- 1Department of Physical Education, Seoul National University, Seoul, South Korea
- 2Institute of Sport Science, Seoul National University, Seoul, South Korea
- 3Institute on Aging, Seoul National University, Seoul, South Korea
Anxiety disorders are the most frequently diagnosed psychological condition, associated with serious comorbidities including excessive fear and interference with daily life. Drugs for anxiety disorders are typically prescribed but the side effects include weight gain, nausea, and sleepiness. Exercise is an effective treatment for anxiety. Exercise induces the release of extracellular vesicles (EVs) into the circulation, which transmit signals between organs. However, the effects of exercise-induced EVs on anxiety remain poorly understood. Here, we isolated EVs from the sera of mice that were sedentary or that voluntarily exercised. We characterized the changes in the miRNA profile of serum EVs after 4 weeks of voluntary exercise. miRNA sequencing showed that 82 miRNAs (46 of which were positive and 36 negative regulators) changed after exercise. We selected genes affected by at least two miRNAs. Of these, 27.27% were associated with neurotrophin signaling (9.09% with each of central nervous system neuronal development, cerebral cortical cell migration, and peripheral neuronal development). We also analyzed behavioral changes in mice with 3 weeks of restraint stress-induced anxiety after injection of 20 μg amounts of EVs from exercised or sedentary mice into the left cerebral ventricle. We found that exercise-derived EVs reduced anxiety (compared to a control group) in a nest-building test but found no between-group differences in the rotarod or open field tests. Exercise-derived EVs enhanced the expression of neuroactive ligand-receptor interaction genes. Thus, exercise-derived EVs may exhibit anti-anxiety effects and may be of therapeutic utility.
Introduction
Anxiety disorders are the most common mental illnesses in the United States; 31% of adults experience anxiety disorders at some time during life (Ritchie and Roser, 2018). Anxiety disorders reduce the quality-of-life (QoL) and increase mortality (Pande et al., 2013; Sarma and Byrne, 2014; Walker et al., 2015). Such disorders are accompanied by depression and other mental illnesses, imposing major societal burdens (Wittchen, 2002). The key anxiety-related brain regions are the amygdala, the ventromedial prefrontal cortex (vmPFC), the anterior cingulate cortex (ACC), and the hippocampus (Milad and Quirk, 2012). Poor coordination among these regions may cause anxiety and fear. Also, a dysfunctional hypothalamic-pituitary-adrenal (HPA) axis may trigger anxiety; the HPA axis becomes hyperactive during stress (Craske et al., 2017). Platelet 5-hydroxytryptamine (5-HT, serotonin)-reuptake-site binding was decreased and brain-derived neurotrophic factor (BDNF) downregulated in patients with anxiety disorders (Iny et al., 1994; Janke et al., 2015).
Typical treatments include selective serotonin reuptake inhibitors (SSRIs), and serotonin and norepinephrine reuptake inhibitors (SNRIs). However, the side effects such as weight gain, nausea, sleepiness (Lord et al., 2017) and anticholinergic effects (Bet et al., 2013). Therefore, other therapies are required. Exercise can be an effective treatment for anxiety disorder. Those who engaged in aerobic exercise and resistance training had lower anxiety symptom scores than controls (Herring et al., 2012). In addition, a meta-analysis found that physical activity positively affected post-traumatic stress disorder (PTSD), which is a type of anxiety disorder (Rosenbaum et al., 2015).
Physical activity plays a key role in human health, affecting protein synthesis and degradation, gene regulation, and epigenetic controls including methylation and acetylation (Widmann et al., 2019; Yoon et al., 2019). Exercise triggers exerkines release into the circulation and changes Extracellular vesicles (EVs) composition (Safdar et al., 2016). EVs contain cellular proteins, nucleic acids, lipids, and metabolites within bilayered membranes. EVs transfer signals among organs (Pitt et al., 2016; Maji et al., 2017). Acute aerobic exercise did not change EVs size but the levels of concentration increased (Oliveira et al., 2018). EVs protein profile changed after a 1-h cycling exercise in healthy humans (Whitham et al., 2018); exercise-derived EVs contained neurogenesis-related proteins including BDNF, apelin (APLN), and cathepsin B (CTSB) (Safdar and Tarnopolsky, 2018). The microRNAs (miRNAs) of rat EVs changed after acute uphill or downhill exercise (Yin et al., 2019).
Among those, the miRNA profiles of EVs are attracting increasing attention. PubMed shows that only five relevant reports appeared in 2010, while 202 were published in 2019. miRNAs are small non-coding RNAs regulating protein expression via mRNA degradation or inhibition of mRNA translation. Exercise-induced miRNAs in EVs protect against cardiovascular disease and strengthen the immune system (Bei et al., 2017; Maji et al., 2017). Two weeks of treadmill exercise changed the EVs miRNA profile to favor neural maturation (Moon et al., 2020). However, no study has explored the effects of exercise-induced EVs miRNAs on anxiety, which is the focus of this study.
Materials and Methods
Neuro2A Cell Culture
Neuro2A cells (ATCC CCL-131, USA), a mouse neuroblastoma cell line, were seeded into 6-well plates at an initial density of 104 cells/well and grown in Eagle's Minimum Essential Medium (EMEM; Gibco, NY, USA) supplemented with 10% fetal bovine serum (FBS, Gibco) in a 37 °C incubator with 95% air and 5% carbon dioxide (CO2). The cells were passaged every second day and confluency was maintained below 90%.
Cell Viability Assay
Cell viability was assessed using Cell Counting Kit-8 (CCK-8; Dojin Laboratories, Kumamoto, Japan). Briefly, Neuro2A cells were seeded into 96 well plates at an initial density of 103cells/well. After 24 h on EMEM with 10% exosome-depleted fetal bovine serum (FBS, Gibco), the medium was changed to serum free EMEM. After incubation with the FBS or the indicated concentraiont (0.1, 1, 10 ng) of EVs from sedentary or exercise group for the indicated time, reagent was added and the mixture was incubated for 3 h. To assess cell viability, absorbance at 450 nm was measured using a Tecan (Infinite 200 PRO series) 96-well microplate spectrophotometer (Mannedorf, Switzerland).
Animal Care
All animal experiments were approved by the Institutional Animal Care and Use Committee (IACUC) of Seoul National University (no. IACUC-190730-6-1). Male C57BL/6J mice were housed at 22–25°C under a 12-h:12-h light:dark cycle with free access to water and food. Mice were housed singly for 4 weeks with or without access to a running wheel.
Restraint Stress
Restraint was performed 6 h per day during 21 days consecutively. Mice were randomly divided into two groups. Controls (N, n = 5) were left completely undetained, while restraint (R, n = 15) were received restraint stress for 3 weeks. The restrainers were purchased commercially (JEUNG DO BIO&PLANT CO, LTD, JD-R-02.).
EVs Collection
After 4 weeks of exercise, mice were anesthesized with isoflurane and whole heart blood collected into BD SST Gold tubes (BD Microtainer, #365957) and centrifuged at 4,000 rpm for 10 min at 4°C; the sera were transferred to fresh tubes. An ExoLutE Plasma and Serum Kit (Rosetta Exosome, #EX-03) was used to remove lipoproteins. Sera (0.5-mL amounts) were centrifuged at 2,000 × g for 10 min at 4°C, the supernatants transferred to fresh microcentrifuge tubes, and the debris removed, followed by two centrifugations with the application of liporemoval column each for 1 min at 500 × g and 4°C. A miRCURY Exosome Cell/Urine/CSF Kit (Qiagen, #76743) was used to precipitate EVs. Precipitation buffer B was added to pretreated serum, followed by overnight incubation and centrifugation at 10,000 × g for 30 min at 20°C. The supernatants were discarded.
Nanoparticle Tracking Analysis (NTA)
All samples were thawed at room temperature and diluted in PBS to final volume of 1 ml. Smaple was introduced to the NanoSight LM10 (NanoSight Ltd., Amesbury, United Kingdom) through glass prism.
Following settings were set acoording to the previous study (Dragovic et al., 2011; Soo et al., 2012). All particles that dispersed enough light to be above the detection threshold are counted by the NTA software version 2.3 and this is used to determine the concentration.
miRNA Sequencing
The RNA isolated from each sample was used to construct sequencing libraries with the SMARTer smRNA-Seq Kit for Illumina, following the manufacturer's protocol. Briefly, Input RNA is first polyadenylated in order to provide a priming sequence for an oligo-(dT) primer. cDNA synthesis is primed by the 3′ smRNA dT Primer, which incorporates an adapter sequence at the 5′ end of each RNA template, it adds non templated nucleotides which are bound by the SMRT smRNA Oligo-enhanced with locked nucleic acid (LNA) technology for greater sensitivity. In the template-switching step, PrimeScript RT uses the SMART smRNA Oligo as a template for the addition of a second adapter sequence to the 3′ end of each first-strand cDNA molecule. In the next step, full-length Illumina adapters (including index sequences for sample multiplexing) are added during PCR amplification. The Forward PCR Primer binds to the sequence added by the SMART smRNA Oligo, while the Reverse PCR Primer binds to the sequence added by the 3' smRNA dT Primer. Resulting library cDNA molecules include sequences required for clustering on an Illumina flow cell.
