- UMR 8256 Biological Adaptation and Ageing, Sorbonne Université and CNRS, IBPS-B2A, Paris, France
Errors of cerebellar development are increasingly acknowledged as risk factors for neuro-developmental disorders (NDDs), such as attention deficit hyperactivity disorder (ADHD), autism spectrum disorder (ASD), and schizophrenia. Evidence has been assembled from cerebellar abnormalities in autistic patients, as well as a range of genetic mutations identified in human patients that affect the cerebellar circuit, particularly Purkinje cells, and are associated with deficits of motor function, learning and social behavior; traits that are commonly associated with autism and schizophrenia. However, NDDs, such as ASD and schizophrenia, also include systemic abnormalities, e.g., chronic inflammation, abnormal circadian rhythms etc., which cannot be explained by lesions that only affect the cerebellum. Here we bring together phenotypic, circuit and structural evidence supporting the contribution of cerebellar dysfunction in NDDs and propose that the transcription factor Retinoid-related Orphan Receptor alpha (RORα) provides the missing link underlying both cerebellar and systemic abnormalities observed in NDDs. We present the role of RORα in cerebellar development and how the abnormalities that occur due to RORα deficiency could explain NDD symptoms. We then focus on how RORα is linked to NDDs, particularly ASD and schizophrenia, and how its diverse extra-cerebral actions can explain the systemic components of these diseases. Finally, we discuss how RORα-deficiency is likely a driving force for NDDs through its induction of cerebellar developmental defects, which in turn affect downstream targets, and its regulation of extracerebral systems, such as inflammation, circadian rhythms, and sexual dimorphism.
1. Introduction
Neurodevelopmental disorders (NDDs) include a wide range of dysfunction such as autism spectrum disorder (ASD), schizophrenia, attention deficit hyperactivity disorder (ADHD), dyslexia etc. While each disorder is characterized by a well-defined set of symptoms described in the Diagnosis and Statistical Manual of Mental Disorders (DSM-5 TR), there is also a large overlap in symptomatology, such as learning difficulties and diminished social interaction, as well as genetic abnormalities (Carroll and Owen, 2009).
Of all the different brain components involved in NDDs, the cerebellum was proposed as a key region because NDD patients often present with multiple sensory-motor integration deficits that are symptomatic of altered cerebellar function (Fatemi et al., 2012). Also the cerebellum’s broad connectivity indicates its involvement in sensory-motor, cognitive and affective processing (Figure 1), wherein cerebellar integration is necessary for their correct organization (Glickstein and Doron, 2008; Strick et al., 2009; Buckner, 2013; Koziol et al., 2014; D’Mello and Stoodley, 2015). Thus, we can apply the theory of cognitive dysmetria, originally applied to cerebellar neurodegenerative disease (Schmahmann, 1998), to understand the pertinence of the cerebellum to the dysfunctions observed in NDDs.
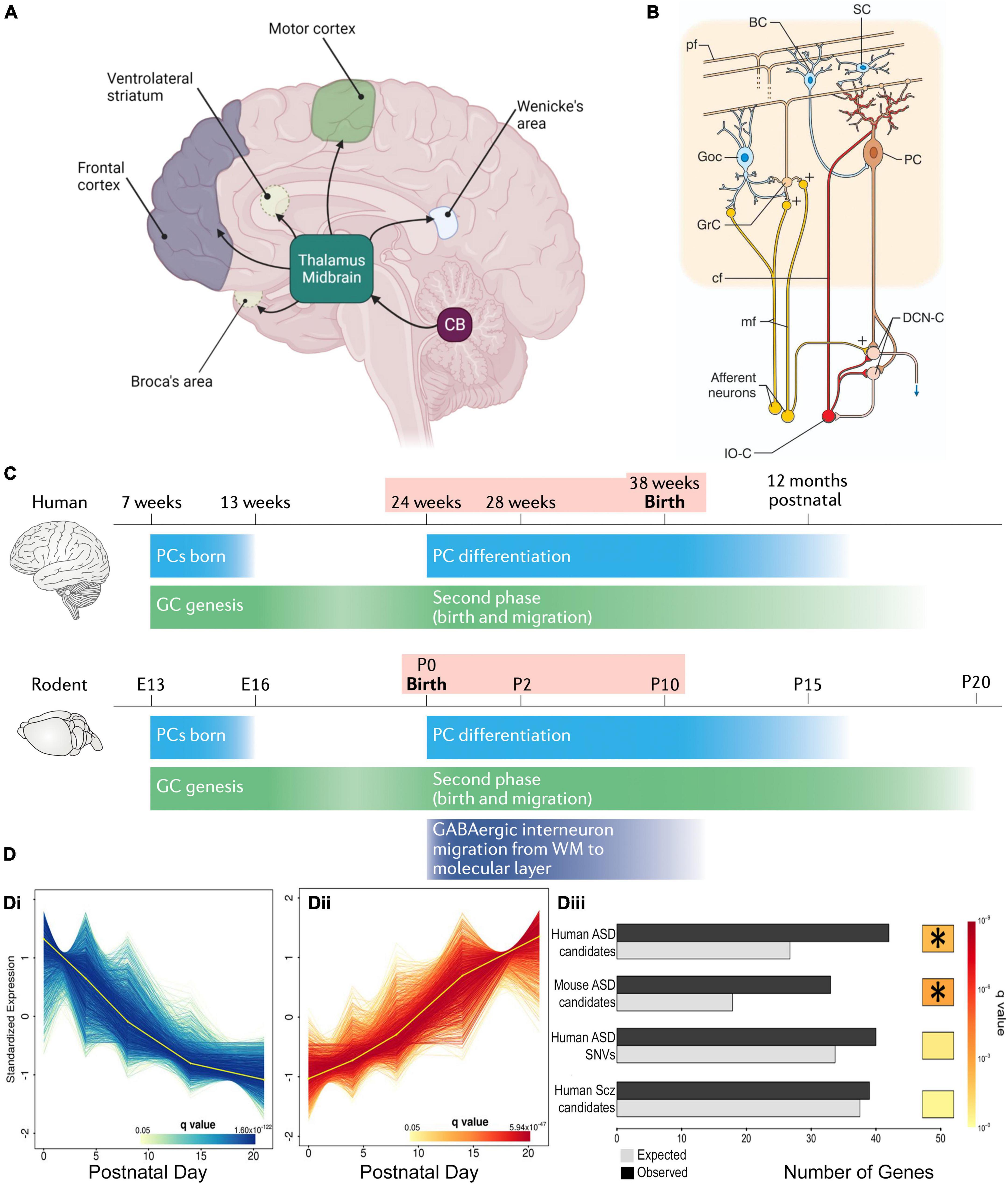
Figure 1. Cerebellar connectivity and development underlies its link to NDDs. (A) The cerebellum has extensive connectivity with the forebrain via the thalamus which underlie its many cognitive and affective roles that are concentrated in different regions. Reproduced with permission from Hatten (2020). (B) Cerebellar cortical circuitry, in which Purkinje cells (PCs) integrate signals from olivary climbing fibers (cf) and granule cell (GrC) parallel fibers (pf), which have received cortical information via pontine mossy fibers (mf), in order to modify deep cerebellar nuclear (DCN) activity, which in turn projects back to the cortex. Neuronal responses are modulated by GABAergic interneurons: feedforward inhibition through basket (BC) and stellate (SC) cells to PCs, and feedback inhibition of GrC-pf activity by Golgi cells (GoC). Reproduced from D’Angelo and Casali (2013) with permission. (C) Humans and rodents have a relatively similar time-course of events leading to cerebellar development, although the human brain inevitably takes longer. Both human and rodent cerebella have relatively similar prolonged vulnerability (pink shading) around birth. Reproduced with permission from Sathyanesan et al. (2019). (D) DESeq2 analysis of mouse PC RNA differentially expressed during early post-natal development (Di, Dii). The gene group that increases expression with development (Dii) was significantly enriched with ASD risk candidate genes (Diii) but, interestingly, not schizophrenia risk genes. *Indicates a significant difference. Modified from Clifford et al. (2019).
