- 1Brain and Mind Research, Central European Institute of Technology, Masaryk University, Brno, Czechia
- 2Faculty of Medicine, Masaryk University, Brno, Czechia
- 3First Department of Neurology, Faculty of Medicine, St. Anne’s University Hospital, Masaryk University, Brno, Czechia
- 4Department of Health Promotion, Faculty of Sports Studies, Masaryk University, Brno, Czechia
- 5Department of Gymnastics and Combatives, Faculty of Sports Studies, Masaryk University, Brno, Czechia
Background: Dance is a complex activity combining physical exercise with cognitive, social, and artistic stimulation.
Objectives: We aimed to assess the effects of dance intervention (DI) on intra and inter-network resting-state functional connectivity (rs-FC) and its association to cognitive changes in a group of non-demented elderly participants.
Methods: Participants were randomly assigned into two groups: DI and life as usual (LAU). Six-month-long DI consisted of supervised 60 min lessons three times per week. Resting-state fMRI data were processed using independent component analysis to evaluate the intra and inter-network connectivity of large-scale brain networks. Interaction between group (DI, LAU) and visit (baseline, follow-up) was assessed using ANOVA, and DI-induced changes in rs-FC were correlated with cognitive outcomes.
Results: Data were analyzed in 68 participants (DI; n = 36 and LAU; n = 32). A significant behavioral effect was found in the attention domain, with Z scores increasing in the DI group and decreasing in the LAU group (p = 0.017). The DI as compared to LAU led to a significant rs-FC increase of the default mode network (DMN) and specific inter-network pairings, including insulo-opercular and right frontoparietal/frontoparietal control networks (p = 0.019 and p = 0.023), visual and language/DMN networks (p = 0.012 and p = 0.015), and cerebellar and visual/language networks (p = 0.015 and p = 0.003). The crosstalk of the insulo-opercular and right frontoparietal networks were associated with attention/executive domain Z-scores (R = 0.401, p = 0.015, and R = 0.412, p = 0.012).
Conclusion: The DI led to intervention-specific complex brain plasticity changes that were of cognitive relevance.
Introduction
Dance is a complex activity combining physical exercise with cognitive, social, and artistic stimulation (Burzynska et al., 2017). While the beneficial effect of dance intervention (DI) programs on physical fitness have been repeatedly proven, papers assessing the effects of DI on cognition have mixed results (Alpert et al., 2009; Coubard, 2011; Kim et al., 2011; Kattenstroth et al., 2013; Müller et al., 2017; Rehfeld et al., 2017, 2018; Brustio et al., 2018; Qi et al., 2018b). The effects of dance intervention on brain plasticity include various structural changes, such as an increase in gray matter volume (left hippocampus, left dentate gyrus, left precentral gyrus, etc.) as well as an increase in white matter integrity (fornix, corpus callosum), for a full review please see Teixeira-Machado et al. (2019). These findings are in concordance with our recent works, where 6-month-long DI as compared to life activities as usual (LAU) and resulted in significant improvement in a Five Point Test (FPT) which evaluated attention and executive functions (Kropacova et al., 2019), and in increases of cortical thickness of the right lateral occipitotemporal cortex (Rektorova et al., 2020) implicated in learning new skilled movements (Gatti et al., 2017). Physical fitness also significantly improved due to the 6-month DI, which was linked to changes in diffusion tensor imagining measures in the whole white matter skeleton and in the corticospinal tract and the superior longitudinal fascicle, which are engaged in motor learning and movement execution, as well as in spatial attention, manipulation of mental representations, and speech comprehension (Sejnoha Minsterova et al., 2020).
Brain connectivity changes following aerobic exercise of various types and intensities were studied by several authors. Increase of resting-state functional connectivity (rsFC) following aerobic exercise was often reported in the default mode network (DMN) and hippocampus and parahippocampal gyrus regions (Burdette et al., 2010; Rosano et al., 2010; Voss et al., 2010; Leavitt et al., 2014; Boraxbekk et al., 2016; Tozzi et al., 2016; Chirles et al., 2017; McGregor et al., 2018; Ikuta et al., 2019). A 6-month-long aerobic exercise intervention in MCI patients led to increased rsFC of the prefrontal cortex (Hugenschmidt et al., 2017), similarly, the aerobic exercise intervention of the same duration led to increased rsFC in the prefrontal cortex, as well as superior parietal gyrus/precuneus in older overweight adults compared to the control group (Prehn et al., 2019). Not only regular but also a single session of moderate aerobic exercise resulted in increased FC in the sensorimotor areas (pre/post central gyri, thalamus and secondary somatosensory area) of healthy young adults (Rajab et al., 2014). Similarly, a 30-min long exercise bout was reported to increase rsFC in the right fronto parietal network (rFPN), sensorimotor network (SMN), and right affect and reward and network (ARN) in male athletes (Schmitt et al., 2019). However, some studies reported limited or no effect of aerobic exercise on rsFC (Flodin et al., 2017; Cui et al., 2019).
Although dance, unlike simple aerobic activity, involves learning and attention, emotions, coordination and balance, acoustic stimulation and social interaction (Kshtriya et al., 2015) only one study to date (Qi et al., 2018b) used rs-fMRI to examine the effect of the intervention on FC. The results of this pilot 3-month-long aerobic dance intervention in older adults with MCI showed an increase in brain spontaneous activity (analysis of the amplitude of low-frequency fluctuations) in bilateral fronto temporal, hippocampal, entorhinal and anterior cingulate cortices, compared to baseline (Qi et al., 2018b).
