- 1Departamento de Ciencias Médicas Básica and Instituto de Tecnologías Biomédicas, Centro de Investigaciones Biomédicas de Canarias, Universidad de La Laguna, La Laguna, Spain
- 2Centro de Biología Molecular “Severo Ochoa” (CSIC-UAM), Universidad Autónoma de Madrid, Madrid, Spain
- 3Centro de Investigación Biomédica en Red de Enfermedades Neurodegenerativas, Madrid, Spain
- 4Instituto de Química Avanzada de Cataluña (IQAC-CSIC), Barcelona, Spain
- 5Departamento de Biología Animal, Edafología y Geología, and Instituto de Tecnologías Biomédicas, Centro de Investigaciones Biomédicas de Canarias, Universidad de La Laguna, Tenerife, Spain
- 6Unidad de Estadística Médica, Universidad Católica de Murcia, Murcia, Spain
- 7Instituto Cajal (CSIC) and Centro de Investigación Biomédica en Red de Fragilidad y Envejecimiento Saludable, Madrid, Spain
Different dietary ratios of n−6/n−3 long-chain polyunsaturated fatty acids (LC-PUFAs) may alter brain lipid profile, neural activity, and brain cognitive function. To determine whether ovarian hormones influence the effect of diet on the brain, ovariectomized and sham-operated mice continuously treated with placebo or estradiol were fed for 3 months with diets containing low or high n−6/n−3 LC-PUFA ratios. The fatty acid (FA) profile and expression of key neuronal proteins were analyzed in the cerebral cortex, with intact female mice on standard diet serving as internal controls of brain lipidome composition. Diets containing different concentrations of LC-PUFAs greatly modified total FAs, sphingolipids, and gangliosides in the cerebral cortex. Some of these changes were dependent on ovarian hormones, as they were not detected in ovariectomized animals, and in the case of complex lipids, the effect of ovariectomy was partially or totally reversed by continuous administration of estradiol. However, even though differential dietary LC-PUFA content modified the expression of neuronal proteins such as synapsin and its phosphorylation level, PSD-95, amyloid precursor protein (APP), or glial proteins such as glial fibrillary acidic protein (GFAP), an effect also dependent on the presence of the ovary, chronic estradiol treatment was unable to revert the dietary effects on brain cortex synaptic proteins. These results suggest that, in addition to stable estradiol levels, other ovarian hormones such as progesterone and/or cyclic ovarian secretory activity could play a physiological role in the modulation of dietary LC-PUFAs on the cerebral cortex, which may have clinical implications for post-menopausal women on diets enriched with different proportions of n−3 and n−6 LC-PUFAs.
Introduction
Phospholipids are major components of neural cell membranes, playing critical roles in synaptic transmission and neuronal signaling through interactions with specific membrane proteins (Bazan, 2014). The brain's complement of phospholipids and other complex lipids contains large amounts of long-chain polyunsaturated fatty acids (LC-PUFAs) such as arachidonic acid (ARA; 20:4n−6) and docosahexaenoic acid (DHA; 22:6n−3), but low levels of other omega-3 LC-PUFAs, especially eicosapentaenoic acid (EPA; 20:5n−3) (Brenna and Diau, 2007; Chen et al., 2009). While saturated and monounsaturated fatty acids can be synthesized de novo within the brain, LC-PUFAs are mainly supplied by the blood and are synthesized from two dietary precursors: linoleic acid (LNA; 18:2n−6) and α-linoleic acid (ALA; 18:3n−3) (Carrié et al., 2000; Williard et al., 2001; Bazinet and Layé, 2014). Even though the specific mechanisms involved in phospholipid signaling are not completely understood, several pro-resolving lipid mediators (SPMs) have been identified, including 17S-hydroxy-DHA (17S-DHA), neuroprotectin D1 (NPD-1), resolvin D5 (RvD5), 14S-HDHA and maresin 1 (MaR1) (Orr et al., 2013; Serhan, 2014). LC-PUFAs and SPMs participate in a plethora of signaling processes, such as cell survival and neuroinflammation, neurotransmission, and cognitive function (Bannenberg and Serhan, 2010; Serhan and Chang, 2013). One of the best-characterized SPMs is NPD-1, which is synthesized in response to brain injury and may have therapeutic potential in a wide range of neurological conditions (Bazan et al., 2011a,b, 2013).
In rodents, daily intake of omega-3 LC-PUFAs (n−3 LC-PUFAs) is necessary for neural development and maintenance of synaptic circuitry (Brenna and Diau, 2007; Dyall and Michael-Titus, 2008; Bazan et al., 2011a,b). In addition, experimental and clinical data support that their dietary inclusion has positive effects on numerous pathological conditions (Gorjão et al., 2009; Mozaffarian and Wu, 2012; Russell and Bürgin–Maunder, 2012). Diets enriched in n−3 LC-PUFAs have been associated with a lower incidence of dementia and neurological disorders (Mazza et al., 2007), while diets containing low percentage of DHA may be linked to cognitive impairment (Ikemoto et al., 2001; Catalan et al., 2002). Interestingly, the ratio of n−3 to n−6 LC- PUFA in the diet appears to be a critical factor for the effect on the brain lipidome (Bourre et al., 1984; Jumpsen et al., 1997; Carrié et al., 2000). On the other hand, women have significantly lower blood levels of docosapentaenoic acid (DPA; 22:5-3) and EPA, but significantly higher levels of DHA than men (Metherel et al., 2009). In studies with female mice, it has been reported that the effect of diet on the brain lipidome may be partially dependent on circulating levels of gonadal hormones (Díaz et al., 2016). Furthermore, experimental evidence has indicated that estrogen may modulate neuronal signaling through interactions with specific estrogen receptors in neural membrane microdomains, such as lipid rafts (Herrera et al., 2011; Marín et al., 2013).
The aim of this study was to investigate the putative synergistic interaction between estradiol and differential dietary fatty acid (FA) supplementation, and observe how this combination may affect the long-term lipid and protein profile in the brain. A multifactorial design was established to investigate the effects of diets containing two different ratios of n−3 and n−6 LC-PUFAs, specifically EPA and DHA, and their potential interaction with reproductive status in placebo and estradiol-treated ovariectomized mice. At the end point, analysis of the cerebral cortex showed that both diets modified the brain lipidome when compared to standard diet, and may influence neural function by modifying the content of several proteins involved in intracellular signaling and synaptic transmission. In addition, some of these effects were sensitive to the presence or absence of the ovaries and, at least partially, to circulating levels of estradiol.
Materials and Methods
Animals and Husbandry
Female C57BL/6J mice (Mus musculus) were purchased from Charles River Laboratories, and housed under constant temperature (22 ± 2°C) and humidity (50 ± 5%) and a 12:12 h light-dark cycle in specific pathogen–free conditions. Mice (10 per cage) were housed in cages fitted with microbarrier filter tops, and allowed access to food (A03/R03, SAFE-Panlab) and tap water ad libitum. One month after birth, the standard laboratory food was gradually replaced in all animals with the experimental diets (see below) at a rate of 25% per week; all mice were fed with 100% experimental diet from the age of 2 months until the time of sacrifice. For lipid analysis, a group of intact females fed with standard laboratory food was used as a control. La Laguna University Animal Care and Use Committee approved the protocol for this study.
Ovariectomy and Hormone Treatments
In preliminary experiments it was found that ovariectomized mice implanted with 0.05 mg estradiol pellets (Innovative Research of America, Sarasota, Florida) for 90 days showed plasma estradiol levels of 7.2 ± 4.54 pg/ml (mean ± SEM) as determined by RIA (Architect System, ref #B7K720, Abbot, Germany). Since these values are within the range of those found in cycling animals of the same age at proestrus (20.9 ± 6.03 pg/ml) and estrus (1.8 ± 4.54 pg/ml), this dose of estradiol was chosen for the dietary experiments. Thus, female mice were anesthetized with inhaled isoflurane (2 ± 0.5%) after analgesic injection (buprenorphine hydrochloride, Buprex), and then bilaterally ovariectomized or sham-operated at 90 ± 1 days-of-age through a 1 cm dorsal incision that was closed with surgical clips. The day after ovariectomy mice received a 3 mm pellet containing a 90-day timed-release 0.05 mg 17β-estradiol or placebo. The pellet was subcutaneously implanted in the subscapular region by using a trocar according to manufacturer's instructions. Animals still showing inflammation around the implantation area 13 days after receiving the pellet were discarded. Mice were then housed according to the different diets and hormonal treatments until the end-point 90 days later, as shown in Table 1.
Diets
Two specific experimental diets were used in these experiments. The high n−6/n−3 ratio diet (DI) containing safflower oil, which had undetectable levels of EPA (20:5n−3) and DHA (22:6n−3), had abundant oleic acid (18:1n−9) and linoleic acid (18:2n−6), but was poor in α-linolenic acid (18:3n−3). The low n−6/n−3 ratio diet (DII) had the same basic composition, but was supplemented with extra EPA and DHA in a proportion of 7 g/kg of diet, added in the form of fish oil as a lipid source to give a particularly high DHA content. These diets were designed in the Instituto de Nutrición y Tecnología de los Alimentos at the University of Granada, Spain, and produced by Mucedola (Mucedola srl, Milano, Italy). Both diets and the standard laboratory food (SF) were subsequently lab-analyzed to determine the final percentage and absolute quantity (g/kg) of each FA (Table 2). Summarizing the main diet differences: (a) DI had a n−6/n−3 ratio 13–14 times higher than that of SF and 59 times higher than that of DII; (b) DII had a n−6/n−3 ratio 4–5 times lower than that of SF; (c) DI did not have traces of either DHA or EPA, while DII contained relevant amounts of both LC-PUFAs (Table 2). For simplicity, high and low n−6/n−3 LC-PUFA ratio diets are referred to in the text and graphics as DI and DII, respectively. Mice were fed these diets ad libitum for 90 days until the time of sacrifice.
Tissue Processing and Sample Preparation
Mice were sacrificed using CO2 90 days after ovariectomy or sham operation, and their brains were collected. Cerebral cortex tissue for western blot analysis was homogenized in 3 volumes of ice-cold lysis buffer containing 20 mM HEPES, 100 mM NaCl, 100 mM NaF, 1 mM Na3VO4, 5 mM EDTA, 1% Triton X100 plus protease inhibitor cocktail (Roche Diagnostic) and 1 μM Okadaic acid (Calbiochem). Homogenates were centrifuged at 16,000 × g for 20 min at 4°C, and supernatants were stored at −80°C. Protein concentration was measured using BioRad DC Protein Assay (BioRad) following the manufacturer's protocol. Buffer containing 10% sodium dodecyl sulfate (SDS), 0.5 mM dithiothreitol, 325 mM Tris HCl, pH 6.8, 87% glycerol and bromophenol blue was added to samples before loading into polyacrylamide gels for electrophoresis. For all samples Western blot determinations were repeated 3–4 times. For lipid analysis, tissues were homogenized at a concentration of 5 mg/ml in PBS with 0.01% 3,5-Di-tert-4-butylhydroxytoluene (HBT) as an antioxidant.
