- 1HABITAT, GIPPCL, Biofertilizer Production Unit, CCS Haryana Agricultural University, Hisar, India
- 2Department of Veterinary Physiology and Biochemistry, Sardar Vallabhbhai Patel University of Agriculture and Technology, Meerut, India
- 3Department of Zoology, Chaudhary Bansi Lal University, Bhiwani, India
- 4Department of Veterinary Physiology and Biochemistry, Lala Lajpat Rai University of Veterinary and Animal Sciences, Hisar, India
- 5Department of Veterinary Physiology and Biochemistry, RGSC, Banaras Hindu University, Varanasi, India
- 6Department of Chemistry and Biochemistry, CCS Haryana Agricultural University, Hisar, India
- 7Department of Animal Biotechnology, Lala Lajpat Rai University of Veterinary and Animal Sciences, Hisar, India
Colorectal cancer (CRC) is the third most frequently occurring tumor in the human population. CRCs are usually adenocarcinomatous and originate as a polyp on the inner wall of the colon or rectum which may become malignant in the due course of time. Although the therapeutic options of CRC are limited, the early diagnosis of CRC may play an important role in preventive and therapeutic interventions to decrease the mortality rate. The CRC-affected tissues exhibit several molecular markers that may be exploited as the novel strategy to develop newer approaches for the treatment of the disease. Nanotechnology consists of a wide array of innovative and astonishing nanomaterials with both diagnostics and therapeutic potential. Several nanomaterials and nano formulations such as Carbon nanotubes, Dendrimer, Liposomes, Silica Nanoparticles, Gold nanoparticles, Metal-organic frameworks, Core-shell polymeric nano-formulations, Nano-emulsion System, etc can be used to targeted anticancer drug delivery and diagnostic purposes in CRC. The light-sensitive photosensitizer drugs loaded gold and silica nanoparticles can be used to diagnose as well as the killing of CRC cells by the targeted delivery of anticancer drugs to cancer cells. This review is focused on the recent advancement of nanotechnology in the diagnosis and treatment of CRC.
Introduction
Colorectal cancer (CRC) is a severe health problem and has the third-highest occurrence within the cancer-causing conditions that influence the populations of developing and developed countries (da Paz et al., 2012). Among populations of western countries, a major cause of death and morbidity is CRC (Arvelo et al., 2015). Various routine factors and the growing age are the common reason of CRC with only a few cases being the outcome of genetic disorders (Bours et al., 2015; Gulbake et al., 2016). It generally starts in the bowel lining and can extend into the wall of the bowel and beneath muscle layers if not treated properly at the earliest (Datta et al., 2016). In addition to these, there are genetic and environmental factors that can interact in various ways to enhance carcinogenesis (Bours et al., 2015). Regarding the CRC pathogenesis four central theories have been established. In the first theory, epigenetic and genetic variation generate the formation of colon cancer promotes CRC. Second, cancer emerges due to a multistep process at both molecular and morphological levels. The third and crucial molecular step is the loss of genetic stability in cancer formation. Fourth, hereditary cancer diseases usually belong to germline forms of an important genetic disorder, for which somatic occurrences force the manifestation of sporadic colon cancers (Kheirelseid et al., 2013). However, various factors that contribute to and affect the start and establishment of disease, continue to increase the number of potential targets (Hutchinson et al., 2015). Ultimately, this targeting potential will show the way for the success of an effective technique for disease management and will provide better treatments to the patients. Several decades of research have been concentrating on the establishment of novel methods and techniques in cancer research, especially in the areas of detection, giving rise to earlier cancer therapy to decrease mortality.
CRC is the most devastating disease identified among the most common malignancies and can be recorded as the second leading reason for cancer-associated deaths in women and the third-highest common disease in men worldwide (Huyghe et al., 2020). Current situations have been shown that up to 2030, the chances and mortality rate of CRC will enhance by 60% worldwide (Brand et al., 2017; Huyghe et al., 2020). With the existing diagnostic techniques and treatments, patients suffer long invasive techniques with unwanted side effects to remove CRC. Hence, a new detection method requires further research to improve their specificity and affectivity (Bray et al., 2019).
Nanotechnology has opened up a new way to the development of novel and efficiently organized nanomaterials with the capability of enhanced performance in screening, detection, and treatment of CRC cancer along with other types of tumors (Gulbake et al., 2016; Bray et al., 2019). The role of nanotechnology interventions to CRC includes the nanomaterial-based screening of tumors, customization of targeted drug delivery systems, and advanced treatment modalities (Upendra et al., 2016; Minakshi et al., 2017; Minakshi et al., 2018; Minakshi et al., 2020). Currently, nanotechnologies have found worldwide consideration due to their capability to improve existing standards and methods for the screening, diagnosis, and treatment of CRC. The present review has the main emphasis on the discussion of nanotechnology-based precision methods for screening, detection, and treatment of colorectal cancer.
During the preparation of this review, recent and appropriate information was collected using several scientific search engines including PubMed, Medline, Google Scholar. Several research and review articles were collected, thoroughly studied and a comprehensive manuscript is prepared. Under this review article several aspects of colorectal cancer (CRC) including their different clinical stages, recent diagnostic and therapeutic approaches using several nano-formulations such as Quantum dots, Iron oxide, Carbon nanotubes, Liposomes, Silica Nanoparticles, Nanoemulsion, Gold nanoparticles, etc. have been discussed. Apart from the use of individual medicines, the efficacy of combinatorial nanomedicine against CRC has also been explored.
Colorectal Cancer Stages
The survival rate in CRC mainly depends upon the stages of CRC disease and it usually ranges from 90% in the case of localized stage to 10% in the case of patients diagnosed with metastatic cancer. If the stage of diagnosis is earlier, the higher the chance of survival rate (Haggar and Boushey, 2009). The exact stage of the tumor, which explains the level of cancer in a patient’s body, is one of the very significant factors in deciding which therapy is useful and how successful therapy might be (Schroy et al., 2016). Figure 1 describes the various stages of colorectal cancer. Abnormal cells arise from colonic wall mucosa, may become tumors, and divides in stage 0. During stage I, cancer has formed in the colon wall mucosa and emerges into submucosa as well as muscularis propria. Stage II cancer further progresses from muscularis propria into pericolorectal tissues (IIA), and then emerges to the visceral peritoneum (IIB), then directly penetrates into the attached organs (IIC). Stage III cancer emerges into muscularis propria metastases in nearby tissues or 1–3 regional lymph nodes and spreads in submucosa with metastases in 4–6 lymph nodes in IIIB and 7 or more regional lymph nodes (IIIC). Metastasis confines to one organ such as liver ovary, lung, regional node, etc. in stage IV, and again stage IV cancer is further divided into IV A and IV B stages (Xynos et al., 2013; Lai et al., 2016). Five years survival rate percent researches showed 90% survival in the case of stage 1 CRC and 10% survival in the case of stage IV CRC (Pesta et al., 2016). Physical inactivity, eating more processed meat, long-term smoking, obesity, alcohol addiction, and a diet with fewer vegetables and fruits are the most common lifestyle-related threats and major causes of CRC. Family history is also connected with CRC, a person with chronic inflammatory bowel disease, type 2 diabetes, or genetic disease likelynch syndrome has been linked with augmented risk (Young et al., 2014). Stages 0, I, II, and III may be curable but stage IV CRC is not often curable but it may be managed based on growth and spreading disease (Young et al., 2014; Sun et al., 2016).
Existing Screening Methods for Colorectal Cancer
Various screening methods have been established to screen CRC before symptoms start, when it may be easily treatable. Few of the screening methods that identify polyps and adenomas at an early stage may assist in the detection and early removal of tumor growth which might otherwise lead to further progression of cancers. Hence early detection of colorectal cancer may be another method of cancer prevention. Different tests are prevalent for suitable screening of colorectal cancer (Table 1). Stool tests detect minute quantities of blood that cannot be possibly seen visually from both adenomas and polyp cancers (Imperiale et al., 2004; Burch et al., 2007; Ansa et al., 2018; Qaseem et al., 2019).
In the colonoscopy entire colon and rectum are screened for cancer using a colonoscope, having a flexible tube light with a lens for seeing and a tool for excising the abnormal tissue (Kahi et al., 2016). A complex analysis of six studies showed that screening using colonoscopy reduces the chances of establishment and dying of people from CRC (Brenner et al., 2014). Conversely, the test is costly and unpleasant. Virtual colonoscopy or computed tomographic (CT) colonography and double-contrast barium enema are the optional choices for patients, who cannot go through a routine colonography due to the anesthesia risk, which prevents a complete examination. In both the methods, x-rays are used to observe the colon from outside the body (Pickhardt, 2013; Kuipers and Spaander, 2015). The occurrence of colorectal neoplasm was detected using double-contrast barium enema (DBEM) screening in people of Thailand and reported a diagnostic outcome of 0.4% for CRC and 0.7% for advanced adenoma (Lohsiriwat et al., 2013). Insigmoidoscopy test for checking cancer, polyps, and other abnormalities using a flexible tube having light at the end is inserted into the lower colon and rectum (Guo et al., 2015). Many of the clinical trials reported that sigmoidoscopy reduces the chances of establishment and prevents persons from dying from CRC (Atkin et al., 2010; Segnanet al., 2011; Elmunzer et al., 2012; Schoen et al., 2012; Holme Kalager, 2014). All these screening tests for CRC have differed in respect to their preparation requirements, patient expediency, amount of colon and rectum evaluated along with their limitations.
Along with these general tests, the CellSerch® assay (Janssen Diagnostics, LLC, South Raritan, NJ, United States) which is based on circulating tumor cell (CTC) diagnostic technology is used for the diagnosis of meta-static colorectal cancer along with other cancers such as breast and prostate cancers. US FDA cleared circulating tumor cell (CTC) diagnostic technology for metastatic CRC along with other cancers such as prostate and breast cancers (Allen et al., 2014). It gives prediction-related information in metastatic CRC, regardless of the metastatic site. Currently, various new systems such as MagSweeper, Cynvenio, IsoFlux, VerIFASt, AdnaGen, and magnetic sifters have been established to further enhance the identification speed and efficiency (Mostert et al., 2015). Most of the current screening methods are very expensive and not easy at the point of care. Hence, the development of more sensitive, fast, low cost, and specific screening of CRC is very essential.
Recent Advancements of Nanotechnology in Cancer Theranostics
Recent development in nano-sciences has allowed fabrication of several nanoparticles (NPs)-based systems for therapeutics and diagnostics. Although the clinical applications of nano-based theranostics are still limited probably due to their complex pharmacokinetics, NPs can improve the knowledge of biochemical and physiological principles of several diseases and their treatments (Siddique and Chow, 2020). NPs have been used in the enhancement of capability of several imaging techniques such as positron emission tomography (PET) by use of radioisotope chelator-free NPs, and iron oxide-based NPs in magnetic resonance imaging (MRI) (Rosado-de-Castro et al., 2018). Similarly, in the optical imaging system, persistent luminescence nanoparticles (PLNPs) have been used as a novel optical nanoprobe to utilize the characteristic long-lasting near-infrared (NIR) luminescence (Lecuyer et al., 2016) which allows the functioning of optical imaging without constant excitation and autofluorescence (Liu et al., 2019). The NPs act as excellent contrast agents because of their small size, high sensitivity, and specific chemical composition. Similarly, NPs are also widely used for the therapeutic purpose of cancer. In therapeutic use, it improves the accumulation and release of pharmacologically active compounds at the pathological site leading to enhanced therapeutic efficacy and reduced toxic side effects. Moreover, recently developed NPs have the capability for integrating therapeutic and diagnostic agents into a single NP that can be easily used for theranostic purposes (Siddique and Chow, 2020). Studies have also revealed that theranostic NPs may have the capability for future use as personalized nanomedicine-based therapies (Baetke et al., 2015). In the coming future, multifunctional NPs can be designed by using various functional materials leading to the development of simultaneous diagnosis and therapeutics known as theranostics (Kim et al., 2017).
Existing Treatment Methods for Colorectal Cancer
At present, various treatment methods with their merits and demerits are available (Table 2). The most general method for the treatment of CRC is surgery and most commonly known as surgical resection. Surgery is the most common treatment for CRC and is often called surgical resection. It is a significant first line of the protective system against any particularly well-defined tumor or any cancer (Lee, 2009; Chu, 2012). During surgical treatment in CRC, a portion of the healthy rectum or colon will also be excised. In addition to the surgical resection, other surgical options for CRC comprise colostomy for rectal cancer, radiofrequency ablation, laparoscopic surgery, etc. Radiation therapy is most widely used for the treatment of rectal cancer for the reason that this cancer tends to continue to the site where it grows initially. Radiation therapy is given to the patient in a scheduled manner comprising a defined number of treatments at defined time intervals (Joye and Haustermans, 2014). Stomach upset, mild skin infections, fatigue, etc. are the main side effects of radiation therapy. In the chemotherapy treatment method, drugs are used to deactivate cancer cells, generally by stopping the activity of tumor cells to grow and multiply. At present various approved drugs are used for the treatment of CRC (Jayakumar et al., 2015). Vomiting, nausea, neuropathy, mouth sores, and diarrhea are the main causes of chemotherapy. Treatment that targets the defined genes of tumor cells or defined tissue environment is known as targeted therapy. Such type of treatment inhibits the growth and division of the cancerous cells although limiting the harm to normal cells. To find out an efficient and reliable treatment of CRC, new methods should be established for effective detection of proteins, genes, and additional factors in a patient’s cancer. For CRC treatment epidermal growth factor receptor (EGFR) inhibitor therapy, anti-angiogenesis therapy is an option. The most common side effects of the targeted therapy include rashes on upper body parts and the face.
During the treatment of CRC, poor drug response to chemotherapy is frequently reported which might be primarily due to the appearance of the multidrugresistance (MDR) in tumor cells (Wu et al., 2018). Several studies have shown the mechanism of drug resistance in colorectal cancer cells. Some of the studies revealed that the effect of cetuximab and panitumumab can be inactivated by the mutations in PIK3CA or BRAF gene, or due to gene amplification of MET, KRAS, and ERBB2 resulting in the prevention of EGFR signaling (Dienstmann et al., 2015). The overexpression of dihydropyrimidine dehydrogenase (DPD) gene may lead to resistance against 5-FU, because DPD may convert the 5-FU into the inactive metabolite (DeNardo and DeNardo, 2012). In addition, decreased expression of RFC-1 (reduced folate transporter 1) and PCFT (proton-coupled folate transporter) may block the LV (Odin et al., 2015). Similarly, altered levels of metallothioneins and glutathione reductase (GR) may affect the response of oxaliplatin (OXA). Moreover, the increased activity of UDP-glucuronosyltransferases (UGT) or induced DNA damage repair mechanism such as TDP1 (tyrosyl-DNA phosphodiesterase 1) may limit the effect of IRI (Jensen et al., 2015). To overcome the multidrug resistance, nanomedicine appears as a recent strategy to enhance the prognosis in CRC patients.
Applications of Nanotechnology in Screening, Detection, and Treatment of Colorectal Cancer
More than four decades ago application of nanotechnology foundations is laid down for their diagnostic and therapeutic uses in a very précised manner (Fortina et al., 2007). Nanotechnology is a most important branch of research which plays a very important role in screening, detection, and treatment of day-by-day increasing diseases (Upendra et al., 2016). It plays a major role in the discovery and delivery of drugs with specific action at a specific target site with an enhanced success rate. The development of these nano-formulations includes liposomes, quantum dots, silica NPs, gold NPs, liposomes, dendrimers, nano-emulsions as a coating material in imaging at pathological sites, and nano-drug delivery (Bose et al., 2015; Yallapu et al., 2015).
