- 1Laboratory for Zoonosis and Environmental Microbiology, Centre for Infectious Disease Control, National Institute of Public Health and the Environment (RIVM), Bilthoven, Netherlands
- 2Institute for Risk Assessment Sciences, Utrecht University, Utrecht, Netherlands
Micro- and nanoplastics (MNPs) are everywhere: in the air we breathe, in our food and in virtually every type of water. Currently, it is unknown whether, and to what extent, these MNPs are hazardous to human health. Identifying risks of physical and chemical aspects of MNPs has gained a lot of attention over the last few years, and efforts have been made to quantify these risks. In our opinion, the momentum delivered by these efforts should be used to highlight the relevance of including another important aspect of MNPs: their associated microbes. Although more and more studies describe MNP-associated microbes, the interplay between physical, chemical and microbiological aspects are lacking. Hazard identification parameters describing this interplay are crucial to risk assessment strategies, yet the majority of effort has been directed towards optimizing human exposure parameters. Here, we address the importance of including microbiological aspects of MNPs in the risk assessment of MNPs. Physical and chemical aspects of MNPs impact the diversity and abundance of microbes and antibiotic resistance genes (ARGs). In turn, environmental factors (e.g., UV radiation, antibiotics) may impact the microbial composition directly, or indirectly by impacting physical or chemical MNP aspects. Future efforts should be directed towards investigating this interplay in order to determine the effects of these dynamic outcomes on human health. We believe that elucidating these pieces of the puzzle is needed to ultimately perform a more holistic risk assessment of MNPs on human health.
1 Introduction
Plastic debris including microplastics (<5 mm, MPs) and nanoplastics (<1 μm, NPs) are ubiquitous in environmental compartments such as air, soil, water and certain foods (Vethaak and Legler, 2021). Humans are exposed to MNPs mainly via inhalation, ingestion or skin contact (WHO, 2019a). Although the European Union has adopted several proposals aimed at limiting intentional plastic pollution, 200–600 olympic size swimming pools of MPs are unintentionally released in the environment every year (European Comission, 2024). Due to their ubiquity, toxicity and ability to cover large distances in aquatic environments and in the atmosphere, MNPs are a human health concern (Vethaak and Legler, 2021). However, the (quantitative) risks of physical and chemical properties of MNPs on human health are unclear. Literature addresses the current limitations in MNP reference material, available analytical methodology and study comparability (Brachner et al., 2020) and highlights the effects of MNPs on environmental ecosystems (Sana et al., 2020) and human health (Gonzalez-Acedo et al., 2021; Yee et al., 2021; Gruber et al., 2023). Additionally, MNPs may be colonized by microbes, hereby potentially posing microbiological threats to human health (Loiseau and Sorci, 2022).
The human body is continuously exposed and home to microbes. Some microbes are beneficial to human health (e.g., Lactobacilli in the gut), while others are pathogenic and may cause respiratory infections (e.g., Legionella pneunomphila), gastrointestinal infections (e.g., noroviruses) or skin infections (e.g., Pseudomonas aeruginosa). Whether microbes are pathogenic depends on the levels and nature of their virulence factors and thus their ability to evade or inhibit host defense mechanisms. Infectious diseases caused by water- and airborne pathogenic microbes and antimicrobial resistance (AMR) are considered some of the most important threats to human health (WHO, 2019b), of which the latter one contributed to 4.95 million global deaths in 2019 (Antimicrobial Resistance Collaborators, 2022). Understanding the role that plastic debris plays in the spread of pathogenic microbes and AMR in environmental compartments is critical for quantification of the overall impact of aspects (physical, chemical and microbiological) of MNPs on human health.
Although an increasing number of studies assess the microbiological aspects of MNPs, information on the physical or chemical properties of MNPs are often lacking since no standardized material is used or since laboratory equipment, funding or expertise only allows for the detection of one of those MNP aspects. This results in only static microbiological parameters, while understanding the dependability between MNP aspects helps to understand and predict these microbiological parameters. Here, we provide perspective on how this dynamic interplay can be addressed. The parameters contributing to this interplay are, despite the increasing number of publications on the role of MNPs in the spreading of microbes via air, soil and foods, best characterized in aquatic environments. Notably, aquatic environments combine the presence of (pathogenic) microbes while facilitating long-distance transport of plastic debris and MNPs, hereby enabling particle dissemination and human exposure. First, we provide perspective on which different microbiological aspects of MNPs may impact human health (Figure 1). Next, we describe how physical (particle size) and chemical (polymer type) MNP properties affect MNP-associated microbes. Lastly, we describe how environmental factors (e.g., antibiotics and UV radiation) may affect these microbes directly or indirectly, via MNPs.
