- 1Department of Preventive Medicine, Institute of Nutrition and Diseases, Wenzhou Medical University, Wenzhou, China
- 2Department of Neurology, Institute of Geriatric Neurology, The Second Affiliated Hospital and Yuying Children’s Hospital, Wenzhou Medical University, Wenzhou, China
- 3Department of Clinical Laboratory, The Second Affiliated Hospital and Yuying Children’s Hospital, Wenzhou Medical University, Wenzhou, China
Background: Environmental substances such as pesticides are well-known in link with Parkinson’s disease (PD) risk. Enzymes including cytochromes P450 (CYPs), esterases and glutathione S-transferases (GSTs) are responsible for the xenobiotic metabolism and may functionally compensate each other for subtypes in the same class. We hypothesize that the genetic effects of each class modulate PD risk stronger in a synergistic way than individually.
Methods: We selected 14 polymorphic loci out of 13 genes which encode enzymes in the classes of CYP, esterase, and GST, and recruited a cohort of 1,026 PD and control subjects from eastern China. The genotypes were identified using improved multiplex ligation detection reaction and analyzed using multiple models.
Results: A total of 13 polymorphisms remained after Hardy-Weinberg equilibrium analysis. None of the polymorphisms were independently associated with PD risk after Bonferroni correction either by logistic regression or genetic models. In contrast, interaction analyses detected increased resistance to PD risk in individuals carrying the rs12441817/CC (CYP1A1) and rs2070676/GG + GC (CYP2E1) genotypes (P = 0.002, OR = 0.393, 95% CI = 0.216–0.715), or carrying the GSTM1-present, GSTT1-null, rs156697/AG + GG (GSTO2) and rs1695/AA (GSTP1) genotypes (P = 0.003, OR = 0.348, 95% CI = 0.171–0.706). The synergistic effect of GSTs on PD was primarily present in females (P = 0.003). No synergistic effect was observed within genotypes of esterases.
Conclusion: We demonstrate a presence of synergistic but not individual impact on PD susceptibility in polymorphisms of CYPs and GSTs. The results indicate that the genetic interplay leads the way to PD development for xenobiotic metabolizing enzymes.
Introduction
Parkinson’s disease (PD) is the second most common neurodegenerative disease, affecting 1.14 and 1.06% of people over 60 years old worldwide and in China, respectively (Pringsheim et al., 2014; Cui et al., 2020). Only a minority of PD are known with genetic causes and familial hereditary, such as mutations in SNCA, LRRK2, and PARKIN. In contrast, most PD cases are sporadic with unclear etiology. Complicated interactions between genetic susceptibilities and environmental exposures are considered to be responsible for the pathogenesis of sporadic PD (Kalia and Lang, 2015).
The chemical 1-methyl-4phenyl-1,2,3,6-tetrahydropyridine (MPTP) was discovered to induce parkinsonism in humans in the 1980s (Langston et al., 1984). MPTP was later found to be structurally similar to some known pesticides such as paraquat, raising the question whether pesticide exposures were associated with PD (Wirdefeldt et al., 2011). Multiple kinds and classes of pesticides have since been identified to link to PD development. For example, exposure to herbicide paraquat, insecticide rotenone or fungicide maneb leads to toxic effects similar to those of MPTP in animal models (Islam et al., 2021). Benomyl, another fungicide, inhibits aldehyde dehydrogenase activity, and results in accumulation of 3,4-dihydroxyphenylacetaldehyde (DOPAL), a dopamine metabolite highly toxic to dopaminergic neurons (Fitzmaurice et al., 2013). Organophosphate pesticides are also shown to be associated with increased risk of PD, probably due to the inhibition of acetylcholinesterase activity and the induction of oxidative stress (Sanchez-Santed et al., 2016). In addition, pesticide exposure exhibits the strongest association with PD among 11 environmental risk factors identified in a meta-analysis (Noyce et al., 2012).
Pesticides are metabolized in vivo by phase I enzymes such as cytochromes P450 (CYPs) and esterases into reactive metabolites. Phase II enzymes such as glutathione S-transferases (GSTs) then detoxify the reactive metabolites through catalytic conjugation with charged species such as reduced glutathione (Omiecinski et al., 2011). Genetic liabilities of these enzymes to diseases have been widely studied such as in context of diabetes, cancer and cardiovascular disorders (Gao et al., 2017; Elfaki et al., 2018). It has also been investigated whether the enzymes are genetically associated with PD. However, some of the results remain inconsistent and inconclusive, such as for CYP2D6, and GSTM1 (Ahmadi et al., 2000; Chan et al., 2003; Elbaz et al., 2004; Perez-Pastene et al., 2007).
Since the same class of xenobiotic metabolizing enzymes may functionally compensate each other or overlap, we hypothesize that the genetic effects of each class modulate PD risk stronger in a synergistic way than individually. We selected 14 polymorphic loci out of 13 genes which encode metabolizing enzymes of three classes, GSTs, esterases, and CYPs (Supplementary Table 1). Amongst, GSTM1 and GSTT1 are two deletion polymorphisms, and the remaining are single nucleotide polymorphisms (SNPs). These polymorphisms are either functional in modulating protein expression or enzyme activity or are reportedly associated with PD risk (Supplementary Table 1). Their genetic effects on PD were herein investigated individually and synergistically in a large Chinese cohort.
