- 1Department of Psychology, University of Cambridge, Cambridge, United Kingdom
- 2School of Psychology, College of Life and Environmental Sciences, University of Exeter, Exeter, United Kingdom
- 3Department of Psychiatry, University of Cambridge, Cambridge, United Kingdom
- 4Cambridgeshire and Peterborough NHS Foundation Trust, Cambridge, United Kingdom
COVID-19, caused by severe acute respiratory syndrome coronavirus 2 (SARS-CoV-2), has been often characterized as a respiratory disease. However, it is increasingly being understood as an infection that impacts multiple systems, and many patients report neurological symptoms. Indeed, there is accumulating evidence for neural damage in some individuals, with recent studies suggesting loss of gray matter in multiple regions, particularly in the left hemisphere. There are several mechanisms by which the COVID-19 infection may lead to neurological symptoms and structural and functional changes in the brain, and cognitive problems are one of the most commonly reported symptoms in those experiencing Long COVID – the chronic illness following the COVID-19 infection that affects between 10 and 25% of patients. However, there is yet little research testing cognition in Long COVID. The COVID and Cognition Study is a cross-sectional/longitudinal study aiming to understand cognitive problems in Long COVID. The first paper from the study explored the characteristics of our sample of 181 individuals who had experienced the COVID-19 infection, and 185 who had not, and the factors that predicted ongoing symptoms and self-reported cognitive deficits. In this second paper from the study, we assess this sample on tests of memory, language, and executive function. We hypothesize that performance on “objective” cognitive tests will reflect self-reported cognitive symptoms. We further hypothesize that some symptom profiles may be more predictive of cognitive performance than others, perhaps giving some information about the mechanism. We found a consistent pattern of memory deficits in those that had experienced the COVID-19 infection, with deficits increasing with the severity of self-reported ongoing symptoms. Fatigue/Mixed symptoms during the initial illness and ongoing neurological symptoms were predictive of cognitive performance.
Introduction
Traditionally, COVID-19 has been considered a respiratory disease. However, around 35% of patients – and up to 85% of those who become severely ill – report neurological symptoms including headache, dizziness, myalgia, or loss of taste and smell (e.g., Mao et al., 2020). The most well-known neurological symptom – alteration in taste or smell (anosmia/dysgeusia) – is also one of the most common symptoms of the disease (e.g., Lechien et al., 2020), often the first symptom to manifest (Mao et al., 2020; Romero-Sánchez et al., 2020) and last to abate (Lechien et al., 2020).
There is accumulating evidence that COVID-19 is associated with neural damage, particularly in the presence of neurological symptoms (Helms et al., 2020; Kandemirli et al., 2020). Post-mortem studies of patients who have died of COVID-19 show evidence for ischemic lesions and indications of neuro-inflammation (Matschke et al., 2020). Multiple studies have indicated abnormalities such as hemorrhagic lesions in the orbitofrontal cortex (Le Guennec et al., 2020), the medial temporal lobe, and the hippocampus (Moriguchi et al., 2020; Poyiadji et al., 2020), bilateral thalamic lesions, and sub-insular regions (Poyiadji et al., 2020). The changes may be functional as well as structural, with nearly 90% of electroencephalography (EEG) studies conducted in patients with COVID-19 revealing epileptiform discharges, mostly within the frontal lobes (Galanopoulou et al., 2020). A study using the UK Biobank cohort conducted structural and functional brain scans before and after infection with COVID-19 on 394 patients compared with 388 matched controls who had not experienced the COVID-19 infection (Douaud et al., 2021). Significant loss of gray matter was identified in areas with high connectivity to the olfactory system (the hypothesized route of viral entry into the brain). The parahippocampal gyrus, the lateral orbitofrontal cortex, and the insula were particularly affected, and gray matter reductions were notably concentrated in the left hemisphere. The mechanistic implications of this left hemisphere bias are not clear but may reflect asymmetry in the connectivity of the olfactory system (Royet and Plailly, 2004). An analysis of the small subset of this sample (n = 15) who had been hospitalized indicated more severe gray matter loss in these participants, particularly in the left cingulate cortex, and the right amygdala, and the hippocampus. Bougakov et al. (2021) have argued that depending on the mechanism and location of neural damage, there are several cognitive deficits that might be expected to be detectable in patients with COVID-19. For example, SARS-CoV-2 may be able to attack the brain directly, perhaps via the olfactory nerve (Lechien et al., 2020; Politi et al., 2020), causing encephalitis. Besides, severe hypoxia from respiratory issues may induce hypoxic/anoxic encephalopathy (Guo et al., 2020). The unusual clotting seen in patients with COVID-19 may be associated with acute ischemic and hemorrhagic cerebrovascular events (CVAs: Beyrouti et al., 2020; Li et al., 2020; Wang et al., 2020; Kubánková et al., 2021), leading to more lasting brain lesions. Finally, a maladaptive immune response to infection can negatively impact neural systems via hemorrhagic encephalopathy (Das et al., 2020; Poyiadji et al., 2020) or peripheral neuropathy (e.g., Guillain-Barre syndrome; Alberti et al., 2020; Whittaker et al., 2020; Zhao et al., 2020).
Much of the evidence suggesting that cognitive dysfunction may occur following the COVID-19 infection comes from those who experience “post-COVID-19 syndrome”/“post-acute sequalae SARS-CoV-2” (PASC)/“Long COVID.” The National Institute for Health and Care Excellence (NICE) guidelines describe “post-COVID-19 syndrome” as “Signs or symptoms that develop during or after infection consistent with COVID-19, continue for more than 12 weeks and are not explained by an alternative diagnosis” (National Institute for Health and Care Excellence, 2020). Disagreements exist as to the exact symptoms, longevity, and severity required to qualify for a diagnosis of PASC, making it difficult to ascertain prevalence precisely. However, estimates of patients with COVID-19 having some degree of chronic illness range from 10 to 25% (e.g., Cirulli et al., 2020; Ding et al., 2020; Sudre et al., 2020; Tenforde et al., 2020; Nehme et al., 2021; Office for National Statistics, 2021). The patient-created term “Long COVID” has increasingly been used as an umbrella term to describe this highly heterogeneous condition (Callard and Perego, 2021).
Cognitive dysfunction is one of the most common symptoms reported in research into Long COVID, occurring in around 70% of patients (Cirulli et al., 2020; Bliddal et al., 2021; Davis et al., 2021; Ziauddeen et al., 2021), and in many cases appearing second only to fatigue. In one study, 86% of participants indicated that cognitive dysfunction and/or memory impairment was impacting their ability to work (Davis et al., 2021). In our first paper in the COVID and Cognition study (Guo et al., 2022), we found a similar prevalence of cognitive symptoms to previous studies, with 77.8% reporting difficulty concentrating, 69% reporting brain fog, 67.5% reporting forgetfulness, 59.5% reporting tip-of-the-tongue (ToT) word-finding problems, and 43.7% reporting semantic disfluency (saying or typing the wrong word). In that analysis, we found that the experience of chronic fatigue-like (“Fatigue/Mixed”) and neurological symptoms during the first 3 weeks significantly predicted the experience of cognitive symptoms later in the subsequent illness. Those individuals experiencing ongoing “Cardiopulmonary/Fatigue,” “Neurological” and “Gastrointestinal/Autoimmune” symptoms were also found to be more likely to be experiencing cognitive symptoms.
It is often difficult to ascertain to what extent quite broadly defined self-reported cognitive deficits such as “difficulty concentrating” and “brain fog” translate into measurable changes in cognitive performance. While there are multiple lines of evidence to suggest that individuals experiencing Long COVID experience cognitive symptoms, there is, to date, little research objectively measuring cognition post-COVID-19.
The study of Alemanno et al. (2021) investigated cognitive function in the post-acute phase (1 month after discharge) in patients with COVID-19 that had experienced severe illness. Using the Montreal Cognitive Assessment (MoCA) score, they showed that 80% of patients showed indications of cognitive deficit, particularly in memory, executive function, and language. Similarly, Helms et al. (2020) found that, at discharge from the intensive care unit (ICU), 33% of patients showed evidence of dysexecutive syndrome, with symptoms such as inattention, disorientation, or poorly organized movements in response to the command. In their study of 29 patients (average age 65) presenting at least one new neurological symptom since COVID-19 infection, Hosp et al. (2021) found that cognitive performance may be linked to neurological abnormalities and symptoms. A positron emission tomography (PET) analysis revealed predominant frontoparietal hypometabolism, correlating to lower MoCA scores and extended neuropsychological testing. In particular, patients with COVID-19 showed deficits in tests of verbal memory and executive functions. One issue with all of these studies’ data is limited to severely ill patients, mostly of older age (65+). It is thus difficult to determine whether these deficits are specific to COVID-19 or a more general response to acute respiratory distress (ARD) and ventilation. It is known, for example, that survivors of critical illness are known to experience long-term cognitive impairment (Hopkins et al., 1999; Jackson et al., 2003; Ehlenbach et al., 2010; Iwashyna et al., 2010; Pandharipande et al., 2013), particularly if they experience delirium (e.g., Girard et al., 2010; Pandharipande et al., 2013). Thus, it is important to establish to what extent cognitive dysfunction is a feature of post-COVID-19 pathology, or merely reflective of the large number of patients with COVID-19 that experience ARD. Furthermore, it must be investigated whether these deficits extend to younger populations. In an early indication that this might be the case, Almeria et al. (2020) assessed younger (aged 24–60) patients 10–40 days post-discharge, of which only 20% had been in intensive care, but 60% required oxygen. They found that those reporting neurological symptoms had lower performance on attention, memory, and executive function, once again suggesting a degree of association between symptomatology and degree of cognitive deficit.
In a very large study using 81,337 participants in the Great British Intelligence Test (GBIT; mean age 46.75), Hampshire et al. (2021) compared participants who reported having had the COVID-19 infection to concurrently tested control participants. The authors conducted an analysis of the association between symptom severity and cognitive performance controlled for age, gender, education level, income, racial-ethnic group, and pre-existing medical disorders. Among 12,689 participants that suspected that they had had COVID-19, 326 had a positive test, and 192 were hospitalized. Participants who had received a positive test had a lower global score and this deficit scaled with the severity of initial respiratory illness: There was not only a substantial effect size for people who had been hospitalized but also a clear effect for mild but biologically confirmed cases who reported no breathing difficulties. The largest effect sizes were seen in tests of verbal reasoning, multi-stage planning, and spatial attention. Most participants had fully recovered at the time they took the test; however, 24% of those with test-confirmed COVID-19 reported residual symptoms. Controlling for residual symptoms, respiratory severity during the initial illness remained a strong predictor of global cognitive performance, while the presence of ongoing symptoms did not predict significant variance. There was no significant association between time since illness and cognitive performance; however, this analysis excluded those with ongoing symptoms.
The study conducted by Graham et al. (2021) investigated cognition and quality of life measures in 100 non-hospitalized patients (mean age 43) presenting to a neuro-COVID clinic with neurological symptoms persisting for at least 6 weeks from symptom onset. These patients reported a median of five neurologic symptoms and over 80% reported having experienced brain fog. Some, but not all, of these symptoms, had resolved at the time of cognitive assessment. A subset of participants was assessed with the National Institutes of Health (NIH) Toolbox covering processing speed, attention and executive memory, executive function, and working memory, and these scores were compared to established baselines. The authors reported 53% of participants as having abnormal findings, with short-term memory and attention being most commonly impaired. Participants also had significantly reduced cognition- and fatigue-related quality of life indices. However, given that performance in this study was compared to established baselines rather than a control group, it is difficult to be confident of the proportion of the seen deficit that is attributable to COVID-19 rather than the general stress and disruption caused by the pandemic.
Despite being probable that there is a relationship between the COVID-19 infection, neurological symptoms, and cognitive dysfunction, many questions remain about the specific nature of the cognitive impairment in Long COVID. We distinguish three main ones that drive our research program and which it attempts to answer: First, what are the associations between reported symptoms and cognitive outcomes? Second, given the heterogeneous nature of Long COVID, is diversity reflected in a diversity of cognitive issues, or is there a specific sub-phenotype of Long COVID that is associated with cognitive deficits? Finally, are those that report “subjective” cognition and memory complaints more likely to demonstrate impairments in “objective” cognitive assessments of the same functions?
In this study, we reported on the first stage of a mixed cross-sectional/longitudinal study, the COVID and Cognition Study (COVCOG), aimed at understanding cognition following the COVID-19 infection relative to that of concurrently tested controls. Using the online assessment platform Gorilla1, we set out to bring together information about symptom profiles both during and following initial infection and detailed analysis of cognitive performance across a range of domains including memory, language, and executive function. The aims of this study do not include identifying a specific mechanism of cognitive deficit (as that requires types of tests and analysis not feasible in an online study) but rather to “map the terrain,” providing sufficient breadth and detail of mechanism-relevant information to facilitate and inform the future mechanistic investigation.
The first aim of this investigation was to ascertain whether differences could be found in cognitive performance between those that had and those that had not experienced the COVID-19 infection. Problems with memory and with speech and language are the most commonly reported cognitive symptoms (after “brain fog”) in Long COVID, affecting around 70 and 40% of patients, respectively (Davis et al., 2021). Given this, we hypothesize that where cognitive differences exist, these will be larger, or more likely, in tests assessing memory or language relative to those assessing (for example) executive function.
A second hypothesis, following previous findings (e.g., Hampshire et al., 2021; Hosp et al., 2021) is that the degree of cognitive deficit will relate to the severity and nature of the initial illness. In particular, it seems likely that the number and severity of neurological symptoms during the initial illness may be indicative of the degree of impact of the disease on neural function (whether that be via direct infection, inflammation or CVA, or another route), which would be most likely to result in subsequent cognitive deficits. Our previous publication on this study (Guo et al., 2022) found that ongoing cognitive symptoms were predicted by Fatigue/Mixed, Neurological/Psychiatric, and Respiratory/Infectious (e.g., cough, fever, loss of taste and smell) symptoms experienced during the initial illness. We predict that similar associations will be found between symptom factors during the initial illness and performance on cognitive tasks and that these may be most pronounced for neurological symptoms.
We further hypothesize that not just the presence but the nature of ongoing illness will be associated with cognitive deficits. We predict that those with severe ongoing symptoms will be more likely to show concomitantly more severe deficits in cognitive tasks. Our first paper from this study found that ongoing Cardiopulmonary/Fatigue, Neurological and Gastrointestinal/Autoimmune symptoms were associated with greater cognitive symptoms. We hypothesize that these symptom factors will be similarly associated with performance on cognitive tests.