The libraries were validated by checking the size, purity, and concentration on the Agilent Bioanalyzer. The libraries were pooled in equimolar amounts, and sequenced on an Illumina HiSeq 2500 instrument to generate 51 base reads. Image decomposition and quality values calculation were performed using the modules of the Illumina pipeline.
Intracerebroventricular (ICV) Injection
Mice were anesthetized with isoflurane and placed in a stereotactic frame. The lateral ventricle(AP: −0.5 mm, ML: 1 mm, DV: −2.5 mm) was defined using the bregma and lambda values and 20 μg of EVs injected.
Tissue Collection
Tissues were collected the day after the 4 weeks of exercise and 3 days after ICV injection. Mice were anesthetized with isoflurane and whole heart blood collected. After perfusion with phosphate-buffered saline, whole brains were collected and samples placed in 4% (v/v) paraformaldehyde or frozen at −80°C.
Biochemical Tests
(i) Reverse transcription-quantitative polymerase chain reaction (RT-qPCR)
Total RNA was isolated and purified using TRIzol reagent (Invitrogen, #15596026) according to the manufacturer's protocol. cDNA was prepared to employ a CycleScript RT premix (Bioneer, #K-2044-CFG). All primer sequences are listed in Table 1. Expression levels were calculated using a SensiFAST SYBR Lo-ROX Kit (Bioline, #BIO-94020) and a commercial detection system (BioRad, #CFX96).
(ii) Western blotting
Total proteins were extracted into RIPA buffer (Thermo Fisher Scientific, #89900) containing a phosphatase inhibitor (Sigma-Aldrich, #4906845001) and a protease inhibitor (Roche, #43693159001), subjected to 10–16% (w/v) Tris-glycine SDS-PAGE, transferred to PVDF membranes using Iblot 2 NC ministacks (Invitrogen, #IB23002), and the membranes blocked with 5% (w/v) skim milk. The primary antibodies were anti-pAMPK (Cell Signaling Technology, #2531S), anti-AMPK (Cell Signaling Technology, #5831S), anti-pACC (Cell Signaling Technology, #3661S), anti-ACC (Cell Signaling Technology, #3662S), and anti-GAPDH (Cell Signaling Technology, #2118S) diluted 1:1000 (primary antibodies) or 1:5000 (secondary antibodies) in TBST (Biosesang, #HT2007) containing 5% (w/v) skim milk. The membranes were then incubated with a peroxidase-conjugated anti-rabbit secondary antibody and signals quantitated using the Immobilion Western Chemiluminescent HRP Substrate (Millipore, #WBKLS0500).
Behavioral Tests
All behavioral tests were blindly scored by three individuals.
(i) Nest building test
Nest-building behavior commenced at 7:00 pm. Pressed cotton squares (~3 g) were placed in all cages. After dark, every cage was photographed and the cotton removed. Nest-building was scored using the Deacon method (Deacon, 2006).
(ii) Open field test
The open-field test square was a 40 × 40 cm black, polyvinyl chloride, plastic board surrounded by a 40-cm wall. Areas within 10 cm of the wall were considered to be peripheral and other areas central. Time spent in the center and the number of center entries increase when anxiety is low. At the beginning of the test, mice were placed in the central area and were allowed to move freely for 5 min. All sessions were recorded by a video camera placed 145 cm above the square and the data analyzed with the aid of the ANY-maze Video Tracking System (Stoelting Co., Wood Dale, IL, USA). The software detects an animal-based on its contrast with the background. After every session, the square was cleaned with a paper towel dampened with 70% (v/v) alcohol. All open field tests were conducted at 3:00 PM.
(iii) Tail suspension test
The tail suspension test was performed using the method of (Can et al., 2012). Each mouse was suspended on a climbstopper for 6 min by applying adhesive tape to the tail. The climbstopper disturbs the tail-climbing habit of the C57BL/6J mouse. The total duration of immobility was measured in seconds. Mobility was defined as any bodily shaking or limb movement akin to running. Immobility was defined as the absence of movement apart from breathing motion.
(iv) Forced swim test
The forced swim test was rehearsed on the day before the test. Each mouse was placed into a 2-L beaker containing a 20-cm depth of water at 23–25°C for 5 min; and immobility, and swimming and climbing behavior, was scored every 5 s.
Microarray and Bioinformatic Analysis
Each hippocampus from non-restrained vehicle-injected group (NV, n = 3), restraint-stressed sedentary EV-injected group (RS, n = 4), restraint-stressed exercise EV-injected group (RE, n = 4) were pooled and RNA purity and integrity were confirmed by ND-1000 Spectrophotometer (Nano-Drop, Wilmington, USA). The Affymetrix Whole transcript Expression array process was performed according to the protocol of the manufacturer (Gene Chip Whole Transcript PLUS reagent Kit). cDNA was synthesized according to the manufacturer's method using Gene Chip Whole Transcript (WT) Amplification kit, and the hybridized array was scanned using a GCS3000 Scanner (Affymetrix). Signal values were calculated using Affymetrix® Gene ChipTM Command Console software. Gene enrichment and functional annotation analysis were performed on genes showing significant expression level (≥2-fold) based on gene ontology and KEGG pathway analysis, respectively.
Statistical Analysis
Statistical analysis was performed with the aid of Graph Pad Prism ver. 7 (Graph Pad Software Inc., La Jolla, CA, USA); all data are presented as means with SEMs. A p-value < 0.05 was considered to reflect statistical significance. Student's t-test was used to compare data between the two groups.
Results
Effects of 4 Weeks of Voluntary Wheel Exercise on Skeletal Muscle Exercise-Related AMPK Signaling Protein and Oxidative Phosphorylated mRNA Levels
To validate the effects of 4 weeks of voluntary wheel exercise, mice were individually housed with or without an exercise wheel for 4 weeks (Figure 1A) and the levels of exercise-related proteins (phosphorylated AMPK and ACC) measured, as were the levels of encoding mRNAs (oxidative phosphorylation [Nudfa, Sdhb, and Cox5b], and Pgc1α). AMPK and ACC are an important signaling molecule that is activated during exercise training (Chen et al., 2003). The p-AMPK/AMPK and p-ACC/ACC ratios of exercise group was significantly higher than sedentary group (Figures 1B–D, p-AMPK/AMPK, p < 0.05 and p-ACC/ACC, p = 0.0507). Endurance exercises upregulates the gene expressions of oxidative phosphorylation and Pgc1α that assist promote mitochondrial biogenesis in skeletal muscles (Gureev et al., 2019). Nudfa expression levels did not differ between the sedentary and exercise groups. Sdhb expression was upregulated in the exercise group (p < 0.05) and the levels of Cox5b and Pgc1α tended (p = 0.06, p = 0.07, respectively) to increase in that group (Figures 1E–H). These experiments indicated that 4 weeks of voluntary wheel exercise was sufficient for further study.
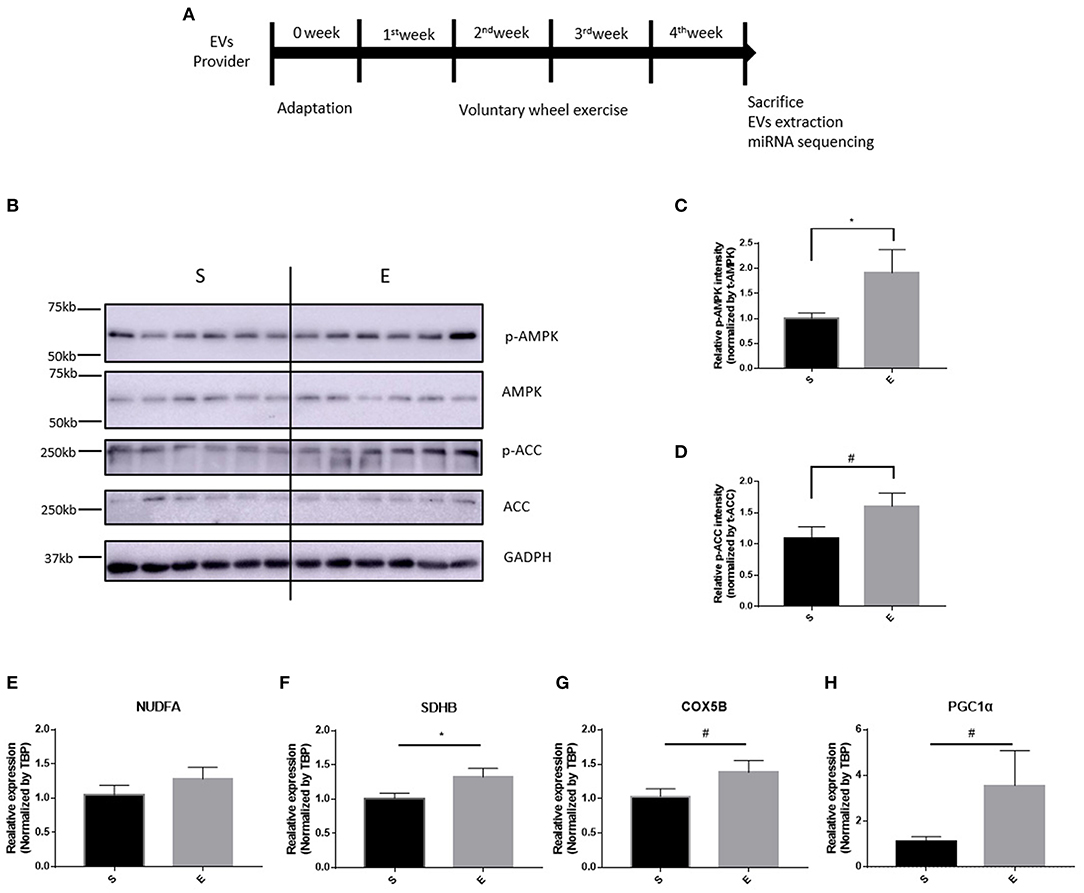
Figure 1. The effects of 4 weeks of voluntary wheel exercise on skeletal muscle proteins and their encoding mRNA levels. (A) The experimental schedule. (B–D) p-AMPK/AMPK and p-ACC/ACC protein levels. (E–H) OXPHOS and Pgc1α mRNA expression levels in the gastrocnemius. *p < 0.05 vs. S. Statistical analysis was performed with the aid of the one-tailed Student t-test. EVs, Extracellular vesicles; S, sedentary group (n = 6); E, exercise group (n = 6). The data represent means ± SEM (*p < 0.05). #p < 0.05.