Western Blot Analysis
Cerebral cortex tissue extracts were resolved by SDS-PAGE and transferred onto nitrocellulose (Whatman) or PVDF (Millipore) membranes, and subsequently blocked by incubation in 10% non-fat milk for 1 h at room temperature. Membranes were incubated overnight at 4°C with appropriate primary antibodies (Table 3), then washed in 0.1% Tween–PBS and incubated with secondary horseradish peroxide-conjugated antibody (Santa Cruz Biotechnology, Santa Cruz, CA, USA). Antibody binding was detected with SuperSignalTM (Thermo-Fisher Scientific), using β-actin as internal loading control. Densitometry analysis was performed using a GS-800 Calibrated Densitometer (Bio-Rad).
Lipid Analysis
Lipid extraction from dietary samples and cerebral tissue was performed using a modification of the Folch's method (Folch et al., 1957). Dietary FA profiles (g FA/kg diet fresh weight) were obtained by means of acid-catalyzed transmethylation of the lipid fractions followed by GC-MS analysis (Fabelo et al., 2014). Similarly, the cerebral cortex lipid fractions were subjected to further analysis of FA and complex lipids as previously described (Cingolani et al., 2014). Sphingolipids were analyzed by HPLC-MS (Garanto et al., 2013) by using 0.2 nmol of C17-sphinganine, N–dodecanoylsphingosine, N–dodecanoylglucosylsphingosine, and N–dodecanoyl sphingosylphosphorylcholine as internal standards. Sphingolipids were annotated as <lipid subclass> <total fatty acyl chain length>: <total number of unsaturated bonds>. If the sphingoid base residue was dihydrosphingosine, the lipid class contained a <DH> prefix. Since our chromatographic separation did not discriminate between dh-glucosylceramides (GlcdhCer) and dh-galactosylceramides (GaldhCer), their mixture was represented as monohesoxylceramides (MHC). In all cases, the final tissue data was indicated as pmol/mg of protein, except in the case of total FA, which were calculated as pmol equivalents per mg of protein with respect to C12 ceramide. For statistical analysis, all lipids were shown as percentage of variation relative to its content in brain tissue from animals fed with SF.
Statistical Analysis
For lipidomic outcomes, we performed a 2 × 3 factorial analysis with an extra control group. General ANOVA was performed, followed by linear contrasts to answer the questions posed during the design phase of the study. Regardless, no more than 6 contrasts were performed. In this way, 12 response variables were tested in addition to the “multi-testing effect,” whose accurate quantification is unfeasible. A reasonable approach to take this into account is to consider only those effects or interactions in which the p-value of the test is <0.01. However, when any interaction was found to be significant in several of the analyzed lipids, it is discussed, even if the p-value of the test is slightly higher than 0.01. In addition, the 95% confidence interval was calculated for all effects and interactions of interest.
In the case of changes in cerebral cortex protein expression levels, since the effect of diet under each reproductive status was monitored in different electrophoresis gels, it was not feasible to evaluate possible effects of each hormone condition. However, we were still able to evaluate the interaction of the hormonal condition with the effect of the diet. Thus, for each reproductive status and within the same gel, we calculated the percentage of variation induced by DII vs. DI diets. Then, in order to estimate effects and interactions between the different factors, we ran a saturated regression model which includes the dichotomous variable diet and the polytomous variable hormone condition with three levels (Armitage et al., 2004). The latter entered the model as two dummy variables, the first with a value of “1” in ovariectomized animals receiving placebo pellets, and the second with value “1” in ovariectomized mice treated with estradiol. Intact, sham-operated controls had a value of “0” in each dummy variable. This model provides us with confidence intervals and tests for the three effects of the diet conditioned to the hormonal status and the interaction between the two factors, adding up to the 5 comparisons “a priori” expected in the experimental design. Although there are four animals per group, the 2 × 3 factorial design includes 6 × 4 = 24 animals, which allows us to estimate the intragroup population variance with 6 × 3 = 18 degrees of freedom (df). In this way, any contrast that compares two means follows a t-Student distribution with 18 df. This provides a higher statistical power than the simple comparison of two groups with 4 animals per group.
For all tests, actual p-values are given for each comparison and the significance of the main effects detected by the analysis. The statistical software used was Stata 14 (StataCorp LLC, College Station, Texas) for inference factorial analysis and GraphPad Prisma 7 (GraphPad Software, Inc., San Diego, California) for graphic representations.
Results
The Cerebral Cortex Lipidome Is Affected by Dietary Differences in the Ratio of n−3 and n−6 LC-PUFAs
In intact, sham-operated females (CO), both experimental diets (DI and DII) induced a dramatic rise in total brain cortex FAs (Figure 1 and Table 4A), compared with animals fed with SF (DI: p = 0.0001; DII: p = 0.015) and the effect of DI was even higher than that of DII (p = 0.004). The effect of diet was not significantly affected by reproductive status (p = 0.821), and no significant interaction was detected (p = 0.451). Thus, cerebral cortex levels of total FAs were altered by changes in the ratios of dietary n−3 and n−6 LC-PUFAs, apparently regardless of the presence of ovaries and circulating levels of estradiol. With regard to the LC-PUFAs analyzed, each was significantly altered by both experimental diets compared to their levels in animals fed with SF (p < 0.01 or p < 0.001). In the case of C20:4n−6 (arachidonic acid, ARA) almost no modifications were seen (Figure 1 and Table 4B), in parallel with the dietary content of its precursor, C18:2n−6 (linoleic acid), which had the lowest levels in mice fed with SF, and the highest in those fed with DI (Table 2). In contrast, levels of C22:4n−6 (adrenic acid; ADA) and C22:5n−6 (DPA) were elevated in animals fed with DI (high n−6/n−3 ratio) when compared to mice fed with SF or DII (with lower n−6/n−3 ratio), as shown in Figure 1, Table 4C (p = 0.0091) and Table 4D (p < 0.0001). Regarding the levels of C22:6n−3 (DHA), they were reduced in animals fed with DI (high n−6/n−3 ratio) compared to mice fed with either SF or DII (low n−6/n−3 ratio), as shown in Figure 1 and Table 4E (p < 0.0001). However, no significant differences were found between the levels of DHA in animals fed with SF and those fed with DII, in agreement with the high amount of its precursor, C18:3n−3 (α-linolenic acid) in both. Despite its high concentration in diet DII, we were unable to detect any trace of 20:5n−3 (EPA), the most direct DHA biosynthetic precursor. Interestingly, the ratio between C22:6n−3 (DHA) and C22:5n−6 (DPA) was found to be significantly reduced in animals fed with DI and elevated in those fed with DII as shown in Figure 1 and Table 4F (p < 0.0001, and p = 0.0001), in correlation with their higher n−6/n−3 and lowest n−6/n−3 ratios, respectively.
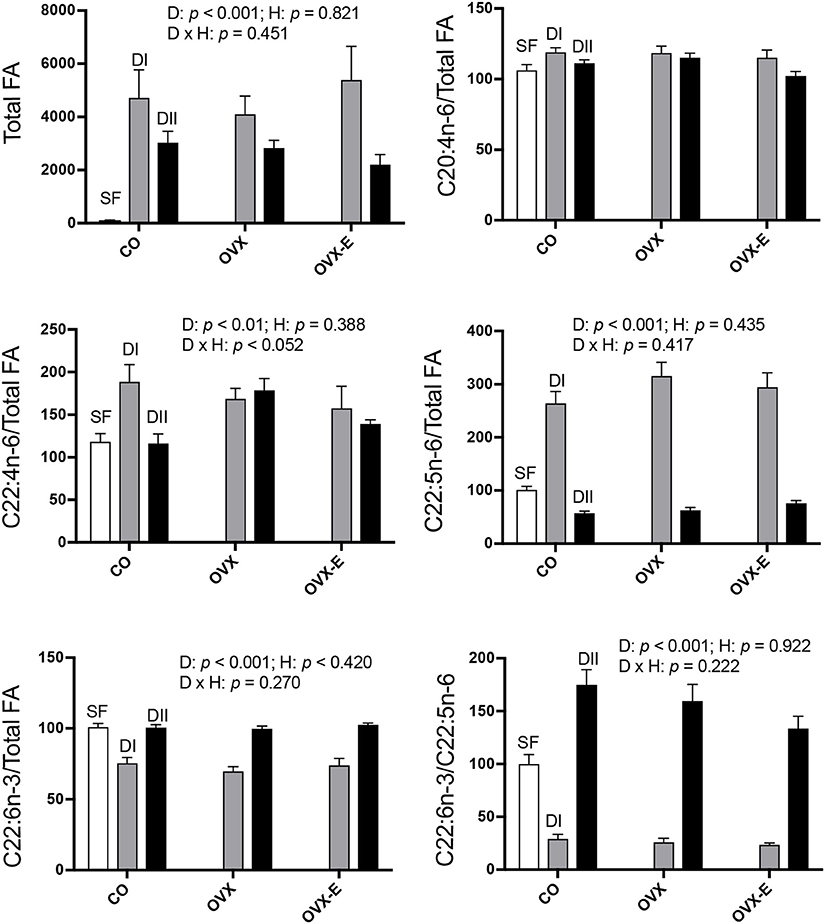
Figure 1. Differential effects of dietary LC-PUFAs on the relative cerebral cortex fatty acid content in female mice under different reproductive conditions. Fatty acid (FA) composition of each diet is shown in Table 1. The FAs from brain cortex were obtained and analyzed as described in section Materials and Methods. White bars represent the relative FA content from cerebral cortex of intact animals fed with standard food (SF). Gray and black bars represent the data from animals fed with DI or DII n−6/n−3 LC-PUFA diets, respectively. Vertical axis represents the percentage of variation induced by each diet relative to SF. Data are represented as mean ± SEM of 4 animals per group, except those fed with SF (n = 3). Horizontal axis indicates the different reproductive status: CO, intact, sham-operated; OVX, ovariectomized, placebo-treated; OVX-E, ovariectomized, estradiol-treated. Only some representative p-values for the main effects of diet (D), hormone status (H) and their interaction (D × H) were indicated in each graph and the remaining data were detailed in Table 4.