Various important nanotechnological applications have been verified in cancer biology, comprising early screening and detection of cancers and establishment of new treatment approaches that cannot be gained using the existing conventional methods (Laroui et al., 2013). In de facto, in certain tumors, nano-sized particles of different shapes and compositions have emerged out as important and promising novel tools for colorectal cancer staging, diagnosis, and therapeutics (Figure 2) (Dong et al., 2016). Early screening and detection of CRC is the main solution for impediments and it can impact the long-lasting survival of CRC patients. Early detection of CRC is the key, and it can impact the long-term survival of patients with CRC. In this review, we discuss the current achievements of NPs that provide a new way for early screening and flourishing treatment of CRC.
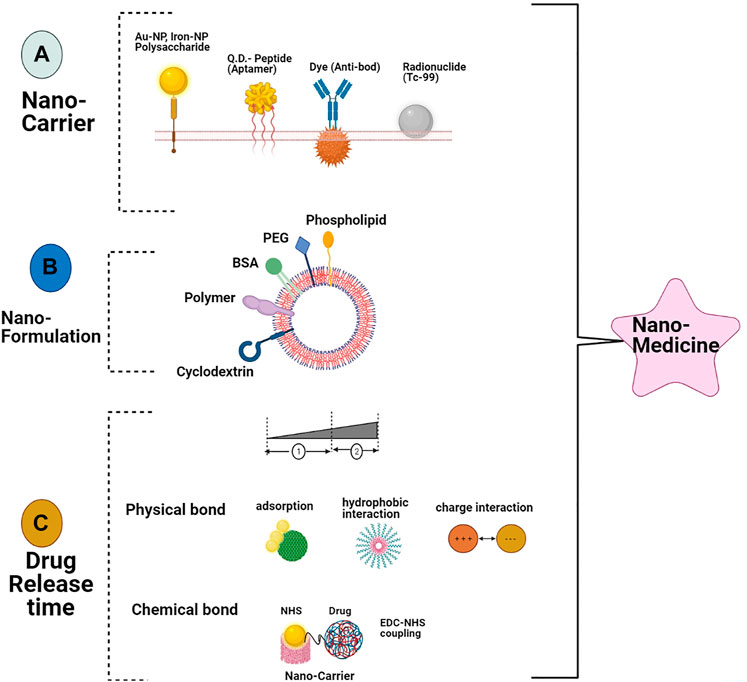
FIGURE 2. Schematic explanation role of nanotechnology in CRC. (A) NPs routes for administration, (B) various kinds of polymer NPs and metallic along with necessary parameters for use in CRC therapy cancer therapy (C) screening and detecting the tumor respectability using multimodal imaging and involvement of increased permeability and retention effect on antitumor drug synthesis for CRC targeting therapy.
Quantum Dots
Quantum dots (QDs) are semiconductor nanocrystals which fluoresce with light excitation and have special optical features, comprising high brightness, the ability to emit fluorescence at varying wavelengths, and resistance to photo-bleaching. Owing to QDs chemical and optical advantages, QD-based nanotechnology is acting as a promising platform for studying various tumors including the CRC (Fang et al., 2012; Pericleou et al., 2012; Zeng et al., 2015). QD-based immune histochemistry (QD-IHC) has been used for qualitatively analyzing the expression of large external antigens from CRC tissue samples and compared with conventional-IHC (Wang et al., 2016), QD-IHC offered various detectable advantages for quantification of protein marker. These showed simpler operation, higher sensitivity, less human interference, and better capability for concurrent multifactor analysis; would produce more accurate clinical detections. Therefore, QD-IHC is a better option for conventional IHC in therapeutic applications. QD-based immune cytochemistry using QD-probes studies mainly focuses on marker identification in tissue sections, IHC combined with imaging quantitative analysis for screening of large external antigen in living cells showed results that were the same as obtained using flow cytometry and expending the applications of QD-probes for clinical applications (Wang et al., 2016). In another study, a sensitive method was developed to identify Aldo-keto reductase family 1 member B10 (AKR1B10) in the serum that acts as a therapeutic target and prognostic predictor for CRC using QDs. The QDs possess stability against photo bleaching along with size-controlled luminescence activity that makes QDs a suitable agent for photoelectrochemical-based tumor marker detection from biological samples. However, QDs are still not used for the detection of AKR1B10 from serum samples (Wang et al., 2015; Liu et al., 2021). This technique played a great role in the early detection of CRC with high specificity and sensitivity.
A highly sensitive and specific technology was developed, which enables concurrent detection of biochemical fluorescence and morphological changes during CRC progression and development using optical coherence tomography or laser-induced tomography that allows nondestructive internal visualization of CRC (Carbary-Ganz et al., 2015). A probe QDot655 specific to vascular endothelial growth factor receptor 2 (QD655-GEGFR2) restricted to the colon used in carcinogen-treated mice and provides significantly high contrast between infected and healthy tissues with specificity and sensitivity ex-vivo (Carbary-Ganz et al., 2015). These probes act for in vivo magnetic resonance imaging (MRI) and further biopsy of CRC, an exceptional cell-specific, paramagnetic double-signal fluorescent molecular multipurpose nanoprobe (GdDTPA-BSA@QDs-PCAb) was designed using surface engineering of QDs with DTPA-BSA-Gd3+ large molecular complex by ultrasonic conditions. These nanoprobes act as promising tools for use in contrast-enhanced MRI diagnosis of CRC and have no cytotoxic effects in MTT assay (Xing et al., 2015). QDs-bevacizumab nanoprobes have been successfully used for targeted CRC detection in both in-vitro and in-vivo systems. During detection, they enhanced the tumor-specific signal after subsequent injection of the QDs and act as a significant achievement in VEGF-directed noninvasive imaging systems during clinical practices (Gazouli et al., 2014).
Under defined conditions subtractive Cell-SELEX technology is used for the production of a panel of seven aptamers (Apts) that precisely attach metastatic CRC cells (LoVo) along with other metastatic tumor cells with great affinity, and hence provides a broad spectrum specific detection of metastatic tumor cells (Li et al., 2014b). This research showed that Apts selected from a single Cell-SELEX can alone act as functionalized for several purposes based on target biochemical properties, thereby increasing the applications of the Cell-SEARCH approach. This receptor-specific Apt W14 was used as the targeted carrier for doxorubicin-specific delivery to defined cells to reduce the toxicity of this drug (Li et al., 2014b).
QDs conjugated with non-membrane receptor binding Apt W3 were used as a molecular probe for specified imaging of metastatic tumor-bearing sections, metastatic tumor cell lines, and formalin-fixed paraffin-embedded tissues from the CRC persons (Li et al., 2014). Hence QDs are great technological modalities in progress that can reform the CRC treatment and diagnosis.
Iron Oxidenano-Formulations
Iron oxide NPs have the two-fold capability to work as both photo-thermal and magnetic agents for human applications as MRI agents. They also have brilliant biodegradability in vivo and after dissolution, iron ions can be adjusted by the body by a highly regulated physiological phenomenon (Espinosa et al., 2016). A smart multi functional magnetic nano vehicles encapsulating an antibody targeting peptide AP1 (MPVA AP1), which are consistent in size and also water soluble, anti-cancerous drug has been developed (Kuo and Liu, 2016). These nano-vehicles are easily dispersed in aqueous solutions and exhibit no cytotoxic effects in L929 fibroblasts, and showed their capability for therapeutic applications (Kuo and Liu, 2016). A CRC cell (CT26-IL4R) trial discovered that the MPVA-AP1 showed outstanding selectivity and targeting. A steady storage test revealed no leakage of encapsulated drugs without the stimulus of the magnetic field. In disparity, nano-vehicles loaded with doxorubicin burst upon treatment of high frequency of magnetic field, which is fast, accurate, and controlled release. Furthermore, in vivo investigations recognized that magnetic nano-vehicles exhibited obvious chemotherapeutic and thermotherapeutic effects. Hence, smart magnetic nano-vehicles e.g. MPVA-AP1 have the noteworthy capability for specified doses and precise controlled release in antitumor applications (Kuo and Liu, 2016).
The usefulness of polylactide-co-glycolic acid (PLGA) nanoparticle as a5-fluorouracil (5-FU) vehicles with or without an iron oxide and hyperthermia at DNA damage point in an HT-29 colon tumor cell line spheroid culture model by alkaline comet assay (Esmaelbeygi et al., 2015) and found that less DNA damage in case 5-Fu loaded nanoparticles as compared with hyperthermia. Hence hyperthermia is a damaging agent and NPs are an efficient drug delivery system to CRC. The iron oxide NPs enhanced the effect of hyperthermia and could be extremely beneficial in the diagnosis of CRC (Esmaelbeygi et al., 2015).
The paclitaxel (PTX), as well as super-paramagnetic iron oxide (SPIO), is encapsulated inside the core of PEAL Ca micelles and studied for significant tumor therapy (Feng et al., 2014). The drug release in this research showed that PTX in the micelles was released with a lower rate at neutral pH and a faster rate at pH 5.0. Cell culture studies also showed that PTX-SPIO-PEALCa was successfully absorbed by the CRCLoVo cells and PTX was likely internalized by lysosomal cells. Additionally, successful inhibition of CRC LoVo cell growth was verified. Hence, micelles play a great role in MRI visible drug release methods for CRC treatment (Feng et al., 2014).
The lectin-Fe2O3 @AuNPs are synthesized by joining lectins on the Fe2O3@AuNPs via bifunctional polyethylene glycol (PEG) NHS ester disulfide (NHS-PEG-S-S-PEG-NHS) linkers. Both in vitro and in vivo studies are done for checking the activity of lectins- Fe2O3@AuNPs and found that it could be useful for dual-mode MRI and X-ray CT imaging of CRC. Hence results obtained proposed that lectins could be applicable as cancer-targeting ligands in nano-formulations based on contrast agents (He et al., 2014).
Poly Lactic-co-Glycolic Acid Nanoparticles
Eco-friendly Poly lactic-co-glycolic acid (PLGA) NPs have been used as carriers for proteins, peptides, vaccines, drugs, and nucleotides. These can help in shielding drug moieties from breakdown and subsequently ensure the effective release of drugs (Sah et al., 2013) and are extensively studied for their potential use in tumor therapy, specifically for CRC. Using the emulsion-solvent evaporation method curcumin-loaded PGLA NPs (C-PNPs) are successfully synthesized for colon delivery (Akl et al., 2016). C-PNPs exhibit significantly higher cellular uptake in HT-29 cells in comparison with pure curcumin solution due to their sustained release, greater colloidal stability in gastrointestinal fluids, and smaller size. Therefore, C-PNPs have great potential as an early platform for the further establishment of a sufficient oral targeted drug system to the colon, mainly if it is additionally functionalized with a specific targeting ligand (Akl et al., 2016).
Scientists also prepared chitosan polymeric NPS using the solvent extraction emulsification technique, with different ratios of polymer, and showed potential application in the successful delivery of active pharmaceutical components to the CRCs (Tummala et al., 2015). Total health center complex N-38 is an effective treatment against many cancers but the delivery system is not easy due to its low solubility. SN-38encapsulatedin poly(D,L-lactide-co-glycolide)NPs were prepared by the spontaneous emulsification solvent diffusion method to improve its solubility, stability, and cellular uptake (Essa et al., 2015). To study cellular uptake and cytotoxicity of SN-38 encapsulated NPs, the colorectal adenocarcinoma cell line-205 (COLO-205) was used and found considerably reduced cell proliferation and death. Hence, SN-38 loaded NPs are potentially essential drug delivery systems for the treatment of CRC (Essa et al., 2015).
Carbon Nanotubes
Carbon nanotubes (CNTs), allotropes of carbon cylindrical in shape, have rolled graphene sheets with a diameter of fewer than 1 μm and a few nanometers in length (Rastogi et al., 2014). Owing to CNT chemical and physical properties such as high surface area, needle-like structure, heat conductivity, and chemical stability enable its potential uses in several fields such as immunotherapy, diagnosis, gene therapy, and a carrier in the drug delivery system (Hampel et al., 2008).
Various researches showed that several strategies have been developed in which CNTs have been used for carrying antitumor drugs. For the delivery of paclitaxel in Caco-2 cells single-walled carbon nanotubes conjugated with a synthetic polyampholyte were used. Paclitaxel-SWCNT-treated Caco-2 and HT-29 cells showed greater anticancer effects when compared with alone paclitaxel (Lee and Geckeler, 2012; Wu et al., 2015). The same types of results have been found in Eudragit®-irinotecan loaded CNTs (Zhou et al., 2014). Oxaliplatin and mitomycin C-coated CNTs, induced by infrared light rays in colon cell lines, found considerably higher drug delivery and localization in cancer using thermal treatment of cell membrane (Levi-Polyachenko et al., 2009). One study revealed that SWCNTs modified with TRAIL (a ligand that binds to specific receptors and causes apoptosis process in cancer) increased the cell death 10 times in comparison with alone delivery of TRAIL in carcinoma cell lines (Zakaria et al., 2015).
Dendrimer
They are three-dimensional macromolecular structures. They possess central core molecules surrounded by consecutive layers. The dendrimer exhibit a high degree of molecular consistency along with shaping characteristics, fine molecular weight distribution, and multivalency. The specific physicochemical properties along with biodegradable backbones facilitate several applications of dendrimer in nanopharmaceuticals development (Abbasi et al., 2014; Huang et al., 2015a; Wu et al., 2015). Some of the studies revealed that dual antibody conjugates can provide advantages of capturing circulating tumor cells (CTCs) in contrast to single-antibody counterparts (Xie et al., 2015). The study also revealed that the surface-active dendrimers can be successively shielded with two antibodies against the human colorectal CTCs surface biomarkers Slex and EpCAM. Dual antibody-coated dendrimers exhibit improved specificity in the detection of CTCs in both patient blood and nudemicein comparison to single antibody-coated dendrimers. Moreover, dual antibody-coated conjugates may downregulate the captured CTCs. Thus, theoretically, it can be assumed that biocompatible two antibodies conjugated to a nanomaterial may have the capability to capture and downregulate the CTCs that can be used as a new strategy for the prevention of metastasis (Xie et al., 2015).
Similarly in another study, HT29 cells (colon cancer cells) were successfully captured by employing Sialyl Lewis X antibodies (aSlex)-conjugated Poly(amidoamine) dendrimers (Xie et al., 2015b). The colon cancer cells were characterized using aSlex-coated dendrimer conjugate and examined by flow cytometry and microscopy. The study revealed that conjugate possessed an enhanced capacity to capture HT29 cells in a concentration-dependent manner. The aSlex-coated dendrimer conjugate showed optimum potential in capturing and detaining CTCs in the blood (Xie et al., 2015b). The maximum capture competence was obtained within 1 h of exposure.
Apart from the diagnostic value, dendrimer may also be used for therapeutic purposes. In one of the studies, telodendrimers were suspended with linear PEG-blocking dendritic oligomer of vitamin E and cholic acid, designed for the delivery of gambogic acid (GA) and other natural anticancer compounds were used (Huang et al., 2015a). The study revealed that high GA-loading ability and subsequent drug release were obtained with these optimized telodendrimers. Moreover, these novel nano-formulations of GA were found to exhibit similar in vitro cytotoxic activity as the free drug against the colon cancer cells (Huang et al., 2015a).