4 Weeks of Running Wheel Exercise Did Not Changed Serum-Derived EVs External Properties: Size and Number
After isolation of extracellular vesicles from each group's sera, we performed a nanoparticle tracking assay for investigating the difference between groups; Figure 2A shows the sample frame. Neither EVs diameter nor size changed over the 4 weeks of exercise (Figures 2B,C,E, p > 0.05). Figure 2D shows that the level of CD9 (an EVs biochemical marker) was 5 μg/EVs in both groups.
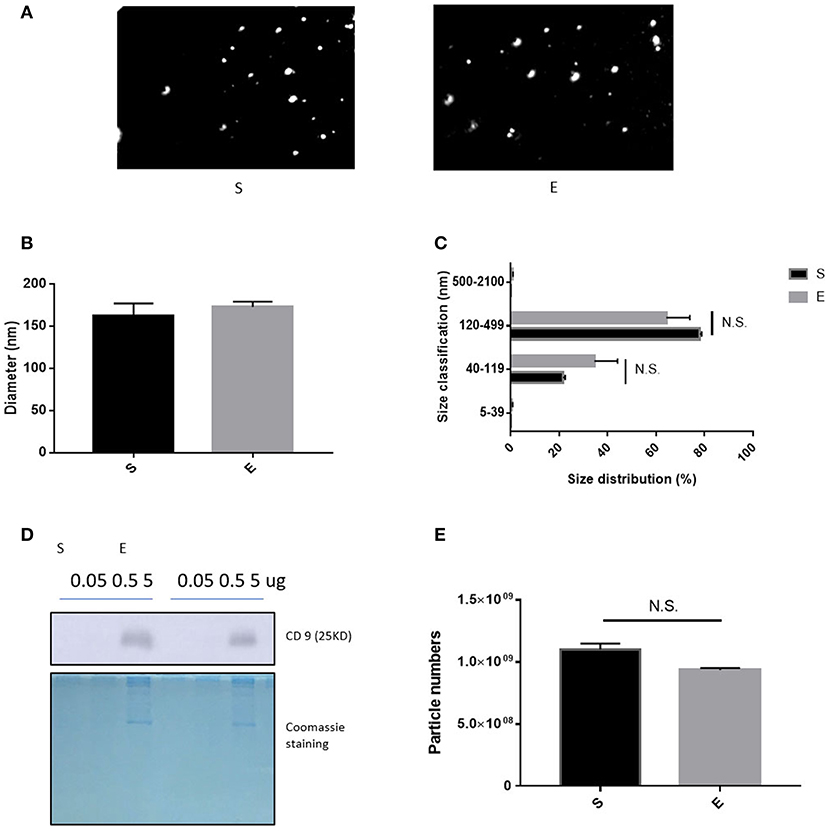
Figure 2. The features of EVs induced by 4 weeks of voluntary wheel exercise. (A) A representative video frame. (B) The average diameter. (C) The size distribution. (D) Western blotting for CD9 (an EV-specific marker). (E) EV particle numbers. N.S., not significant. Statistical analysis was performed with the aid of the two-tailed Student t-test.
EVs miRNA Internal Profiles Were Altered After 4 Weeks of Voluntary Wheel Exercise
To figure out the internal composition of extracellular vesicles, we designed the study comparing sedentary and exercise group's miRNA. The EVs of mice that engaged in 4 weeks of voluntary wheel exercise exhibited 46 upregulated and 36 downregulated miRNAs compared to sedentary group (Tables 2A,B). Particularly, we confirmed 4 miRNAs to validate the miRNA sequencing (Supplementary Figures 1A–D). All changed miRNAs were functionally annotated by DAVID ver. 6.7 software, except those lacking ENTERZ GENE IDs. The miRNAs affected the micro-ribonucleoprotein complex, cancer development, the responses to oxygen and amino acids, and gene silencing (Table 3).
The 82 miRNAs' predicted target genes were identified with the aid of miRNA databases, Target Scan and the miRDB. Both databases identified 129 and 119 genes regulated by more than two miRNAs (Table 4); 13 genes were common to both the Target Scan and miRDB lists. Target scan genes were subjected to functional analysis using Cytoscape (Figure 3A). Of the 129 genes, 27.27% were associated with the neurotrophin signaling pathway (9.09% with each of central nervous system neuronal development, cerebral cortex cell migration, and peripheral neuron development; Figure 3B). These data implied exercise-induced EVs can affect the neuronal cell.
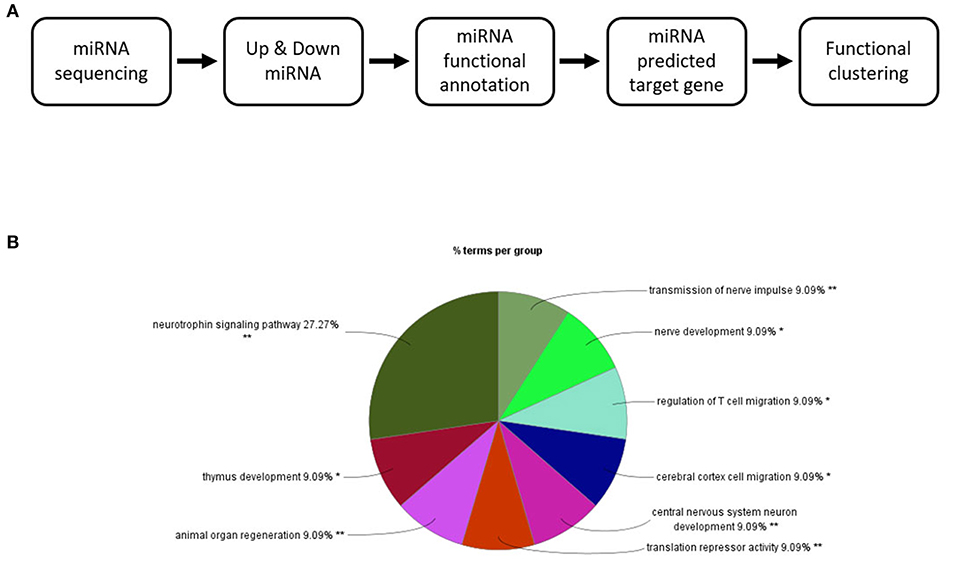
Figure 3. Changes in the EV miRNA profiles after 4 weeks of voluntary wheel exercise. (A) A bioinformatic schematic. (B) The target genes predicted by functional clustering. Each sedentary, exercise group animal number was 6.
EVs Derived by 4 Weeks of Voluntary Wheel Exercise Increased Neuro2A Cell Viability
To verify the hypothesis, we conducted cellular viability experiments using Neuro2A, a neuroblastoma cell line. The addition of fetal bovine serum to 10% (v/v) increased Neuro2A cell viability 1.2-fold relative to the MEM-only control (Figure 4). Twenty-four hours treatment of 10 ng of exercise-induced EVs enhanced the viability of Neuro2A cell compared to the same dosage of sedentary (Figure 4). This result that exercise-derived EVs affect positively on Neuro2A cell viability.
