date (Littlefield et al., 2022; Su et al., 2022), making it difficult to untangle abnormalities expected to occur after a severe systemic illness, which might disproportionately affect older adults, from those directly related to SARS-CoV-2. Additionally, with the exception of smell and taste disorders, the most commonly reported long COVID symptoms are all relatively frequent in the general population (Subramanian et al., 2022), and a relapsing and remitting pattern of symptoms is common (Davis et al., 2021). A number of studies have attempted to overcome the heterogeneity of this condition by correlating individual immune abnormalities with specific symptoms, but such multiple comparisons increases the risk of chance findings and potentially erroneous conclusions.
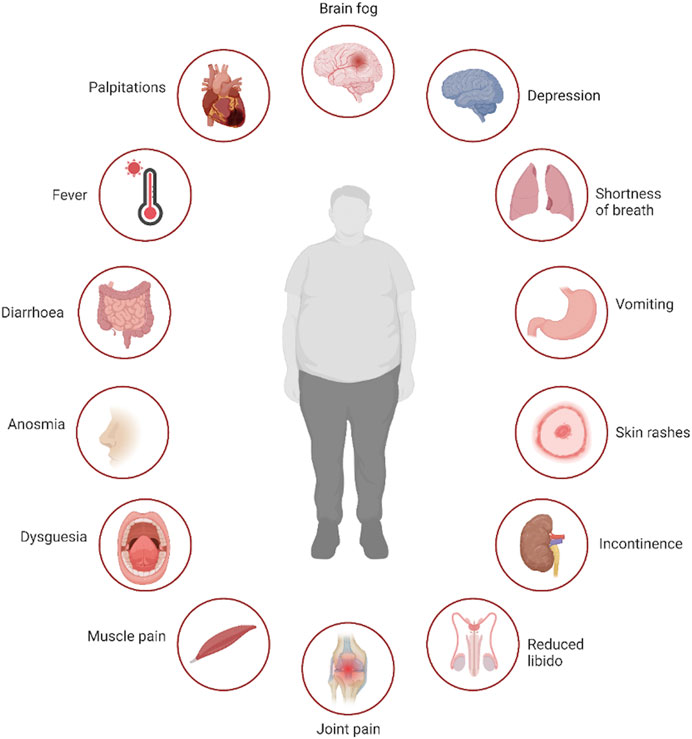
FIGURE 1. Multisystem symptoms associated with long COVID. Legend: Selection of symptoms reported by patients suffering with long COVID, demonstrating the multi-system nature of the disease. Created with BioRender.com.
Identifying distinct long COVID clinical phenotypes or syndromes that may have unique underlying pathophysiologic mechanisms (Figure 2) has been the focus of a number of studies. Some have proposed a manual subgrouping of symptom profiles or grading of symptom severity, while others have used unsupervised statistical methods to identify data driven phenotypes appropriate for this novel condition. While these studies differ in terms of cohorts, data collection methods, symptoms included and analytic approaches, four common themes across studies are emerging.
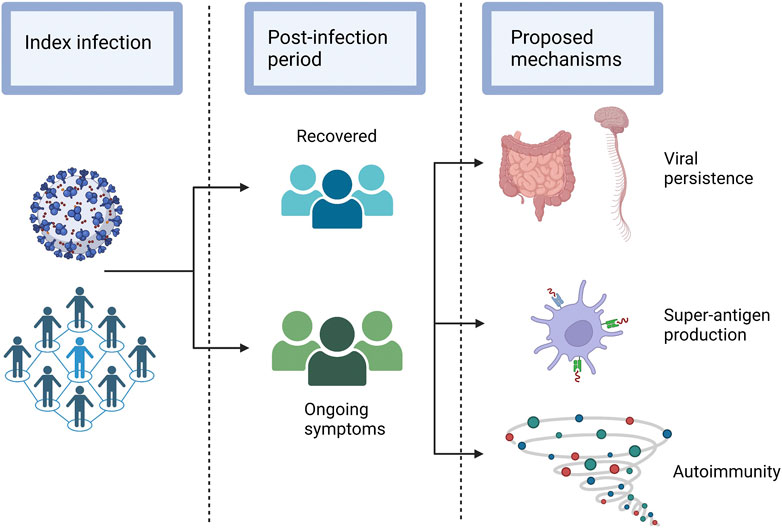
FIGURE 2. Hypothesised sequelae of SARS-CoV-2 that lead to long COVID. Legend: A selection of the possible sequelae of SARS-CoV-2 that may lead to the various post-acute pathologies that occur in a subset of individuals.
The phenotype most commonly reported across studies using unsupervised clustering is a cardiorespiratory phenotype (Kenny et al., 2022a; Zhang et al., 2022a; Canas et al., 2022; Caspersen et al., 2022; Danesh et al., 2022; Frontera et al., 2022; Sahanic et al., 2022; Whitaker et al., 2022; Reese et al., 2023), with breathlessness reported as a predominant symptom, while cough, chest pain and palpitations are reported to a varying degree. Similarly, a number of studies have identified a cluster with a high number of symptoms, including pain and musculoskeletal symptoms such as joint pain and myalgia (Kenny et al., 2022a; Zhang et al., 2022a; Canas et al., 2022; Fernández-de-Las-Peñas et al., 2022; Frontera et al., 2022; Sahanic et al., 2022; Ziauddeen et al., 2022; Reese et al., 2023). A cluster with a lower number of overall symptoms and either predominant anosmia or no characteristic symptoms has been reported in four studies (Kenny et al., 2022a; Fernández-de-Las-Peñas et al., 2022; Frontera et al., 2022; Whitaker et al., 2022), and finally a neuropsychiatric cluster with symptoms such as brain fog, depression and insomnia has also been reported in four individual studies (Canas et al., 2022; Caspersen et al., 2022; Danesh et al., 2022; Reese et al., 2023). In electronic health record based studies, laboratory abnormalities tend to cluster together (Zhang et al., 2022a; Reese et al., 2023). Whether these emerging phenotypes are impacted by elements such as vaccination and infecting variant is less well studied, but early evidence suggests infecting variant may affect clinical phenotype, while vaccination seems to play a less important role (Kenny et al., 2022b; Canas et al., 2022). Similarly, patients may transition across phenotypes over the course of their long COVID illness (Davis et al., 2021). A summary of studies using unsupervised clustering of long COVID phenotypes is shown in 1. While this phenotyping approach to studying long COVID is becoming increasingly common, there is also a large body of work looking at organ-specific symptoms and their possible underlying pathologic mechanisms. Table 1.
Organ specific sequelae of COVID-19
Common organ specific symptoms and sequelae of long COVID are summarised in Table 2. Severe COVID-19 is characterised by pneumonitis leading to acute respiratory distress syndrome (ARDS). As in other aetiologies of ARDS, direct pulmonary injury can lead to fibrosis or persistent inflammatory changes on lung imaging in the post-acute period, and abnormal pulmonary function in terms of restrictive patterns on spirometry or impaired diffusion capacity (Gao et al., 2021; Vargas Centanaro et al., 2022). In a subset of individuals, severity of pulmonary fibrosis post COVID-19 may necessitate lung transplant (Roach et al., 2022). In addition to fibrotic changes, COVID-19 may also result in damage to the pulmonary vasculature even in the absence of parenchymal abnormalities. These include pulmonary embolism-like perfusion defects, or disseminated patchy perfusion defects, that may reflect a widespread angiopathy (Remy-Jardin et al., 2021). Pulmonary embolism or angiopathy can lead to chronic thromboembolic pulmonary hypertension in some cases (Cueto-Robledo et al., 2022). However, abnormal respiratory radiological or functional findings are predominantly seen in survivors of severe acute COVID-19, while pulmonary function tests and routine imaging are often normal in those with a mild initial illness (Alba et al., 2021; Guler et al., 2021). Despite these normal findings, dyspnoea remains one of the most commonly reported long COVID symptoms across the spectrum of disease severity, whether measured by self-report, physician assessment, or increase in prescription of medications for dyspnoea such as bronchodilators in those with previous confirmed SARS-CoV-2 (Lund et al., 2021; Ziauddeen et al., 2022).
Cardiac complications of SARS-CoV-2 infection have been noted since the beginning of the pandemic, with elevations of biomarkers of cardiac injury noted in an initial case series from Wuhan, China (Huang et al., 2020a). Since then a much broader array of long term cardiac complications have been observed. A case control study from the Veteran’s Affairs healthcare service in the United States of America found increased risks of ischaemic heart disease, pericarditis, myocarditis, arrhythmias and heart failure in the 30 days to 12 month period post COVID-19 compared to matched controls (Xie et al., 2022). Additionally, chest pain, palpitations and objective tachycardia are often reported (Estiri et al., 2021), and in one study, individuals recovering from COVID-19 were noted to have a relative tachycardia for a median of 79 days post symptom onset (Radin et al., 2021).
Musculoskeletal manifestations of long COVID include myalgia, joint pain and muscle weakness. Individuals admitted to the ICU may develop weakness comparable to critical illness myopathy from other causes, which has been associated with persistent impairments out to 5 years (Herridge et al., 2011; Soares et al., 2022). Female sex and corticosteroid use during acute illness have also been associated with muscle weakness up to 1 year after infection (Huang et al., 2021a). Muscle weakness has been described in those with a mild initial illness (Stoffels et al., 2022), but whether this reflects more than just deconditioning or muscle disuse has not been determined. The underlying cause and pathogenesis of muscle weakness is poorly understood, with viral myositis, ongoing inflammation, and deconditioning all being proposed.
New gastrointestinal symptoms including diarrhoea, constipation, vomiting, abdominal pain and heartburn have been reported in individuals recovering from COVID-19 (Blackett et al., 2022), and presence of these symptoms does not necessarily correlate with having had these symptoms acutely (Weng et al., 2021). Liver enzymes have been reported to be elevated in 25% of people hospitalised with COVID-19 at 1 month follow-up post-discharge, although these elevations are usually transient in the absence of concomitant liver pathology (Liao et al., 2022).
There is an excess risk of both incident diabetes and hyperglycemia in the 12 month period post COVID-19, with the degree of risk correlating to the severity of acute illness (Xie and Al-Aly, 2022). Elevated risks have been shown for both type 1 and type 2 diabetes, and a greater risk with COVID-19 than with other upper respiratory tract infections (Zhang et al., 2022b).
A registry of dermatologic findings in COVID-19 found skin rashes occurred for various durations, with morbiliform, urticarial and papulosquamous eruptions relatively short lived, while pernio was more frequently associated with a duration of >60 days (McMahon et al., 2021). Other manifestations including pustular dermatoses (Goyal et al., 2022) have been reported. Hair loss has been reported in 22% of hospitalised individuals at follow-up, regardless of acute oxygen requirement (Huang et al., 2021b). Telogen effluvium, a diffuse non-scarring hair loss disorder which typically lasts <6 months and is associated with stress, hormonal changes or medications (Hughes and Saleh, 2022) are the most common suspected aetiologies underlying hair loss, but alopecia areata and other forms of alopecia have also been reported (Chularojanamontri et al., 2022).