Later, a new platform for drug delivery was developed with the reengineering of nano-scale dendrimers for the capture of CTCs in the blood (Xie et al., 2014). These nano-scale dendrimers were lodged with dual antibodies to aim the two surface biomarkers specific to colorectal CTCs with the capacity to particularly recognize and bind CTCs and downregulate the activity of CTCs by arresting cell division in the S phase. Moreover, dual-antibody conjugates revealed enhanced specificity and competence in controlling the CTCs in vitro as well as in vivo in comparison with their single-antibody counterparts. Thus, these studies revealed an innovative way of effective prevention of metastatic initiation by binding and restraining CTCs that were usually achieved by the traditional cytotoxic killing of cancer cells (Xie et al., 2014).
Liposomes
Chemically, liposomes are lipid-based vesicles that act as artificial carriers with a small and spherical aqueous core that are nontoxic (Silva et al., 2011). Owing to their smaller size, ability to incorporate various substances, and phospholipid bilayer in nature, they are considered the most effective drug delivery systems into cells with decreased side effects (Suntres, 2011; Patil and Jadhav, 2014). In 1961, liposome was described as the first nanoparticle platform for drug delivery in clinical medicine (Bangham et al., 1965). It is one of the most used for drug delivery systems especially for peptides, nucleic acids, and proteins as nano-liposomes (Abreu et al., 2011).
Liposomes can be divided into three categories viz., long-circulating liposomes, active targeting liposomes, and liposomes with special properties that include thermo-sensitive, pH-sensitive, magnetic, and positive (Akbarzadeh et al., 2013; Nag and Awasthi, 2013; Noble et al., 2014). For clinical practice, liposome formulation carrying the drug such as daunorubicin (DaunoXome®) and Doxorubicin (Doxil®) has been approved by FDA in the mid-1990s (Barenholz, 2012). The study revealed that Doxil is approximately 100 nm in size and has much less gastrointestinal and cardiac toxicity (Rivera, 2003). Later, FDA has approved Marqibo® as a liposomal drug that is a cell cycle-dependent anticancer drug (Lammers et al., 2008; Lam and Ho, 2009; Allen and Cullis, 2013).
Drug resistance is a common problem in anticancer therapy. Several efforts have been made to fight drug resistance obtained when administrating liposome-based drugs such as Doxorubicin. In one of the studies during the administration of high dosages of an antitumor drug such as Doxorubicin (DOX) an aptamer-based drug delivery system assists in delivering high dosages of the active drug toward the target cancer cells (Li et al., 2014). Similarly, Thermodox® (thermo-sensitive liposome Doxorubicin) is used for colorectal liver metastases treatment in combination with radiofrequency ablation (Figure 3). In this treatment procedure, liposomal Doxorubicin formulation releases the active drug in response to the mild hyperthermic signal (Stang et al., 2012). The study revealed that Thermodox can effectively deliver twenty-five-fold more Doxorubicin to tumor cells than ordinary intravenous. Doxorubicin dose is five fold more effective than to standard liposomal formulations in animal models.
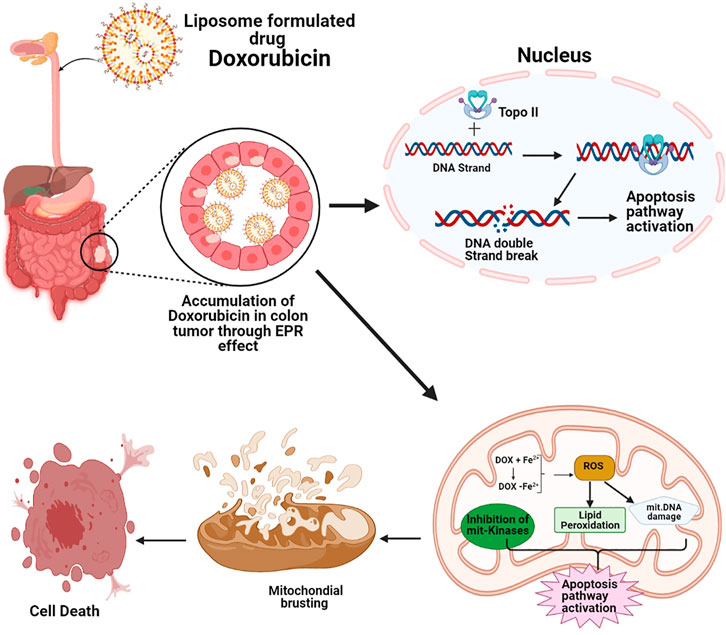
FIGURE 3. Schematic representation of Doxorubicin mechanism for colorectal cancer treatment, Doxorubicin accumulation in colon tumor, Doxorubicin molecular mechanism in the nucleus increase of topoisomerase II and induced by Doxorubicin, causes more DNA breaking which consequently gives rise to apoptosis but in mitochondria molecular mechanism of Doxorubicin, Fe2+-conjugated Doxorubicin causes Reactive oxygen species production that induces apoptosis and Doxil inhibits the mitochondrial kinases, consequential in apoptosis induction.
Silica Nanoparticles
Silica materials are broadly categorized as xerogels and mesoporous silica nanoparticles (MSN). Silica nanoparticles possess numerous advantages such as a highly porous framework, biocompatibility, and easy functionalization (Amato, 2010; Wei et al., 2010). MSNs consist of a bee's-hive-like porous structure that assists in packing large amounts of bioactive molecules. Moreover, MSN also possesses important features such as adjustable size of cavities in the range of 50–300 and 2–6 nm respectively (Stang et al., 2012), very low level of toxicity, easy endocytosis, the ability of large quantity of medicine loading along with resistance to heat and pH (Bharti et al., 2015). For more precise drug delivery and action to cancer cells, amesoporous silica nanoparticle-protamine hybrid system (MSN-PRM) was also used which can selectively release the drugs in the proximity of cancer cells and get activated with specific enzymes to trigger the anticancer activity (Radhakrishnan et al., 2013). In another study conjugated the hyaluronic acid to MSNs was done as HA-MSN and it was proved that the amount of DOX loading into HA-MSNs increases significantly than bare MSNs (Yu et al., 2013). Moreover, the cellular uptake of DOX-HA-MSNs conjugate was also increased which was reflected by the enhanced cytotoxicity to the human colon carcinoma cell line (HCT-116 cell line) (Gidding et al., 1999). Similarly, in another study, MSNs were functionalized with polyethyleneimine-polyethylene glycol (PEI-PEG) and polyethylene glycol (PEG) which was resulted in an increased amount of loading of Epirubicin hydrochloride (EPI) and exhibited improved antitumor activity (Hanafi-Bojd et al., 2015). Silica nanoparticles can also be used along with photosensitizer chemicals to kill the CRC cells. Researchers at the University of Buffalo and Roswell Park Cancer Institute have developed a silica-based nano shell that encases photosensitizer molecules. The nano shells are designed in such a way that they are easily taken up by the tumor cells and, upon exposure of cells to light the photosensitizers are activated and release the reactive oxygen molecules to kill the cancer cells (Advances in Colorectal Cancer Research, 2016). Currently, this technique is under clinical trials. Moreover, the second-generation photosensitizer-loaded nano shell is also in the developing phase where tumor-targeting and imaging agents will be incorporated to deliver at the tumor-specific sites that will assist in image-guided therapy for cancer (Advances in Colorectal Cancer Research, 2016). Thus, nanoparticles may also be allowed for molecular imaging of cancer cells followed by earlier diagnosis and targeted drug delivery.
Nano-Emulsion System
It is a transparent solution consisting of oil, water, and surfactant. It possessed thermodynamically uniform and stable physical properties. Nano-emulsions possess specific features such as facilitation of transferring drugs and drug combinations protection against external factors such as heat and pH (Bilensoy et al., 2009), lower toxicity, higher stability, and better efficiency along with the dissolution of nonpolar compounds (Patil and Jadhav, 2014). In one of the studies, the synergistic effect of gold nanoparticles (AuNPs) and lycopene (LP) on the HT-29 colon cancer cell line was studied. The experiment was designed in such a way that the first case consisted of a system of nano-emulsion having Tween 80 as emulsifiers along with LP and AuNPs and the second system consisted of a mixture of AuNPs and LP without any emulsion. The results revealed that the nano-emulsion system consists of dosages of LP and AuNPs as 250 and 125 times respectively lesser in comparison with their mixture mode, but the apoptosis induced by nano-emulsion was found three times higher than the mixture model (Chen et al., 2015; Huang et al., 2015b). Similarly, in another study, an ion-pairing complex of Oxaliplatin (OXA) with a deoxycholic acid derivative (Nα-deoxycholyl-l-lysyl-methylester, DCK) (OXA/DCK) was prepared for oral delivery of OXA and 5-fluorouracil (5-FU) to the colorectal cancer patient. The study revealed that in CT26 tumor-bearing mice, the tumor was inhibited by 73.9, 48.5, and 38.1% in comparison with tumor volumes in the control group and the oral OXA and 5-FU groups, respectively, which indicated the application of OXA/DCK and 5-FU as an oral combination therapy for CRC (Pangeni et al., 2016).
Core-Shell Polymeric Nano-Formulations
Nowadays there is an increasing interest in the manufacturing of core/shell nanoparticles with two or more materials (Zhou et al., 1994; Kumar et al., 2020). Such nanomaterials possess a core-shell structure where the outer surface atoms differ from those of the interior core atoms. The surface chemistry of core-shell type nanomaterials is usually characterized by multiple techniques such as secondary ion mass spectroscopy and X-rayphotoelectron spectroscopy (Simonet and Valcárcel, 2009; Zielińska et al., 2020). In core/shell nanoparticles combinations of different materials such as organic/organic, organic/inorganic, inorganic/inorganic can be used (Ghosh and Paria, 2012) with specific purposes including increasing the stability, functionality, and dispersibility of the core particles. Moreover, specifically designed core-shell particles may also provide a controlled release of the core and therefore, allow the reduced consumption of precious materials (Kalele et al., 2006). The core/shell particles possess specific applications in the biomedical field such as bio-imaging for cell labeling, controlled drug delivery, and tissue engineering practices (Bai et al., 2006; Sounderya and Zhang, 2008; Stanciu et al., 2009). Some other types of nanomaterial such as Lactobacillus reuteri biofilm coated with zinc gallogermanate (ZGGO) mesoporous silica may also be used as a bacterial inspired bio-nanoparticle system (ZGGO@SiO2@LRM). This nano system was found possessed with the unique property of targeted delivery of 5-FU to the tumor of colorectum area. Further study revealed that in comparison with 5-FU alone, the ZGGO@SiO2@LRM nanosystem decreased the tumor number per mouse to one-half during in-vivo chemotherapy. Additionally, this system was found capable of tolerating the digestion of gastric acid and thus may support the targeted drug delivery of oral medicines into the colorectum region. Moreover, ZGGO also assists in the hassle-free photo luminescence (PL) bioimaging where LRM coating accurately targets the tumor of the colorectum region (Wang et al., 2019). Similarly, other silica-based core-shell nanoparticles such as mesoporous silica nanoparticles coated with PEG or hydrochloride dopamine along with epithelial cell adhesion molecule aptamer (MSN@PDA-PEGApt) were also used for targeted delivery of maytansine derivative (DM1) with 94% drug loading efficiency to treat colorectal cancer in mice (Li et al., 2017).
Metal-Organic Frameworks
Metal-organic frameworks (MOFs) are manufactured using metal nodes and organic linkers (Jiao et al., 2018). The MOFs-based biosensors have currently been applied for the detection of various targets, such as heavy metal ions (Zhang et al., 2017), hazard molecules (Liu et al., 2017), and living cancer cells (Gu et al., 2019). Most of the biosensors have been designed either for the detection of cancer markers (Jayanthi et al., 2017; Huang et al., 2018) or small biomolecules released from cancer cells for the early diagnosis such as the PBA(Ni-Fe):MoS2 hollow nanocubes for hydrogen peroxide detection (Zhang et al., 2019) and 3D bimetallic Au/Pt nanoflowers for the identification of cellular ATP secretion (Zhu et al., 2020).
Later on, integrated MOFs with electrochemically active other components have also become an efficient strategy to exploit the MOF-based cytosensors. In one such study bimetallic TbFe-MOFs have been developed using MOF on MOF strategy and was used for the anchoring of carbohydrate antigen 125 aptamers for identification of living Michigan Cancer Foundation-7 (MCF-7) cells (Li et al., 2020). Similarly, the bimetallic ZrHf-MOF doped with carbon dots was used in the identification of human epidermal growth factor receptor-2 (HER2) and HER2 expressed in MCF-7 cells (Gu et al., 2019). In another study, multicomponent Zr-MOFs were used for the detection of MCF-7 cells with an extremely low limit of detection as 31 cell ml−1 (Li et al., 2020).
Later, combinations of MOFs with different types of nanomaterials such as metal oxide, metal nanoparticles, and carbon-based nanomaterials were used to create newer forms of multifunctional composites/hybrids (Liang et al., 2018; Al-Sagur et al., 2019). Several organic compounds such as metallophthalocyanines (MPcs) (e.g., CuPc, FePc, CoPc, and NiPc) possess electro catalysis activity for the oxidation of biological compounds (Liu et al., 2017). The biological tissue of CRC possessed CT26 cells. Therefore, it is essential to detect the living CT26 cells for the early diagnosis of CRC (Peng, et al., 2020). In one of the studies a specific electrochemical cytosensor was described which was constructed on the Cr-MOF@CoPc nano hybrids for the detection of living CT26 cells (Duan et al., 2020). A Chlorin-Based nanoscale metal-organic framework was used for photodynamic therapy of colon cancers using mouse models and found that these have a great potential for clinical translation (Kuangda et al., 2015). In a recent study, zirconium-based metal-organic framework (MOF), PCN-223, was synthesized and used as a potential vehicle for 5FU for rectal delivery in diagnosis for CRC (Nea et al., 2020).
Gold Nanoparticles
Among the noble metal, gold nanoparticles (AuNPs) are considered the most stable nanomaterial that is used for the preparation of nanostructure with various structures and shapes such as nanocubes, nanospheres, nanorods, nanoflowers, nanobranches, nanowires, nano-bipyramids, nanoshells, and nanocages (Chen et al., 2008; Ramalingam et al., 2014; Li et al., 2015; Xiao et al., 2019). The recent advancement in technology has led to accurate surface coating of Au NPs with specific particle shape and size. These specificities of gold nanomaterials make it safer and specific anticancer and drug delivery agent (Siddique and Chow, 2020). Moreover, Artificial Intelligence and mathematical modeling-based study revealed that gold nanoparticle has the capability to adjust its optical densities, light absorbency, wavelengths. Therefore, adjusting the ideal wavelength with nanoparticle size may allow the higher amount of light absorbance within the nanoparticle itself leading to enhanced efficacy of gold nanoparticle against cancer cells (Moore and Chow, 2021). In a recent study gold NPs increase cisplatin delivery and potentiate chemotherapy by decompressing of CRC vessels (Zhao et al., 2018). Gold NPs along with nucleic acids used as molecular method for cellular uptake (Graczyk et al., 2020).
AuNPs have the property of easy to functionalize with biologically active organic molecules along with high physical and chemical stability leading to their excellent biocompatibility that is an essential feature to be used in the medical field (Pissuwan et al., 2019). Moreover, AuNPs can directly conjugate with several other molecules including antibodies, nucleic acids, proteins, enzymes, fluorescent dyes, and drugs which enhance their applications in medical and biological activities (Ramalingam, 2019). Gold nanoshells or gold nanospheres have also been intensively studied over the past decade because of their specific and localized surface plasmon resonance. Gold nanospheres can also easily be conjugated with several imaging reporters and can carry genes, drug payloads, and other chemotherapeutic agents for theranostic applications. Gold nanoparticles usually passively accumulate in tumors and exert specific pharmaceutical effects with active targeting ligands such as Apts, antibodies, and peptides to the required targets (Singhana et al., 2015).