Finally, we predict that any deficits will be greatest in those individuals experiencing ongoing cognitive symptoms. Indeed, we might expect those reporting specific cognitive symptoms (e.g., “forgetfulness”) to be particularly impaired on tests of cognition that assess the associated skill (e.g., memory).
Materials and Methods
Participants
A total of 421 participants aged 18 and over were recruited through word of mouth, student societies, and online/social media platforms such as the Facebook Long COVID Support Group and the Prolific recruitment site. They were recruited from the majority English-speaking countries (the United Kingdom, Ireland, United States, Canada, Australia, New Zealand, or South Africa) and were English speakers. Of these, 181 (130 women) had experienced the COVID-19 infection (65 test-confirmed, 96 suspected) and 185 (118 women) had not. A further 55 had “unknown” infection status (did not think they had had COVID-19 but had had an illness that could potentially have been). Among those that had had COVID-19, 42 (29 women) had recovered by the time of test (“Recovered group,” R), 53 (36 women) continued to experience mild or moderate ongoing symptoms (“Ongoing (Mild/Moderate) group,” C+), and 66 (54 women) experienced severe ongoing symptoms (“Ongoing (Severe) group,” C++). The other 20 participants were too early in the illness to indicate ongoing symptoms. Comorbidity was not an exclusion criterion. Full details of our sample, including demographic and medical history characterizations, are provided in our previous publication on this study (Guo et al., 2022).
Procedure
The study was reviewed by the University of Cambridge Psychology Ethics Committee (PRE.2020.106, September 8, 2020). This is a mixed cross-sectional/longitudinal online study conducted using Gorilla (Anwyl-Irvine et al., 2020; see text footnote 1). The results reported here are for the baseline session of the study only. The baseline session consisted of a questionnaire covering demographics, previous health, and experience of COVID-19, followed by a series of cognitive tests.
Participants answered questions relating to their age, sex, education level, country of permanent residence, ethnicity, and profession. They were then asked a series of questions relating to their medical history and health-related behaviors (such as smoking and exercise). Next, they were asked for details of their experience of COVID-19. The COVID-status was established based on their response to a series of questions (starting with “Have you had COVID-19?”) and their response to a series of questions regarding the presence and severity of ongoing symptoms. Full details of the questionnaires and grouping dynamics are provided in our previous publication on this study (Guo et al., 2022). Finally, participants were asked to give details on a large number of individual symptoms during three time periods: the initial 3 weeks, “in the time since then,” and the past 1–2 days. Participants were also asked to report a 5-point Likert scale, from very bad (1) to very good (5) on how current symptom severity was on the day of the test.
Cognitive Tests
Figures 1A–D,F shows the 6 cognitive tasks that were presented. All participants completed tasks, while only the “No COVID” group completed task e.
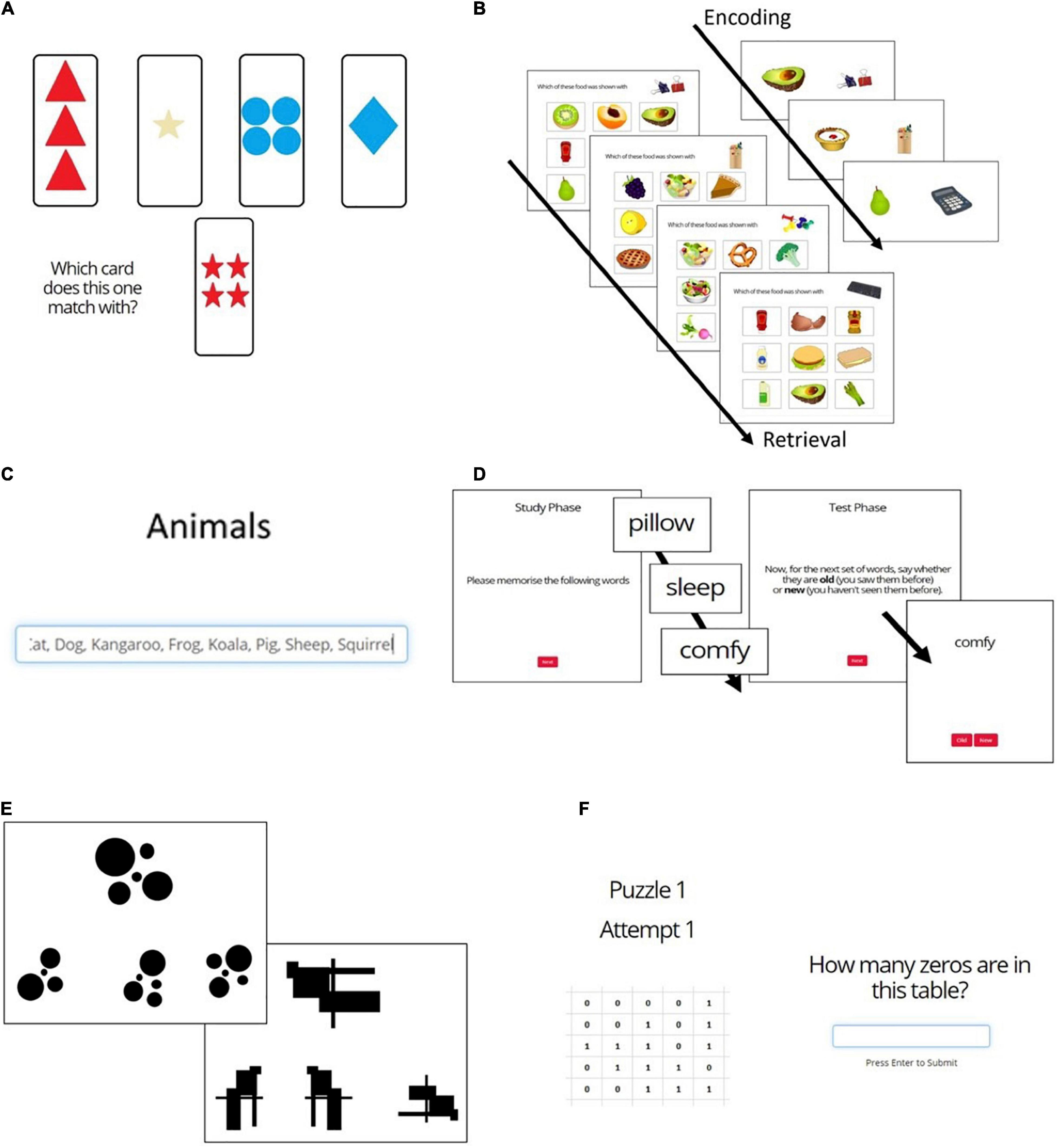
Figure 1. Cognitive tasks. (A) Wisconsin Card Sorting Test; (B) Pictorial Associative Memory Test; (C) Category Fluency Test; (D) Word List Recognition Memory Test; (E) 2D Mental Rotation Test; (F) Number Counting Test (Attention/Bot Check).
Word List Recognition Memory Test (Figure 1D)
Participants were shown a list of 16 words one by one with the instruction to memorize as many as possible. They were then shown 32 (16 old and 16 new) words and asked to report which had been on the original list (Figure 1D). Target and distractor words were scored and matched for imagery and concreteness. The dependent variables on this task were % correct, d′, and reaction time (RT).
Pictorial Associative Memory Test (Figure 1B)
Participants were required to memorize a series of 17 stationery and food-item pairs each displayed on the screen for 3 s. The recall phase took place immediately thereafter and involved 15 trials, each of which presented an item of stationery and asked participants to select the associated food item from 9 options (Figure 1B). The dependant variables were % correct and reaction time.
Category Fluency Test (Figure 1C)
Participants were presented with the category word “Animals” and had 1 min to type every example of that category they could think of. The words were entered into a scrolling text box such that, after around 6 words, earlier words started to move out of view (Figure 1C). Dependent variables were number of correct words, % produced words that were correct, number of incorrect (unrelated) words (e.g., “table”), number of incorrect (related) words (e.g., “fur”), and number of repetitions.
Mental Rotation Test (Figure 1E)
Participants were presented with 16 trials in which they saw an abstract image and had to select which of three possible options represented that image rotated (Figure 1E). This is a test of visual working memory. Outcome variables were % correct and reaction time.
Wisconsin Card Sorting Test (Figure 1A)
This executive function (EF) task assesses task switching and inhibition. Across 64 trials, participants were required to match a given card to one of the four cards based on either color, shape, or number (Figure 1A). They were not explicitly told the matching rule but must infer this from the feedback on their choices. Every few trials the rule changed, and participants must find and follow the new rule based on feedback.
Number Counting Test (Figure 1F)
This task was included in the baseline as an attention/“bot” control for data quality. It presented a grid of 1s and 0s and asked the participants to count the 0s (Figure 1F). This is not cognitively difficult but requires concentration. Because the grid is an image, this is also difficult for most Ais. Participants were given 3 attempts at this task. The numbers given by participants giving 3 incorrect answers were manually checked. If the numbers appeared to be genuine attempts (i.e., close but incorrect), then the participant was considered genuine and was included in the dataset. No participants were removed due to failing this task.
Relational Reasoning Test
Across 35 trials, participants were shown a 3 × 3 matrix of images with one missing and were asked to select from 4 options which image should fill the gap. This task was given only to the No COVID group and was intended as a means by which to IQ-match control participants for potential pre-post infection longitudinal explorations. Data from this task are not reported in this paper.
Data Processing and Analysis
Analyses were conducted using IBM SPSS Statistics for Windows, version 232. We describe quantitative variables using means and standard deviations, and numbers and percentages for qualitative variables. Sidak’s correction for multiple comparisons was employed where appropriate, and both corrected and uncorrected analyses are shown.
As there were a large number of cognitive test variables, we reduced these via factor analysis to produce 4 factors representing Executive Functions (Performance), Executive Functions (Reaction Time), Memory, and Category Fluency. Analyses were conducted first on these factors to give an overview of the pattern of cognitive performance and then on the individual variables to give a more detailed picture.
We investigated differences in cognitive performance, first, by dividing the sample into two groups (COVID/No COVID), and, second, by subdividing the COVID group by symptom longevity and severity (Recovered, Ongoing Mild/Moderate infection, and Ongoing Severe infection). Where parametric analysis was not appropriate, we employed the Pearson’s chi-square (χ2) for categorical variables and the Mann–Whitney test and the Kruskal–Wallis test for continuous variables depending on the number of COVID groups. To explore what variables were associated with infection or ongoing symptoms, we employed various independent multinomial logistic regression models (backward elimination method). To investigate differences between groups (COVID/No COVID; Recovered, Ongoing Mild/Moderate, Ongoing Severe) and the outcome of the cognitive tasks, we employed independent t-test/Mann–Whitney and ANOVA/Kruskal-Wallis tests. We also performed general linear models (GLM) controlling for sex, age, country, and education level. We also examined whether any total score from the cognitive tasks could be associated with variance in initial illness severity (Asymptomatic/Very mild, Mild, and Moderate/Severe) using independent simple regression models.
As reviewed in detail in our previous publication (Guo et al., 2022), we used exploratory principal component analysis to cluster the symptoms experienced during the initial infection, and the symptoms subsequently experienced since that time. We identified 5 factors for symptoms experienced during the first 3 weeks of illness. These included a “Neurological/Psychiatric” factor characterized by disorientation, delirium, and visual disturbances; a “Fatigue/Mixed” factor characterized by fatigue, chest pain/tightness, and muscle/body pains; a “Gastrointestinal” factor characterized by diarrhea, nausea, and vomiting; a “Respiratory/Infectious” factor characterized by fever, cough, and breathing issues; and a “Dermatological” factor characterized by rash, itchy welts, and foot sores. For symptoms experienced in the time since the initial illness, 6 factors were identified: A “Neurological” factor characterized by disorientation, confusion, and delirium; a “Gastrointestinal/Autoimmune” factor characterized by hot flushes, nausea, and diarrhea; a “Cardiopulmonary/Fatigue” factor characterized by breathing issues, chest pain/tightness, and fatigue; a “Dermatological/Fever” factor characterized by face/lips swelling, foot sores, and itchy welts; an “Appetite Loss” factor characterized by weight loss and loss of appetite, and finally, a “Mood” factor characterized by depression, anxiety, and vivid dreams. To assess currently experienced symptom factors, we employed the sum scores by factor method using the “since then” symptom factors as a base. We used linear multiple regression models (backward elimination method) to test whether ongoing factors predicted performance on cognitive tests.
Results
Factor Analysis of Cognitive Variables
The cognitive task variables were a priori divided into two groups: language and memory (incorporating all Word List, Associative Memory, and Category Fluency variables), and executive functions (including all WCST and 2D Mental Rotation variables), and factor analyses were conducted on these separately. Each exploratory factor analysis (EFA) was limited to two factors. Two items (one in each analysis: WCST perseverative error reaction time and Category Fluency repetitions) that did not load into any factor were removed. The re-run analyses explained 48.9% and 58.9% of the variance, respectively. We thus ended with four performance factors: Executive Functions Performance (including score and errors for WCST and performance on 2D Mental Rotation), Executive Functions Reaction Times (including all reaction times from both EF tasks), Memory (including all variables from both Word List and Associative Memory), and Category Fluency (including all Category Fluency variables). See Supplementary Table 1 for rotated component matrix.
Ten extreme outliers (identified by Q plot) were removed from each of the Category Fluency and EF Reaction Time factors to bring skewness and kurtosis within acceptable bounds [Category: skew = –0.623 (0.139); kurtosis = –0.181 (0.276); EF RT: skew = –0.508 (0.138); kurtosis = –0.153 (0.274)]. Similarly, 9 extreme outliers (identified by Q plot) were removed from the Memory factor [skew = –0.623 (0.139); kurtosis = –0.181 (0.276)].
COVID-19 and Cognition
Memory and Word Finding
A first analysis was run using the task factors comparing the “COVID” and “No COVID” groups. There was a significant negative influence of the COVID-19 infection on memory performance, even when controlling for age, sex, country, and education level [F(1,304) = 10.903, p = 0.001].
There was also a significant difference between groups on the Category Fluency factor [F(1,307) = 6.297, p = 0.013, = 0.02], but this disappeared when controlling for demographic variables (see Figure 2 and Table 1).