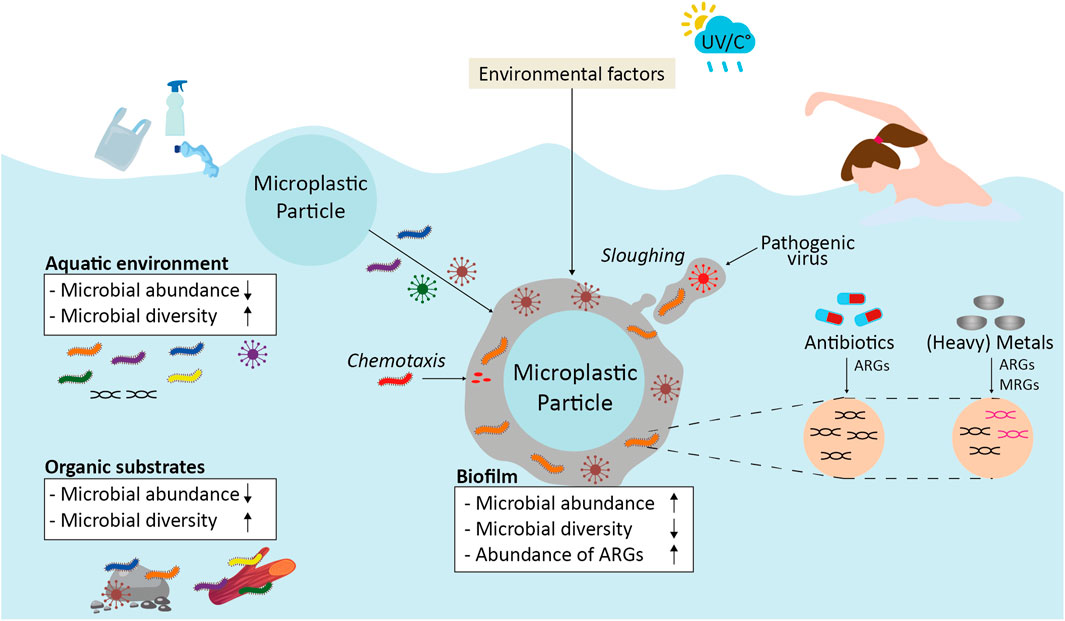
FIGURE 1. Overview of the different microbiological aspects of MNPs that may impact human health. Microbiological aspects may be affected by environmental factors and via physical and chemical aspects of MNPs. ARGs; antibiotic resistance genes, MRGs; metal resistance genes, UV; ultra violet radiation; °C; temperature in degrees Celsius.
2 MNP-associated biofilms are dynamic
MNPs were collected in various aquatic environments ranging from marine environments to freshwater. Planktonic microbes that stick to the surface of MNPs may form a microbial biofilm in which different types of microbes form a complex network of cells that stick to each other and to the MNP surface. The ecosystem that surrounds the MNP surface is referred to as the ‘plastisphere’ (Zettler et al., 2013). Potentially pathogenic bacteria (e.g., Vibrio spp., Arcobacter spp. and members of the Campylobacteraceae) (Zettler et al., 2013; Amaral-Zettler et al., 2020; Mughini-Gras et al., 2021; Metcalf et al., 2022), diatoms and algae (Audrezet et al., 2021), fungi (e.g., Aspergillus) and viruses (e.g., Rotavirus, Poliovirus, Norovirus, SARS-CoV-2) (Moresco et al., 2021) may inhabit the plastisphere of various MNP polymer types. Thus, MNPs that accumulate in these aquatic environments may serve as a habitat for a wide range of pathogenic microbes. The composition of biofilms is dynamic, since it is affected by physical and chemical properties of the MNP, as well as by environmental factors such as temperature, pH, salinity, UV radiation, nutrient limitations and natural organic matter (NOM). These combined factors might lead to competition between microbes for nutrients or binding sites on the particles, constantly allowing the biofilm to change in composition. Continuous subjection to changes in environmental factors allows for growth, attachment and detachment of (pathogenic) microbes which affect the environmental fate of MNPs. Detachment of a larger portion of the biofilm is called ‘sloughing’. Sloughs may allow for the sudden release of (multiple) pathogenic microbes from the biofilm at once (Skraber et al., 2005).