Based on this promising preliminary finding, we decided to use fMRI and a data-driven independent componential analysis (ICA) approaches with a primary objective to assess the effect of DI on rsFC in a mixed group of healthy elderly with or without MCI but no dementia. We hypothesized that DI-induced task-specific changes in both within-network and between-network rsFC in the DI group compared to the LAU group. Our secondary objective was to evaluate the association between the DI-induced changes in rsFC and cognitive performance. Based on our previous results (Kropacova et al., 2019; Rektorova et al., 2020), we particularly focused on DI-induced changes in attention and executive function domains. We hypothesized that DI-induced brain plasticity changes will be linked with our cognitive outcomes of interest.
Methods
Study Participants and Intervention
Volunteers older than 60 were recruited using several sources, such as free advertising in community centers (senior centers, libraries), University of the Third Age courses, and in public media such as local newspapers and leaflets, etc. The exclusion criteria included serious brain injury, major psychiatric disorder or central nervous system disease, other serious neurological, orthopedic, oncological or internal disease disabling participation in the dance-exercise intervention, as well as any contraindications of MRI scans as followed in clinical practice. The abuse of alcohol, other addictive substances and smoking were other exclusion criteria. Volunteers practizing sport activities more frequently or at a more demanding level than the dance exercise intervention were not included in the study.
Participants were randomly assigned into two groups in a 1:1 ratio: DI (dance intervention) and LAU (life as usual) using the opaque envelope method. The study sample was estimated based on pilot data analysis, when a mean change of 0.5 points was observed in the five-point test score (Kropacova et al., 2019). Considering the type 1 error probability α of 0.05 and expecting the test power β of 0.8, and also considering a dropout rate of 25%, the minimum number of participants in one group was calculated to be 42. It should be noted that in the presented paper, the five point test is not included in the analysis. Participants who were eventually included in the analysis were those who completed the study protocol (at least 66.6% attendance of dance-exercise intervention in DI group, 2 fMRI scans, 2 detailed neuropsychological examinations before and after the intervention and physical fitness assessment).
Participants included in the DI group followed a 6-month-long dance exercise course taking place three times a week, the duration of a single lesson was 60 min and it took place at the Faculty of Sports Studies, Masaryk University in Brno. The dance exercise training was prepared and led by professionals from the faculty. The intervention included various dance types, e.g., Irish country, African, and Greek, etc., which were combined into a final choreography and performed at medium physical load intensity three times per week (Kropacova et al., 2019; Rektorova et al., 2020). Participants in the LAU group led normal lives.
Neuropsychological Examination
The complex neuropsychological testing evaluated global cognitive functions (Montreal Cognitive Assessment—MOCA) and five domains: memory, attention, executive functions, visuospatial functions, and language. Subjects who scored below –1.5 SD in two tests in at least one cognitive domain compared to normative data were categorized as having MCI. Activities of daily living and depressive symptoms were also evaluated to exclude subjects with dementia or depression (Kropacova et al., 2019). For further details, see the Supplementary Material.
MRI Data Acquisition and Preprocessing
All subjects were scanned in 3T Siemens Prisma MR scanner (Siemens Corp., Erlangen, Germany) at the Central European Institute of Technology (CEITEC), Masaryk University in Brno, using the following sequences: magnetization-prepared rapid gradient-echo (MPRAGE) high-resolution T1-weighted images (240 sagittal slices, slice thickness = 1 mm, TR = 2,300 ms, TE = 2.34 ms, FA = 8°, FOV = 224 mm, matrix size 224 × 224) and gradient-echo echo-planar imaging sequence for resting state fMRI (200 images, 34 transversal slices, slice thickness = 3.5 mm, TR = 1,990 ms, TE = 35 ms, FA = 70°, FOV = 192 mm, matrix size 64 × 64).
The resting state fMRI data were preprocessed using the SPM 12 toolbox and Matlab 2014b. Preprocessing included realignment and unwarping, normalization into standard anatomical space (MNI) and spatial smoothing with 5 mm FWHM. The level of motion was thoroughly checked in terms of frame-wise displacement (FD) (Power et al., 2012). No FD was higher than 3 mm and scans that displayed FD > 0.75 mm were scrubbed (Power et al., 2012). No more than 2.5% of subject scans were removed. Moreover, the six movement regressors (obtained during realignment and unwarping), FD and extracted signals from white matter and cerebrospinal fluid were regressed out of the data in subsequent analysis. Assessment of functional connectivity changes using independent component analysis (ICA).
Regarding the rs-fMRI data analyses, we utilized the data-driven approach using independent component analysis (ICA) methods. The intra-network and inter-network connectivity (intra-NC and inter-NC) of major large-scale brain networks were also evaluated, see the details below.
The data from all subjects (n = 68, 36 DI and 32 LAU) and both sessions (a total of 136 fMRI datasets) were entered into single group spatial Independent Component Analysis (gsICA) (Calhoun et al., 2001) implemented in the GIFT toolbox running under MATLAB. At first, each dataset was further preprocessed to remove the mean per each voxel time series and reduced by Principal Component Analysis (PCA) with the number of components set to the maximum Minimum Description Length (MDL) estimate for all datasets (Li et al., 2007). Then, a second PCA reduction was applied to all PCA components from all datasets with the number of components set to median over MDL estimates. Finally, the Infomax algorithm and ICASSO framework (Himberg et al., 2004) was used to derive maximally spatially group-specific independent components and the GICA algorithm was used to render dataset-specific spatial maps and time-series (20 runs with random initialization, minimal cluster size 16, maximal cluster size 24). We visually inspected spatial maps of the resulting components and those that represent functional networks were used for ensuing intra-NC analysis and the respective back-reconstructed time-series were used to evaluate inter-NC connectivity.
