- 1Electronic Information School, Wuhan University, Wuhan, China
- 2Peng Cheng Laboratory, Shenzhen, China
- 3Wuhan Institute of Quantum Technology, Wuhan, China
- 4Hubei Luojia Laboratory, Wuhan, China
Metasurfaces provide a novel platform for multifunctional devices due to their incomparable competence for the manipulation of different optical properties. Recently, many works have emerged to merge distinct functions into a single metasurface, which effectively increase the information density and capacity of meta-devices. In this work, combining the dual-wavelength polarizer and the orientation degeneracy of the Malus law, we further exploit the design degree of freedom of the metasurface, and realize color control and phase manipulation simultaneously with single-sized nanostructures. We experimentally demonstrate our concept by integrating the function of pseudo-color nanoprinting and holographic image display together. Our research can effectively improve the functionalities of metasurface and promote advanced research of multimode displays, information encryption, optical multiplexing, and many other related fields.
1 Introduction
Benefiting from the incomparable capability of manipulation for optical properties such as phase (Hao et al., 2021; Wang et al., 2021; Zhang H. et al., 2022; Cheng et al., 2022; Ma et al., 2022; Ming et al., 2022; Tao et al., 2022), polarization (Ouyang et al., 2021; Xu et al., 2021; Deng J. et al., 2022; Zhang S. et al., 2022; Kim et al., 2022; Zhou et al., 2022) and amplitude (Li Z. et al., 2021; Ren et al., 2021; Zheng et al., 2021; Deng L. et al., 2022; Chen et al., 2022; Yang et al., 2022), metasurface has emerged as a powerful platform for implementing multifunctional devices (Deng J. et al., 2020; Deng L. et al., 2020; Chen et al., 2020; Dai et al., 2020; Li et al., 2020; Li J. et al., 2021). By dividing the metasurface into different parts, the segmenting metasurfaces have showcased their ability to integrate different functions, e.g., imaging and nanoprinting (Chen et al., 2020) or near- and far-field display together (Dai et al., 2020). However, these works simply hybrid the single-function by spaces and sacrifice the device size to increase the functionality, in which the information density is not increased in essential. Another attempt to realize multifunction is employing the nanostructure with distinct dimensions, i.e., combining the geometric and propagation phase to realize different functions (Li et al., 2020; Li J. et al., 2021), which decreases the crosstalk between different functions and improves the information density. Nevertheless, the complicated design and fabrication process hamper the wide applicability of the metasurface. To settle this issue, the orientation degeneracy hidden in the Malus law is proposed and the multimode gray-scale image display is realized (Deng J. et al., 2020; Deng L. et al., 2020). Such approaches, however, only work for one single wavelength and the chromatic images display is still elusive.
Herein, we employ the dual-wavelength polarizer and successfully achieve the Fourier holographic and pseudo-color nanoprinting images display based on single-sized nanostructures, as shown in Figure 1. Specifically, the proposed metasurface works as polarizer arrays at two distinct wavelengths (i.e., 510 and 620 nm) with orthogonal polarization direction, and the mixture of these two colors with different ratio can be realized merely by rotating the orientation angle of the nanostructure. More importantly, employing the orientation degeneracy, the phase manipulation can be simultaneously implemented by selecting the nanostructure orientations through the simulated annealing algorithm (SAA) (Deng L. et al., 2020), without influencing the color modulation. As a result, the pseudo-color control and phase manipulation can be fulfilled at the same time. The present research provides an exciting opportunity to advance our knowledge of multifunctional devices based on a single-sized metasurface, and can make major contribution to fields such as image display, optical anticounterfeiting, information encryption, and etc.