3 Humans may be exposed to MNP-associated biofilms
MNPs with biofilms have been found in virtually every type of water, including drinking-water (WHO, 2019a). The human health risk of these MNP-associated biofilms in drinking-water has been described as low considering that 1) drinking-water sources can contain pollution leading to a higher concentration and diversity of pathogens and 2) the concentrations of MNPs in fresh water are relatively low compared to other possible attachment sites for microbes or biofilms (e.g., drinking-water pipes) (WHO, 2019a). In contrast, a recent study by Yang et al. has detected higher levels of MPs in drinking-water (Yang et al., 2024). They showed that changes in water quality (chlorination, heating) and in environmental factors (freeze-thawing) expedited the release of MPs from polypropylene pipes, suggesting that this phenomenon occurs in a wide range of drinking-water pipes. Additionally, MNPs (that are for instance released from drinking-water pipes) are mostly light weight and persistent and may therefore, together with their associated biofilms, be transported over a long distance. Through sloughing, pathogenic microbes could be released miles from where microbes first attached (Skraber et al., 2005), in environments where these microbes are otherwise not present. Therefore, even whilst overall concentrations found in drinking-water may be relatively low, MNPs and their biofilms are still relevant for human health.
Once MNPs enter the human body they may impact human health via gastric, intestinal, pulmonary and dermal exposure. Local inflammation, apoptosis or oxidative stress may be induced, depending on the cellular uptake mechanism (Yee et al., 2021). It has been described that MNPs can lead to dysbiosis in the human intestinal microbiome (Fackelmann and Sommer, 2019). It is currently unclear whether pathogenic microbes in MNP-associated biofilms affect cellular uptake mechanisms and aggravate the effects elicited by MNPs, for instance via enhancing intestinal dysbiosis.
4 MNP size and chemical composition define their microbial composition
The dominant narrative is that the microbial content in biofilms of different types of MNPs found in aquatic environments is less diverse than the microbial content in biofilms of natural substrates (such as stone, wood and plant materials), or in the surrounding aquatic environment (Miao et al., 2019; Mughini-Gras et al., 2021). In contrast, microbial abundance was found to be higher in biofilms of MNPs compared to biofilms of organic material (McCormick et al., 2016; Miao et al., 2019). Furthermore, metabolic pathways in microbes in MNP-associated biofilms are different compared to microbes in biofilms on other substrates (Miao et al., 2019), possibly due to toxic properties of the plastics. Investigating the effect of diversity, abundance as well as the (metabolic) function of MNP-associated microbes is important to get a complete picture of the microbiological risks of MNPs.
The composition of MNP-associated biofilms appears to be determined by physical properties of MNPs such as particle size. In general, the microbial composition of biofilms on MPs is less diverse than those of NPs (Mughini-Gras et al., 2021). NPs are small in size but more abundant than MPs, therefore, the total surface to which microbes can attach is larger for NPs compared to MPs. The larger the total surface, the more space will be available for different microbes to attach. An increased surface-to-volume ratio also means an increase in chemical reactivity and hydrophobicity of the particle, which may contribute to the difference in microbial composition. For instance, adsorption rates for viruses are higher on smaller particles (2 µm) than on larger particles (100 µm), possibly due to a higher electrical (zeta) potential in smaller particles, allowing for enhanced adsorption of viral particles to the plastic surface (Lu et al., 2022). Whether this is similar for virus adsorption to NPs, needs to be investigated. After virus absorption, the zeta potential of the plastic particle surface changes (Lu et al., 2022), which may affect the binding of other viruses, or other types of microbes. Studies with pharmaceuticals have shown that in addition to hydrophobicity of the MNPs, ionic strength also affects the sorption potential of MPs (Puckowski et al., 2021; YangLi et al., 2023). When measuring biofilm compositions, these physical properties of MNPs should be measured and reported as well, to correlate the biofilm composition to physical properties and link this to human exposure and uptake mechanisms.