Materials and Methods
Subjects
A total of 1026 Han Chinese subjects were recruited from eastern China, including 527 sporadic PD cases (270 males and 257 females) and 499 controls (270 males and 229 females). The median age of the cases and controls was 66 (interquartile range, 59–73) and 58 (interquartile range, 50–68), respectively. PD cases were diagnosed by two neurologists according to the UK Parkinson’s Disease Society Brain Bank Criteria (Hughes et al., 1992). Patients with a family history of PD, or with secondary and atypical parkinsonism were excluded. Control subjects were free of neurological disorders determined by medical history, physical and laboratory examinations. Informed written consents were obtained from all participants or their legal representatives. The study was approved by the Ethics Committee of the Second Affiliated Hospital and Yuying Children’s Hospital, Wenzhou Medical University.
Genotyping
Genomic DNA was extracted from peripheral blood using TIANamp Genomic DNA kit (Tiangen, Beijing, China) according to the manufacturer’s instruction. The candidate polymorphisms were genotyped using the improved multiplex ligation detection reaction (iMLDR) at Genesky Biotechnologies (Shanghai, China). Specifically, the alleles of each polymorphism were distinguished by different fluorescent labels of allele specific oligonucleotide probe pairs. Different polymorphisms were further distinguished by different extended lengths at 3′ end. Four percent of the total samples were randomly selected and repeated as quality control, which showed consistent results.
Statistical Analysis
All data were analyzed using the Statistical Package for Social Science program (version 23.0). The χ2-test was used to assess Hardy-Weinberg equilibrium in genotype distribution and the difference in gender. Following Kolmogorov-Smirnov test for normality, Mann-Whitney U-test was used to evaluate age difference. The differences in genotype and allele frequencies, as well as the interactions between polymorphisms were analyzed using logistic regression model with gender and age as covariates. Homozygous genotypes of low frequency less than 0.05 were with limited sample size and were then grouped into their respective heterozygous genotypes during the interaction analysis to increase the statistical power (Mazaheri et al., 2006; Oliveira-Paula et al., 2021). Genetic models were analyzed using SNPStats software at https://www.snpstats.net. A backward elimination method as reported previously was used to identify the highest-risk genotype combination for PD (Ionita-Laza et al., 2014). Significance was set at α = 0.05.
Results
Association Analyses of the Candidate Polymorphisms With Parkinson’s Disease Susceptibility
The PD cases and controls were comparable in gender (P > 0.05) but different in age (P < 0.05). Genotype distributions of the polymorphisms in controls met with Hardy-Weinberg equilibrium (P > 0.05), except for CYP2D6-rs1065852 (P = 0.002). This variant was then removed from later analyses. Differences of the remaining 13 variants in genotype and allele frequencies were analyzed between the PD cases and controls (Table 1). A difference (P < 0.05) appeared in the allele frequencies of rs762551 (CYP1A2) and rs156697 (GSTO2). However, after Bonferroni correction (threshold for significance = 0.05/13, that is, 0.0038), none of the polymorphisms were significantly different in genotype and allele frequencies between the PD cases and controls.
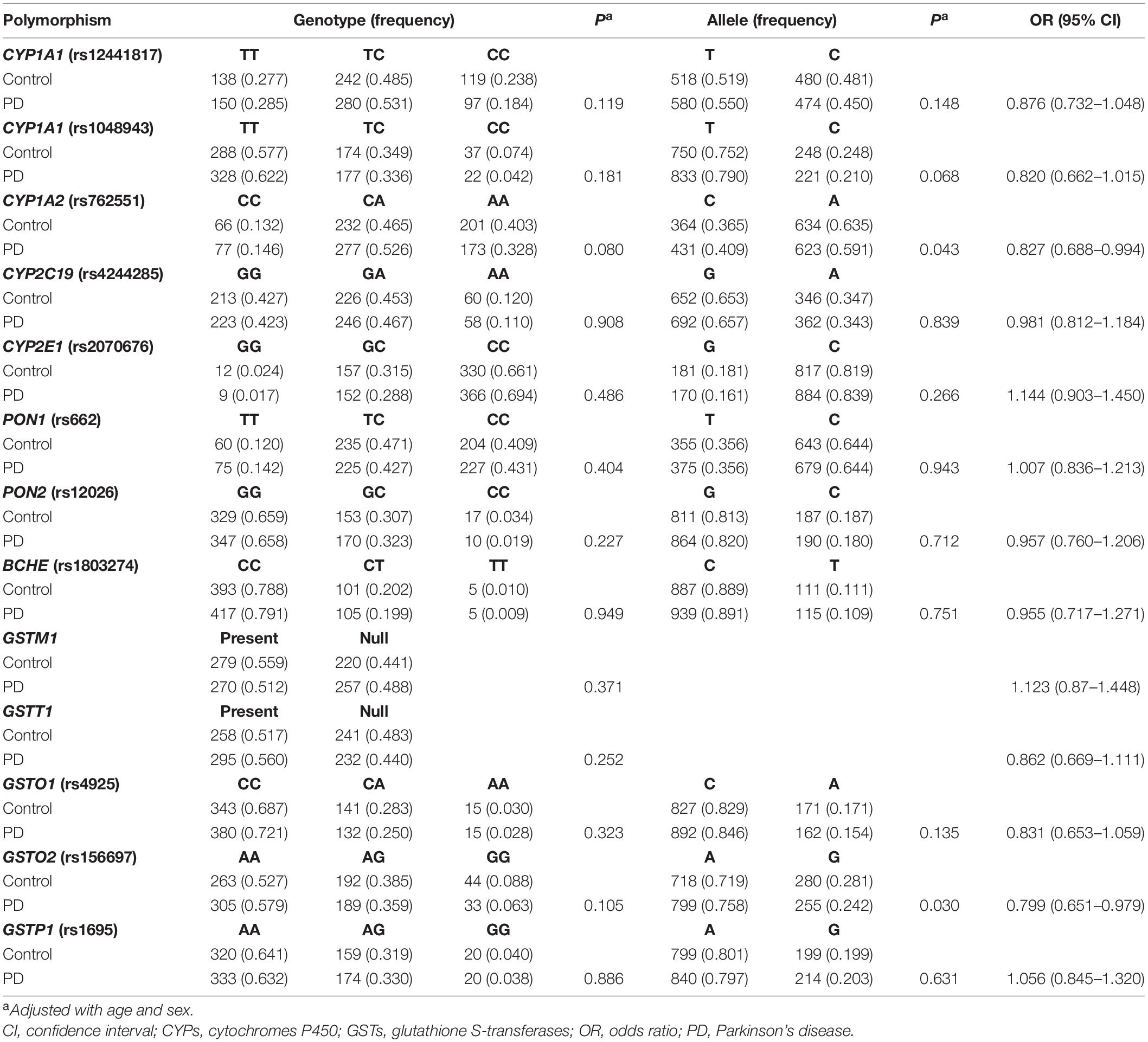
Table 1. Genotype and allele frequencies of polymorphisms of CYPs, esterases and GSTs in PD patients and controls.