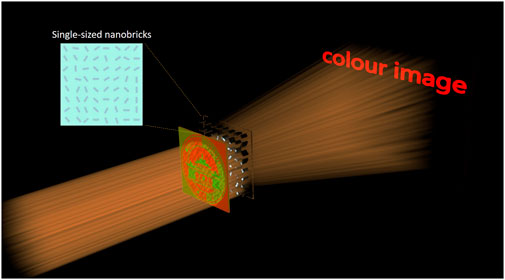
FIGURE 1. Schematic illustration of the single-sized metasurface for pseudo-color control and holographic image display. The metasurface can display a pseudo-color nanoprinting in the near-field under the illumination of incident beam with red (620 nm) and green (510 nm) light, and a Fourier holographic image in the far-field when the wavelength of incident beam is 620 or 510 nm.
2 Materials and methods
2.1 Working principle for simultaneous color control and phase manipulation
To realize pseudo-color control with the single-sized nanostructures, each unit-cell of the metasurface is supposed to modulate the polarization direction of incident beam at two wavelengths, i.e., each nanostructure acts as dual-wavelength nano-polarizer. Herein we employ the silver nanobricks sitting on the fused silica to implement the proposed function. Figures 2A, B show the diagram of a unit-cell, in which the orientation angle θ is defined as the angle between the x-axis and the long axis of nanobrick. Here, CST Microwave Studio software is used to simulate the optical response of the nanobricks. After careful optimization, each nanobrick is designed with length L of 140 nm, width W of 85 nm, unit-cell dimension CS of 340 nm, and H of 70 nm. Figure 2C shows the simulated reflectivities and transmissivities with incident beam polarized along the long (rl and tl) and short (rs and ts) axes of the nanobrick. We can see that at the working wavelength of 510 nm, rs reaches 69.93% and ts is 3.98%; while at the working wavelength of 620 nm, rl reaches 90.9% and tl is 0.3%, which demonstrates that the nanobrick works as polarizer at two designed wavelengths. According to the Malus law, when the incident light beam polarized along the x-axis and with wavelength of 510 nm or 620 nm passes through the nanobrick, the transmitted beam’s intensity can be expresses as:
or
where I0 represents the intensity of the incident beam, I510 and I620 are the intensity of the output light at wavelength of 510 and 620 nm, respectively. As a result, when the incident beam is with two wavelengths of 510 and 620 nm, the ratio of the red (620 nm) and green (510 nm) components I620/510 in the output beam can be adjusted by changing the orientation angle of the nanobrick:
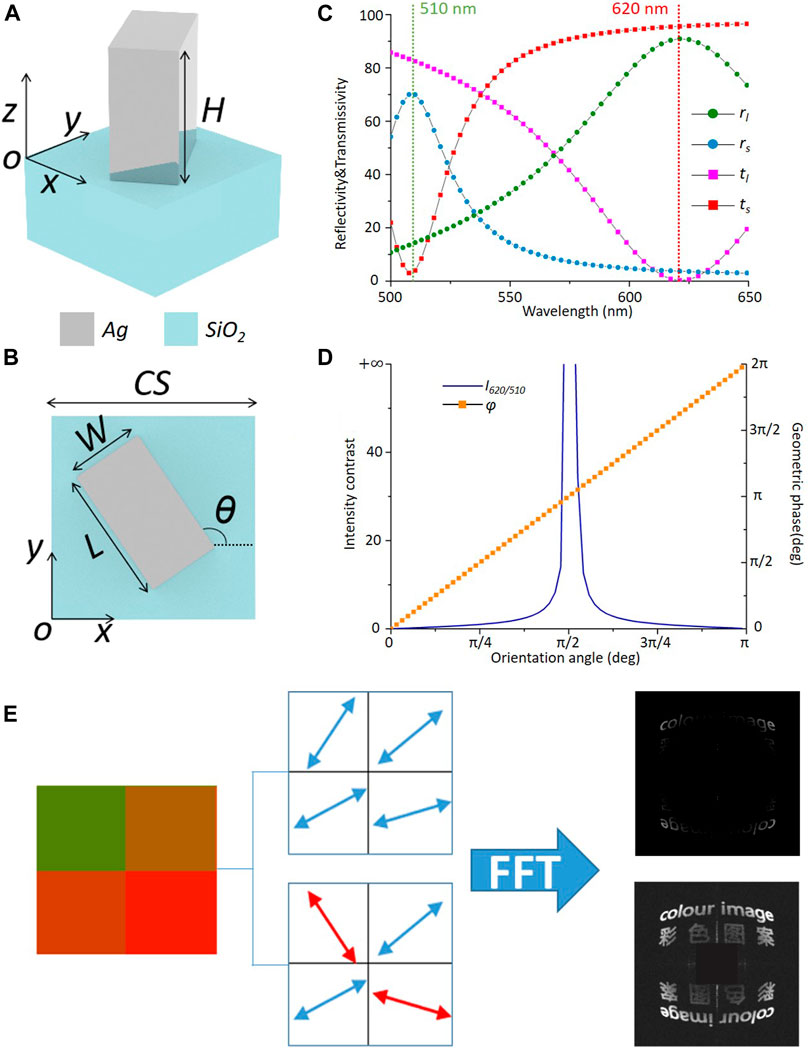
