- 1Department of Neurosurgery, Spine and Peripheral Nerve Surgery, Medical University of Lodz, Lodz, Poland
- 2Department of Pathology, Chair of Oncology, Medical University of Lodz, Lodz, Poland
- 3Department of Molecular Pathology and Neuropathology, Medical University of Lodz, Lodz, Poland
- 4Department of Neurosurgery and Neurooncology, Medical University of Lodz, Lodz, Poland
- 5Department of Normal and Clinical Anatomy, Medical University of Lodz, Lodz, Poland
- 6Department of Sleep Medicine and Metabolic Disorders, Medical University of Lodz, Lodz, Poland
- 7Department of Neurosurgery and Ochsner Neuroscience Institute, Ochsner Health System, New Orleans, LA, United States
- 8Department of Neurosurgery, Tulane University School of Medicine, New Orleans, LA, United States
- 9Department of Neurology, Tulane University School of Medicine, New Orleans, LA, United States
- 10Department of Structural and Cellular Biology, Tulane University School of Medicine, New Orleans, LA, United States
- 11Department of Surgery, Tulane University School of Medicine, New Orleans, LA, United States
- 12Department of Anatomical Sciences, St. George's University, St. George's, Grenada
- 13University of Queensland, Brisbane, QLD, Australia
Neurovascular compression syndromes (NVC) are challenging disorders resulting from the compression of cranial nerves at the root entry/exit zone. Clinically, we can distinguish the following NVC conditions: trigeminal neuralgia, hemifacial spasm, and glossopharyngeal neuralgia. Also, rare cases of geniculate neuralgia and superior laryngeal neuralgia are reported. Other syndromes, e.g., disabling positional vertigo, arterial hypertension in the course of NVC at the CN IX-X REZ and torticollis, have insufficient clinical evidence for microvascular decompression. The exact pathomechanism leading to characteristic NVC-related symptoms remains unclear. Proposed etiologies have limited explanatory scope. Therefore, we have examined the underlying pathomechanisms stated in the medical literature. To achieve our goal, we systematically reviewed original English language papers available in Pubmed and Web of Science databases before 2 October 2021. We obtained 1694 papers after eliminating duplicates. Only 357 original papers potentially pertaining to the pathogenesis of NVC were enrolled in full-text assessment for eligibility. Of these, 63 were included in the final analysis. The systematic review suggests that the anatomical and/or hemodynamical changes described are insufficient to account for NVC-related symptoms by themselves. They must coexist with additional changes such as factors associated with the affected nerve (e.g., demyelination, REZ modeling, vasculature pathology), nucleus hyperexcitability, white and/or gray matter changes in the brain, or disturbances in ion channels. Moreover, the effects of inflammatory background, altered proteome, and biochemical parameters on symptomatic NVC cannot be ignored. Further studies are needed to gain better insight into NVC pathophysiology.