Experimental Design and Statistical Analysis
The study was completed between September 2015 and June 2018 and was divided into three parts. In September and October, the participants underwent neuropsychological and physical assessment and fMRI data were acquired, the data acquisition took place at CEITEC, Masaryk University, and at Faculty of Sports Studies, Masaryk University in Brno.
The intervention itself took place from November to April. In May and June, the re-assessments were performed in both groups.
Two sample t-test and Fischer Exact tests were used to compare the demographic data and cognitive performance between the two groups before the intervention. The effect of the intervention on cognitive performance was tested using ANOVA with three factors (“subject,” “group”—DI or LAU, and “visit”—before and after intervention), with age, sex and education as covariates.
Intra-Network Connectivity Analysis
For intra-network connectivity analysis, the groups at baseline were first compared using ANOVA, with a group (DI or LAU) as a factor. Then, the group differences between “before” and “after” intervention were compared using ANOVA, with three factors: group (DI or LAU), subject and visit (before, after), and an interaction group x visit. Both models included three nuisance covariates (age, gender and years of education). Both models were analyzed for each selected component and the analysis included only voxels significantly active within the given network. Altogether, 20% of voxels with the highest absolute value were included in the analysis. The significance level was set to p < 0.05 FWE corrected (cluster level, initial cut p = 0.001 uncorrected) corrected for multiple comparisons using Random Field Theory (Nichols and Hayasaka, 2003).
Inter-Network Connectivity Analysis
For inter-network connectivity analysis, subject-specific static functional connectivity between all pairs of selected ICA components (correlation coefficient) was calculated based on the components’ time series. The resulting correlation coefficients were transformed into Z-scores using Fisher Z-transform. Again, the groups at baseline were first compared using ANOVA with a single factor—group (DI or LAU). The group differences between “before” and “after” intervention were compared using ANOVA, with three factors: group (DI or LAU), subject and visit (before, after) and an interaction group x visit. The main effect of all three factors and interaction of group (DI or LAU) and “visit” (before, after) was modeled. For both models, three covariates were included (age, gender and years of education). Both models were calculated for each connection (altogether 36 connections, 9 components between each other). The significance level was set to p < 0.05 with Bonferroni corrections on two contrasts (p < 0.025, 2 × main effect of a factor “group” (DI or LAU) or 2 × interaction “group” X “visit”).
All ANOVAs with three factors included the “subject” factor, which modeled an effect of repeated measures. In case of any significant group vs. visit results, we also performed a correlation between changes in the rs-FC and cognitive domain z-scores in the DI group. The connectivity changes were corrected for age, sex and education.
Results
Participant Characteristics
Altogether, 147 subjects were recruited, 120 were included in the study after reviewing the inclusion and exclusion criteria. Eleven participants included in the DI group did not meet the criterion of the minimum attendance (66.6%), those whose attendance was lower than 10% were offered to be moved to the LAU group (6 participants). Eleven participants of the LAU group were lost to follow-up.
In the present study, we analyzed data from 68 subjects from the final cohort who had good quality fMRI data, 36 DI participants and 32 LAU participants. There were no differences in age, education and MOCA scores (two sample t-test, p < 0.05) and men/women ratio (Fischer Exact test, chi square = 1.24, p = 0.265) between the two groups. A significant difference between the groups was found for gender distribution (Fischer Exact test, chi square = 3.95, p = 0.047). Both DI and LAU were heterogeneous groups consisting of both healthy controls (HC) and subjects with mild cognitive impairment (MCI). There was no difference between the DI and LAU groups in healthy seniors (HS) and MCI ratio.
Cognitive Outcomes
The results of cognitive examinations are summarized in Supplementary Table 1. There were no differences in cognitive domain Z scores between the groups before the intervention (two sample t-test, p < 0.05) (see Table 1).
The interaction of factors “group” and “visit” was significant for the attention domain, with Z scores increasing in the DI group and decreasing in the LAU group (F = 6.00, p = 0.017) (see Supplementary Figure 2).
Independent Componential Analysis Resting-State fMRI Results
Altogether 20 components were identified using the Independent Component Analysis. We visually inspected the spatial maps of the components and for further analysis of inter and intra-network connectivity, we selected those representing functional networks—cerebellum, DMN, visual network, right and left frontoparietal network, language network, salience (insulo-opercular) network, frontoparietal control network and sensory-motor network (see Supplementary Figure 1).
Dance Intervention-Induced Changes in Intra-Network Connectivity
There were no significant differences in intra-network connectivities between DI and LAU groups at baseline. We observed significant interaction “group” x “visit” for the DMN. Based on extracted average values, the connectivity increases in the DI group and decreases in the LAU group (ANOVA, p = 0.048 FWE). The rs-FC in the precuneus (MNI coordinate –9, –58, 25) increased in the DI group and it decreased in the LAU group (p = 0.048) (see Supplementary Figure 3).
Dance Intervention-Induced Changes in Inter-Network Connectivity
There were three significant differences in inter-NC between the DI and LAU groups before the intervention, in all three the connectivity was higher in the LAU group compared to the DI group (cerebellum –visual network—p = 0.0088, cerebellum—language—p = 0.01, language—sensory-motor network—p = 0.0218).