Neuropsychiatric complications in the months following SARS-CoV-2 infection have been frequently reported. Anosmia, dysosmia and taste disorders which are characteristic features of acute COVID-19, may persist for months after the initial infection (Zayet et al., 2021; Tan et al., 2022). Severity of initial infection is most strongly associated with increased risk of new neurological diagnoses, but higher incidences of neurological sequelae are reported across all categories of initial COVID-19 disease severity. Cerebrovascular events, including intracranial haemorrhage and ischaemic stroke, are increased in the post-infectious convalescence period, with the most marked risk in those with encephalopathy at the time of initial infection (Taquet et al., 2021). Cognitive dysfunction is a major long COVID symptom, and objective cognitive impairment has been found even in those without subjective impairment (Ceban et al., 2022). Interestingly, longitudinal follow-up has shown an increased incidence of both anxiety and depression at 12 months compared to 6 months from the initial diagnosis (Huang et al., 2021a). Additionally, there is increased prescribing of anxiolytic and anti-depressant agents, as well as analgesics, in the 6 months following a diagnosis of COVID-19 (Al-Aly et al., 2021). Although the association between initial disease severity and subsequent psychiatric diagnoses is less clear than with neurological disorders, the underlying pathogenesis remains unclear. Postulated mechanisms include direct viral cytotoxicity in the central nervous system as well as altered coagulation. It is likely that there are other contributors, especially to the increase in reported psychiatric symptoms, including consequences of living under pandemic restrictions, income security, and the psychological and psychiatric impact of ongoing, and in some cases debilitating physical ill-health.
Proposed mechanisms of long COVID
There are multiple proposed pathogenic mechanisms that may contribute to the development of long COVID. These are summarised in Figure 3 and explained in greater detail below.
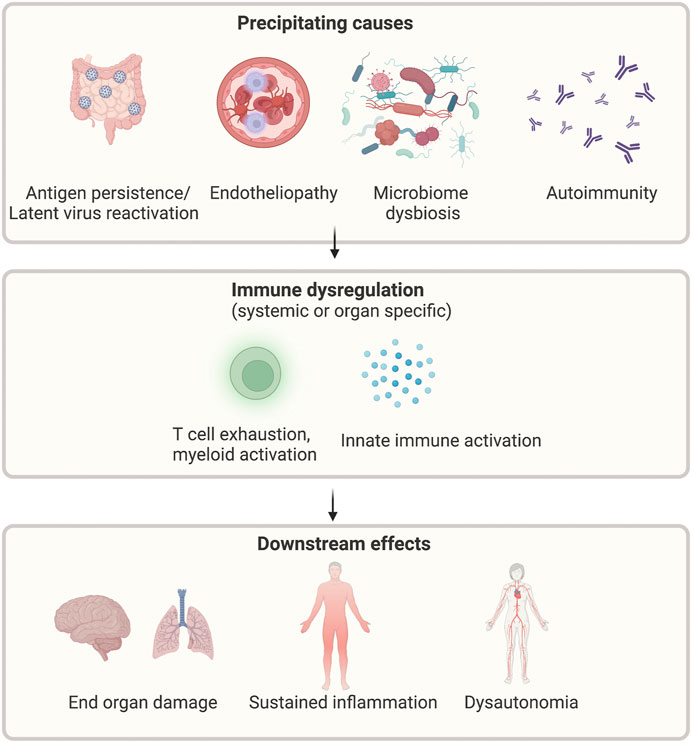
FIGURE 3. Proposed mechanisms of long COVID. Legend: Multiple possible precipitants, including persistent virus, reactivation of latent viruses, altered microbiome, endothelial dysfunction, and autoimmunity, may lead to ongoing immune activation and subsequent systemic symptoms. Created with BioRender.com.
Immune dysregulation
A small number of studies have examined changes in immune profiles or function following other acute viral infections. Expansion of activated CD8+ T cells have been reported in the setting of parvovirus B19 infection, as well as in the aftermath of severe influenza A H7N9 infection (Isa et al., 2005; Zhao et al., 2018). There is also increasing evidence of quantitative and qualitative changes in immune populations after SARS-CoV-2 infection. A prolonged period of T cell activation is seen in individuals, both hospitalised and non-hospitalised, after resolution of initial SARS-CoV-2 infection, with increased expression of the T cell exhaustion markers PD-L1 and TIGIT (Files et al., 2021). The persistent expansion of activated T cells appears most marked in older individuals (Townsend et al., 2022), which may in part be due to age-related immunosenescence and/or poorer T cell cross-reactivity between different coronaviruses in older individuals (Saletti et al., 2020). There is a similar reduction in naïve B cell numbers in convalescent samples from individuals with long-COVID-19 compared to recovered individuals or healthy controls (Phetsouphanh et al., 2022). Interestingly, blockade of PD-1 ex vivo can reverse the exhausted T cell state and restored T cell function, with increased responsiveness upon exposure to SARS-CoV-2 peptides (Loretelli et al., 2021). T cell exhaustion is demonstrated 1 year after infection (Taeschler et al., 2022). The mechanisms driving loss of T cell function post COVID-19, and how or if they contribute to the development of long COVID remains unclear. It may in part be due to the large number of viral-specific T cells that are produced during acute infection, especially in those with prolonged viral shedding (Moderbacher et al., 2020).
The immunological abnormalities seen following SARS-CoV-2 are not limited to lymphoid populations. There is evidence of increased innate cell activation, with myeloid cells displaying an activated phenotype (HLADR+CD38+), as well as production of type I and type III interferons. These abnormalities are most pronounced in patients with long COVID (Phetsouphanh et al., 2022). Persistent interferon abnormalities are perhaps not surprising, given the key role played by dysfunctional interferon responses in the development of severe acute COVID-19 (Bastard et al., 2021; Smith et al., 2022). Additional cytokines including IL-6, TNFα and IP-10 have also been shown to be elevated in long COVID (Peluso et al., 2021). Individuals with neurological features of long COVID, in particular brain fog, may have distinct circulating cytokines, including markers of blood brain barrier (BBB) disruption such as TGFβ and IL-8 when compared to those who have recovered fully from SARS-CoV-2 infection (Campbell et al., 2022). Specific inflammatory changes may be seen at the organ level. For example, individuals with persistent pulmonary parenchymal changes demonstrated both persistent upper airway type 1 interferon signalling and upregulated neutrophil associated signalling (George et al., 2022). A delayed but exaggerated interferon response in severe disease may promote neutrophil chemotaxis to lung tissue and the formation of neutrophil extracellular traps (NETs), which release proteases and cause extracellular membrane degradation, leading to fibrogenesis (George et al., 2022). A summary of the major immune changes described in long COVID are shown in Table 3. The aetiology of these immunological changes remains poorly understood, with antigen persistence, response to host tissue damage, or host factors in immune regulation all proposed as possible contributing factors.
Antibody responses to pandemic coronaviruses (SARS-CoV, SARS-CoV-2 and MERS) have been studied in detail. Most, but not all, individuals mount a measurable serologic response following infection with SARS-CoV-2 (Wajnberg et al., 2020), and while increased disease severity leads to a more robust serologic response (Huang et al., 2020b), even individuals with a mild initial illness can retain protective immunity against reinfection or development of severe disease from similar variants (Hall et al., 2021). Waning of serologic responses over time, as well as the emergence of new variants has meant that reinfection is now common, but development or recurrence of severe disease remains rare. There is ample evidence that quantitative neutralising antibody response correlates with protection against both re-infection and development of severe disease (Khoury et al., 2021) but a defined threshold of protection has yet to be determined. Similarly, vaccination has been associated with a lower risk of development of long COVID, but there are conflicting results regarding the magnitude of protection (Al-Aly et al., 2022; Antonelli et al., 2022), and it is unknown if there is a threshold of antibody that would provide protection against long COVID in the event of a breakthrough infection. Additionally, there is some evidence that vaccination in the convalescent setting may ameliorate symptoms, although reports are conflicting (Notarte et al., 2022). It is unknown why vaccination may prevent or improve long COVID symptoms. One hypothesis is that vaccination attenuates acute disease severity, and thus subsequent immune derangements and organ damage. A limitation to this hypothesis is that long COVID occurs frequently even in those with a mild initial illness (Maglietta et al., 2022). A second theory is that long COVID is due to a dysfunctional antibody response, which either fails to clear viral remnants or leads to the induction of self-reactive antibodies or proinflammatory antibodies. Vaccination prior to natural infection may prime the immune system and avoid this dysfunctional response. Findings in support of this proposed mechanism include the identification of an immunoglobulin signature in acute infection that predicts long COVID (Cervia et al., 2022), and that the antibodies induced by vaccination have different glycosylation patterns to those induced by infection (Chakraborty et al., 2022).
Viral persistence and latent virus reactivation
Unlike DNA viruses, most RNA viruses cause acute infections with a relatively short period of host infectivity and subsequent recovery and host immunity. However, full or partial viral persistence in tissues has been demonstrated after the infectious period of a number of RNA viruses, including Polio, Chikungunya, Ross River and Measles viruses, and is implicated in prolonged or late complications of infection (Griffin, 2022). The clinical pattern of relapsing and remitting symptoms seen in long COVID has led to the hypothesis that viral persistence may also be contributing to the development of this condition (Brodin et al., 2022). A potential source of viral persistence is the gut, with SARS-CoV-2 nucleocapsid protein detected in intestinal biopsies 4 months after initial infection, even in mild cases (Gaebler et al., 2021). Similarly, viral RNA can be detected in the faeces of patients post-SARS-CoV-2 infection, even in the setting of negative nasopharyngeal PCR (Natarajan et al., 2022). There have also been reports of detectable viral spike protein in blood (Swank et al., 2022), circulating monocytes (Patterson et al., 2022), lung tissue (Ceulemans et al., 2021) and urine (Tejerina et al., 2022), weeks to months after initial SARS-CoV-2 infection, though correlation with persistent symptoms or development of long COVID phenotypes is variable. Furthermore in a cohort of predominantly individuals requiring hospitalisation, SARS-CoV-2 RNAemia in acute disease was found to be associated with a higher odds of subsequent subjective long COVID memory complaints, independent of acute disease severity. While viral persistence may serve a functional role in allowing for evolution of the humoral immune response, increasing the breadth and potency of circulating anti SARS-CoV-2 IgG (Gaebler et al., 2021), it may also lead to sustained innate immune activation or demonstrate molecular mimicry with host antigens leading to the induction of autoimmunity.
