- 1Deparment of Neurology, The First Affiliated Hospital, China Medical University, Shenyang, China
- 2Clinical Department one, China Medical University, Shenyang, China
- 3Department of Endodontics, School of Stomatology, China Medical University, Shenyang, China
- 4Deparment of Geriatrics, Shengjing Hospital, China Medical University, Shenyang, China
Background and Purpose: Conclusions of previous cohort studies on the relationship between 25-hydroxyvitamin D level and the risk of dementia and Alzheimer's disease were not consistent. Thus, we performed a dose–response meta-analysis to evaluate this relationship by summarizing cohort studies.
Methods: Pubmed, Embase, Cochrane, and Web of Science databases were searched for relevant studies. Cohort studies concerning the association between 25-hydroxyvitamin D level and dementia or Alzheimer's disease were included. Results of studies were pooled and the dose–response relationship was determined using a random-effect model.
Results: Ten cohort studies, with 28,640 participants were included. A significant inverse relationship was found between 25-hydroxyvitamin D level and the risk of dementia and Alzheimer's disease. In addition, we found a linear dose–response relationship in that a 10 nmol/L increase in 25-hydroxyvitamin D level may lead to a 5% decrease in the risk of dementia (relative risk, 0.95; 95% confidence interval, 0.93–0.98) and 7% in the risk of Alzheimer's disease (relative risk, 0.93; 95% confidence interval, 0.89–0.97).
Conclusion: Plasma or serum 25-hydroxyvitamin D concentration was inversely related to the risk of dementia and Alzheimer's disease, consistent with a linear dose–response relationship.
Introduction
Despite being a common disease, dementia can neither be effectively prevented nor cured at present. With the prolongation of people's lives, the incidence of dementia is also increasing (Abbott, 2011). Nowadays, about 47 million people all over the world suffer from dementia, and the numbers will increase to 131 million by 2050 (Martin et al., 2016). More seriously, the death toll from dementia is high. Alzheimer's disease is the most common type of dementia—death from Alzheimer's disease accounts for 3.4% of all deaths in the United States, ranking it sixth (Kochanek et al., 2015). Therefore, it is of great importance to determine the cause of dementia and spare no effort to prevent it.
Vitamin D is a fat-soluble secosteroid which is generated mainly in our skin by ultraviolet B (UVB) sunlight, and can also be provided by diet (Webb, 2006; Kimlin, 2008; Cashman et al., 2017). Vitamin D is all hydroxylated to 25-hydroxyvitamin D [25(OH)D] in the liver regardless of the source. Thus, 25(OH)D is considered to reflect the vitamin D status in humans (Webb, 2006; Cashman et al., 2017). An increasing number of studies show that vitamin D has a neuroprotective effect (Naveilhan et al., 1996a,b; Brewer et al., 2001; Garcion et al., 2002; Masoumi et al., 2009; Briones and Darwish, 2012). Some cross-sectional studies and subsequent meta-analysis showed that the level of 25(OH)D in patients with Alzheimer's disease is lower than in healthy controls (Balion et al., 2012; Annweiler et al., 2013; Zhao et al., 2013). However, a cross-sectional study cannot determine a causal relationship between low 25(OH)D level and dementia or Alzheimer's disease. Although some cohort studies overcame this problem, their conclusions were not consistent. Some studies showed that low 25(OH)D level increased the risk of dementia and Alzheimer's disease (Annweiler et al., 2011; Afzal et al., 2014; Knekt et al., 2014; Littlejohns et al., 2014; Feart et al., 2017; Licher et al., 2017) but others did not (Graf et al., 2014; Schneider et al., 2014; Karakis et al., 2016; Olsson et al., 2017).
The meta-analysis of Shen and Ji (2015) showed an association between lower vitamin D status and increased risk of dementia and Alzheimer's disease, but a causal relationship cannot be confirmed because it included few cohort studies. Another meta-analysis (Sommer et al., 2017) found a similar result; however, this did not evaluate the risk of Alzheimer's disease. The recent meta-analysis by Jayedi et al. (2018) did not make full use of data because of restricting categories of 25(OH)D level. All previous analyses used the I2 statistic to describe and quantify the heterogeneity; however, Borenstein et al. (2017) showed that this is not an absolute measure of heterogeneity. Thus, we conducted a random-effects dose–response meta-analysis on cohort studies using prediction interval (PI) to describe the heterogeneity, with the hope of better exploring the relationship between 25(OH)D level and dementia and Alzheimer's disease.
Materials and Methods
Search Strategy
This meta-analysis was carried out according to the PRISMA guidelines and was registered in International Prospective Register of Systematic Reviews (number CRD42018085851; Moher et al., 2009).