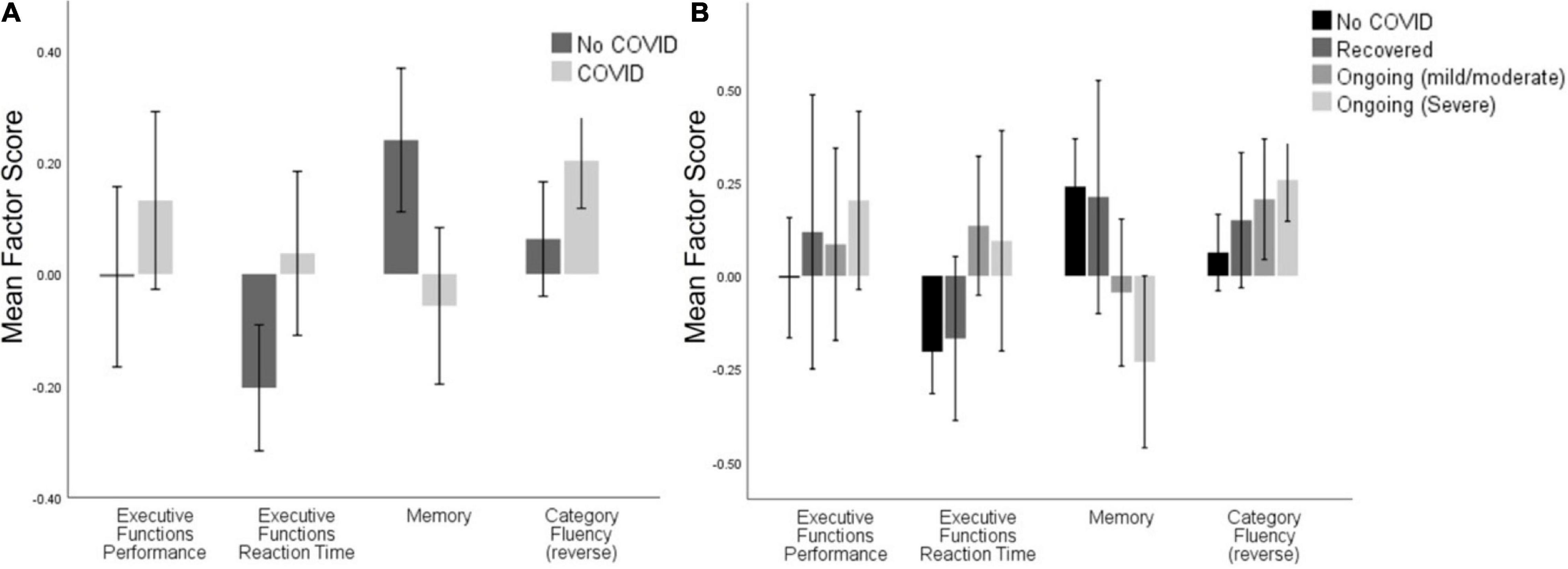
Figure 2. Cognitive task factor scores across (A) the No COVID group and the COVID group, and (B) the No COVID group and the three ongoing severity groups. Significant differences were seen between the No COVID group and Ongoing (Mild/Moderate) on Memory [t(87.6) = 2.4, p = 0.018], and between No COVID and Ongoing (Severe) on Memory [t(99.8) = 3.9, p < 0.001] and Category Fluency [t(152) = 3.05, p < 0.003]. After controlling for demographic variables, only the differences in Memory maintained significance (see Supplementary Table 2). Error bars: ± 2 SE.
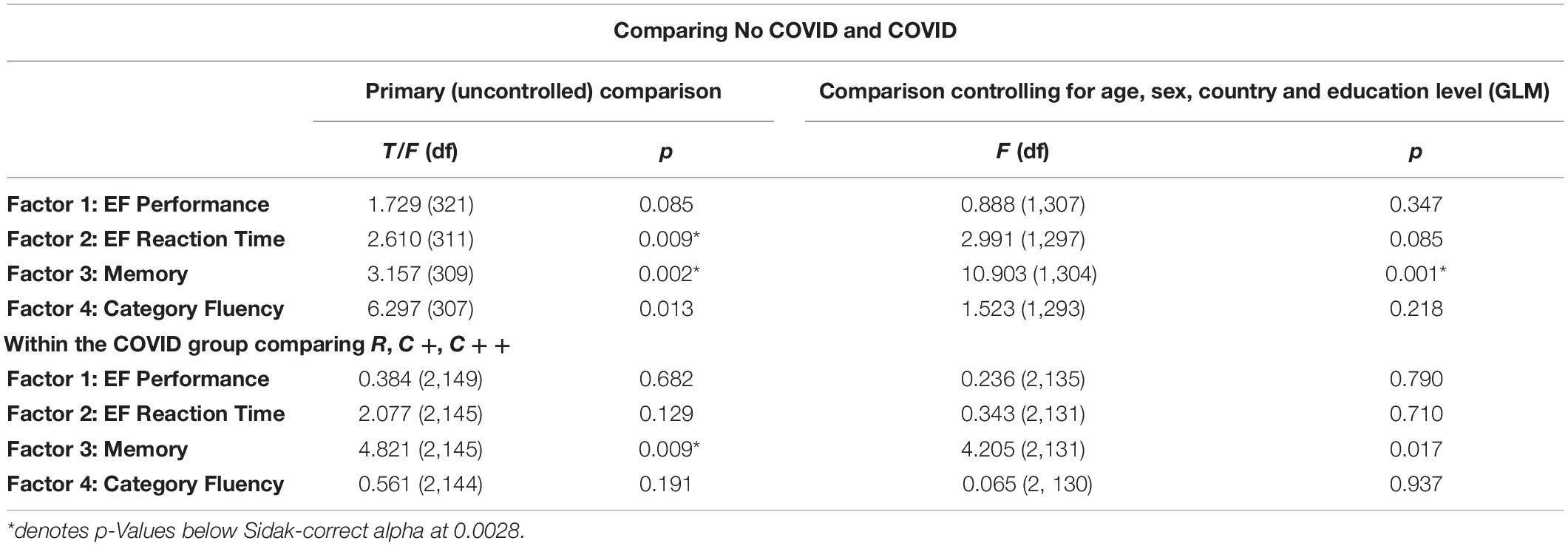
Table 1. Cognitive performance factors across COVID and No COVID groups (top) and symptom severity levels (bottom).
For individual variables, primary analysis suggested that individuals who had experienced the COVID-19 infection had significantly lower performance (U = 3.29, p < 0.001) and slower reaction time (U = 3.53, p < 0.001) than the No COVID group on the Word List Recognition Memory Test (Table 2). After controlling for age, sex, country, and education level, these effects were maintained [% correct: F(1,315) = 6.77, p = 0.01; RT: F(1,315) = 12.66, p < 0.001)], with d′ becoming significant [F(1,315) = 5.78, p = 0.017]. A much weaker trend was seen in the Pictorial Associative Memory Test, suggesting a reduced performance in the COVID group (t = 1.91, p = 0.056) and no impact on reaction time (p = 0.671). When controlling for age, sex, country and education level, the significance of this group effect strengthened, suggesting that those who had experienced the COVID-19 infection scored lower than the No COVID group [F(1,319) = 4.01, p = 0.046]. Considering only analyses controlling for demographic factors, only reaction time on the Word List Recognition survived conservative correction for multiple comparisons (Sidak α = 0.0028).
For Category Fluency, uncorrected analysis found that, although the COVID group repeated more words (U = 2.35, p = 0.019), they gave fewer incorrect (related) words (U = 2.23, p = 0.026) than the No COVID group. However, these effects disappeared after factoring out age, sex, country, and education level.
Other Tasks
There were no significant differences between the groups on the Executive Function Performance factor, but there was a significant group difference in Executive Function Reaction Time [t(311) = 2.610, p = 0.009], but this dropped below significance once age, sex, country, and education were accounted for (see Figure 2 and Table 1).
In terms of individual variables, there were no group differences in performance on the WCST; however, the COVID-group had significantly slower reaction time on trials with both correct responses (U = 3.03, p = 0.002; see Table 2) and non-perseverative errors (U = 2.86, p = 0.004). No significant difference was found after controlling for age, sex, country, and education level. There were no significant differences between groups on performance on the 2D Mental Rotation Test.
Ongoing Symptom Severity and Cognition
Memory and Word Finding
There was a significant difference between ongoing symptom severity groups in the Memory factor [F(2,150) = 5.724, p = 0.004], which was weakened but still significant when demographic factors were accounted for [F(2,136) = 3.653, p = 0.028]. Pairwise analysis controlling for demographic variables showed a significant difference between the Recovered and Ongoing (Severe) groups [F(1,88) = 6.414, p = 0.013]. There was no association between symptom severity and the Category Fluency factor (see Table 1).
In terms of individual variables (see Table 3), significant differences between ongoing symptom sub-groups were found on Word List % correct [H(3) = 22.51, p < 0.001; Figure 3] and reaction time [H(3) = 24.07, p < 0.001]. Pairwise tests with Sidak α = 0.008 revealed that those with severe ongoing symptoms had lower % correct than the No COVID group (p < 0.001) and those that had recovered (p < 0.001) and had slower reaction time than the No COVID group (p < 0.001). Those with mild/moderate ongoing symptoms also had slower reaction time than the No COVID group (p < 0.001) and the Recovered group (p = 0.004). When age, sex, country and education level were factored out by GLM, d′ [F(3,310) = 2.90, p = 0.035], % correct [F(3,310) = 4.99, p = 0.002], and reaction time [F(3,310) = 6.88, p < 0.001] differences were all significant, but only % correct and reaction time survived correction for multiple comparisons (Sidak α = 0.0028). Pairwise tests suggested that those with severe ongoing symptoms had significant lower d′ (p = 0.004), lower % correct (p < 0.001), and slower reaction time than the No COVID group (p < 0.001). Those with mild/moderate ongoing symptoms still had slower reaction time than the No COVID group (p < 0.001). In contrast to these findings with Word List Recognition Memory, primary analysis did not find significant group differences on Pictorial Associative Memory on either performance or reaction time. However, after controlling age, sex, country and education level, a main effect emerged for % correct [F(3,314) = 2.94, p = 0.034]; however, this did not survive correction for multiple comparisons (Sidak α = 0.0028). Nonetheless, pairwise comparisons suggested that those with severe ongoing symptoms scored lower than the No COVID group (p = 0.005, Sidak α = 0.008).
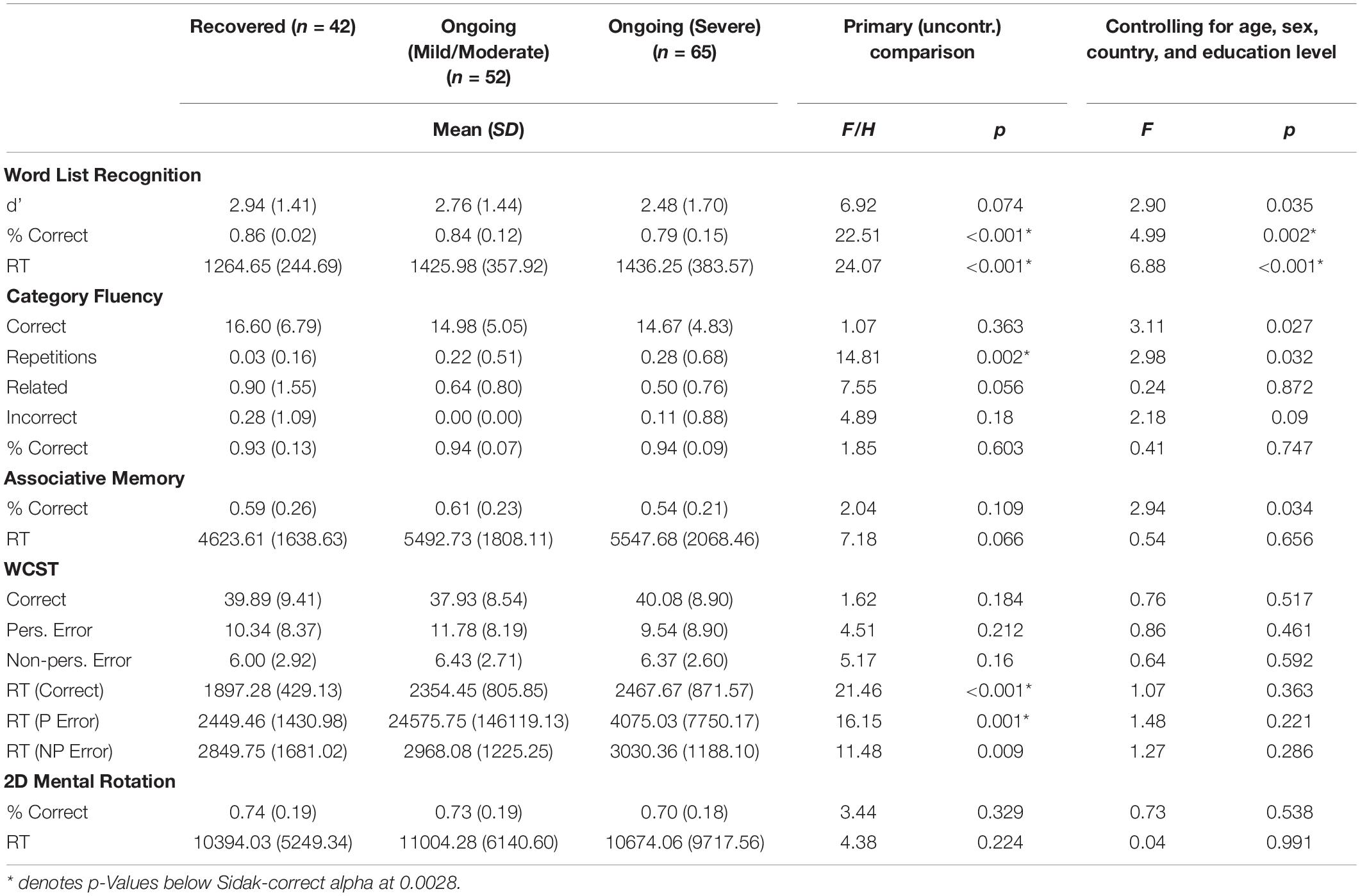
Table 3. Cognitive task results among Recovered, Ongoing (Mild/Moderate), and Ongoing (Severe) groups.