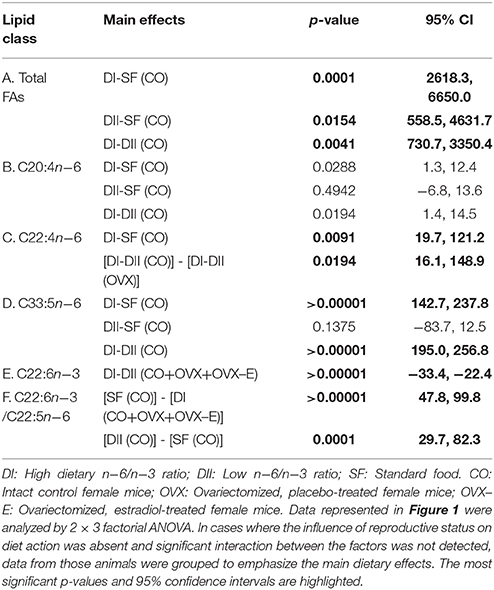
Table 4. Linear contrasts of the differential effects of dietary n−6/n−3 ratio on cerebral cortex fatty acid content under different reproductive conditions.
In addition to the effect of dietary composition on brain FA content, we studied its possible dependence on reproductive status. As shown in Figure 1, no clear effect was found in either ovariectomized or estradiol-treated mice, and only in the case of C22:4n−6 (ADA) was a nearly significant interaction detected (p = 0.052). In addition, when comparing the differential effect of DI and DII in control (CO) vs. ovariectomized (OVX) mice, a truly significant difference was found (Table 4C: p = 0.0194). Since the effect of diet was quite similar under all reproductive conditions for the rest of FAs analyzed, we grouped the data from CO, OVX, and OVX-E mice to emphasize the effect and ratio of DI and DII in the case of C22:5n−6 (DPA) and C22:6n−3 (DHA) (Tables 4E,F). It was found that, irrespective of reproductive condition, the effect of each diet on cortical levels of these LC-PUFAs correlated comparatively more with the total amount of FAs or relatively with the amount of linoleic acid in the diet (Table 2).
Results from the analysis of complex lipids are shown in Figure 2 and Table 5; for all lipids analyzed, the global effect of diet was highly significant (p ≤ 0.001–0.0001). Thus, control animals fed alternative diets had increased cerebral cortex complex lipids compared to those fed SF, although the effect was significantly, quantitatively higher in mice fed with DI (p ≤ 0.0001) than those fed with DII (p ≤ 0.02–0.003). On the other hand, though no significant effect of hormone status was observed, certain interactions between diet and reproductive condition (D × H) were detected (Figure 2), a finding that justified further comparisons of the differences between the effects of both diets under each reproductive condition (Table 5; p-values and 95% confidence intervals). In all cases, the differences between the DI and DII effects observed in control animals were also significant in ovariectomized, estradiol-treated mice, and were even more evident when data from CO and OVX-E groups were combined. In contrast, the effects of DI and DII were similar in ovariectomized, placebo-treated females and no significant differences were detected for any of the different lipids analyzed. Furthermore, when comparing the dietary effect observed in CO animals (intact ovaries) plus OVX-E animals (continuous estradiol treatment) with that of OVX mice, a reasonable level of significance was found in all cases (Tables 5A–F, p ≤ 0.025).
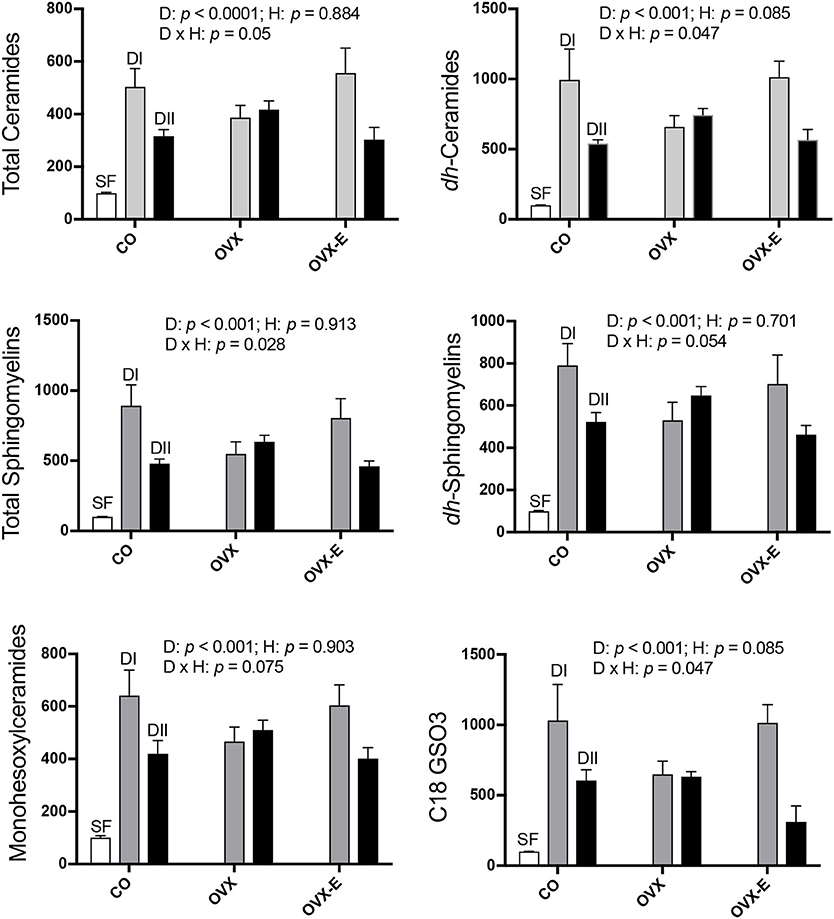
Figure 2. Differential effects of dietary LC-PUFAs on the relative complex lipid content in the cerebral cortex of female mice under different reproductive conditions. Some sphingolipids and complex lipids were obtained and analyzed as described in section Materials and Methods. White bars represent the relative lipid content from cerebral cortex of intact animals fed with standard laboratory food (SF). Gray and black bars represent the same data from animals fed with DI or DII n−6/n−3 LC-PUFA diets, respectively. Vertical axis represents the percentage of variation induced by each diet relative to SF. Data are represented as mean ± SEM of 4 animals per group, except for those fed with SF (n = 3). Horizontal axis indicates the different reproductive status: CO, intact, sham-operated; OVX, ovariectomized, placebo-treated; OVX-E, ovariectomized, estradiol-treated The data were analyzed as previously described, and only some representative p-values for the main effects of diet (D), hormone status (H) and their interaction (D × H) were indicated in each graph, the remaining data were detailed in Table 5.
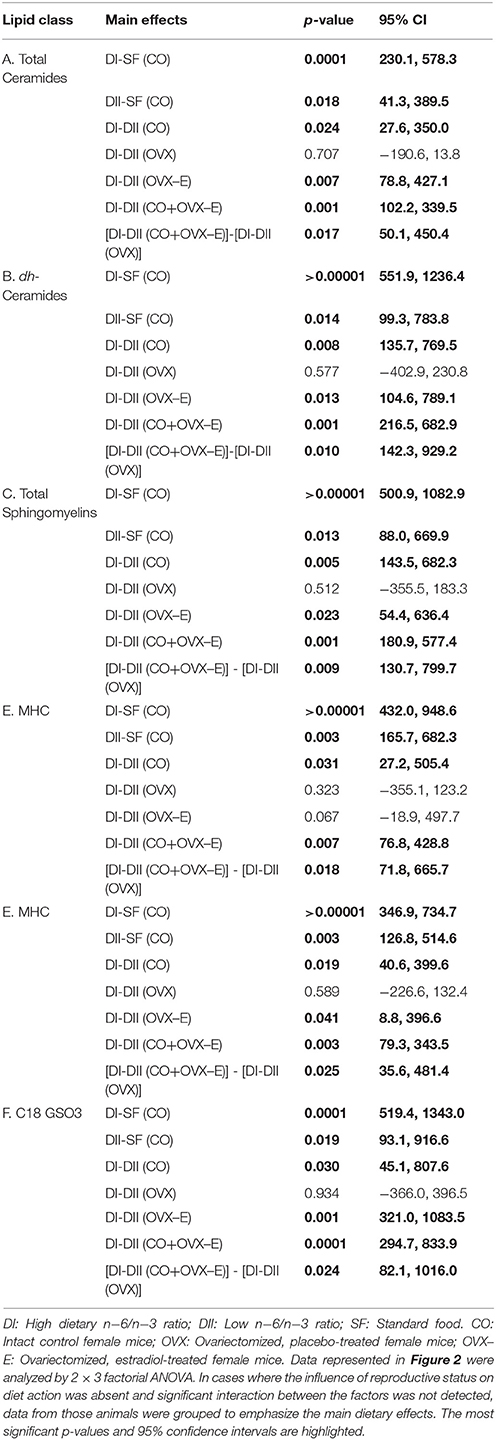
Table 5. Linear contrasts of the differential effects of dietary n−6/n−3 ratio on cerebral cortex lipid content under different reproductive conditions.
As an indication of the levels of estradiol, the dry uterine weight was determined at the endpoint. Intact females showed similar uterine weights, with independence of being fed with DI or DII (DI: 16.00 ± 2.40 mg; DII: 18.40 ± 2.00 mg). Also, OVX animals treated with estradiol showed similar uterine weights with the two diets (DI: 38.40 ± 8.00 mg; DII: 34.40 ± 3.20 mg). In contrast, OVX mice treated with placebo and fed with DI had uterine weights significantly higher than those fed with DII (DI: 8.00 ± 1.20 mg; DII: 4.00 ± 0.40 mg; p < 0.001).
In summary, these findings suggest interaction of diet and ovarian hormones on the levels of complex lipids in the mouse cerebral cortex, even though the presence or absence of ovaries and normal circulating estradiol levels do not appear to significantly modify the main effect of each diet.