In one of the studies, cellular prion protein (PrPC) aptamer (Apt) conjugated with AuNPs was used for targeted delivery of doxorubicin (Dox) to CRC as PrPC-Apt-functionalized doxorubicin-oligomer-AuNPs (PrPC-Apt DOA). The result revealed that in comparison with free Dox treatment, the oligomer PrPC-Apt DOA decreased the growth and increased the apoptosis of CRC cells to a significant extent. This indicated the possibility of the application of PrPC-Apt DOA as a therapeutic agent for CRC (Go et al., 2021). The gold nanoparticles (GNPs) may also be used to enhance the anticancer efficacy of 5-FU and reduce its side effects. The 5-FU can be loaded to GNPs using thiol-containing ligands, thioglycolic acid (TGA), and glutathione (GSH) as 5-FU/GSH-GNPs. Further study revealed that the release of 5-FU from GNPs was slow and induced apoptosis in colorectal cancer cells. Overall, 5-FU/GSH-GNPs showed two-fold higher anticancer efficacies than that with free 5-FU (Safwat et al., 2016). Moreover, the electroporation-GNPs technique may also provide the opportunity for colon cancer therapy especially for the highly immunogenic cancers where otherwise tumors are unresectable (Arab-Bafrani et al., 2020). The gold nanoparticle can also be used for the early diagnosis of CRC cells using colonoscopy. The Center of Cancer Nanotechnology at Stanford University developed a technique where gold nanoparticles are specifically allowed to bind the CRC cells. Subsequently, light from a colonoscope is allowed to shine, the gold nanoparticle bounded cancer cells stand out from the normal cells that can be removed easily. This technique is going to begin a clinical trial for safety evaluation in human patients soon (Advances in Colorectal Cancer Research, 2016).
Other Nanoparticles in Colorectal Cancer Detection and Treatment
Nanodrug delivery system can be used to encapsulate the chemotherapeutics agent to targeted drug delivery to cancer cells which can avoid the adverse effect of conventional treatment. In recent years several nano carriers with diverse characteristics have already been tested where polypeptide-based copolymers were found substantial consideration for their biocompatibility, slow biodegradability, and considerably lower toxicity. One such example assessed is poly(trimethylene carbonate)-block-poly(L-glutamic acid) derived polymersomes, targeted against the EGFR. It was loaded with plitidepsin and tested for efficacy and specificity in LS174T and HT29 cell lines (Goni-de-Cerio et al., 2015). Moreover, a systematic in vitro cytotoxicity study was also conducted with the unloaded polymersomes to determine the cell membrane asymmetry, viability, biocompatibility check, etc. which recognized the fine biocompatibility of plitidepsin-unloaded polymersomes. Further study revealed that the cellular uptake and cytotoxic effect exhibited by the EGFR-targeted plitideps in loaded polymersomes in CRC cell lines were found much more sensitive to anti-EGFR drug-loaded in comparison with targeted drug-loaded polymersomes. Moreover, untargeted polymersomes reduce the plitideps in cytotoxicity and cellular uptake which revealed the use of targeted nano-carrier in cell lines may be a line of treatment for CRC without any adverse effect on normal cells (Goni-de-Cerio et al., 2015).
Recently, one of the near-infra-red fluorescent proteinoid-poly(L-lactic acid)(PLLA) NPs preparation was described where a P(EF-PLLA) random copolymer was prepared using thermal copolymerization of L-phenylalanine(F), L-glutamicacid(E), and PLLA (Kolitz-Domb et al., 2014). The study revealed that under optimal conditions, the proteinoid-PLLA copolymer can self-assemble into hollow nano-sized particles that can be used to encapsulate the indocyanine green and NIR dye. Further study revealed that the encapsulation process enhances the photo stability of the dye. The anti-CEA antibodies and tumor-targeting ligands such as peanut agglutinin can be covalently conjugated to the surface of P (EF-PLLA) NPs and increases the detectable fluorescent signal from tumors. The efficacy of P (EF-PLLA) NPs for colon tumor detection has been demonstrated in the chicken embryo (Kolitz-Domb et al., 2014).
Over the years, several nano-formulations were being used to improve curcumin delivery to cancer sites (Figure 4). Nano-formulations are primarily used to enhance curcumin water solubility and to present a better consistent delivery for curcumin (Wong et al., 2019). Preferably, nano-formulation of curcumin for tumors should have improved anticancer activity as compared when curcumin alone and also nontoxic to normal cells. For CRC nano-formulation of curcumin has been documented in several investigations comprising micelles (Javadi et al., 2018), nano-gels (Madhusudana Rao et al., 2015), liposomes (Sesarman et al., 2018), polymeric NPs (Xiao et al., 2015), cyclodextrins (Ndong Ntoutoume et al., 2016), phytosomes (Marjaneh et al., 2018), solid lipid nanoparticles (Chirio et al., 2011), and gold NPs (SanojRejinold et al., 2015). Different nanomaterials for CRC detection and treatment have been discussed in Table 3. Although several nano formulations are undergoing clinical trials, the number of nano formulations used in clinical trials against the CRC is limited. Some of the nano formulations used for the appropriate clinical trials against the CRC are summarized in Table 4.
Combinatorial Nanomedicine and Colorectal Cancer Therapy
Nanomedicine has proved its efficacy in revolutionizing the therapeutics and diagnostics of cancers with the advancement in the development of nano devices. NPs have been used for the delivery of multidrug especially to mediate the drug resistance in relapsing cancers (Maya et al., 2014; Anitha et al., 2016). Recently, a study showing an improved efficacy of 5-FU assisted chemotherapy as a combinatorial strategy for colon cancer treatment was described (Anitha et al., 2014a; Maya et al., 2014). In combinatorial nanomedicine efficacy of both the drugs was enhanced due to nano encapsulation where 5-FU was used along with a nontoxic polymeric carrier system thiolated chitosan. In combinatorial use the dosage of 5-FU was decreased which was reflected with enhanced chemotherapeutic efficacy and beneficiary effect on CRC patient cases. The nano-sized drug-encapsulation systems possess specific efficacy in terms of passive retention of more drug-loaded NPs in the vicinity of cancer cells. These strategies have assisted in the development of the next phase of anticancer nanomedicine (Anitha et al., 2014; Malarvizhi et al., 2014; Maya et al., 2014; Linton et al., 2016).
Nanotechnology and Chemoprevention
Given side effects associated with chemotherapy, implementation of the nanotechnology-based formulation is very essential for cancer prevention, diagnosis, and treatment because it allows drug targeting and possible drug reduction which can furthermore increase the safety of drugs by minimizing nontargeted toxicities.
NSAIDs are most extensively used for colon cancer prevention (Alizadeh et al., 2012; Drew et al., 2016; U.S. Preventative Service Task Force, 2016; Pan et al., 2018). The epidemiological investigations demonstrate that out of all the NSAIDs, the most promising agent reported is aspirin in reducing adenomatous tumor recurrence due to the accessibility consistent result with minimum gastrointestinal toxicity and no cardiovascular risk (Umezawa et al., 2019). Additionally, Grade B recommendation has been provided for aspirin by the U.S. Preventative Service Task Force (2016) for its utilization as chronic prophylaxis means for CRC (Dehmer et al., 2015). Although aspirin alone or in combination has been proved for colon chemo preventive activity, nano encapsulation of aspirin can enhance its efficacy with a reduced dose. The chemo preventive effect of the combination of calcium, folic acid, and aspirin has been studied on azoxymethane-treated 7-week-old Sprague-Dawley male rats and found that 1.7 fold higher effective during chemoprevention as compared with their un-modified complement regimen (Prabhu et al., 2007; Chaudhary et al., 2011). Another NSAID is Celecoxib that is being broadly explored in clinical uses for its chemoprevention potential; however, it offers pharmacokinetic variability and cardiotoxicity (Solomon et al., 2005). Celecoxib polymeric NPs have been prepared using ethyl cellulose with lipid hybrid NPs, sodium casein ate bile salt and micro emulsions enhanced its bioavailability allowing a decrease in dose, crystallization, and related toxicity (Margulis-Goshen et al., 2011; Tan et al., 2011; Morgen et al., 2012). Naturally extracted plant-based chemicals are phytochemicals that are broadly studied as potential chemo preventive sources for their nontoxicity and pleiotropic effects (Thomasset et al., 2007; Zubair et al., 2017; Wong et al., 2019). In colon and intestinal cancer, curcumin has reported competent chemo protective activity, but it has poor absorption, minimum water solubility, and low bioavailability. To conquer the problem, curcumin whey protein nano capsules were synthesized, which not only found >70% release within 48 h but also showed improved bioavailability and cell internalization (Jayaprakasha et al., 2016). In other research, it was found that curcumin encapsulated in polymeric nano carrier enhanced the solubility of curcumin and showed less structural abnormalities, considerable reduction in tumors and beta-catenin in the treated group with curcumin NPs as compared with the group treated only with curcumin (Alizadeh et al., 2012).
Conclusion and Future Prospective
With the advancement in nanotechnologies, there has been increased research on the development of medical devices, targeted therapies, and novel drug delivery systems. Nanotechnology enabled the development of medical products from a single mode of action to multifunctional platforms such as nano theranostics to combine diagnostics and therapeutics. The preclinical study of nanomaterials has proved its efficacy as a therapeutics purpose of cancer. The detailed study of predictive immuno toxicity assays, nanoparticle surface characterization, and quantitative evaluation of encapsulated versus free drug fractions has highlighted the importance of nanotechnology in modern medicine. The advancement in drug delivery systems with the capability to modify the tissue uptake, bio-distribution of drugs, and pharmacokinetics of therapeutic agents has immense significance in biomedical research. Some of the nano carriers are even able to cross the blood-brain barrier (BBB) and may act at the cellular level. Moreover, several nanoparticle-based studies have focused on the development of techniques to customize novel drug conjugates and diagnostics as well as therapeutic devices. Nano carriers can also be programmed to release the therapeutic agents, fluorescent molecules, or even magnetic materials to the colorectal cancer site to increase the bioavailability, drug solubility, stability, and tumor specificity of therapeutics agent in comparison with free molecular cargo. Moreover, nano carriers-based therapeutics agents may decrease the tumor multidrug resistance resulting in ineffective treatment by reducing the overall drug requirement and potential side effects. In recent years, the nanotechnology applied to CRC has evolved enough to complement the latest advances in tumor diagnosis and therapy far beyond the traditional systems. It can be combined with completely newer concepts of diagnostics and therapeutics synergistically with available methodology. Thus, in the coming future despite the several challenges in the application of nanotechnology, nanomedicine is going to gain the capability to play a critical role in the management of human CRC. The specific research on new methods directed at the understanding of nano-bio interface may reveal some additional relationships between the nanoparticle structure and its biological activity. Such information may be used in devising new strategies for further development of nanotechnology to improve the existing pharmaceuticals and development of novel therapeutics products in the future.
Author Contributions
BB first prepare the whole manuscript. BB, KR, AP, MG, RK, and SS edited manuscript. Finally BB and PM critically analyse and finalize the manuscript.
Conflict of Interest
The authors declare that the research was conducted in the absence of any commercial or financial relationships that could be construed as a potential conflict of interest.
Publisher’s Note
All claims expressed in this article are solely those of the authors and do not necessarily represent those of their affiliated organizations, or those of the publisher, the editors and the reviewers. Any product that may be evaluated in this article, or claim that may be made by its manufacturer, is not guaranteed or endorsed by the publisher.
Acknowledgments
The authors highly acknowledge the HABITAT, GIPPCL Biofertilizer Production Unit CCSHAU, Hisar and Department of Animal Biotechnology, LUVAS, Hisar for providing support facility for preparation of the manuscript.
References
Abbasi, E., Aval, S. F., Akbarzadeh, A., Milani, M., Nasrabadi, H. T., Joo, S. W., et al. (2014). Dendrimers: Synthesis, Applications, and Properties. Nanoscale Res. Lett. 9 (1), 247. doi:10.1186/1556-276x-9-247
Abreu, A. S., Castanheira, E. M., Queiroz, M. J., Ferreira, P. M., Vale-Silva, L. A., and Pinto, E. (2011). Nanoliposomes for Encapsulation and Delivery of the Potential Antitumoral Methyl 6-Methoxy-3-(4-Methoxyphenyl)-1H-Indole-2-Carboxylate. Nanoscale Res. Lett. 6 (1), 482. doi:10.1186/1556-276X-6-482
Advances in Colorectal Cancer Research (2016). Nanotechnology to Improve Early Detection and Treatment of Colorectal Cancer. Available at: https://www.nih.gov/research-training/nanotechnology-improve-early-detection-treatment-colorectal-cancer.