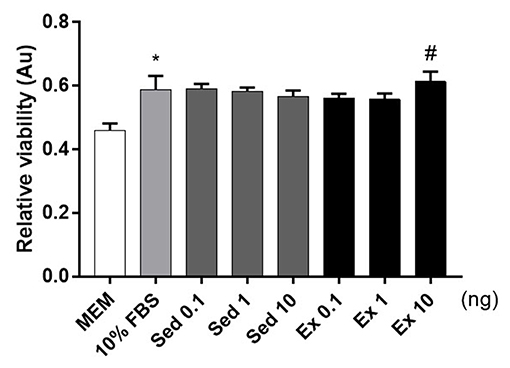
Figure 4. Effects of 4-week, voluntary running wheel exercise-induced EVs on Neuro2A cell viability levels. After 24 h of treatment with MEM alone, 10% (v/v) FBS, or 0.1, 1, or 10 ng of EVs from the sedentary or exercised group. *p < 0.05 compared to the MEM control. #p < 0.05 compared to the Sed 10 group. Statistical analysis was performed with the aid of the ANOVA test.
EVs Induced by Running Exercise Ameliorated Anxiety Behaviors
It is well-known that emotionally afflicted mice are accompanied by decreased neuronal cell viability. Exercise, on the contrary, promotes the production of nerve cells and increases resistance to stress. Therefore, we conducted a behavioral experiment to find out whether exercise-derived EV directly affects beneficial changes in stressed mice. Mice were housed for 4 weeks with or without a wheel after 1 week of acclimatization and restraint stressed mice received EVs from exercised mice via ICV injection (Figure 5A). Three weeks of restraint stress-induced a tendency of depression, as revealed by reduced mobility on the tail suspension test (Figure 5B, p = 0.15). Restraint stress-treated mice also scored lower than the non-treated group in the nest-building test, thus exhibiting anxiety-like behavior (Figure 5C, p = 0.0519).
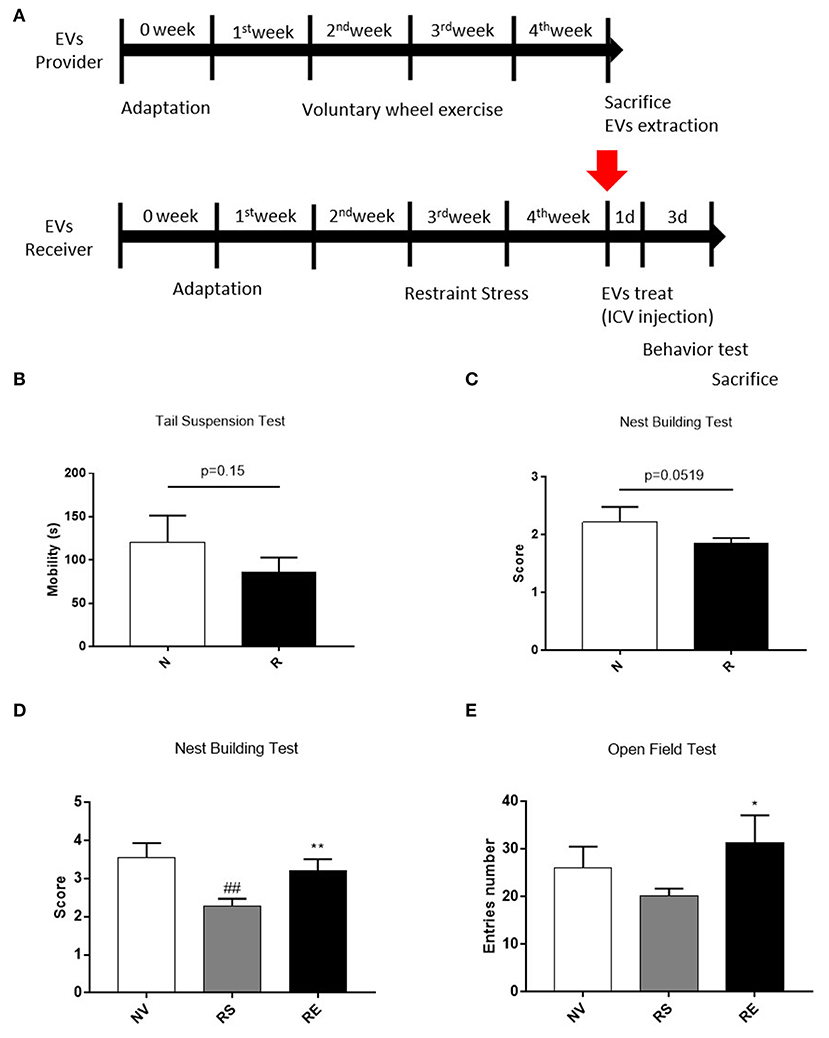
Figure 5. The effects of 4 weeks of voluntary running wheel exercise-induced EVs on anxiety. (A) The experimental design (red arrows indicate EV ICV injection). (B) The mobility durations of the non-restrained and restrained groups on the tail-suspension test. (C) The group scores on the nest- building test prior to ICV injection. (D) The group scores on the nest-building test after ICV injection. (E) The center entry numbers in the open field test after ICV injection. All mice were male. N, non-restrained (6-week-old, n = 5); R, restrained (thus stressed) (6-week-old, n = 15); NV, non-restrained vehicle-injected group (11-week-old, n = 5); RS, restraint-stressed sedentary EV-injected group (11-week-old, n = 5); RE, restraint-stressed exercise EV-injected group (11-week-old, n = 5). *p < 0.05, **p < 0.001 compared to the NV group, ##p < 0.001 compared to the RS group. Statistical analysis was performed using the one-tailed Student t-test.
Exercise-induced EVs injection increased the nest-building test scores compared to sedentary-induced EVs injected (Figure 5D, p < 0.001). The number of center entries in the open field test was higher in exercise-induced EVs injected mice (Figure 5E, p < 0.05). However, basic behavior did not differ between the two groups (data not shown). These results elucidated that the exercise-derived EVs have the positive effect on anxiety behaviors.
Restraint Stress and Exercise-Induced EVs Altered Hippocampal mRNA Levels
Through microarray experiments, we directly investigated changes of gene profiles in the hippocampus of EVs injected mice. Restraint stress transformed the microarray hierarchical clustering heat map image (Figure 6). However, few changes were noted after EVs injection (Figure 6). Restraint stress significantly changed the expression levels of genes associated with long-term depression (Plcb4, Gucy1a3, Prkg2, Ppp1r17, Grid2, and Crhr1) in the Kyoto Encyclopedia of Genes and Genomes (KEGG) map (Table 5). Exercise-induced EVs injection upregulated the expression of genes associated with neuroactive ligand-receptor interaction (Tacr3, Gabrr1, Cysltr1, Oprm1, and Trhr) in the KEGG map (Table 6).
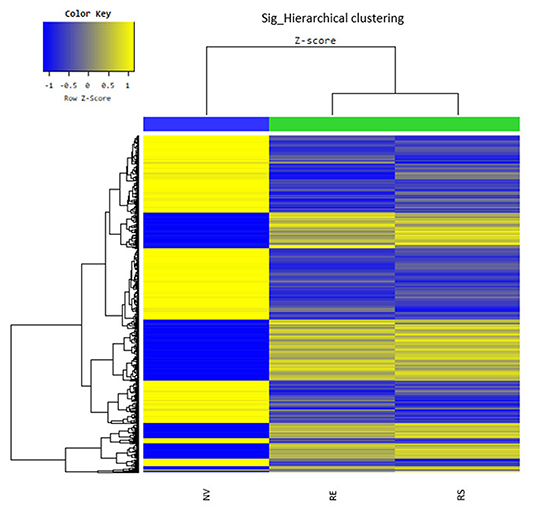
Figure 6. The hippocampal effects of 4 weeks of voluntary wheel exercise-induced EVs. The microarray hierarchical clustering heat-map image. NV, non-restrained vehicle-injected group (n = 3); RS, restraint-stressed sedentary EV-injected group (n = 4); RE, restraint-stressed exercise EV-injected group (n = 4).
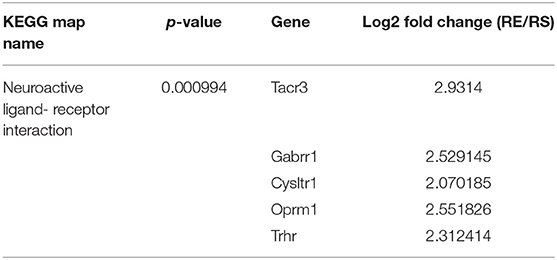
Table 6. The list of changed gene by exercise-induced EVs, fold change means restraint exercise/restraint sedentary.
Discussion
Anxiety disorders are characterized by excessive worry about events or activities that persists for at least 6 months (American Psychiatric Association, 2013). Large-scale surveys have found that 33.7% of subjects suffer anxiety disorders during life (Alonso and Lépine, 2007). Anxiety disorders are comorbid with other mental illnesses, creating major societal burdens. However, the common drugs trigger side effects including nausea, weight gain, and sleepiness (Lord et al., 2017). Thus, new therapies are required (Wegner et al., 2014; Stonerock et al., 2015).