The Expression of Synaptic Proteins in the Cerebral Cortex Are Affected by Dietary Differences in the Ratio of n−3 and n−6 LC-PUFAs
Brain lipids constitute a basic component of neural organization and play a critical role in the interactions of membrane proteins that are involved in cell signaling and synaptic functions (Bazan, 2014), processes that are also regulated by circulating levels of reproductive hormones and locally-synthesized steroids (Varea et al., 2009; Arevalo et al., 2015). Thus, it was interesting to explore whether differences in dietary n−6/n−3 LC-PUFA ratios affect expression and/or phosphorylation of synaptic proteins and key neuronal markers. Statistical analyses of observed changes for each protein were performed as follows: (a) the effect of differences in dietary content of LC-PUFAs in control animals; (b) the global interaction between the effect of diet and reproductive status; and (c) the specific differences between the effect of diet in controls and those observed in OVX or OVX-E mice.
CO mice fed a low n−6/n−3 ratio diet (DII) showed higher levels of both synapsin (p = 0.023) and PSD-95 (p = 0.006) than those fed with a high n−6/n−3 ratio diet (DI) (Figure 3 and Tables 6A, D). In both cases, a global interaction between diet and reproductive status was observed (p = 0.013 and p = 0.001, respectively). Significant differences were also found in the effect of diet on the levels of synapsin (Table 6A: p = 0.001 and p = 0.009) and PSD-95 (Table 6D: p = 0.0004 and p = 0.004) when intact (CO) mice were compared with ovariectomized (OVX) or ovariectomized, estradiol-treated animals (OVX-E), respectively. These findings suggest that the presence of ovaries may be necessary for the effect of dietary lipid composition on the expression of these synaptic proteins, and that continuous treatment with estradiol alone was not able to compensate for ovariectomy. In contrast, levels of p-synapsin were reduced in animals fed with a low n−6/n−3 ratio diet (DII) compared to those fed with a high n−6/n−3 ratio diet (DI), regardless of reproductive status (Figure 3 and Table 6B: p = 0.015). These differences in p-synapsin were additionally confirmed by a supplementary Western blots from the three diets (DI, DII and SF), and using PDK1 and Actin as internal controls (Supplementary Figure 1). No significant effects were found in the case of synaptophysin (Figure 3 and Table 6C), P120 or β-catenin (Tables 6E,F, respectively). The slight effect observed in synaptophysin in the case of OVX-E animals fed DII when compared to CO mice was not considered significant as it was quantitatively small, and the 95% confidence intervals did not allow further accurate interpretation of the results. Neither significant effects of diet nor reproductive status were found in the case of several neuronal markers such as BACE, PHF1, Tau1, and Tau5 (data not shown). However, CO mice fed with low n−6/n−3 ratio diet (DII) showed higher levels of APP and GFAP than those fed with high n−6/n−3 ratio diet (DI) (Figure 4 and Table 7; p = 0.003 and p < 0.0012, respectively). These effects were partially affected by reproductive status, as certain global interaction was detected, albeit of a small magnitude. Significant differences were also detected in the effect of diet on both APP and GFAP between controls and ovariectomized animals (Tables 7A,B; p = 0.018 and p = 0.029, respectively), which was partially compensated by continuous estradiol treatment.
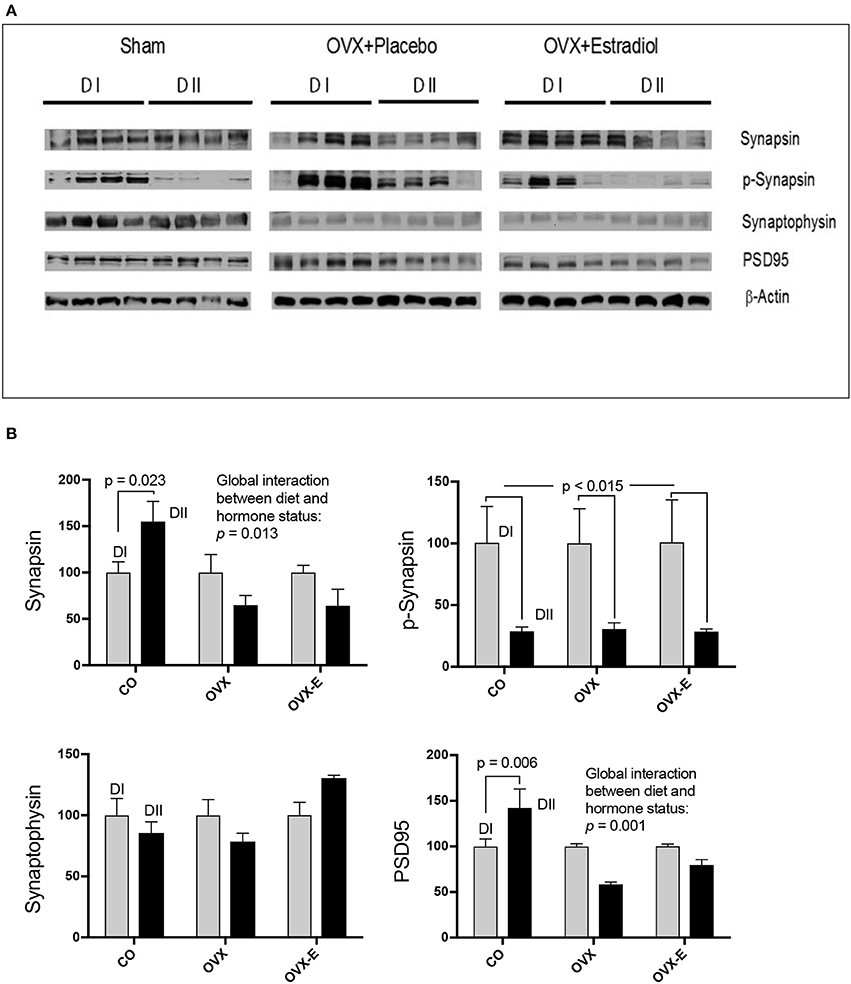
Figure 3. Differential effects of dietary LC-PUFAs on the expression of synaptic proteins (synapsin, synaptophysin, and PSD95) in the cerebral cortex of female mice under different reproductive conditions. (A) Represents the Western blots, and (B) represents means ± SEM of densitometric quantification (4 mice per group). Western blots were quantified and normalized with respect to the loading control, actin. The normalized data corresponding to intact, sham-operated mice from the DI diet was arbitrarily considered 100 relative units. Vertical axis represents the percentage of variation induced by DII vs. DI n−6/n−3 LC-PUFA ratio diet. Horizontal axis indicates the different reproductive status as follows: CO, intact, sham-operated; OVX, ovariectomized, placebo-treated; OVX-E, ovariectomized, estradiol-treated. The data were statistically analyzed as previously described, and for simplicity, only the main effects of diet are given in the figure, as well as the interaction between diet and reproductive status. Other details of the analysis including the differences between the effect of diet in controls and ovariectomized mice treated with either placebo or estradiol were presented in Table 6.
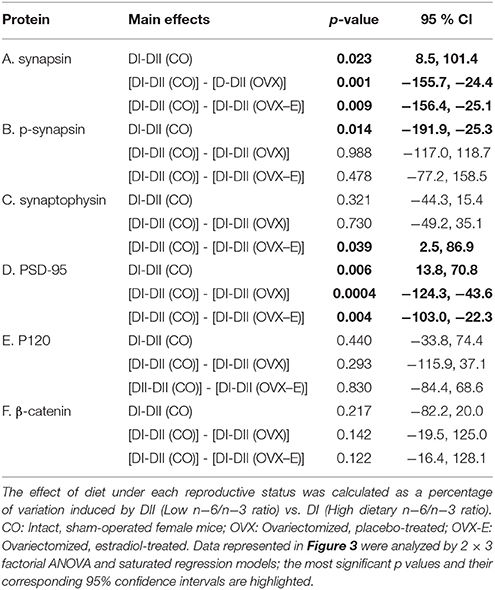
Table 6. Linear contrasts of the differential effects of dietary n−6/n−3 ratio on the expression of synaptic proteins in the cerebral cortex of female mice under different reproductive conditions.
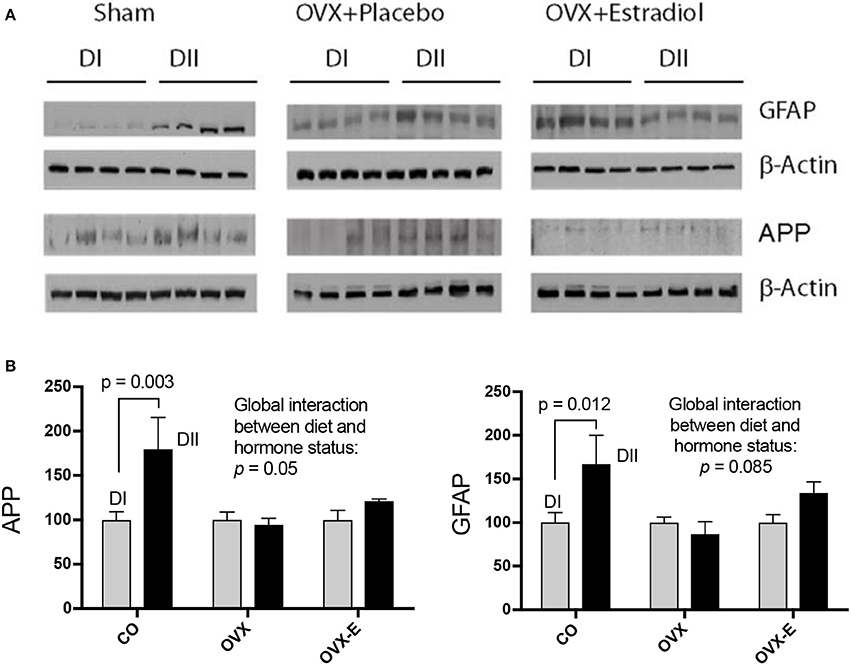
Figure 4. Differential effects of dietary LC-PUFAs on the expression of APP and GFAP in the cerebral cortex of female mice under different reproductive conditions. (A) Represent the western blots, and (B) represent the densitometric data as means ± SEM of relative densitometric quantification (4 mice per group). Vertical axis represents the percentage of variation induced by DII vs. DI n−6/n−3 LC-PUFA ratio diet. Horizontal axis indicates the different reproductive status as follows: CO, intact, sham-operated; OVX, ovariectomized, placebo-treated; OVX-E, ovariectomized, estradiol-treated. Western blots were quantified and normalized with respect to the loading control, β-Actin. The normalized data corresponding to intact, sham-operated mice from the DI diet was arbitrarily considered 100 relative units. The data were statistically analyzed as previously described, and only some relevant data are included in this Figure whereas the remaining data was presented in Table 7.