Reactivation of other latent viruses, such as EBV, has also been implicated in long COVID. EBV RNAemia in acute illness is associated with subsequent long COVID fatigue, cough and memory complaints. While EBV RNAemia is generally absent in long COVID (Peluso et al., 2022a), higher levels of anti EBV antibodies that are associated with lytic infection have been demonstrated in those with long COVID compared to those without (Gold et al., 2021; Klein et al., 2022a).
Autoimmunity
Early development of neutralising antibody response after initial infection is associated with survival in acute COVID-19, but abnormal humoral responses have been implicated in the uncontrolled excessive immune activation seen in severe acute COVID-19. Multisystem Inflammatory Syndrome in Children (MIS-C), described in paediatric cases of SARS-CoV-2, is mediated by B cell autoantibody production secondary to T cell activation following exposure to superantigen, and has also been described in cases of severe acute COVID-19 in adults (Consiglio et al., 2020; Sancho-Shimizu et al., 2021). Autoantibodies against a variety of targets have been described in acute SARS-CoV-2 infection, including anti-nuclear antibodies, antibodies associated with myositis, systemic sclerosis and connective tissue diseases (Chang et al., 2021), and antibodies against various immunomodulatory proteins (Wang et al., 2021), including type I interferon autoantibodies (Bastard et al., 2020; Bastard et al., 2021). In addition, afucosylated anti spike IgG may promote excessive inflammation through recruitment of macrophages, NK cells and production of cytokines (Hoepel et al., 2021; Chakraborty et al., 2022).
Similarly, development of autoantibodies have been implicated in long COVID, though there are conflicting reports in the published literature. Autoantibodies directed towards a wide variety of targets have been reported in long COVID, including antibodies against angiotensin, ACE, and muscarinic receptors (Klein et al., 2022a; Sotzny et al., 2022; Thurner et al., 2022). Associations between autoantibody detection and specific symptoms in long COVID has not yet been demonstrated (Klein et al., 2022a). In addition, autoantibodies such as neutralising anti-interferon antibodies, generated in acute infection, may theoretically promote the development of viral persistence and therefore contribute to the development of long COVID. However, detection of these antibodies in the acute phase has been associated with persistent respiratory symptoms (Su et al., 2022), there is a low prevalence of anti-interferon antibodies in the post-acute period (Peluso et al., 2022b).
Microbiome and microbial translocation
Viral persistence within the intestinal tract may also impact the gut microbiota and contribute to dysbiosis. The gut microbiome is significantly altered in individuals with acute SARS-CoV-2 when compared with uninfected matched controls. Changes include depletion of species with immunomodulatory roles within the gut such as Faecalibacterium prausnitzii and Eubacterium rectale (Yeoh et al., 2021). Furthermore, the degree of dysbiosis was associated with both initial COVID-19 disease severity and levels of circulating inflammatory cytokines. Interestingly, gut microbial diversity remains depleted 6 months after SARS-CoV-2 infection, with those with more severe initial infection having the most marked differences (Chen et al., 2022). In a cohort of hospitalised individuals, both microbiome composition at time of hospital admission and lack of recovery of microbiome composition were associated with the development of long COVID (Liu et al., 2022). Furthermore, the dysbiosis observed in acute SARS-CoV-2 is associated with increased intestinal permeability and subsequent translocation of bacterial and fungal products into the circulation, further contributing to systemic inflammation (Giron et al., 2021; Bernard-Raichon et al., 2022). Similarly, the dysbiosis observed in the convalescent setting may also contribute to increased intestinal permeability. Zonulin, a physiologic driver of intestinal permeability, and Beta-D-glucan, a marker of fungal translocation, have both been shown to be elevated in individuals with long COVID, and positively correlate with plasma concentrations of IL-6, TNFa, IP10, and negatively correlate with quality of life scores (Giron et al., 2022).
Dysautonomia
Autonomic dysfunction has been described following multiple infections, but the underlying mechanisms remain elusive (Carod-Artal, 2018). Orthostatic intolerance occurs when an individual cannot maintain an upright position due to either sympathetic system over-activation or cerebral hypoperfusion. The typical features of dysautonomia occur as a result of catecholamine release and include tachycardia, hypotension, fatigue, palpitations, and syncope (Chen-Scarabelli and Scarabelli, 2004). There have been small studies examining the role of autonomic dysfunction in individuals with these long COVID symptoms. Tilt-table testing has demonstrated orthostatic intolerance in a small number of those affected (Jamal et al., 2022). However, post-COVID-19 fatigue has not been shown to be associated with autonomic dysfunction (Townsend et al., 2021a). Interestingly, findings of dysautonomia post-COVID-19 have been shown to correlated poorly with symptoms, but are associated with objective reduction in functional capacity on cardiopulmonary exercise testing (CPET) (Ladlow et al., 2022).
While correlation with all symptoms is poor, dysautonomia may be implicated in some individuals with a cardiorespiratory phenotype of post COVID-19. In one study that performed invasive CPET in individuals with persistent dyspnea more than 1 year post acute COVID-19 and normal pulmonary and cardiac imaging, exercise limitation was demonstrated to be due to impaired peripheral systemic oxygen extraction, rather than deconditioning or cardiopulmonary limitation (Singh et al., 2022). During exercise, production of local vasodilatory substances as well as sympathetic nervous system mediated vasoconstriction to non-exercising areas improves systemic oxygen extraction (Singh et al., 2021). In chronic fatigue syndrome, this process is impaired due to small fibre neuropathy affecting microvascular tone and causing microvascular shunting (Joseph et al., 2021). A similar mechanism of autonomic dysfunction may be at play in long COVID, given the similarities between these two conditions, but confirmatory studies are needed.
There are multiple possible aetiologies for post-COVID autonomic dysfunction. The ACE-2 receptor, which is widely expressed on endothelial cells lining blood vessels, is bound by SARS-CoV-2 (Hamming et al., 2004). Lower cortisol in individuals with long COVID compared to recovered controls suggests dysfunction of the hypothalamic-pituitary-adrenal axis (Klein et al., 2022a; Su et al., 2022). Persistent cardiac dysfunction and inflammation, which has been demonstrated in cardiac MRI studies (Puntmann et al., 2020), may also contribute.
Endotheliopathy
Acute severe SARS-CoV-2 infection is associated with a distinct coagulopathy, characterised by markedly elevated D-dimers and increased thrombotic complications (Fogarty et al., 2020; Sakr et al., 2020). SARS-CoV-2 can also directly infect endothelial cells, which may further contribute to coagulopathy (Varga et al., 2020). Given the role played by coagulopathy in acute SARS-CoV-2 infection, there has been extensive investigation of coagulation parameters in the setting of long-COVID. Sustained disturbances in coagulation pathways have been identified in post-COVID patients, with up to a quarter of those assessed having an elevated D-dimer 4 months after initial infection (Townsend et al., 2021b) Additionally, those hospitalised with acute COVID-19 have been shown to have reduced fibrinolytic potential and demonstrate increased thrombin generation at a similar timepoint (von Meijenfeldt et al., 2021). Sustained endothelial cell activation has been shown up to 10 weeks after initial acute infection in both hospitalised and non-hospitalised individuals, with increased factor VIII and soluble thrombomodulin levels (Fogarty et al., 2022).
Von Willebrand Factor, a marker of endothelial cell activation, also remains elevated in these individuals. It has been hypothesised that these changes are due to persistent endothelial dysfunction, and may result in ongoing, low-grade thrombus formation in the microvasculature, contributing to the symptoms reported in those with long COVID.
Disruption of the endothelium at the BBB has been proposed as a possible pathological process driving neurocognitive symptoms of long COVID. The BBB is a collection of endothelial cells, astrocytes, microglial cells and neurons, and acts as a bridge between the blood and the central nervous system (Langen et al., 2019). The neurological consequences and cerebral microvascular injuries seen in acute SARS-CoV-2 infection are well-described (Mao et al., 2020; Lee et al., 2021). The SARS-CoV-2 spike protein has been shown to impair BBB function (Buzhdygan et al., 2020). The prevalence of persistent cognitive dysfunction following SARS-CoV-2 infection has been reported as being above 50%, in contrast to results from prior pandemic coronaviruses (Kwong et al., 2020; Taquet et al., 2021). The detection of SARS-CoV-2 within the cerebrospinal fluid (CSF) of individuals with COVID-19 further supports disruption of BBB integrity during acute infection (Espíndola et al., 2021). Neuro-invasion of SARS-CoV-2 may result in neuronal loss and subsequent neurological impairment, while secondary inflammation may further contribute. Indeed, there is increasing evidence of structural brain changes following SARS-CoV-2 infection, associated with cognitive decline (Douaud et al., 2022) and abnormalities reminiscent of post chemotherapy neuronal changes have been observed in a mouse model of mild to moderate COVID-19 (Fernández-Castañeda et al., 2022). It is important to acknowledge that these changes seen in endothelial cells and at the BBB have not been shown to be directly pathogenic. The above studies, while demonstrating correlation between viral markers, symptoms, and markers of endothelial dysfunction, do not demonstrate direct causation. This is an area that warrants further mechanistic investigation, as it is of therapeutic potential.
In addition to structural changes, alterations in cerebral bloodflow are seen in the aftermath of infection, with changes persisting up to 10 months after infection (Tian et al., 2022). This may in part be due to the systemic inflammatory response triggered by SARS-CoV-2, which may increase BBB permeability (Alam et al., 2020). In patients with neurological features of long COVID, in particular brain fog, there are increasing circulating markers of BBB disruption such as TGFβ and IL-8 when compared to individuals who have recovered fully from SARS-CoV-2 infection (Campbell et al., 2022).
Therapeutics
The absence of clear pathogenic pathways to explain the myriad of presentations of long COVID has contributed to slow progress in the development of effective therapeutics. There remains no unifying treatment for long COVID. Both pharmacological and non-pharmacological therapies have been trialled for specific long COVID symptoms. The most common non-pharmacological interventions include physical and cognitive pacing for chronic fatigue and cognitive dysfunction. These have shown to have some improvement in functional outcomes (Bateman et al., 2021; O’Brien et al., 2022).
The potential persistence of viral reservoirs have led to suggestions that antiviral therapies such nirmatrelvir-ritonavir and remdesivir may be of benefit. Evidence from clinical trials including those being conducted by the STIMULATE-ICP group and others (Clinicaltrials, 2022) is awaited to clarify the role of these treatments in long COVID. The STIMULATE-ICP consortium in the United Kingdom are undertaking a large, randomised, platform clinical trial in long COVID, seeking to improve on the definition, the diagnosis and the management of long COVID. The planned interventions focus on community-based therapies as well as multi-organ MRI imaging, with a view to expanding in pharmacological therapies. Proposed therapeutics include anti-inflammatories such as colchicine, as well as anti-histamines.