Exercise is a powerful treatment, improving PTSD, sub-element anxiety disorders, and generalized anxiety disorder (Herring et al., 2012; Rosenbaum et al., 2015). Exercise increased neural precursor cell proliferation in the mouse dentate gyrus (Pons-Espinal et al., 2019). Besides, exercise intervention may ameliorate not only cognitive but also behavior function in individuals with Parkinson's disease. Physical activity can protect from cognitive impairment in Alzheimer's disease. These experiments attest that exercise helps individuals to mitigate neural disease (Petzinger et al., 2013; Pin-Barre and Laurin, 2015; Cui et al., 2018).
EVs may modulate the beneficial effects of exercise (Safdar and Tarnopolsky, 2018; Whitham et al., 2018). EVs contain proteins, nucleic acids, lipids, and cell metabolites (Maji et al., 2017); and engage in inter-organ signaling (Pitt et al., 2016). Previous studies showed that exercise-induced EVs exerted positive effects on neuronal systems (Safdar and Tarnopolsky, 2018; Moon et al., 2020). However, whether such EVs reduce anxiety remains unclear. Therefore, we designed the experiments and found that anxiety was relieved through intracerebroventricularly injection of such EVs.
Most previous studies found that 4 weeks of voluntary wheel exercise significantly increased exercise-related signaling (Tanner et al., 2013; Kurauti et al., 2016; Liao et al., 2017). Phosphorylated AMPK and ACC, which are activated by exercise, promote ATP production and increase rates of myocardial fatty acid oxidation. Also, oxidative phosphorylation and Pgc1α mRNAs that promotes mitochondrial biogenesis are increased by endurance exercise. Thus, these factors are commonly used as exercise markers. Our results showed that the p-AMPK and p-ACC levels in the gastrocnemius muscle were upregulated and the levels of mRNAs encoding PGC1α, oxidative phosphorylated genes tended to rise; 4 weeks of voluntary wheel exercise was sufficient for the following studies.
EVs consist of a bilayer membrane that makes it possible to travel inter-organs. We isolated EVs from each group's sera for demonstrating the differences between groups. The EVs of both the exercised and sedentary groups 5 μg of EVs contained CD9 which is considered as EVs positive marker. The EVs were characterized via nanoparticle tracking. In accordance with the previous result (Oliveira et al., 2018), the EVs diameter, size, and number did not differ between the groups. Recent studies showed that EVs were released during cycling and declined when resting (Frühbeis et al., 2015; Whitham et al., 2018; Brahmer et al., 2019). Another study demonstrated that plyometric jumping and downhill running did not change vesicle number or size (Lovett et al., 2018). Differences in EVs diameter, numbers, and size vary by species, the type and intensity of exercise, and the timing of measurements.
EVs can carry proteins nucleic acids, lipids, and metabolites. Among that, miRNAs are small non-coding RNAs that regulate protein expression via mRNA degradation or inhibition of translation. Specific miRNAs are associated with chronic fatigue syndrome, depression, Alzheimer's disease, and Huntington's disease (Hoss, 2015; Wei et al., 2016; Baraniuk and Shivapurkar, 2017; Wu et al., 2017). However, few reports focused on miRNA changes in exercise-induced EVs (D'Souza et al., 2018; Lovett et al., 2018; Hou et al., 2019; Yin et al., 2019; Nair et al., 2020). Therefore, We compared the EVs miRNAs through miRNA sequencing in sedentary and exercised mice; 46 miRNAs were upregulated and 36 downregulated in the exercise group. Among that, miR-10b-5p was upregulated in the rat hippocampus after 4 weeks of treadmill exercise (Fernandes et al., 2018) and miR-let-7i was downregulated in the plasma of athletes after exercise (Nielsen et al., 2014), consistent with our findings. Furthermore, as our results, the elevation of miR-342-5p in exercise-released exosome showed that reduced apoptosis and LDH release (a marker of tissue damage), increased cardiomyocyte viability (Hou et al., 2019). Wang et al. (2019) demonstrated that upregulated exosomal miR-29 plays important role in muscle atrophy and kidney fibrosis in mice. Thus, we confirmed those miRNA sequencing data through RT-qPCR in sera isolated miRNAs (Supplementary Figure 1) and illustrated cumulative distribution plots of miR-29b-3p target and non-target mRNAs (Supplementary Figure 2).
We defined all miRNA-targeted genes with the aid of Target Scan and miRDB, and performed a functional analysis with the aid of Cytoscape. The genes were involved in neurotrophin signaling, central nervous system neuronal development and cerebral cortex cell migration, and peripheral nerve development. Previous studies also confirmed that exercise-induced EVs play roles in nervous system communication (Galieva et al., 2019; Liu et al., 2019). Physical activity modulates the miRNA level which can affect on memory (Fernandes et al., 2017). Also, Exercise-induced extracellular derivatives enhanced neural maturation (Moon et al., 2020). Therefore, we treated Neuro2A cells with exercise-induced EVs 10 μg and it exhibited enhanced viability; the effects of sedentary EVs were less clear. This result indicates that exercise-derived EVs might be actively involved in anxiety disorders. However, because we could not have conducted the miRNA-mediated gene silencing, it might not relevant between miRNAs and cell viability.
A previous study shows that EVs are delivered hippocampus through ICV injection (Nakano et al., 2016; Micci et al., 2019). According to the positive effect on Neuro2A cell viability result of in vitro study, the anxiety model was made by 3 weeks of restraint stress and then treated EVs directly through ICV injection. Chronic stress diminishes the neurogenesis (Duman, 2009). Restraint is well-known to induce depressive- and anxiety-like behavior (Chiba et al., 2012; Ampuero et al., 2015). The nest-building test showed that restraint stress evoked anxiety-like behavior. However, tail suspension test demonstrated a tendency in depressive-like behavior. In earlier work, 2 h per day over 7 days of restraint stress-triggered adaptive behavioral changes in anxiety- and depressive-like phenotype (Sadler and Bailey, 2016). Likewise, a previous study demonstrated that differential effects between the restraint methods (Shoji and Miyakawa, 2020).
After ICV injection, exercise-induced EVs treated group was rescued nest-building and central zone entries to significantly greater extents than sedentary. Exercise-derived EVs, thus alleviating anxiety. However, depressive behavior was not affected. We inject only one EV dose; this may be a limitation of the study. In addition, the 3 days of rehabilitation may have diluted the EV effects. Repeat EV injections via a cannula or osteomic pump are required in a future study.
We used a microarray to explore changes in hippocampal gene expression. In the restrained group, genes involved in long-term depression (Plcb4, Gucy1a3, Prkg2, Ppp1r17, Grid2, and Crhr1) were downregulated compared to the control group. These genes are also associated with anxiety; medial septal Plcb4 knockout mice exhibited more anxiety-like behaviors (Shin et al., 2009). Gucy1a3 expression in the amygdala correlated positively with social behavior in the rhesus monkey (Sabatini et al., 2007). Prkg2 encodes cGK type II (cGKII), which regulates hippocampal synaptic plasticity (Serulle et al., 2007). Anxiety-like behaviors increased in cGKII knockout mice (Werner et al., 2004). Crhr1 encodes corticotropin-releasing factor 1 (CRF1); a CRF1 antagonist was anxiolytic in mice (Marcinkiewcz et al., 2016), associated with dose-related changes in regional cerebral glucose metabolism in regions involved in mood and anxiety (Schmidt et al., 2009). Ppp1r17 and Grid2 have not been studied in the context of anxiety.
Exercise-induced EVs injected restrained group exhibited higher levels of the neuroactive ligand-receptor interaction genes (Tacr3, Gabrr1, Cysltr1, Oprm1, and Trhr) than sedentary-derived EVs injected group. Tacr3 overexpression in the unilateral habenula significantly reduced anxiety-like behavior (Cui et al., 2020). By contrast, Tacr3 expression in the amygdala of the NIH-HS rat was higher in a high-anxious group compared with a low-anxious group (Díaz-Morán et al., 2013). Gabrr1 and Cysltr1 has few previous studies related to anxiety and depression. Gabrr1 encodes an ionotropic GABAA receptor that inhibits neuronal activity. Early-life stress such as maternal separation can evoke anxiety-like behavior later in life (Wang et al., 2020). Gabrr1 was upregulated in the pineal gland of rats subjected to long-term maternal separation (Steine et al., 2016). Cysltr1 encodes cysteinyl leukotriene receptor 1; hippocampal knockdown prevented depressive-like behavior in mice (Yu et al., 2016). Oprm1 has been extensively studied in the context of single nucleotide polymorphisms (SNPs) (Mague and Blendy, 2010; Mura et al., 2013; Boparai et al., 2018). The gene encodes the μ opioid receptor. The G allele is a minor form associated with higher resting cortisol levels, blunting of the cortisol response to stress, and separation anxiety disorder (Mague and Blendy, 2010; Boparai et al., 2018). Also, the SNP affects the mRNA expression level, reducing expression by 50% compared to that of the A allele, inducing anxiety (Mura et al., 2013). Trhr encodes the receptor for thyrotropin-releasing hormone (TRH); TRH-R1 knockout mice exhibited increased anxiety- and depressive-like behaviors (Zeng et al., 2007). Low levels of exploratory activity in novel environment rats which are highly inhibited and anxious, depressive-like behavior expressed low Trhr level in dentate gyrus where neural proliferation within the hippocampus (Birt et al., 2021).