However, cerebellar behavioral dysfunction will also involve many non-cerebellar areas, and is therefore only indirect support for the cerebellar-NDD hypothesis. Also, it does not identify whether the cerebellar deficit generates the NDD or is merely a reflection of disordered brain development. Support for a causal role for the cerebellum comes from it having the highest co-expression of NDD-associated genes (Menashe et al., 2013; Wang et al., 2014), many of which are temporally regulated in developing Purkinje neurons (Clifford et al., 2019), and are thus central to cerebellar development (Parenti et al., 2020). Conversely, cerebellar dysfunction alone is not sufficient to explain the systemic comorbidities often seen in NDDs, such as abnormal circadian rhythm, chronic inflammation and sexual dimorphism (Hu et al., 2015; Siniscalco et al., 2018).
Here we propose a potential unifying theory in which a pleiotropic nuclear receptor, Retinoic acid-related Orphan Receptor alpha (RORα), could account for the cerebellar, neuropsychiatric and systemic components of neurodevelopmental disorders. Of the 1,353 mouse genes that have been linked to NDDs (SFARI Gene 3.0, 2022) 14% are likely RORA targets (Hu et al., 2015). Moreover, we chose RORα because in addition to its regulation of critical stages of cerebellar development and function, it also has generic physiological roles in many systems including inflammation, circadian rhythms and sex steroid metabolism (Jarvis et al., 2002; Boukhtouche et al., 2006b; Hu et al., 2015), processes that also affected in NDDs. Moreover, while the cerebellum-NDD hypothesis has been extensively reviewed (Wang et al., 2014; Sathyanesan et al., 2019; Thabault et al., 2022), including its potential genetic basis, how a gene that affects a process of cerebellar neurodevelopment can generate such wide ranging abnormalities remains unclear.
2. The cerebellum and neurodevelopmental disorders
2.1. Cerebro-cerebellar interactions
As indicated above, NDD-associated behavioral abnormalities are consistent with cerebellar dysfunction. This is due to the extensive cerebellar connectivity (Figure 1A) with brain regions such as the prefrontal cortex, thalamus and ventral tegmental area (VTA). These broad networks underlie the cerebellar contribution to higher cognitive and affective processing, according to the specific region of the cerebellum that is activated (King et al., 2019).
However, functional impairment in NDDs is not limited to the cerebellum but involves the entire cerebellocortical circuit (Sathyanesan et al., 2019; Thabault et al., 2022). In schizophrenia, for example, there is reduced blood flow in the cerebello-thalamo-cortical circuit during a cognitive task (Daskalakis et al., 2005) and children with ASD display poor motor coordination that correlates with reduced cerebellar white matter fractional anisotropy (white matter integrity) (Dickinson et al., 2016). Cortico-cerebellar interactions are clearly demonstrated by fMRI and the functional connectivity between Right Crus 1 (RC1) and the infra-parietal lobule of the default mode network is disrupted in autistic patients (Stoodley et al., 2017). The infra-parietal lobule is involved in the imitation and interpretation of the gestures of other people, and an impairment of this network causes disruption of social development (Stoodley et al., 2017). The same functional connectivity occurs in mice, and when RC1 activity is experimentally inhibited, the mice display autistic-like behavior (Stoodley et al., 2017). The importance of RC1 is reinforced by its altered morphology in ASD patients, which correlates with an eye tracking abnormality (Laidi et al., 2017). Eye-tracking is thought to be involved in face recognition, thus an alteration in this function would account for decreased social interaction. Moreover, oculomotor impairment has recently been suggested as an early diagnostic feature for ASD (Laidi et al., 2017). The cerebellum also has direct projections to the VTA, which are involved in reward, particularly for social interaction, and cerebellar dysfunction in this pathway leads to abnormal social behavior, a classic NDD trait (Carta et al., 2019).
2.2. Cerebellar structural abnormalities in NDDs
In addition to connectivity errors, patients with NDDs often have reduced cerebellar volume (Daskalakis et al., 2005; Becker and Stoodley, 2013; Hampson and Blatt, 2015; Stoodley and Kuschner, 2016), and perinatal cerebellar injury forms the highest non-genetic risk for NDDs (Wang et al., 2014). Within the cerebellum, the cortex has a highly uniform network centered around Purkinje cells (PC), that receive excitatory input from climbing fiber axons of medullary inferior olive neurons and parallel fiber axons of cerebellar granular cells, in addition to modulation by local inhibitory interneurons. The PC is the sole cortical outflow, impacting on deep cerebellar neurons and thus downstream cerebral centers (Figure 1B). Patients with NDDs often show PC loss with different lobules being affected for each disorder (Daskalakis et al., 2005; Becker and Stoodley, 2013; Hampson and Blatt, 2015; Stoodley and Kuschner, 2016). The importance of PCs is confirmed in NDD mouse models in which gene mutations/deletions are limited to Purkinje cells, wherein PCs malfunction and there is associated ASD-like repetitive and social behaviors (Reith et al., 2013; Piochon et al., 2015; Thabault et al., 2022).