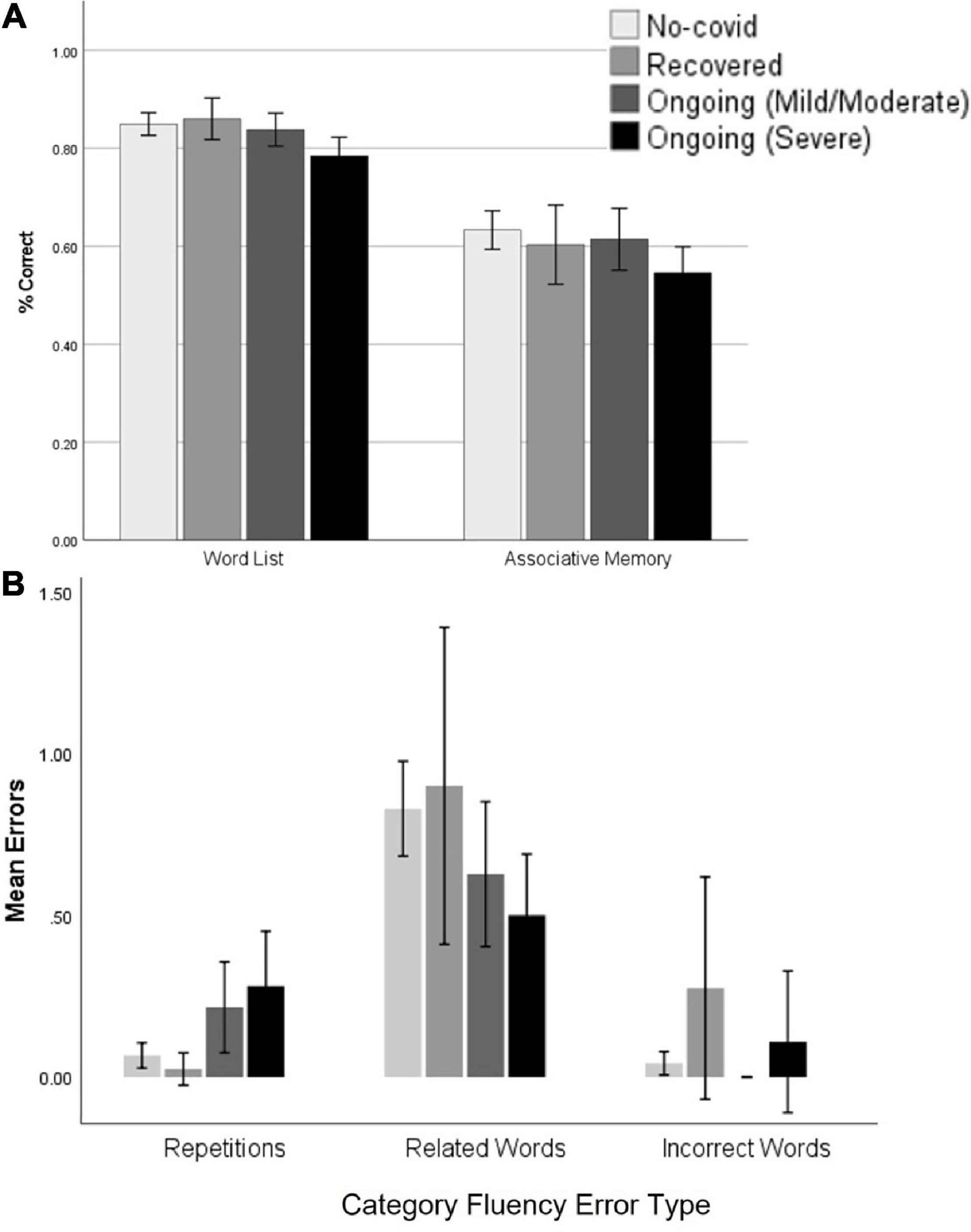
Figure 3. Word List and Associative Memory performance across ongoing symptom groups (A); Category Fluency errors across groups on ongoing symptom severity (B). Error bars: ±2 SE.
For Category Fluency, primary analysis showed a significant group effect in repetitions [H(3) = 14.81, p = 0.002; Figure 3]. Pairwise comparison with Sidak α = 0.008 found that those with severe ongoing symptoms had more repeated words than both the No COVID (p = 0.002) and Recovered groups (p = 0.004). When GLM controlling for age, sex, country, and education level was conducted, there were significant main effects on the number of correct words [F(3,301) = 3.11, p = 0.027] and repetitions [F(3,301) = 2.98, p = 0.032], but neither of these survived correction for multiple comparisons (Sidak α = 0.0028). Pairwise tests showed that those with severe ongoing symptoms had fewer correct words than the Recovered group (p = 0.008), but no pairwise comparisons were significant for repetitions.
Other Tasks
There was no effect of symptom severity group on either of the Executive Function factors (see Table 1).
There were significant group effects for the WCST in reaction time for trials with correct responses [H(3) = 21.46, p < 0.001], perseverative terrorism [H(3) = 16.15, p = 0.001], and non-perseverative errors [H(3) = 11.48, p = 0.009]. Pairwise tests with Sidak α = 0.008 showed that those with mild/moderate ongoing symptoms had a slower reaction time for trials with correct responses than the No COVID group (p = 0.005) and the Recovered groups (p = 0.008). Similarly, those with severe ongoing symptoms were slower for correct responses than the Recovered (p < 0.001) and the No COVID groups (p < 0.001). For trials containing perseverative errors, both those with mild/moderate (p = 0.002) and severe (p = 0.002) ongoing symptoms have slower reaction times than those who recovered. Those with mild/moderate ongoing symptoms were also slower than the No COVID group for trials containing non-perseverative errors (p = 0.005). However, after controlling for age, sex, country, and education level, all these significances disappeared. There were no significant effects in 2D Mental Rotation.
Initial Illness and Subsequent Cognitive Performance
The Severity of Initial Illness
We assessed whether more severe initial illness [grouped into three: Asymptomatic/Very mild; Mild (bed-bound); Moderate/Severe (very ill or hospitalized)] was associated with cognitive performance at the time of test (often weeks or months later). First, we examined this in terms of the cognitive task factors. There was no effect of initial symptom severity on any of the cognitive task factor scores (EF Performance: F(2,149) = 0.479, p = 0.620; EF RT: F(2,146) = 0.019, p = 0.982; Memory: F(2,146) = 1.087, p = 0.340; Category Fluency: F(1,145) = 1.171, p = 0.313).
Next, we examined which (if any) individual cognitive task variables could be associated with variance in initial illness severity (Asymptomatic/Very mild, Mild, Moderate/Severe) using independent simple regression models with COVID-19 illness severity as the dependent variable and all cognitive task variable as predictors. There was a significant association for Word List Recognition [F(1,142) = 6.369, p = 0.013, standardized B = –0.207, = 0.036], but no other cognitive task was associated with initial illness severity. These associations did not survive correction for multiple comparisons (Sidak α = 0.0028).
We also examined whether any particular diagnoses during the initial illness were related to subsequent cognitive performance. After removing diagnoses with very low prevalence (< 4%), none of the remaining diagnoses (hypoxia, blood clots, and Inflammatory syndrome) presented any significant association with cognitive performance.
Nature of Initial Illness and Cognitive Performance
Individual Neurological Symptoms
To test whether any of the specific neurological symptoms experienced during the first 3 weeks of illness (initial symptoms) were related to subsequent cognitive performance, we carried out multiple linear regressions with cognitive performance factors as the dependent variable and the neurological symptoms as possible predictors. Almost no participants showed hallucination or delirium (< 10% of participants), so these were removed from the analysis.
A single early neurological symptom emerged as predicting variance in cognitive task factors. Both Executive Function Performance ( = 0.03) and Memory ( = 0.038) were predicted by initial disorientation (EF Performance: Radj2 = 0.024, p = 0.032; Memory: Radj2 = 0.031, p = 0.017). Variance in Executive Function RT and Category Fluency factors were not predicted by early neurological symptoms. With individual cognitive tests as the dependent variable, several models emerged; however, none of the models survived correction for multiple comparisons (Sidak α = 0.0028; Supplementary Table 3). Headache severity was associated with slower reaction time of the Word List Recognition Test (p = 0.005) and fewer correct answers on the Category Fluency (p = 0.003) and Pictorial Associative Memory (p = 0.036) Tests. Confusion predicted the percentage of correct answers of the Category Fluency (p = 0.047) and the Word List Recognition Tests (p = 0.006). Altered consciousness predicted Word List Recognition d′ (p = 0.003), and dizziness predicted perseverative errors in the WCST (p = 0.035). Disorientation predicted WCST correct answers (p = 0.019), and numbness predicted WCST reaction time for trials with correct answers (p = 0.003). Speech difficulty, disturbed vision, and loss of smell/taste did not predict any cognitive outcome.
Initial Symptom Factors
As reported in our previous publication with this sample (Guo et al., 2022), we used exploratory factor analysis to reduce reported symptoms into related factors. For initial symptoms, 5 factors were identified: “Neurological/Psychiatric” (characterized by disorientation, delirium, and visual disturbances); “Fatigue/Mixed” (characterized by fatigue, chest pain/tightness, and muscle/body pains); “Gastrointestinal” (characterized by diarrhea, vomiting, and nausea); “Respiratory/Infectious” (characterized by fever, cough, and breathing issues); and “Dermatological” (characterized by itchy welts, rash and foot sores). To assess whether any of the symptom-factors predicted any aspect of the different cognitive tasks, we conducted various multiple linear regression models (backward elimination method) with the symptom factors as predictors and cognitive task as the dependent variables.
No model significantly predicted variation in the EF Performance or Category Fluency factors. Individual differences in EF Reaction Time were significantly predicted by a model which contained only the Dermatological factor ( = 0.079) and predicted over 8% of variance (Radj2 = 0.081, p < 0.001; see Figure 4). Individual differences on the Memory factor were significantly predicted by a model containing the Fatigue/Mixed factor ( = 0.061) and predicted 5.4% of variance (Radj2 = 0.054, p = 0.002).
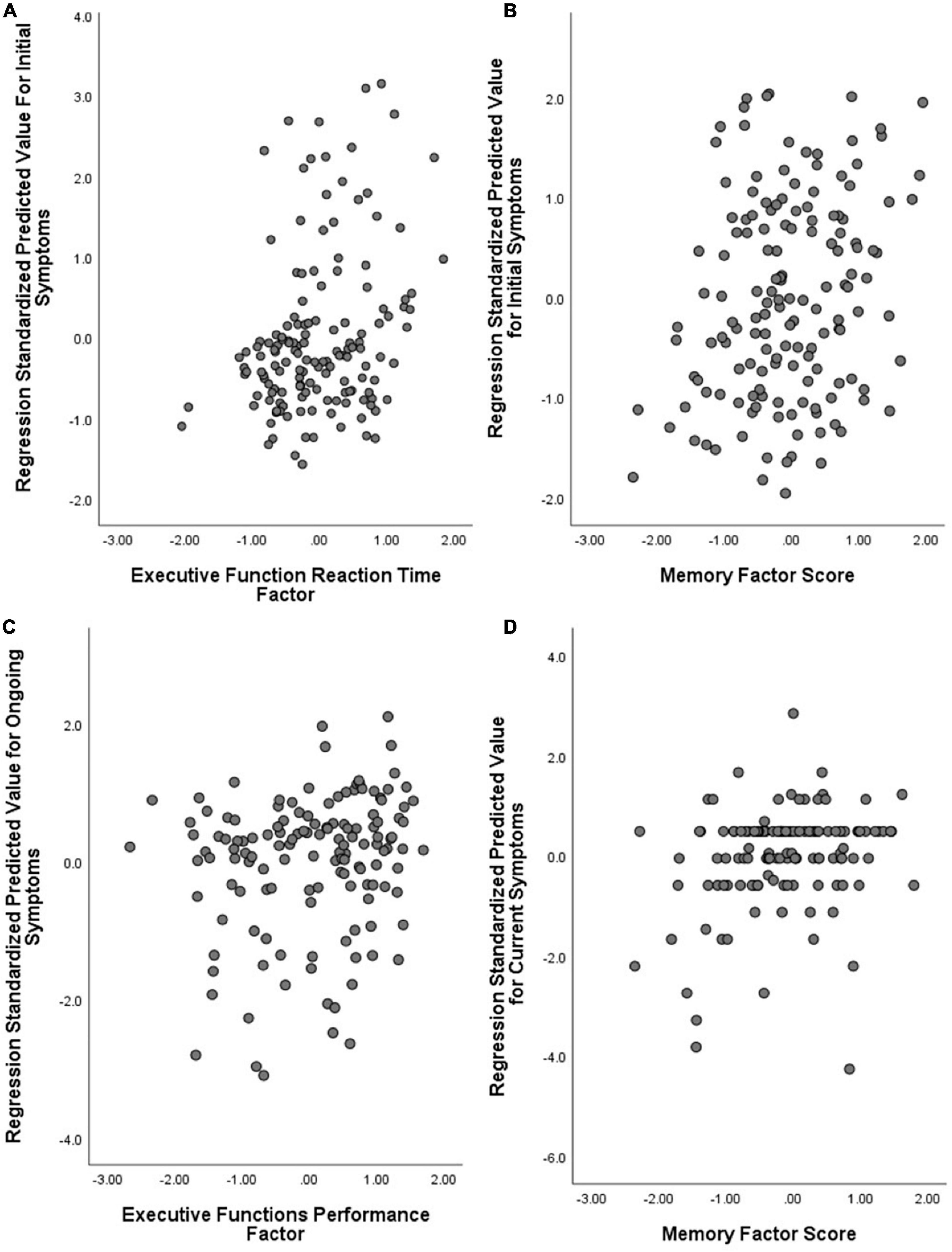
Figure 4. Symptom factors predicting cognitive task factors. (A) Initial symptoms model (Dermatological) predicting Executive Function Reaction Time; (B) Initial symptoms model (Fatigue/Mixed) predicting Memory; (C) Ongoing symptoms model (Neurological) predicting Executive Function Performance; and (D) Current symptoms model (Neurological) predicting Memory. Note that symptom factors are reversely coded (lower numbers translate to more severe symptoms).