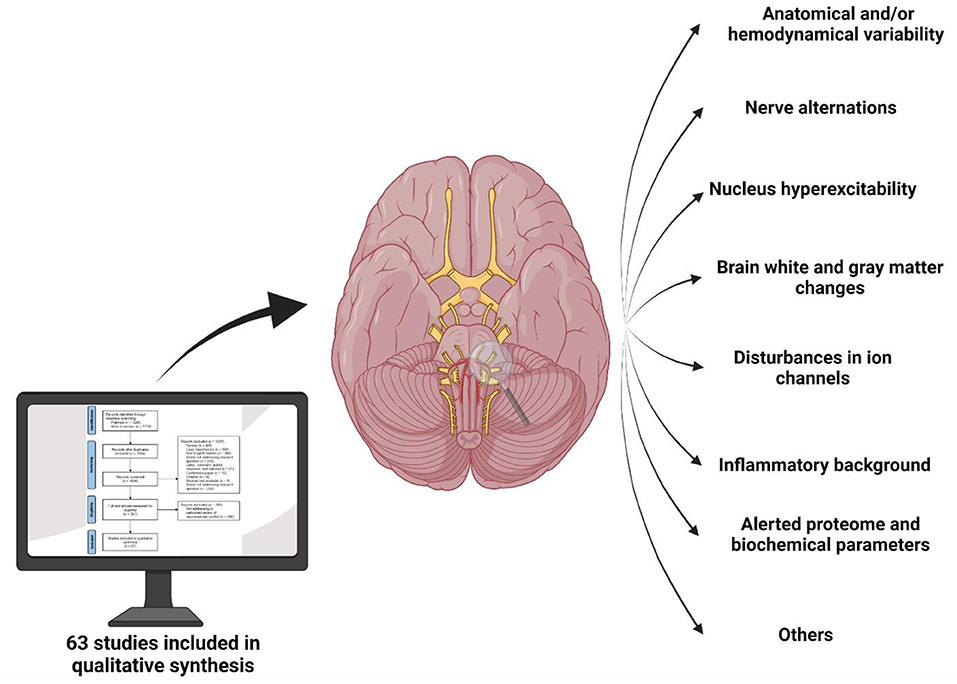
Graphical Abstract. So far, proposed etiologies of neurovascular compression syndromes have limited explanatory scope. Therefore, we have examined the underlying pathomechanisms stated in the medical literature. The systematic review suggests that the anatomical and/or hemodynamical changes described are insufficient to account for NVC-related symptoms by themselves. They must coexist with additional factors such as affected nerves (e.g., demyelination, REZ modeling, vasculature pathology), nucleus hyperexcitability, white and/or gray matter changes in the brain, or disturbances in ion channels. Moreover, the effects of inflammatory background, altered proteome, and biochemical parameters on symptomatic neurovascular compression syndromes cannot be ignored in the future.
Introduction
Neurovascular compression syndromes (NVC) are challenging disorders resulting from the compression of cranial nerves at the root entry/exit zone (REZ) also known as Redlich–Obersteiner's zone. Although the REZ is equated with the transition zone (TZ) between the central myelin produced by oligodendroglia and the peripheral myelin comprising Schwann cells, some publications have revealed differences between the two. Peker et al., who tested 100 trigeminal nerves from 50 cadavers, showed that on average the TZ is 3 mm from the REZ into the pons (Peker et al., 2006). This observation has a significant clinical consequence. During the surgery, it is important to visualize the compression of an appropriate vessel on the nerve in the TZ, e.g., in the situation in which TZ is retracted toward the pons, the neurosurgeon should look for conflict between vessel and pons rather than nerve.
Clinically, we can distinguish the following major NVC conditions: trigeminal neuralgia (TN), hemifacial spasm (HFS), and glossopharyngeal neuralgia. Also, rare cases of geniculate neuralgia and superior laryngeal neuralgia have been described (Greenberg, 2020). Disabling positional vertigo, arterial hypertension in the course of NVC at the CN IX-X REZ, and torticollis have insufficient clinical evidence for microvascular decompression (MVD; see Table 1). Nerve compression by a vessel is insufficient to cause NVC-related symptoms by itself. The pathomechanism leading to characteristic NVC-related symptoms remains unclear. Proposed etiologies have limited explanatory scope. Therefore, we have examined the underlying pathomechanisms described in the medical literature and subjected them to a systematic review.