We sought cohort studies about the association between the level of vitamin D and the risk of dementia or Alzheimer's disease by searching Pubmed, Embase, Cochrane, and Web of Science databases. Pubmed search terms were: (Alzheimer* OR dement*) AND (humans OR persons OR inpatients OR outpatients OR patients OR volunteers OR subjects OR participants) AND (vitamin D OR cholecalciferol OR ergocalciferol OR 25-hydroxyvitamin D) AND (cohort OR cohort studies [Mesh] OR risk [Mesh]). We also carried out manual retrieval using references from literature. No time or language restriction existed during the whole searching process.
Study Selection
Studies included had to meet the following criteria: (1) subjects were humans; (2) exposure was the level of 25(OH)D in plasma or serum; (3) outcome of the study was dementia or Alzheimer's disease and the diagnostic standards were provided; (4) dementia or Alzheimer's disease risk of different 25(OH)D levels was compared; (5) relative risk (RR), hazard ratio (HR) or odds ratio (OR) with 95% confidence intervals (95% CI) was provided; and (6) they were cohort studies. We excluded articles that met the following standards: (1) case–control or cross-sectional study; (2) letter, review, editorial or supplement; and (3) non-human study. If the same study was published repeatedly, we included the one with longer follow-up time.
Data Extraction and Quality Assessment
Two investigators were independently responsible for data extraction and study quality assessment. Disagreement was solved by asking a third investigator. The following information was extracted from included studies: authors, year of publication, location, study design, follow-up time, categories of 25(OH)D levels, number of participants, number of cases, age, and sex of the participants, adjusted factors, and RRs, HRs or ORs with 95% CI. The quality of studies was assessed based on the Newcastle–Ottawa scale (Wells et al., 2011), and studies with more than six stars were considered to be high quality.
Statistical Analysis
For all studies, we took RRs and 95% CI as effect size, both HRs and ORs were considered to be equivalent to RRs. The study stratified by sex or race was considered as two independent studies. For the study that the unit of 25(OH)D concentration was ng/ml, we multiplied the value to 2.5, converting the units into nmol/L (Holick, 2007; Ross et al., 2011). The 25(OH)D level was divided into different categories in different studies, and we extracted the mean or median 25(OH)D concentration of each category. If the mean or median was not provided, we extracted the midpoint of each category. If the upper boundary of the highest category was not provided, we considered 1.2 times of its lower boundary as the midpoint. If the lower boundary of the lowest category was not provided, we considered it to be 0 nmol/L.
We pooled the RRs for the highest vs. lowest category of 25(OH)D level in different studies using a random-effect model (DerSimonian and Laird, 2015). The publication bias was evaluated by funnel plots, Egger's test as well as Begg's test (Egger et al., 1997). Moreover, we estimated the potential non-linear trends between 25(OH)D level and the risk of dementia and Alzheimer's disease using restricted cubic spline models with three knots (10, 50, and 90%) (Durrleman and Simon, 1989). After calculating the p-value for non-linearity (Greenland and Longnecker, 1992), we evaluated the linear trend using the method of generalized least square (Orsini et al., 2012).
The Q-test statistic was chose to evaluate heterogeneity among studies (Higgins et al., 2003), with p-values < 0.10 considered to have significant heterogeneity. The I2 statistic was chose to evaluate the proportion of the variance resulting from heterogeneity in the observed variance and PI was also chosen to describe the heterogeneity (Borenstein et al., 2017).
Finally, we performed a subgroup analysis of the risk of dementia. This analysis was stratified by age, percentage of women, number of participants, number of cases, location, follow-up time, study quality, sample, and whether adjusted for season.
All analyses were conducted using STATA version 12.0 (StataCorp, TX) with a random-effect model. Significant level in this meta-analysis was set as 0.05.
Results
Study Characteristics
Our search identified 1,120 studies, of which 1,110 were excluded from, and ten were included in our meta-analysis (Annweiler et al., 2011; Afzal et al., 2014; Graf et al., 2014; Knekt et al., 2014; Littlejohns et al., 2014; Schneider et al., 2014; Karakis et al., 2016; Feart et al., 2017; Licher et al., 2017; Olsson et al., 2017) (Figure 1). These ten studies were published between 2011 and 2017, and had a total of 28,640 participants; the details of them are shown in Table 1. Three studies (Littlejohns et al., 2014; Schneider et al., 2014; Karakis et al., 2016) were conducted in the USA and seven (Annweiler et al., 2011; Afzal et al., 2014; Graf et al., 2014; Knekt et al., 2014; Feart et al., 2017; Licher et al., 2017; Olsson et al., 2017) in Europe. One study (Annweiler et al., 2011) was carried out for women and another for men (Olsson et al., 2017). In addition, one study (Knekt et al., 2014) reported information of men and women separately, and another (Schneider et al., 2014) reported blacks and whites separately. Because results stratified by sex or race were considered as two independent studies, we finally included 12 studies in our meta-analysis. The result of study quality assessment is shown in Table 2. All these studies were of high quality except one (Annweiler et al., 2011). The measurements of 25(OH)D level, diagnostic criteria for dementia and Alzheimer's disease, and the extracted RRs in these studies are shown in Supplementary Table S1.