Akbarzadeh, A., Rezaei-Sadabady, R., Davaran, S., Joo, S. W., Zarghami, N., Hanifehpour, Y., et al. (2013). Liposome: Classification, Preparation, and Applications. Nanoscale Res. Lett. 8 (1), 102. doi:10.1186/1556-276x-8-102
Akl, M. A., Kartal-Hodzic, A., Oksanen, T., Ismael, H. R., Afouna, M. M., Yliperttula, M., et al. (2016). Factorial Design Formulation Optimization and In Vitro Characterization of Curcumin-Loaded PLGA Nanoparticles for Colon Delivery. J. Drug Deliv. Sci. Tech. 32 (A), 10–20. doi:10.1016/j.jddst.2016.01.007
Al-Sagur, H., Shanmuga Sundaram, K., Kaya, E. N., Durmuş, M., Basova, T. V., and Hassan, T. V. A. (2019). Amperometric Glucose Biosensing Performance of a Novel Graphene Nanoplatelets-Iron Phthalocyanine Incorporated Conducting Hydrogel. Biosens. Bioelectron. 139, 111–323. doi:10.1016/j.bios.2019.111323
Alizadeh, A. M., Khaniki, M., Azizian, S., Mohaghgheghi, M. A., Sadeghizadeh, M., and Najafi, F. (2012). Chemoprevention of Azoxymethane-Initiated colon Cancer in Rat by Using a Novel Polymeric Nanocarrier-Curcumin. Eur. J. Pharmacol. 689, 226–232. doi:10.1016/j.ejphar.2012.06.016
Allen, J. E., Saroya, B. S., Kunkel, M., Dicker, D. T., Das, A., Peters, K. L., et al. (2014). Apoptotic Circulating Tumor Cells (CTCs) in the Peripheral Blood of Metastatic Colorectal Cancer Patients Are Associated with Liver Metastasis But Not CTCs. Oncotarget 5 (7), 1753–1760. doi:10.18632/oncotarget.1524
Allen, T. M., and Cullis, P. R. (2013). Liposomal Drug Delivery Systems: From Concept to Clinical Applications. Adv. Drug Deliv. Rev. 65 (1), 36–48. doi:10.1016/j.addr.2012.09.037
Amato, G. (2010). Silica-Encapsulated Efficient and Stable Si Quantum Dots with High Biocompatibility. Nanoscale Res. Lett. 5 (7), 1156–1160. doi:10.1007/s11671-010-9619-9
Anitha, A., Deepa, N., Chennazhi, K. P., Lakshmanan, V. K., and Jayakumar, R. (2014). Combinatorial Anticancer Effects of Curcumin and 5-Fluorouracil Loaded Thiolated Chitosan Nanoparticles Towards Colon Cancer Treatment. Biochim. Biophys. Acta 1840 (9), 2730–2743. doi:10.1016/j.bbagen.2014.06.004
Anitha, A., Maya, S., Sivaram, A. J., Mony, U., and Jayakumar, R. (2016). Combinatorial Nanomedicines for Colon Cancer Therapy. Wiley Interdiscip. Rev. Nanomed. Nanobiotechnol. 8 (1), 151–159. doi:10.1002/wnan.1353
Anitha, A., Sreeranganathan, M., Chennazhi, K. P., Lakshmanan, V. K., and Jayakumar, R. (2014a). In Vitro combinatorial Anticancer Effects of 5-Fluorouracil and Curcumin Loaded N,O-Carboxymethyl Chitosan Nanoparticles Toward Colon Cancer and In Vivo Pharmacokinetic Studies. Eur. J. Pharm. Biopharm. 88 (1), 238–251. doi:10.1016/j.ejpb.2014.04.017
Ansa, B. E., Coughlin, S. S., Alema-Mensah, E., and Smith, S. A. (2018). Evaluation of Colorectal Cancer Incidence Trends in the United States (2000–2014). J. Clin. Med. 7 (2), 22. doi:10.3390/jcm7020022
Arab-Bafrani, Z., Shahbazi-Gahrouei, D., and Abbasian, M. (2020). Rapid Delivery of Gold Nanoparticles Into Colon Cancer HT-29 Cells by Electroporation: In-Vitro Study. J. Biomed. Phys. Eng. 10 (2), 161–166. doi:10.31661/jbpe.v0i0.579
Arvelo, F., Sojo, F., and Cotte, C. (2015). Biology of Colorectal Cancer. Ecancermedicalscience 9, 520. doi:10.3332/ecancer.2015.520
Atkin, W. S., Edwards, R., and Kralj-Hans, I. (2010). Once-Only Flexible Sigmoidoscopy Screening in Prevention of Colorectal Cancer: A Multicentrerandomised Controlled Trial. Lancet 375 (9726), 1624–1633. doi:10.1016/s0140-6736(10)60551-x
Baetke, S. C., Lammers, T., and Kiessling, F. (2015). Applications of Nanoparticles for Diagnosis and Therapy of Cancer. Br. J. Radiol. 88 (1054), 20150207. doi:10.1259/bjr.20150207
Bai, Y., Teng, B., Chen, S., Chang, Y., and Li, Z. (2006). Preparation of Magnetite Nanoparticles Coated with an Amphiphilic Block Copolymer: A Potential Drug Carrier with a Core-Shell-Corona Structure for Hydrophobic Drug Delivery. Macromol. Rapid Commun. 27 (24), 2107–2112. doi:10.1002/marc.200600504
Bala, V., Rao, S., Boyd, B. J., and Prestidge, C. A. (2013). Prodrug and Nanomedicine Approaches for the Delivery of the Camptothecin Analogue SN38. J. Control Release 172, 48–61. doi:10.1016/j.jconrel.2013.07.022
Bangham, A. D., Standish, M. M., and Watkins, J. C. (1965). Diffusion of Univalent Ions across the Lamellae of Swollen Phospholipids. J. Mol. Biol. 13 (1), 238–IN27. doi:10.1016/s0022-2836(65)80093-6
Barenholz, Y. (2012). Doxil® - the First FDA-Approved Nano-Drug: Lessons Learned. J. Control. Release 160 (2), 117–134. doi:10.1016/j.jconrel.2012.03.020
Bharti, C., Gulati, N., Nagaich, U., and Pal, A. (2015). Mesoporous Silica Nanoparticles in Target Drug Delivery System: A Review. Int. J. Pharma Investig. 5 (3), 124. doi:10.4103/2230-973x.160844
Bibbins-Domingo, K., and Grossman, D. C. (2016). Screening for Colorectal Cancer: US Preventive Services Task Force Recommendation Statement. JAMA 315 (23), 2564–2575. doi:10.1001/jama.2016.5989
Bilensoy, E., Sarisozen, C., Esendaglı, G., Dogan, A. L., Aktaş, Y., and Şen, M. (2009). Intravesical Cationic Nanoparticles of Chitosan and Polycaprolactone for the Delivery of Mitomycin C to Bladder Tumors. Int. J. Pharm. 371 (1–2), 170–176. doi:10.1016/j.ijpharm.2008.12.015
Bose, S., Panda, A. K., Mukherjee, S., and Sa, G. (2015). Curcumin and Tumor Immune-Editing: Resurrecting the Immune System. Cell Div. 10 (1), 6. doi:10.1186/s13008-015-0012-z
Bours, M. J., Beijer, S., Winkels, R. M., van Duijnhoven, F. J., Mols, F., Breedveld-Peters, J. J., et al. (2015). Dietary Changes and Dietary Supplement Use, and Underlying Motives for These Habits Reported by Colorectal Cancer Survivors of the Patient Reported Outcomes Following Initial Treatment and Long-Term Evaluation of Survivorship (PROFILES) Registry. Br. J. Nutr. 114 (2), 286–296. doi:10.1017/s0007114515001798
Brand, M., Gaylard, P., and Ramos, J. (2017). Colorectal Cancer in South Africa: An Assessment of Disease Presentation, Treatment Pathways and 5-Year Survival. S. Afr. Med. J. 108, 118–122. doi:10.7196/SAMJ.2017.v108i2.12338
Bray, F., Ferlay, J., Soerjomataram, I. R. L., Siegel, L. A., and Jemal, T. A. (2019). Global Cancer Statistics 2018: GLOBOCAN Estimates of Incidence and Mortality Worldwide for 36 Cancers in 185 Countries. CA Cancer J. Clin. 68, 394–424. doi:10.3322/caac.21492
Brenner, H., Stock, C., and Hoffmeister, M. (2014). Effect of Screening Sigmoidoscopy and Screening Colonoscopy on Colorectal Cancer Incidence and Mortality: Systematic Review and Meta-Analysis of Randomised Controlled Trials and Observational Studies. BMJ 348, g2467. doi:10.1136/bmj.g2467
Burch, J. A., Soares-Weiser, K., and John, D. J. (2007). Diagnostic Accuracy of Faecal Occult Blood Tests Used Inscreening for Colorectal Cancer: A Systematic Review. J. Med. Screen. 14 (3), 132–137. doi:10.1258/096914107782066220
Cabeza, L., Perazzoli, G., Mesas, C., Jiménez-Luna, C., Prados, J., Rama, A. R., et al. (2020). Nanoparticles in Colorectal Cancer Therapy: Latest In Vivo Assays, Clinical Trials, and Patents. AAPS PharmSciTech. 21 (5), 178. doi:10.1208/s12249-020-01731-y
Canton, A. S., Broek, N. V. D., and Danelon, C. (2019). Development of a Lipid-Based Delivery System for the Chemotherapeutic Compound SN-38. bioRxiv. doi:10.1101/792317
Carbary-Ganz, J. L., Welge, W. A., Barton, J. K., and Utzinger, U. (2015). In Vivo Molecular Imaging of Colorectal Cancer Using Quantum Dots Targeted to Vascular Endothelial Growth Factor Receptor 2 and Optical Coherence Tomography/Laser-Induced Fluorescence Dual-Modality Imaging. J. Biomed. Opt. 20 (9), 096015. doi:10.1117/1.jbo.20.9.096015
Celsion (2016). Phase 2 Study of Thermodox as Adjuvant Therapy with Thermal Ablation (RFA) in Treatment of Metastatic Colorectal Cancer (mCRC) (ABLATE). Clinical Trials. Available at: https://clinicaltrials.gov/ct2/show/NCT01464593.
Chaudhary, A., Sutaria, D., Huang, Y., Wang, J., and Prabhu, S. (2011). Chemo prevention of Colon Cancer in a Rat Carcinogenesis Model Using a Novel Nanotechnology-Based Combined Treatment System. Cancer Prev. Res. 4, 1655–1664. doi:10.1158/1940-6207.CAPR-11-0129
Chen, B. H., Huang, R. F. S., Wei, Y. J., and Stephen Inbaraj, B. (2015). Inhibition of Colon Cancer Cell Growth by Nano Emulsion Carrying Gold Nanoparticles and Lycopene. Int. J. Nanomed. 10 (1), 2823–2846. doi:10.2147/ijn.s79107
Chen, H., Kou, X., Yang, Z., Ni, W., and Wang, J. (2008). Shape- and Size Dependent Refractive index Sensitivity of Gold Nanoparticles. Langmuir 24, 5233–5237. doi:10.1021/la800305j
Chibaudel, B., Maindrault-Gœbel, F., Bachet, J-B., Louvet, C., Khalil, A., Dupuis, O., et al. (2016). PEPCOL: A GERCOR Randomized Phase II Study of Nano Liposomal Irinotecan PEP02 (MM-398) or Irinotecan with Leucovorin/5-Fluorouracil as Second-Line Therapy in Metastatic Colorectal Cancer. Cancer Med. 5 (4), 676–683. doi:10.1002/cam4.635
Chirio, D., Gallarate, M., Peira, E., Battaglia, L., Serpe, L., and Trotta, M. (2011). Formulation of Curcumin-Loaded Solid Lipid Nanoparticles Produced by Fatty Acids Coacervation Technique. J. Microencapsul. 28, 537–548. doi:10.3109/02652048.2011.590615
Chu, E. (2012). An Update on the Current and Emerging Targeted Agents in Metastatic Colorectal Cancer. Clin. Colorectal Cancer 11, 1–13. doi:10.1016/j.clcc.2011.05.005
Chuah, L. H., Roberts, C. J., Billa, N., Abdullah, S., Rosli, R., and Manickam, S. (2014). Using Nanoparticle Tracking Analysis (NTA) to Decipher Mucoadhesion Propensity of Curcumin-Containing Chitosan Nanoparticles and Curcumin Release. J. Dispersion Sci. Technol. 35 (9), 1201–1207. doi:10.1080/01932691.2013.800458
Collins, J. F., Lieberman, D. A., Durbin, T. E., and Weiss, D. G. (2005). Accuracy of Screening for Fecal Occult Blood on a Single Stool Sample Obtained by Digital Rectal Examination: A Comparison with Recommended Sampling Practice. Ann. Intern. Med. 142 (2), 81–85. doi:10.7326/0003-4819-142-2-200501180-00006
da Paz, M. C., Santos, M. F., and Santos, C. M. (2012). Anti-CEA Loaded Maghemite Nanoparticles as a Theragnostic Device for Colorectal Cancer. Int. J. Nanomed. 7, 5271–5282. doi:10.2147/IJN.S32139
Datta, K., Suman, S., Kumar, S., and Fornace, A. J. (2016). Colorectal Carcinogenesis, Radiation Quality, and the Ubiquitin-Proteasome Pathway. J. Cancer 7 (2), 174–183. doi:10.7150/jca.13387
Dehmer, S. P., Maciosek, M. V., and Flottemesch, T. J. (2015). “U.S. Preventive Services Task Force Evidence Syntheses, Formerly Systematic Evidence Reviews,” in Aspirin Use to Prevent Cardiovascular Disease and Colorectal Cancer: A Decision Analysis: Technical Report. Rockville, MD: Agency for Healthcare Research and Quality (US).
DeNardo, G. L., and DeNardo, S. J. (2012). Concepts, Consequences, and Implications of Theranosis. Semin. Nucl. Med. 42, 147–150. doi:10.1053/j.semnuclmed.2011.12.003
Dienstmann, R., Salazar, R., and Tabernero, J. (2015). Overcoming Resistance to Anti-EGFR Therapy in Colorectal Cancer. Am. Soc. Clin. Oncol. Educ. Book 35, e149–e156. doi:10.14694/EdBook_AM.2015.35.e149
Dong, Z., Cui, M. Y., and Peng, Z. (2016). Nanoparticles for Colorectal Cancer-Targeted Drug Delivery and MR Imaging: Current Situation and Perspectives. Curr. Cancer Drug Targets 16 (6), 536–550. doi:10.2174/1568009616666151130214442
Drew, D. A., Cao, Y., and Chan, A. T. (2016). Aspirin and Colorectal Cancer: The Promise of Precision Chemoprevention. Nat. Rev. Cancer 16, 173–186. doi:10.1038/nrc.2016.4
Duan, F., Hu, M., Guo, C., Song, Y., Wang, M., He, L., et al. (2020). Chromium-based Metal-Organic Framework Embedded with Cobalt Phthalocyanine for the Sensitively Impedimetric Cytosensing of Colorectal Cancer (CT26) Cells and Cell Imaging. Chem. Eng. J. 398, 125452. doi:10.1016/j.cej.2020.125452
Elmunzer, B. J., Hayward, R. A., Schoenfeld, P. S., Saini, S. D., Deshpande, A., and Waljee, A. K. (2012). Effect of Flexible Sigmoidoscopy-Based Screening Onincidence and Mortality of Colorectal Cancer: A Systematic Review and Meta-Analysis of Randomized Controlled Trials. PLoS Med. 9 (12), 1001352. doi:10.1371/journal.pmed.1001352
Esmaelbeygi, E., Khoei, S., Khoee, S., and Eynali, S. (2015). Role of Iron Oxide Core of Polymeric Nanoparticles in the Thermo Sensitivity of Colon Cancer Cell Line HT-29. Int. J. Hyperthermia 31 (5), 489–497. doi:10.3109/02656736.2015.1035766
Espinosa, A., Di Corato, R., Kolosnjaj Tabi, J., Flaud, P., Pellegrino, T., and Wilhelm, C. (2016). Duality of Iron Oxide Nanoparticles in Cancer Therapy:amplification of Heating Efficiency by Magnetic Hyperthermia Andphotothermal Bimodal Treatment. ACS Nano 10 (2), 2436–2446. doi:10.1021/acsnano.5b07249
Essa, S., Daoud, J., Lafleur, M., Martel, S., and Tabrizian, M. (2015). SN-38 Active Loading in Poly(Lactic-Co-Glycolic Acid) Nanoparticles and Assessment of Their Anticancer Properties on COLO-205 Human colon Adenocarcinoma Cells. J Microencapsul 32 (8), 784–793. doi:10.3109/02652048.2015.1081416
Fang, M., Peng, C. W., Pang, D. W., and Li, Y. (2012). Quantum Dots for Cancer Research: Current Status, Remaining Issues, and Future Perspectives. Cancer Biol. Med. 9 (3), 151–163. doi:10.7497/j.issn.2095-3941.2012.03.001
Feng, S. T., Li, J., and Luo, Y. (2014). pH-Sensitive Nanomicelles for Controlled and Efficient Drug Delivery to Human Colorectal Carcinoma LoVo Cells. PLoS One 9 (6), 100732. doi:10.1371/journal.pone.0100732
Fortina, P., Kricka, L. J., Graves, D. J., Park, J., Hyslop, T., Tam, F., et al. (2007). Applications of Nanoparticles to Diagnostics and Therapeutics in Colorectal Cancer. Trends. Biotechnol. 25 (4), 145–155. doi:10.1016/j.tibtech.2007.02.005
Gazouli, M., Bouziotis, P., Lyberopoulou, A., Ikonomopoulos, J., Papalois, A., Anagnou, N. P., et al. (2014). Quantum Dots-Bevacizumab Complexes for In Vivo Imaging of Tumors. In Vivo 28 (6), 1091–1095.