FIGURE 2. (A) The space and (B) top views of a unit-cell consisting of Ag nanobrick and SiO2 substrate. (C) Simulated reflectivity and transmissivity of the nanobricks when illuminated by light polarized along the long (rl, tl) and short axes (rs, ts), which shows that the nanobrick works as a dual-wavelength polarizer with orthogonal polarization direction at the wavelengths of 510 and 620 nm. (D) Calculated intensity contrast (I620/510) and geometric phase φ as the function of orientation angle θ, from which we can see that there are always two orientation angles to produce the same pseudo-color but different geometric phase. (E) The optimization process to determine the orientation arrays. There are different orientation arrays producing the same pseudo-color nanoprinting, the FFT to which will obtain holographic images with different correlation coefficient. And the SAA is employed to select the orientation angle pixel by pixel until the correlation coefficient finally satisfies the requirement.
Consequently, the color between red and green can be modulated, and this is the basic principle of pseudo-color manipulation.
Further, due to the orientation degeneracy of the trigonometric function, each nanobrick has two angles (θ or π-θ) to generate the same output color, as shown in Figure 2D of the blue line. And it’s known that when the circularly polarized light passes through an anisotropic structure, the output light with opposite chirality will carry the geometric phase
The SAA is employed to select the orientation angle of the nanobrick. As shown in Figure 2E, for one pixel of the nanobrick arrays, there are two angles generating the same color, which is presented by the blue and red arrows, respectively. And these two angle arrays will generate PB phase with different value in the above-mentioned pixel. Subsequently, the fast Fourier transform (FFT) to the PB phase will obtain different calculated images, and we employ the correlation coefficient between the calculated and target images to select the orientation angle of a single nanobrick, the definition of which is given as:
where I and M represent the calculated and target image,
2.2 Sample fabrication
Firstly, we employ the spin coater to spin and coat a polymethyl methacrylate (PMMA) film and a conducting layer on the SiO2 substrate. Subsequently, the electron beam lithography (EBL) is utilized to pattern the nanobrick structures. Then the conducting layer is washed away by the deionized water, and the exposed resist is developed in a mixture of methyl isobutyl ketone (MIBK) and isopropyl alcohol (IPA) with the ratio of 1/3 for 80 s, dipped in IPA for 30 s and blown dry by the nitrogen. Next, electron beam evaporator is employed to deposit a layer of chromium (3 nm) to increase the adhesion and a 70 nm silver film. Finally, we obtain the designed silver metasurface structure through lift-off process in hot acetone with temperature of 85°C.