2.3. Cerebellar development
Cerebellar development is protracted extending from 30 days post-conception to the second post-natal year in humans and ∼E10 to P28 in mice (Figure 1C; Leto et al., 2016; van Essen et al., 2020) making it vulnerable to environmental change (Haldipur et al., 2011). The perinatal period is particularly vulnerable because it is the time of multiple changes in the cerebellum, including neurogenesis, neuronal migration and connectivity, all necessary for formation of the mature structure (Leto et al., 2016). But at the same time, major cerebellar projections to the thalamus, cortex and other regions are maturing. All these concurrent processes can explain the 36-fold increase in risk of ASD following perinatal cerebellar injury (Wang et al., 2014). The human perinatal period is equivalent to early post-natal stages in the mouse, in which the cerebellar cortical circuit is generated and refined (Leto et al., 2016). This period includes the genesis, migration and connectivity of granule cells (GCs) and their parallel fiber axons (PFs). Purkinje cells extend a large ramified dendritic tree to receive many PF synaptic inputs. They also receive multiple climbing fibers (CFs) from the brainstem inferior olive (Leto et al., 2016), which are refined to a monoinnervation by the end of the 3rd post-natal week. Lastly, basket, then stellate, inhibitory interneurons create negative feedback loops on PC activity (Ango et al., 2004). These processes occur in a defined order and if their appropriate timing is disturbed, PCs and their cortical circuit do not mature correctly (Letellier et al., 2009; Bailly et al., 2018), having modified activity that in turn will affect downstream cortical and subcortical areas and their associated cognitive and affective regulation. This hypothesis is supported by the existence of important changes in cerebrocerebellar interaction during maturation, which would indicate that disrupted cerebellar development could alter correct brain functioning (Moore et al., 2017): i.e., abnormal cerebellar development will not only alter its own function, but will also perturb the maturation of connected forebrain regions and their associated cognitive and affective processes. This impact that the development of one structure can have on another with which it is connected, was described by Wang et al. (2014) as developmental diaschisis.
Consistent with this hypothesis, a perinatal cerebellar lesion leads to a relative volume reduction of the contralateral prefrontal cortex (PFC; Limperopoulos et al., 2012). This relative size abnormality between the PFC and cerebellum also occurs in 3 to 9-year-old boys suffering from ASD (Carper, 2000; Sparks et al., 2002), thus supporting the importance of cerebellar development in NDDs and its developmental impact on the whole brain.
With so many interconnected processes taking place simultaneously during post-natal cerebellar development, multiple genes need to be expressed at a given time and in a given place. For example, sonic hedgehog (SHH) is secreted by PCs to stimulate granule cell precursor (GCP) division, thus shaping the cerebellum during pre and post-natal stages (Lewis et al., 2004). Synaptogenesis requires other genes such as neuroligins or shanks, mutations in which are known NDD risk factors (Parenti et al., 2020). Importantly, many genes expressed during cerebellar development, including 58 in PCs, are established risk-candidates for NDDs (Figure 1D; Clifford et al., 2019; Parenti et al., 2020). One such molecule is the Retinoic acid-related Orphan Receptor α (RORα), which is essential for PC development and maturation during the embryonic phase, for the refinement of their connections, and their maintenance throughout life (Figure 2A; Boukhtouche et al., 2006a; Chen et al., 2013; Takeo et al., 2015). Consequently, a lack of RORα will lead to PC malformation and death, and by interfering with PC secretion of SHH (Gold et al., 2003), it will reduce GC genesis, resulting in malformation of the whole cerebellar network.
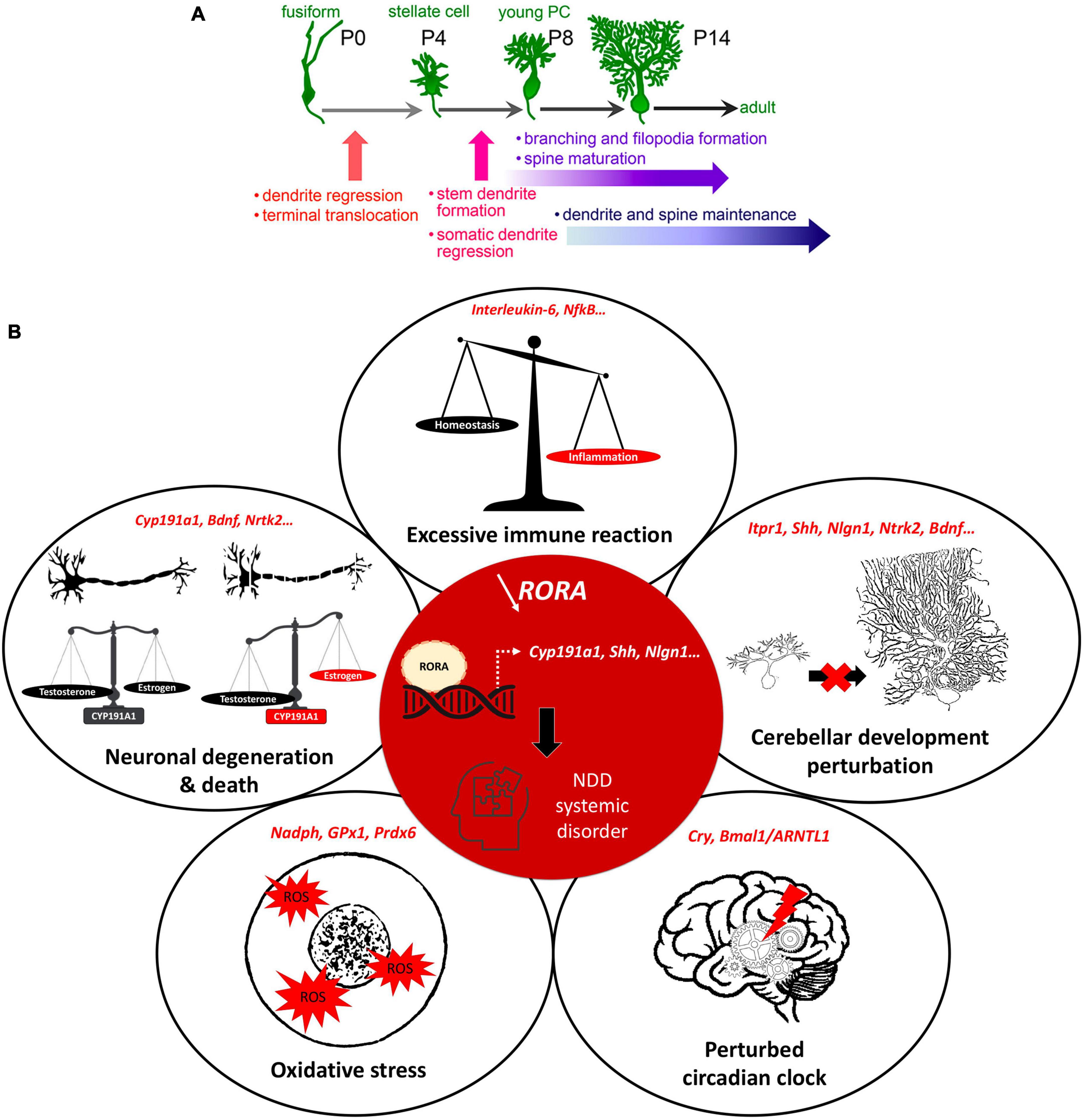
Figure 2. RORα regulates multiple genes and plays extensive roles in cerebellar development. (A) Key stages of PC development which are regulated by RORα. These are at all stages from embryonic development to adult maintenance. Reproduced from Takeo et al. (2015) with permission. (B) A schema showing the central role of RORα in multiple cellular processes, that are modified in NDDs. When RORα is reduced (central red circle), its regulation of gene transcription is altered. Here we include the known RORα target genes that are also involved in NDDs. The effects in red illustrate the induced abnormalities according to the direction of change: estrogen and PC development are reduced, circadian rhythms are perturbed, but inflammation and ROS are increased.