25(OH)D Level and Risk of Dementia
All 12 studies were included in highest vs. lowest meta-analysis of the relationship between 25(OH)D level and risk of dementia. In one study (Afzal et al., 2014), the combined Alzheimer's disease and vascular dementia were treated as all-cause dementia. The pooled RR of dementia was 0.72 (95% CI: 0.59–0.88), without significant heterogeneity (p = 0.125, I2 = 33.2%, PI: 0.45–1.14; Figure 2). Egger's test (p = 0.102) and Begg's test (p = 0.115) showed no significant publication bias. Sensitivity analysis conducted by removing one study at a time and analyzing the rest showed that no single study influenced the pooled RRs (range 0.68–0.77).
In the next dose–response meta-analysis, we excluded three of the 12 studies. One of the studies divided the 25(OH)D level into only two categories (Annweiler et al., 2011), and the other two studies did not provide numbers of participants and cases in each category (Graf et al., 2014; Karakis et al., 2016). The non-linear association between 25(OH)D level and the risk of dementia was non-significant (p for non-linearity = 0.176). The linear trend showed that the risk of dementia decreased by 5% (RR: 0.95, 95% CI: 0.93–0.98, p = 0.000) for every 10 nmol/L increment in 25(OH)D level, without significant heterogeneity (p = 0.145; Figure 3).
25(OH)D Level and Risk of Alzheimer's Disease
Six studies (Annweiler et al., 2011; Afzal et al., 2014; Littlejohns et al., 2014; Karakis et al., 2016; Feart et al., 2017; Olsson et al., 2017) with data concerning the risk of Alzheimer's disease were included in the highest vs. lowest meta-analysis. The pooled RR of Alzheimer's disease was 0.78 (95% CI: 0.60–1.00), with significant heterogeneity (p = 0.041, I2 = 56.8%, PI: 0.39–1.54; Figure 4). The RRs after sensitivity analysis were in the range of 0.69–0.88. It is noteworthy that the effect became 0.88 (0.76–1.03) without heterogeneity (p = 0.287, I2 = 20.0%, PI: 0.61–1.31) when we removed one study (Feart et al., 2017) that had not adjusted for age.
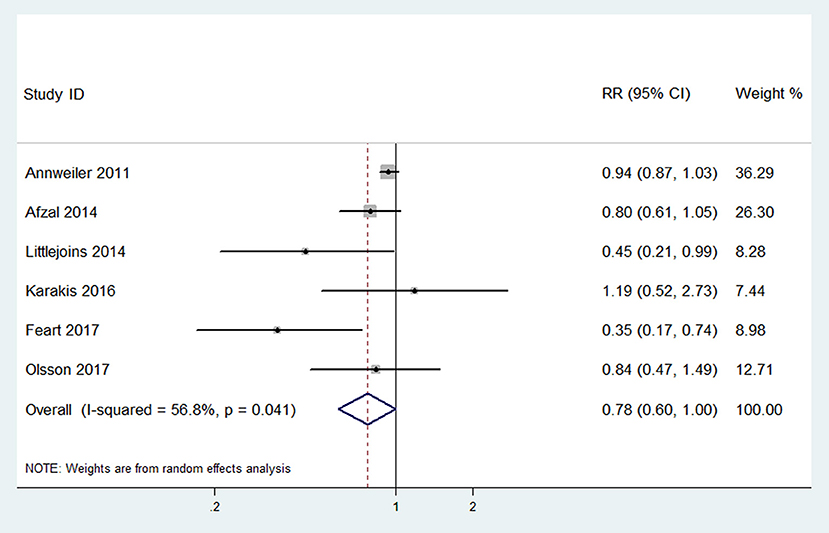
Figure 4. Forest plot of the highest vs. lowest meta-analysis of 25(OH)D level and the risk of Alzheimer's disease.
In the next dose–response meta-analysis, we excluded two (Annweiler et al., 2011; Karakis et al., 2016) of these six studies, because they did not provide enough categories of exposure or numbers of participants and cases in each category. The non-linear association between 25(OH)D level and the risk of Alzheimer's disease was non-significant (p for non-linearity = 0.804). The linear trend showed that the risk of Alzheimer's disease decreased by 7% (RR: 0.93, 95% CI: 0.89–0.97, p = 0.001) for every 10 nmol/L increment in 25(OH)D level without significant heterogeneity (p = 0.200; Figure 5).