Ghosh, C. R., and Paria, S. (2012). Core/Shell Nanoparticles: Classes, Properties, Synthesis Mechanisms, Characterization, and Applications. Chem. Rev. 112 (4), 2373–2433. doi:10.1021/cr100449n
Gidding, C. E., Kellie, S. J., Kamps, W. A., and de Graaf, S. S. (1999). Vincristine Revisited. Crit. Rev. Oncol. Hematol. 29 (3), 267–287. doi:10.1016/s1040-8428(98)00023-7
Giglio, V., Sgarlata, C., and Vecchio, G. (2015). Novel Amino-Cyclodextrin Cross-Linked Oligomer as Efficient Carrier for Anionic Drugs: A Spectroscopic and Nanocalorimetric Investigation. RSC Adv. 5, 16664–16671. doi:10.1039/c4ra16064a
Go, G., Lee, C. S., Yoon, Y. M., Lim, J. H., Kim, T. H., and Lee, S. H. (2021). PrPC Aptamer Conjugated-Gold Nanoparticles for Targeted Delivery of Doxorubicin to Colorectal Cancer Cells. Int. J. Mol. Sci. 22 (4), 1976. doi:10.3390/ijms22041976
Goni-de-Cerio, F., Thevenot, J., and Oliveira, H. (2015). Cellular Uptake and Cytotoxic Effect of Epidermal Growth Factor Receptor Targeted and Plitidepsin Loaded Co-Polymeric Polymersomes on Colorectal Cancer Cell Lines. J. Biomed. Nanotechnology 11 (11), 2034–2049. doi:10.1166/jbn.2015.2148
Graczyk, A., Pawlowska, R., Jedrzejczyk, D., and Chworos, A. (2020). Gold Nanoparticles in Conjunction with Nucleic Acids as a Modern Molecularsystem for Cellular Delivery. Molecules 25, 204. doi:10.3390/molecules25010204
Gu, C., Guo, C., Li, Z., Wang, M., Zhou, M. N., He, L., et al. (2019). Bimetallic ZrHf Based Metal-Organic Framework Embedded with Carbon Dots: Ultra-Sensitive Platform for Early Diagnosis of HER2 and HER2-Overexpressed Living Cancer Cells. Biosens. Bioelectron. 134, 8–15. doi:10.1016/j.bios.2019.03.043
Gulbake, A., Jain, A., Jain, A., Jain, A., and Jain, S. K. (2016). Insight to Drug Delivery Aspects for Colorectal Cancer. World J. Gastroenterol. 22 (2), 582–599. doi:10.3748/wjg.v22.i2.582
Guo, C., Liu, Q., and Dai, M. (2015). Colorectal Cancer Screening: Situation and prospect. Zhonghua Yu Fang Yi Xue Za Zhi 49 (5), 377–380. doi:10.1093/geront/gnv170.03
Haggar, F., and Boushey, R. (2009). Colorectal Cancer Epidemiology: Incidence, Mortality, Survival, and Risk Factors. Clin. Colon Rectal Surg. 22 (04), 191–197. doi:10.1055/s-0029-1242458
Hamaguchi, T., Tsuji, A., Yamaguchi, K., Takeda, K., Uetake, H., Esaki, T., et al. (2018). A Phase II Study of NK012, a Polymeric Micelle Formulation of SN-38, in Unresectable, Metastatic or Recurrent Colorectal Cancer Patients. Cancer Chemother. Pharmacol. 82 (6), 1021–1029. doi:10.1007/s00280-018-3693-6
Hampel, S., Kunze, D., Haase, D., Kramer, K., Rauschenbach, M., Ritschel, M., et al. (2008). Carbon Nanotubes Filled with a Chemotherapeutic Agent: A Nanocarrier Mediates Inhibition of Tumor Cell Growth. Nanomedicine 3 (2), 175–182. doi:10.2217/17435889.3.2.175
Hanafi-Bojd, M. Y., Jaafari, M. R., Ramezanian, N., Xue, M., Amin, M., Shahtahmassebi, N., et al. (2015). Surface Functionalized Mesoporous Silica Nanoparticles as an Effective Carrier for Epirubicin Delivery to Cancer Cells. Eur. J. Pharm. Biopharm. 89, 248–258. doi:10.1016/j.ejpb.2014.12.009
He, X., Liu, F., Liu, L., Duan, T., Zhang, H., and Wang, Z. (2014). Lectin-Conjugated Fe2O3@Au Core@Shell Nanoparticles as Dual Mode Contrast Agents for In Vivo Detection of Tumor. Mol. Pharm. 11 (3), 738–745. doi:10.1021/mp400456j
Holme, L., M., and Kalager, M. (2014). Effect of Flexible Sigmoidoscopy Screening on Colorectal Cancer Incidence and Mortality: A Randomized Clinical Trial. JAMA 312 (6), 606–615. doi:10.1001/jama.2014.8266
Hosseinifar, T., Sheybani, S., Abdouss, M., Najafabadi, S. A., and Ardestani, M. (2018). Pressure Responsive Nanogel Base on Alginate-Cyclodextrin with Enhanced Apoptosis Mechanism for colon Cancer Delivery. J. Biomed. Mater. Res. A. 106 (2), 349–359. doi:10.1002/jbm.a.36242
Huang, R. F., Wei, Y. J., Inbaraj, B. S., and Chen, B. H. (2015b). Inhibition of Colon Cancer Cell Growth by Nanoemulsion Carrying Gold Nanoparticles and Lycopene. Int. J. Nanomed. 10, 2823–2846. doi:10.2147/IJN.S79107
Huang, R., He, N., and Li, Z. (2018). Recent Progresses in DNA Nanostructure-Based Biosensors for Detection of Tumor Markers. Biosens. Bioelectron. 109, 27–34. doi:10.1016/j.bios.2018.02.053
Huang, W., Wang, X., and Shi, C. (2015a). Fine-tuning Vitamin E-Containing Telodendrimers for Efficient Delivery of Gambogic Acid in Colon Cancer Treatment. Mol. Pharm. 12 (4), 1216–1229. doi:10.1021/acs.molpharmaceut.5b00051
Hutchinson, R. A., McArt, D. G., Salto-Tellez, M., Jasani, B., and Hamilton, P. W. (2015). Epidermal Growth Factor Receptor Immunohistochemistry: New Opportunities in Metastatic Colorectal Cancer. J. Transl. Med. 13, 217. doi:10.1186/s12967-015-0531-z
Huyghe, N., Baldin, P., and Van den Eynde, M. (2020). Immunotherapy with Immune Checkpoint Inhibitors in Colorectal Cancer: what Is the Future beyond Deficient Mismatch-Repair Tumors? Gastroenterol. Rep. 8, 11–24. doi:10.1093/gastro/goz061
Imperiale, T. F., Ransohoff, D. F., Itzkowitz, S. H., Turnbull, B. A., and Ross, M. E. (2004). Fecal DNA Versus Fecal Occult Blood for Colorectal-Cancer Screening in an Average-Risk Population. New Engl. J. Med. 351 (26), 2704–2714. doi:10.1056/NEJMoa033403
Javadi, S., Rostamizadeh, K., Hejazi, J., Parsa, M., and Fathi, M. (2018). Curcumin Mediated Down-Regulation of alphaV Beta3 Integrin and Up-Regulation of Pyruvate Dehydrogenase Kinase 4 (PDK4) in Erlotinib Resistant SW480 Colon Cancer Cells. Phytother. Res. 32, 355–364. doi:10.1002/ptr.5984
Jayakumar, J., Mohammed, Z. H., Jayaprakash, B. U., Ramzi, M. M., and Bhat, A. A. (2015). “Recent Developments in Nanomedicine; Treatment Options for Colorectal Cancer,” in Modern Technology: Present and Future of Cancer. Editor M. A. Macha (San Mateo, CA: OMICS Group eBooks).
Jayanthi, V. S. P. K., Das, A. B., and Saxena, U. (2017). Recent Advances in Biosensor Development for the Detection of Cancer Biomarkers. Biosens. Bioelectron. 91, 15–23. doi:10.1016/j.bios.2016.12.014
Jayaprakasha, G. K., Chidambara Murthy, K. N., and Patil, B. S. (2016). Enhanced colon Cancer Chemoprevention of Curcumin by Nanoencapsulation with Whey Protein. Eur. J. Pharmacol. 789, 291–300. doi:10.1016/j.ejphar.2016.07.017
Jensen, N. F., Stenvang, J., Beck, M. K., Hanáková, B., Belling, K. C., Do, K. N., et al. (2015). Establishment and Characterization of Models of Chemotherapy Resistance in Colorectal Cancer: towards a Predictive Signature of Chemoresistance. Mol. Oncol. 9 (6), 1169–1185. doi:10.1016/j.molonc.2015.02.008
Jiao, L., Wang, Y., Jiang, H.-L., and Xu, Q. (2018). Metal-Organic Frameworks as Platforms for Catalytic Applications. Adv. Mater. 30, 1703663. doi:10.1002/adma.201703663
Johdi, N. A., and Sukor, N. F. (2020). Colorectal Cancer Immunotherapy: Options and Strategies. Front. Immunol. 11, 1624. doi:10.3389/fimmu.2020.01624pp1-17
Joye, I., and Haustermans, K. (2014). “Early and Late Toxicity of Radiotherapy for Rectal Cancer,” in Early Gastrointestinal Cancers II: Rectal Cancer. Editors F. Otto, and M. P. Lutz (Switzerland: Springer International Publishing), 189–201. doi:10.1007/978-3-319-08060-4_13
Kahi, C. J., Boland, C. R., and Dominitz, J. A. (2016). Colonoscopy Surveillance after Colorectal Cancer Resection: Recommendations of the US Multi-Society Task Force on Colorectal Cancer. Am. J. Gastroenterol. 111 (3), 337–346. doi:10.1038/ajg.2016.22
Kalele, S., Gosavi, S., Urban, J., and Kulkarni, S. (2006). Nanoshell Particles: Synthesis, Properties and Applications. Curr. Sci. 91 (8), 1038–1052.
Kheirelseid, E., Miller, N., and Kerin, M. (2013). Molecular Biology of Colorectal Cancer: Review of the Literature. Am. J. Mol. Biol. 3, 72–80. doi:10.4236/ajmb.2013.32010
Kim, J., Lee, N., and Hyeon, T. (2017). Recent Development of Nanoparticles for Molecular Imaging. Philos. Trans. A. Math. Phys. Eng. Sci. 375 (2107), 20170022. doi:10.1098/rsta.2017.0022
Kolitz-Domb, M., Corem-Salkmon, E., Grinberg, I., and Margel, S. (2014). Synthesis and Characterization of Bioactive Conjugated Near-Infrared Fluorescent Proteinoid-poly (L-Lactic Acid) Hollow Nanoparticles for Optical Detection of Colon Cancer. Int. J. Nanomed. 9, 5041–5053. doi:10.2147/IJN.S68582
Kuangda, Lu., Chunbai, He., and Wenbin, L. (2015). A Chlorin-Based Nanoscale Metal–Organic Framework for Photodynamic Therapy of Colon Cancers. J. Am. Chem. Soc. 137 (24), 7600–7603. doi:10.1021/jacs.5b04069
Kuipers, E. J., and Spaander, M. C. (2015). Colorectal Cancer Screening by Colonoscopy, CT-Colonography, or Fecal Immunochemical Test. J. Natl. Cancer Inst. 108 (2), djv383. doi:10.1093/jnci/djv383
Kumar, R., Mondal, K., Panda, P. K., Kaushik, A., Abolhassani, R., Ahuja, R., et al. (2020). Core-Shell Nanostructures: Perspectives Towards Drug Delivery Applications. J. Mater. Chem. B 8.39, 8992–9027. doi:10.1039/d0tb01559h
Kuo, C. Y., Liu, T. Y., and Chan, T. Y. (2016). Magnetically Triggered Nano Vehicles for Controlled Drug Release as a Colorectal Cancer Therapy. Colloids Surf. B Biointerfaces 140, 567–573. doi:10.1016/j.colsurfb.2015.11.008
Lai, Y., Wang, C., and Civan, J. M. (2016). Effects of Cancer Stage and Treatment Differences on Racial Disparities in Survival from colon Cancer: a United States Population-Based Study. Gastroenterology 150 (5), 1135–1146. doi:10.1053/j.gastro.2016.01.030
Lam, R., and Ho, D. (2009). Nanodiamonds as Vehicles for Systemic and Localized Drug Delivery. Expert Opin. Drug Deliv. 6 (9), 883–895. doi:10.1517/17425240903156382
Lammers, T., Hennink, W. E., and Storm, G. (2008). Tumour-targeted Nanomedicines: Principles and Practice. Br. J. Cancer 99 (3), 392–397. doi:10.1038/sj.bjc.6604483
Laroui, H., Rakhya, P., Xiao, B., Viennois, E., and Merlin, D. (2013). Nanotechnology Diagnostics and Therapeutics for Gastrointestinal Disorders. Dig. Liver Dis. 45 (12), 995–1002. doi:10.1016/j.dld.2013.03.019
Lecuyer, T., Teston, E., Ramirez-Garcia, G., Maldiney, T., Viana, B., Seguin, J., et al. (2016). Chemically Engineered Persistent Luminescence Nanoprobes for Bioimaging. Theranostics 6 (13), 2488–2524. doi:10.7150/thno.16589
Lee, S. W. (2009). Laparoscopic Procedures for colon and Rectal Cancer Surgery. Clin. Colon Rectal Surg. 22, 218–224. doi:10.1055/s-0029-1242461
Lee, Y., and Geckeler, K. E. (2012). Cellular Interactions of a Water-Soluble Supramolecular Polymer Complex of Carbon Nanotubes with Human Epithelial Colorectal Adenocarcinoma Cells. Macromol. Biosci. 12 (8), 1060–1067. doi:10.1002/mabi.201200085
Levi-Polyachenko, N. H., Merkel, E. J., Jones, B. T., Carroll, D. L., and Stewart, J. H. (2009). Rapid Photothermal Intracellular Drug Delivery Using Multiwalled Carbon Nanotubes. Mol. Pharm. 6 (4), 1092–1099. doi:10.1021/mp800250e
Li, S., Zhang, L., Wang, T., Li, L., Wang, C., and Su, Z. (2015). The Facile Synthesis of Hollow Au Nanoflowers for Synergistic Chemo-Photothermal Cancer Therapy. Chem. Commun. 51, 14338–14341. doi:10.1039/C5CC05676D
Li, W., Chen, H., Yu, M., and Fang, J. (2014). Targeted Delivery of Doxorubicin Using a Colorectal Cancer-Specific ssDNA Aptamer. Anat. Rec. 297 (12), 2280–2288. doi:10.1002/ar.22990
Li, W. M., Bing, T., Wei, J. Y., Chen, Z. Z., Shangguan, D. H., and Fang, J. (2014b). Cell-SELEX-Based Selection of Aptamers that Recognized is Tinct Target Son Metastatic Colorectal Cancer Cells. Biomaterials 35 (25), 6998–7007. doi:10.1016/j.biomaterials.2014.04.112
Li, Y., Duo, Y., Bao, S., He, L., Ling, K., Luo, J., et al. (2017). EpCAM Aptamer-Functionalized Polydopamine-Coated Mesoporous Silica Nanoparticles Loaded with DM1 for Targeted Therapy in Colorectal Cancer. Int. J. Nanomed. 12, 6239–6257. doi:10.2147/ijn.s143293
Li, Y., Hu, M., Huang, X., Wang, M., He, L., Song, Y., et al. (2020). Multicomponent Zirconium-Based Metal-Organic Frameworks for Impedimetric Aptasensing of Living Cancer Cells. Sens. Actuat. B-Chem. 306, 127608. doi:10.1016/j.snb.2019.127608
Liang, J., Xie, Y., Wang, X., Wang, Q., Liu, T., Huang, Y., et al. (2018). An Imidazoliumfunctionalized Mesoporous Cationic Metal-Organic Framework for Cooperative CO2 Fixation into Cyclic Carbonate. Chem. Commun. 54, 342–345. doi:10.1039/c7cc08630j
Lima, S. A. C., Gaspar, A., Reis, S., and Duraes, L. (2017). Multifunctional Nanospheres for Co-delivery of Methotrexate and Mild Hyperthermia to colon Cancer Cells. Mater. Sci. Eng. C 75, 14201426. doi:10.1016/j.msec.2017.03.049
Linton, S., Sherwood, S. S. G., Drews, K. C., and Kester, M. (2016). Targeting Cancer Cells in the Tumor Microenvironment: Opportunities and Challenges in Combinatorial Nanomedicine, Wiley Interdiscip. Rev. Nanomed. Nanobiotechnol. 8, 208–222. doi:10.1002/wnan.1358
Liu, J., Lecuyer, T., Seguin, J., Mignet, N., Scherman, D., Viana, B., et al. (2019). Imaging and Therapeutic Applications of Persistent Luminescence Nanomaterials. Adv. Drug Deliv. Rev. 138, 193–210. doi:10.1016/j.addr.2018.10.015
Liu, R., Zheng, S., Yang, C. Y., Yu, Y., Peng, S., Ge, Q., et al. (2021). Prognostic Value of Aldo-Keto Reductase Family 1 Member B10 (AKR1B10) in Digestive System Cancers: A Meta-Analysis. Medicine 100 (14), e25454. doi:10.1097/md.0000000000025454
Liu, X., Hu, M., Wang, M., Song, Y., Zhou, N., He, L., et al. (2017). Novel Nanoarchitecture of Co-MOF-on-TPN-COF Hybrid: Ultralowly Sensitive Bioplatform of Electrochemical Platform for High Trace Determination of Heavy Metal Ions. Biosens. Bioelectron. 94, 358–364.