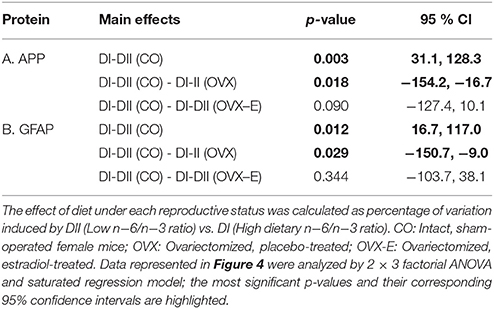
Table 7. Linear contrasts of the differential effects of dietary n−6/n−3 ratio on the expression of APP and the glial marker GFAP, in the cerebral cortex of female mice under different reproductive conditions.
Discussion
Experimental evidence has suggested that the ratio between n−6 and n−3 LC-PUFAs is a critical factor for their developmental and neuroprotective activity (Miller et al., 2016; Dyall, 2017). Therefore, the present study tested the effects of two experimental diets, DI and DII, whose major difference in composition was the global ratio of n−6 and n−3 LC-PUFAs. In addition, the two diets contained higher total levels of FA content (DI: 158%; DII: 147%) compared to the standard diet (SF), which explains the dramatic increases of total FAs observed in the cerebral cortex of mice fed with both experimental diets.
As expected, DI had increased percentages of n−6 FAs including C22:4n−6 (ADA) and C22:5n−6 (DPA), and reduced levels of C22:6n−3 (DHA). In addition, both diets caused minor elevation in C20:4n−6 (ARA) levels in the cerebral cortex, though the effect of DI was slightly higher, in agreement with the dietary content of its precursor, linoleic acid. Since ARA is a critical intermediate in the biosynthesis of thromboxanes, prostaglandins, and leukotrienes, which play an essential role in the inflammatory/anti-inflammatory response (Stables and Gilroy, 2011), some regulatory mechanisms to maintain balanced levels may exist. On the other hand, levels of DHA were higher in the cortex of mice fed with DII than in those fed with DI, in good correlation with the absence of DHA and the extremely low levels of its precursor, C18:3n−3 (ALA) in the composition of DI. Interestingly, the levels of DHA in animals fed with DII were similar to those fed with SF, despite the absence of DHA in the standard diet. However, the fact that the SF diet contains twice as much of the DHA precursor indicates that ALA-derived DHA may be sufficient to maintain brain DHA levels and preserve its function, as it has been suggested (Anderson et al., 2005; André et al., 2005). Furthermore, this interpretation is in agreement with evidence from animal models that brain DHA levels are similar when fed with diets with ALA as the only PUFA compared to those fed with DHA or ALA+DHA (reviewed in Barcelo-Coblijn and Murphy, 2009). Regarding other LC-PUFA intermediates in DHA biosynthesis, such as eicosatetranoic acid (ETA, C20:4n−3) or EPA (C20:5n−3), we did not find detectable amounts of either in brain samples, in agreement with previous reports (Miller et al., 2016; Harauma et al., 2017).
In summary, the most relevant finding regarding the effect of DI and DII on LC-PUFAs was a dramatic reduction in the ratio between C22:6n−3 and C22:5n−6 in animals fed with DI, and a marked increase in those fed with DII. However, this effect does not seem to be due to DHA enrichment in DII, but rather to the differential dietary levels of LNA (DI) and ALA (DII), in agreement with other reports (Domenichiello et al., 2016). Interestingly, no significant effects of reproductive status were detected for the dietary effects on cerebral cortex LC-PUFA levels, since the p-values obtained from the factorial analysis did not support relevant interactions between diet and gonadal conditions.
Our findings also show that the two experimental diets induced profound and highly significant elevations in the levels of all complex lipids analyzed, though for all of them the effect was stronger in animals fed a diet with the highest n−6/n−3 LC-PUFA ratio. Interestingly, the effects of DI and DII on cortical ceramide levels observed in control mice were not detected in ovariectomized mice. Furthermore, the differential effect in all complex lipids of each diet was reverted in ovariectomized animals receiving continuous estradiol treatment. These results suggest a synergistic action between dietary PUFAs and ovarian hormones on the cerebral cortex lipidome, which would be in agreement with other reports using hippocampal tissue from female mice and multivariate statistical approaches (Díaz et al., 2016).
The influence of ovarian hormones on the dietary effects on cortical ceramides is highly relevant given the important function of these lipids in a wide range of cell processes including growth, differentiation, apoptosis, and oncogenesis (Kashara and Sanai, 2000; Simons and Toomre, 2000; Anderson and Jacobson, 2002; Sengupta et al., 2007; Pruett et al., 2008). Increase in ceramide concentration in cell membranes affects not only the structural organization and dynamic properties of lipid rafts (Cremesti et al., 2002) but also myelin formation and stability (Pan et al., 2005; Susuki et al., 2007), neural differentiation (Wang and Yu, 2013; Wang et al., 2014), synapse formation (Hering et al., 2003; Mendez-Otero and Santiago, 2003), synaptic plasticity and transmission, neurotoxicity, and neurodegeneration (Hering et al., 2003; Besshoh et al., 2005; Ferrer, 2009; Fabelo et al., 2014; Attiori Essis et al., 2015; Sonnino and Prinetti, 2016; Marín et al., 2017). In addition to experimental evidence in rodents, a number of postmortem studies support the role of age-dependent or genetic alterations of ceramide and sphingolipid metabolism in several neurological disorders, including Alzheimer's (AD) and Parkinson's diseases (He et al., 2010; Fabelo et al., 2011; Gegg et al., 2012; Bouti et al., 2016; Olsen and Færgeman, 2017). However, despite the importance of ceramide and sphingolipids for brain function, the effect of dietary interventions on their levels in the cerebral cortex has not been previously explored.
In addition, neuronal lipid microdomains are also targets for estrogen hormones that act via classical estrogen receptors or membrane-associated receptors to trigger a variety of signaling pathways (Marín et al., 2003, 2005, 2007; Guerra et al., 2004). Activation of these pathways is involved in the regulation of brain development, neuronal survival and synaptic plasticity, which are the basis for the neuroprotective role of estrogens (Marín et al., 2005; Herrera et al., 2011; Arevalo et al., 2015). Therefore, dietary and estrogen-induced modification of brain sphingolipid composition may be expected to alter the expression of specific signaling and synaptic proteins. Thus, the second aim of this work was to determine whether circulating ovarian hormones could interact with the effects of dietary lipid composition, altering expression of neuronal proteins in the mouse cerebral cortex. We analyzed the levels of key neuronal proteins such as P120, PSD-95, β-catenin, synaptophysin, and synapsin. Though no significant effects were detected on the expression of P120, β-catenin, or synaptophysin, both PSD-95 and synapsin were found to be sensitive to the different dietary n−6/n−3 ratios. In addition, the effect of diet was blocked by ovariectomy, suggesting a potential synergy between diet and reproductive hormones at both presynaptic and postsynaptic levels.
Synapsins are a family of presynaptic proteins that interact with synaptic vesicles through phosphorylation-dependent processes (Chi et al., 2003; Cousin et al., 2003; Menegon et al., 2006; Sun et al., 2006; Giachello et al., 2010; Messa et al., 2010). In this work, a diet with a low n−6/n−3 LC-PUFA ratio (with relatively high levels of DHA and EPA) induced higher expression of synapsin, but lower levels of p-synapsin (Ser-9) than a diet with a higher n−6/n−3 ratio (lacking supplemental DHA and EPA). Interestingly, regarding total synapsin, it was found that ovariectomy blocked the dietary effect, and that continuous estradiol-treatment neither reverted nor prevented the effect of ovariectomy, suggesting that, in addition to estradiol, other ovarian secretions might be involved. In this respect, estrogen-progesterone interactions in the modulation of synaptic plasticity and the expression of both pre-and post-synaptic proteins in several brain regions of monkeys and rodents have been reported (Choi et al., 2003; Foy et al., 2008; Williams et al., 2011; Baudry et al., 2013). On the contrary, the profound dietary effect observed for p-synapsin was not influenced by ovariectomy. It should be pointed out that synapsin phosphorylation is a short-term regulated event at the level of presynaptic terminals which depends on action potential firing, activation dynamics of highly localized voltage-dependent calcium channels, and a complex interplay between several Ca++-dependent protein kinases (Chi et al., 2003; Menegon et al., 2006; Sun et al., 2006; Giachello et al., 2010). Thus, protein-lipid interactions at the level of presynaptic membranes can be expected to depend on dietary lipid composition and being additionally influenced by ovarian function. However, rapid modulation of short-term presynaptic phenomena, such as those involved in p-synapsin phosphorylation and its potential dependence on ovarian hormone levels, must be difficult to be observed under our experimental approach.
PSD-95 is an integral component of the postsynaptic density that participates in neurotransmission (Cho et al., 1992; Kim and Sheng, 2004; Berry and Nedivi, 2017), synaptic plasticity and learning (Migaude et al., 1998; EI-Husseini et al., 2000; Schnell et al., 2002; Ehrlich and Malonow, 2004; Ehrlich et al., 2007). In intact female mice, we found that a diet with a low n−6/n−3 LC-PUFA ratio (enriched in DHA and EPA) induced higher expression levels of PSD-95 in the cerebral cortex than a diet with a higher n−6/n−3 ratio (lacking DHA or EPA enrichment). This is in agreement with previous reports of reduced PSD-95 expression in aged mice fed with DHA-deficient diets (Sidhu et al., 2016). This effect was not observed in ovariectomized animals, indicating that circulating ovarian hormones might interact with brain dietary inputs for regulation of the synaptic proteome. However, administration of estradiol alone was unable to either revert or prevent the effect of ovariectomy. Even though we cannot reach clear conclusions from these findings, they suggest that, in addition to estradiol, oscillations in other cyclic ovarian secretions may play a physiological role in the modulation of brain cortex synaptic proteins by dietary differences in n−6/n−3 ratios. In this respect, as mentioned above, there are several reports supporting the potential effect of estrogen-progesterone interactions on synaptic plasticity and post-synaptic proteins (Foy et al., 2008; Baudry et al., 2013; for review see Williams et al., 2011).
The second group of proteins analyzed were those involved in some neurodegenerative pathologies like AD and other taupathies, such as APP, Tau, BACE or GFAP (for review see Laird et al., 2005; LaFerla and Green, 2012; Rosenberg et al., 2016). Our data indicate that only APP and GFAP were differentially expressed in the cerebral cortex of female mice fed with either DI or DII. In both cases, ovariectomy blocked the differential effect of DII, even though only a slight estradiol-induced reversion was observed. It is tantalizing to suggest that the increase of APP expression induced by DII over DI might be a positive response to certain stress, influencing the balance of neuronal-glia interactions. It will be interesting to further studying the effects of these dietary and reproductive manipulations in specific mouse models of neurodegenerative disorders.