The evidence supporting post-COVID endotheliopathy and coagulopathy, as well as the coagulopathy seen in acute infection, has stimulated trials on prolonged anti-thrombotic therapy. Use of the factor Xa inhibitor rivaroxaban in patients with high risk of thrombotic complications, based on predictive scoring tools and D-dimer levels, has been shown improve reduce the risk of venous thromboembolism 1 month after discharge (Ramacciotti et al., 2022). However, extended administration of these therapies beyond 1 month, their use in individuals who were not hospitalised with COVID-19, or impact on sequelae other than venous thromboembolism has not been extensively studied. Furthermore, the American Society of Haematology have issued a conditional recommendation against the use of anticoagulant prophylaxis post hospital discharge, although they acknowledge that there is very low certainty in the evidence and that high-quality, randomised controlled trials are needed (Cuker et al., 2022). The STIMULATE-ICP group hope to address the need for high-quality trial data by assessing response to rivaroxaban in this population (Forshaw et al., 2022).
The suggestion of ongoing immune dysfunction has also been the target of research into potential therapeutics for long COVID. Aberrant type-I interferon responses can be modulated by the histamine 2 receptor antagonist famotidine. This has shown earlier resolution in serum type-I interferon levels in patients with acute SARS-CoV-2 infection, and this was also associated with shorter time to symptom resolution (Brennan et al., 2022). However, to date there have been no RCTs examining the effect of anti-histamines in long COVID.
There has also been recent reports of positive impact of targeted therapeutics for management of persistent brain fog and cognitive dysfunction in long COVID. In a small case series, a combination of guanfacine, an α2 adrenoceptor agonist, and n-acetylcysteine, an anti-oxidant, was assessed in twelve individuals, eight of which reported symptomatic improvement (Fesharaki-Zadeh et al., 2023). Although preliminary, this pilot data can provide the basis for design of appropriately powered, placebo-controlled study to evaluate its efficacy.
Given the lack of evidence-based treatments, prevention of long COVID is a key to reducing the overall burden of this condition. Both vaccination against SARS-CoV-2 and certain modifiable lifestyle factors are associated with lower risk of long COVID-19 (Wang et al., 2023), and there is evidence that acute treatments including antivirals and metformin may be of benefit (Carfì et al., 2020; Xu et al., 2020).
Future directions
The lack of understanding of the pathological basis for the range of manifestations of long COVID continues to hamper the development of effective therapies and remains a significant unmet clinical need. As outlined here, a large number of symptoms and underlying abnormalities have been reported, but consistent, robust evidence of causal association is lacking in most cases. Studies to date have been limited by a lack of consensus definition or classification of long COVID, a focus on individual symptoms rather than defined phenotypes, disproportionate inclusion of individuals with a history of initial severe, acute COVID-19 illness, under-representation of minority groups and paucity of longitudinal studies. Furthermore, local changes at an organ level may be found in the absence of a measurable systemic inflammatory response, and requires examination of specimens such as CSF and respiratory samples which have been less well studied. Improving care for this large cohort will require a coordinated, structured and comprehensive research programme encompassing epidemiologic, basic science, translational and clinical studies. Immune perturbations within affected organs, including the lungs, gastrointestinal tract and the central nervous system, the effects of disease-independent factors such as sociodemographic factors, socioeconomic status and lifetime stress on chronic inflammation, immune response to acute infection, and immune recovery in the aftermath of infection are all areas that require further investigation.
Given the scale and economic burden of long COVID (Kingdom OonsU, 2022), clinical trials should be performed in parallel with mechanistic studies. A significant coordinated, international, interdisciplinary approach will be required to improve our understanding and management of this condition. While challenging, this effort offers the opportunity to significantly advance our understanding of host, pathogen and environmental factors urgently needed to understand post infectious conditions beyond long COVID.
Author contributions
GK and LT drafted the manuscript. All authors conceived and designed the manuscript, contributed to manuscript revision, read, and approved the submitted version.
Conflict of interest
The authors declare that the research was conducted in the absence of any commercial or financial relationships that could be construed as a potential conflict of interest.
Acknowledgments
Smurfit Kappa supported this work through an unrestricted grant. The funder was not involved in the study design, collection, analysis, interpretation of data, the writing of this article or the decision to submit it for publication.
Publisher’s note
All claims expressed in this article are solely those of the authors and do not necessarily represent those of their affiliated organizations, or those of the publisher, the editors and the reviewers. Any product that may be evaluated in this article, or claim that may be made by its manufacturer, is not guaranteed or endorsed by the publisher.
References
Al-Aly, Z., Bowe, B., and Xie, Y. (2022). Long COVID after breakthrough SARS-CoV-2 infection. Nat. Med. 28 (7), 1461–1467. doi:10.1038/s41591-022-01840-0
Al-Aly, Z., Xie, Y., and Bowe, B. (2021). High-dimensional characterization of post-acute sequelae of COVID-19. Nature 594 (7862), 259–264. doi:10.1038/s41586-021-03553-9
Alam, S., Willows, S., Kulka, M., and Sandhu, J. K. (2020). Severe acute respiratory syndrome coronavirus 2 may be an underappreciated pathogen of the central nervous system. Eur. J. neurology 27 (11), 2348–2360. doi:10.1111/ene.14442
Alba, G. A., Ziehr, D. R., Rouvina, J. N., Hariri, L. P., Knipe, R. S., Medoff, B. D., et al. (2021). Exercise performance in patients with post-acute sequelae of SARS-CoV-2 infection compared to patients with unexplained dyspnea. EClinicalMedicine 39, 101066. doi:10.1016/j.eclinm.2021.101066
Antonelli, M., Penfold, R. S., Merino, J., Sudre, C. H., Molteni, E., Berry, S., et al. (2022). Risk factors and disease profile of post-vaccination SARS-CoV-2 infection in UK users of the COVID symptom study app: A prospective, community-based, nested, case-control study. Lancet Infect. Dis. 22 (1), 43–55. doi:10.1016/S1473-3099(21)00460-6
Bastard, P., Gervais, A., Le Voyer, T., Rosain, J., Philippot, Q., Manry, J., et al. (2021). Autoantibodies neutralizing type I IFNs are present in∼ 4% of uninfected individuals over 70 years old and account for∼ 20% of COVID-19 deaths. Sci. Immunol. 6 (62), eabl4340. doi:10.1126/sciimmunol.abl4340
Bastard, P., Rosen, L. B., Zhang, Q., Michailidis, E., Hoffmann, H-H., Zhang, Y., et al. (2020). Autoantibodies against type I IFNs in patients with life-threatening COVID-19. Science 370 (6515), eabd4585. doi:10.1126/science.abd4585
Bernard-Raichon, L., Venzon, M., Klein, J., Axelrad, J. E., Zhang, C., Sullivan, A. P., et al. (2022). Gut microbiome dysbiosis in antibiotic-treated COVID-19 patients is associated with microbial translocation and bacteremia. Nat. Commun. 13 (1), 5926. doi:10.1038/s41467-022-33395-6
Blackett, J. W., Wainberg, M., Elkind, M. S. V., and Freedberg, D. E. (2022). Potential long coronavirus disease 2019 gastrointestinal symptoms 6 Months after coronavirus infection are associated with mental health symptoms. Gastroenterology 162 (2), 648–650.e2. doi:10.1053/j.gastro.2021.10.040
Bohnacker, S., Hartung, F., Henkel, F., Quaranta, A., Kolmert, J., Priller, A., et al. (2022). Mild COVID-19 imprints a long-term inflammatory eicosanoid-and chemokine memory in monocyte-derived macrophages. Mucosal Immunol. 15 (3), 515–524. doi:10.1038/s41385-021-00482-8
Brennan, C. M., Nadella, S., Zhao, X., Dima, R. J., Jordan-Martin, N., Demestichas, B. R., et al. (2022). Oral famotidine versus placebo in non-hospitalised patients with COVID-19: A randomised, double-blind, data-intense, phase 2 clinical trial. Gut 71 (5), 879–888. doi:10.1136/gutjnl-2022-326952
Brodin, P., Casari, G., Townsend, L., O’Farrelly, C., Tancevski, I., Löffler-Ragg, J., et al. (2022). Studying severe long COVID to understand post-infectious disorders beyond COVID-19. Nat. Med. 28 (5), 879–882. doi:10.1038/s41591-022-01766-7
Buzhdygan, T. P., DeOre, B. J., Baldwin-Leclair, A., Bullock, T. A., McGary, H. M., Khan, J. A., et al. (2020). The SARS-CoV-2 spike protein alters barrier function in 2D static and 3D microfluidic in-vitro models of the human blood–brain barrier. Neurobiol. Dis. 146, 105131. doi:10.1016/j.nbd.2020.105131
Campbell, M., Greene, C., Connolly, R., Brennan, D., Laffan, A., O'Keeffe, E., et al. (2022). Blood-brain barrier disruption in Long COVID-associated cognitive impairment. Berlin, Germany: Researchgate.
Canas, L. S., Molteni, E., Deng, J., Sudre, C. H., Murray, B., Kerfoot, E., et al. (2022). Profiling post-COVID syndrome across different variants of SARS-CoV-2. medRxiv 2022, 22278159. doi:10.1101/2022.07.28.22278159
Carfì, A., Bernabei, R., and Landi, F.Gemelli Against COVID-19 Post-Acute Care Study Group (2020). Persistent symptoms in patients after acute COVID-19. Jama 324 (6), 603–605. doi:10.1001/jama.2020.12603
Carod-Artal, F. J. (2018). Infectious diseases causing autonomic dysfunction. Clin. Aut. Res. 28 (1), 67–81. doi:10.1007/s10286-017-0452-4
Caspersen, I. H., Magnus, P., and Trogstad, L. (2022). Excess risk and clusters of symptoms after COVID-19 in a large Norwegian cohort. Eur. J. Epidemiol. 37 (5), 539–548. doi:10.1007/s10654-022-00847-8
Ceban, F., Ling, S., Lui, L. M. W., Lee, Y., Gill, H., Teopiz, K. M., et al. (2022). Fatigue and cognitive impairment in post-COVID-19 syndrome: A systematic review and meta-analysis. Brain, Behav. Immun. 101, 93–135. doi:10.1016/j.bbi.2021.12.020
Centers for Disease Control and Prevention (2022). Post-COVID conditions: Information for healthcare providers 2022. Available at: https://www.cdc.gov/coronavirus/2019-ncov/hcp/clinical-care/post-covid-conditions.html.