The initial-symptom factors predicted aspects of all the individual cognitive tasks (Table 4). The Fatigue/Mixed factor predicted d′ (p = 0.008) and reaction time (p = 0.003) within the Word List Recognition Test, as well as Category Fluency correct answers (p = 0.014). The Fatigue/Mixed factor also predicted WCST reaction time (for correct answers, p = 0.002) in combination with the Dermatological factor. When the Fatigue/Mixed factor was combined with the Respiratory/Infectious factor, the significant variance was predicted in Word List % correct (p = 0.003), and the Respiratory/Infectious factor independently predicted correct choices on the WCST (p = 0.042). Finally, the Dermatological factor independently predicted reaction time in the 2D Mental Rotation Test (p = 0.001) and the Pictorial Associative Memory Test (p = 0.048). Compared against a corrected alpha (Sidak α = 0.0028), the models predicting WCST reaction time and 2D Mental Rotation maintained significance (see Table 4).
Nature of Ongoing Illness and Cognitive Performance
Ongoing Symptoms and Cognitive Performance
As reported in our previous publication with this sample (Guo et al., 2022), 6 factors were identified within the ongoing symptoms: “Neurological” (characterized by disorientation, confusion, and delirium); “Gastrointestinal/Autoimmune” (characterized by diarrhea, hot flushes, and nausea); “Cardiopulmonary/Fatigue” (characterized by breathing issues, chest pain/tightness, and fatigue); “Dermatological/Fever” (characterized by face/lips swelling, foot sores, and itchy welts); “Appetite Loss” (characterized by weight loss and loss of appetite); and “Mood” (characterized by depression, anxiety, and vivid dreams). To assess whether symptoms experienced in the time since the initial infection predicted any aspect of the different cognitive tasks, we entered the ongoing symptom factors into a series of regressions with the cognitive task variables as dependents.
For these ongoing symptoms, no model significantly predicted variance in the EF Reaction Time, Memory, or Category Fluency factors. The Neurological factor alone predicted variance in EF Performance ( = 0.031; Radj2 = 0.024, p = 0.037). Different symptom factors were able to explain variance in different individual cognitive tasks (Table 5). The Cardiopulmonary/Fatigue factor predicted a significant amount of variance in Word List % correct (p = 0.03) and reaction time in WCST trials containing correct answers (p = 0.01). The Neurological factor predicted variance in WCST correct answers (p = 0.046), and in combination with the Dermatological/Fever factor predicted performance on the WCST (p = 0.013). The Neurological factor and Mood factors together predicted % of words produced that were correct in the Category Fluency Test (p = 0.004) Finally, the Gastrointestinal/Autoimmune factor predicted variation in Word List reaction time (p = 0.046). None of these associations survived correction for multiple comparisons (Sidak α = 0.0028).
Nature of Current Illness and Cognitive Performance
The Severity of Current Illness
Given the often-cyclical nature of symptoms, participants were asked to report to what degree they were experiencing a “bad day” in terms of symptoms on the day of testing. To address the question of whether group differences in performance were due to severity of illness on the day of testing, we first assessed whether completing the test on a “bad day” impacted cognitive performance. No cognitive task factor showed any significant associations with current symptom severity. In terms of individual cognitive task variables, there were group effects in Category Fluency repetitions [F(4,117) = 5.809, p < 0.001] and 2D Mental Rotation reaction time [F(4,118) = 5.371, p = 0.001], both of which survived correction for multiple comparisons (Sidak α = 0.0028). However, no effect was directional [with the only significant correlation being with 2D Mental Rotation performance (r = –0.184, p = 0.042, which did not survive correction for multiple comparisons (Sidak α = 0.0028)].
To test whether associations between ongoing symptoms and cognitive performance were not better explained by the symptoms’ severity on the day of testing, rather than the presence of ongoing symptoms per se, we performed stepwise regressions with the cognitive task factors as the dependent, current symptom severity (good/bad day) as the first step and the ongoing symptom subgroup (R/C + /C + +) as the second step. Current symptom severity was not a significant predictor of any cognitive outcome.
Current Symptom Factors
As reported in our previous publication (Guo et al., 2022), factor scores for current symptoms were calculated from the 6 ongoing symptom factors. No current symptom factors significantly predicted individual differences in either Executive Function factors or the Category Fluency factor. A model containing the Neurological factor ( = 0.041) predicted variance in the Memory factor (Radj2 = 0.034, p = 0.018).
In terms of individual task variables, the degree to which current symptoms aligned with the Mood factor ( = 0.043) predicted the percentage of correct words in the Category Fluency Test (Radj2 = 0.037, p = 0.013), while alignment with the Dermatological/Fever factor ( = 0.029) predicted variance in the number of repetitions (Radj2 = 0.022, p = 0.043). The extent to which current symptoms aligned with the Neurological factor ( = 0.028) predicted the number of WCST perseveration errors (Radj2 = 0.021, p = 0.047). Alignment with the Cardiopulmonary/Fatigue factor ( = 0.035) predicted WCST reaction time of correct answers (Radj2 = 0.028 p = 0.025). None of the factors were associated with any variables within the Associative Memory or Word List tests. After correcting for multiple comparison (Sidak α = 0.0028), no associations were significant.
Cognitive Symptoms and Cognitive Performance
As reported in our previous publication with this sample (Guo et al., 2022), cognitive symptoms were highly prevalent. Within those currently experiencing symptoms (n = 126), 77.8% reported difficulty concentrating, 69% reported brain fog, 67.5% reported forgetfulness, 59.5% reported ToT problems and 43.7% reported semantic disfluency (saying or typing the wrong word).
A cognitive symptom factor was created separately to the non-cognitive symptoms (see Guo et al., 2022) for both ongoing and current symptoms. There was no association between the ongoing cognitive symptom factor and any cognitive task factor. In terms of individual cognitive task variables, the ongoing cognitive symptom factor significantly predicted variance in the Word List Recognition Memory Test, with more severe reported cognitive symptoms associated with lower % correct ( = 0.038; Radj2 = 0.031, p = 0.02) and slower reaction times ( = 0.039; Radj2 = 0.032, p = 0.018). Ongoing cognitive symptoms were also associated with a number of repetitions in the Category Fluency Test ( = 0.032; Radj2 = 0.025, p = 0.032) and reaction time in the 2D Mental Rotation ( = 0.029; Radj2 = 0.022, p = 0.042). However, none of these associations survived correction for multiple comparisons (Sidak α = 0.0028).
Current cognitive symptoms significantly predicted variance in the Memory factor ( = 0.046; Radj2 = 0.039, p = 0.012) only. In terms of individual variables, current cognitive symptoms significantly predicted variance in Word List performance (but not RT) metrics (d′: = 0.03; Radj2 = 0.024, p = 0.036; % correct: = 0.06; Radj2 = 0.053, p = 0.003), Category Fluency repetitions ( = 0.048; Radj2 = 0.041, p = 0.009), and 2D Mental Rotation reaction time ( = 0.041; Radj2 = 0.034, p = 0.015). However, none of these associations survived correction for multiple comparisons (Sidak α = 0.0028).
Some specific cognitive symptoms can be related directly to tests of the associated ability. Participants that reported currently experiencing forgetfulness were compared to those not reporting this symptom on measures of memory. Forgetfulness was associated with a reduced score on the overall Memory factor [t(134) = 2.111, p = 0.037; Figure 5], even when demographic variables were accounted for [F(1,120) = 8.840, p = 0.03]. For individual memory variables, those reporting forgetfulness scored significantly lower on the Word List Recognition Memory Test (U = 2.48, p = 0.013) but no difference was found for Associative Memory. After controlling for age, sex, country, and education level, no differences were significant among the individual variables.
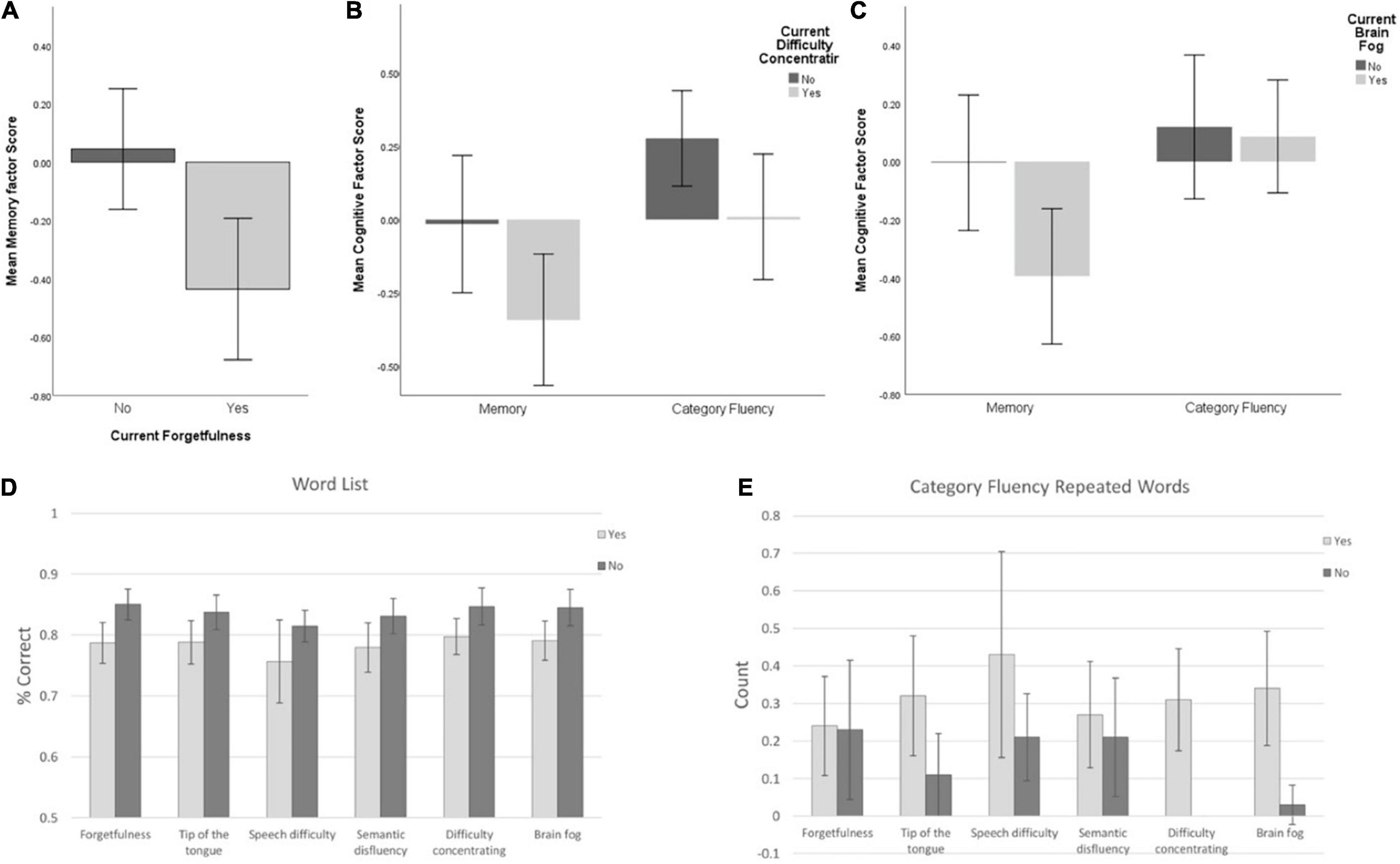
Figure 5. (A) Memory factor score for those reporting (or not) current forgetfulness. (B) Memory and Category Fluency factor scores for those reporting (or not) current difficulty concentrating or (C) brain fog. (D) Word List % correct among groups with/without current cognitive symptoms; (E) Category Fluency repetitions among groups with/without current cognitive symptoms. Error bars: ± 2 SE.
Participants reporting linguistic problems (two cognitive symptoms: ToT, semantic disfluency; one neurological symptom: speech difficulty, e.g., slurring) were compared to those not reporting these symptoms on measures of involving verbal/linguistic challenge. For the Category Fluency factor, there was no effect of ToT [t(135) = 0.414, p = 0.680], semantic disfluency [t(135) = 0.671, p = 0.503], or speech difficulty [t(16.4) = 0.039, p = 0.969]. In terms of individual linguistic (Word list and Category Fluency) variables, those reporting ToT problems trended toward lower % correct of Word List Recognition (U = 1.91, P = 0.057) and repeated significantly more words on the Category Fluency factor (U = 2.22, p = 0.026) than those without this symptom. Those reporting semantic disfluency had significant lower % correct (U = 2.49, p = 0.013) and d′ (U = 1.99, p = 0.047) on Word List Recognition than those without this symptom. Finally, those reporting speech difficulty had significantly lower % correct on Word List Recognition (U = 2.15, p = 0.031) and more repetitions on Category Fluency (U = 2.37, p = 0.018) than those not reporting this symptom. Again, after controlling for age, sex, country and education level, no differences were significant.
Finally, to establish whether any cognitive performance differences were due to “general” issues with cognition, we compared individuals experiencing “general” cognitive issues (difficulty concentrating and brain fog) to those not reporting these symptoms across all cognitive tests.
Difficulty concentrating was not associated with variance in any cognitive task factor. However, controlling for demographic variables revealed an association between reporting difficulty concentrating and lower scores on the Category Fluency factor [F(1,121) = 4.199, p = 0.043]. Brain fog was associated with significantly reduced performance on the Memory factor only [t(134) = 2.151, p = 0.033], which dropped below significance (p = 0.054) when demographic variables were accounted for. Neither Executive Function factors showed any significant association with these symptoms.
In terms of individual variables, those reporting difficulty concentrating had fewer correct words (U = 2.11, p = 0.034) and more repetitions (U = 2.74, p = 0.006) on Category Fluency but had faster reaction time on 2D Mental Rotation (U = 2.26, p = 0.024) than those not reporting this symptom. After controlling for age, sex, country and education level, these differences remained significant: Those reporting difficulty concentrating produced fewer correct words [F(1,106) = 8.19, p = 0.005] and more repetitions [F(1,106) = 4.28, p = 0.04] on Category Fluency, and reacted faster on the 2D Mental Rotation Test [F(1,107) = 5.68, p = 0.019]. However, none of these survived correction for multiple comparisons (Sidak α = 0.0028).
Those reporting brain fog had lower performance on Word List Recognition (U = 2.35, p = 0.019) and produced more repetitions in Category Fluency (U = 3.04, p = 0.002) than those not reporting this symptom. After controlling for age, sex, country and education level, the difference on Word List Recognition disappeared but those reporting brain fog still had more repetitions on Category Fluency [F(1,106) = 6.9, p = 0.01]. However, this did not survive correction for multiple comparisons (Sidak α = 0.0028).