The results of the current work indicate that different dietary n−6/n−3 LC-PUFA ratios are able to remodel the lipidome in the cerebral cortex of female mice, and that these effects are partially influenced by the circulating levels of ovarian hormones. A variety of results from animal models of neurodegenerative diseases have suggested that dietary interventions may be useful therapeutic tools against several neurodegenerative disorders (Lim et al., 2005; Green et al., 2007; Bazan et al., 2011a,b; Bazan, 2014). However, the potential for these therapeutic approaches in human health and disease is still unclear, and the current dietary recommendations have been considered specific to particular age groups and physiological conditions (Zárate et al., 2017). The interactions between the effect of dietary lipid composition and reproductive status shown here indicate the importance the specific n−6/n−3 composition in therapeutic diets, which must also be well-balanced in regards to the hormonal context and physiological situations of age-associated impairment of reproductive function, such as menopause.
Author Contributions
JH performed the animal experiments and was in charge of most experimental manipulations, hormone treatments, and collection of brain samples; JH and LO-G prepared brain samples and western blotting analysis; AM and GH participated in experimental designs, animal treatments, and collection of samples; GF and JC performed the lipidomic analysis of brain samples; NA and CR analyzed the lipid composition of the experimental diets; CR collaborated in the interpretation of results; LP-V performed statistical analysis of quantitative results; RA conceived and designed the experiments and contributed together with LP-V to the design of the specific statistical analysis; LG-S, RA, and FW were in charge of the final interpretation of results and wrote the paper.
Funding
RA was supported in part by SAF2007-66148-C02-01 & FP7-REGPOT-2012-31637-IMBRAIN grants. LG-S was supported by BFU2014-51836-C2-1-R grant, CIBERFES and Fondos Feder. FW was supported by grants from the MICINN (SAF2012-39148-C03-0), the European Union (EU-FP7-2009-CT222887), CAM (B2017/BMD-3700), Centro de Investigación Biomédica en Red sobre Enfermedades Neurodegenerativas (CIBERNED), CIBERNED Proyectos Colaborativos (PI2016/01), and by an institutional grant from the Fundación Ramón Areces to CBMSO and Fondos FEDER.
Conflict of Interest Statement
The authors declare that the research was conducted in the absence of any commercial or financial relationships that could be construed as a potential conflict of interest.
Acknowledgments
The authors gratefully acknowledge Mario Diaz (Universidad de La Laguna) and Emilio Martinez de la Victoria (Instituto de Nutrición y Tecnología de los Alimentos, Universidad de Granada) for their advice in the design of experimental diets, and Maria Rosa Arnaiz for her help in the management of mice in the University of La Laguna animal facility. The antibody PHF-1 was generously provided by Prof. P. Davies (The Feinstein Institute for Medical Research, NY, USA).
Supplementary Material
The Supplementary Material for this article can be found online at: https://www.frontiersin.org/articles/10.3389/fncel.2018.00103/full#supplementary-material
References
Anderson, G. J., Neuringer, M., Lin, D. S., and Connor, W. E. (2005). Can prenatal N−3 fatty acid defficiency be completely reversed after birth? Effects on retinal and brain biochemistry and visual function in Rhesus monkeys. Pediatr. Res. 58, 865–872. doi: 10.1203/01.pdr.0000182188.31596.5a
Anderson, R. G., and Jacobson, K. (2002). A role for lipid shells in targetting proteins to caveolae, rafts, and other lipid domains. Science 296, 1821–1825. doi: 10.1126/science.1068886
André, A., Juanéda, P., Sébédio, J. L., and Chardigny, J. M. (2005). Effects of aging and dietary n−3 fatty acids on rat brain phospholipids: focus on plasmalogens. Lipids 40, 799–806. doi: 10.1007/s11745-005-1441-x
Arevalo, M. A., Azcoitia, I., and Garcia-Segura, L. M. (2015). The neuroprotective actions of oestradiol and oestrogen receptors. Nat. Rev. Neurosci. 16, 17–29. doi: 10.1038/nrn3856
Armitage, P., Berry, G., and Mathews, J. N. S. (2004). Statistical Methods in Medical Research. London: Wiley-Blackwell.
Attiori Essis, S., Laurier-Laurin, M. E., Pépin, É., Cyr, M., and Massicote, G. (2015). GluN2B-containing NMDA receptors are upregulated in plasma membranes by the sphingosine-1-phosphate analog FTY720P. Brain Res. 1624, 349–358. doi: 10.1016/j.brainres.2015.07.055
Bannenberg, G., and Serhan, C. N. (2010). Specialized pro-resolving lipid mediators in the inflamatory response: an update. Biochem. Biophys. Acta 1891, 1260–1273. doi: 10.1016/j.bbalip.2010.08.002
Barcelo-Coblijn, G., and Murphy, E. J. (2009). Alpha-linoleic acid and its conversion to longer chain n−3 fatty acids: benefits for human health and a role in maintaining tissue n−3 fatty acid levels. Prog. Lipid Res. 48, 355–374. doi: 10.1016/j.plipres.2009.07.002
Baudry, M., Xiaoning, B., and Aguirre, C. (2013). Progesterone-estrogen interactions in synaptic plasticity and neuroprotection. Neuroscience 239, 280–294. doi: 10.1016/j.neuroscience.2012.10.051
Bazan, N. G. (2014). Is there a molecular logic that sustains neuronal functional integrity and survival?: lipid signaling is necessary for neuroprotective neuronal transcriptional programs. Mol. Neurobiol. 50, 1–5. doi: 10.1007/s12035-014-8897-0
Bazan, N. G., Calandria, J. M., and Gordon, W. C. (2013). Docosahexanoic acid and its derivative neuroprotectin D1 display neuroprotective properties in the retina, brain and central nervous system. Nestle Nutr. Inst. Workshop Ser. 77, 121–131. doi: 10.1159/000351395
Bazan, N. G., Molina, M. F., and Gordon, W. C. (2011a). Docosahexaenoic acid signalolipidomics in nutrition: significance in aging, neuroinflamation, macular degeneration, Alzheimer's, and other neurodegenerative diseases. Ann. Rev. Nutr. 31, 321–351. doi: 10.1146/annurev.nutr.012809.104635
Bazan, N. G., Musto, A. E., and Knott, E. J. (2011b). Endogenous signaling by omega-3 docosahexanoic acid-derived mediators sustains homeostatic synaptic and circuitry integrity. Mol. Neurobiol. 44, 216–222. doi: 10.1007/s12035-011-8200-6
Bazinet, R. P., and Layé, S. (2014). Polyunsaturated fatty acids and their metabolites in brain function and disease. Nat. Rev. Neurosci. 15, 771–785. doi: 10.1038/nrn3820
Berry, K. P., and Nedivi, E. (2017). Spine dynamics: are they all the same? Neuron 27, 43–55. doi: 10.1016/j.neuron.2017.08.008
Besshoh, S., Bawa, D., Teves, L., Wallace, M. C., and Gurd, J. W. (2005). Increased phosphorylation and redistribution of NMDA receptors between synaptic lipid rafts and postsynaptic densities following transient global ischemia in the rat brain. J. Neurochem. 93, 186–194. doi: 10.1111/j.1471-4159.2004.03009.x
Bourre, J. M., Pascal, G., Durand, G., Masson, M., Dumont, O., and Piciotti, M. (1984). Alterations in the fatty acid composition of rat brain cells (neurons, astrocytes, and oligodendrocytes) and of subcellular fractions (myelin ans synaptosomes) induced by a diet devoid of n−3 fatty acids. J. Neurochem. 43, 342–348. doi: 10.1111/j.1471-4159.1984.tb00906.x
Bouti, M., Sun, Y., Shacka, J. J., and Auray-Blais, C. (2016). Tandem mass spectometry multiplex analysis of glucoceramide and galactosyceramide isoforms in brain tissues at different stages of Parkinson disease. Anal Chem. 88, 1856–1863. doi: 10.1021/acs.analchem.5b04227
Brenna, J. T., and Diau, G.-Y. (2007). The influence of dietary docosahexanoic acid and arachdonic acid on central nervous system polyunsaturated fatty acid composition. Prostaglandins Leukot. Essent. Fatty Acids. 77, 247–250. doi: 10.1016/j.plefa.2007.10.016
Carrié, I., Clément, M., de Javel, D., Francès, H., and Bourre, J.-M. (2000). Specific phospholipid fatty acid composition of brain regions in mice: effects of n−3 polyunsaturated fatty acid deficiency and phospholipid supplementation. J. Lipid Res. 41, 465–472.