Taken together, the high vulnerability of cerebellar development, including all developmental processes from gene expression to long-distance connectivity, combined with its close ties with cerebral structures such as the PFC, reinforce the role of this structure in the onset of NDDs.
3. RORα and neurodevelopmental disorders
Given the wide range of symptoms seen in NDDs, including impaired cognition, disruption of circadian clock, abnormal inflammatory episodes etc., it is difficult to imagine a single point of origin, including localized perinatal cerebellar injury. However, nuclear receptors, which are transcription factors activated by hormones, such as thyroid hormone, steroids and retinoic acid, have wide-ranging functions. Again, RORα, is of particular interest given its broad function and role in cerebellar development.
Retinoid-related Orphan Receptor alpha is a transcription factor with a classical structure including a ligand binding domain (LBD) and a DNA binding domain (DBD) that binds to ROR response elements (RORE) on the DNA (Becker-André et al., 1993). It exists in four isoforms (α1 to α4) differing by their N-terminal domain and by their level of expression in different tissues: RORα4 in leukocytes and skin, RORα2 and 3 in the testes and RORα1 and 4 in the brain (Matsui et al., 1995). Within the brain, RORα is widely expressed in the cerebral cortex, thalamus, hypothalamus and cerebellum (Ino, 2004). Consistent with being a transcription factor, RORα regulates a very large number of genes which results in RORα regulating many physiological processes including those that are disordered in NDDs (for review, see Cook et al., 2015). Moreover, studies on purified neurons show that RORα can bind to the promoter regions of over 2,500 genes (Hu et al., 2015), 438 of which are included in autism gene databases (Xu et al., 2012; Parenti et al., 2020).
More specifically for NDDs, RORα target genes are either confirmed NDD candidate genes (e.g., ITPR1, NLGN1, NTRK2) or regulate processes affected in NDDs (e.g., CYP19A1, A2BP1, HSD17B10) (Supplementary Table 1; Sarachana et al., 2011; Sarachana and Hu, 2013). For example, CYP19A1 (aromatase) converts testosterone to estrogen, which upregulates RORα (Sarachana et al., 2011). Thus, in boys, less RORA expression and reduced aromatase will increase circulating testosterone, which in turn inhibits RORA expression (Sarachana et al., 2011), reinforcing the RORA deficiency. Moreover, less estrogen, which is neuroprotective, would exacerbate PC death, thus increasing the risk of developing autism (Janmaat et al., 2011; Hu et al., 2015) and explaining the sexual dimorphism of NDDs. This hypothesis is corroborated by post-mortem studies of autistic patients showing fewer PCs, which is more severe in males (Skefos et al., 2014), and also by the “extreme male brain theory” where patients with ASD traits have abnormal sex hormone balance, with higher testosterone (Greenberg et al., 2018). In addition, RORα also has anti-inflammatory functions through upregulating the antioxidant enzymes, glutathione peroxidase-1 and peroxiredoxin-6 (Boukhtouche et al., 2006b) that protect neurons and glia from the adverse effects of oxidative stress (Boukhtouche et al., 2006b; Jolly et al., 2012). RORα also regulates expression of the inflammatory cytokine interleukin 6 (IL6; Journiac et al., 2009). Thus, reduction of RORα function can underlie the greater inflammatory state found in NDD patients (Jiang et al., 2018). Lastly, RORα binds to Bmal1 and Cry and is a central part of the circadian cycle, a cycle known to be disrupted in ASD and schizophrenia patients (Jarvis et al., 2002; Hu et al., 2009). Taken together these data strongly suggest that RORα regulates cellular processes that are perturbed in NDDs (Figure 2B).
Further evidence suggesting that RORα is strongly implicated in NDDs comes from its expression in patient’s brains. RORα is reduced in the cerebellum and PFC of post-mortem ASD brains (Hu et al., 2015), and the RORA gene is hypermethylated (and therefore less expressed) in lymphoblastoid cells of these patients (Nguyen et al., 2010). Additionally, not only are RORA genetic variants associated with ASD (Sayad et al., 2017), but treatment of adult BTBR mice (an ASD model) with a synthetic RORα agonist improved repetitive behaviors by upregulating RORα target genes that are down-regulated in ASD (Wang et al., 2016). But the role of RORα is not confined to ASD; its expression is altered in schizophrenia (Devanna and Vernes, 2014) and many of its single nucleotide polymorphisms are found in ADHD (Miller et al., 2013; Liu et al., 2021). Furthermore, RORA missense variants can occur in either the DNA binding domain, conferring a dominant toxic effect, or in the ligand binding domain, which results in loss-of-function (Guissart et al., 2018). These data show how different mutations, and mutations at different loci in the RORA gene, can produce overlapping but distinct NDD phenotypes.
4. Discussion: Cerebellum, ROR, and NDDs
We have discussed separately the evidence for cerebellar and RORα involvement in neurodevelopmental disorders, but this does not automatically mean that the effects are linked. For example, there is greater PFC oxidative stress in schizophrenia and ASD (Rossignol and Frye, 2014; Maas et al., 2017), but there is also less PFC RORα expression in these disorders (Sarachana and Hu, 2013), which can directly explain the greater oxidative stress without involving the cerebellum.
However, RORα regulates multiple events during cerebellar development, and adult cerebellar maintenance, whose alteration can result in NDD-type dysfunction. This overlapping function reinforces the involvement of both the cerebellum and RORα in the development of these disorders. In the cerebellum, RORα is expressed in stellate and basket interneurons, but more particularly in Purkinje cells where it is required for their survival and growth (Boukhtouche et al., 2006a,b; Dusart and Flamant, 2012). As stated above, this effect on PC survival likely passes through RORα’s direct target genes, CYP19A1 (aromatase) and NTRK2 (the BDNF TrkB receptor), with subsequent reduction in activity of the trophic factors, estrogen and BDNF, respectively (Janmaat et al., 2011; Tsutsui et al., 2011). Moreover, in early post-natal development PCs secrete SHH to promote granule cell genesis and differentiation (Hamilton et al., 1996). However, RORα also directly regulates SHH expression (Gold et al., 2003). Thus, we propose that reduced RORα function explains not only poor PC maturation, but also their defective SHH secretion and subsequent impaired GC development (Leto et al., 2016).