Association Analyses of the Polymorphisms With Parkinson’s Disease in Genetic Models
Except for the deletion polymorphisms of GSTM1 and GSTT1, we analyzed the remaining 11 polymorphisms in association with PD using multiple genetic models including dominant, recessive and additive. As shown in Table 2, an association at P < 0.05 was present in rs12441817 (CYP1A1) and rs762551 (CYP1A2) when using the recessive model, and in rs762551 (CYP1A2) and rs156697 (GSTO2) when using the additive model. However, the associations were no longer considered valid following Bonferroni correction (threshold for significance = 0.05/11, that is, 0.0045).
Analyses of the Synergistic Effect of the Polymorphisms on Parkinson’s Disease Risk
We divided the 13 polymorphisms into three enzyme classes, GSTs, esterases and CYPs, and assessed the potential synergistic effect of each class on PD risk. To serve this purpose, the genotypes of each SNP were sorted into two groups with the following rationales: the homozygous genotype with frequency lower than 0.05 was clustered into the heterozygous genotype as indicated in “Materials and Methods” section, otherwise the dominant or recessive model with smaller P-value was adopted for sorting. The genotypes of GSTM1 and GSTT1 were as it is. Group details were listed in the table footnotes. Results showed that none of the genotype combinations were significantly associated with PD within the class of five GSTs, three esterases or five CYPs (threshold for significance at 0.0038 after Bonferroni correction; Supplementary Table 2).
The backward elimination model was then used to detect stronger synergistic effect of the enzymes on PD starting from the combinations with the smallest P-value in each class (Supplementary Table 2 and Table 3). Results for CYPs showed that individuals simultaneously carrying the rs12441817/CC (CYP1A1), rs762551/AA (CYP1A2), and rs2070676/GG + GC genotypes (CYP2E1) were more resistant to PD susceptibility (P = 0.002, OR = 0.354, 95% CI = 0.184–0.682), while simultaneous presence of the rs12441817/CC and rs2070676/GG + GC genotypes appeared to play the core protective effect (P = 0.002, OR = 0.393, 95% CI = 0.216–0.715; Table 3). No significant difference was observed in the gender subgroups. Results for GSTs showed that individuals carrying the GSTM1-present, GSTT1-null, rs156697/AG + GG GSTO2), and rs1695/AA (GSTP1) genotypes displayed an increased resistance to PD (P = 0.003, OR = 0.348, 95% CI = 0.171–0.706), wherein a gender-dependent effect was observed with significance in females (P = 0.003) but not in males (Table 3). Results for esterases suggested no synergistic effect on PD in the total cohort, neither in the gender subgroups (Table 3).
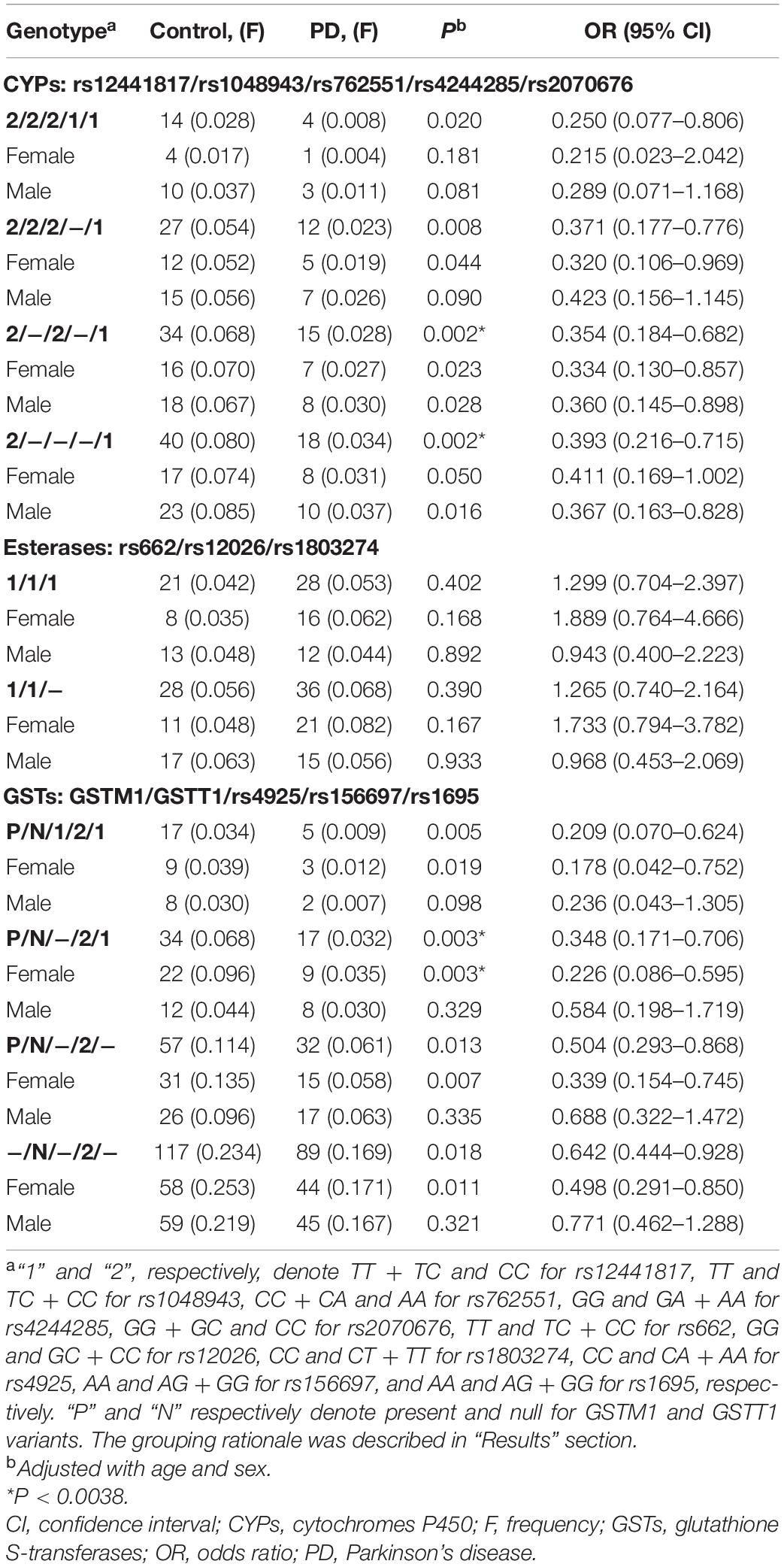
Table 3. Interaction analyses of polymorphisms of CYPs, esterases and GSTs using backward elimination model.
Discussion