Catalan, J., Moriguchi, T., Slotnick, B., Murthy, M., Greiner, R. S., and Salem, N. Jr (2002). Cognitive deficits in docosahexanoic acid-deficiency rats. Behav. Neurosci. 116, 1022–1031. doi: 10.1037/0735-7044.116.6.1022
Chen, C. T., Liu, Z., Ouellet, M., Calon, F., and Bazinet, R. P. (2009). Rapid beta-oxidation of eicosapentanoic acid in mouse brain: an in situ study. Prostaglandins Leukot. Essent. Fatty Acids. 80, 157–163. doi: 10.1016/j.plefa.2009.01.005
Chi, P., Greengard, P., and Ryan, T. A. (2003). Synaptic vesicle mobilization is regulated by distinct synapsin I phosphorylation pathways at different frequencies. Neuron 38, 69–78. doi: 10.1016/S0896-6273(03)00151-X
Cho, K. O., Hunt, C. A., and Kennedy, M. B. (1992). The rat brain postsynaptic density fraction contains a homolog of the Drosophila discs-large tumor suppressor protein. Neuron 9, 929–942. doi: 10.1016/0896-6273(92)90245-9
Choi, J. M., Romeo, R. D., Brake, W. G., Bethea, C. L., Rosenwaks, Z., and McEwen, B. S. (2003). Estradiol increases pre- and post-synaptic proteins in the CA1 region of the hippocampus in female Rhesus macaques (Macaca mulata). Endocrinology 144, 4734–4738. doi: 10.1210/en.2003-0216
Cingolani, F., Casasampere, M., Sanllehi, P., Casas, J., Bujons, J., and Fabrias, G. (2014). Inhibition of dihydroceramide desaturase activity by the sphingosine kinase inhibitor SKII. J. Lipid Res. 55, 1711–1720. doi: 10.1194/jlr.M049759
Cousin, M. A., Maladi, C. S., Tan, T. C., Raymond, C. R., Smillie, K. J., and Robinson, P. J. (2003). Synapsin I-associated phosphatidylinositol 3-kinase mediates synaptic vesicle delivery to the readily releasable pool. J. Biol. Chem. 278, 29065–29071. doi: 10.1074/jbc.M302386200
Cremesti, A. E., Goni, F. M., and Kolesnick, R. (2002). Role of sphingomyelinase and ceramide in regulationg rafts: do biophysical properties determine biologic outcomes? FEBS Lett. 531, 47–53. doi: 10.1016/S0014-5793(02)03489-0
Díaz, M., Fabelo, N., Casañas-Sánchez, V., Marin, R., Gómez, T., Quinto-Alemany, D., et al. (2016). Hippocampal lipid homeostasis in APP/PS1 mice is modulated by a complex interplay between dietary DHA and estrogens: relevance for Alzheimer's disease. J. Alzheimers Dis. 49, 459–481. doi: 10.3233/JAD-150470
Domenichiello, A. F., Kitson, A. P., Chen, C. T., Trépanier, M. O., Stavro, P. M., and Bazinet, R. P. (2016). The effect of linoleic acid on the whole body synthesis rates of polyunsaturated fattu acids from α-linolenic and linoleic acid in free-living rats. J. Nutr. Biochem. 30, 167–176. doi: 10.1016/j.jnutbio.2015.11.016
Dyall, S. C. (2017). Interplay between n−3 and n−6 long-chain polyunsaturated fatty acids and the endocannabinoid system in brain protection and repair. Lipids 52, 885–900. doi: 10.1007/s11745-017-4292-8
Dyall, S. C., and Michael-Titus, A. T. (2008). Neurological benefits of omega-3 fatty acids. Neuromol. Med. 10, 219–235. doi: 10.1007/s12017-008-8036-z
Ehrlich, I., Klein, M., Rumpel, S., and Malinow, R. (2007). PSD-95 is required for activity-driven synapse stabilization. Proc. Natl. Acad. Sci. U.S.A. 104, 4176–4181. doi: 10.1073/pnas0609307104
Ehrlich, I., and Malonow, R. (2004). Postsynaptic density 95 controls AMP receptor incorporation during long-term potentiation and experienced-driven synaptic plasticity. J. Neurosci. 24, 916–927. doi: 10.1523/JNEUROSCI.4733-03.2004
EI-Husseini, A. E., Schnell, E., Chetkovich, D. M., Nicoll, R. A., and Bredt, D. S. (2000). PSD-95 involvement in maduration of excitatory synapses. Science 290, 1364–1368.
Fabelo, N., Martín, V., Marín, R., Moreno, D., Ferrer, I., and Díaz, M. (2014). Altered lipid composition in cortical lipid rafts occurs at early stages of sporadic Alzheimer's diseas and facilitates APP/BACE1 interactions. Neurobiol. Aging. 35, 1801–1812. doi: 10.1016/j.neurobiolaging.2014.02.005
Fabelo, N., Martin, V., Santpere, G., Marín, R., Torrent, L., Ferrer, I., et al. (2011). Severe alterations in lipid composition of frontal cortex lipid rafts from Parkinson's disease and incidental Parkinson's disease. Mol. Med. 17, 1107–1118. doi: 10.2119/molmed.2011.00119
Ferrer, I. (2009). Altered mitochondria, energy metabolism, voltage-dependent anion channel, and lipid rafts converge to exaust neurons in Alzheimer's disease. J. Bioenerg. Biomembr. 41, 425–431. doi: 10.1007/s10863-009-9243-5
Folch, J., Lees, M., and Sloane Stanley, G. H. (1957). A simple method for the isolation and purification of total lipids from animal tissues. J. Biol. Chem. 226, 497–509.
Foy, M. R., Akopian, G., and Thompson, R. F. (2008). Progesterone regulation of synaptic transmission and plasticity in rodent hippocampus. Learn. Mem. 15, 820–822. doi: 10.1101/lm.1124708
Garanto, A., Mandal, N. A., Egido-Gabás, M., Marfany, G., Fabriàs, G., Anderson, R. E., et al. (2013). Specific spjingolipid content decrease in Cerkl knockdown mouse retinas. Exp. Eye Res. 110, 96–106. doi: 10.1016/j.exer.2013.03.003
Gegg, M. E., Burke, D., Heales, S. J., Cooper, J. M., Hardy, J., Wood, N. W., et al. (2012). Glucocerebrosidade deficiency in substantia nigra of Parkinson disease brains. Ann. Neurol. 72, 455–463. doi: 10.1002/ana.23614
Giachello, C. N., Fiumara, F., Giacomini, C., Corradi, C., Milanese, C., Ghirardi, M., et al. (2010). MAPK/Erk-dependent phosphorylation of synapsin mediates formation of functional synapses and short-term homosynaptic plasticity. J. Cell Sci. 123, 881–893. doi: 10.1242/jcs.056846
Gorjão, R., Acevedo-Martins, A. K., Rodrigues, H. G., Abdulkader, F., Arcisio-Miranda, M., Procopio, J., et al. (2009). Comparative effects of DHA and EPA on cell function. Pharmacol. Ther. 122, 56–64. doi: 10.1016/j.pharmthera.2009.01.004
Green, K. N., Martinez-Coria, H., Khahwji, H., Hall, E. B., Yurko-Mauro, K. A., Ellis, L., et al. (2007). Dietary docosahexaenoic acid and docosapentaenoic acid ameliorate amyloid-βand tau pathology via a mechanism involving presenelin 1 levels. Neurobiol. Dis. 27, 4385–4395. doi: 10.1523/JNEUROSCI.0055-07.2007
Guerra, B., Díaz, M., Alonso, R., and Marín, R. (2004). Plasma membrane oestrogen receptor mediates neuroprotection against β-amyloid toxicity through activation of Raf-1/MEK/ERK cascade. J. Neurochem. 91, 99–109. doi: 10.1111/j.1471-4159.2004.02695.x
Harauma, A., Yasuda, H., Hatanaka, E., Nakamura, M., Salem, N. Jr, and Moriguchi, T. (2017). The essentiality of arachidonic acid in addition to docosahexaenoic acid for brain growth and function. Prostaglandins Leukot. Essent. Fatty Acids 116, 9–18. doi: 10.1016/j.plefa.2016.11.002
He, X., Huang, Y., Li, B., Gong, C. X., and Schuchman, E. H. (2010). Deregulation of sphingolipid metabolism in Alzheimer's disease. Neurobiol. Aging 31, 398–408. doi: 10.1016/j.neurobiolaging.2008.05.010
Hering, H., Lin, C. C., and Sheng, M. (2003). Lipid rafts in the maintenance of synapses, dendritic spines, and surface AMPA receptor stability. J. Neurosci. 23, 3262–3271.
Herrera, J. L., Díaz, M., Hernández-Fernaud, J. R., Salido, E., Alonso, R., Fernandez, C., et al. (2011). Voltage-dependent anion channel (VDAC) as a resident protein of lipid rafts: post-transductional regulation by estrogens and involvement in neuronal preservation against Alzheimer's disease. J. Neurochem. 116, 820–827. doi: 10.1111/j.1471-4159.2010.06987.x
Ikemoto, A., Ohishi, M., Sato, Y., Hata, N., Misawa, Y., Fujii, Y., et al. (2001). Okuyama, H. Reversibility of n−3 fatty acid deficiency-induced alterations of learning nehaviour in the rat: level of n−6 fatty acids as another critical factor. Lipid Res. 42, 1655–1663.