Discussion
In this study, we present that the second subset of initial findings from a cross-sectional/longitudinal study investigating cognition post-COVID-19: The COVID and Cognition Study (COVCOG). In the first paper (Guo et al., 2022), we described the characteristics of the sample of 181 (130 women) individuals who had experienced the COVID-19 infection (74% of which self-identified as experiencing “Long COVID”) and 185 (118 women) who had not. Those who had had COVID-19 had a relatively even spread of those that had fully recovered at the time of test (n = 42) or had mild/moderate (n = 53) or severe (n = 66) ongoing symptoms. The majority of the sample fell between ages 18–60, were of White Northern European ethnicity, had attended college/university, and lived in the United Kingdom. In this second investigation, we explored how factors associated with COVID-19 infection may impact the performance on cognitive tests.
Participants were assessed on a range of cognitive tasks intended to cover different aspects of memory (verbal memory and associative memory), language (word finding), and executive functions (task switching and visual working memory). Our first hypothesis was that those who had experienced the COVID-19 infection would be likely to show deficits in tasks challenging memory and language, given the prevalence of self-reported cognitive symptoms in these areas.
We found that the fact of the COVID-19 infection (irrespective of ongoing symptoms) was associated with reduced performance on a factor created from memory task variables, but not other cognitive task factors (once demographic variables were accounted for). Detailed analysis of individual variables showed an increased reaction time when performing a verbal memory task (alongside several other Word List and Associative Memory variables, which did not survive correction for multiple comparisons). When considering the severity of ongoing symptoms, once again memory emerged as a significant factor, with those with severe ongoing symptoms performing significantly worse than those that had recovered. Looking at individual variables, the impact on verbal memory specifically became clear, with both performance (% correct) and reaction time being significantly affected by the severity of ongoing illness in a dose-dependent manner (those with severe symptoms were worse than those with mild symptoms who were worse than those that had recovered). The picture was less clear for non-verbal associative memory, which did not show the main effect (after correcting for multiple comparisons) but pairwise analyses did demonstrate a clear performance advantage in those who had not experienced the COVID-19 infection relative to those with severe ongoing symptoms. The Category Fluency word-finding task showed a similar pattern, with main effects falling below the threshold for significance once multiple comparisons were accounted for, but pairwise analysis revealing a strong negative impact of severe ongoing illness on the ability to produce category words. Looking at executive functions, similar to Hampshire et al. (2021), we found little to no effect of the COVID-19 infection on 2D Mental Rotation, which is thought to assess visuospatial working memory (Hyun and Luck, 2007). While some group differences emerged in reaction times during the WCST, these disappeared after controlling for demographic factors, suggesting that they may have been an artifact of the slightly older age of those with ongoing COVID-19 symptoms.
Long COVID is often reported to be a cyclical illness, with symptoms changing in severity over time. As such, it was important to establish whether the severity of symptoms on the day of the test (rather than in general) might account for significant variance in cognitive performance. We found that the extent to which participants reported that they were having a “bad day” in terms of symptoms on the day of the test was not directionally associated with performance on any task and did not contribute to models predicting cognitive performance from the severity of ongoing symptoms. This suggests that it was the general severity of the ongoing illness, rather than feeling ill on that day in particular, that was driving alterations in cognitive performance.
Given these findings, we suggest that, as others have found (e.g., Hampshire et al., 2021), “objective” cognitive differences do exist between those that have and have not experienced the COVID-19 infection. In particular, we found that these are related to the severity of ongoing illness (with those who report having fully recovered being, in our sample, indistinguishable from those who have not had the infection) and that they may be most pronounced in tests of verbal memory. Particular difficulties with language and verbal memory align with the frequency of self-reported deficits in these areas in other studies of Long COVID (e.g., Davis et al., 2021; Ziauddeen et al., 2021) as well as evidence for the concentration of gray matter loss in the left hemisphere (Douaud et al., 2021).
In our previous publication on the COVCOG sample (Guo et al., 2022), we reported that differences in long-term severity of Long COVID symptoms could be partially predicted by the severity and nature of the initial illness. In this study, we found that the reported severity of initial illness did not influence later performance on cognitive tasks taken. However, there was an influence on the nature of the initial illness. Using the symptom factors we introduced previously (Guo et al., 2022), we found that individual differences in the initial Dermatological symptom factor predicted around 8% of the variance in Executive Functions Reaction Times, while around 5% of the variance in Memory was predicted by individual differences in the Fatigue/Mixed initial symptom factor. These results were reflected in the individual cognitive variables, where the Fatigue/Mixed symptom factor predicted multiple memory variables (e.g., word list d′, % correct, and reaction time), while the Dermatological factor predicted Associative Memory and 2D Mental Rotation reaction time. Interestingly, the initial symptom factors predicting cognitive performance were not quite the same as those that were found to predict cognitive symptoms in our previous analysis. In our previous publication (Guo et al., 2022), we showed that a model containing all factors except the Dermatological symptom factor predicted around 20% of the variance in ongoing cognitive symptoms and that a similar model (omitting Respiratory symptoms) predicted around 14% of the variance in current cognitive symptoms. One explanation for the differential findings in this study may be that measures of reaction time may not align so closely to individuals’ perceived cognitive issues.
One hypothesis was that neurological symptoms during the acute phase may signal an increased likelihood of subsequent cognitive issues. While we found no clear association between the initial Neurological factor and cognitive function, one specific symptom, disorientation, experienced during this period predicted variance in both Executive Functions and Memory. There were also several associations between neurological symptoms experienced in the first 3 weeks and individual cognitive task variables (notably headache, altered consciousness, and numbness); however, these did not survive correction for multiple comparisons. As discussed in our previous report (Guo et al., 2022), the Fatigue/Mixed factor, while not labeled “Neurological” contains a large number of neurological symptoms, including confusion, numbness, headache, and dizziness, the latter two of which loaded more highly on the Fatigue/Mixed factor than on the Neurological/Psychiatric factor (which was more characterized by disorientation, visual disturbances, delirium, and altered consciousness). The fact that it was this factor, rather than the Neurological/Psychiatric factor, that predicted later cognitive task performance may be informative as to the mechanism of action. The Fatigue/Mixed factor might be considered to incorporate many of the expected features of systemic inflammation, in contrast to the Neurological/Psychiatric factor that is more closely linked to the neurological system only. This account accords with the other factors that emerged as predictors. While named for the fact that they affect the skin, the symptoms in the “Dermatological” factor are also linked with systemic inflammation, incorporating cross-loading symptoms such as limb weakness. These findings suggested that systemic inflammation associated with acute COVID-19 infection may have contributed to cognitive deficits across different domains up to 6 months later.
Links between systemic inflammation and cognitive functions have been previously reported in experimental (Harrison et al., 2009) and population-level studies (Gimeno et al., 2009). For example, Typhoid-vaccine-induced inflammation can lead to poorer performance in a reaction time task that was associated with systemic IL-6 levels and substantia nigra activation (Brydon et al., 2008). In an epidemiological study, higher levels of the inflammation marker, IL-6, were associated with reduced hippocampal volumes in middle-aged healthy volunteers (Marsland et al., 2008). Certain brain circuits involving the amygdala, the hippocampus, and the striatum have been particularly noted to be more sensitive to the impact of peripheral inflammation (Kraynak et al., 2018). The role of such limbic circuits in autonomic and visceromotor regulation suggests a link between peripheral inflammation physiology and implicated brain circuits. On the other hand, some studies proposed a role of the dorsolateral prefrontal cortex on peripheral inflammation via projections on the adrenal medulla that can affect attentional control (Miller et al., 2013). As such, there is a good reason to implicate systemic inflammation as a candidate causal mechanism for cognitive impacts.
In terms of ongoing symptoms, the main finding to emerge was that the Neurological factor predicted variance in Executive Function Performance, perhaps driven by an influence of this cluster of symptoms on the WCST (though no individual task variable survived correction for multiple comparisons). The Neurological factor also emerged as a significant predictor of cognitive performance among the current symptoms, this time significantly predicting variance in Memory. These associations align to some degree with the previous finding that the current cognitive symptoms were well predicted by models containing ongoing Neurological, Gastrointestinal, and Cardiopulmonary/Fatigue symptoms, and current Neurological and Cardiopulmonary/Fatigue symptoms (Guo et al., 2022). The shift in predictive power from predominantly inflammatory variables during the acute phase, to more classic neurological symptoms during the ongoing illness, raises the possibility that damages or processes instigated by an excessive immune response to infection may lead to disruption of neural function with neurological and cognitive consequences that linger independently. Such a mechanistic hypothesis would require targeted investigation of inflammatory markers, as well as functional and structural imaging.
As has been noted, the symptom factors that predicted performance on cognitive tasks were not always the same as those that predicted individual differences in cognitive symptoms. Indeed, individual differences in ongoing cognitive symptoms did not predict variance in any cognitive task performance factor. Currently experienced cognitive symptoms were, however, associated with reduced memory performance, driven by differences in multiple verbal memory tasks (particularly Word List and repetitions within the Category Fluency Test). When investigating specific cognitive symptoms, those who reported currently experiencing forgetfulness showed significantly lower Memory factor score, while those reporting linguistic issues did not score differently on the Category Fluency factor (although there were some associations with individual Category Fluency and Word List task variables that did not withstand controlling for demographic factors). The finding that those currently reporting cognitive issues-particularly memory problems—scored significantly lower on objective cognitive tasks than those experiencing ongoing symptoms but not reporting such symptoms, and that both are linked with ongoing neurological symptoms is important. It suggests that subjective experience of cognitive deficits in this population may be considered predictive of the need for neurological assessment and treatment.
In this study, one of the symptom factors included mood symptoms. Although we did not specifically examine the interplay between mood symptoms and inflammation, it is an area that warrants attention. There is substantial literature highlighting the crucial link between depression and low-grade inflammation (Dantzer et al., 2008). The extent to which COVID19-induced mood symptoms and inflammation interact—together leading to poorer cognitive performance—is an important clinical aspect for future investigation. A recent study with depressed patients showed that reaction time and processing speed were more sensitive to peripheral inflammation whereas executive functions were relatively spared (Kaser et al., 2021). Longitudinal results from our study can help understand the longstanding impact of COVID-19 induced inflammation on mood as well as cognition, and the interaction between the two.
Limitations
Many of the limitations of this study have been reviewed in our previous report (Guo et al., 2022). One major limitation of this study is that, due to the novelty of the topic, it was not designed with clear, specific hypotheses, and as such, much of the analysis was necessarily exploratory, resulting in a large number of analyses and comparisons. To account for these, Sidak alpha adjustments were used, with the result that only the very strongest effects survived at conventional statistical thresholds. We consider this conservative approach appropriate but note that it is likely to be associated with a high type 2 error rate and, thus, that some associations that did not reach these thresholds may yet be upheld upon further investigation/replication. A stated aim of this study was to generate hypotheses that could be tested in later, more targeted research, and thus while only the strongest statistical outputs should be treated as concrete findings, those that do not reach this threshold are also reported, such that they can inform and motivate future research. Of particular note is that, while rarely surviving corrections for multiple comparisons, variables associated with the Word List Recognition Memory Test repeatedly emerged as being modulated by facets of Long COVID. This is particularly relevant since it was predominantly this task that was influenced by the severity of ongoing symptoms. All elements of this task (performance and reaction time) were predicted by Fatigue/Mixed symptoms during the initial illness, and performance was related to ongoing Cardiopulmonary/Fatigue symptoms and current Neurological symptoms and Appetite Loss. Word List performance was also linked with the severity of cognitive symptoms, both ongoing and current. The consistent implication of verbal memory as vulnerable to factors associated with the COVID-19 infection should certainly warrant, further, more targeted investigation.
Another potentially notable finding that may be somewhat obscured by alpha corrections is the consistency in the association between neurological symptoms and executive function, particularly within the WCST. While the more “encephalitis-like” Neurological/Psychiatric initial symptom factor did not show associations with later WCST performance, individual elements of it (dizziness, disorientation, numbness) did. As already stated, during the ongoing illness, the Neurological factor strongly predicted the number of perseveration errors but was also associated with reduced correct responses and slower reaction times. This pattern was carried over into currently experienced symptoms, with neurological symptoms once again predicting perseveration errors. Taken as a pattern, these findings (though not all individually strong) may suggest that more severe neurological symptoms may be indicative of alterations in the frontal lobe function, evidenced by problems with response inhibition. This, again, should be investigated in more targeted future studies.
An additional limitation of this study was that the data was collected online. While online assessment facilitated cognitive testing during lockdown, and with patients from around the world, it meant that we were less able to guarantee high-quality data by ensuring that participants were in a suitable environment or concentrating properly on the task. This was mitigated to some degree by the use of the “concentration/bot check” task, which did not highlight a problem with lack of concentration. It is also increasingly becoming accepted that online cognitive testing can produce highly robust and reliable results and that Gorilla.sc is a reliable platform on which to conduct this type of research (e.g., Hilbig, 2016; Anwyl-Irvine et al., 2020). Nonetheless, future research should confirm these findings using full lab-based cognitive testing batteries.
Our study contained very few individuals who fell at either end of the severity spectrum (e.g., were asymptomatic or required ventilation). The deficits identified in the study of Hampshire et al. (2021) were substantial and related to severity, with ventilated participants showing performance reductions larger than those seen (using the same tasks) following a stroke and greater than the average 10-year decline. They also found that detectable deficits were also present in those that experienced no respiratory symptoms at all and those that did not have ongoing symptoms. In contrast, our present results suggest that those who report being completely recovered from COVID-19 were indistinguishable from those that had not experienced infection at all. This difference may be due to the relative power of the two studies (with Hampshire and colleagues having a large sample). It may also be related to differences in how symptomatology was recorded. Hampshire and colleagues only asked about “breathing difficulties” in the initial illness, and their assessment of ongoing symptoms was a sub-choice within “have you had, or suspect you have had symptoms of COVID-19” (“No”/“Yes but the symptoms passed”/“Yes currently experiencing symptoms”). Given that people’s experience of symptoms during the long-term sequelae of COVID-19 can be very different from the “Classic” COVID-19 symptoms of breathing difficulties, cough, and loss of sense of taste and smell, many individuals who were experiencing, for example, ongoing cognitive or neurological symptoms may not have considered these to qualify in this context. Further research will be necessary to clarify these discrepancies.
Long Term Risks
The accumulating neural and cognitive findings in Long COVID patient groups present a concerning picture when considering long-term cognitive health. In particular, loss of gray matter within the temporal lobe in COVID-19 (Douaud et al., 2021), along with the evidence for reduced memory performance presented here, supports the suggestion that those who have experienced the COVID-19 infection may be at increased risk for later neurodegeneration and dementia (de Erausquin et al., 2021).