Xenobiotic metabolizing enzymes are key to the retention and deposition of environmental substances in human bodies. The exposures, in particular pesticide, have been well-recognized to aggravate PD risk. The association between pesticide and PD may be more prominent in eastern China due to the higher pesticide residues (Yu et al., 2020). By analyzing 13 functional or potential PD-associated polymorphisms in genes which encode three classes of metabolizing enzymes, including GSTs, CYPs, and esterases, we demonstrate that none of the polymorphisms are independently associated with PD risk. These observations are in line with results of a previous genome-wide association study (GWAS) in East Asian (Foo et al., 2020). On the other hand, there are synergistic effects on PD in polymorphisms of CYPs and GSTs, that is, the protection conferred, respectively, by simultaneous presence of the rs12441817/CC (CYP1A1) and rs2070676/GG + GC (CYP2E1) genotypes, as well as of the GSTM1-present, GSTT1-null, rs156697/AG + GG (GSTO2) and rs1695/AA (GSTP1) genotypes.
CYPs are the class of the most important phase I enzymes responsible for metabolism of various xenobiotic compounds, including drugs and pesticides (Elfaki et al., 2018). Genetic polymorphisms in CYPs are associated with altered enzymatic activities and disturbed expression levels. The changes in activity or expression may lead to inter-individual variations in xenobiotic metabolisms, and are implicated in various disease susceptibilities (Dardiotis et al., 2013; Elfaki et al., 2018). In context of PD, the C allele of rs12441817 (CYP1A1) was shown to be associated with reduced risk of PD (P = 5.22 × 10–5, OR = 0.87, 95% CI = 0.81–0.93) in a European-descent based GWAS (Nalls et al., 2014). However, this association is not confirmed by the current study and the Asian GWAS (Foo et al., 2020). We are not sure whether it is ethnicity specific. In addition, rs2070676 (CYP2E1) showed an association with PD in a Swedish population (Shahabi et al., 2009), rs1048943 (CYP1A1) was associated with PD in a Japanese population (Takakubo et al., 1996), and rs762551 (CYP1A2) was reported to have a marginal association with PD risk in the United States and Denmark (Palacios et al., 2010; Chuang et al., 2016). Nonetheless, these associations are not independently observed in the current study, the Asian GWAS (Foo et al., 2020), the European GWAS (Nalls et al., 2014), and other case-control studies (Chan et al., 2002; Facheris et al., 2008; Siokas et al., 2021). As noted in Supplementary Table 1, the rs1048943 is a missense variant of CYP1A1 (T > C, Ile462Val) and functionally regulates the CYP1A1 enzyme activity (Shah et al., 2009). The CYP1A2 activity is also influenced by its rs762551 polymorphism (Denden et al., 2016). The rs4244285 is the most studied polymorphism of CYP2C19, and can alter the CYP2C19 mRNA reading frame leading to a truncated non-functional protein (Scott et al., 2012). As discussed above, these polymorphisms are not, at least not consistently, shown to be associated with PD, suggesting that bluntness of each enzyme per se may not be sufficient to affect PD susceptibility. On the other hand, our results demonstrate that simultaneous presence of the rs12441817/CC (CYP1A1) and rs2070676/GG + GC (CYP2E1) genotypes confers protection against PD risk, suggesting a synergistic genetic effect from these two enzymes. Coincidentally, the rs12441817/C and the rs2070676/G indeed served as the protective allele in the two positive studies (Shahabi et al., 2009; Nalls et al., 2014), which to some extent supports our finding. These results together indicate that a synergistic play more likely leads to a modulation of PD risk than individually.