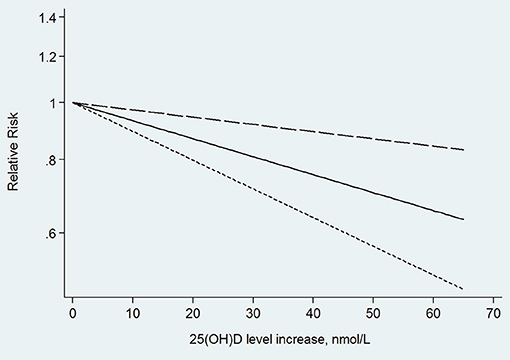
Figure 5. Linear trend of the relationship between 25(OH)D level increase and the risk of Alzheimer's disease.
Subgroup Analysis
The results of subgroup analysis are shown in Table 3. The associations between 25(OH)D level and the risk of dementia and Alzheimer's disease were consistent in different subgroups, which were defined by age, percentage of women, number of participants, number of cases, location, follow-up time, quality of studies, sample, and whether they were adjusted for season.
Discussion
In our meta-analysis, 25(OH)D level was inversely associated with the risk of dementia and Alzheimer's disease, and the subgroup analysis indicated a similar result. In addition, we found a linear dose–response relationship showing that a 10 nmol/L increase in 25(OH)D level may lead to a 5% decrease in dementia and 7% in Alzheimer's disease.
A previous meta-analysis (Shen and Ji, 2015) that included only three cross-sectional studies and two prospective cohort studies was not regarded as convincing evidence because there were too few participants, and was inadequate to determine any causal relationship between low 25(OH)D level and dementia or Alzheimer's disease. Two years later, a meta-analysis (Sommer et al., 2017) of five cohort studies showed that low 25(OH)D level may lead to a high risk of dementia. However, this analysis included studies published only in 2014. After that, four studies (Karakis et al., 2016; Feart et al., 2017; Licher et al., 2017; Olsson et al., 2017) of high quality appeared, and two (Karakis et al., 2016; Olsson et al., 2017) found no association between 25(OH)D level and dementia or Alzheimer's disease. A more recent meta-analysis (Jayedi et al., 2018) included only studies with more than three categories of 25(OH)D level and excluded information from studies with fewer categories of 25(OH)D level. Our meta-analysis included 28,640 participants and quantified the relationship between 25(OH)D level and dementia or Alzheimer's disease and provided a clearer understanding about 25(OH)D level and the risk of dementia or Alzheimer's disease.
Several mechanisms may explain the relationship between vitamin D and dementia and Alzheimer's disease. Vitamin D can play a neuroprotective role by up-regulating nerve growth factor, brain-derived neurotrophic factor and glial cell-derived neurotrophic factor (Naveilhan et al., 1996a,b; Garcion et al., 2002). Both animal and human studies have found that vitamin D can promote the removal of amyloid-β (Aβ; Masoumi et al., 2009; Briones and Darwish, 2012; Mizwicki et al., 2012). Vitamin D can also inhibit the expression of inducible nitric oxide synthase (Dursun et al., 2013). Furthermore, vitamin-D-binding protein can reduce Aβ aggregation and cell death induced by Aβ (Moon et al., 2013).
There are some strengths in our study. On the one hand, we included only cohort studies in our meta-analysis, and most were of high quality. Thus, selection bias and reverse causality were largely reduced. On the other hand, unlike previous meta-analyses, we assessed the relationship between 25(OH)D level and dementia or Alzheimer's disease with a dose–response model which enabled a better use of information. Moreover, we used PI to evaluate the heterogeneity in this random-effects meta-analysis. The PI ranged within 0.45–1.14 for risk of dementia and 0.39–1.54 for risk of Alzheimer's disease. Thus, it is possible that future studies will show an increased dementia and Alzheimer's disease risk in people with higher compared with lower 25(OH)D level.
However, our meta-analysis has several limitations. Firstly, we focused on the relationship between baseline 25(OH)D levels and the risk of dementia and Alzheimer's disease. It was unclear whether the 25(OH)D level had changed during the follow-up. However, this effect is likely to be small because studies have shown that 25(OH)D concentration can be relatively stable for a long period (Jorde et al., 2010; Sonderman et al., 2012). Secondly, all participants involved in our meta-analysis were Americans and Europeans, thus our findings may not apply to people elsewhere in the world. Thirdly, in studies included in our meta-analysis, the diagnostic criteria for dementia and Alzheimer's disease as well as measurements of 25(OH)D concentration were not completely unified and this may lead to some bias. However, diagnostic criteria such as NINCDS-ADRDA, DSM-IV, DSM-III, ICD-8, ICD-9, and ICD-10 are all well accepted and the measurements of 25(OH)D concentration were accurate. Considering the different test samples (plasma and serum) in these studies, we conducted a subgroup analysis and found similar results. Fourthly, there are several subtypes of dementia, such as Alzheimer's disease, vascular dementia and dementia with Lewy bodies. In our meta-analysis, the only dementia subtype was Alzheimer's disease, which is the most common subtype. The relationship between 25(OH)D and other subtypes of dementia remains to be discussed. Error in distinguishing different types of dementia may result in attenuation of the true association between 25(OH)D level and Alzheimer's disease. Moreover, the confounding factors adjusted in each study differed, and the risk factors for dementia were still not clear. Accordingly, there may be some risk factors to be adjusted. In this meta-analysis, we extracted RRs with most adjusted factors, and the study (Annweiler et al., 2011) without any adjusted factors was excluded in the dose–response analysis because of its categories of exposure. Lastly, due to the observational design of all the studies, the possibility of potential bias and residual confounding cannot be excluded.