We found six significant results for the group vs. visit interaction. Based on the extracted average values, the inter-NC increased in the DI group (five results) and decreased in the LAU group (one result). The inter-NC between the networks decreased significantly more in LAU than in the DI group (see Table 2 and Figure 1). Briefly, DI, when compared to LAU, induced increases in the crosstalk between the insulo-opercular and right frontoparietal/frontoparietal control networks (p = 0.019 and p = 0.023), visual and language/DMN networks (p = 0.012 and p = 0.015), and cerebellar and visual/language networks (p = 0.015 and p = 0.003).
Association Between Resting-State Functional Connectivity Changes and Cognitive Outcomes of Interest
The DI-induced changes in inter-NC between the right frontoparietal network and salience network significantly correlated with changes in attention domain z-scores (R = 0.402, p = 0.015) and executive function domains (R = 0.412, p = 0.012) in the DI group (see Figures 2A–C).
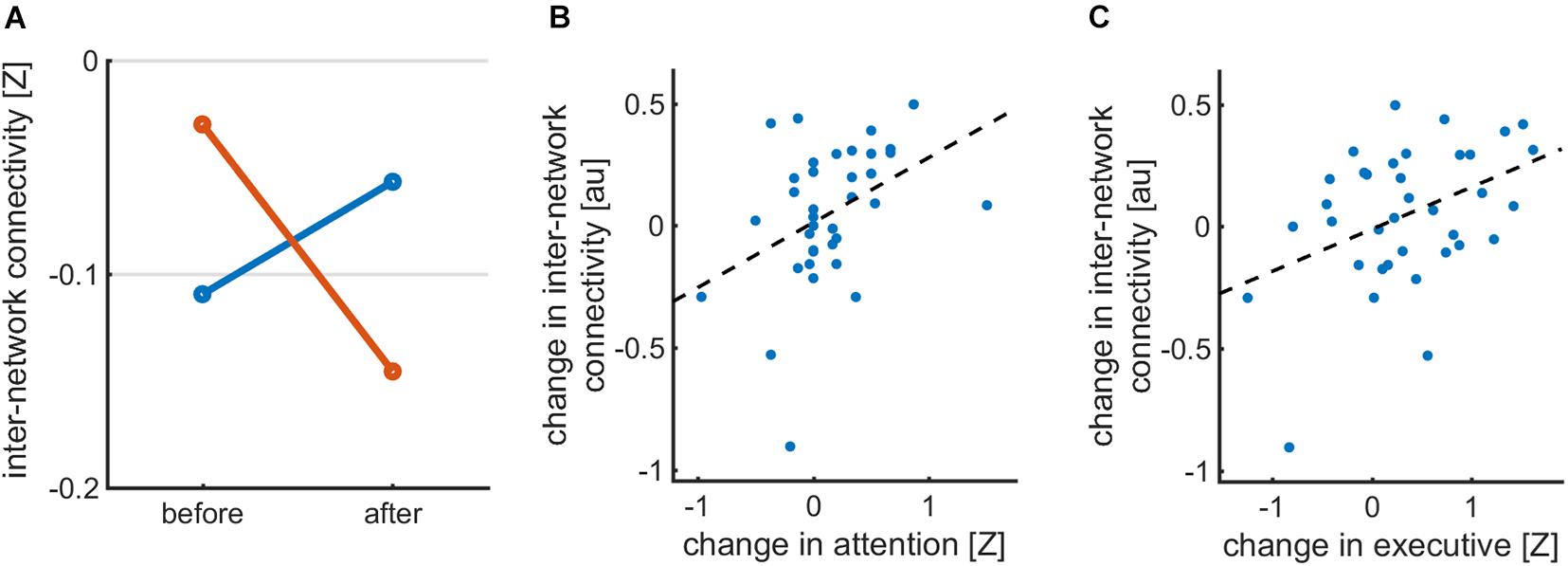
Figure 2. (A) Inter-network connectivity between RFPN and SAL networks in DI and LAU groups before and after the intervention (B) correlation between inter-NC between FRP and SAL in DI group and z-scores in attention domain (C) correlation between inter-NC between FRP and SAL in DI group and z-scores in executive domain.
Discussion
The significant behavioral effect of DI was found in the attention domain, which is in concordance with previous research (Diamond, 2015; Xiong et al., 2021) and our other research, which was performed in the same final cohort with a larger sample size (Kropacova et al., 2019). The current cohort remains smaller due to discarding data from participants with motion artifacts during fMRI scanning.
Our main goal was to assess brain rsFC changes that reflect DI-induced ability to enhance the recruitment of relevant cognitive large-scale brain networks and/or communication between them. For this purpose, we analyzed rsFC changes within specific networks as well as those of individual network pairings. We observed group vs. visit interaction for the DMN intra-network connectivity which increased in the DI group and decreased in the LAU group. DMN is a main resting state cognitive network with high degrees of functional connectivity (Greicius et al., 2003). Reduced rsFC within DMN has been described in both normal and pathological aging (such as in MCI or Alzheimer’s disease), with DMN having a strong correlation with cognitive processes (Agosta et al., 2012; Krajcovicova et al., 2014; Vidal-Piñeiro et al., 2014; La et al., 2015; Qi et al., 2018a; Zonneveld et al., 2019). The positive effect of exercise, both short and long-term, on the plasticity of DMN, has already been described by several studies (Boraxbekk et al., 2016; Li et al., 2017; McGregor et al., 2018).