3 Results
To demonstrate our concept, we design and fabricate a metasurface with 500 × 500 pixels (150 × 150 μm) to implement the near-field pseudo-color encoding and the far-field Fourier holographic images display. Firstly, the function of pseudo-color image display is characterized. Figure 3A displays the optical microscope (Motic BA310Met) for observing the nanoprinting image. In the experimental process, a polarizer is employed to decode the image right at the metasurface plane. Figure 3B shows the captured transmitted image when a polarizer with polarization angle of 0° is placed before the metasurface, which possesses ultra-high resolution of 75 k dpi (dots per inch) and the scale bar is 30 μm. We can see the images with color changing from red to green clearly, which demonstrates that the proposed metasurface can realize pseudo-color control merely by changing the orientation angle of the single-sized nanobrick.
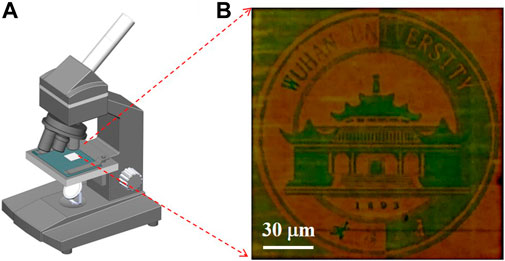
FIGURE 3. (A) The diagram of the optical microscope for observing the pseudo-color image recording by the metasurface. (B) The captured images when a polarizer with polarization directions of 0° is inserted before the metasurface. Scale bar: 30 μm.
Next, the function of the far-field holographic image display is demonstrated. As shown in Figure 4A of the optical setup, the light source is a linearly polarized super-continuum laser (YSL SC-pro), which is modulated to be LCP by a quarter-wave plate (QWP) after passing through an iris, and the far-field holographic image can be observed after the metasurface being illuminated by the incident LCP light. The inset is the scanning electron microscope (SEM) image of the nanobricks, the scale bar of which is 600 nm. The experimentally observed images at the working wavelengths of 510 and 620 nm are shown in Figures 4B,C, respectively, from which we can recognize the words clearly. Because the correction of geometric distortion is performed at the wavelength of 620 nm, the captured image at 510 nm shows slight distortion. These experiment results effectively prove that the metasurface can display a pseudo-color nanoprinting in the near-field and a Fournier holographic image in the far-field.
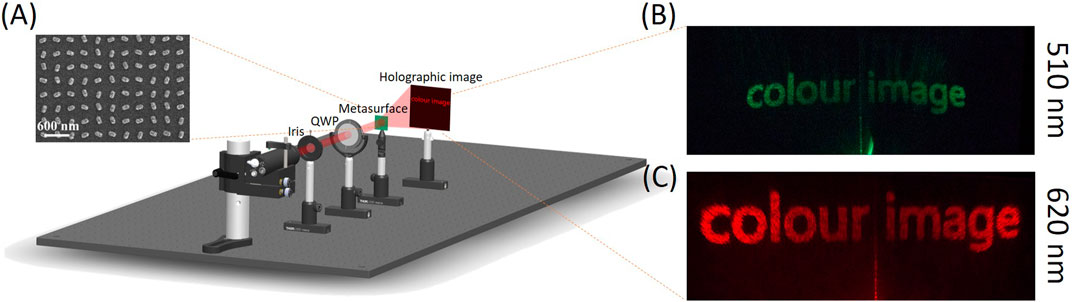
FIGURE 4. (A) Schematic illustration of the optical setup to decode far-field holographic image. The light source is a linearly polarized super-continuum laser. A QWP before the metasurface is employed to modulate the laser beam to be LCP, and the transmitted holographic image can be obtained in the far-field when the incident beam illuminates the metasurface. The inset shows partial view of the SEM image of the metasurface with the scale bar of 600 nm. (B) Experimental Fourier holographic images under LCP light illumination at 510 nm and (C) 620 nm.