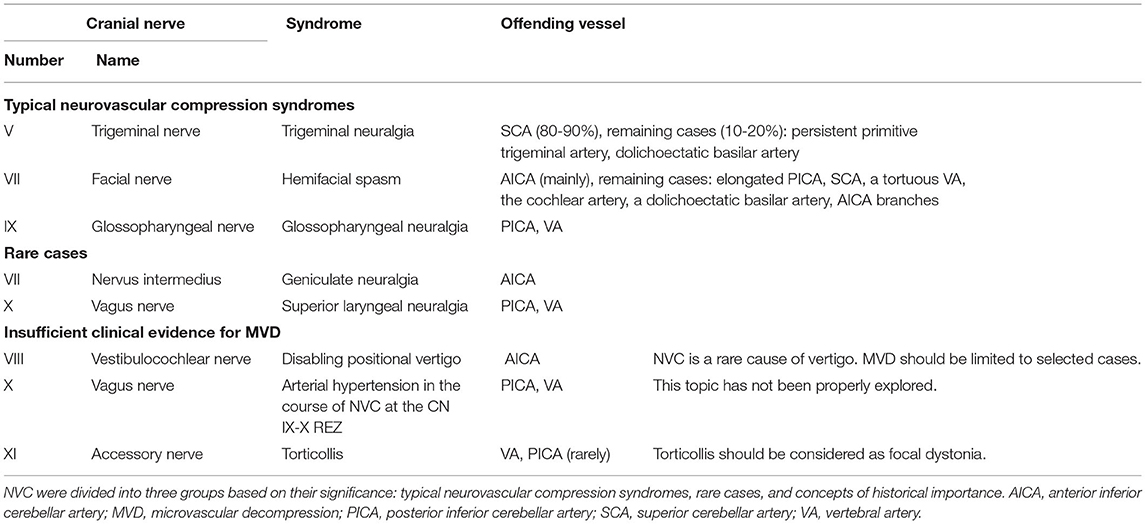
Table 1. Typical offending vessel depending on specific neurovascular compression syndromes (NVC) in accordance with Greenberg (2020).
Materials and Methods
Two of us (BSz and JS) screened all the relevant original English language papers published in the Pubmed and Web of Science databases before 2 October 2021. We decided to include all available subtypes of original papers to maximize the coverage of possible pathomechanisms of NVC. The exact queries are given in the Supplementary Materials. We obtained 1694 articles after eliminating duplicates. These records were double screened. In the case of any discrepancies between the two authors extracting data, the final decision was made by the senior author (MR). Only 357 original papers potentially pertaining to the pathogenesis of NVC were enrolled in the full-text assessment for eligibility (see Figure 1), and 63 of these were included in the final analysis.
The Influence of Anatomical and Hemodynamical Changes on the Course of NVC
Anatomical Variability
Selected anatomical variabilities such as a duplicate posterior inferior cerebellar artery, a short basilar artery, and an aberrant arterial course can affect the course of a NVC (Karki et al., 2019). Among other known factors, we can distinguish narrowness of the posterior fossa, a small petrous angle, and a sharp nerve-pontine angle.
Posterior fossa narrowness is an important example of the anatomical variabilities predisposing to NVC. This topic was explored by Kamiguchi et al., who measured the petrous angle and pons diameter index in 34 HFS patients. They observed a significantly smaller petrous angle combined with a significantly greater pons diameter index in the HFS patients than the control group. This anatomical variability led to more crowding of cranial nerves and vascular structures, which could contribute to symptomatic NVC (Kamiguchi et al., 1997).
Zhu et al. suggested that a small cross-sectional area of the cerebellopontine angle (CPA) could promote HFS pathogenesis. This hypothesis was corroborated by preoperative findings. Interestingly, these measurements can be considered predictors of the microvascular decompression (MVD) effect: they were significantly lower in the recurrent group than in the non-recurrent group (Zhu et al., 2020). This hypothesis is consistent with available literature (Dou et al., 2016; Cheng et al., 2017). Moreover, a crowded CPA space and a tendency toward attrition of the neurovascular components are considered likely to promote the occurrence of bilateral HFS (Dou et al., 2016).
Not only a small CPA but also a sharp nerve-pontine angle can lead to the NVC and also to the exacerbation of nerve degeneration (Pang et al., 2019; Zhu et al., 2020). From the clinical point of view, these anatomical factors are insufficient to cause NVC-related symptoms by themselves. As there are observed among many patients without NVC-related symptoms.
Hemodynamical Factors
Lorenzoni et al. observed that the following factors increased the risk of TN: compression of the superior cerebellar, anterior inferior cerebellar, basilar or vertebral arteries, and of venous structures. Moreover, they observed nerve dislocation and/or distortion by the vessel in 32% of cases (Lorenzoni et al., 2012).