Esterases, including paraoxonase 1 (PON1), paraoxonase 2 (PON2), and butyrylcholinesterase (BCHE), are responsible for catalyzing ester hydrolysis during Phase I reactions, and are critical in organophosphate metabolism (Satoh et al., 2002). Two of the most studied polymorphisms of PON1 are rs854560 (A > T, Leu55Met) and rs662 (T > C, Gln192Arg). The rs854560/A and rs662/C alleles result in higher PON1 enzyme activity (Rejeb et al., 2013). The rs854560 was excluded from the current study due to a low minor allele frequency in East Asians (T allele at 0.034; from gnomAD).1 The rs12026 of PON2 (G > C, Ala148Gly) and rs1803274 of BCHE (G > A, Ala539Thr) are linked with lower enzyme activity at presence of the C and A allele, respectively (Rejeb et al., 2013; Habieb et al., 2021). Nonetheless, neither individual nor synergistic effect on PD risk is detected in these variants, suggesting that esterases may not be important in modulating the environmental and genetic interplay toward PD risk. Besides, there are two previous reports for rs662 and PD with results showing positive association (Kondo and Yamamoto, 1998) and no association (Wang and Liu, 2000).
The current study detects no individual association between the GST polymorphisms and PD risk. The rs4925 (GSTO1), rs156697 (GSTO2), and rs1695 (GSTP1) have not been reported in Chinese PD cohorts till now. The GSTM1 and GSTT1 deletion polymorphisms have been previously reported in relatively small Chinese populations, also showing no association with PD (Tao et al., 1998; Fong et al., 2006). The lack of PD association in these variants has been additionally observed in other ethnicities, including Caucasians, Australians and Japanese (Menegon et al., 1998; Ahmadi et al., 2000; Harada et al., 2001; Whitbread et al., 2004). Nonetheless, reports of significant association with PD also exist, such as for the GSTM1 variant in Chileans (Perez-Pastene et al., 2007), the GSTT1 variant in a UK population (De Palma et al., 1998), and the rs4925 in Americans (Wahner et al., 2007). Regardless of the individual performance, the present study for the first time reports a protection against PD synergistically conferred by the genotypes of GSTM1-present, GSTT1-null, rs156697/AG + GG (GSTO2) and rs1695/AA (GSTP1). The GSTM1-present and the rs1695/A allele are linked with elevated enzyme activities (Johansson et al., 1998; Hayes and Strange, 2000). In contrast, the GSTT1-null and the rs156697/G lead to reduced enzyme activities or protein levels (Hayes and Strange, 2000; Allen et al., 2012). Thus, the combination of these four genotypes appears to be mediocre in terms of collective enzyme activity. Interestingly, there is a similar case showing a synergistic effect from the alleles of GSTM1-null, GSTT1-present, GSTA1/T (low activity), and GSTP1/G (low activity) on promoting risk for clear cell renal cell carcinoma (Coric et al., 2016). In certain cases, high GST activity may lead to increased toxicity, such that GSTT1 dehalogenates and transforms halogenated organic compounds to toxic products (Landi, 2000). In addition, the expression of GSTs may be regulated by sex hormones (Coecke et al., 2000), which may partially explain our observed female-specific synergistic effect.
Conclusion
In conclusion, the current study demonstrates that polymorphisms of CYPs and GSTs, but not those of esterases, have synergistic impact on PD susceptibility. The variants are not associated with PD individually. Our findings suggest that more attention should be paid to the synergistic interplay for the genetic variations of xenobiotic metabolizing enzymes to better understand their roles in PD risk.
Data Availability Statement
The datasets presented in this study can be found in online repositories. The names of the repository/repositories and accession number(s) can be found below: https://datadryad.org/stash, https://datadryad.org/stash/share/bcA9u9G-dGMdGLw_TnpEtY9qPeJg7aXdRA7u35_tgxs.
Ethics Statement
The studies involving human participants were reviewed and approved by the Ethics Committee of the Second Affiliated Hospital and Yuying Children’s hospital, Wenzhou Medical University. The patients/participants provided their written informed consent to participate in this study.
Author Contributions
H-HF designed the study. H-HF, K-YW, H-DY, M-JG, X-HY, and H-JD conducted the experiments. H-HF and J-HZ analyzed the data. B-QL and XZ contributed samples. H-HF and J-HZ wrote the manuscript. XZ and J-HZ supervised the study. All authors contributed to the article and approved the submitted version.
Funding
This work was supported by the Zhejiang Provincial Natural Science Foundation (LD19H090001 and LZ19H090002), the National Natural Science Foundation of China (82071585), and Science and Technology Department of Zhejiang Province (2018C37111).
Conflict of Interest
The authors declare that the research was conducted in the absence of any commercial or financial relationships that could be construed as a potential conflict of interest.
Publisher’s Note
All claims expressed in this article are solely those of the authors and do not necessarily represent those of their affiliated organizations, or those of the publisher, the editors and the reviewers. Any product that may be evaluated in this article, or claim that may be made by its manufacturer, is not guaranteed or endorsed by the publisher.
Acknowledgments
We are thankful to their colleagues for clinical data collection and management of patients. We appreciate all the subjects for participating in this study.