The role of RORα continues during later stages of cerebellar development in particular the development of the Purkinje cell dendritic tree and cortical circuitry. In order to permit somatic polarization and growth of the dendritic tree, PCs regress their transient perisomatic dendrites; a process for which RORα is essential (Boukhtouche et al., 2006a). Subsequent dendritic expansion requires the genesis of GCs, with their PF axons and BDNF secretion (Altman, 1972; Tsutsui et al., 2011), both of which will be reduced by RORα deficiency through its regulation of SHH and NTRKR2 (TrkB) expression (Sarachana and Hu, 2013). PF-PC synaptogenesis takes place on PC dendritic spines, whose formation depends on intracellular calcium regulation by IP3-induced Ca2+ release from the endoplasmic reticulum. RORα directly regulates ITPR1 expression, thus its dysfunction will reduce ITPR1 transcription and hence, spine formation (Sugawara et al., 2017) altering the correct formation of the cerebellar cortical circuit. Indeed, in staggerer mice, which do not have any functional RORα, PCs do not develop dendritic spines, but this abnormality can be rescued by viral vector-induced rora expression, which induces the expression of RORα target genes (Iizuka et al., 2016). Furthermore, a second RORA target gene, A2BP1, codes for an RNA splicing enzyme, whose dysfunction in PCs causes abnormal splicing of SCN8A mRNA encoding the Nav1.6 sodium channel, a key mediator of Purkinje cell pace-making. Thus, although PCs appear normal, they have abnormal spiking activity (Gehman et al., 2012), which will impact upon cerebello-cerebral function. Finally in the 2nd−3rd post-natal weeks, PC activity becomes closely modulated by inhibitory molecular layer interneurons, stellate (SC) and basket cells (BCs), which are the last to form in the immature cerebellar cortex (Leto et al., 2016). The post-synaptic cell adhesion protein, Neuroligin 1, is required for normal GABAergic input to PCs through synaptogenesis at PF-SCs and at the BC-PC axon synaptic complex (Nozawa et al., 2018). Importantly, RORα upregulates the NLGN1 gene (Sarachana and Hu, 2013) thus contributing to GABAergic regulation of cerebellar cortical activity. Therefore, when RORα is deficient, there will be an alteration in excitatory/inhibitory balance in PC afferents. In addition to these key functions during development, RORα is required throughout life to maintain PC dendrites (Chen et al., 2013) and survival, for example ITPR1 deficiency causes the adult-onset spinocerebellar ataxia 15 with its associated PC loss (Sugawara et al., 2017).
Although we present multiple roles for RORα in cerebellar development and function that are likely mechanisms underlying NDDs, we do not claim that it is the cause. There is often an environmental component to NDDs, which is consistent with the prolonged period of cerebellar development, and this will be independent from RORα dysfunction. Moreover, the genetics of NDDs is vast, with numerous small missense mutations in many “risk” genes (Parenti et al., 2020), which often have to occur in combination to cause an NDD phenotype. Therefore, combining RORα ’s role in regulating circadian rhythms, oxidative stress and inflammation (Jarvis et al., 2002; Boukhtouche et al., 2006b; Hu et al., 2015), all key comorbidities in NDDs, with its role in cerebellar development, we hypothesize that RORα has a major causative role in NDD pathophysiology. Further investigation to confirm this possibility is essential because it points to an NDD-risk gene that has appropriate widespread actions, and which can be replaced therapeutically to upregulate the abnormal low expression of RORA target genes, thus improving abnormal NDD-linked behaviors.
Data availability statement
The original contributions presented in this study are included in the article/Supplementary material, further inquiries can be directed to the corresponding author.
Author contributions
SR wrote the original draft. SR and RS edited and finalized the manuscript. Both authors contributed to the article and approved the submitted version.
Funding
This work was supported by a French Laboratory of Excellence in Biological Psychiatry (LabEx BioPsy) doctoral scholarship to SR, and ANR-19-CE37-0021 grant BrainMag.
Acknowledgments
We would like to acknowledge the helpful comments of Ann Lohof on this manuscript.
Conflict of interest
The authors declare that the research was conducted in the absence of any commercial or financial relationships that could be construed as a potential conflict of interest.
Publisher’s note
All claims expressed in this article are solely those of the authors and do not necessarily represent those of their affiliated organizations, or those of the publisher, the editors and the reviewers. Any product that may be evaluated in this article, or claim that may be made by its manufacturer, is not guaranteed or endorsed by the publisher.
Supplementary material
The Supplementary Material for this article can be found online at: https://www.frontiersin.org/articles/10.3389/fncel.2023.1108339/full#supplementary-material
References
Altman, J. (1972). Postnatal development of the cerebellar cortex in the rat. III. Maturation of the components of the granular layer. J. Comp. Neurol. 145, 465–513. doi: 10.1002/cne.901450403
Ango, F., di Cristo, G., Higashiyama, H., Bennett, V., Wu, P., and Huang, Z. J. (2004). Ankyrin-based subcellular gradient of neurofascin, an immunoglobulin family protein, directs GABAergic innervation at Purkinje axon initial segment. Cell 119, 257–272. doi: 10.1016/j.cell.2004.10.004
Bailly, Y., Rabacchi, S., Sherrard, R., Rodeau, J., Demais, V., Lohof, A., et al. (2018). Elimination of all redundant climbing fiber synapses requires granule cells in the postnatal cerebellum. Sci. Rep. 8:10017. doi: 10.1038/s41598-018-28398-7