Finally, because we found that a higher level of 25(OH)D could help to prevent dementia and Alzheimer's disease, ways to increase 25(OH)D level should be given attention. Approximately 80–100% of the vitamin D required by humans is produced by the UVB radiation in sunshine (Kimlin, 2008). However, at higher latitudes, UVB availability decreases and the number of months in which vitamin D cannot be effectively synthesized increases (O'Neill et al., 2016). Nevertheless, this does not mean that people in higher latitudes have a higher prevalence of vitamin D deficiency because diet, clothing, skin color as well as behavior habits also influence synthesis of vitamin D (Jablonski and Chaplin, 2012; O'Neill et al., 2016). Having more outdoor activities and eating more food rich in vitamin D such as fish (cod liver), meat, eggs and milk (Ovesen et al., 2003; Brustad et al., 2004; Hayes and Cashman, 2017) may help to improve vitamin D status. In addition, vitamin D supplementation and some vitamin D-fortified food can also improve vitamin D intake (O'Donnell et al., 2008; Black et al., 2012; Cashman and Kiely, 2016; Manios et al., 2017). Moreover, it is important to note that the safe upper intake level for vitamin D is 100 μg/day for adults, and long-term intake of too much vitamin D can be harmful (IOM, 2011; EFSA, 2012).
Conclusion
In summary, this dose–response meta-analysis showed that plasma or serum 25(OH)D level was inversely associated with the risk of dementia and Alzheimer's disease, consistent with a linear dose–response relationship. Maintaining adequate 25(OH)D status may lower risk of dementia and Alzheimer's disease.
Author Contributions
HC and WT designed the study. HC and LT searched the databases. WT and WX selected studies. JL and KF evaluated the quality of studies. HC and WT performed all analyses. HS and BZ wrote the manuscript. All authors revised the manuscript.
Conflict of Interest Statement
The authors declare that the research was conducted in the absence of any commercial or financial relationships that could be construed as a potential conflict of interest.
Acknowledgments
The work was supported by the Science and Technology Program Foundation of Liaoning Province (2014226033) and the Science and Technology Program Foundation of Shenyang (17-230-9-31, F16-206-9-03). We want to thank Dr. David J. Llewellyn and Dr Ilianna Lourida for generously providing related data for us.
Supplementary Material
The Supplementary Material for this article can be found online at: https://www.frontiersin.org/articles/10.3389/fnagi.2018.00368/full#supplementary-material
References
Afzal, S., Bojesen, S. E., and Nordestgaard, B. G. (2014). Reduced 25-hydroxyvitamin D and risk of Alzheimer's disease and vascular dementia. Alzheimers Dement. 10, 296–302. doi: 10.1016/j.jalz.2013.05.1765
Annweiler, C., Llewellyn, D. J., and Beauchet, O. (2013). Low serum vitamin D concentrations in Alzheimer's disease: a systematic review and meta-analysis. J. Alzheimers Dis. 33, 659–674. doi: 10.3233/jad-2012-121432
Annweiler, C., Rolland, Y., Schott, A. M., Blain, H., Vellas, B., and Beauchet, O. (2011). Serum vitamin D deficiency as a predictor of incident non-Alzheimer dementias: a 7-year longitudinal study. Dement. Geriatr. Cogn. Disord. 32, 273–278. doi: 10.1159/000334944
Balion, C., Griffith, L. E., Strifler, L., Henderson, M., Patterson, C., Heckman, G., et al. (2012). Vitamin D, cognition, and dementia: a systematic review and meta-analysis. Neurology 79, 1397–1405. doi: 10.1212/WNL.0b013e31826c197f
Black, L. J., Seamans, K. M., Cashman, K. D., and Kiely, M. (2012). An updated systematic review and meta-analysis of the efficacy of vitamin D food fortification. J. Nutr. 142, 1102–1108. doi: 10.3945/jn.112.158014