We found more significant results for the DI-induced rs-FC enhancement of the inter-network crosstalk than of the intra-network connectivity. This finding may relate to the fact that aging is associated with the network de-differentiation and enhanced inter-network pairing, which is one of the well-described neural compensatory mechanisms for decreased modularity and network segregation with age (Geerligs et al., 2015). While several significant results have been found for the group vs. visit interaction, only the inter-network rsFC increases between right frontoparietal network and salience (insulo-opecular) network in the DI group were associated with our cognitive outcome measures, i.e., with z-scores in attention and executive function domains. The salience network comprises anterior cingulate and ventral anterior insular cortices, bilateral Rolandic opercula, with nodes in the amygdala, thalamus, hypothalamus, ventral striatum and specific brainstem nuclei (Menon, 2015; Blefari et al., 2017; Seeley, 2019). The right FP network is located within the right frontal and parietal cortices. Both salience/fronto-opercular and right FP networks (the latter is also referred to as the ventral attention network (Farrant and Uddin, 2015) are engaged in the control of salient (i.e., behaviorally relevant) stimuli processing from internal (interoceptive awareness and bodily self-consciousness) as well as external (outside world) environment (Blefari et al., 2017; Seeley, 2019). They also play important roles in the control of working memory (Wallis et al., 2015). ACC is repeatedly associated with executive functions (Carter et al., 1999), the anterior insula is also functionally connected with frontal regions implicated in executive functions (Eckert et al., 2009). The finding that the DI enhanced this specific inter-network crosstalk related to attention to behaviorally relevant stimuli and to executive functions is novel and future studies should assess the long-term cognitive and behavioral sequelae of these changes.
We also identified increased rsFC between the cerebellum and visual/language networks, and between DMN and visual/language networks. Some of these results have to be taken with caution because of the significant differences in the inter-network connectivity strength between DI and LAU groups already at baseline. On the whole, the abovementioned networks are known to be engaged in movement coordination, and visual and speech processing (Solé et al., 2010; van den Heuvel and Hulshoff Pol, 2010; Manto et al., 2012), thus supporting the notion that DI is an enjoyable multimodal cognitive, movement and social activity that modulates brain plasticity in a specific behaviorally relevant manner.
Study Limitations
One of the study limitations of this study is that there was no active control for the DI group, who would perform, e.g., aerobic activity, such as jogging (Müller et al., 2017). Moreover, due to the low number of MCI subjects in both DI and LAU groups, we could not perform analyses for these groups separately.
In conclusion, dance is a multimodal activity that led to complex intervention-specific brain plasticity changes that were of cognitive relevance.
Data Availability Statement
The original contributions presented in the study are included in the article/Supplementary Material, further inquiries can be directed to the corresponding author/s.
Ethics Statement
The studies involving human participants were reviewed and approved by the Masaryk University Ethical Committee. The patients/participants provided their written informed consent to participate in this study.
Author Contributions
IR and ZB contributed to conception, design of the study, and wrote the first draft of the manuscript. ZB, L’N, NN-E, SK, LB, RG, PV, and LS participated in data acquisition. RG, PV, and LS were responsible for DI program preparation and evaluation. RM performed statistical analysis of data. L’N, RM, and IR wrote sections of the manuscript. All authors contributed to manuscript revision, read, and approved the submitted version.
Funding
This study was supported by the grant project of the Agency of Health Research AZV 15-33854A and by the European Regional Development Fund-Project “National infrastructure for biological and medical imaging” CZ.02.1.01/0.0/0.0/16_013/0001775. This project has received funding from the European Union’s Horizon 2020 Research and Innovation Programme under the Marie Skłodowska-Curie grant agreement No. 734718 (CoBeN).
Conflict of Interest
The authors declare that the research was conducted in the absence of any commercial or financial relationships that could be construed as a potential conflict of interest.
Publisher’s Note
All claims expressed in this article are solely those of the authors and do not necessarily represent those of their affiliated organizations, or those of the publisher, the editors and the reviewers. Any product that may be evaluated in this article, or claim that may be made by its manufacturer, is not guaranteed or endorsed by the publisher.
Acknowledgments
We acknowledge the core facility MAFIL supported by the Czech-BioImaging large RI project (LM2018129 funded by MEYS CR) for their support with obtaining the scientific data presented in this paper.
Supplementary Material
The Supplementary Material for this article can be found online at: https://www.frontiersin.org/articles/10.3389/fnagi.2021.724064/full#supplementary-material
References
Agosta, F., Pievani, M., Geroldi, C., Copetti, M., Frisoni, G. B., and Filippi, M. (2012). Resting state fMRI in Alzheimer’s disease: beyond the default mode network. Neurobiol. Aging 33, 1564–1578. doi: 10.1016/j.neurobiolaging.2011.06.007
Alpert, P. T., Miller, S. K., Wallmann, H., Havey, R., Cross, C., Chevalia, T., et al. (2009). The effect of modified jazz dance on balance, cognition, and mood in older adults. J. Am. Acad. Nurse Pract. 21, 108–115. doi: 10.1111/j.1745-7599.2008.00392.x
Blefari, M. L., Martuzzi, R., Salomon, R., Bello-Ruiz, J., Herbelin, B., Serino, A., et al. (2017). Bilateral Rolandic operculum processing underlying heartbeat awareness reflects changes in bodily self-consciousness. Eur. J. Neurosci. 45, 1300–1312. doi: 10.1111/ejn.13567
Boraxbekk, C.-J., Salami, A., Wåhlin, A., and Nyberg, L. (2016). Physical activity over a decade modifies age-related decline in perfusion, gray matter volume, and functional connectivity of the posterior default-mode network—A multimodal approach. NeuroImage 131, 133–141. doi: 10.1016/j.neuroimage.2015.12.010
Brustio, P. R., Liubicich, M. E., Chiabrero, M., and Rabaglietti, E. (2018). Dancing in the golden age: a study on physical function, quality of life, and social engagement. Geriatr. Nurs. 39, 635–639. doi: 10.1016/j.gerinurse.2018.04.013
Burdette, J. H., Laurienti, P. J., Espeland, M. A., Morgan, A., Telesford, Q., Vechlekar, C. D., et al. (2010). Using network science to evaluate exercise-associated brain changes in older adults. Front. Ag. Neurosci. 2:23. doi: 10.3389/fnagi.2010.00023
Burzynska, A. Z., Finc, K., Taylor, B. K., Knecht, A. M., and Kramer, A. F. (2017). The Dancing brain: structural and functional signatures of expert dance training. Front. Hum. Neurosci. 11:566. doi: 10.3389/fnhum.2017.00566
Calhoun, V. D., Adali, T., Pearlson, G. D., and Pekar, J. J. (2001). A method for making group inferences from functional MRI data using independent component analysis. Hum. Brain Mapp. 14, 140–151.