Cervia, C., Zurbuchen, Y., Taeschler, P., Ballouz, T., Menges, D., Hasler, S., et al. (2022). Immunoglobulin signature predicts risk of post-acute COVID-19 syndrome. Nat. Commun. 13 (1), 446. doi:10.1038/s41467-021-27797-1
Ceulemans, L. J., Khan, M., Yoo, S-J., Zapiec, B., Van Gerven, L., Van Slambrouck, J., et al. (2021). Persistence of SARS-CoV-2 RNA in lung tissue after mild COVID-19. Lancet Respir. Med. 9 (8), e78–e79. doi:10.1016/S2213-2600(21)00240-X
Chakraborty, S., Gonzalez, J. C., Sievers, B. L., Mallajosyula, V., Chakraborty, S., Dubey, M., et al. (2022). Early non-neutralizing, afucosylated antibody responses are associated with COVID-19 severity. Sci. Transl. Med. 14 (635), eabm7853. doi:10.1126/scitranslmed.abm7853
Chang, S. E., Feng, A., Meng, W., Apostolidis, S. A., Mack, E., Artandi, M., et al. (2021). New-onset IgG autoantibodies in hospitalized patients with COVID-19. Nat. Commun. 12 (1), 5417. doi:10.1038/s41467-021-25509-3
Chen, Y., Gu, S., Chen, Y., Lu, H., Shi, D., Guo, J., et al. (2022). Six-month follow-up of gut microbiota richness in patients with COVID-19. Gut 71 (1), 222–225. doi:10.1136/gutjnl-2021-324090
Chen-Scarabelli, C., and Scarabelli, T. M. (2004). Neurocardiogenic syncope. Bmj 329 (7461), 336–341. doi:10.1136/bmj.329.7461.336
Chularojanamontri, L., Tuchinda, P., Rujitharanawong, C., Hunnangkul, S., Pochanapan, O., Panjapakkul, W., et al. (2022). New-onset and exacerbated skin diseases after COVID-19 infection: A systematic review. J. Dermatol 49 (11), e419–e421. doi:10.1111/1346-8138.16501
Clinicaltrials (2022). Paxlovid for treatment of long covid (STOP-PASC). Available at: https://clinicaltrials.gov/ct2/show/NCT05576662.
Consiglio, C. R., Cotugno, N., Sardh, F., Pou, C., Amodio, D., Rodriguez, L., et al. (2020). The immunology of multisystem inflammatory syndrome in children with COVID-19. Cell 183 (4), 968–981. doi:10.1016/j.cell.2020.09.016
Cueto-Robledo, G., Roldan-Valadez, E., Graniel-Palafox, L. E., Garcia-Cesar, M., Torres-Rojas, M. B., Enriquez-Garcia, R., et al. (2022). Chronic thromboembolic pulmonary hypertension (cteph): A review of another sequel of severe post-covid-19 pneumonia. Curr. Probl. Cardiol. 2022, 101187. doi:10.1016/j.cpcardiol.2022.101187
Cuker, A., Tseng, E. K., Nieuwlaat, R., Angchaisuksiri, P., Blair, C., Dane, K., et al. (2022). American Society of Hematology living guidelines on the use of anticoagulation for thromboprophylaxis in patients with COVID-19: July 2021 update on postdischarge thromboprophylaxis. Blood Adv. 6 (2), 664–671. doi:10.1182/bloodadvances.2021005945
Cutler, D. M. (2022). The costs of long COVID. JAMA Health Forum 3 (5), e221809. doi:10.1001/jamahealthforum.2022.1809
Danesh, V., Arroliga, A. C., Bourgeois, J. A., Boehm, L. M., McNeal, M. J., Widmer, A. J., et al. (2022). Symptom clusters seen in adult COVID-19 recovery clinic care seekers. J. General Intern. Med. 38, 442–449. doi:10.1007/s11606-022-07908-4
Davis, H. E., Assaf, G. S., McCorkell, L., Wei, H., Low, R. J., Re'em, Y., et al. (2021). Characterizing long COVID in an international cohort: 7 months of symptoms and their impact. EClinicalMedicine 38, 101019. doi:10.1016/j.eclinm.2021.101019
Douaud, G., Lee, S., Alfaro-Almagro, F., Arthofer, C., Wang, C., McCarthy, P., et al. (2022). SARS-CoV-2 is associated with changes in brain structure in UK Biobank. Nature 604 (7907), 697–707. doi:10.1038/s41586-022-04569-5
Espíndola, O. M., Brandão, C. O., Gomes, Y. C. P., Siqueira, M., Soares, C. N., Lima, M. A. S. D., et al. (2021). Cerebrospinal fluid findings in neurological diseases associated with COVID-19 and insights into mechanisms of disease development. Int. J. Infect. Dis. 102, 155–162. doi:10.1016/j.ijid.2020.10.044
Estiri, H., Strasser, Z. H., Brat, G. A., Semenov, Y. R., Aaron, J. R., Agapito, G., et al. (2021). Evolving phenotypes of non-hospitalized patients that indicate long COVID. BMC Med. 19 (1), 249. doi:10.1186/s12916-021-02115-0
Bateman L., Bested A. C., Bonilla H. F., Chheda B. V., Chu L., Curtin J. M.et al. (2021). Myalgic encephalomyelitis/chronic fatigue syndrome: Essentials of diagnosis and management. Mayo clinic proceedings (Amsterdam, Netherlands: Elsevier).
Kenny G., McCann K., O’Brien C., Savinelli S., Tinago W., Yousif O.et al. (2022). Identification of distinct long COVID clinical phenotypes through cluster analysis of self-reported symptoms. Open forum infectious diseases (Oxford, United Kingdom: Oxford University Press US).
Fernández-Castañeda, A., Lu, P., Geraghty, A. C., Song, E., Lee, M-H., Wood, J., et al. (2022). Mild respiratory COVID can cause multi-lineage neural cell and myelin dysregulation. Cell 185 (14), 2452–2468.e16. doi:10.1016/j.cell.2022.06.008
Fernández-de-Las-Peñas, C., Martín-Guerrero, J. D., Florencio, L. L., Navarro-Pardo, E., Rodríguez-Jiménez, J., Torres-Macho, J., et al. (2022). Clustering analysis reveals different profiles associating long-term post-COVID symptoms, COVID-19 symptoms at hospital admission and previous medical co-morbidities in previously hospitalized COVID-19 survivors. Infection 51, 61–69. doi:10.1007/s15010-022-01822-x
Fesharaki-Zadeh, A., Lowe, N., and Arnsten, A. F. (2023). Clinical experience with the α2A-adrenoceptor agonist, guanfacine, and N-acetylcysteine for the treatment of cognitive deficits in “Long-COVID19”. Neuroimmunol. Rep. 3, 100154. doi:10.1016/j.nerep.2022.100154
Files, J. K., Boppana, S., Perez, M. D., Sarkar, S., Lowman, K. E., Qin, K., et al. (2021). Sustained cellular immune dysregulation in individuals recovering from SARS-CoV-2 infection. J. Clin. investigation 131 (1), e140491. doi:10.1172/JCI140491
Fogarty, H., Townsend, L., Ni Cheallaigh, C., Bergin, C., Martin-Loeches, I., Browne, P., et al. (2020). More on COVID-19 coagulopathy in Caucasian patients. Br. J. Haematol. 189, 1060–1061. doi:10.1111/bjh.16791
Fogarty, H., Ward, S. E., Townsend, L., Karampini, E., Elliott, S., Conlon, N., et al. (2022). Sustained VWF-ADAMTS-13 axis imbalance and endotheliopathy in long COVID syndrome is related to immune dysfunction. J. Thrombosis Haemostasis 20, 2429–2438. doi:10.1111/jth.15830
Forshaw, D., Wall, E. C., Prescott, G., Dehbi, H-M., Green, A., Attree, E., et al. (2022). STIMULATE-ICP: A pragmatic, multi-centre, cluster randomised trial of an integrated care pathway with a nested, phase III, open label, adaptive platform randomised drug trial in individuals with long COVID: A structured protocol. medRxiv 2022, 22277893. doi:10.1101/2022.07.21.22277893
Frontera, J. A., Thorpe, L. E., Simon, N. M., de Havenon, A., Yaghi, S., Sabadia, S. B., et al. (2022). Post-acute sequelae of COVID-19 symptom phenotypes and therapeutic strategies: A prospective, observational study. Plos one 17 (9), e0275274. doi:10.1371/journal.pone.0275274
Gaebler, C., Wang, Z., Lorenzi, J. C., Muecksch, F., Finkin, S., Tokuyama, M., et al. (2021). Evolution of antibody immunity to SARS-CoV-2. Nature 591 (7851), 639–644. doi:10.1038/s41586-021-03207-w
Gao, J., Chu, W., Duan, J., Li, J., Ma, W., Hu, C., et al. (2021). Six-month outcomes of post-ARDS pulmonary fibrosis in patients with H1N1 pneumonia. Front. Mol. Biosci. 8, 640763. doi:10.3389/fmolb.2021.640763
George, P. M., Reed, A., Desai, S. R., Devaraj, A., Faiez, T. S., Laverty, S., et al. (2022). A persistent neutrophil-associated immune signature characterizes post–COVID-19 pulmonary sequelae. Sci. Transl. Med. 14 (671), eabo5795. doi:10.1126/scitranslmed.abo5795
Giron, L. B., Dweep, H., Yin, X., Wang, H., Damra, M., Goldman, A. R., et al. (2021). Plasma markers of disrupted gut permeability in severe COVID-19 patients. Front. Immunol. 12, 686240. doi:10.3389/fimmu.2021.686240
Giron, L. B., Peluso, M. J., Ding, J., Kenny, G., Zilberstein, N. F., Koshy, J., et al. (2022). Markers of fungal translocation are elevated during post-acute sequelae of SARS-CoV-2 and induce NF-κB signaling. JCI Insight 7 (15), e164813. doi:10.1172/jci.insight.164813
Gold, J. E., Okyay, R. A., Licht, W. E., and Hurley, D. J. (2021). Investigation of long COVID prevalence and its relationship to Epstein-Barr virus reactivation. Pathogens 10 (6), 763. doi:10.3390/pathogens10060763
Goyal, P. K., Mohammed, T. O., Mahmoud, A., Zaidi, A. J., and Nguyen, C. V. (2022). COVID-19 infection leading to acute pustular dermatoses. Arch. Dermatol Res. 2022, 1–13. doi:10.1007/s00403-022-02450-z
Griffin, D. E. (2022). Why does viral RNA sometimes persist after recovery from acute infections? PLoS Biol. 20 (6), e3001687. doi:10.1371/journal.pbio.3001687
Guler, S. A., Ebner, L., Aubry-Beigelman, C., Bridevaux, P. O., Brutsche, M., Clarenbach, C., et al. (2021). Pulmonary function and radiological features 4 months after COVID-19: First results from the national prospective observational Swiss COVID-19 lung study. Eur. Respir. J. 57 (4), 2003690. doi:10.1183/13993003.03690-2020
Hall, V. J., Foulkes, S., Charlett, A., Atti, A., Monk, E. J., Simmons, R., et al. (2021). SARS-CoV-2 infection rates of antibody-positive compared with antibody-negative health-care workers in england: A large, multicentre, prospective cohort study (SIREN). Lancet 397 (10283), 1459–1469. doi:10.1016/S0140-6736(21)00675-9
Hamming, I., Timens, W., Bulthuis, M., Lely, A., Gv, N., and van Goor, H. (2004). Tissue distribution of ACE2 protein, the functional receptor for SARS coronavirus. A first step in understanding SARS pathogenesis. J. Pathology 203 (2), 631–637. doi:10.1002/path.1570
Herridge, M. S., Tansey, C. M., Matté, A., Tomlinson, G., Diaz-Granados, N., Cooper, A., et al. (2011). Functional disability 5 Years after acute respiratory distress syndrome. N. Engl. J. Med. 364 (14), 1293–1304. doi:10.1056/NEJMoa1011802
Hickie, I., Davenport, T., Wakefield, D., Vollmer-Conna, U., Cameron, B., Vernon, S. D., et al. (2006). Post-infective and chronic fatigue syndromes precipitated by viral and non-viral pathogens: Prospective cohort study. Bmj 333 (7568), 575. doi:10.1136/bmj.38933.585764.AE
Hoepel, W., Chen, H-J., Geyer, C. E., Allahverdiyeva, S., Manz, X. D., de Taeye, S. W., et al. (2021). High titers and low fucosylation of early human anti–SARS-CoV-2 IgG promote inflammation by alveolar macrophages. Sci. Transl. Med. 13 (596), eabf8654. doi:10.1126/scitranslmed.abf8654
Huang, A. T., Garcia-Carreras, B., Hitchings, M. D., Yang, B., Katzelnick, L. C., Rattigan, S. M., et al. (2020). A systematic review of antibody mediated immunity to coronaviruses: Kinetics, correlates of protection, and association with severity. Nat. Commun. 11 (1), 4704–4716. doi:10.1038/s41467-020-18450-4
Huang, C., Huang, L., Wang, Y., Li, X., Ren, L., Gu, X., et al. (2021). 6-month consequences of COVID-19 in patients discharged from hospital: A cohort study. Lancet 397 (10270), 220–232. doi:10.1016/S0140-6736(20)32656-8
Huang, C., Wang, Y., Li, X., Ren, L., Zhao, J., Hu, Y., et al. (2020). Clinical features of patients infected with 2019 novel coronavirus in Wuhan, China. Lancet 395 (10223), 497–506. doi:10.1016/S0140-6736(20)30183-5
Huang, L., Yao, Q., Gu, X., Wang, Q., Ren, L., Wang, Y., et al. (2021). 1-year outcomes in hospital survivors with COVID-19: A longitudinal cohort study. Lancet 398 (10302), 747–758. doi:10.1016/S0140-6736(21)01755-4
Hughes, E. C., and Saleh, D. (2022). Telogen effluvium. StatPearls. Treasure island (FL). Florida, United States: StatPearls Publishing LLC. StatPearls Publishing Copyright © 2022.