Subsequently, to explore the broadband characteristic of the metasurface, we change the wavelength of the incident light beam from 490 nm to 640 nm with a step of 30 nm. The experimentally captured holographic images are shown in Figures 5A–F, which display the designed words clearly although they show different sizes and geometric distortion versus the wavelength due to different diffraction angles. These experimental results prove that the proposed metasurface possesses broadband characteristic for displaying holographic images.
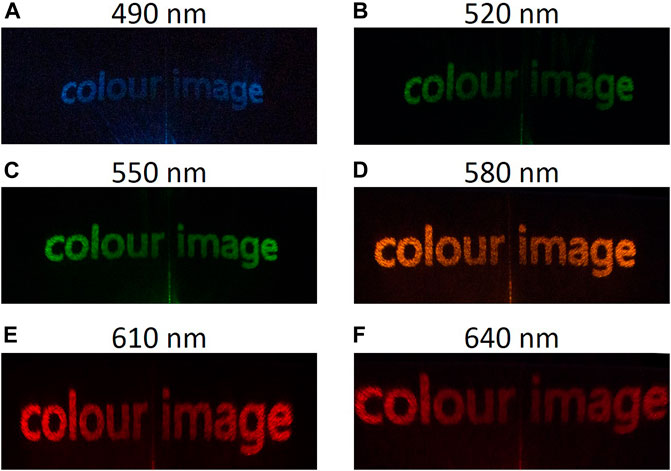
FIGURE 5. (A-F) Experimentally captured holographic meta-images from 490 to 640 nm with steps of 30 nm.
4 Discussion
In conclusion, combining the dual-wavelength polarizer and orientation degeneracy hidden in the Malus law, a general platform for simultaneous pseudo-color control and phase manipulation is proposed. The employment of the dual-wavelength polarizer effectively converts the gray scale to pseudo color. On this basis, the employment of the orientation degeneracy allows the metasurface to further control the phase of the output light, thus realizing the holographic image and chromatic nanoprinting image display at the same time. In addition, benefiting from the single-sized strategy, our work effectively simplifies the process of design and fabrication. This study provides new insights into the multifunctional devices design based on the metasurface, and can be applied in fields such as optical anticounterfeiting, image display, information encryption, and etc.
Data availability statement
The original contributions presented in the study are included in the article/supplementary material, further inquiries can be directed to the corresponding authors.
Author contributions
ZL and GZ proposed the idea and initiated the project. ZL and JL conducted the metasurface design and numerical simulations. JL fabricated the samples. ZZ and JL performed the experimental characterization. GZ and ZL analyzed the experimental data. GZ, ZL, JL, and ZZ co-wrote the paper. GZ and ZL supervised the project. The manuscript was discussed and corrected by all authors.
Funding
The authors would like to acknowledge the support from the National Key Research & Development Program of China (2021YFE0205800), National Natural Science Foundation of China (11904267, 12174292, 91950110), Natural Science Foundation of Hubei Province (ZRMS2021000211), and the Fundamental Research Funds for the Central Universities (2042021kf0018, 2042022kf0024, 2042022kf1013 and 2042022kf1011).
Conflict of interest
The authors declare that the research was conducted in the absence of any commercial or financial relationships that could be construed as a potential conflict of interest.
Publisher’s note
All claims expressed in this article are solely those of the authors and do not necessarily represent those of their affiliated organizations, or those of the publisher, the editors and the reviewers. Any product that may be evaluated in this article, or claim that may be made by its manufacturer, is not guaranteed or endorsed by the publisher.