Carter, C. S., Botvinick, M. M., and Cohen, J. D. (1999). The contribution of the anterior cingulate cortex to executive processes in cognition. Rev. Neurosci. 10, 49–57. doi: 10.1515/REVNEURO.1999.10.1.49
Chirles, T. J., Reiter, K., Weiss, L. R., Alfini, A. J., Nielson, K. A., and Smith, J. C. (2017). Exercise training and functional connectivity changes in mild cognitive impairment and healthy elders. J. Alzheimers Dis. 57, 845–856. doi: 10.3233/JAD-161151
Coubard, O. (2011). Practice of contemporary dance improves cognitive flexibility in aging. Front. Ag. Neurosci 3:13. doi: 10.3389/fnagi.2011.00013
Cui, L., Yin, H., Lyu, S., Shen, Q., Wang, Y., Li, X., et al. (2019). Tai chi chuan vs general aerobic exercise in brain plasticity: a multimodal mri study. Sci. Rep. 9:17264. doi: 10.1038/s41598-019-53731-z
Diamond, A. (2015). Effects of physical exercise on executive functions: going beyond simply moving to moving with thought. Ann. Sports Med. Res. 2:1011.
Eckert, M. A., Menon, V., Walczak, A., Ahlstrom, J., Denslow, S., Horwitz, A., et al. (2009). At the heart of the ventral attention system: the right anterior insula. Hum. Brain Mapp. 30, 2530–2541. doi: 10.1002/hbm.20688
Farrant, K., and Uddin, L. Q. (2015). Asymmetric development of dorsal and ventral attention networks in the human brain. Dev. Cogn. Neurosci. 12, 165–174. doi: 10.1016/j.dcn.2015.02.001
Flodin, P., Jonasson, L. S., Riklund, K., Nyberg, L., and Boraxbekk, C. J. (2017). Does Aerobic exercise influence intrinsic brain activity? An aerobic exercise intervention among healthy old adults. Front. Aging Neurosci. 9:267. doi: 10.3389/fnagi.2017.00267
Gatti, R., Rocca, M. A., Fumagalli, S., Cattrysse, E., Kerckhofs, E., Falini, A., et al. (2017). The effect of action observation/execution on mirror neuron system recruitment: an fMRI study in healthy individuals. Brain Imaging Behav. 11, 565–576. doi: 10.1007/s11682-016-9536-3
Geerligs, L., Renken, R. J., Saliasi, E., Maurits, N. M., and Lorist, M. M. (2015). A brain-wide study of age-related changes in functional connectivity. Cereb. Cortex 25, 1987–1999. doi: 10.1093/cercor/bhu012
Greicius, M. D., Krasnow, B., Reiss, A. L., and Menon, V. (2003). Functional connectivity in the resting brain: a network analysis of the default mode hypothesis. Proc. Natl. Acad. Sci.U.S.A. 100, 253–258. doi: 10.1073/pnas.0135058100
Himberg, J., Hyvärinen, A., and Esposito, F. (2004). Validating the independent components of neuroimaging time series via clustering and visualization. NeuroImage 22, 1214–1222. doi: 10.1016/j.neuroimage.2004.03.027
Hugenschmidt, C. E., Johnston, M. E., Jung, Y., Kraft, R. A., Laurienti, P. J., Whitlow, C. T., et al. (2017). Effects of aerobic exercise on functional connectivity of prefrontal cortex in mci: results of a randomized controlled trial. Alzheimers Dement. 13, 569–570. doi: 10.1016/j.jalz.2017.07.184
Ikuta, T., Frith, E., Ponce, P., and Loprinzi, P. D. (2019). Association of physical activity on the functional connectivity of the hippocampal–orbitofrontal pathway. Phys. Sportsmed. 47, 290–294. doi: 10.1080/00913847.2018.1549461
Kattenstroth, J.-C., Kalisch, T., Holt, S., Tegenthoff, M., and Dinse, H. R. (2013). Six months of dance intervention enhances postural, sensorimotor, and cognitive performance in elderly without affecting cardio-respiratory functions. Front. Aging Neurosci. 5:5. doi: 10.3389/fnagi.2013.00005
Kim, S.-H., Kim, M., Ahn, Y.-B., Lim, H.-K., Kang, S.-G., Cho, J.-H., et al. (2011). Effect of dance exercise on cognitive function in elderly patients with metabolic syndrome: a pilot study. J. Sports Sci. Med. 10, 671–678.