Jumpsen, J., Elien, E., Goh, Y., and Clandinin, M. (1997). Small changes of dietary (n−6) and (n−3)/fatty acid content ratio alter phosphatidylethanolamine and phosphatidylcholine fatty acid composition during development of neuronal and glial cells in rats. J. Nutr. 127, 724–731. doi: 10.1093/jn/127.5.724
Kashara, K., and Sanai, Y. (2000). Functional roles of glycospingolipids in signal transduction via lipid rafts. Glyconj J. 17, 153–162. doi: 10.1023/A:1026576804247
Kim, E., and Sheng, M. (2004). PDZ domain proteins of synapses. Nat. Rev. Neurosci. 5, 771–781. doi: 10.1038/nrn1517
LaFerla, F. M., and Green, K. N. (2012). Animal models of Alzheimer disease. Cold Spring Harb. Perspect. Med. 11:a005329. doi: 10.1101/cshperspect.a006320
Laird, F. M., Huaibi, C., Savonenko, A. V., Farah, M. H., He, K., Melnikova, T., et al. (2005). BACE1, a major determinant of selective vulnerability of the brain to amlyoid-β amyloidogenesis, is essential for dognitive, emotional, and synaptic function. J. Neurosci. 25, 111693–111709. doi: 10.1523/JNEUROSCI.2766-05.2005
Lim, G. P., Calon, F., Morihara, T., Yang, F., Teter, B., Ubeda, O., et al. (2005). A diet enriched with the omega-3 fatty acid docosahexaenoic acid reduces amyloid burden in an aged Alzheimer mouse model. J. Neurosci. 25, 3032–3040. doi: 10.1523/JNEUROSCI.4225-04.2005
Marín, R., Casañas, V., Pérez, J. A., Fabelo, N., Fernández, C. E., and Díaz, M. (2013). Oestrogens as modulators of neuronal signalosomes and brain lipid homeostasis related to protection against neurodegeneration. J. Neuroendocrinol. 25, 1104–1115. doi: 10.1111/jne.12068
Marín, R., Fabelo, N., Martín, V., García-Esparcia, P., Ferrer, I., Quinto-Alemany, D., et al. (2017). Anomalies occurring in lipid profiles and protein distribution in frontal cortex lipid rafts in dementia with Lewy bodies disclose neurochemical traits partially shared by Alzheimer's and Parkinson's disease. Neurobiol. Aging. 49, 52–59. doi: 10.1016/j.neurobiolaging.2016.08.027
Marín, R., Guerra, B., Alonso, R., Ramírez, C. M., and Díaz, M. (2005). Estrogen activates classical and alternative mechanisms to orchestrate neuroprotection. Curr. Neurovasc. Res. 2, 287–301. doi: 10.2174/156720205774322629
Marín, R., Guerra, B., Morales, A., Diaz, M., and Alonso, R. (2003). An estrogen membrane receptor participates in the neuroprotective action of estradiol against amyloid β-peptide1−40-induced toxicity in SN56 neurons. J. Neurochem. 85, 1180–1189. doi: 10.1046/j.1471-4159.2003.01767.x
Marín, R., Ramírez, C., Morales, A., González, M., González-Muñoz, E., Zorzano, A., et al. (2007). Voltage-dependent anion channel (VDAC) participates in amiloid beta-induced toxicity and interacts with plasma membrane estrogen receptor α in septal and hippocampal neurons. Mol. Membr. Biol. 24, 148–160. doi: 10.1080/09687860601055559
Mazza, M., Pomponi, M., Janiri, L., Bria, P., and Mazza, S. (2007). Omega-3 fatty acids and antioxidant in neurological and psychiatric diseases: an overview. Progr. Neuropsychopharmacol. Biol. Psychiatr. 31, 12–26. doi: 10.1016/j.pnpbp.2006.07.010
Mendez-Otero, R., and Santiago, M. F. (2003). Functional role of a specific ganglioside in neuronal migration and neurite outgrowth. Braz. J. Med. Biol. Res. 36, 1003–1013. doi: 10.1590/S0100-879X2003000800006
Menegon, A., Bonanomi, D., Albertinazzi, C., Lotti, F., Ferrari, G., Kao, H. T., et al. (2006). Protein kinase A-mediated synapsin I phosphorylation is a central modulator of Ca2+-dependent synapticactivity. J. Neurosci. 26, 11670–11681. doi: 10.1523/JNEUROSCI.3321-06.2006
Messa, M., Congia, S., Defranchi, E., Valtorta, F., Fassio, A., Onofri, F., et al. (2010). Benfenati, F. Tyrosine phosphorylation of synapsin I by Src regulates synaptic-vesicle trafficking. J. Cell Sci. 123, 2256–2265. doi: 10.1242/jcs.068445
Metherel, A. H., Armstrong, J. M., Patterson, A. C., and Stark, K. D. (2009). Assesment of blood measurements of n−3 polyunsaturated fatty acids with acute fish oil supplementation and washout in men and women. Prostaglandins Leukot. Essent. Fatty Acids 81, 23–29. doi: 10.1016/j.plefa.2009.05.018
Migaude, M., Charlesworth, P., Dempster, M., Webster, L. C., Watabe, A. M., Makhinson, M., et al. (1998). Enhanced long-term potentiation and impaired learning in mice with mutant postsynaptic density-95 protein. Nature 396, 433–439. doi: 10.1038/24790
Miller, L. R., Jorgensen, M. J., Kaplan, J. R., Seeds, M. C., Rahbar, E., Morgan, T. M., et al. (2016). Alterations in levels and ratios of n−3 and n−6 polyunsaturated fatty acids in the temporal cortex and liver of Vernet monkeys from brith to adulhood. Physiol. Behav. 156, 71–78. doi: 10.1016/j.physbeh.2015.12.009
Mozaffarian, D., and Wu, J. H. Y. (2012). (n−3) fatty acids and cardiovascular health: are effects of EPA and DHA shared or complementary? J. Nutr. 142, 614S–625S. doi: 10.3945/jn.111.149633
Olsen, A. S. B., and Færgeman, N. J. (2017). Sphingolipids: membrane microdomains in brain development, function and neurological diseases. Open Biol. 7:170069. doi: 10.1098/rsob.170069
Orr, S. K., Palumbo, S., Bosetti, F., Mount, H. T., Kang, J. X., Greenwood, C. E., et al. (2013). Unesterified docosahexaenoic acid is protective in neuroinflammation. J. Neurochem. 213, 378–393. doi: 10.1111/jnc.12392
Pan, B., Frombolt, S. E., Hess, E. J., Crawford, T. O., Griffin, J. W., Sheikh, K. A., et al. (2005). Myelin–associated glycoproteins and complementary axonal ligands, gangliosides, mediate axonal stability in the CNS and PNS: neuropathology and behavioral deficits in single- and double-null mice. Exp. Neurol. 195, 208–217. doi: 10.1016/j.expneurol.2005.04.017
Pruett, S. T., Bushnev, A., Hagedorn, K., Adiga, M., Haynes, C. A., Sullards, M. C., et al. (2008). Biodiversity of sphingoid bases (“sphingosines”) and related aminoalcoholes. Lipid Res. 49, 1621–1639. doi: 10.1194/jlr.R800012-JLR200
Rosenberg, R. N., Lambracht-Washington, D., Yu, G., and Xia, W. (2016). Genomics of Alzheimer disease: a review. JAMA Neurol. 73, 867–874. doi: 10.1001/jamaneurol.2016.0301
Russell, F. D., and Bürgin–Maunder, C. S. (2012). Distinguishing health benefits of eicosapentaenoic and docosahexaenoic acids. Mar. Drugs 10, 2535–2559. doi: 10.3390/md10112535
Schnell, E., Sizemore, M., Karimzadegan, S., Chen, L., Bredt, D. S., and Nicholl, R. A. (2002). Direct interaction betweeb PSD-95 and stargazing control synaptic AMPA receptor number. Proc. Natl. Acad. Sci. U.S.A. 99, 13902–13907. doi: 10.1073/pnas.172511199
Sengupta, P., Barird, B., and Holowka, D. (2007). Lipid rafts, fluid/fluid phase separation, and their relevance to plasma membrane structure and function. Semin. Cell. Dev. Biol. 18, 583–590. doi: 10.1016/j.semcdb.2007.07.010
Serhan, C. N. (2014). Pro-resolving lipid mediators are leads for resolution physiology. Nature 510, 92–101. doi: 10.1038/nature13479
Serhan, C. N., and Chang, N. (2013). Resolution phase lipid mediators of inflamation: agonists of resolution. Curr. Opin. Pharmacol. 13, 632–640. doi: 10.1016/j.coph.2013.05.012
Sidhu, V. K., Huang, B. X., Desai, A., Kevala, K., and Kim, H.-Y. (2016). Role of DHA in aging-related change in mouse brain synaptic plasma membrane proteome. Neurobiol. Aging 41, 73–85. doi: 10.1016/j.neurobiolaging.2016.02.007
Simons, K., and Toomre, D. (2000). Lipid rafts and signal transduction. Nat. Rev. Mol. Cell. Biol. 1, 31–39. doi: 10.1038/35036052
Sonnino, S., and Prinetti, A. (2016). The role of sphingolipids in neural plasticity of the brain. J. Neurochem. 137, 485–488. doi: 10.1111/jnc.13589
Stables, M. J., and Gilroy, D. W. (2011). Old and new generation lipid mediators in acute inflammation and resolution. Progr. Lipid Res. 50, 35–51. doi: 10.1016/j.plipres.2010.07.005
Sun, J., Bronk, P., Liu, X., Han, W., and Sudhof, T. C. (2006). Synapsins regulate use-dependent synaptic plasticity in the calyx of Held by a Ca2+/calmodulin–dependent pathway. Proc. Natl. Acad. Sci. U.S.A. 103, 2880–2885. doi: 10.1073/pnas.0511300103
Susuki, K., Baba, H., Tohyama, K., Kanai, K., Kuwabara, S., Hirata, K., et al. (2007). Gangliosides contribute to stability of paranodal junctions and ion channel clusters in myelinated nerve fibers. Glia 55, 746–757. doi: 10.1002/glia.20503
Varea, O., Garrido, J. J., Dopazo, A., Mendez, P., Garcia-Segura, L. M., and Wandosell, F. (2009). Estradiol activates beta-catenin dependent transcription in neurons. PLoS ONE 4:e5153. doi: 10.1371/journal.pone.0005153
Wang, J., Cheng, A., Wakade, C., and Yu, R. K. (2014). Ganglioside GD3 is required for neurogenesis and long-term maintenance of neural stem cells in the postnatal mouse brain. J. Neurosci. 34, 13790–13800. doi: 10.1523/JNEUROSCI.2275-14.2014
Wang, J., and Yu, R. K. (2013). Interaction of ganglioside GD3 with n EGF receptor sustains the self-renewal ability of mouse neural stem cells in vitro. Proc. Natl. Acad. Sci. U.S.A. 110, 137–142. doi: 10.1073/pnas.1307224110
Williams, T. J., Mitterling, K. L., Thompson, L. I., Torres-Reveron, A., Waters, E. M., McEwen, B. S., et al. (2011). Age- and hormone-regulation of opiod peptides and synaptic proteins in the rat dorsal hippocampal formation. Brain Res. 1379, 71–85. doi: 10.1016/j.brainres.2010.08.103
Williard, D. E., Harmon, S. D., Kaduce, T. L., Preuss, M., Moore, S. A., Robbins, M. E. C., et al. (2001). Docosahexanoic acid synthesis from n−3 polyunsaturated fatty acids in differentiated rat brain astrocytes. J. Lipid Res. 42, 1368–1376.
Keywords: cerebral cortex lipidome, long-chain polyunsaturated fatty acids (LC-PUFAs), docosahexaenoic acid (DHA), sphingolipids, ovarian hormones, synaptic proteins
Citation: Herrera JL, Ordoñez-Gutierrez L, Fabrias G, Casas J, Morales A, Hernandez G, Acosta NG, Rodriguez C, Prieto-Valiente L, Garcia-Segura LM, Alonso R and Wandosell FG (2018) Ovarian Function Modulates the Effects of Long-Chain Polyunsaturated Fatty Acids on the Mouse Cerebral Cortex. Front. Cell. Neurosci. 12:103. doi: 10.3389/fncel.2018.00103
Received: 28 November 2017; Accepted: 29 March 2018;
Published: 24 April 2018.
Edited by:
Merce Pallas, Universitat de Barcelona, SpainReviewed by:
Mustapha Umar Imam, Zhengzhou University, ChinaAmy Christensen, University of Southern California, United States
Copyright © 2018 Herrera, Ordoñez-Gutierrez, Fabrias, Casas, Morales, Hernandez, Acosta, Rodriguez, Prieto-Valiente, Garcia-Segura, Alonso and Wandosell. This is an open-access article distributed under the terms of the Creative Commons Attribution License (CC BY). The use, distribution or reproduction in other forums is permitted, provided the original author(s) and the copyright owner are credited and that the original publication in this journal is cited, in accordance with accepted academic practice. No use, distribution or reproduction is permitted which does not comply with these terms.
*Correspondence: Francisco G. Wandosell, ZndhbmRvc2VsbEBjYm0uY3NpYy5lcw==
Rafael Alonso, cmFsb25zb3NvbGlzQGdtYWlsLmNvbQ==
Luis M. Garcia-Segura, bG1nc0BjYWphbC5jc2ljLmVz