Isa, A., Kasprowicz, V., Norbeck, O., Loughry, A., Jeffery, K., Broliden, K., et al. (2005). Prolonged activation of virus-specific CD8+ T cells after acute B19 infection. PLoS Med. 2 (12), e343. doi:10.1371/journal.pmed.0020343
Jamal, S. M., Landers, D. B., Hollenberg, S. M., Turi, Z. G., Glotzer, T. V., Tancredi, J., et al. (2022). Prospective evaluation of autonomic dysfunction in post-acute sequela of COVID-19. J. Am. Coll. Cardiol. 79 (23), 2325–2330. doi:10.1016/j.jacc.2022.03.357
Jing, Y., Luo, L., Chen, Y., Westerberg, L. S., Zhou, P., Xu, Z., et al. (2021). SARS-CoV-2 infection causes immunodeficiency in recovered patients by downregulating CD19 expression in B cells via enhancing B-cell metabolism. Signal Transduct. Target. Ther. 6 (1), 345–413. doi:10.1038/s41392-021-00749-3
Joli, J., Buck, P., Zipfel, S., and Stengel, A. (2022). Post-COVID-19 fatigue: A systematic review. Front. Psychiatry 13, 947973. doi:10.3389/fpsyt.2022.947973
Joseph, P., Arevalo, C., Oliveira, R. K. F., Faria-Urbina, M., Felsenstein, D., Oaklander, A. L., et al. (2021). Insights from invasive cardiopulmonary exercise testing of patients with myalgic encephalomyelitis/chronic fatigue syndrome. Chest 160 (2), 642–651. doi:10.1016/j.chest.2021.01.082
Kenny, G., McCann, K., O'Brien, C., Savinelli, S., O'Broin, C., Tinago, W., et al. (2022). Identification of distinct long COVID clinical phenotypes through cluster analysis of self-reported symptoms. Open Forum Infect. Dis. 9 (2), ofac060. doi:10.1093/ofid/ofac060
Khoury, D. S., Cromer, D., Reynaldi, A., Schlub, T. E., Wheatley, A. K., Juno, J. A., et al. (2021). Neutralizing antibody levels are highly predictive of immune protection from symptomatic SARS-CoV-2 infection. Nat. Med. 27 (7), 1205–1211. doi:10.1038/s41591-021-01377-8
Kingdom OonsU (2022). Self-reported long COVID and labour market outcomes, UK. Available at: https://www.ons.gov.uk/peoplepopulationandcommunity/healthandsocialcare/conditionsanddiseases/bulletins/selfreportedlongcovidandlabourmarketoutcomesuk2022/selfreportedlongcovidandlabourmarketoutcomesuk2022.
Klein, J., Wood, J., Jaycox, J., Lu, P., Dhodapkar, R. M., Gehlhausen, J. R., et al. (2022). Distinguishing features of Long COVID identified through immune profiling. medRxiv 2022, 22278592. doi:10.1101/2022.08.09.22278592
Klein, J., Wood, J., Jaycox, J., Lu, P., Dhodapkar, R. M., Gehlhausen, J. R., et al. (2022). Distinguishing features of Long COVID identified through immune profiling. medRxiv 2022, 22278592. doi:10.1101/2022.08.09.22278592
Kwong, K. C. N. K., Mehta, P. R., Shukla, G., and Mehta, A. R. (2020). COVID-19, SARS and MERS: A neurological perspective. J. Clin. Neurosci. 77, 13–16. doi:10.1016/j.jocn.2020.04.124
Ladlow, P., O’Sullivan, O., Houston, A., Barker-Davies, R., May, S., Mills, D., et al. (2022). Dysautonomia following COVID-19 is not associated with subjective limitations or symptoms but is associated with objective functional limitations. Heart rhythm. 19 (4), 613–620. doi:10.1016/j.hrthm.2021.12.005
Lam, M. H-B., Wing, Y-K., Yu, M. W-M., Leung, C-M., Ma, R. C., Kong, A. P., et al. (2009). Mental morbidities and chronic fatigue in severe acute respiratory syndrome survivors: Long-term follow-up. Archives Intern. Med. 169 (22), 2142–2147. doi:10.1001/archinternmed.2009.384
Langen, U. H., Ayloo, S., and Gu, C. (2019). Development and cell biology of the blood-brain barrier. Annu. Rev. Cell Dev. Biol. 35, 591–613. doi:10.1146/annurev-cellbio-100617-062608
Lee, M-H., Perl, D. P., Nair, G., Li, W., Maric, D., Murray, H., et al. (2021). Microvascular injury in the brains of patients with Covid-19. N. Engl. J. Med. 384 (5), 481–483. doi:10.1056/NEJMc2033369
Lee, S. H., Shin, H-S., Park, H. Y., Kim, J. L., Lee, J. J., Lee, H., et al. (2019). Depression as a mediator of chronic fatigue and post-traumatic stress symptoms in Middle East respiratory syndrome survivors. Psychiatry investig. 16 (1), 59–64. doi:10.30773/pi.2018.10.22.3
Liao, X., Li, D., Ma, Z., Zhang, L., Zheng, B., Li, Z., et al. (2022). 12-Month post-discharge liver function test abnormalities among patients with COVID-19: A single-center prospective cohort study. Front. Cell. Infect. Microbiol. 12, 864933. doi:10.3389/fcimb.2022.864933
Littlefield, K. M., Watson, R. O., Schneider, J. M., Neff, C. P., Yamada, E., Zhang, M., et al. (2022). SARS-CoV-2-specific T cells associate with inflammation and reduced lung function in pulmonary post-acute sequalae of SARS-CoV-2. PLOS Pathog. 18 (5), e1010359. doi:10.1371/journal.ppat.1010359
Liu, Q., Mak, J. W. Y., Su, Q., Yeoh, Y. K., Lui, G. C-Y., Ng, S. S. S., et al. (2022). Gut microbiota dynamics in a prospective cohort of patients with post-acute COVID-19 syndrome. Gut 71 (3), 544–552. doi:10.1136/gutjnl-2021-325989
Loretelli, C., Abdelsalam, A., D’Addio, F., Nasr, M. B., Assi, E., Usuelli, V., et al. (2021). PD-1 blockade counteracts post–COVID-19 immune abnormalities and stimulates the anti–SARS-CoV-2 immune response. JCI insight 6 (24), e146701. doi:10.1172/jci.insight.146701
Lund, L. C., Hallas, J., Nielsen, H., Koch, A., Mogensen, S. H., Brun, N. C., et al. (2021). Post-acute effects of SARS-CoV-2 infection in individuals not requiring hospital admission: A Danish population-based cohort study. Lancet Infect. Dis. 21 (10), 1373–1382. doi:10.1016/S1473-3099(21)00211-5
Maglietta, G., Diodati, F., Puntoni, M., Lazzarelli, S., Marcomini, B., Patrizi, L., et al. (2022). Prognostic factors for post-COVID-19 syndrome: A systematic review and meta-analysis. J. Clin. Med. 11 (6), 1541. doi:10.3390/jcm11061541
Mao, L., Jin, H., Wang, M., Hu, Y., Chen, S., He, Q., et al. (2020). Neurologic manifestations of hospitalized patients with coronavirus disease 2019 in Wuhan, China. JAMA neurol. 77 (6), 683–690. doi:10.1001/jamaneurol.2020.1127
McMahon, D. E., Gallman, A. E., Hruza, G. J., Rosenbach, M., Lipoff, J. B., Desai, S. R., et al. (2021). Long COVID in the skin: A registry analysis of COVID-19 dermatological duration. Lancet Infect. Dis. 21 (3), 313–314. doi:10.1016/S1473-3099(20)30986-5
Moderbacher, C. R., Ramirez, S. I., Dan, J. M., Grifoni, A., Hastie, K. M., Weiskopf, D., et al. (2020). Antigen-specific adaptive immunity to SARS-CoV-2 in acute COVID-19 and associations with age and disease severity. Cell 183 (4), 996–1012. doi:10.1016/j.cell.2020.09.038
Moldofsky, H., and Patcai, J. (2011). Chronic widespread musculoskeletal pain, fatigue, depression and disordered sleep in chronic post-SARS syndrome; a case-controlled study. BMC Neurol. 11 (1), 37. doi:10.1186/1471-2377-11-37
Natarajan, A., Zlitni, S., Brooks, E. F., Vance, S. E., Dahlen, A., Hedlin, H., et al. (2022). Gastrointestinal symptoms and fecal shedding of SARS-CoV-2 RNA suggest prolonged gastrointestinal infection. Med 3, 371–387.e9. doi:10.1016/j.medj.2022.04.001
Newton, J., Okonkwo, O., Sutcliffe, K., Seth, A., Shin, J., and Jones, D. (2007). Symptoms of autonomic dysfunction in chronic fatigue syndrome. QJM Int. J. Med. 100 (8), 519–526. doi:10.1093/qjmed/hcm057
Notarte, K. I., Catahay, J. A., Velasco, J. V., Pastrana, A., Ver, A. T., Pangilinan, F. C., et al. (2022). Impact of COVID-19 vaccination on the risk of developing long-COVID and on existing long-COVID symptoms: A systematic review. eClinicalMedicine 53, 101624. doi:10.1016/j.eclinm.2022.101624
O’Brien, K., Townsend, L., Dowds, J., Bannan, C., Nadarajan, P., Kent, B., et al. (2022). 1-year quality of life and health-outcomes in patients hospitalised with COVID-19: A longitudinal cohort study. Respir. Res. 23 (1), 115–211. doi:10.1186/s12931-022-02032-7
Patterson, B. K., Francisco, E. B., Yogendra, R., Long, E., Pise, A., Rodrigues, H., et al. (2022). Persistence of SARS CoV-2 S1 protein in CD16+ monocytes in post-acute sequelae of COVID-19 (PASC) up to 15 months post-infection. Front. Immunol. 12, 5526. doi:10.3389/fimmu.2021.746021