Krajcovicova, L., Marecek, R., Mikl, M., and Rektorova, I. (2014). Disruption of resting functional connectivity in Alzheimer’s patients and at-risk subjects. Curr. Neurol. Neurosci. Rep. 14:491. doi: 10.1007/s11910-014-0491-3
Kropacova, S., Mitterova, K., Klobusiakova, P., Brabenec, L., Anderkova, L., Nemcova-Elfmarkova, N., et al. (2019). Cognitive effects of dance-movement intervention in a mixed group of seniors are not dependent on hippocampal atrophy. J Neural Transm 126, 1455–1463. doi: 10.1007/s00702-019-02068-y
Kshtriya, S., Barnstaple, R., Rabinovich, D. B., and DeSouza, J. F. X. (2015). Dance and aging: a critical review of findings in neuroscience. Am. J. Dance Ther. 37, 81–112. doi: 10.1007/s10465-015-9196-7
La, C., Mossahebi, P., Nair, V. A., Bendlin, B. B., Birn, R., Meyerand, M. E., et al. (2015). Age-related changes in inter-network connectivity by component analysis. Front. Aging Neurosci. 7:237. doi: 10.3389/fnagi.2015.00237
Leavitt, V. M., Cirnigliaro, C., Cohen, A., Farag, A., Brooks, M., Wecht, J. M., et al. (2014). Aerobic exercise increases hippocampal volume and improves memory in multiple sclerosis: preliminary findings. Neurocase 20, 695–697. doi: 10.1080/13554794.2013.841951
Li, M., Huang, M., Li, S., Tao, J., Zheng, G., and Chen, L. (2017). The effects of aerobic exercise on the structure and function of DMN-related brain regions: a systematic review. Int. J. Neurosci. 127, 634–649. doi: 10.1080/00207454.2016.1212855
Li, Y.-O., Adalı, T., and Calhoun, V. D. (2007). Estimating the number of independent components for functional magnetic resonance imaging data. Hum. Brain Mapp. 28, 1251–1266. doi: 10.1002/hbm.20359
Manto, M., Bower, J. M., Conforto, A. B., Delgado-García, J. M., da Guarda, S. N. F., Gerwig, M., et al. (2012). Consensus paper: roles of the cerebellum in motor control—the diversity of ideas on cerebellar involvement in movement. Cerebellum 11, 457–487. doi: 10.1007/s12311-011-0331-9
McGregor, K. M., Crosson, B., Krishnamurthy, L. C., Krishnamurthy, V., Hortman, K., Gopinath, K., et al. (2018). Effects of a 12-week aerobic spin intervention on resting state networks in previously sedentary older adults. Front. Psychol. 9:2376. doi: 10.3389/fpsyg.2018.02376
Müller, P., Rehfeld, K., Schmicker, M., Hökelmann, A., Dordevic, M., Lessmann, V., et al. (2017). Evolution of neuroplasticity in response to physical activity in old age: the case for dancing. Front. Aging Neurosci. 9:56. doi: 10.3389/fnagi.2017.00056
Nichols, T., and Hayasaka, S. (2003). Controlling the familywise error rate in functional neuroimaging: a comparative review. Stat. Methods Med. Res. 12, 419–446. doi: 10.1191/0962280203sm341ra
Power, J. D., Barnes, K. A., Snyder, A. Z., Schlaggar, B. L., and Petersen, S. E. (2012). Spurious but systematic correlations in functional connectivity MRI networks arise from subject motion. NeuroImage 59, 2142–2154. doi: 10.1016/j.neuroimage.2011.10.018
Prehn, K., Lesemann, A., Krey, G., Witte, A. V., Köbe, T., Grittner, U., et al. (2019). Using resting-state fMRI to assess the effect of aerobic exercise on functional connectivity of the DLPFC in older overweight adults. Brain Cogn. 131, 34–44. doi: 10.1016/j.bandc.2017.08.006
Qi, M., Zhu, Y., Zhang, L., Wu, T., and Wang, J. (2018b). The effect of aerobic dance intervention on brain spontaneous activity in older adults with mild cognitive impairment: a resting-state functional MRI study. Exp. Ther. Med. 17, 715–722. doi: 10.3892/etm.2018.7006
Qi, H., Liu, H., Hu, H., He, H., and Zhao, X. (2018a). Primary disruption of the memory-related subsystems of the default mode network in Alzheimer’s disease: resting-state functional connectivity MRI study. Front. Aging Neurosci. 10:344. doi: 10.3389/fnagi.2018.00344
Rajab, A. S., Crane, D. E., Middleton, L. E., Robertson, A. D., Hampson, M., and MacIntosh, B. J. (2014). A single session of exercise increases connectivity in sensorimotor-related brain networks: a resting-state fMRI study in young healthy adults. Front. Hum. Neurosci. 8:625. doi: 10.3389/fnhum.2014.00625
Rehfeld, K., Lüders, A., Hökelmann, A., Lessmann, V., Kaufmann, J., Brigadski, T., et al. (2018). Dance training is superior to repetitive physical exercise in inducing brain plasticity in the elderly. PLoS One 13:e0196636. doi: 10.1371/journal.pone.0196636
Rehfeld, K., Müller, P., Aye, N., Schmicker, M., Dordevic, M., Kaufmann, J., et al. (2017). Dancing or fitness sport? The effects of two training programs on hippocampal plasticity and balance abilities in healthy seniors. Front. Hum. Neurosci. 11:305. doi: 10.3389/fnhum.2017.00305