We found no significant difference in EV physical properties between the exercise and sedentary groups, but their physiological characteristics varied (in a manner that may affect the nervous system). Exercise-induced EVs contributed to neuronal cell viability and mitigated anxiety-like behavior. Thus, exercise-induced EVs may serve as anxiolytics.
Data Availability Statement
The datasets presented in this study can be found in online repositories. The names of the repository/repositories and accession number(s) can be found at: https://www.ncbi.nlm.nih.gov/geo/query/acc.cgi?acc=GSE168765.
Ethics Statement
The animal study was reviewed and approved by Institutional Animal Care and Use Committee of Seoul National University.
Author Contributions
KY wrote the paper and collected the data. SP contributed data and performed the analysis. SK performed the analysis. HM designed the experiments. All authors contributed to the article and approved the submitted version.
Funding
This work was supported by the National Research Foundation (NRF, grant number: 2020R1C1C1006414).
Conflict of Interest
The authors declare that the research was conducted in the absence of any commercial or financial relationships that could be construed as a potential conflict of interest.
Supplementary Material
The Supplementary Material for this article can be found online at: https://www.frontiersin.org/articles/10.3389/fnmol.2021.665800/full#supplementary-material
Supplementary Figure 1. The validation of 4 weeks of voluntary wheel exercise mice sera-isolated EVs miRNAs through RT-qPCR. (A–D) miR-10b-5p, miR-let-7i-5p, miR-342-3p, and miR-29b-3p miRNA levels normalized by miR-16. ***p < 0.001, ****p < 0.0001 vs. S. Statistical analysis was performed with the aid of the one-tailed Student t-test. S, sedentary group (n = 6); E, exercise group (n = 6). The data represent means ± SEM (*p < 0.05).
Supplementary Figure 2. Cumulative distribution plots of miR-29-3p target and non-target genes. (A) Fold change means restraint / non-restraint group. (B) Fold change means restraint exercise / restraint sedentary group.
References
Alonso, J., and Lépine, J. P. (2007). Overview of key data from the European Study of the Epidemiology of Mental Disorders (ESEMeD). J. Clin. Psychiatry 68(Suppl.2), 3–9.
American Psychiatric Association (2013). DSM-5: Diagnostic and Statistical Manual of Mental Disorders, 947. doi: 10.1176/appi.books.9780890425596
Ampuero, E., Luarte, A., Santibañez, M., Varas-Godoy, M., Toledo, J., Diaz-Veliz, G., et al. (2015). Two chronic stress models based on movement restriction in rats respond selectively to antidepressant drugs: aldolase C as a potential biomarker. Int. J. Neuropsychopharmacol. 18:pyv038. doi: 10.1093/ijnp/pyv038
Baraniuk, J. N., and Shivapurkar, N. (2017). Exercise – induced changes in cerebrospinal fluid miRNAs in Gulf War Illness, Chronic Fatigue Syndrome and sedentary control subjects. Sci. Rep. 7:15338. doi: 10.1038/s41598-017-15383-9
Bei, Y., Xu, T., Lv, D., Yu, P., Xu, J., Che, L., et al. (2017). Exercise-induced circulating extracellular vesicles protect against cardiac ischemia-reperfusion injury. Basic Res. Cardiol. 112:38. doi: 10.1007/s00395-017-0628-z
Bet, P. M., Hugtenburg, J. G., Penninx, B. W., and Hoogendijk, W. J. (2013). Side effects of antidepressants during long-term use in a naturalistic setting. Eur. Neuropsychopharmacol. 23, 1443–1451. doi: 10.1016/j.euroneuro.2013.05.001
Birt, I. A., Hagenauer, M. H., Clinton, S. M., Aydin, C., Blandino, P., Stead, J. D. H., et al. (2021). Genetic liability for internalizing versus externalizing behavior manifests in the developing and adult hippocampus: insight from a meta-analysis of transcriptional profiling studies in a selectively bred rat model. Biol. Psychiatry 89, 339–355. doi: 10.1016/j.biopsych.2020.05.024
Boparai, S., Borelli, J. L., Partington, L., Smiley, P., Jarvik, E., Rasmussen, H. F., et al. (2018). Interaction between the opioid receptor OPRM1 gene and mother-child language style matching prospectively predicts children's separation anxiety disorder symptoms. Res. Dev. Disabil. 82, 120–131. doi: 10.1016/j.ridd.2018.03.002
Brahmer, A., Neuberger, E., Esch-Heisser, L., Haller, N., Jorgensen, M. M., Baek, R., et al. (2019). Platelets, endothelial cells and leukocytes contribute to the exercise-triggered release of extracellular vesicles into the circulation. J. Extracell. Vesicles 8:1615820. doi: 10.1080/20013078.2019.1615820
Can, A., Dao, D. T., Terrillion, C. E., Piantadosi, S. C., Bhat, S., Gould, T. D., et al. (2012). The tail suspension test. J. Vis. Exp. 59:e3769. doi: 10.3791/3769
Chen, Z. P., Stephens, T. J., Murthy, S., Canny, B. J., Hargreaves, M., Witters, L. A., et al. (2003). Effect of exercise intensity on skeletal muscle AMPK signaling in humans. Diabetes 52, 2205–2212. doi: 10.2337/diabetes.52.9.2205
Chiba, S., Numakawa, T., Ninomiya, M., Richards, M. C., Wakabayashi, C., and Kunugi, H. (2012). Chronic restraint stress causes anxiety- and depression-like behaviors, downregulates glucocorticoid receptor expression, and attenuates glutamate release induced by brain-derived neurotrophic factor in the prefrontal cortex. Prog. Neuropsychopharmacol. Biol. Psychiatry 39, 112–119. doi: 10.1016/j.pnpbp.2012.05.018
Craske, M. G., Stein, M. B., Eley, T. C., Milad, M. R., Holmes, A., Rapee, R. M., et al. (2017). Anxiety disorders. Nat. Rev. Dis. Primers 3:17024. doi: 10.1038/nrdp.2017.24
Cui, M. Y., Lin, Y., Sheng, J. Y., Zhang, X., and Cui, R. J. (2018). Exercise intervention associated with cognitive improvement in Alzheimer's disease. Neural Plasticity 2018:9234105. doi: 10.1155/2018/9234105
Cui, W. Q., Zhang, W. W., Chen, T., Li, Q., Xu, F., Mao-Ying, Q. L., et al. (2020). Tacr3 in the lateral habenula differentially regulates orofacial allodynia and anxiety-like behaviors in a mouse model of trigeminal neuralgia. Acta Neuropathol. Commun. 8:44. doi: 10.1186/s40478-020-00922-9
Deacon, R. M. (2006). Assessing nest building in mice. Nat. Protoc. 1, 1117–1119. doi: 10.1038/nprot.2006.170
Díaz-Morán, S., Palència, M., Mont-Cardona, C., Cañete, T., Blázquez, G., Martínez-Membrives, E., et al. (2013). Gene expression in amygdala as a function of differential trait anxiety levels in genetically heterogeneous NIH-HS rats. Behav. Brain Res. 252, 422–431. doi: 10.1016/j.bbr.2013.05.066
Dragovic, R. A., Gardiner, C., Brooks, A. S., Tannetta, D. S., Ferguson, D. J. P., Hole, P., et al. (2011). Sizing and phenotyping of cellular vesicles using Nanoparticle Tracking Analysis. Nanomed. Nanotechnol. Biol. Med. 7, 780–788. doi: 10.1016/j.nano.2011.04.003
D'Souza, R. F., Woodhead, J. S. T., Zeng, N., Blenkiron, C., Merry, T. L., Cameron-Smith, D., et al. (2018). Circulatory exosomal miRNA following intense exercise is unrelated to muscle and plasma miRNA abundances. Am. J. Physiol. Endocrinol. Metab. 315, E723–e733. doi: 10.1152/ajpendo.00138.2018
Duman, R. S. (2009). Neuronal damage and protection in the pathophysiology and treatment of psychiatric illness: stress and depression. Dialog. Clin. Neurosci. 11, 239–255. doi: 10.31887/DCNS.2009.11.3/rsduman
Fernandes, J., Arida, R. M., and Gomez-Pinilla, F. (2017). Physical exercise as an epigenetic modulator of brain plasticity and cognition. Neurosci. Biobehav. Rev. 80, 443–456. doi: 10.1016/j.neubiorev.2017.06.012