Lohsiriwat, V., Siriluck, P., and Wanwarang, S. (2013). Colorectal Cancer Screening by Double Contrast Barium Enema in Thai People. Asian Pac. J. Cancer Prev 13, 1273–1276. doi:10.7314/apjcp.2012.13.4.1273
Madhusudana Rao, K., Krishna Rao, K. S., Ramanjaneyulu, G., and Ha, C. S. (2015). Curcumin Encapsulated pH Sensitive Gelatin Based Interpenetrating Polymeric Network Nanogels for Anti Cancer Drug Delivery. Int. J. Pharm. 478, 788–795. doi:10.1016/j.ijpharm.2014.12.001
Maksimenko, A., Alami, M., Zouhiri, F., Brion, J. D., Pruvost, A., Mougin, J., et al. (2014). Therapeutic Modalities of Squalenoyl Nanocomposites in Colon Cancer: an Ongoing Search for Improved Efficacy. ACS Nano 8 (3), 2018–2032. doi:10.1021/nn500517a
Malarvizhi, G. L., Retnakumari, A. P., Nair, S., and Koyakutty, M. (2014). Transferrin Targeted Core-Shell Nanomedicine for Combinatorial Delivery of Doxorubicin and Sorafenib Against Hepatocellular Carcinoma. Nanomedicine 10 (8), 1649–1659. doi:10.1016/j.nano.2014.05.011
Margulis-Goshen, K., Weitman, M., Major, D. T., and Magdassi, S. (2011). Inhibition of Crystallization and Growth of Celecoxib Nanoparticles Formed from Volatile Micro Emulsions. J. Pharm. Sci. 100, 4390–4400. doi:10.1002/jps.22623
Marjaneh, R. M., Rahmani, F., Hassanian, S. M., Rezaei, N., Hashemzehi, M., Bahrami, A., et al. (2018). Phytosomal Curcumin Inhibits Tumor Growth in Colitis-Associated Colorectal Cancer. J. Cel. Physiol. 233, 6785–6798. doi:10.1002/jcp.26538
Maya, S., Sarmento, B., Lakshmanan, V. K., Menon, D., and Jayakumar, R. (2014). Actively Targeted Cetuximab Conjugated Gamma-Poly(glutamic Acid)-Docetaxel Nanomedicines for Epidermal Growth Factor Receptor Over Expressing Colon Cancer Cells. J. Biomed. Nanotechnol 10 (8), 1416–1428. doi:10.1166/jbn.2014.1841
Minakshi, P., Kumar, R., GhoshBrar, M. B., Barnela, M., and Lakhani, P. (2020). Application of Polymeric Nano-Materials in Management of Inflammatory Bowel Disease. Curr. Top. Med. Chem. 20, 982–1008. doi:10.2174/1568026620666200320113322
Minakshi, P., Lambe, U., Ranjan, K., Patil, S., Brar, B., Devi, B., et al. (2018). An Insight in Biomarkers for Colorectal Cancer. Gastroenterol. Liver Dis. 3 (1), 1–15.
Minakshi, P., Upendra, P. L., Brar, B., Ikbal, S., Manimegalai, J., Koushlesh, R., et al. (2017). Nanotherapeutics: An Insight into Healthcare and Multi-Dimensional Applications in Medical Sector of the Modern World. Biomed. Pharmacother. 97, 1521–1537. doi:10.1016/j.biopha.2017.11.026
Moore, J. A., and Chow, J. C. L. (2021). Recent Progress and Applications of Gold Nanotechnology in Medical Biophysics Using Artificial Intelligence and Mathematical Modelling. Nano Express 2 (2021), 022001. doi:10.1088/2632-959x/abddd3
Morgen, M., Bloom, C., Beyerinck, R., Bello, A., Song, W., Wilkinson, K., et al. (2012). Polymeric Nanoparticles for Increased Oral Bioavailability and Rapid Absorption Using Celecoxib as a Model of a Low-Solubility, High-Permeability Drug. Pharm. Res. 29, 427–440. doi:10.1007/s11095-011-0558-7
Mostert, B., Sieuwerts, A. M., and Bolt-deVries, J. (2015). mRNA Expression Profiles in Circulating Tumor Cells of Metastatic Colorectal Cancer Patients. Mol. Oncol. 9 (4), 920–932. doi:10.1016/j.molonc.2015.01.001
Nag, O., and Awasthi, V. (2013). Surface Engineering of Liposomes for Stealth Behavior. Pharmaceutics 5 (4), 542–569. doi:10.3390/pharmaceutics5040542
Ndong Ntoutoume, G. M. A., Granet, R., Mbakidi, J. P., Brégier, F., Léger, D. Y., Fidanzi-Dugas, C., et al. (2016). Development of Curcumin-Cyclodextrin/Cellulose Nanocrystals Complexes: New Anticancer Drug Delivery Systems. Bioorg. Med. Chem. Lett. 26, 941–945. doi:10.1016/j.bmcl.2015.12.060
Nea, C. Y., Kim, N. S., Choi, S. Y., and Choy, B. Y. (2020). PCN-223 as a Drug Carrier for Potential Treatment of Colorectal Cancer. J. Ind. Eng. Chem. 84, 290–296. doi:10.1016/j.jiec.2020.01.010
Noble, G. T., Stefanick, J. F., Ashley, J. D., Kiziltepe, T., and Bilgicer, B. (2014). Ligand-targeted Liposome Design: Challenges and Fundamental Considerations. Trends Biotechnol. 32 (1), 32–45. doi:10.1016/j.tibtech.2013.09.007
Odin, E., Sondén, A., Gustavsson, B., Carlsson, G., and Wettergren, Y. (2015). Expression of Folate Pathway Genes in Stage III Colorectal Cancer Correlates with Recurrence Status Following Adjuvant Bolus 5-FU-Based Chemotherapy. Mol. Med. Camb. Mass. 21, 597–604. doi:10.2119/molmed.2014.00192
Ouyang, D. L., Chen, J. J., Getzenberg, R. H., and Schoen, R. E. (2005). Noninvasive Testing for Colorectal Cancer: A Review. Am. J. Gastroenterol. 100 (6), 1393–1403. doi:10.1111/j.1572-0241.2005.41427.x
Pan, P., Huang, Y. W., Oshima, K., Yearsley, M., Zhang, J., Yu, J., et al. (2018). Could Aspirin and Diets High in Fiber Act Synergistically to Reduce the Risk of Colon Cancer in Humans?. Int. J. Mol. Sci. 19, E166. doi:10.3390/ijms19010166
Pangeni, R., Choi, S. W., Jeon, O. C., Byun, Y., and Park, J. W. (2016). Multiple Nanoemulsion System for an Oral Combinational Delivery of Oxaliplatin and 5-fluorouracil: Preparation and In Vivo Evaluation. Int. J. Nanomed. 11, 6379–6399. doi:10.2147/ijn.s121114
Patil, Y. P., and Jadhav, S. (2014). Novel Methods for Liposome Preparation. Chem. Phys. Lipids 177, 8–18. doi:10.1016/j.chemphyslip.2013.10.011
Peng, J., Han, S., Chen, Z., Yang, J., Pei, Y., Bao, C., et al. (2020). Chaperone-Mediated Autophagy Regulates Apoptosis and the Proliferation of colon Carcinoma Cells. Biochem. Biophys. Res. Commun. 522, 348–354. doi:10.1016/j.bbrc.2019.11.081
Pericleous, P., Gazouli, M. L., Lyberopoulou, A., Rizos, S., Nikiteas, N., and Efstathopoulos, E. P. (2012). Quantum Dot Should Promise for Early Cancer Imaging and Detection. Int. J. Cancer 131 (3), 519–552. doi:10.1002/ijc.27528
Pesta, M., Kulda, V., Narsanska, A., Fichtl, J., and Topolcan, O. (2016). May CTC Technologies Promote Better Cancer Management? EPMA J. 6 (1), 1. doi:10.1186/s13167-014-0023-x
Pickhardt, P. J. (2013). Missed Lesions at CT Colonography: Lessons Learned. Abdom. Imaging 38 (1), 82–97. doi:10.1007/s00261-012-9897-z
Pillai, G. (2014). Nanomedicines for Cancer Therapy: An Update of FDA Approved and Those under Various Stages of Development. SOJ Pharm. Pharm. Sci. 1 (2), 13. doi:10.15226/2374-6866/1/2/00109
Pissuwan, D., Camilla, G., Mongkolsuk, S., and Cortie, M. B. (2019). Single and Multiple Detections of Foodborne Pathogens by Gold Nanoparticle Assays. Wires Nanomed. Nanobiotechnol. 12, 1584. doi:10.1002/wnan.1584
Prabhu, S., Kanthamneni, N., and Wang, J. (2007). Synergistic Chemoprevention of Colorectal Cancer Using Colon-Targeted Polymer Nanoparticles. Cancer Res. 67, 1.
Qaseem, A., Crandall, C. J., and Mustafa, R. A. (2019). Screening for Colorectal Cancer in Asymptomatic Average-Risk Adults: A Guidance Statement from the American College of Physicians. Ann. Intern. Med. 171 (9), 643–654. doi:10.7326/m19-0642
Radhakrishnan, K., Gupta, S., Gnanadhas, D. P., Ramamurthy, P. C., Chakravortty, D., and Raichur, A. M. (2013). Protamine-Capped Mesoporous Silica Nanoparticles for Biologically Triggered Drug Release. Part. Syst. Char 31 (4), 449–458. doi:10.1002/ppsc.201300219
Ramalingam, V. (2019). Multifunctionality of Gold Nanoparticles: Plausible and Convincing Properties. Adv. Colloid Int. Sci. 271, 101989. doi:10.1016/j.cis.2019.101989
Ramalingam, V., Rajaram, R., Premkumar, C., Santhanam, P., Dhinesh, P., Vinothkumar, S., et al. (2014). Biosynthesis of Silver Nanoparticles from Deep Sea Bacterium Pseudomonas aeruginosa JQ989348 for Antimicrobial, Antibiofilm, and Cytotoxic Activity. J. Basic Microbiol. 54, 928–936. doi:10.1002/jobm.201300514
Rampado, R., Crotti, S., Caliceti, P., Pucciarelli, S., and Agostini, M. (2019). Nanovectors Design for Theranostic Applications in Colorectal Cancer. J. Oncol. 2019, 2740923. doi:10.1155/2019/2740923
Rastogi, V., Yadav, P., Bhattacharya, S. S., Mishra, A. K., Verma, N., Verma, A., et al. (2014). Carbon Nanotubes: An Emerging Drug Carrier for Targeting Cancer Cells. J. Drug. Deliv. 2014, 1–23. doi:10.1155/2014/670815
Rivera, E. (2003). Liposomal Anthracyclines in Metastatic Breast Cancer: Clinical Update. Oncologist 8 (Suppl. 2), 3–9. doi:10.1634/theoncologist.8-suppl_2-3
Rosado-de-Castro, P. H., Morales, M. D. P., Pimentel-Coelho, P. M., Mendez-Otero, R., and Herranz, F. (2018). Development and Application of Nanoparticles in Biomedical Imaging. Contrast Media Mol. Imaging 2018, 1403826. doi:10.1155/2018/1403826
Safwat, M. A., Soliman, G. M., Sayed, D., and Attia, M. A. (2016). Gold Nanoparticles Enhance 5-Fluorouracil Anticancer Efficacy against Colorectal Cancer Cells. Int. J. Pharm. 513 (1–2), 648–658. doi:10.1016/j.ijpharm.2016.09.076
Sah, H., Thoma, L. A., Desu, H. R., Sah, E., and Wood, G. C. (2013). Concepts and Practices Used to Develop Functional PLGA-Based Nanoparticulate Systems. Int. J. Nanomedicine 8, 747–765. doi:10.2147/ijn.s40579
SanojRejinold, N., Thomas, R. G., Muthiah, M., Chennazhi, K. P., Manzoor, K., Park, I. K., et al. (2015). Anti-cancer, Pharmacokinetics and Tumor Localization Studies of pH-, RF- and Thermo-Responsive Nanoparticles. Int. J. Biol.Macromol. 74, 249–262. doi:10.1016/j.ijbiomac.2014.11.044
Schoen, R. E., Pinsky, P. F., and Weissfeld, J. L. (2012). Colorectal-Cancer Incidence and Mortality with Screening Flexible sigmoidoscopy. New Engl. J. Med. 366 (25), 2345–2357. doi:10.1056/nejmoa1114635
Schroy, P. C., Duhovic, E., Chen, C. A., and etal, (2016). Risk Stratification and Shared Decision Making for Colorectal Cancer Screening: A Randomized Controlled Trial. Med. Decis. Making 36 (4), 526–535. doi:10.1177/0272989x15625622
Segnan, N., Armaroli, P., and Bonelli, L. (2011). Once-Only Sigmoidoscopy in Colorectal Cancer Screening: Follow-Up Findings of the Italian Randomized Controlled Trial-SCORE. J. Natl. Cancer Inst. 103 (17), 1310–1322. doi:10.1093/jnci/djr284
Sesarman, A., Tefas, L., Sylvester, B., Licarete, E., Rauca, V., Luput, L., et al. (2018). Anti-Angiogenic and Anti-Inflammatory Effects of Long-Circulating Liposomes Co-encapsulating Curcumin and Doxorubicin on C26 Murine Colon Cancer Cells. Pharmacol. Rep. 70, 331–339. doi:10.1016/j.pharep.2017.10.004
Shapiro, J. A., Bobo, J. K., and Church, T. R. (2017). A Comparison of Fecal Immunochemical and High-Sensitivity Guaiactests for Colorectal Cancer Screening. Am. J. Gastroenterol. 112 (11), 1728–1735. doi:10.1038/ajg.2017.285
Siddique, S., and Chow, J. C. L. (2020). Application of Nanomaterials in Biomedical Imaging and Cancer Therapy. Nanomaterials 10 (9), 1700. doi:10.3390/nano10091700
Siddique, S., and Chow, J. C. L. (2020). Gold Nanoparticles for Drug Delivery and Cancer Therapy. Appl. Sci. 10, 3824. doi:10.3390/app10113824
Silva, R., Ferreira, H., and Cavaco-Paulo, A. (2011). Sonoproduction of Liposomes and Protein Particles as Templates for Delivery Purposes. Biomacromolecules 12 (10), 3353–3368. doi:10.1021/bm200658b
Simonet, B. M., and Valcárcel, M. (2009). Monitoring Nanoparticles in the Environment. Anal. Bioanal. Chem. 393 (1), 17–21. doi:10.1007/s00216-008-2484-z
Singhana, B., Slattery, P., and Melancon, M. P. (2015). “Targeted Gold Nanoshells,” in Applications of Nanoscience in Photomedicine. Editors M. R. Hamblin, and P. Avci (Oxfordshire, United Kingdom: Woodhead Publishing Limited-Chandos Publishing), 267–290. doi:10.1533/9781908818782.267
Solomon, S. D., Mcmurray, J. J., Pfeffer, M. A., Wittes, J., Fowler, R., Finn, P., et al. (2005). Cardiovascular Risk Associated with Celecoxib in a Clinical Trial for Colorectal Adenoma Prevention. N. Engl. J. Med. 352, 1071–1080. doi:10.1056/NEJMoa050405
Sounderya, N., and Zhang, Y. (2008). Use of Core/Shell Structured Nanoparticles for Biomedical Applications. Recent Pat Biomed. Eng. 1 (1), 34–42. doi:10.2174/1874764710801010034
Stanciu, L., Won, Y-H., Ganesana, M., and Andreescu, S. (2009). Magnetic Particle-Based Hybrid Platforms for Bioanalytical Sensors. Sensors 9 (4), 2976–2999. doi:10.3390/s90402976
Stang, J., Haynes, M., Carson, P., and Moghaddam, M. (2012). A Preclinical System Prototype for Focused Microwave Thermal Therapy of the Breast. IEEE Trans. Biomed. Eng. 59 (9), 2431–2438. doi:10.1109/tbme.2012.2199492
Sun, Y., Liu, Y., and Cogdell, D. (2016). Examining Plasma microRNA Markers for Colorectal Cancer at Different Stages. Oncotarget 7 (10), 11434–11449. doi:10.18632/oncotarget.7196
Suntres, Z. E. (2011). Liposomal Antioxidants for Protection against Oxidant-Induced Damage. J. Toxicol. 2011, 1–16. doi:10.1155/2011/152474
Tan, A., Davey, A. K., and Prestidge, C. A. (2011). Silica-Lipid Hybrid (SLH) Versus Non-Lipid Formulations for Optimising the Dose-dependent Oral Absorption of Celecoxib. Pharm. Res. 28, 2273–2287. doi:10.1007/s11095-011-0458-x
Thomasset, S. C., Berry, D. P., Garcea, G., Marczylo, T., Steward, W. P., and Gescher, A. J. (2007). Dietary Polyphenolic Phytochemicals–Promising Cancer Chemopreventive Agents in Humans? A Review of Their Clinical Properties. Int. J. Cancer 120, 451–458. doi:10.1002/ijc.22419
Tummala, S., Kumar, S. M. N., and Prakash, A. (2015). Formulation and Characterization of 5-Fluorouracil Enteric Coated Nanoparticles for Sustainedand Localized Release in Treating Colorectal Cancer. Saudi Pharm. J. 23 (3), 308–314. doi:10.1016/j.jsps.2014.11.010
Umezawa, S., Higurashi, T., Komiya, Y., Arimoto, J., Horita, N., Kaneko, T., et al. (2019). Chemoprevention of Colorectal Cancer: Past, Present, and Future. Cancer Sci. 110, 3018–3026. doi:10.1111/cas.14149
Upendra, L., Minakshi, P., Brar, B., Guray, M., and Ranjan, K. (2016). Nanodiagnostics: a New Frontier for Veterinary and Medical Sciences. J. Exp. Biol. Agric. Sci. 4 (3S), 307–318.