Supplementary Material
The Supplementary Material for this article can be found online at: https://www.frontiersin.org/articles/10.3389/fnagi.2022.888942/full#supplementary-material
Footnotes
References
Ahmadi, A., Fredrikson, M., Jerregard, H., Akerback, A., Fall, P. A., Rannug, A., et al. (2000). GSTM1 and mEPHX polymorphisms in Parkinson’s disease and age of onset. Biochem. Biophys. Res. Commun. 269, 676–680. doi: 10.1006/bbrc.2000.2338
Allen, M., Zou, F., Chai, H. S., Younkin, C. S., Miles, R., Nair, A. A., et al. (2012). Glutathione S-transferase omega genes in Alzheimer and Parkinson disease risk, age-at-diagnosis and brain gene expression: an association study with mechanistic implications. Mol. Neurodegener. 7:13. doi: 10.1186/1750-1326-7-13
Chan, D. K., Mellick, G. D., Buchanan, D. D., Hung, W. T., Ng, P. W., Woo, J., et al. (2002). Lack of association between CYP1A1 polymorphism and Parkinson’s disease in a Chinese population. J. Neural Transm. 109, 35–39. doi: 10.1007/s702-002-8234-8
Chan, D. K., Mellick, G. D., Hung, W. T., and Woo, J. (2003). Genetic and environmental risk factors and their interactions for Parkinson’s disease in a Chinese population. J. Clin. Neurosci. 10, 313–315. doi: 10.1016/s0967-5868(03)00014-6
Chuang, Y. H., Lill, C. M., Lee, P. C., Hansen, J., Lassen, C. F., Bertram, L., et al. (2016). Gene-Environment Interaction in Parkinson’s Disease: coffee, ADORA2A, and CYP1A2. Neuroepidemiology 47, 192–200. doi: 10.1159/000450855
Coecke, S., Vanhaecke, T., Foriers, A., Phillips, I. R., Vercruysse, A., Shephard, E. A., et al. (2000). Hormonal regulation of glutathione S-transferase expression in co-cultured adult rat hepatocytes. J. Endocrinol. 166, 363–371. doi: 10.1677/joe.0.1660363
Coric, V. M., Simic, T. P., Pekmezovic, T. D., Basta-Jovanovic, G. M., Savic Radojevic, A. R., Radojevic-Skodric, S. M., et al. (2016). Combined GSTM1-Null, GSTT1-Active, GSTA1 low-activity and GSTP1-variant genotype is associated with increased risk of clear cell renal cell carcinoma. PLoS One 11:e0160570. doi: 10.1371/journal.pone.0160570
Cui, L., Hou, N. N., Wu, H. M., Zuo, X., Lian, Y. Z., Zhang, C. N., et al. (2020). Prevalence of Alzheimer’s disease and Parkinson’s Disease in China: an updated systematical analysis. Front. Aging Neurosci. 12:603854. doi: 10.3389/fnagi.2020.603854
Dardiotis, E., Xiromerisiou, G., Hadjichristodoulou, C., Tsatsakis, A. M., Wilks, M. F., and Hadjigeorgiou, G. M. (2013). The interplay between environmental and genetic factors in Parkinson’s disease susceptibility: the evidence for pesticides. Toxicology 307, 17–23. doi: 10.1016/j.tox.2012.12.016
De Palma, G., Mozzoni, P., Mutti, A., Calzetti, S., and Negrotti, A. (1998). Case-control study of interactions between genetic and environmental factors in Parkinson’s disease. Lancet 352, 1986–1987. doi: 10.1016/s0140-6736(05)61332-3
Denden, S., Bouden, B., Haj Khelil, A., Ben Chibani, J., and Hamdaoui, M. H. (2016). Gender and ethnicity modify the association between the CYP1A2 rs762551 polymorphism and habitual coffee intake: evidence from a meta-analysis. Genet. Mol. Res. 15:gmr7487. doi: 10.4238/gmr.15027487
Elbaz, A., Levecque, C., Clavel, J., Vidal, J. S., Richard, F., Amouyel, P., et al. (2004). CYP2D6 polymorphism, pesticide exposure, and Parkinson’s disease. Ann. Neurol. 55, 430–434. doi: 10.1002/ana.20051
Elfaki, I., Mir, R., Almutairi, F. M., and Duhier, F. M. A. (2018). Cytochrome P450: polymorphisms and roles in cancer, diabetes and atherosclerosis. Asian Pac. J. Cancer Prev. 19, 2057–2070. doi: 10.22034/APJCP.2018.19.8.2057
Facheris, M. F., Schneider, N. K., Lesnick, T. G., de Andrade, M., Cunningham, J. M., Rocca, W. A., et al. (2008). Coffee, caffeine-related genes, and Parkinson’s disease: a case-control study. Mov. Disord. 23, 2033–2040. doi: 10.1002/mds.22247
Fitzmaurice, A. G., Rhodes, S. L., Lulla, A., Murphy, N. P., Lam, H. A., O’Donnell, K. C., et al. (2013). Aldehyde dehydrogenase inhibition as a pathogenic mechanism in Parkinson disease. Proc. Natl. Acad. Sci. U.S.A. 110, 636–641. doi: 10.1073/pnas.1220399110
Fong, C., Cheng, C., and Wu, R. (2006). Modification of pesticide exposure in correlation with glutathione transferase (GST) polymorphisms for the susceptibility risk of sporadic Parkinson’s diseases: P641. Mov. Disord. 21, S501–S502.