References
Chen, K., Xu, C., Zhou, Z., Li, Z., Chen, P., and Zheng, G. (2022). Multifunctional liquid crystal device for grayscale pattern display and holography with tunable spectral-response. Laser Phot. Rev. 16, 2100591. doi:10.1002/lpor.202100591
Chen, R., Zhou, Y., Chen, W., Chen, R., Iqbal, N., and Ma, Y. (2020). Multifunctional metasurface: Coplanar embedded design for metalens and nanoprinted display. ACS Photonics 7, 1171. doi:10.1021/acsphotonics.9b01795
Cheng, J., Sha, X., Zhang, H., Chen, Q., Qu, G., Song, Q., et al. (2022). Ultracompact orbital angular momentum sorter on a CMOS chip. Nano Lett. 22, 3993. doi:10.1021/acs.nanolett.2c00572
Dai, Q., Guan, Z., Chang, S., Deng, L., Tao, J., Li, Zhong., et al. (2020). A single-celled tri-functional metasurface enabled with triple manipulations of light. Adv. Funct. Mat. 30, 2003990. doi:10.1002/adfm.202003990
Deng, J., Deng, L. G., Guan, Z., Tao, J., Li, G. F., Li, Z., et al. (2020a). Multiplexed anticounterfeiting meta-image displays with single-sized nanostructures. Nano Lett. 20, 1830. doi:10.1021/acs.nanolett.9b05053
Deng, J., Gao, F., Yuan, P., Li, Y., and Yan, B. (2022a). Bidirectional nanoprinting based on bilayer metasurfaces. Opt. Express 30, 448136. doi:10.1364/OE.448136
Deng, L., Deng, J., Guan, Z., Tao, J., Chen, Y., Yang, Y., et al. (2020b). Malus-metasurface-assisted polarization multiplexing. Light-Sci. Appl. 9, 101. doi:10.1038/s41377-020-0327-7
Deng, L., Li, Z., Zhou, Z., He, Z., Zeng, Y., Zheng, G., et al. (2022b). Bilayer-metasurface design, fabrication, and functionalization for full-space light manipulation. Adv. Opt. Mat. 10, 2102179. doi:10.1002/adom.202102179
Fu, R., Deng, L., Guan, Z., Chang, S., Tao, J., Li, Z., et al. (2020). Zero-order-free meta-holograms in a broadband visible range. Photonics Res. 8, 723. doi:10.1364/PRJ.387397
Hao, Z., Liu, W., Li, Z., Li, Z., Geng, G., Wang, Y., et al. (2021) Full complex-amplitude modulation of second harmonic generation with nonlinear metasurfaces. 15, 2100207. doi:10.1002/lpor.202100207
Kim, J., Jeon, D., Seong, J., Badloe, T., Jeon, N., Kim, G., et al. (2022). Photonic encryption platform via dual-band vectorial metaholograms in the ultraviolet and visible. ACS Nano 16, 3. doi:10.1021/acsnano.1c10100
Li, J., Wang, Y., Chen, C., Fu, R., Zhou, Z., Li, Z., et al. (2021a). From lingering to rift: Metasurface decoupling for near- and far-field functionalization. Adv. Mat. 33, 2007507. doi:10.1002/adma.202007507
Li, Z., Chen, C., Guan, Z., Tao, J., Chang, S., Dai, Q., et al. (2020). Three-Channel metasurfaces for simultaneous meta-holography and meta-nanoprinting: A single-cell design approach. Laser Phot. Rev. 14, 2000032. doi:10.1002/lpor.202000032
Li, Z., Dai, Q., Mehmood, M., Hu, G., Yanchuk, B., Tao, J., et al. (2018). Full-space cloud of random points with a scrambling metasurface. Light-Sci. Appl. 7, 63. doi:10.1038/s41377-018-0064-3
Li, Z., Deng, L., Deng, J., He, Z., Tao, J., Zheng, G., et al. (2021b). Metasurface-enabled three-in-one nanoprints by multifunctional manipulations of light. iScience 24, 103510. doi:10.1016/j.isci.2021.103510
Ma, Q., Gao, W., Xiao, Q., Ding, L., Gao, T., Zhou, Y., et al. (2022). Directly wireless communication of human minds via non-invasive brain-computer-metasurface platform. eLight 2, 11. doi:10.1186/s43593-022-00019-x