Becker, E. B. E., and Stoodley, C. J. (2013). Autism spectrum disorder and the cerebellum. Int. Rev. Neurobiol. 113, 1–34. doi: 10.1016/B978-0-12-418700-9.00001-0
Becker-André, M., André, E., and DeLamarter, J. F. (1993). Identification of nuclear receptor mRNAs by RT-PCR amplification of conserved zinc-finger motif sequences. Biochem. Biophys. Commun. 194, 1371–1379. doi: 10.1006/bbrc.1993.1976
Boukhtouche, F., Janmaat, S., Vodjdani, G., Gautheron, V., Mallet, J., Dusart, I., et al. (2006a). Retinoid-related orphan receptor α controls the early steps of Purkinje cell dendritic differentiation. J. Neurosci. 26, 1531–1538. doi: 10.1523/JNEUROSCI.4636-05.2006
Boukhtouche, F., Vodjdani, G., Jarvis, C., Bakouche, J., Staels, B., Mallet, J., et al. (2006b). Human retinoic acid receptor-related orphan receptor α1 overexpression protects neurones against oxidative stress-induced apoptosis. J. Neurochem. 96, 1778–1789. doi: 10.1111/j.1471-4159.2006.03708.x
Buckner, R. L. (2013). The cerebellum and cognitive function: 25 years of insight from anatomy and neuroimaging. Neuron 80, 807–815. doi: 10.1016/j.neuron.2013.10.044
Carper, R. A. (2000). Inverse correlation between frontal lobe and cerebellum sizes in children with autism. Brain 123, 836–844. doi: 10.1093/brain/123.4.836
Carroll, L. S., and Owen, M. J. (2009). Genetic overlap between autism, schizophrenia and bipolar disorder. Genome Med. 1, 1–7. doi: 10.1186/gm102
Carta, I., Chen, C., Schott, A., Dorizan, S., and Khodakhah, K. (2019). Cerebellar modulation of the reward circuitry and social behavior. Science 363:eaav0581. doi: 10.1126/science.aav0581
Chen, X., Heck, N., Lohof, A., Rochefort, C., Morel, M., Wehrl, R., et al. (2013). Mature purkinje cells require the retinoic acid-related orphan receptor-α (rorα) to maintain climbing fiber mono-innervation and other adult characteristics. J. Neurosci. 33, 9546–9562. doi: 10.1523/JNEUROSCI.2977-12.2013
Clifford, H., Dulneva, A., Ponting, C. P., Haerty, W., and Becker, E. B. (2019). A gene expression signature in developing Purkinje cells predicts autism and intellectual disability co-morbidity status. Sci. Rep. 9:485. doi: 10.1038/s41598-018-37284-1
Cook, D. N., Kang, H. S., and Jetten, A. M. (2015). Retinoic acid-related orphan receptors (RORs): Regulatory functions in immunity, development, circadian rhythm, and metabolism. Nucl. Receptor Res. 2:101185. doi: 10.1016/j.physbeh.2017.03.040
D’Angelo, E., and Casali, S. (2013). Seeking a unified framework for cerebellar function and dysfunction: From circuit operations to cognition. Front. Neural Circuits 6, 1–23. doi: 10.3389/fncir.2012.00116
Daskalakis, Z. J., Christensen, B. K., Fitzgerald, P. B., Fountain, S. I., and Chen, R. (2005). Reduced cerebellar inhibition in schizophrenia: A preliminary study. Am. J. Psychiatry 162, 1203–1205. doi: 10.1176/appi.ajp.162.6.1203
Devanna, P., and Vernes, S. C. (2014). A direct molecular link between the autism candidate gene RORa and the schizophrenia candidate MIR137. Sci. Rep. 4:3994. doi: 10.1038/srep03994
Dickinson, A., Jones, M., and Milne, E. (2016). Measuring neural excitation and inhibition in autism: Different approaches, different findings and different interpretations. Brain Res. 1648, 277–289. doi: 10.1016/j.brainres.2016.07.011
D’Mello, A. M., and Stoodley, C. J. (2015). Cerebro-cerebellar circuits in autism spectrum disorder. Front. Neurosci. 9:408. doi: 10.3389/fnins.2015.00408
Dusart, I., and Flamant, F. (2012). Profound morphological and functional changes of rodent Purkinje cells between the first and the second postnatal weeks: A metamorphosis? Front. Neuroanat. 6:11. doi: 10.3389/fnana.2012.00011
Fatemi, S. H., Aldinger, K. A., Ashwood, P., Bauman, M. L., Blaha, C. D., Blatt, G. J., et al. (2012). Consensus paper: Pathological role of the cerebellum in Autism. Cerebellum 11, 777–807. doi: 10.1007/s12311-012-0355-9
Gehman, L. T., Meera, P., Stoilov, P., Shiue, L., O’Brien, J, E., Meisler, M. H., et al. (2012). The splicing regulator Rbfox2 is required for both cerebellar development and mature motor function. Genes Dev. 26, 445–460. doi: 10.1101/gad.182477.111
Glickstein, M., and Doron, K. (2008). Cerebellum: Connections and functions. Cerebellum 7, 589–594. doi: 10.1007/s12311-008-0074-4
Gold, D. A., Baek, S. H., Schork, N. J., Rose, D. W., Larsen, D. D., Sachs, B. D., et al. (2003). RORα coordinates reciprocal signaling in cerebellar development through Sonic hedgehog and calcium-dependent pathways. Neuron 40, 1119–1131. doi: 10.1016/s0896-6273(03)00769-4
Greenberg, D. M., Warrier, V., Allison, C., and Baron-Cohen, S. (2018). Testing the empathizing–systemizing theory of sex differences and the extreme male brain theory of autism in half a million people. Proc. Natl. Acad. Sci. U.S.A. 115, 12152–12157. doi: 10.1073/pnas.1811032115
Guissart, C., Latypova, X., Rollier, P., Khan, T., Stamberger, H., McWalter, K., et al. (2018). Dual molecular effects of dominant RORA mutations cause two variants of syndromic intellectual disability with either autism or cerebellar ataxia. Am. J. Hum. Genet. 102, 744–759. doi: 10.1016/j.ajhg.2018.02.021
Haldipur, P., Bharti, U., Alberti, C., Sarkar, C., Gulati, G., Iyengar, S., et al. (2011). Preterm delivery disrupts the developmental program of the cerebellum. PLoS One 6:e23449. doi: 10.1371/journal.pone.0023449
Hamilton, B. A., Frankel, W., Kerrebrock, A., Hawkins, T., FitzHugh, W., Kusumi, K., et al. (1996). Disruption of the nuclear hormone receptor RORα in staggerer mice. Nature 379, 736–739. doi: 10.1038/379736a0
Hampson, D. R., and Blatt, G. J. (2015). Autism spectrum disorders and neuropathology of the cerebellum. Front. Neurosci. 9:420. doi: 10.3389/fnins.2015.00420
Hatten, M. E. (2020). Adding cognitive connections to the cerebellum. Science 370, 1411–1412. doi: 10.1126/science.abf4483