Borenstein, M., Higgins, J. P., Hedges, L. V., and Rothstein, H. R. (2017). Basics of meta-analysis: I2 is not an absolute measure of heterogeneity. Res. Synth. Methods 8, 5–18. doi: 10.1002/jrsm.1230
Brewer, L. D., Thibault, V., Chen, K. C., Langub, M. C., Landfield, P. W., and Porter, N. M. (2001). Vitamin D hormone confers neuroprotection in parallel with downregulation of L-type calcium channel expression in hippocampal neurons. J. Neurosci. 21, 98–108. doi: 10.1523/JNEUROSCI.21-01-00098.2001
Briones, T. L., and Darwish, H. (2012). Vitamin D mitigates age-related cognitive decline through the modulation of pro-inflammatory state and decrease in amyloid burden. J Neuroinflamm. 9:244. doi: 10.1186/1742-2094-9-244
Brustad, M., Alsaker, E., Engelsen, O., Aksnes, L., and Lund, E. (2004). Vitamin D status of middle-aged women at 65-71 degrees N in relation to dietary intake and exposure to ultraviolet radiation. Public Health Nutr. 7, 327–335. doi: 10.1079/phn2003536
Cashman, K. D., and Kiely, M. (2016). Tackling inadequate vitamin D intakes within the population: fortification of dairy products with vitamin D may not be enough. Endocrine 51, 38–46. doi: 10.1007/s12020-015-0711-x
Cashman, K. D., van den Heuvel, E. G., Schoemaker, R. J., Preveraud, D. P., Macdonald, H. M., and Arcot, J. (2017). 25-Hydroxyvitamin D as a biomarker of vitamin D status and its modeling to inform strategies for prevention of vitamin D deficiency within the population. Adv. Nutr. 8, 947–957. doi: 10.3945/an.117.015578
DerSimonian, R., and Laird, N. (2015). Meta-analysis in clinical trials revisited. Contemp. Clin. Trials 45(Pt A), 139–145. doi: 10.1016/j.cct.2015.09.002
Durrleman, S., and Simon, R. (1989). Flexible regression models with cubic splines. Stat. Med. 8, 551–561. doi: 10.1002/sim.4780080504
Dursun, E., Gezen-Ak, D., and Yilmazer, S. (2013). A new mechanism for amyloid-beta induction of iNOS: vitamin D-VDR pathway disruption. J. Alzheimers. Dis. 36, 459–474. doi: 10.3233/jad-130416
EFSA (2012). Scientific opinion on the tolerable upper intake level of vitamin D. EFSA J. 10:2813. doi: 10.2903/j.efsa.2012.2813
Egger, M., Davey Smith, G., Schneider, M., and Minder, C. (1997). Bias in meta-analysis detected by a simple, graphical test. BMJ 315, 629–634. doi: 10.1136/bmj.315.7109.629
Feart, C., Helmer, C., Merle, B., Herrmann, F. R., Annweiler, C., Dartigues, J.-F., et al. (2017). Associations of lower vitamin D concentrations with cognitive decline and long-term risk of dementia and Alzheimer's disease in older adults. Alzheimers Dement. 13, 1207–1216. doi: 10.1016/j.jalz.2017.03.003
Garcion, E., Wion-Barbot, N., Montero-Menei, C. N., Berger, F., and Wion, D. (2002). New clues about vitamin D functions in the nervous system. Trends Endocrinol. Metab. 13, 100–105. doi: 10.1016/S1043-2760(01)00547-1
Graf, C. E., Rossi, C., Giannelli, S. V., Nobari, B. H., Gold, G., Herrmann, F. R., et al. (2014). Vitamin D is not associated with cognitive status in a cohort of very old hospitalized patients. J. Alzheimers Dis. 42(Suppl. 3), S53–61. doi: 10.3233/jad-132612
Greenland, S., and Longnecker, M. P. (1992). Methods for trend estimation from summarized dose-response data, with applications to meta-analysis. Am. J. Epidemiol. 135, 1301–1309. doi: 10.1093/oxfordjournals.aje.a116237
Hayes, A., and Cashman, K. D. (2017). Food-based solutions for vitamin D deficiency: putting policy into practice and the key role for research. Proc. Nutr. Soc. 76, 54–63. doi: 10.1017/s0029665116000756
Higgins, J. P., Thompson, S. G., Deeks, J. J., and Altman, D. G. (2003). Measuring inconsistency in meta-analyses. BMJ 327, 557–560. doi: 10.1136/bmj.327.7414.557
Holick, M. F. (2007). Vitamin D deficiency. N. Engl. J. Med. 357, 266–281. doi: 10.1056/NEJMra070553
IOM (2011). Dietary Reference Intakes for Calcium and Vitamin D. Washington, DC: The National Academies Press.