US National Institute of Health (2016). ClinicalTrial.gov. Available at: https://clinicaltrials.gov/.
U.S. Preventive Services Task Force (2016). Aspirin Use to Prevent Cardiovascular Disease and Colorectal Cancer: Preventive Medication. Available at: https://www.uspreventiveservicestaskforce.org/Page/Document/Recommendation Statement Final/aspirin-to-preventcardiovascular-disease-and-cancer.
Wang, S., Li, W., Yuan, D., Song, J., and Fang, J. (2016). Quantitative Detection of the Tumor-Associated Antigen Large External Antigen in Colorectal Cancertissues and Cells Using Quantum Dot Probe. Int. J. Nanomedicine 12, 235–247. doi:10.2147/IJN.S97509
Wang, Y., Li, Y., Wang, T., Gu, J., Zhao, J., and Pan, Z. (2015). Detection of AKR1B10 in Peripheral Blood by Anti-akr1b10-Conjugated CdTe/CdS Quantum Dots. Clin. Lab. 61 (9), 1267–1274. doi:10.7754/clin.lab.2015.150203
Wang, Z. H., Liu, J. M., Li, C. Y., Wang, D., Lv, H., Lv, S. W., et al. (2019). Bacterial Biofilm Bioinspired Persistent Luminescence Nanoparticles with Gut-Oriented Drug Delivery for Colorectal Cancer Imaging and Chemotherapy. ACS Appl. Mater. Inter. 11 (40), 36409–36419. doi:10.1021/acsami.9b12853
Wei, L., Hu, N., and Zhang, Y. (2010). Synthesis of Polymer Mesoporous Silica Nanocomposites. Materials 3 (7), 4066–4079. doi:10.3390/ma3074066
Wong, K. E., Ngai, S. C., Chan, K.-G., Lee, L.-H., Goh, B.-H., and Chuah, L.-H. (2019). Curcumin Nano-Formulations for Colorectal Cancer: A Review. Front. Pharmacol. 10, 152. doi:10.3389/fphar.2019.00152
Wu, C., Zhang, Y., Yang, D., Zhang, J., Ma, J., Cheng, D., et al. (2019). Novel SN38 Derivative-Based Liposome as Anticancer Prodrug: an In Vitro and In Vivo Study. Int. J. Nanomedicine 14, 75–85. doi:10.2147/IJN.S187906
Wu, L. P., Ficker, M., Christensen, J. B., Trohopoulos, P. N., and Moghimi, S. M. (2015). Dendrimers in Medicine: Therapeutic Concepts and Pharmaceutical Challenges. Bioconjug. Chem. 26 (7), 1198–1211. doi:10.1021/acs.bioconjchem.5b00031
Wu, S. Y., Huang, Y. J., Tzeng, Y. M., Huang, C. F., Hsiao, M., Wu, A. T. H., et al. (2018). Destruxin B Suppresses Drug-Resistant Colon Tumorigenesis and Stemness Is Associated with the Upregulation of miR-214 and Downregulation of mTOR/β-Catenin Pathway. Cancers 10 (10), 353. doi:10.3390/cancers10100353
Xiao, B., Si, X., Han, M. K., Viennois, E., Zhang, M., and Merlin, D. (2015). Codelivery of Camptothecin and Curcumin by Cationic Polymeric Nanoparticles for Synergistic Colon Cancer Combination Chemotherapy. J. Mater. Chem. B 3, 7724–7733. doi:10.1039/C5TB01245G
Xiao, B., Zhang, M., Viennois, E., Zhang, Y., Wei, N., Baker, M. T., et al. (2015). Inhibition of MDR1 Gene Expression and Enhancing Cellular Uptake for Effective colon Cancer Treatment Using Dual-Surface-Functionalized Nanoparticles. Biomaterials 48, 147–160. doi:10.1016/j.biomaterials.2015.01.014
Xiao, T., Huang, J., Wang, D., Meng, T., and Yang, X. (2019). Au and Au-Based Nanomaterials: Synthesis and Recent Progress in Electrochemical Sensor Applications. Talanta 206, 120210. doi:10.1016/j.talanta.2019.120210
Xie, J., Gao, Y., Zhao, R., Sinko, P. J., Gu, S., Wang, J., et al. (2015). Ex Vivo and In Vivo Capture and Deactivation of Circulating Tumor Cells by Dual-Antibody-Coated Nanomaterials. J. Control. Release 209, 159–169. doi:10.1016/j.jconrel.2015.04.036
Xie, J., Wang, J., and Chen, H. (2015b). Multivalent Conjugation of Antibody to Dendrimers for the Enhanced Capture and Regulation on colon Cancer Cells. Sci. Rep. 5, 9445. doi:10.1038/srep09445
Xie, J., Zhao, R., and Gu, S. (2014). The Architecture and Biological Function of Dual Antibody-Coated Dendrimers: Enhanced Control of Circulating Tumor Cells and Their Hetero-Adhesion to Endothelial Cells for Metastasis Prevention. Theranostics 4 (12), 1250–1263. doi:10.7150/thno.8775
Xie, Y. H., Chen, Y. X., and Fang, J. Y. (2020). Comprehensive Review of Targeted Therapy for Colorectal Cancer. Signal Transduct. Target. Ther. 5, 22. doi:10.1038/s41392-020-0116-z
Xing, X., Zhang, B., Wang, X., Liu, F., Shi, D., and Cheng, Y. (2015). An “Imaging-Biopsy” Strategy for Colorectal Tumor Reconfirmation by Multipurposeparamagnetic Quantum Dots. Biomaterials 48, 16–25. doi:10.1016/j.biomaterials.2015.01.011
Xynos, I. D., Kavantzas, N., and etal, Tsaousi. S. (2013). Factors Influencing Survival in Stage IV Colorectal Cancer: the Influence of DNA Ploidy. ISRN Gastroenterol. 2013, 490578. doi:10.1155/2013/490578
Yallapu, M. M., Nagesh, P. K. B., Jaggi, M., and Chauhan, S. C. (2015). Therapeutic Applications of Curcumin Nano-Formulations. AAPS J. 17 (6), 1341–1356. doi:10.1208/s12248-015-9811-z
Yang, C. C., Yang, S. Y., Ho, C. S., Chang, J. F., Liu, B. H., and Huang, K. W. (2014). Development of Antibody Functionalized Magnetic Nanoparticles for the Immunoassay of Carcinoembryonic Antigen: a Feasibility Study for Clinical Use. J. Nanobiotechnology 12, 44. doi:10.1186/s12951-014-0044-6
Young, P. E., Womeldorph, C. M., and Johnson, E. K. (2014). Early Detection of Colorectal Cancer Recurrence in Patients Undergoing Surgery With Curative Intent: Current Status and Challenges. J. Cancer 5 (4), 262–271. doi:10.7150/jca.7988
Yu, M., Jambhrunkar, S., Thorn, P., Chen, J., Gu, W., and Yu, C. (2013). Hyaluronic Acid Modified Mesoporous Silica Nanoparticles for Targeted Drug Delivery to CD44-Overexpressing Cancer Cells. Nanoscale 5 (1), 178–183. doi:10.1039/c2nr32145a
Zakaria, A. B., Picaud, F., Rattier, T., Pudlo, M., Dufour, F., Saviot, L., et al. (2015). Nanovectorization of TRAIL with Single wall Carbon Nanotubes Enhances Tumor Cell Killing. Nano Lett. 15 (2), 891–895. doi:10.1021/nl503565t
Zeng, W.-J., Peng, C.-W., Yuan, J.-P., Cui, R., and Li, Y. (2015). Quantum Dot-Based Multiplexed Imaging in Malignant Ascites: A New Model for Malignant Ascites Classification. Int. J. Nanomedicine 10, 1759–1768. doi:10.2147/IJN.S70228
Zhang, W., Wang, C., Guan, L., Peng, M., Li, K., and Lin, Y. (2019). A Non-Enzymatic Electrochemical Biosensor Based on Au@PBA(Ni-Fe): MoS2 Nanocubes for Stable and Sensitive Detection of Hydrogen Peroxide Released from Living Cells. J. Mater. Chem. B 7, 7704–7712. doi:10.1039/c9tb02059d
Zhang, Z., Ji, H., Song, Y., Zhang, S., Wang, M., Jia, C., et al. (2017). Fe(III)-Based Metal-Organic Framework-Derived Core-Shell Nanostructure: Sensitive Platform for High Trace Determination of Heavy Metal Ions. Biosens. Bioelectron. 94, 358–364. doi:10.1016/j.bios.2017.03.014
Zhao, X., Pan, J., Li, W., Yang, W., Qin, L., and Pan, Y. (2018). Gold Nanoparticles Enhance Cisplatin Delivery and Potentiate Chemotherapy by Decompressing Colorectal Cancer Vessels. Int. J. Nanomed. 13, 6207–6221. doi:10.2147/ijn.s176928
Zheng, Y., You, X., Guan, S., Huang, J., Wang, L., Zhang, J., et al. (2019). Poly(Ferulic Acid) with an Anticancer Effect as a Drug Nanocarrier for Enhanced Colon Cancer Therapy. Adv. Funct. Mater. 29 (15), 1808646. doi:10.1002/adfm.201808646
Zhou, H. S., Sasahara, H., Honma, I., Komiyama, H., and Haus, J. W. (1994). Coated Semiconductor Nanoparticles: The CdS/PbS System’s Photoluminescence Properties. Chem. Mater. 6 (9), 1534–1541. doi:10.1021/cm00045a010
Zhou, M., Peng, Z., Liao, S., Li, P., and Li, S. (2014). Design of Microencapsulated Carbon Nanotube-Based Microspheres and its Application in colon Targeted Drug Delivery. Drug. Deliv. 21 (2), 101–109. doi:10.3109/10717544.2013.834413
Zhu, Q., Liang, B., Liang, Y., Ji, L., Cai, Y., Wu, K., et al. (2020). 3D Bimetallic Au/Pt Nanoflowers Decorated Needle-type Microelectrode for Direct In Situ Monitoring of ATP Secreted from Living Cells. Biosens. Bioelectron 153, 112019. doi:10.1016/j.bios.2020.112019
Zielińska, A., Carreiró, F., Oliveira, A. M., Neves, A., Pires, B., Venkatesh, D. N., et al. (2020). Polymeric Nanoparticles: Production, Characterization, Toxicology and Ecotoxicology. Molecules 25 (16), 3731. doi:10.3390/molecules25163731
Keywords: colorectal cancer, nanotechnology, detection, treatment, targeted therapy
Citation: Brar B, Ranjan K, Palria A, Kumar R, Ghosh M, Sihag S and Minakshi P (2021) Nanotechnology in Colorectal Cancer for Precision Diagnosis and Therapy. Front. Nanotechnol. 3:699266. doi: 10.3389/fnano.2021.699266
Received: 23 April 2021; Accepted: 13 August 2021;
Published: 16 September 2021.
Edited by:
Christiane Pienna Soares, Sao Paulo State University, BrazilReviewed by:
Luciana Dini, Sapienza University of Rome, ItalyJames Chow, University of Toronto, Canada
Copyright © 2021 Brar, Ranjan, Palria, Kumar, Ghosh, Sihag and Minakshi. This is an open-access article distributed under the terms of the Creative Commons Attribution License (CC BY). The use, distribution or reproduction in other forums is permitted, provided the original author(s) and the copyright owner(s) are credited and that the original publication in this journal is cited, in accordance with accepted academic practice. No use, distribution or reproduction is permitted which does not comply with these terms.
*Correspondence: Basanti Brar, YmFzYW50aWJyYXJAZ21haWwuY29t; Prasad Minakshi, bWluYWtzaGkuYWJ0QGx1dmFzLmVkdS5pbg==