Hu, V. W., Sarachana, T., Kim, K. S., Nguyen, A., Kulkarni, S., Steinberg, M. E., et al. (2009). Gene expression profiling differentiates autism case-controls and phenotypic variants of autism spectrum disorders: Evidence for circadian rhythm dysfunction in severe autism. Autism Res. 2, 78–97. doi: 10.1002/aur.73
Hu, V. W., Sarachana, T., Sherrard, R., and Kocher, K. (2015). Investigation of sex differences in the expression of RORA and its transcriptional targets in the brain as a potential contributor to the sex bias in autism. Mol. Autism 6:7. doi: 10.1186/2040-2392-6-7
Iizuka, A., Matsuzaki, Y., Konno, A., and Hirai, H. (2016). Plasticity of the developmentally arrested staggerer cerebellum in response to exogenous RORα. Brain Struct. Funct. 221, 2879–2889. doi: 10.1007/s00429-015-1077-9
Ino, H. (2004). Immunohistochemical characterization of the orphan nuclear receptor RORα in the mouse nervous system. J. Histochem. Cytochem. 52, 311–323. doi: 10.1177/002215540405200302
Janmaat, S., Akwa, Y., Doulazmi, M., Bakouche, J., Gautheron, V., Liere, P., et al. (2011). Age-related Purkinje cell death is steroid dependent: RORα haplo-insufficiency impairs plasma and cerebellar steroids and Purkinje cell survival. Age 33, 565–578. doi: 10.1007/s11357-010-9203-3
Jarvis, C. I., Staels, B., Brugg, B., Lemaigre-Dubreuil, Y., Tedgui, A., and Mariani, J. (2002). Age-related phenotypes in the staggerer mouse expand the ROR nuclear receptors role beyond the cerebellum. Mol. Cell. Endocrinol. 186, 1–5. doi: 10.1016/s0303-7207(01)00668-2
Jiang, N. M., Cowan, M., Moonah, S. N., and William, A. P. Jr. (2018). The impact of systemic inflammation on neurodevelopment. Trends Mol. Med. 24, 794–804. doi: 10.1016/j.molmed.2018.06.008
Jolly, S., Journiac, N., Garabedian, B. V., and Mariani, J. (2012). RORalpha, a key to the development and functioning of the brain. Cerebellum 11, 451–452. doi: 10.1007/s12311-011-0339-1
Journiac, N., Jolly, S., Jarvis, C., Gautheron, V., Rogard, M., Trembleau, A., et al. (2009). The nuclear receptor RORα exerts a bi-directional regulation of IL-6 in resting and reactive astrocytes. Proc. Natl. Acad. Sci. U.S.A. 106, 21365–21370. doi: 10.1073/pnas.0911782106
King, M., Hernandez-Castillo, C. R., Poldrack, R. A., Ivry, R. B., and Diedrichsen, J. (2019). Functional boundaries in the human cerebellum revealed by a multi-domain task battery. Nat. Neurosci. 22, 1371–1378. doi: 10.1038/s41593-019-0436-x
Koziol, L., Budding, D., Andreasen, N., D’Arrigo, S., Bulgheroni, S., Imamizu, H., et al. (2014). Consensus paper: The cerebellums role in movement and cognition. Cerebellum 13, 151–177. doi: 10.1038/jid.2014.371
Laidi, C., Boisgontier, J., Chakravarty, M., Hotier, S., d’Albis, M., Mangin, J., et al. (2017). Cerebellar anatomical alterations and attention to eyes in autism. Sci. Rep. 7:12008. doi: 10.1038/s41598-017-11883-w
Letellier, M., Wehrl, R., Mariani, J., and Lohof, A. (2009). Synapse elimination in olivo-cerebellar explants occurs during a critical period and leaves an indelible trace in Purkinje cells. Proc. Natl. Acad. Sci. U.S.A. 106, 14102–14107. doi: 10.1073/pnas.0902820106
Leto, K., Arancillo, M., Becker, E., Buffo, A., Chiang, C., Ding, B., et al. (2016). Consensus paper: Cerebellar development. Cerebellum 15, 789–828. doi: 10.1007/s12311-015-0724-2
Lewis, D. A., Cruz, D., Eggan, S., and Erickson, S. (2004). Postnatal development of prefrontal inhibitory circuits and the pathophysiology of cognitive dysfunction in schizophrenia. Ann. N. Y. Acad. Sci. 1021, 64–76. doi: 10.1196/annals.1308.008
Limperopoulos, C., Chilingaryan, G., Sullivan, N., Guizard, N., Robertson, R. L., and Plessis, A. (2012). Injury to the premature cerebellum : Outcome is related to remote cortical development. Cereb. Cortex 24, 728–736. doi: 10.1093/cercor/bhs354
Liu, Y., Chang, X., Qu, H., Tian, L., Glessner, J., Qu, J., et al. (2021). Rare recurrent variants in noncoding regions impact attention-deficit hyperactivity disorder (ADHD) gene networks in children of both African American and European American ancestry. Genes 12:310. doi: 10.3390/genes12020310
Maas, D. A., Vallès, A., and Martens, G. J. M. (2017). Oxidative stress, prefrontal cortex hypomyelination and cognitive symptoms in schizophrenia. Transl. Psychiatry 7:e1171. doi: 10.1038/TP.2017.138
Matsui, T., Sashihara, S., Oh, Y., and Waxman, S. G. (1995). An orphan nuclear receptor, mROR α, and its spatial expression in adult mouse brain. Mol. Brain Res. 33, 217–226. doi: 10.1016/0169-328X(95)00126-D
Menashe, I., Grange, P., Larsen, E. C., Banerjee-Basu, S., and Mitra, P. P. (2013). Co-expression profiling of autism genes in the mouse brain. PLoS Comput. Biol. 9:e1003128. doi: 10.1371/journal.pcbi.1003128
Miller, M. W., Wolf, E. J., Logue, M. W., and Baldwin, C. T. (2013). The retinoid-related orphan receptor alpha (RORA) gene and fear-related psychopathology. J. Affect. Disord. 151, 702–708. doi: 10.1016/j.jad.2013.07.022
Moore, D. M., D’Mello, A. M., McGrath, L. M., and Stoodley, C. J. (2017). The developmental relationship between specific cognitive domains and grey matter in the cerebellum. Dev Cogn Neurosci. 24, 1–11. doi: 10.1016/j.dcn.2016.12.001
Nguyen, A., Rauch, T. A., Pfeifer, G. P., and Hu, V. W. (2010). Global methylation profiling of lymphoblastoid cell lines reveals epigenetic contributions to autism spectrum disorders and a novel autism candidate gene, RORA, whose protein product is reduced in autistic brain. FASEB J. 24, 3036–3051. doi: 10.1096/fj.10-154484
Nozawa, K., Hayashi, A., Motohashi, J., Takeo, Y. H., Matsuda, K., and Yuzaki, M. (2018). Cellular and subcellular localization of endogenous neuroligin-1 in the cerebellum. Cerebellum 17, 709–721. doi: 10.1007/s12311-018-0966-x
Parenti, I., Rabaneda, L. G., Schoen, H., and Novarino, G. (2020). Neurodevelopmental disorders: From genetics to functional pathways. Trends Neurosci. 43, 608–621. doi: 10.1016/j.tins.2020.05.004