Jablonski, N. G., and Chaplin, G. (2012). Human skin pigmentation, migration and disease susceptibility. Philos. Trans. R. Soc. Lond. B. Biol. Sci. 367, 785–792. doi: 10.1098/rstb.2011.0308
Jayedi, A., Rashidy-Pour, A., and Shab-Bidar, S. (2018). Vitamin D status and risk of dementia and Alzheimer's disease: a meta-analysis of dose-response. Nutr Neurosci. 2018, 1–10. doi: 10.1080/1028415x.2018.1436639
Jorde, R., Sneve, M., Hutchinson, M., Emaus, N., Figenschau, Y., and Grimnes, G. (2010). Tracking of serum 25-hydroxyvitamin D levels during 14 years in a population-based study and during 12 months in an intervention study. Am. J. Epidemiol. 171, 903–908. doi: 10.1093/aje/kwq005
Karakis, I., Pase, M. P., Beiser, A., Booth, S. L., Jacques, P. F., Rogers, G., et al. (2016). Association of serum vitamin D with the risk of incident dementia and subclinical indices of brain aging: the framingham heart study. J. Alzheimers. Dis. 51, 451–461. doi: 10.3233/jad-150991
Kimlin, M. G. (2008). Geographic location and vitamin D synthesis. Mol. Aspects Med. 29, 453–461. doi: 10.1016/j.mam.2008.08.005
Knekt, P., Saaksjarvi, K., Jarvinen, R., Marniemi, J., Mannisto, S., Kanerva, N., et al. (2014). Serum 25-hydroxyvitamin D concentration and risk of dementia. Epidemiology 25, 799–804. doi: 10.1097/EDE.0000000000000175
Kochanek, K. D., Murphy, S. L., and Xu, J. (2015). Deaths: final data for 2011. Natl. Vital Stat. Rep. 63, 1–120.
Licher, S., de Bruijn, R., Wolters, F. J., Zillikens, M. C., Ikram, M. A., and Ikram, M. K. (2017). Vitamin D and the risk of dementia: the rotterdam study. J. Alzheimers. Dis. 60, 989–997. doi: 10.3233/jad-170407
Littlejohns, T. J., Henley, W. E., Lang, I. A., Annweiler, C., Beauchet, O., Chaves, P. H. M., et al. (2014). Vitamin D and the risk of dementia and Alzheimer disease. Neurology 83, 920–928. doi: 10.1212/WNL.0000000000000755
Manios, Y., Moschonis, G., Mavrogianni, C., van den Heuvel, E., Singh-Povel, C. M., Kiely, M., et al. (2017). Reduced-fat Gouda-type cheese enriched with vitamin D3 effectively prevents vitamin D deficiency during winter months in postmenopausal women in Greece. Eur. J. Nutr. 56, 2367–2377. doi: 10.1007/s00394-016-1277-y
Martin, P., Adelina, C.-H., Martin, K., Maëlenn, G., and Maria, K. (2016). World Alzheimer Report 2016. London: Alzheimer's Disease International.
Masoumi, A., Goldenson, B., Ghirmai, S., Avagyan, H., Zaghi, J., Abel, K., et al. (2009). 1alpha,25-dihydroxyvitamin D3 interacts with curcuminoids to stimulate amyloid-beta clearance by macrophages of Alzheimer's disease patients. J. Alzheimers. Dis. 17, 703–717. doi: 10.3233/jad-2009-1080
Mizwicki, M. T., Menegaz, D., Zhang, J., Barrientos-Duran, A., Tse, S., Cashman, J. R., et al. (2012). Genomic and nongenomic signaling induced by 1alpha,25(OH)2-vitamin D3 promotes the recovery of amyloid-beta phagocytosis by Alzheimer's disease macrophages. J. Alzheimers. Dis. 29, 51–62. doi: 10.3233/jad-2012-110560
Moher, D., Liberati, A., Tetzlaff, J., and Altman, D. G. (2009). Preferred reporting items for systematic reviews and meta-analyses: the PRISMA statement. Ann Intern Med 151, 264–269, w264. doi: 10.7326/0003-4819-151-4-200908180-00135
Moon, M., Song, H., Hong, H. J., Nam, D. W., Cha, M. Y., Oh, M. S., et al. (2013). Vitamin D-binding protein interacts with Abeta and suppresses Abeta-mediated pathology. Cell Death Differ. 20, 630–638. doi: 10.1038/cdd.2012.161
Naveilhan, P., Neveu, I., Baudet, C., Funakoshi, H., Wion, D., Brachet, P., et al. (1996a). 1,25-Dihydroxyvitamin D3 regulates the expression of the low-affinity neurotrophin receptor. Brain Res. Mol. Brain Res. 41, 259–268. doi: 10.1016/0169-328X(96)00103-9
Naveilhan, P., Neveu, I., Wion, D., and Brachet, P. (1996b). 1,25-Dihydroxyvitamin D3, an inducer of glial cell line-derived neurotrophic factor. Neuroreport 7, 2171–2175. doi: 10.1097/00001756-199609020-00023
O'Donnell, S., Cranney, A., Horsley, T., Weiler, H. A., Atkinson, S. A., Hanley, D. A., et al. (2008). Efficacy of food fortification on serum 25-hydroxyvitamin D concentrations: systematic review. Am. J. Clin. Nutr. 88, 1528–1534. doi: 10.3945/ajcn.2008.26415