While some authors have particularly highlighted the neurodegenerative risks posed via viral invasion of the central nervous system (CNS) (Douaud et al., 2021), in fact, almost all candidate mechanisms of neural impact raise the possibility of increased vulnerability to dementia. SARS-CoV-2 is increasingly being recognized as an inflammatory disease (Pearce et al., 2020; Sims et al., 2021). In addition to having major physical impacts, excessive and chronic inflammation is also associated with considerable damage in the brain. Chronic neuroinflammation is heavily implicated in the pathophysiology of neurodegenerative diseases (Chen et al., 2016), with evidence of inflammation commonly being found in the brains of patients with Alzheimer’s disease (AD) (McGeer and McGeer, 2010; Zotova et al., 2010). The dramatic impact of infections, such as Escherichia coli on survival and proliferation of hippocampal neurons (Ekdahl et al., 2003; Monje et al., 2003), has previously indicated that this region may be vulnerable to deleterious effects of inflammatory viral infection, and development of dementia following viral infections such as influenza have been previously noted (e.g., Honjo et al., 2009). COVID-19 has also been linked to abnormal blood clotting, which again has been linked to disease severity and death (Xiang-Hua et al., 2010; Tang et al., 2020; Wang et al., 2020; Wichmann et al., 2020), with microthrombi in multiple organs, including the brain (Zhang et al., 2020). Clotting is a significant factor when considering the risk for neurological damage and cognitive impairment because of the risk of CVAs and stroke (e.g., Klok et al., 2020). Indeed, an increased incidence of stroke has been reported in hospitalized patients with COVID-19 (Li et al., 2020; Oxley et al., 2020). A large proportion of stroke survivors experience cognitive impairment, and, unlike physical impairments, these tend to worsen rather than improve over time, leading to the description of “post-stroke dementia” (Mijajlović et al., 2017). Many small stroke events [“transient ischemic attacks” (TIAs)] go unnoticed at the time but may cause cumulative damage, leading to cognitive decline and dementia vulnerability. Indeed, recent studies have indicated that the proportion of dementia that is caused by small vessel ischemia may be as high as 36–67% (e.g., Seshadri and Wolf, 2007; Grau-Olivares and Arboix, 2009).
Summary
In this second investigation of the first baseline session of the COVID and Cognition study, we explored whether those who had experienced the COVID-19 infection showed measurable differences in assessments of cognitive performance. We found a consistent association between the COVID-19 infection and reduced memory performance, with those with ongoing symptoms being less accurate and slower in a test of verbal memory, but (once demographics and multiple comparisons were accounted for) there were no significant group effects in any other cognitive domain. When considering the nature of symptoms experienced, Fatigue/Mixed and Dermatological symptoms during the initial 3 weeks of illness were associated with reduced memory performance and slower reaction times on Executive Function Performance and Reaction Time tasks, respectively. Neurological symptoms during the ongoing illness were associated with performance in the Executive Function tasks, while the same symptoms experienced at the time of test predicted variance in memory. These were the most robust findings, with a conservative correction for multiple comparisons, suggesting that other identified associations may be worthy of further investigation.
In combination with previous evidence for cognitive dysfunction (e.g., Hampshire et al., 2021) and neural damage following the COVID-19 infection (Douaud et al., 2021), these findings are concerning and suggest that COVID-19 is an illness that may be associated with considerable cognitive and neurological sequelae of unknown longevity. This is particularly concerning given the potential for these changes to translate into greater vulnerability to neurodegeneration. These findings should be of note to policymakers, both in the context of post-COVID support provision and in the nature of the response to the ongoing pandemic. It is yet to be seen whether the proportion of infections that translate into Long COVID remains similar in the face of changes in both population immunity (via both vaccination and previous infection) and disease variants. However, if the current patterns persist, the long-term societal impacts of unmitigated spread may be considerable. In terms of follow-up support for patients, we reported in our previous publication (Guo et al., 2022) that a large proportion of our sample reported difficulty in getting support from medical professionals, and one reason for this may be a reluctance to consider self-reported cognitive deficits as a concrete indicator (rather than, for example, a component of general fatigue). It is thus notable that, in this study, self-reported memory issues were associated with measurable reductions in memory ability and that these are linked with other neurological symptoms. This suggests that neurological and neuropsychological assessment should be made more widely available to patients with Long COVID reporting cognitive deficits.
The COVID and Cognition participants were followed up multiple times following this assessment, and future publications with this cohort will prove informative as to the likely progression in symptoms and cognitive performance over time. However, given the associations shown in our previous publication with the number of weeks since infection (Guo et al., 2022), it seems likely that a considerable proportion of individuals may show stable cognitive symptoms over many months.
Data Availability Statement
The raw data supporting the conclusions of this article will be made available by the authors, without undue reservation.
Ethics Statement
The studies involving human participants were reviewed and approved by the Psychology Research Ethics Committee, University of Cambridge. The patients/participants provided their written informed consent to participate in this study.
Author Contributions
LGC and PG designed the study. PG, SY, AB, RL, AS, LC, and LGC recruited and collected the data. PG, AB, SY, and LC analyzed the data. LGC, PG, AB, and SY wrote the manuscript. MH provided statistical advice. MK provided medical advice and oversight. All authors contributed to the article and approved the submitted version.
Funding
This study was not supported by any funding bodies but did benefit from research funds from the Department of Psychology, University of Cambridge.
Conflict of Interest
The authors declare that the research was conducted in the absence of any commercial or financial relationships that could be construed as a potential conflict of interest.
Publisher’s Note
All claims expressed in this article are solely those of the authors and do not necessarily represent those of their affiliated organizations, or those of the publisher, the editors and the reviewers. Any product that may be evaluated in this article, or claim that may be made by its manufacturer, is not guaranteed or endorsed by the publisher.
Acknowledgments
The COVID and Cognition study has benefitted from help and support from a large number of individuals, not least the participants who gave their time. We would especially like to thank members of the Long COVID support group, particularly Claire Hastie, Barbara Melville-Johannesson, Margaret O’Hara, and Talya Varga for their time and insights. We would also like to thank Emma Weisblatt, Mirjana Bozic, and Keir Shiels who gave valuable suggestions, Honor Thompson, Aashna Malik, Connor Doyle, Abel Ashby, and Seraphina Zhang for research support, and Helen Spencer for statistical assistance.
Supplementary Material
The Supplementary Material for this article can be found online at: https://www.frontiersin.org/articles/10.3389/fnagi.2022.804937/full#supplementary-material
Footnotes
- ^ www.gorilla.sc; Cauldron Science, Cambridge, United Kingdom.
- ^ IBM, Armonk, New York, NY, United States.
References
Alberti, P., Beretta, S., Piatti, M., Karantzoulis, A., Piatti, M. L., Santoro, P., et al. (2020). Guillain-Barré syndrome related to COVID-19 infection. Neurol.Neuroimmunol. Neuroinflamm. 7, e741.
Alemanno, F., Houdayer, E., Parma, A., Spina, A., Del Forno, A., Scatolini, A., et al. (2021). COVID-19 cognitive deficits after respiratory assistance in the subacute phase: a COVID-rehabilitation unit experience. Plos one 16:e0246590. doi: 10.1371/journal.pone.0246590
Almeria, M., Cejudo, J. C., Sotoca, J., Deus, J., and Krupinski, J. (2020). Cognitive profile following COVID-19 infection: clinical predictors leading to neuropsychological impairment. Brain Behav. Immunityhealth 9:100163. doi: 10.1016/j.bbih.2020.100163
Anwyl-Irvine, A. L., Massonnié, J., Flitton, A., Kirkham, N., and Evershed, J. K. (2020). Gorilla in our midst: an online behavioral experiment builder. Behav. Res. Methods 52, 388–407. doi: 10.3758/s13428-019-01237-x
Beyrouti, R., Adams, M. E., Benjamin, L., Cohen, H., Farmer, S. F., Goh, Y. Y., et al. (2020). Characteristics of ischaemic stroke associated with COVID-19. J. Neurol. Neurosurg. Psychiatr. 91, 889–891. doi: 10.1136/jnnp-2020-323586
Bliddal, S., Banasik, K., Pedersen, O. B., Nissen, J., Cantwell, L., Schwinn, M., et al. (2021). Acute and persistent symptoms in non-hospitalized PCR-confirmed COVID-19 patients. Scientific Rep. 11, 1–11. doi: 10.1038/s41598-021-92045-x
Bougakov, D., Podell, K., and Goldberg, E. (2021). Multiple neuroinvasive pathways in COVID-19. Mol. Neurobiol. 58, 564–575. doi: 10.1007/s12035-020-02152-5
Brydon, L., Harrison, N. A., Walker, C., Steptoe, A., and Critchley, H. D. (2008). Peripheral inflammation is associated with altered substantia nigra activity and psychomotor slowing in humans. Biol. Psychiatr. 63, 1022–1029. doi: 10.1016/j.biopsych.2007.12.007
Callard, F., and Perego, E. (2021). How and why patients made Long Covid. Soc. Sci. Med. 268:113426.
Chen, W. W., Zhang, X., and Huang, W. J. (2016). Role of neuroinflammation in neurodegenerative diseases. Mol. Med. Rep. 13, 3391–3396.
Cirulli, E., Barrett, K. M. S., Riffle, S., Bolze, A., Neveux, I., Dabe, S., et al. (2020). Long-term COVID-19 symptoms in a large unselected population. [preprint]. doi: 10.1101/2020.10.07.20208702
Dantzer, R., O’Connor, J. C., Freund, G. G., Johnson, R. W., and Kelley, K. W. (2008). From inflammation to sickness and depression: when the immune system subjugates the brain. Nat. Rev. Neurosci. 9, 46–56. doi: 10.1038/nrn2297
Das, G., Mukherjee, N., and Ghosh, S. (2020). Neurological insights of COVID-19 pandemic. ACS Chem. Neurosci. 11, 1206–1209.
Davis, H. E., Assaf, G. S., McCorkell, L., Wei, H., Low, R. J., Re’em, Y., et al. (2021). Characterizing long COVID in an international cohort: 7 months of symptoms and their impact. EClin. Med. 38:3820561. doi: 10.1016/j.eclinm.2021.101019
de Erausquin, G. A., Snyder, H., Carrillo, M., Hosseini, A. A., Brugha, T. S., Seshadri, S., et al. (2021). The chronic neuropsychiatric sequelae of COVID-19: the need for a prospective study of viral impact on brain functioning. Alzheimer’s Dement. 17, 1056–1065. doi: 10.1002/alz.12255
Ding, X., Xu, J., Zhou, J., and Long, Q. (2020). Chest CT findings of COVID-19 pneumonia by duration of symptoms. Euro. J. Radiol. 127:109009. doi: 10.1016/j.ejrad.2020.109009
Douaud, G., Lee, S., Alfaro-Almagro, F., Arthofer, C., Wang, C., Lange, F., et al. (2021). Brain imaging before and after COVID-19 in UK Biobank. medRxiv [preprint]. doi: 10.1101/2021.06.11.21258690
Ehlenbach, W. J., Hough, C. L., Crane, P. K., Haneuse, S. J., Carson, S. S., Curtis, J. R., et al. (2010). Association between acute care and critical illness hospitalization and cognitive function in older adults. Jama 303, 763–770. doi: 10.1001/jama.2010.167
Ekdahl, C. T., Claasen, J.-H., Bonde, S., Kokaia, Z., and Lindvall, O. (2003). Inflammation is detrimental for neurogenesis in adult brain. Proc. Natl. Acad. Sci. 100, 13632–13637. doi: 10.1073/pnas.2234031100
Galanopoulou, A. S., Ferastraoaru, V., Correa, D. J., Cherian, K., Duberstein, S., Gursky, J., et al. (2020). EEG findings in acutely ill patients investigated for SARS-CoV-2/COVID-19: a small case series preliminary report. Epilepsia Open 5, 314–324. doi: 10.1002/epi4.12399
Gimeno, D., Kivimäki, M., Brunner, E. J., Elovainio, M., De Vogli, R., Steptoe, A., et al. (2009). Associations of C-reactive protein and interleukin-6 with cognitive symptoms of depression: 12-year follow-up of the Whitehall II study. Psychol. Med. 39, 413–423. doi: 10.1017/S0033291708003723
Girard, T. D., Jackson, J. C., Pandharipande, P. P., Pun, B. T., Thompson, J. L., Shintani, A. K., et al. (2010). Delirium as a predictor of long-term cognitive impairment in survivors of critical illness. Critic. care Med. 38:1513. doi: 10.1097/CCM.0b013e3181e47be1
Graham, E. L., Clark, J. R., Orban, Z. S., Lim, P. H., Szymanski, A. L., Taylor, C., et al. (2021). Persistent neurologic symptoms and cognitive dysfunction in non-hospitalized Covid-19 “long haulers”. Ann. Clin. Transl. Neurol. 8, 1073–1085. doi: 10.1002/acn3.51350
Grau-Olivares, M., and Arboix, A. (2009). Mild cognitive impairment in stroke patients with ischemic cerebral small-vessel disease: a forerunner of vascular dementia? Exp. Rev. Neurother. 9, 1201–1217. doi: 10.1586/ern.09.73
Guo, P., Ballesteros, A. B., Yeung, S. P., Liu, R., Saha, A., Curtis, L., et al. (2022). COVCOG 1: factors predicting cognitive symptoms in Long COVID. A first publication from the COVID and Cognition Study.”. medRxiv [preprint]. doi: 10.1101/2021.10.26.21265525
Guo, Y.-R., Cao, Q.-D., Hong, Z.-S., Tan, Y.-Y., Chen, S.-D., Jin, H.-J., et al. (2020). The origin, transmission and clinical therapies on coronavirus disease 2019 (COVID-19) outbreak–an update on the status. Military Med. Res. 7, 1–10. doi: 10.1186/s40779-020-00240-0
Hampshire, A., Trender, W., Chamberlain, S. R., Jolly, A. E., Grant, J. E., Patrick, F., et al. (2021). Cognitive deficits in people who have recovered from COVID-19. EClin. Med. 39:101044. doi: 10.1016/j.eclinm.2021.101044
Harrison, N. A., Brydon, L., Walker, C., Gray, M. A., Steptoe, A., and Critchley, H. D. (2009). Inflammation causes mood changes through alterations in subgenual cingulate activity and mesolimbic connectivity. Biol. Psychiatr. 66, 407–414. doi: 10.1016/j.biopsych.2009.03.015
Helms, J., Kremer, S., Merdji, H., Clere-Jehl, R., Schenck, M., Kummerlen, C., et al. (2020). Neurologic features in severe SARS-CoV-2 infection. N. Eng. J. Med. 382, 2268–2270.