Notably, the composition of MNP-associated biofilms is also affected by the chemical composition of MNPs, including the release (leaching) of chemicals (Li et al., 2022; Nelis et al., 2023). This (chemical) composition varies per plastic polymer type and may therefore differentially affect the microbial composition of MNPs. For instance, MNP polymer types polyethylene and polypropylene can release dissolved organic matter (Amaral-Zettler et al., 2020). Microbes may migrate towards these hydrocarbons to take up dissolved organic matter via chemotaxis (Amaral-Zettler et al., 2020). In contrast, leachates may exert cytotoxic effects on microbes (Sarker et al., 2020; Liu et al., 2022), potentially leading to microbial degradation or inactivation and altered environmental fate of the plastic particles. Notably, the presence of different microplastic-specific functional groups (COOH, NH2) affect the microbial composition. COOH groups enhance, while NH2 groups decrease, virus adsorption rate to plastics compared to plastic particles without functional groups (Lu et al., 2022), hereby selecting for the type of pathogen present on the particle.
The detection and composition of MNP-associated pathogenic microbes (e.g., E. coli and Vibrio spp.) is subject of study and generally performed with sequencing techniques or quantitative molecular detection methods. However, the virulence (pathology) of these microbes is severely understudied, yet provide important pieces to the risk assessment puzzle. A clear example was found in the virulence genes of Rhodococcus equi, a bacterium that can infect immunocompromised humans causing pulmonary infections. These virulence genes were upregulated when in contact with disposable polypropylene, but not when in contact with glass (Hansen et al., 2022), suggesting that MNPs have the potency to affect virulence factors of microbes. However, which properties (physical, chemical or both) attributed to this observation, remains unclear. Studies should therefore focus on the effect of (standardized) MNPs on virulence factors of pathogenic microbes in different exposure-relevant environments. These studies would aid in understanding the plastic-mediated infection risks upon inhalation, ingestion or direct contact of MNPs.
5 MNPs may facilitate the emergence of antimicrobial resistance
Aquatic environments are often polluted with antibiotics, responsible for the rapid emergence of AMR (WHO, 2019b). AMR is achieved via various antibiotic resistance mechanisms including the reduction in bacterial cell permeability, intra- and extracellular sequestration of antibiotics, efflux pumps, mutations at target sites and the formation of biofilms (Imran et al., 2019). Bacteria in the plastisphere harbor different antibiotic resistance genes (ARGs) and pathogenic bacteria profiles than biofilms on natural substrates or bacteria in the surrounding aquatic environment (Wu et al., 2019). Furthermore, the abundance of ARGs (e.g., sul1, ermB) in MNP-associated biofilms was higher compared to bacteria in the surrounding environment (Wu et al., 2019; Shi et al., 2020). ARGs can be transferred to other (pathogenic) bacteria via horizontal gene transfer of mobile genetic elements, leading to antibiotic resistance in the receiving bacterial cell. Horizontal gene transfer was found to occur faster between microbes on MPs than between planktonic microbes (Arias-Andres et al., 2018). This was corroborated by the increase in abundance of ARGs and mobile genetic elements on MNPs over time (Imran et al., 2019; Shi et al., 2020). Considering that MNPs might serve as a vector for AMR and are ideal substrates for the long-distance transport of biofilms, MNPs might expose people to resistant microbes, for example, while swimming, hereby posing a human health threat.
Since the size of MNPs affects the microbial composition of the MNP-associated biofilm, it may also affect the diversity or abundance of ARGs (Yang et al., 2019). NPs contain more reactive oxygen species (ROS) and microbes with a lower membrane permeability than MPs, which may lead to more bacterial cell damage (Shi et al., 2020). This lower membrane permeability found in NP-associated microbes may enhance AMR. Overall, the effects of the physical and chemical properties of MNPs on the diversity, abundance and function of ARGs is understudied, yet would help in assessing the integrated risks of MNPs and would predict which measures could be taken to minimize the change of MNP-facilitated AMR emergence.