Peluso, M. J., Deveau, T-M., Munter, S. E., Ryder, D. M., Buck, A. M., Beck-Engeser, G., et al. (2022). Impact of pre-existing chronic viral infection and reactivation on the development of long COVID. J. Clin. Investigation 2022, 22276660. doi:10.1101/2022.06.21.22276660
Peluso, M. J., Lu, S., Tang, A. F., Durstenfeld, M. S., Ho, H., Goldberg, S. A., et al. (2021). Markers of immune activation and inflammation in individuals with postacute sequelae of severe acute respiratory syndrome coronavirus 2 infection. J. Infect. Dis. 224 (11), 1839–1848. doi:10.1093/infdis/jiab490
Peluso, M. J., Mitchell, A., Wang, C. Y., Takahashi, S., Hoh, R., Tai, V., et al. (2022). Low prevalence of interferon α autoantibodies in people experiencing symptoms of post–coronavirus disease 2019 (COVID-19) conditions, or long COVID. J. Infect. Dis. 227, 246–250. doi:10.1093/infdis/jiac372
Pérez-Gómez, A., Vitallé, J., Gasca-Capote, C., Gutierrez-Valencia, A., Trujillo-Rodriguez, M., Serna-Gallego, A., et al. (2021). Dendritic cell deficiencies persist seven months after SARS-CoV-2 infection. Cell. Mol. Immunol. 18 (9), 2128–2139. doi:10.1038/s41423-021-00728-2
Phetsouphanh, C., Darley, D. R., Wilson, D. B., Howe, A., Munier, C., Patel, S. K., et al. (2022). Immunological dysfunction persists for 8 months following initial mild-to-moderate SARS-CoV-2 infection. Nat. Immunol. 23 (2), 210–216. doi:10.1038/s41590-021-01113-x
Puntmann, V. O., Carerj, M. L., Wieters, I., Fahim, M., Arendt, C., Hoffmann, J., et al. (2020). Outcomes of cardiovascular magnetic resonance imaging in patients recently recovered from coronavirus disease 2019 (COVID-19). JAMA Cardiol. 5, 1265–1273. doi:10.1001/jamacardio.2020.3557
Radin, J. M., Quer, G., Ramos, E., Baca-Motes, K., Gadaleta, M., Topol, E. J., et al. (2021). Assessment of prolonged physiological and behavioral changes associated with COVID-19 infection. JAMA Netw. Open 4 (7), e2115959–e. doi:10.1001/jamanetworkopen.2021.15959
Ramacciotti, E., Agati, L. B., Calderaro, D., Aguiar, V. C. R., Spyropoulos, A. C., de Oliveira, C. C. C., et al. (2022). Rivaroxaban versus no anticoagulation for post-discharge thromboprophylaxis after hospitalisation for COVID-19 (MICHELLE): An open-label, multicentre, randomised, controlled trial. Lancet 399 (10319), 50–59. doi:10.1016/S0140-6736(21)02392-8
Reese, J. T., Blau, H., Casiraghi, E., Bergquist, T., Loomba, J. J., Callahan, T. J., et al. (2023). Generalisable long COVID subtypes: Findings from the NIH N3C and RECOVER programmes. EBioMedicine 87, 104413. doi:10.1016/j.ebiom.2022.104413
Remy-Jardin, M., Duthoit, L., Perez, T., Felloni, P., Faivre, J-B., Fry, S., et al. (2021). Assessment of pulmonary arterial circulation 3 months after hospitalization for SARS-CoV-2 pneumonia: Dual-energy CT (DECT) angiographic study in 55 patients. EClinicalMedicine 34, 100778. doi:10.1016/j.eclinm.2021.100778
Renaud, M., Thibault, C., Le Normand, F., Mcdonald, E. G., Gallix, B., Debry, C., et al. (2021). Clinical outcomes for patients with anosmia 1 year after COVID-19 diagnosis. JAMA Netw. open 4 (6), e2115352. doi:10.1001/jamanetworkopen.2021.15352
Roach, A., Chikwe, J., Catarino, P., Rampolla, R., Noble, P. W., Megna, D., et al. (2022). Lung transplantation for covid-19–related respiratory failure in the United States. N. Engl. J. Med. 386 (12), 1187–1188. doi:10.1056/NEJMc2117024
Sahanic, S., Tymoszuk, P., Ausserhofer, D., Rass, V., Pizzini, A., Nordmeyer, G., et al. (2022). Phenotyping of acute and persistent coronavirus disease 2019 features in the outpatient setting: Exploratory analysis of an international cross-sectional online survey. Clin. Infect. Dis. 75 (1), e418–e431. doi:10.1093/cid/ciab978
Sakr, Y., Giovini, M., Leone, M., Pizzilli, G., Kortgen, A., Bauer, M., et al. (2020). Pulmonary embolism in patients with coronavirus disease-2019 (COVID-19) pneumonia: A narrative review. Ann. intensive care 10 (1), 124–213. doi:10.1186/s13613-020-00741-0
Saletti, G., Gerlach, T., Jansen, J. M., Molle, A., Elbahesh, H., Ludlow, M., et al. (2020). Older adults lack SARS CoV-2 cross-reactive T lymphocytes directed to human coronaviruses OC43 and NL63. Sci. Rep. 10 (1), 21447–21510. doi:10.1038/s41598-020-78506-9
Sancho-Shimizu, V., Brodin, P., Cobat, A., Biggs, C. M., Toubiana, J., Lucas, C. L., et al. (2021). SARS-CoV-2–related mis-C: A key to the viral and genetic causes of kawasaki disease? J. Exp. Med. 218 (6), e20210446. doi:10.1084/jem.20210446
Singh, I., Joseph, P., Heerdt, P. M., Cullinan, M., Lutchmansingh, D. D., Gulati, M., et al. (2022). Persistent exertional intolerance after COVID-19: Insights from invasive cardiopulmonary exercise testing. Chest 161 (1), 54–63. doi:10.1016/j.chest.2021.08.010
Singh, I., Oliveira, R. K. F., Naeije, R., Oldham, W. M., Faria-Urbina, M., Waxman, A. B., et al. (2021). Systemic vascular distensibility relates to exercise capacity in connective tissue disease. Rheumatol. Oxf. 60 (3), 1429–1434. doi:10.1093/rheumatology/keaa510
Smith, N., Possémé, C., Bondet, V., Sugrue, J., Townsend, L., Charbit, B., et al. (2022). Defective activation and regulation of type I interferon immunity is associated with increasing COVID-19 severity. Nat. Commun. 13 (1), 7254–7314. doi:10.1038/s41467-022-34895-1
Sneller, M. C., Liang, C. J., Marques, A. R., Chung, J. Y., Shanbhag, S. M., Fontana, J. R., et al. (2022). A longitudinal study of COVID-19 sequelae and immunity: Baseline findings. Ann. Intern. Med. 175, 969–979. doi:10.7326/M21-4905
Soares, M. N., Eggelbusch, M., Naddaf, E., Gerrits, K. H. L., van der Schaaf, M., van den Borst, B., et al. (2022). Skeletal muscle alterations in patients with acute Covid-19 and post-acute sequelae of Covid-19. J. Cachexia Sarcopenia Muscle 13 (1), 11–22. doi:10.1002/jcsm.12896
Sotzny, F., Filgueiras, I. S., Kedor, C., Freitag, H., Wittke, K., Bauer, S., et al. (2022). Dysregulated autoantibodies targeting vaso-and immunoregulatory receptors in Post COVID Syndrome correlate with symptom severity. Front. Immunol. 13, 981532. doi:10.3389/fimmu.2022.981532
Stoffels, A. A. F., van Voorthuizen, E. L., van Hees, H. W. H., Peters, J. B., van Helvoort, H. A. C., Voermans, N. C., et al. (2022). Longitudinal analysis of quadriceps muscle strength in patients with previous COVID-19 hospitalization and in patients with post-acute sequelae following mild COVID-19. Nutrients 14 (20), 4319. doi:10.3390/nu14204319
Su, Y., Yuan, D., Chen, D. G., Ng, R. H., Wang, K., Choi, J., et al. (2022). Multiple early factors anticipate post-acute COVID-19 sequelae. Cell 185 (5), 881–895. doi:10.1016/j.cell.2022.01.014
Subramanian, A., Nirantharakumar, K., Hughes, S., Myles, P., Williams, T., Gokhale, K. M., et al. (2022). Symptoms and risk factors for long COVID in non-hospitalized adults. Nat. Med. 28 (8), 1706–1714. doi:10.1038/s41591-022-01909-w
Sudre, C. H., Murray, B., Varsavsky, T., Graham, M. S., Penfold, R. S., Bowyer, R. C., et al. (2021). Attributes and predictors of long COVID. Nat. Med. 27 (4), 626–631. doi:10.1038/s41591-021-01292-y
Swank, Z., Senussi, Y., Manickas-Hill, Z., Yu, X. G., Li, J. Z., Alter, G., et al. (2022). Persistent circulating severe acute respiratory syndrome coronavirus 2 spike is associated with post-acute coronavirus disease 2019 sequelae. Clin. Infect. Dis. 76, e487–e490. doi:10.1093/cid/ciac722
Taeschler, P., Adamo, S., Deng, Y., Cervia, C., Zurbuchen, Y., Chevrier, S., et al. (2022). T cell recovery and evidence of persistent immune activation 12 months after severe COVID-19. Allergy 77 (8), 2468–2481. doi:10.1111/all.15372
Tan, B. K. J., Han, R., Zhao, J. J., Tan, N. K. W., Quah, E. S. H., Tan, C. J-W., et al. (2022). Prognosis and persistence of smell and taste dysfunction in patients with Covid-19: meta-analysis with parametric cure modelling of recovery curves. BMJ 378, e069503. doi:10.1136/bmj-2021-069503
Taquet, M., Geddes, J. R., Husain, M., Luciano, S., and Harrison, P. J. (2021). 6-month neurological and psychiatric outcomes in 236 379 survivors of COVID-19: A retrospective cohort study using electronic health records. Lancet Psychiatry 8, 416–427. doi:10.1016/S2215-0366(21)00084-5