Hilbig, B. E. (2016). Reaction time effects in lab- versus Web-based research: experimental evidence. Behav. Res. Methods 48, 1718–1724. doi: 10.3758/s13428-015-0678-9
Honjo, K., van Reekum, R., and Verhoeff, N. P. (2009). Alzheimer’s disease and infection: do infectious agents contribute to progression of Alzheimer’s disease? Alzheimer’s Dement. 5, 348–360.
Hopkins, R. O., Weaver, L. K., Pope, D., Orme, J. F. Jr., Bigler, E. D., and Larson-Lohr, V. (1999). Neuropsychological sequelae and impaired health status in survivors of severe acute respiratory distress syndrome. Am. J. Respir. Crit. Care Med. 160, 50–56. doi: 10.1164/ajrccm.160.1.9708059
Hosp, J. A., Dressing, A., Blazhenets, G., Bormann, T., Rau, A., Schwabenland, M., et al. (2021). Cognitive impairment and altered cerebral glucose metabolism in the subacute stage of COVID-19. Brain 144, 1263–1276. doi: 10.1093/brain/awab009
Hyun, J.-S., and Luck, S. J. (2007). Visual working memory as the substrate for mental rotation. Psychon. Bull. Rev. 14, 154–158. doi: 10.3758/bf03194043
Iwashyna, T. J., Ely, E. W., Smith, D. M., and Langa, K. M. (2010). Long-term cognitive impairment and functional disability among survivors of severe sepsis. Jama 304, 1787–1794. doi: 10.1001/jama.2010.1553
Jackson, J. C., Hart, R. P., Gordon, S. M., Shintani, A., Truman, B., May, L., et al. (2003). Six-month neuropsychological outcome of medical intensive care unit patients. Critic. Care Med. 31, 1226–1234. doi: 10.1097/01.CCM.0000059996.30263.94
Kandemirli, S. G., Dogan, L., Sarikaya, Z. T., Kara, S., Akinci, C., Kaya, D., et al. (2020). Brain MRI findings in patients in the intensive care unit with COVID-19 infection. Radiology 297, E232–E235. doi: 10.1148/radiol.2020201697
Kaser, M., Foley, ÉM., and Khandaker, G. M. (2021). Neurocognitive performance in depressed patients with low-grade inflammation and somatic symptoms. Brain Behav. Immunity-Health 19:100409. doi: 10.1016/j.bbih.2021.100409
Klok, F., Kruip, M., Van der Meer, N., Arbous, M., Gommers, D., Kant, K., et al. (2020). Incidence of thrombotic complications in critically ill ICU patients with COVID-19. Thrombo. Res. 191, 145–147. doi: 10.1016/j.thromres.2020.04.013
Kraynak, T. E., Marsland, A. L., Wager, T. D., and Gianaros, P. J. (2018). Functional neuroanatomy of peripheral inflammatory physiology: a meta-analysis of human neuroimaging studies. Neurosci. Biobehav. Rev. 94, 76–92. doi: 10.1016/j.neubiorev.2018.07.013
Kubánková, M., Hohberger, B., Hoffmanns, J., Fürst, J., and Herrmann, M. (2021). Physical phenotype of blood cells is altered in COVID-19. Biophys. J. 120, 2838–2847. doi: 10.1016/j.bpj.2021.05.025
Le Guennec, L., Devianne, J., Jalin, L., Cao, A., Galanaud, D., Navarro, V., et al. (2020). Orbitofrontal involvement in a neuroCOVID-19 patient. Epilepsia 61, e90–e94. doi: 10.1111/epi.16612
Lechien, J. R., Chiesa-Estomba, C. M., De Siati, D. R., Horoi, M., Le Bon, S. D., Rodriguez, A., et al. (2020). Olfactory and gustatory dysfunctions as a clinical presentation of mild-to-moderate forms of the coronavirus disease (COVID-19): a multicenter European study. Euro. Arch. Oto-Rhino-Laryngol. 277, 2251–2261.
Li, Y., Li, M., Wang, M., Zhou, Y., Chang, J., Xian, Y., et al. (2020). Acute cerebrovascular disease following COVID-19: a single center, retrospective, observational study. Stroke Vascular Neurol. 5, 279–284. doi: 10.1136/svn-2020-000431
Mao, L., Jin, H., Wang, M., Hu, Y., Chen, S., He, Q., et al. (2020). Neurologic manifestations of hospitalized patients with coronavirus disease 2019 in Wuhan. China. JAMA Neurol. 77, 683–690. doi: 10.1001/jamaneurol.2020.1127
Marsland, A. L., Gianaros, P. J., Abramowitch, S. M., Manuck, S. B., and Hariri, A. R. (2008). Interleukin-6 covaries inversely with hippocampal grey matter volume in middle-aged adults. Biol. Psychiatr. 64, 484–490. doi: 10.1016/j.biopsych.2008.04.016
Matschke, J., Lütgehetmann, M., Hagel, C., Sperhake, J. P., Schröder, A. S., Edler, C., et al. (2020). Neuropathology of patients with COVID-19 in Germany: a post-mortem case series. Lancet Neurol. 19, 919–929. doi: 10.1016/S1474-4422(20)30308-2
McGeer, E. G., and McGeer, P. L. (2010). Neuroinflammation in Alzheimer’s disease and mild cognitive impairment: a field in its infancy. J. Alzheimer’s Dis. 19, 355–361. doi: 10.3233/JAD-2010-1219
Mijajlović, M. D., Pavlović, A., Brainin, M., Heiss, W.-D., Quinn, T. J., Ihle-Hansen, H. B., et al. (2017). Post-stroke dementia–a comprehensive review. BMC Med. 15:11. doi: 10.1186/s12916-017-0779-7
Miller, A. H., Haroon, E., Raison, C. L., and Felger, J. C. (2013). Cytokine targets in the brain: impact on neurotransmitters and neurocircuits. Depress. Anx. 30, 297–306. doi: 10.1002/da.22084
Monje, M. L., Toda, H., and Palmer, T. D. (2003). Inflammatory blockade restores adult hippocampal neurogenesis. Science 302, 1760–1765. doi: 10.1126/science.1088417
Moriguchi, T., Harii, N., Goto, J., Harada, D., Sugawara, H., Takamino, J., et al. (2020). A first case of meningitis/encephalitis associated with SARS-Coronavirus-2. Int. J. Infect. Dis. 94, 55–58. doi: 10.1016/j.ijid.2020.03.062
National Institute for Health and Care Excellence (2020). COVID-19 Rapid Guideline: Managing The Long-Term Effects of COVID-19. Available online at https://www.nice.org.uk/guidance/ng1889. (accessed on Nov 11, 2021)
Nehme, M., Braillard, O., Alcoba, G., Aebischer Perone, S., Courvoisier, D., Chappuis, F., et al. (2021). COVID-19 symptoms: Longitudinal evolution and persistence in outpatient settings. Ann. Internal Med. 174, 723–725. doi: 10.7326/M20-5926
Office for National Statistics (2021). Prevalence of Ongoing Symptoms following Coronavirus (COVID-19) Infection in the UK: 1 April 2021. Available online at https://www.ons.gov.uk/peoplepopulationandcommunity/healthandsocialcare/conditionsanddiseases/bulletins/prevalenceofongoingsymptomsfollowingcoronavirusCOVID19infectionintheuk/1april2021 (accessed on Apr 1, 2021)
Oxley, T. J., Mocco, J., Majidi, S., Kellner, C. P., Shoirah, H., Singh, I. P., et al. (2020). Large-vessel stroke as a presenting feature of Covid-19 in the young. N. Engl. J. Med. 382:e60. doi: 10.1056/NEJMc2009787
Pandharipande, P. P., Girard, T. D., Jackson, J. C., Morandi, A., Thompson, J. L., Pun, B. T., et al. (2013). Long-term cognitive impairment after critical illness. N. Engl. J. Med. 369, 1306–1316.
Pearce, L., Davidson, S. M., and Yellon, D. M. (2020). The cytokine storm of COVID-19: a spotlight on prevention and protection. Exp. Opin. Ther. Targets 24, 723–730. doi: 10.1080/14728222.2020.1783243
Politi, L. S., Salsano, E., and Grimaldi, M. (2020). Magnetic resonance imaging alteration of the brain in a patient with coronavirus disease 2019 (COVID-19) and anosmia. JAMA Neurol. 77, 1028–1029. doi: 10.1001/jamaneurol.2020.2125
Poyiadji, N., Shahin, G., Noujaim, D., Stone, M., Patel, S., and Griffith, B. (2020). COVID-19–associated acute hemorrhagic necrotizing encephalopathy: imaging features. Radiology 296, E119–E120. doi: 10.1148/radiol.2020201187
Romero-Sánchez, C. M., Díaz-Maroto, I., Fernández-Díaz, E., Sánchez-Larsen, Á, Layos-Romero, A., and García-García, J. (2020). Neurologic manifestations in hospitalized patients with COVID-19: the ALBACOVID registry. Neurology 95, e1060–e1070. doi: 10.1212/WNL.0000000000009937
Royet, J. P., and Plailly, J. (2004). Lateralization of olfactory processes. Chem. Senses 29, 731–745. doi: 10.1093/chemse/bjh067
Seshadri, S., and Wolf, P. A. (2007). Lifetime risk of stroke and dementia: current concepts, and estimates from the Framingham Study. Lancet Neurol. 6, 1106–1114. doi: 10.1016/S1474-4422(07)70291-0
Sims, J. T., Krishnan, V., Chang, C.-Y., Engle, S. M., Casalini, G., Rodgers, G. H., et al. (2021). Characterization of the cytokine storm reflects hyperinflammatory endothelial dysfunction in COVID-19. J. Aller. Clin. Immunol. 147, 107–111. doi: 10.1016/j.jaci.2020.08.031
Sudre, C. H., Murray, B., Varsavsky, T., Graham, M. S., Penfold, R. S., Bowyer, R. C. E., et al. (2020). Attributes and predictors of Long-COVID: analysis of COVID cases and their symptoms collected by the Covid Symptoms Study App. Medrxiv 27, 626–631.
Tang, N., Bai, H., Chen, X., Gong, J., Li, D., and Sun, Z. (2020). Anticoagulant treatment is associated with decreased mortality in severe coronavirus disease 2019 patients with coagulopathy. J. Thrombo. Haemosta. 18, 1094–1099. doi: 10.1111/jth.14817
Tenforde, M. W., Kim, S. S., Lindsell, C. J., Billig Rose, E., Shapiro, N. I., Files, D. C., et al. (2020). Symptom duration and risk factors for delayed return to usual health among outpatients with COVID-19 in a multistate health care systems network—United States. March–June 2020. Morb. Mortal. Weekly Rep. 69, 993–998. doi: 10.15585/mmwr.mm6930e1
Wang, Z., Yang, Y., Liang, X., Gao, B., Liu, M., Li, W., et al. (2020). COVID-19 associated ischemic stroke and hemorrhagic stroke: incidence, potential pathological mechanism, and management. Front. Neurol. 11:1152. doi: 10.3389/fneur.2020.571996
Whittaker, A., Anson, M., and Harky, A. (2020). Neurological manifestations of COVID-19: a systematic review and current update. Acta Neurol. Scandin. 142, 14–22. doi: 10.1111/ane.13266
Wichmann, D., Sperhake, J.-P., Lütgehetmann, M., Steurer, S., Edler, C., Heinemann, A., et al. (2020). Autopsy findings and venous thromboembolism in patients with COVID-19: a prospective cohort study. Annal. Internal Med. 173, 268–277. doi: 10.7326/M20-2003
Xiang-Hua, Y., Le-Min, W., Ai-Bin, L., Zhu, G., Riquan, L., Xu-You, Z., et al. (2010). Severe acute respiratory syndrome and venous thromboembolism in multiple organs. Am. J. Respir. Critic. Care Med. 182, 436–437. doi: 10.1164/ajrccm.182.3.436
Zhang, J., Tecson, K. M., and McCullough, P. A. (2020). Endothelial dysfunction contributes to COVID-19-associated vascular inflammation and coagulopathy. Rev. Cardiovasc. Med. 21, 315–319. doi: 10.31083/j.rcm.2020.03.126
Zhao, H., Shen, D., Zhou, H., Liu, J., and Chen, S. (2020). Guillain-Barré syndrome associated with SARS-CoV-2 infection: causality or coincidence? Lancet Neurol. 19, 383–384. doi: 10.1016/s1474-4422(20)30109-5
Ziauddeen, N., Gurdasani, D., O’Hara, M. E., Hastie, C., Roderick, P., Yao, G., et al. (2021). Characteristics of Long Covid: findings from a social media survey. medRxiv [preprint]. doi: 10.1101/2021.03.21.21253968
Keywords: Long COVID, cognition, neurological, memory, executive functions, language, COVID-19, symptoms
Citation: Guo P, Benito Ballesteros A, Yeung SP, Liu R, Saha A, Curtis L, Kaser M, Haggard MP and Cheke LG (2022) COVCOG 2: Cognitive and Memory Deficits in Long COVID: A Second Publication From the COVID and Cognition Study. Front. Aging Neurosci. 14:804937. doi: 10.3389/fnagi.2022.804937
Received: 29 October 2021; Accepted: 17 February 2022;
Published: 17 March 2022.
Edited by:
Flávia H. Santos, University College Dublin, IrelandReviewed by:
Julio Torales, National University of Asunción, ParaguayOlagide Wagner Castro, Federal University of Alagoas, Brazil
Copyright © 2022 Guo, Benito Ballesteros, Yeung, Liu, Saha, Curtis, Kaser, Haggard and Cheke. This is an open-access article distributed under the terms of the Creative Commons Attribution License (CC BY). The use, distribution or reproduction in other forums is permitted, provided the original author(s) and the copyright owner(s) are credited and that the original publication in this journal is cited, in accordance with accepted academic practice. No use, distribution or reproduction is permitted which does not comply with these terms.
*Correspondence: Lucy G. Cheke, TGdjMjNAY2FtLmFjLnVr