Olsson, E., Byberg, L., Karlstrom, B., Cederholm, T., Melhus, H., Sjogren, P., et al. (2017). Vitamin D is not associated with incident dementia or cognitive impairment: an 18-y follow-up study in community-living old men. American Journal of Clinical Nutrition 105, 936–943. doi: 10.3945/ajcn.116.141531
O'Neill, C. M., Kazantzidis, A., Ryan, M. J., Barber, N., Sempos, C. T., Durazo-Arvizu, R. A., et al. (2016). Seasonal changes in vitamin D-effective UVB availability in europe and associations with population serum 25-hydroxyvitamin D. Nutrients 8:9. doi: 10.3390/nu8090533
Orsini, N., Li, R., Wolk, A., Khudyakov, P., and Spiegelman, D. (2012). Meta-analysis for linear and nonlinear dose-response relations: examples, an evaluation of approximations, and software. Am. J. Epidemiol. 175, 66–73. doi: 10.1093/aje/kwr265
Ovesen, L., Brot, C., and Jakobsen, J. (2003). Food contents and biological activity of 25-hydroxyvitamin D: a vitamin D metabolite to be reckoned with? Ann. Nutr. Metab. 47, 107–113. doi: 10.1159/000070031
Ross, A. C., Manson, J. E., Abrams, S. A., Aloia, J. F., Brannon, P. M., Clinton, S. K., et al. (2011). The 2011 report on dietary reference intakes for calcium and vitamin D from the Institute of Medicine: what clinicians need to know. J. Clin. Endocrinol. Metab. 96, 53–58. doi: 10.1210/jc.2010-2704
Schneider, A. L. C., Lutsey, P. L., Alonso, A., Gottesman, R. F., Sharrett, A. R., Carson, K. A., et al. (2014). Vitamin D and cognitive function and dementia risk in a biracial cohort: the ARIC brain MRI study. Eur. J. Neurol. 21, 1211–1211e1270. doi: 10.1111/ene.12460
Shen, L., and Ji, H. F. (2015). Vitamin D deficiency is associated with increased risk of Alzheimer's disease and dementia: evidence from meta-analysis. Nutr. J. 14:76. doi: 10.1186/s12937-015-0063-7
Sommer, I., Griebler, U., Kien, C., Auer, S., Klerings, I., Hammer, R., et al. (2017). Vitamin D deficiency as a risk factor for dementia: a systematic review and meta-analysis. BMC Geriatr. 17:16. doi: 10.1186/s12877-016-0405-0.
Sonderman, J. S., Munro, H. M., Blot, W. J., and Signorello, L. B. (2012). Reproducibility of serum 25-hydroxyvitamin d and vitamin D-binding protein levels over time in a prospective cohort study of black and white adults. Am. J. Epidemiol. 176, 615–621. doi: 10.1093/aje/kws141
Webb, A. R. (2006). Who, what, where and when-influences on cutaneous vitamin D synthesis. Prog. Biophys. Mol. Biol. 92, 17–25. doi: 10.1016/j.pbiomolbio.2006.02.004
Wells, G., Shea, B., O'Connell, D., Peterson, J., Welch, V., Losos, M., et al. (2011). The Newcastle-Ottawa Scale (NOS) for Assessing the Quality of Nonrandomised Studies in Meta-Analyses [Online]. Available online at: http://www.ohri.ca/programs/clinical_epidemiology/oxford.asp.
Keywords: dementia, Alzheimer's disease, 25-hydroxyvitamin D, meta-analysis, cohort studies
Citation: Chen H, Xue W, Li J, Fu K, Shi H, Zhang B, Teng W and Tian L (2018) 25-Hydroxyvitamin D Levels and the Risk of Dementia and Alzheimer's Disease: A Dose–Response Meta-Analysis. Front. Aging Neurosci. 10:368. doi: 10.3389/fnagi.2018.00368
Received: 09 April 2018; Accepted: 23 October 2018;
Published: 09 November 2018.
Edited by:
Christian Gonzalez-Billault, Universidad de Chile, ChileReviewed by:
Yangmei Chen, Second Affiliated Hospital of Chongqing Medical University, ChinaSakineh Shab-Bidar, Tehran University of Medical Sciences, Iran
Copyright © 2018 Chen, Xue, Li, Fu, Shi, Zhang, Teng and Tian. This is an open-access article distributed under the terms of the Creative Commons Attribution License (CC BY). The use, distribution or reproduction in other forums is permitted, provided the original author(s) and the copyright owner(s) are credited and that the original publication in this journal is cited, in accordance with accepted academic practice. No use, distribution or reproduction is permitted which does not comply with these terms.
*Correspondence: Weiyu Teng, dGVuZ3dlaXl1Y211QHNpbmEuY29t
Li Tian, dGlhbmxpY211MkAxMjYuY29t