Foo, J. N., Chew, E. G. Y., Chung, S. J., Peng, R., Blauwendraat, C., Nalls, M. A., et al. (2020). Identification of risk loci for parkinson disease in asians and comparison of risk between asians and europeans a genome-wide association study. JAMA Neurol. 77, 746–754. doi: 10.1001/jamaneurol.2020.0428
Gao, Y., Gao, F., Hu, T. T., Li, G., and Sui, Y. X. (2017). Combined effects of glutathione S-transferase M1 and T1 polymorphisms on risk of lung cancer: evidence from a meta-analysis. Oncotarget 8, 28135–28143. doi: 10.18632/oncotarget.15943
Habieb, M. S., Elhelbawy, N. G., Alhanafy, A. M., Elhelbawy, M. G., Alkelany, A. S., and Wahb, A. M. (2021). Study of the potential association of the BCHE rs1803274 genetic polymorphism and serum level of its protein with breast cancer. Meta Gene 29:100913. doi: 10.1016/j.mgene.2021.100913
Harada, S., Fujii, C., Hayashi, A., and Ohkoshi, N. (2001). An association between idiopathic Parkinson’s disease and polymorphisms of phase II detoxification enzymes: glutathione S-transferase M1 and quinone oxidoreductase 1 and 2. Biochem. Biophys. Res. Commun. 288, 887–892. doi: 10.1006/bbrc.2001.5868
Hayes, J. D., and Strange, R. C. (2000). Glutathione S-transferase polymorphisms and their biological consequences. Pharmacology 61, 154–166. doi: 10.1159/000028396
Hughes, A. J., Daniel, S. E., Kilford, L., and Lees, A. J. (1992). Accuracy of clinical diagnosis of idiopathic Parkinson’s disease: a clinico-pathological study of 100 cases. J. Neurol. Neurosurg. Psychiatry 55, 181–184. doi: 10.1136/jnnp.55.3.181
Ionita-Laza, I., Capanu, M., De Rubeis, S., McCallum, K., and Buxbaum, J. D. (2014). Identification of rare causal variants in sequence-based studies: methods and applications to VPS13B, a gene involved in Cohen syndrome and autism. PLoS Genet. 10:e1004729. doi: 10.1371/journal.pgen.1004729
Islam, M. S., Azim, F., Saju, H., Zargaran, A., Shirzad, M., Kamal, M., et al. (2021). Pesticides and Parkinson’s disease: current and future perspective. J. Chem. Neuroanat. 115:101966. doi: 10.1016/j.jchemneu.2021.101966
Johansson, A. S., Stenberg, G., Widersten, M., and Mannervik, B. (1998). Structure-activity relationships and thermal stability of human glutathione transferase P1-1 governed by the H-site residue 105. J. Mol. Biol. 278, 687–698. doi: 10.1006/jmbi.1998.1708
Kalia, L. V., and Lang, A. E. (2015). Parkinson’s disease. Lancet 386, 896–912. doi: 10.1016/s0140-6736(14)61393-3
Kondo, I., and Yamamoto, M. (1998). Genetic polymorphism of paraoxonase 1 (PON1) and susceptibility to Parkinson’s disease. Brain Res. 806, 271–273. doi: 10.1016/s0006-8993(98)00586-1
Landi, S. (2000). Mammalian class theta GST and differential susceptibility to carcinogens: a review. Mutat. Res. 463, 247–283. doi: 10.1016/s1383-5742(00)00050-8
Langston, J. W., Irwin, I., Langston, E. B., and Forno, L. S. (1984). 1-Methyl-4-phenylpyridinium ion (MPP+): identification of a metabolite of MPTP, a toxin selective to the substantia nigra. Neurosci. Lett. 48, 87–92. doi: 10.1016/0304-3940(84)90293-3
Mazaheri, S., Hajilooi, M., and Rafiei, A. (2006). The G-308A promoter variant of the tumor necrosis factor-alpha gene is associated with migraine without aura. J. Neurol. 253, 1589–1593. doi: 10.1007/s00415-006-0270-4
Menegon, A., Board, P. G., Blackburn, A. C., Mellick, G. D., and Le Couteur, D. G. (1998). Parkinson’s disease, pesticides, and glutathione transferase polymorphisms. Lancet 352, 1344–1346. doi: 10.1016/s0140-6736(98)03453-9
Nalls, M. A., Pankratz, N., Lill, C. M., Do, C. B., Hernandez, D. G., Saad, M., et al. (2014). Large-scale meta-analysis of genome-wide association data identifies six new risk loci for Parkinson’s disease. Nat. Genet. 46, 989–993. doi: 10.1038/ng.3043
Noyce, A. J., Bestwick, J. P., Silveira-Moriyama, L., Hawkes, C. H., Giovannoni, G., Lees, A. J., et al. (2012). Meta-analysis of early nonmotor features and risk factors for Parkinson disease. Ann. Neurol. 72, 893–901. doi: 10.1002/ana.23687
Oliveira-Paula, G. H., Coeli-Lacchini, F. B., Ferezin, L. P., Ferreira, G. C., Pinheiro, L. C., Paula-Garcia, W. N., et al. (2021). Arginase II polymorphisms modify the hypotensive responses to propofol by affecting nitric oxide bioavailability. Eur. J. Clin. Pharmacol. 77, 869–877. doi: 10.1007/s00228-020-03059-9
Omiecinski, C. J., Vanden Heuvel, J. P., Perdew, G. H., and Peters, J. M. (2011). Xenobiotic metabolism, disposition, and regulation by receptors: from biochemical phenomenon to predictors of major toxicities. Toxicol. Sci. 120(Suppl. 1), S49–S75. doi: 10.1093/toxsci/kfq338
Palacios, N., Weisskopf, M., Simon, K., Gao, X., Schwarzschild, M., and Ascherio, A. (2010). Polymorphisms of caffeine metabolism and estrogen receptor genes and risk of Parkinson’s disease in men and women. Parkinsonism Relat. Disord. 16, 370–375. doi: 10.1016/j.parkreldis.2010.02.012