Tejerina, F., Catalan, P., Rodriguez-Grande, C., Adan, J., Rodriguez-Gonzalez, C., Muñoz, P., et al. (2022). Post-COVID-19 syndrome. SARS-CoV-2 RNA detection in plasma, stool, and urine in patients with persistent symptoms after COVID-19. BMC Infect. Dis. 22 (1), 211. doi:10.1186/s12879-022-07153-4
Thurner, L., Fadle, N., Regitz, E., Preuss, K. D., Neumann, F., Cetin, O., et al. (2022). Autoantibodies against SUMO1-DHX35 in long-COVID. J. Transl. Autoimmun. 5, 100171. doi:10.1016/j.jtauto.2022.100171
Tian, T., Wu, J., Chen, T., Li, J., Yan, S., Zhou, Y., et al. (2022). Long-term follow-up of dynamic brain changes in patients recovered from COVID-19 without neurological manifestations. JCI insight 7 (4), e155827. doi:10.1172/jci.insight.155827
Townsend, L., Dyer, A. H., Jones, K., Dunne, J., Mooney, A., Gaffney, F., et al. (2020). Persistent fatigue following SARS-CoV-2 infection is common and independent of severity of initial infection. Plos one 15 (11), e0240784. doi:10.1371/journal.pone.0240784
Townsend, L., Dyer, A. H., Naughton, A., Imangaliyev, S., Dunne, J., Kiersey, R., et al. (2022). Severe COVID-19 is characterised by inflammation and immature myeloid cells early in disease progression. Heliyon 8 (4), e09230. doi:10.1016/j.heliyon.2022.e09230
Townsend, L., Dyer, A. H., Naughton, A., Kiersey, R., Holden, D., Gardiner, M., et al. (2021). Longitudinal analysis of COVID-19 patients shows age-associated T cell changes independent of ongoing ill-health. Front. Immunol. 12, 676932. doi:10.3389/fimmu.2021.676932
Townsend, L., Fogarty, H., Dyer, A., Martin-Loeches, I., Bannan, C., Nadarajan, P., et al. (2021). Prolonged elevation of D-dimer levels in convalescent COVID-19 patients is independent of the acute phase response. J. Thrombosis Haemostasis 19 (4), 1064–1070. doi:10.1111/jth.15267
Townsend, L., Moloney, D., Finucane, C., McCarthy, K., Bergin, C., Bannan, C., et al. (2021). Fatigue following COVID-19 infection is not associated with autonomic dysfunction. PloS one 16 (2), e0247280. doi:10.1371/journal.pone.0247280
USA DoHaHS (2022). National research action plan on long COVID. Available at: https://www.covid.gov/assets/files/National-Research-Action-Plan-on-Long-COVID-08012022.pdf.
Varga, Z., Flammer, A. J., Steiger, P., Haberecker, M., Andermatt, R., Zinkernagel, A. S., et al. (2020). Endothelial cell infection and endotheliitis in COVID-19. Lancet 395 (10234), 1417–1418. doi:10.1016/S0140-6736(20)30937-5
Vargas Centanaro, G., Calle Rubio, M., Álvarez-Sala Walther, J. L., Martinez-Sagasti, F., Albuja Hidalgo, A., Herranz Hernández, R., et al. (2022). Long-term outcomes and recovery of patients who survived COVID-19: LUNG INJURY COVID-19 study. Open Forum Infect. Dis. 9 (4), ofac098. doi:10.1093/ofid/ofac098
Venkatesan, P. (2021). NICE guideline on long COVID. Lancet Respir. Med. 9 (2), 129. doi:10.1016/S2213-2600(21)00031-X
von Meijenfeldt, F. A., Havervall, S., Adelmeijer, J., Lundström, A., Magnusson, M., Mackman, N., et al. (2021). Sustained prothrombotic changes in COVID-19 patients 4 months after hospital discharge. Blood Adv. 5 (3), 756–759. doi:10.1182/bloodadvances.2020003968
Wajnberg, A., Amanat, F., Firpo, A., Altman, D. R., Bailey, M. J., Mansour, M., et al. (2020). Robust neutralizing antibodies to SARS-CoV-2 infection persist for months. Science 370 (6521), 1227–1230. doi:10.1126/science.abd7728
Wang, E. Y., Mao, T., Klein, J., Dai, Y., Huck, J. D., Jaycox, J. R., et al. (2021). Diverse functional autoantibodies in patients with COVID-19. Nature 595 (7866), 283–288. doi:10.1038/s41586-021-03631-y
Wang, S., Li, Y., Yue, Y., Yuan, C., Kang, J. H., Chavarro, J. E., et al. (2023). Adherence to healthy lifestyle prior to infection and risk of post–COVID-19 condition. JAMA Intern. Med. 183 (3), 232–241. doi:10.1001/jamainternmed.2022.6555
Weng, J., Li, Y., Li, J., Shen, L., Zhu, L., Liang, Y., et al. (2021). Gastrointestinal sequelae 90 days after discharge for COVID-19. Lancet Gastroenterol. Hepatol. 6 (5), 344–346. doi:10.1016/S2468-1253(21)00076-5
Whitaker, M., Elliott, J., Chadeau-Hyam, M., Riley, S., Darzi, A., Cooke, G., et al. (2022). Persistent COVID-19 symptoms in a community study of 606,434 people in England. Nat. Commun. 13 (1), 1957–2010. doi:10.1038/s41467-022-29521-z
WHO (2021). A clinical case definition of post COVID-19 condition by a Delphi consensus, 6 October 2021. Geneva: World Health Organization.
Wiech, M., Chroscicki, P., Swatler, J., Stepnik, D., De Biasi, S., Hampel, M., et al. (2022). Remodeling of T cell dynamics during long COVID is dependent on severity of SARS-CoV-2 infection. Front. Immunol. 13, 886431. doi:10.3389/fimmu.2022.886431
Wu, T. J., Yu, A. C., and Lee, J. T. (2022). Management of post-COVID-19 olfactory dysfunction. Curr. Treat. Options Allergy 9, 1–18. doi:10.1007/s40521-021-00297-9
Xie, Y., and Al-Aly, Z. (2022). Risks and burdens of incident diabetes in long COVID: A cohort study. Lancet Diabetes and Endocrinol. 10 (5), 311–321. doi:10.1016/S2213-8587(22)00044-4
Xie, Y., Xu, E., Bowe, B., and Al-Aly, Z. (2022). Long-term cardiovascular outcomes of COVID-19. Nat. Med. 28 (3), 583–590. doi:10.1038/s41591-022-01689-3
Xu, X-W., Wu, X-X., Jiang, X-G., Xu, K-J., Ying, L-J., Ma, C-L., et al. (2020). Clinical findings in a group of patients infected with the 2019 novel coronavirus (SARS-Cov-2) outside of wuhan, China: Retrospective case series. bmj 2020, m606. doi:10.1136/bmj.m606
Yeoh, Y. K., Zuo, T., Lui, G. C-Y., Zhang, F., Liu, Q., Li, A. Y., et al. (2021). Gut microbiota composition reflects disease severity and dysfunctional immune responses in patients with COVID-19. Gut 70 (4), 698–706. doi:10.1136/gutjnl-2020-323020
Zayet, S., Klopfenstein, T., Mercier, J., Kadiane-Oussou, N. J., Lan Cheong Wah, L., Royer, P. Y., et al. (2021). Contribution of anosmia and dysgeusia for diagnostic of COVID-19 in outpatients. Infection 49 (2), 361–365. doi:10.1007/s15010-020-01442-3
Zhang, H., Zang, C., Xu, Z., Zhang, Y., Xu, J., Bian, J., et al. (2022). Data-driven identification of post-acute SARS-CoV-2 infection subphenotypes. Nat. Med. 29, 226–235. doi:10.1038/s41591-022-02116-3
Zhang, T., Mei, Q., Zhang, Z., Walline, J. H., Liu, Y., Zhu, H., et al. (2022). Risk for newly diagnosed diabetes after COVID-19: A systematic review and meta-analysis. BMC Med. 20 (1), 444. doi:10.1186/s12916-022-02656-y
Zhao, M., Chen, J., Tan, S., Dong, T., Jiang, H., Zheng, J., et al. (2018). Prolonged evolution of virus-specific memory T cell immunity after severe avian influenza A (H7N9) virus infection. J. virology 92 (17), 010244–e1118. doi:10.1128/JVI.01024-18
Keywords: SARS-CoV-2, long COVID, post-acute sequelae of SARS-CoV-2 infection, PASC, post COVID condition
Citation: Kenny G, Townsend L, Savinelli S and Mallon PWG (2023) Long COVID: Clinical characteristics, proposed pathogenesis and potential therapeutic targets. Front. Mol. Biosci. 10:1157651. doi: 10.3389/fmolb.2023.1157651
Received: 02 February 2023; Accepted: 04 April 2023;
Published: 26 April 2023.
Edited by:
Seyed Hassan Seradj, Shiraz University of Medical Sciences, IranReviewed by:
Lianhan Shang, China-Japan Friendship Hospital, ChinaShweta Jakhmola, University of California, San Diego, United States
Copyright © 2023 Kenny, Townsend, Savinelli and Mallon. This is an open-access article distributed under the terms of the Creative Commons Attribution License (CC BY). The use, distribution or reproduction in other forums is permitted, provided the original author(s) and the copyright owner(s) are credited and that the original publication in this journal is cited, in accordance with accepted academic practice. No use, distribution or reproduction is permitted which does not comply with these terms.
*Correspondence: Grace Kenny, Z3JhY2Uua2VubnkxQHVjZC5pZQ==