Ming, Y., Intaravanne, Y., Ahmed, H., Kenney, M., Lu, Y., and Chen, X. (2022). Creating composite vortex beams with a single geometric metasurface. Adv. Mat. 34, 2109714. doi:10.1002/adma.202109714
Ouyang, X., Xu, Y., Xian, M., Feng, Z., Zhu, L., Cao, Y., et al. (2021). Synthetic helical dichroism for six-dimensional optical orbital angular momentum multiplexing. Nat. Photonics 15, 901. doi:10.1038/s41566-021-00880-1
Ren, R., Li, Z., Deng, L., Shan, X., Dai, Q., Guan, Z. Q., et al. (2021). Non-orthogonal polarization multiplexed metasurfaces for tri-channel polychromatic image displays and information encryption. Nanophotonics 10, 2903. doi:10.1515/nanoph-2021-0259
Tao, J., You, Q., Li, Z., Luo, M., Liu, Z., Qiu, Y., et al. (2022). Mass-manufactured beam-steering metasurfaces for high-speed full-duplex optical wireless-broadcasting communications. Adv. Mat. 34, 2106080. doi:10.1002/adma.202106080
Wang, Y., Fan, Q., and Xu, T. (2021). Design of high efficiency achromatic metalens with large operation bandwidth using bilayer architecture. Opto-Electron. Adv. 4. 200008. doi:10.29026/oea.2021.200008
Xu, P., Tian, H., Jiang, W., Chen, Z., Cao, T., Qiu, C.-W., et al. (2021). Phase and polarization modulations using radiation-type metasurfaces. Adv. Opt. Mat. 9, 2100159. doi:10.1002/adom.202100159
Yang, B., Ma, D., Liu, W., Choi, D.-Y., Li, Z., Cheng, H., et al. (2022). Deep-learning-based colorimetric polarization-angle detection with metasurfaces. Optica 9, 217. doi:10.1364/OPTICA.449893
Zhang, H., Sha, X., Chen, Q., Cheng, J., Ji, Z., Song, Q., et al. (2022a). All‐dielectric metasurface‐enabled multiple vortex emissions. Adv. Mat. 34, 2109255. doi:10.1002/adma.202109255
Zhang, S., Huang, L., Geng, G., Li, J., Li, X., and Wang, Y. (2022b). Full-Stokes polarization transformations and time sequence metasurface holographic display. Photonics Res. 10, 1031. doi:10.1364/PRJ.450354
Zheng, P., Dai, Q., Li, Z., Ye, Z., Xiong, J., Liu, H., et al. (2021). Metasurface-based key for computational imaging encryption. Sci. Adv. 7, eabg0363. doi:10.1126/sciadv.abg0363
Zheng, P., Li, J., Li, Z., Ge, M., Zhang, S., Zheng, G., et al. (2022). Compressive imaging encryption with secret sharing metasurfaces. Adv. Opt. Mat. 2200257. doi:10.1002/adom.202200257
Keywords: metasurface, single size, multifunctional device, color control, dual-wavelength polarizer
Citation: Li J, Zhou Z, Li Z and Zheng G (2022) Single-sized metasurface for simultaneous pseudo-color nanoprinting and holographic image display. Front. Nanotechnol. 4:973348. doi: 10.3389/fnano.2022.973348
Received: 20 June 2022; Accepted: 26 July 2022;
Published: 17 August 2022.
Edited by:
Georgios Veronis, Louisiana State University, United StatesCopyright © 2022 Li, Zhou, Li and Zheng. This is an open-access article distributed under the terms of the Creative Commons Attribution License (CC BY). The use, distribution or reproduction in other forums is permitted, provided the original author(s) and the copyright owner(s) are credited and that the original publication in this journal is cited, in accordance with accepted academic practice. No use, distribution or reproduction is permitted which does not comply with these terms.
*Correspondence: Zile Li, bGl6aWxlQHdodS5lZHUuY24=; Guoxing Zheng, Z3h6aGVuZ0B3aHUuZWR1LmNu