AMR is, in addition to antibiotics, driven by other environmental factors, such as the presence of metals in aquatic environments. Some metals are beneficial or even required for physiological functions of microbes (e.g., MNO2, Fe, Co) while other metals are toxic for microbes even when present at a low dosage (e.g., Pb, Cd). The presence of (heavy) metals in aquatic environments drives the selection for metal resistance genes (MRGs) (Bartkova et al., 2021). When MRGs and ARGs are present on the same mobile genetic element, co-resistance can occur (Imran et al., 2019; Li et al., 2021). This co-resistance leads to metal-driven antibiotic resistance in nearby bacterial cells for instance when present in an MNP-associated biofilm. Both MRGs and ARGs have been found in potential clinically relevant bacteria including Salmonella enterica, E. coli, S. aureus, Pseudomonas spp., Acinetobacter spp., Listeria spp., Aeromonas spp. and Proteus spp. (Imran et al., 2019), some of which have the ability to form biofilms. Metals may exert a long-lasting selection pressure on ARGs because they cannot easily be degraded. Thus, the environmental effect of (heavy) metals may also exert a long-lasting effect on AMR via MNPs.
6 Environmental factors affect the physical, chemical and microbiological properties of MNPs
In addition to the presence of antibiotics or (heavy) metals in aquatic environments, other environmental factors, such as UV radiation, temperature and pH also affect the composition and function of microbes in MNP-associated biofilms. The effect of these environmental factors on the microbes are either exerted directly, or indirectly via affecting the MNP.
Different environmental factors may affect the MNPs (Al Harraq et al., 2022). They may lead to leaching of MNP-associated chemicals (Imran et al., 2019), that could in turn affect microbial survival, composition and function. For example, changes in water quality (heating) and freeze-thawing of plastic pipes accelerated chemical leaching with a distinct chemical profile. Incubation of these leachates with indigenous bacteria in drinking-water led to enhanced cytotoxicity as measured by bacterial ROS production and cell wall permeability and led to a higher abundance of pathogenic bacteria and a change in virulence gene and ARG profiles (Yang et al., 2024).
In addition to leaching, environmental factors might lead to the degradation of particles for instance via UV radiation-mediated ageing of the particles. Such a process may result in changes in size, available chemical groups and (bio)availability of surface area of particles (Al Harraq et al., 2022). These smaller particles exhibit more surface area and may therefore lead to more microbial adsorption. The ageing of particles by UV radiation was found to affect virus adsorption rates, with more virus adsorption on UV-aged particles and on particles with no functional groups, or with NH2 groups (Lu et al., 2022). Furthermore, a higher surface area can lead to enhanced adsorption of chemical pollutants (Al Harraq et al., 2022) that may result in altered attraction and selection of, or toxicity to, microbes. Adsorption of antibiotics and metals by MNPs (Imran et al., 2019), could for instance drive the selection mechanisms for AMR. Notably, adsorption rates of chemical and microbiological components are also (positively or negatively) affected by the presence of NOM in aquatic environments, depending on the type of water, source of NOM and other environmental factors (Junaid and Wang, 2021; Ali et al., 2022).
Environmental factors are dynamic and can, due to the high number of relevant factors and rapid changes within these factors, never fully be mimicked in vitro. However, the interplay between environmental factors, microbes and MNPs is unclear yet critical for the risk assessment of MNPs on human health. Studies should therefore focus on elucidating this interplay first, for instance by assessing which factors are dominant when it comes to microbial formation and microbial compositions.
7 Discussion
MNPs may play a facilitating role in two major threats to global health: firstly, the potential spread and, secondly, the emergence of infectious diseases and AMR. Currently, the physical and chemical impacts of MNPs on human health are unclear, and the microbiological impacts of MNPs are even more indeterminate. The impact of microbiological aspects of MNPs should be included when assessing the overall risk of MNPs on human health (Figure 1). For this, quantitative risk assessment frameworks and input data are needed. We believe that steps should be taken towards further characterization of microbial biofilms on MNPs found in different environments. This should include the elucidation of biofilm properties such as the characterization of different biofilm-associated microbes and their persistence and virulence. Since MNPs are continuously exposed to environmental factors, their physical, chemical and microbiological properties are dynamic. The interplay between these different properties of MNPs should be studied, rather than snap shots of their compositions, in order to understand these dynamics better. Investigating AMR traits generated under different environmental conditions and in the presence of antibiotics and metals is of particular importance. Of note, NPs, rather than MPs, and their associated biofilms may enter the human body and translocate across human tissues. The routes of exposure and translocation of NPs impact their location in the human body and thus the concentration of both NPs and microbes in different tissue compartments. Understanding the various ways in which microbiological aspects of MNPs may impact human health will provide insight in the occurrence and emergence of infectious diseases and AMR and thus in selecting the best preventative strategies possible to avert integrated MNP health impacts.