Fernandes, J., Vieira, A. S., Kramer-Soares, J. C., Da Silva, E. A., Lee, K. S., Lopes-Cendes, I., et al. (2018). Hippocampal microRNA-mRNA regulatory network is affected by physical exercise. Biochim. Biophys. Acta Gen. Subj. 1862, 1711–1720. doi: 10.1016/j.bbagen.2018.05.004
Frühbeis, C., Helmig, S., Tug, S., Simon, P., and Krämer-Albers, E. M. (2015). Physical exercise induces rapid release of small extracellular vesicles into the circulation. J. Extracell. Vesicl. 4:28239. doi: 10.3402/jev.v4.28239
Galieva, L. R., James, V., Mukhamedshina, Y. O., and Rizvanov, A. A. (2019). Therapeutic potential of extracellular vesicles for the treatment of nerve disorders. Front. Neurosci. 13:163. doi: 10.3389/fnins.2019.00163
Gureev, A. P., Shaforostova, E. A., and Popov, V. N. (2019). Regulation of mitochondrial biogenesis as a way for active longevity: interaction between the Nrf2 and PGC-1α signaling pathways. Front. Gen. 10:435. doi: 10.3389/fgene.2019.00435
Herring, M. P., Jacob, M. L., Suveg, C., Dishman, R. K. O., and Connor, P. J. (2012). Feasibility of exercise training for the short-term treatment of generalized anxiety disorder: a randomized controlled trial. Psychother. Psychosomat. 81, 21–28. doi: 10.1159/000327898
Hoss, A. G. (2015). miR-10b-5p expression in Huntington's disease brain relates to age of onset and the extent of striatal involvement. BMC Med. Genom. 8:10. doi: 10.1186/s12920-015-0083-3
Hou, Z., Qin, X., Hu, Y., Zhang, X., Li, G., Wu, J., et al. (2019). Longterm exercise-derived exosomal miR-342-5p. Circulat. Res. 124, 1386–1400. doi: 10.1161/CIRCRESAHA.118.314635
Iny, L. J., Pecknold, J., Suranyi-Cadotte, B. E., Bernier, B., Luthe, L., Nair, N. P., et al. (1994). Studies of a neurochemical link between depression, anxiety, and stress from [3H]imipramine and [3H]paroxetine binding on human platelets. Biol. Psychiatry 36, 281–291. doi: 10.1016/0006-3223(94)90625-4
Janke, K. L., Cominski, T. P., Kuzhikandathil, E. V., Servatius, R. J., and Pang, K. C. (2015). Investigating the role of hippocampal BDNF in anxiety vulnerability using classical eyeblink conditioning. Front. Psychiatry 6:106. doi: 10.3389/fpsyt.2015.00106
Kurauti, M. A., Freitas-Dias, R., Ferreira, S. M., Vettorazzi, J. F., Nardelli, T. R., Araujo, H. N., et al. (2016). Acute exercise improves insulin clearance and increases the expression of insulin-degrading enzyme in the liver and skeletal muscle of swiss mice. PLoS ONE 11:e0160239. doi: 10.1371/journal.pone.0160239
Liao, Z. Y., Chen, J. L., Xiao, M. H., Sun, Y., Zhao, Y. X., Pu, D., et al. (2017). The effect of exercise, resveratrol or their combination on Sarcopenia in aged rats via regulation of AMPK/Sirt1 pathway. Exp Gerontol. 98, 177–183. doi: 10.1016/j.exger.2017.08.032
Liu, W., Bai, X., Zhang, A., Huang, J., Xu, S., and Zhang, J. (2019). Role of exosomes in central nervous system diseases. Front. Mol. Neurosci. 12:240. doi: 10.3389/fnmol.2019.00240
Lord, C. C., Wyler, S. C., Wan, R., Castorena, C. M., Ahmed, N., Mathew, D., et al. (2017). The atypical antipsychotic olanzapine causes weight gain by targeting serotonin receptor 2C. J. Clin. Invest. 127, 3402–3406. doi: 10.1172/JCI93362
Lovett, J. A. C., Durcan, P. J., and Myburgh, K. H. (2018). Investigation of circulating extracellular vesicle MicroRNA following two consecutive bouts of muscle-damaging exercise. Front. Physiol. 9:1149. doi: 10.3389/fphys.2018.01149
Mague, S. D., and Blendy, J. A. (2010). OPRM1 SNP (A118G): involvement in disease development, treatment response, and animal models. Drug Alcohol. Depend. 108, 172–182. doi: 10.1016/j.drugalcdep.2009.12.016
Maji, S., Matsuda, A., Yan, I. K., Parasramka, M., and Patel, T. (2017). Extracellular vesicles in liver diseases. Am. J. Physiol. Gastrointest. Liver Physiol. 312, G194–G200. doi: 10.1152/ajpgi.00216.2016
Marcinkiewcz, C. A., Mazzone, C. M., D'Agostino, G., Halladay, L. R., Hardaway, J. A., DiBerto, J. F., et al. (2016). Serotonin engages an anxiety and fear-promoting circuit in the extended amygdala. Nature 537, 97–101. doi: 10.1038/nature19318
Micci, M. A., Krishnan, B., Bishop, E., Zhang, W. R., Guptarak, J., Grant, A., et al. (2019). Hippocampal stem cells promotes synaptic resistance to the dysfunctional impact of amyloid beta oligomers via secreted exosomes. Mol. Neurodegener. 14:25. doi: 10.1186/s13024-019-0322-8
Milad, M. R., and Quirk, G. J. (2012). Fear extinction as a model for translational neuroscience: 10 years of progress. Annu. Rev. Psychol. 63, 129–151. doi: 10.1146/annurev.psych.121208.131631
Moon, H. Y., Yoon, K. J., Lee, W. S., Cho, H. S., Kim, D. Y., and Kim, J. S. (2020). Neural maturation enhanced by exercise-induced extracellular derivatives. Sci. Rep. 10:3893. doi: 10.1038/s41598-020-60930-6
Mura, E., Govoni, S., Racchi, M., Carossa, V., Ranzani, G. N., Allegri, M., et al. (2013). Consequences of the 118A>G polymorphism in the OPRM1 gene: translation from bench to bedside? J. Pain Res. 6, 331–353. doi: 10.2147/JPR.S42040
Nair, V. D., Ge, Y., Li, S., Pincas, H., Jain, N., Seenarine, N., et al. (2020). Sedentary and trained older men have distinct circulating exosomal microRNA profiles at baseline and in response to acute exercise. Front. Physiol. 11:605. doi: 10.3389/fphys.2020.00605
Nakano, M., Nagaishi, K., Konari, N., Saito, Y., Chikenji, T., Mizue, Y., et al. (2016). Bone marrow-derived mesenchymal stem cells improve diabetes-induced cognitive impairment by exosome transfer into damaged neurons and astrocytes. Sci. Rep. 6:24805. doi: 10.1038/srep24805
Nielsen, S., Åkerström, T., Rinnov, A., Yfanti, C., Scheele, C., Pedersen, B. K., et al. (2014). The miRNA plasma signature in response to acute aerobic exercise and endurance training. PLoS ONE 9:e87308. doi: 10.1371/journal.pone.0087308
Oliveira, G. P., Porto, W. F., Palu, C. C., Pereira, L. M., Petriz, B., Almeida, J. A., et al. (2018). Effects of acute aerobic exercise on rats serum extracellular vesicles diameter, concentration and small RNAs content. Front. Physiol. 9:532. doi: 10.3389/fphys.2018.00532
Pande, N., Tantia, V., Javadekar, A., and Saldanha, D. (2013). Quality of life impairment in depression and anxiety disorders. Med. J. D. Y. Patil University 6, 229–235. doi: 10.4103/0975-2870.114640
Petzinger, G. M., Fisher, B. E., McEwen, S., Beeler, J. A., Walsh, J. P., and Jakowec, M. W. (2013). Exercise-enhanced neuroplasticity targeting motor and cognitive circuitry in Parkinson's disease. Lancet Neurol. 12, 716–726. doi: 10.1016/S1474-4422(13)70123-6
Pin-Barre, C., and Laurin, J. (2015). Physical exercise as a diagnostic, rehabilitation, and preventive tool: influence on neuroplasticity and motor recovery after stroke. Neural Plast. 2015:608581. doi: 10.1155/2015/608581
Pitt, J. M., Kroemer, G., and Zitvogel, L. (2016). Extracellular vesicles: masters of intercellular communication and potential clinical interventions. J. Clin. Investig. 126, 1139–1143. doi: 10.1172/JCI87316
Pons-Espinal, M., Gasperini, C., Marzi, M. J., Braccia, C., Armirotti, A., Pötzsch, A., et al. (2019). MiR-135a-5p is critical for exercise-induced adult neurogenesis. Stem Cell Rep. 12, 1298–1312. doi: 10.1016/j.stemcr.2019.04.020
Ritchie, H., and Roser, M. (2018). Mental Health. OurWorldInData.org.