Perez-Pastene, C., Graumann, R., Diaz-Grez, F., Miranda, M., Venegas, P., Godoy, O. T., et al. (2007). Association of GST M1 null polymorphism with Parkinson’s disease in a Chilean population with a strong Amerindian genetic component. Neurosci. Lett. 418, 181–185. doi: 10.1016/j.neulet.2007.03.024
Pringsheim, T., Jette, N., Frolkis, A., and Steeves, T. D. (2014). The prevalence of Parkinson’s disease: a systematic review and meta-analysis. Mov. Disord. 29, 1583–1590. doi: 10.1002/mds.25945
Rejeb, J., Omezzine, A., Rebhi, L., Boumaiza, I., Mabrouk, H., Rhif, H., et al. (2013). Association of PON1 and PON2 polymorphisms with PON1 activity and significant coronary stenosis in a Tunisian population. Biochem. Genet. 51, 76–91. doi: 10.1007/s10528-012-9544-y
Sanchez-Santed, F., Colomina, M. T., and Herrero Hernandez, E. (2016). Organophosphate pesticide exposure and neurodegeneration. Cortex 74, 417–426. doi: 10.1016/j.cortex.2015.10.003
Satoh, T., Taylor, P., Bosron, W. F., Sanghani, S. P., Hosokawa, M., and La Du, B. N. (2002). Current progress on esterases: from molecular structure to function. Drug Metab. Dispos. 30, 488–493. doi: 10.1124/dmd.30.5.488
Scott, S. A., Sangkuhl, K., Shuldiner, A. R., Hulot, J. S., Thorn, C. F., Altman, R. B., et al. (2012). PharmGKB summary: very important pharmacogene information for cytochrome P450, family 2, subfamily C, polypeptide 19. Pharmacogenet. Genomics 22, 159–165. doi: 10.1097/FPC.0b013e32834d4962
Shah, P. P., Saurabh, K., Pant, M. C., Mathur, N., and Parmar, D. (2009). Evidence for increased cytochrome P450 1A1 expression in blood lymphocytes of lung cancer patients. Mutat. Res. 670, 74–78. doi: 10.1016/j.mrfmmm.2009.07.006
Shahabi, H. N., Westberg, L., Melke, J., Hakansson, A., Belin, A. C., Sydow, O., et al. (2009). Cytochrome P450 2E1 gene polymorphisms/haplotypes and Parkinson’s disease in a Swedish population. J. Neural Transm. 116, 567–573. doi: 10.1007/s00702-009-0221-1
Siokas, V., Aloizou, A. M., Tsouris, Z., Liampas, I., Liakos, P., Calina, D., et al. (2021). ADORA2A rs5760423 and CYP1A2 rs762551 Polymorphisms as Risk Factors for Parkinson’s Disease. J. Clin. Med. 10:381. doi: 10.3390/jcm10030381
Takakubo, F., Yamamoto, M., Ogawa, N., Yamashita, Y., Mizuno, Y., and Kondo, I. (1996). Genetic association between cytochrome P450IA1 gene and susceptibility to Parkinson’s disease. J. Neural Transm. 103, 843–849. doi: 10.1007/BF01273362
Tao, E., Liu, Z., and Pan, X. (1998). Glutathione S transferase μ polymorphism in Parkinson’s disease. Chin. J. Nerv. Ment. Dis. 24, 79–81.
Wahner, A. D., Glatt, C. E., Bronstein, J. M., and Ritz, B. (2007). Glutathione S-transferase mu, omega, pi, and theta class variants and smoking in Parkinson’s disease. Neurosci. Lett. 413, 274–278. doi: 10.1016/j.neulet.2006.11.053
Wang, J., and Liu, Z. (2000). No association between paraoxonase 1 (PON1) gene polymorphisms and susceptibility to Parkinson’s disease in a Chinese population. Mov. Disord. 15, 1265–1267. doi: 10.1002/1531-8257(200011)15:6<1265::aid-mds1034>3.0.co;2-0
Whitbread, A. K., Mellick, G. D., Silburn, P. A., Le Couteur, D. G., and Board, P. G. (2004). Glutathione transferase Omega class polymorphisms in Parkinson disease. Neurology 62, 1910–1911. doi: 10.1212/01.wnl.0000125282.09308.b1
Wirdefeldt, K., Adami, H. O., Cole, P., Trichopoulos, D., and Mandel, J. (2011). Epidemiology and etiology of Parkinson’s disease: a review of the evidence. Eur. J. Epidemiol. 26(Suppl. 1), S1–S58. doi: 10.1007/s10654-011-9581-6
Keywords: metabolizing enzymes, Parkinsion’s disease, polymorphism, synergistic effect, genetic association
Citation: Fan H-H, Li B-Q, Wu K-Y, Yan H-D, Gu M-J, Yao X-H, Dong H-J, Zhang X and Zhu J-H (2022) Polymorphisms of Cytochromes P450 and Glutathione S-Transferases Synergistically Modulate Risk for Parkinson’s Disease. Front. Aging Neurosci. 14:888942. doi: 10.3389/fnagi.2022.888942
Received: 03 March 2022; Accepted: 11 April 2022;
Published: 29 April 2022.
Edited by:
Mahendra P. Singh, Lovely Professional University, IndiaReviewed by:
Saurabh Srivastav, Rice University, United StatesVijay Kumar, Panjab University, India
Copyright © 2022 Fan, Li, Wu, Yan, Gu, Yao, Dong, Zhang and Zhu. This is an open-access article distributed under the terms of the Creative Commons Attribution License (CC BY). The use, distribution or reproduction in other forums is permitted, provided the original author(s) and the copyright owner(s) are credited and that the original publication in this journal is cited, in accordance with accepted academic practice. No use, distribution or reproduction is permitted which does not comply with these terms.
*Correspondence: Xiong Zhang, emhhbmd4aW9uZzk4QGdtYWlsLmNvbQ==; Jian-Hong Zhu, amh6aHVAd211LmVkdS5jbg==