Data availability statement
The original contributions presented in the study are included in the article/Supplementary material, further inquiries can be directed to the corresponding author.
Author contributions
MS: Conceptualization, Visualization, Writing–original draft, Writing–review and editing. AR: Funding acquisition, Writing–original draft, Writing–review and editing.
Funding
The author(s) declare financial support was received for the research, authorship, and/or publication of this article. This work is funded by the Netherlands Organisation for Health Research and Development (ZonMw) and Health Holland through the Microplastics and Human Health consortium MOMENTUM (Grant ID: 458001101).
Acknowledgments
We thank F. Pierik, program leader ZonMw Microplastics and Health program, and all collaborators in this program for helpful discussions. We thank C. Deuning from the Dutch National Institute for Public Health and the Environment for help with the illustration.
Conflict of interest
The authors declare that the research was conducted in the absence of any commercial or financial relationships that could be construed as a potential conflict of interest.
Publisher’s note
All claims expressed in this article are solely those of the authors and do not necessarily represent those of their affiliated organizations, or those of the publisher, the editors and the reviewers. Any product that may be evaluated in this article, or claim that may be made by its manufacturer, is not guaranteed or endorsed by the publisher.
References
Al Harraq, A., Brahana, P. J., Arcemont, O., Zhang, D., Valsaraj, K. T., and Bharti, B. (2022). Effects of weathering on microplastic dispersibility and pollutant uptake capacity. ACS Environ. Au 2, 549–555. doi:10.1021/acsenvironau.2c00036
Ali, I., Tan, X., Li, J., Peng, C., Naz, I., Duan, Z., et al. (2022). Interaction of microplastics and nanoplastics with natural organic matter (NOM) and the impact of NOM on the sorption behavior of anthropogenic contaminants – a critical review. J. Clean. Prod. 376, 134314. doi:10.1016/j.jclepro.2022.134314
Amaral-Zettler, L. A., Zettler, E. R., and Mincer, T. J. (2020). Ecology of the plastisphere. Nat. Rev. Microbiol. 18, 139–151. doi:10.1038/s41579-019-0308-0
Antimicrobial Resistance Collaborators (2022). Global burden of bacterial antimicrobial resistance in 2019: a systematic analysis. The Lancet 399(10325), 629–655. doi:10.1016/S0140-6736(21)02724-0
Arias-Andres, M., Klumper, U., Rojas-Jimenez, K., and Grossart, H. P. (2018). Microplastic pollution increases gene exchange in aquatic ecosystems. Environ. Pollut. 237, 253–261. doi:10.1016/j.envpol.2018.02.058
Audrezet, F., Zaiko, A., Lear, G., Wood, S. A., Tremblay, L. A., and Pochon, X. (2021). Biosecurity implications of drifting marine plastic debris: current knowledge and future research. Mar. Pollut. Bull. 162, 111835. doi:10.1016/j.marpolbul.2020.111835
Bartkova, S., Kahru, A., Heinlaan, M., and Scheler, O. (2021). Techniques used for analyzing microplastics, antimicrobial resistance and microbial community composition: a mini-review. Front. Microbiol. 12, 603967. doi:10.3389/fmicb.2021.603967
Brachner, A., Fragouli, D., Duarte, I. F., Farias, P. M. A., Dembski, S., Ghosh, M., et al. (2020). Assessment of human health risks posed by nano-and microplastics is currently not feasible. Int. J. Environ. Res. Public Health 17, 8832. doi:10.3390/ijerph17238832
European Comission (2024). Microplastics. Available at: https://environment.ec.europa.eu/topics/plastics/microplastics_en (Accessed March 28, 2024).