Rektorova, I., Klobusiakova, P., Balazova, Z., Kropacova, S., Sejnoha Minsterova, A., Grmela, R., et al. (2020). Brain structure changes in nondemented seniors after six-month dance-exercise intervention. Acta Neurol. Scand. 141, 90–97. doi: 10.1111/ane.13181
Rosano, C., Venkatraman, V. K., Guralnik, J., Newman, A. B., Glynn, N. W., Launer, L., et al. (2010). Psychomotor speed and functional brain MRI 2 years after completing a physical activity treatment. J. Gerontol. Ser. A 65, 639–647. doi: 10.1093/gerona/glq038
Schmitt, A., Upadhyay, N., Martin, J. A., Rojas, S., Strüder, H. K., and Boecker, H. (2019). Modulation of distinct intrinsic resting state brain networks by acute exercise bouts of differing intensity. BPL 5, 39–55. doi: 10.3233/BPL-190081
Seeley, W. W. (2019). The salience network: a neural system for perceiving and responding to homeostatic demands. J. Neurosci. 39, 9878–9882. doi: 10.1523/JNEUROSCI.1138-17.2019
Sejnoha Minsterova, A., Klobusiakova, P., Kropacova, S., Novakova, L., Brabenec, L., Balazova, Z., et al. (2020). Multishell diffusion MRI reflects improved physical fitness induced by dance intervention. Neural Plast. 2020, 1–9. doi: 10.1155/2020/8836925
Solé, R. V., Corominas-Murtra, B., Valverde, S., and Steels, L. (2010). Language networks: their structure, function, and evolution. Complexity 15, 20–26. doi: 10.1002/cplx.20305
Teixeira-Machado, L., Arida, R. M., and de Jesus Mari, J. (2019). Dance for neuroplasticity: a descriptive systematic review. Neurosc. Biobehav. Rev. 96, 232–240. doi: 10.1016/j.neubiorev.2018.12.010
Tozzi, L., Carballedo, A., Lavelle, G., Doolin, K., Doyle, M., Amico, F., et al. (2016). Longitudinal functional connectivity changes correlate with mood improvement after regular exercise in a dose-dependent fashion. Eur. J. Neurosci. 43, 1089–1096. doi: 10.1111/ejn.13222
van den Heuvel, M. P., and Hulshoff Pol, H. E. (2010). Exploring the brain network: a review on resting-state fMRI functional connectivity. Eur. Neuropsychopharmacol. 20, 519–534. doi: 10.1016/j.euroneuro.2010.03.008
Vidal-Piñeiro, D., Valls-Pedret, C., FernÃindez-Cabello, S., Arenaza-Urquijo, E. M., Sala-Llonch, R., Solana, E., et al. (2014). Decreased default mode network connectivity correlates with age-associated structural and cognitive changes. Front. Aging Neurosci. 6:256. doi: 10.3389/fnagi.2014.00256
Voss, M. W., Prakash, R. S., Erickson, K. I., Basak, C., Chaddock, L., Kim, J. S., et al. (2010). Plasticity of brain networks in a randomized intervention trial of exercise training in older adults. Front. Aging Neurosci. 2:32. doi: 10.3389/fnagi.2010.00032
Wallis, G., Stokes, M., Cousijn, H., Woolrich, M., and Nobre, A. C. (2015). Frontoparietal and cingulo-opercular networks play dissociable roles in control of working memory. J. Cogn. Neurosci. 27, 2019–2034. doi: 10.1162/jocn_a_00838
Xiong, J., Ye, M., Wang, L., and Zheng, G. (2021). Effects of physical exercise on executive function in cognitively healthy older adults: a systematic review and meta-analysis of randomized controlled trials. Int. J. Nurs. Stud. 114:103810. doi: 10.1016/j.ijnurstu.2020.103810
Keywords: dance intervention, resting state fMRI, independent component analysis, intra-network connectivity, attention, cognitive
Citation: Balazova Z, Marecek R, Novakova L’, Nemcova-Elfmarkova N, Kropacova S, Brabenec L, Grmela R, Vaculíková P, Svobodova L and Rektorova I (2021) Dance Intervention Impact on Brain Plasticity: A Randomized 6-Month fMRI Study in Non-expert Older Adults. Front. Aging Neurosci. 13:724064. doi: 10.3389/fnagi.2021.724064
Received: 11 June 2021; Accepted: 28 September 2021;
Published: 27 October 2021.
Edited by:
Eling D. de Bruin, Karolinska Institutet (KI), SwedenReviewed by:
Daniele Corbo, University of Brescia, ItalyPaola Valsasina, San Raffaele Scientific Institute, Scientific Institute for Research, Hospitalization and Healthcare (IRCCS), Italy
Copyright © 2021 Balazova, Marecek, Novakova, Nemcova-Elfmarkova, Kropacova, Brabenec, Grmela, Vaculíková, Svobodova and Rektorova. This is an open-access article distributed under the terms of the Creative Commons Attribution License (CC BY). The use, distribution or reproduction in other forums is permitted, provided the original author(s) and the copyright owner(s) are credited and that the original publication in this journal is cited, in accordance with accepted academic practice. No use, distribution or reproduction is permitted which does not comply with these terms.
*Correspondence: Irena Rektorova, aXJlbmEucmVrdG9yb3ZhQGNlaXRlYy5tdW5pLmN6