Piochon, C., Kloth, A., Grasselli, G., Titley, H., Nakayama, H., Hashimoto, K., et al. (2015). Cerebellar plasticity and motor learning deficits in a copy-number variation mouse model of autism. Nat. Commun. 5:5586. doi: 10.1038/ncomms6586.Cerebellar
Reith, R. M., McKenna, J., Wu, H., Hashmi, S. S., Cho, S., Dash, P. K., et al. (2013). Loss of Tsc2 in Purkinje cells is associated with autistic-like behavior in a mouse model of tuberous sclerosis complex. Neurobiol. Dis. 51, 93–103. doi: 10.1016/j.nbd.2012.10.014
Rossignol, D. A., and Frye, R. E. (2014). Evidence linking oxidative stress, mitochondrial dysfunction, and inflammation in the brain of individuals with autism. Front. Physiol. 5:150. doi: 10.3389/fphys.2014.00150
Sarachana, T., and Hu, V. W. (2013). Genome-wide identification of transcriptional targets of RORA reveals direct regulation of multiple genes associated with autism spectrum disorder. Mol. Autism 4:14. doi: 10.1186/2040-2392-4-14
Sarachana, T., Xu, M., Wu, R., and Hu, V. (2011). Sex hormones in autism: Androgens and estrogens differentially and reciprocally regulate RORA, a novel candidate gene for autism. PLoS One 6:e17116. doi: 10.1371/journal.pone.0017116
Sathyanesan, A., Zhou, J., Scafidi, J., Heck, D. H., Sillitoe, R. V., and Gallo, V. (2019). Emerging connections between cerebellar development, behaviour and complex brain disorders. Nat. Rev. Neurosci. 20, 298–313. doi: 10.1038/s41583-019-0152-2
Sayad, A., Noroozi, R., Omrani, M. D., Taheri, M., and Ghafouri-Fard, S. (2017). Retinoic acid-related orphan receptor alpha (RORA) variants are associated with autism spectrum disorder. Metab. Brain Dis. 32, 1595–1601. doi: 10.1007/s11011-017-0049-6
Schmahmann, J. D. (1998). Dysmetria of thought: Clinical consequences of cerebellar dysfunction on cognition and affect. Trends Cogn. Sci. 2, 362–371. doi: 10.1016/S1364-6613(98)01218-2
SFARI Gene 3.0 (2022). Simons Foundation Autism Research Initiative. Available online at: https://gene.sfari.org
Siniscalco, D., Schultz, S., Brigida, A. L., and Antonucci, N., (2018). Inflammation and neuro-immune dysregulations in autism spectrum disorders. Pharmaceuticals 11, 1–14. doi: 10.3390/ph11020056
Skefos, J., Cummings, C., Enzer, K., Holiday, J., Weed, K., Levy, E., et al. (2014). Regional alterations in Purkinje cell density in patients with autism. PLoS One 9:e81255. doi: 10.1371/journal.pone.0081255
Sparks, B. F., Friedman, S. D., Shaw, D. W., Aylward, E. H., Echelard, D., Artru, A. A., et al. (2002). Brain structural abnormalities in young children with autism spectrum disorder. Neurology 59, 184–192. doi: 10.1212/WNL.59.2.184
Stoodley, C. J., D’Mello, A. M., Ellegood, J., Jakkamsetti, V., Liu, P., Nebel, M. B., et al. (2017). Altered Cerebellar connectivity in autism spectrum disorders and rescue of autism related behaviors in mice. Nat. Neurosci. 20, 1744–1751. doi: 10.1097/CCM.0b013e31823da96d.Hydrogen
Stoodley, C. J., and Kuschner. (2016). The cerebellum and neurodevelopmental disorders. Cerebellum 15, 34–37.
Strick, P. L., Dum, R. P., and Fiez, J. A. (2009). Cerebellum and nonmotor function. Annu. Rev. Neurosci. 32, 413–434. doi: 10.1146/annurev.neuro.31.060407.125606
Sugawara, T., Hisatsune, C., Miyamoto, H., Ogawa, N., and Mikoshiba, K. (2017). Regulation of spinogenesis in mature Purkinje cells via mGluR/PKC-mediated phosphorylation of CaMKIIβ. Proc. Natl. Acad. Sci. U.S.A 114, E5256–E5265. doi: 10.1073/pnas.1617270114
Takeo, Y. H., Kakegawa, W., Miura, E., and Yuzaki, M. (2015). Rorα regulates multiple aspects of dendrite development in cerebellar Purkinje cells in vivo. J. Neurosci. 35, 12518–12534. doi: 10.1523/JNEUROSCI.0075-15.2015
Thabault, M., Turpin, V., Maisterrena, A., Jaber, M., Egloff, M., and Galvan, L. (2022). Cerebellar and striatal implications in autism spectrum disorders: From clinical observations to animal models. Int. J. Mol. Sci. 23:2294. doi: 10.3390/ijms23042294
Tsutsui, K., Ukena, K., Sakamoto, H., Okuyama, S., and Haraguchi, S. (2011). Biosynthesis, mode of action, and functional significance of neurosteroids in the purkinje cell. Front. Endocrinol. 2:61. doi: 10.3389/fendo.2011.00061
van Essen, M. J., Nayler, S., Becker, E. B., and Jacob, J. (2020). Deconstructing cerebellar development cell by cell. PLoS Genet. 16:e1008630. doi: 10.1371/journal.pgen.1008630
Wang, S. S., Kloth, A. D., and Badura, A. (2014). The cerebellum, sensitive periods, and autism. Neuron 83, 518–532. doi: 10.1016/j.neuron.2014.07.016
Wang, Y., Billon, C., Walker, J. K., and Burris, T. P. (2016). Therapeutic effect of a synthetic RORα/γ agonist in an animal model of autism. ACS Chem. Neurosci. 7, 143–148. doi: 10.1021/acschemneuro.5b00159
Keywords: cerebellum, autism, schizophrenia, Purkinje cell, circadian rhythms
Citation: Ribeiro S and Sherrard RM (2023) Cerebellum and neurodevelopmental disorders: RORα is a unifying force. Front. Cell. Neurosci. 17:1108339. doi: 10.3389/fncel.2023.1108339
Received: 25 November 2022; Accepted: 14 March 2023;
Published: 30 March 2023.
Edited by:
Keiko Tanaka-Yamamoto, Korea Institute of Science and Technology (KIST), Republic of KoreaReviewed by:
Aaron Sathyanesan, University of Dayton, United StatesCopyright © 2023 Ribeiro and Sherrard. This is an open-access article distributed under the terms of the Creative Commons Attribution License (CC BY). The use, distribution or reproduction in other forums is permitted, provided the original author(s) and the copyright owner(s) are credited and that the original publication in this journal is cited, in accordance with accepted academic practice. No use, distribution or reproduction is permitted which does not comply with these terms.
*Correspondence: Rachel M. Sherrard, cmFjaGVsLnNoZXJyYXJkQHNvcmJvbm5lLXVuaXZlcnNpdGUuZnI=