Fackelmann, G., and Sommer, S. (2019). Microplastics and the gut microbiome: how chronically exposed species may suffer from gut dysbiosis. Mar. Pollut. Bull. 143, 193–203. doi:10.1016/j.marpolbul.2019.04.030
Gonzalez-Acedo, A., Garcia-Recio, E., Illescas-Montes, R., Ramos-Torrecillas, J., Melguizo-Rodriguez, L., and Costela-Ruiz, V. J. (2021). Evidence from in vitro and in vivo studies on the potential health repercussions of micro- and nanoplastics. Chemosphere 280, 130826. doi:10.1016/j.chemosphere.2021.130826
Gruber, E. S., Stadlbauer, V., Pichler, V., Resch-Fauster, K., Todorovic, A., Meisel, T. C., et al. (2023). To waste or not to waste: questioning potential health risks of micro- and nanoplastics with a focus on their ingestion and potential carcinogenicity. Expo. Health 15, 33–51. doi:10.1007/s12403-022-00470-8
Hansen, P., Von Bargen, K., Jünger-Leif, A., and Haas, A. (2022). Laboratory plasticware induces expression of a bacterial virulence factor. mSphere 7, 1–11. doi:10.1128/msphere.00311-22
Imran, M., Das, K. R., and Naik, M. M. (2019). Co-selection of multi-antibiotic resistance in bacterial pathogens in metal and microplastic contaminated environments: an emerging health threat. Chemosphere 215, 846–857. doi:10.1016/j.chemosphere.2018.10.114
Junaid, M., and Wang, J. (2021). Interaction of nanoplastics with extracellular polymeric substances (EPS) in the aquatic environment: a special reference to eco-corona formation and associated impacts. Water Res. 201, 117319. doi:10.1016/j.watres.2021.117319
Liu, Y., Zhou, H., Yan, M., Liu, Y., Ni, X., Song, J., et al. (2022). Toxicity of tire wear particles and the leachates to microorganisms in marine sediments. Environ. Pollut. 309, 119744. doi:10.1016/j.envpol.2022.119744
Li, Y., Lu, Z., Abrahamsson, D. P., Song, W., Yang, C., Huang, Q., et al. (2022). Non-targeted analysis for organic components of microplastic leachates. Sci. Total Environ. 816, 151598. doi:10.1016/j.scitotenv.2021.151598
Li, Z., Junaid, M., Chen, G., and Wang, J. (2021). Interactions and associated resistance development mechanisms between microplastics, antibiotics and heavy metals in the aquaculture environment. Rev. Aquac. 14, 1028–1045. doi:10.1111/raq.12639
Loiseau, C., and Sorci, G. (2022). Can microplastics facilitate the emergence of infectious diseases? Sci. Total Environ. 823, 153694. doi:10.1016/j.scitotenv.2022.153694
Lu, J., Yu, Z., Ngiam, L., and Guo, J. (2022). Microplastics as potential carriers of viruses could prolong virus survival and infectivity. Water Res. 225, 119115. doi:10.1016/j.watres.2022.119115
Mccormick, A. R., Hoellein, T. J., London, M. G., Hittie, J., Scott, J. W., and Kelly, J. J. (2016). Microplastic in surface waters of urban rivers: concentration, sources, and associated bacterial assemblages. Ecosphere 7. doi:10.1002/ecs2.1556
Metcalf, R., Oliver, D. M., Moresco, V., and Quilliam, R. S. (2022). Quantifying the importance of plastic pollution for the dissemination of human pathogens: the challenges of choosing an appropriate 'control' material. Sci. Total Environ. 810, 152292. doi:10.1016/j.scitotenv.2021.152292
Miao, L., Wang, P., Hou, J., Yao, Y., Liu, Z., Liu, S., et al. (2019). Distinct community structure and microbial functions of biofilms colonizing microplastics. Sci. Total Environ. 650, 2395–2402. doi:10.1016/j.scitotenv.2018.09.378
Moresco, V., Oliver, D. M., Weidmann, M., Matallana-Surget, S., and Quilliam, R. S. (2021). Survival of human enteric and respiratory viruses on plastics in soil, freshwater, and marine environments. Environ. Res. 199, 111367. doi:10.1016/j.envres.2021.111367
Mughini-Gras, L., Van Der Plaats, R. Q. J., Van Der Wielen, P., Bauerlein, P. S., and De Roda Husman, A. M. (2021). Riverine microplastic and microbial community compositions: a field study in The Netherlands. Water Res. 192, 116852. doi:10.1016/j.watres.2021.116852