Rosenbaum, S., Vancampfort, D., Steel, Z., Newby, J., Ward, P. B., and Stubbs, B. (2015). Physical activity in the treatment of Post-traumatic stress disorder: a systematic review and meta-analysis. Psychiatry Res. 230, 130–136. doi: 10.1016/j.psychres.2015.10.017
Sabatini, M. J., Ebert, P., Lewis, D. A., Levitt, P., Cameron, J. L., and Mirnics, K. (2007). Amygdala gene expression correlates of social behavior in monkeys experiencing maternal separation. J. Neurosci. 27, 3295–3304. doi: 10.1523/JNEUROSCI.4765-06.2007
Sadler, A. M., and Bailey, S. J. (2016). Repeated daily restraint stress induces adaptive behavioural changes in both adult and juvenile mice. Physiol. Behav. 167, 313–323. doi: 10.1016/j.physbeh.2016.09.014
Safdar, A., Saleem, A., and Tarnopolsky, M. A. (2016). The potential of endurance exercise-derived exosomes to treat metabolic diseases. Nat. Rev. Endocrinol. 12, 504–517. doi: 10.1038/nrendo.2016.76
Safdar, A., and Tarnopolsky, M. A. (2018). Exosomes as mediators of the systemic adaptations to endurance exercise. Cold Spring Harb. Perspect. Med. 8:a029827. doi: 10.1101/cshperspect.a029827
Sarma, S. I., and Byrne, G. J. (2014). Relationship between anxiety and quality of life in older mental health patients. Austr. J. Age. 33, 201–204. doi: 10.1111/ajag.12102
Schmidt, M. E., Andrews, R. D., van der Ark, P., Brown, T., Mannaert, E., Steckler, T., et al. (2009). Dose-dependent effects of the CRF1 receptor antagonist R317573 on regional brain activity in healthy male subjects. Psychopharmacology 208:109. doi: 10.1007/s00213-009-1714-1
Serulle, Y., Zhang, S., Ninan, I., Puzzo, D., McCarthy, M., Khatri, L., et al. (2007). A GluR1-cGKII interaction regulates AMPA receptor trafficking. Neuron 56, 670–688. doi: 10.1016/j.neuron.2007.09.016
Shin, J., Gireesh, G., Kim, S. W., Kim, D. S., Lee, S., Kim, Y. S., et al. (2009). Phospholipase C beta 4 in the medial septum controls cholinergic theta oscillations and anxiety behaviors. J. Neurosci. 29, 15375–15385. doi: 10.1523/JNEUROSCI.3126-09.2009
Shoji, H., and Miyakawa, T. (2020). Differential effects of stress exposure via two types of restraint apparatuses on behavior and plasma corticosterone level in inbred male BALB/cAJcl mice. Neuropsychopharmacol. Rep. 40, 73–84. doi: 10.1002/npr2.12093
Soo, C. Y., Song, Y., Zheng, Y., Campbell, E. C., Riches, A. C., Gunn-Moore, F., et al. (2012). Nanoparticle tracking analysis monitors microvesicle and exosome secretion from immune cells. Immunology 136, 192–197. doi: 10.1111/j.1365-2567.2012.03569.x
Steine, I. M., Zayats, T., Stansberg, C., Pallesen, S., Mrdalj, J., Håvik, B., et al. (2016). Implication of NOTCH1 gene in susceptibility to anxiety and depression among sexual abuse victims. Transl. Psychiatry 6:e977. doi: 10.1038/tp.2016.248
Stonerock, G. L., Hoffman, B. M., Smith, P. J., and Blumenthal, J. A. (2015). Exercise as treatment for anxiety: systematic review and analysis. Ann. Behav. Med. 49, 542–556. doi: 10.1007/s12160-014-9685-9
Tanner, C. B., Madsen, S. R., Hallowell, D. M., Goring, D. M. J., Moore, T. M., Hardman, S. E., et al. (2013). Mitochondrial and performance adaptations to exercise training in mice lacking skeletal muscle LKB1. Am. J. Physiol. Endocrinol. Metab. 305, E1018–E1029. doi: 10.1152/ajpendo.00227.2013
Walker, E. R., McGee, R. E., and Druss, B. G. (2015). Mortality in mental disorders and global disease burden implications: a systematic review and meta-analysis. JAMA Psychiatry 72, 334–341. doi: 10.1001/jamapsychiatry.2014.2502
Wang, D., Levine, J. L. S., Avila-Quintero, V., Bloch, M., and Kaffman, A. (2020). Systematic review and meta-analysis: effects of maternal separation on anxiety-like behavior in rodents. Transl. Psychiatry 10:174. doi: 10.1038/s41398-020-0856-0
Wang, H., Wang, B., Zhang, A., Hassounah, F., Seow, Y., Wood, M., et al. (2019). Exosome-mediated miR-29 transfer reduces muscle atrophy and kidney fibrosis in mice. Mol. Ther. 27, 571–583. doi: 10.1016/j.ymthe.2019.01.008
Wegner, M., Helmich, I., Machado, S., Nardi, A. E., Arias-Carrion, O., and Budde, H. (2014). Effects of exercise on anxiety and depression disorders: review of meta- analyses and neurobiological mechanisms. CNS Neurol. Disord. Drug Target. 13, 1002–1014. doi: 10.2174/1871527313666140612102841
Wei, Y. B., Liu, J. J., Villaescusa, J. C., Åberg, E., Brené, S., Wegener, G., et al. (2016). Elevation of Il6 is associated with disturbed let-7 biogenesis in a genetic model of depression. Transl. Psychiatry 6:e869. doi: 10.1038/tp.2016.136
Werner, C., Raivich, G., Cowen, M., Strekalova, T., Sillaber, I., Buters, J. T., et al. (2004). Importance of NO/cGMP signalling via cGMP-dependent protein kinase II for controlling emotionality and neurobehavioural effects of alcohol. Eur. J. Neurosci. 20, 3498–3506. doi: 10.1111/j.1460-9568.2004.03793.x
Whitham, M., Parker, B. L., Friedrichsen, M., Hingst, J. R., Hjorth, M., Hughes, W. E., et al. (2018). Extracellular vesicles provide a means for tissue crosstalk during exercise. Cell Metabol. 27, 237–251.e4. doi: 10.1016/j.cmet.2017.12.001
Widmann, M., Nieß, A. M., and Munz, B. (2019). Physical exercise and epigenetic modifications in skeletal muscle. Sports Med. 49, 509–523. doi: 10.1007/s40279-019-01070-4
Wittchen, H.-U. (2002). Generalized anxiety disorder: prevalence, burden, and cost to society. Depr. Anxiety 16, 162–171. doi: 10.1002/da.10065
Wu, Y., Xu, J., Xu, J., Cheng, J., Jiao, D., Zhou, C., et al. (2017). Lower serum levels of miR-29c-3p and miR-19b-3p as biomarkers for Alzheimer's disease. Tohoku J. Exp. Med. 242, 129–136. doi: 10.1620/tjem.242.129
Yin, X., Zhao, Y., Zheng, Y. L., Wang, J. Z., Li, W., Lu, Q. L., et al. (2019). Time-course responses of muscle-specific MicroRNAs following acute uphill or downhill exercise in sprague-dawley rats. Front. Physiol. 10:1275. doi: 10.3389/fphys.2019.01275
Yoon, K. J., Zhang, D., Kim, S. J., Lee, M. C., and Moon, H. Y. (2019). Exercise-induced AMPK activation is involved in delay of skeletal muscle senescence. Biochem. Biophys. Res. Commun. 512, 604–610. doi: 10.1016/j.bbrc.2019.03.086
Yu, X. B., Dong, R. R., Wang, H., Lin, J. R., An, Y. Q., Du, Y., et al. (2016). Knockdown of hippocampal cysteinyl leukotriene receptor 1 prevents depressive behavior and neuroinflammation induced by chronic mild stress in mice. Psychopharmacology 233, 1739–1749. doi: 10.1007/s00213-015-4136-2
Keywords: exercise, extracellular vesicle, anxiety, miRNA, ICV injection
Citation: Yoon KJ, Park S, Kwak SH and Moon HY (2021) Effects of Voluntary Running Wheel Exercise-Induced Extracellular Vesicles on Anxiety. Front. Mol. Neurosci. 14:665800. doi: 10.3389/fnmol.2021.665800
Received: 09 February 2021; Accepted: 20 May 2021;
Published: 01 July 2021.
Edited by:
Dong-Gyu Jo, Sungkyunkwan University, South KoreaReviewed by:
Sung Hyun Kim, Kyung Hee University, South KoreaJe-Hyun Yoon, Medical University of South Carolina, United States
Minseok Song, Yeungnam University, South Korea
Copyright © 2021 Yoon, Park, Kwak and Moon. This is an open-access article distributed under the terms of the Creative Commons Attribution License (CC BY). The use, distribution or reproduction in other forums is permitted, provided the original author(s) and the copyright owner(s) are credited and that the original publication in this journal is cited, in accordance with accepted academic practice. No use, distribution or reproduction is permitted which does not comply with these terms.
*Correspondence: Hyo Youl Moon, aHltb29uODA0M0BnbWFpbC5jb20=