Nelis, J. L. D., Schacht, V. J., Dawson, A. L., Bose, U., Tsagkaris, A. S., Dvorakova, D., et al. (2023). The measurement of food safety and security risks associated with micro- and nanoplastic pollution. TrAC Trends Anal. Chem. 161, 116993. doi:10.1016/j.trac.2023.116993
Puckowski, A., Cwiek, W., Mioduszewska, K., Stepnowski, P., and Bialk-Bielinska, A. (2021). Sorption of pharmaceuticals on the surface of microplastics. Chemosphere 263, 127976. doi:10.1016/j.chemosphere.2020.127976
Sana, S. S., Dogiparthi, L. K., Gangadhar, L., Chakravorty, A., and Abhishek, N. (2020). Effects of microplastics and nanoplastics on marine environment and human health. Environ. Sci. Pollut. Res. Int. 27, 44743–44756. doi:10.1007/s11356-020-10573-x
Sarker, I., Moore, L. R., Paulsen, I. T., and Tetu, S. G. (2020). Assessing the toxicity of leachates from weathered plastics on photosynthetic marine bacteria prochlorococcus. Front. Mar. Sci. 7. doi:10.3389/fmars.2020.571929
Shi, J., Wu, D., Su, Y., and Xie, B. (2020). Nano microplastics promote the propagation of antibiotic resistance genes in landfill leachate. Environ. Sci. Nano 7, 3536–3546. doi:10.1039/d0en00511h
Skraber, S., Schijven, J., Gantzer, C., and De Roda Husman, A. M. (2005). Pathogenic viruses in drinking-water biofilms: a public health risk? Biofilms 2, 105–117. doi:10.1017/s1479050505001833
Vethaak, A. D., and Legler, J. (2021). Microplastics and human health. Science 371, 672–674. doi:10.1126/science.abe5041
World Health Organization (WHO) (2019b). Ten threats to global health in 2019. Available at: https://www.who.int/news-room/spotlight/ten-threats-to-global-health (Accessed January 5, 2024).
Wu, X., Pan, J., Li, M., Li, Y., Bartlam, M., and Wang, Y. (2019). Selective enrichment of bacterial pathogens by microplastic biofilm. Water Res. 165, 114979. doi:10.1016/j.watres.2019.114979
Yang, W., Li, Y., and Boraschi, D. (2023). Association between microorganisms and microplastics: how does it change the host-pathogen interaction and subsequent immune response? Int. J. Mol. Sci. 24, 4065. doi:10.3390/ijms24044065
Yang, X., Zhou, Y., Xia, R., Liao, J., Liu, J., and Yu, P. (2024). Microplastics and chemical leachates from plastic pipes are associated with increased virulence and antimicrobial resistance potential of drinking water microbial communities. J. Hazard Mater 463, 132900. doi:10.1016/j.jhazmat.2023.132900
Yang, Y., Liu, G., Song, W., Ye, C., Lin, H., Li, Z., et al. (2019). Plastics in the marine environment are reservoirs for antibiotic and metal resistance genes. Environ. Int. 123, 79–86. doi:10.1016/j.envint.2018.11.061
Yee, M. S., Hii, L. W., Looi, C. K., Lim, W. M., Wong, S. F., Kok, Y. Y., et al. (2021). Impact of microplastics and nanoplastics on human health. Nanomater. (Basel), 11. doi:10.3390/nano11020496
Keywords: micro-and nanoplastics, microbes, risk assessment, biofilms, pathogens, human health, antimicrobial resistance
Citation: Stunnenberg M and de Roda Husman AM (2024) It’s a matter of microbes: a perspective on the microbiological aspects of micro- and nanoplastics in human health. Front. Nanotechnol. 6:1368437. doi: 10.3389/fnano.2024.1368437
Received: 10 January 2024; Accepted: 04 March 2024;
Published: 15 March 2024.
Edited by:
Brij Pal Singh, Central University of Haryana, IndiaReviewed by:
Bharat Bhushan, National Institute of Food Technology Entrepreneurship and Management, IndiaTan Suet May Amelia, University of Malaysia Terengganu, Malaysia
Copyright © 2024 Stunnenberg and de Roda Husman. This is an open-access article distributed under the terms of the Creative Commons Attribution License (CC BY). The use, distribution or reproduction in other forums is permitted, provided the original author(s) and the copyright owner(s) are credited and that the original publication in this journal is cited, in accordance with accepted academic practice. No use, distribution or reproduction is permitted which does not comply with these terms.
*Correspondence: Melissa Stunnenberg, bWVsaXNzYS5zdHVubmVuYmVyZ0ByaXZtLm5s