Anatomical variations and hemodynamical changes in the vertebrobasilar arterial system are important promoters of vascular compression among HFS patients (Kim et al., 2012; Wang et al., 2019a). The risk of HFS is greater among individuals with a dominant vertebral artery and characteristic direction of the vertebrobasilar junction (Park et al., 2015). Further, lateral deviation of the vertebral artery is significantly more frequent among HFS patients, with a relative risk of 8.44 (Guan et al., 2011).
Kim et al. evaluated the effects of selected parameters of the vertebral artery (severity of compression, indentation, and color change) on surgical treatment outcomes among HFS patients (see Figure 2). They revealed that patients with severe compression have slightly poorer outcomes than those with mild or moderate compression. Moreover, they concluded that CNVII color changes could be related to poor outcomes after MVD (Kim et al., 2012). Other important parameters affecting HFS patients are the angulation and tortuosity of vessels. Computational fluid dynamics models have confirmed that these lead to significant pressure differences between the vascular walls on opposite sides (Wang et al., 2019a).
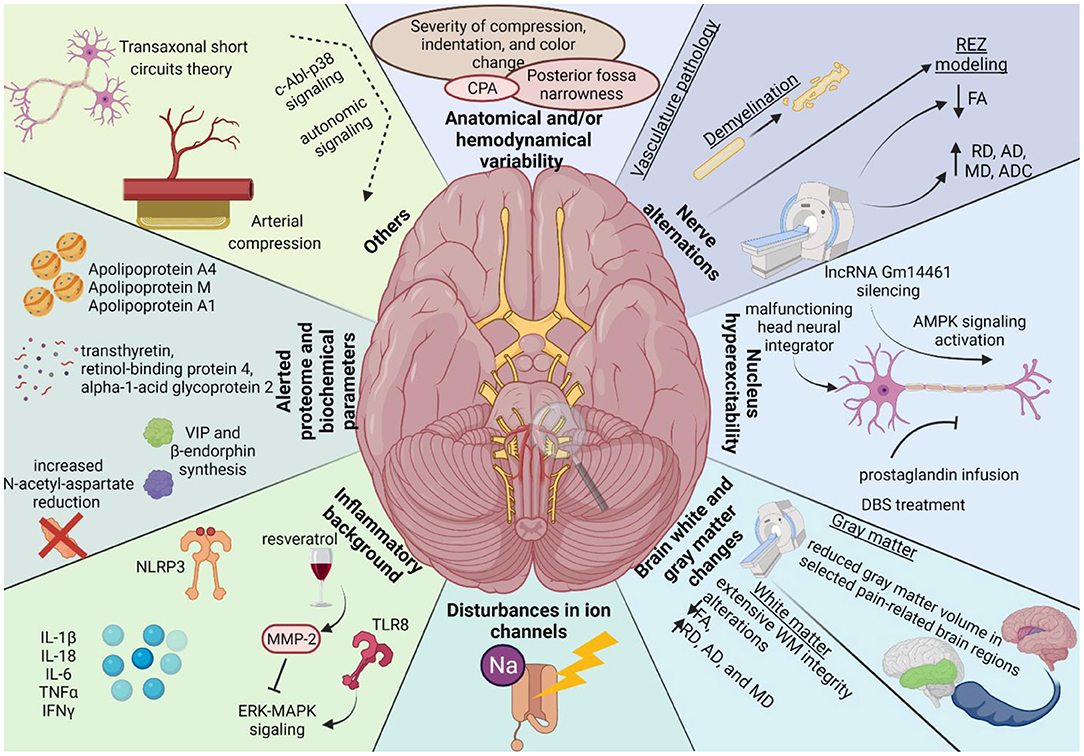
Figure 2. The graphical summary of the underlying pathogenesis of neurovascular compression syndromes based on performed systematic review. Legend as follow: AD, axial diffusivity; ADC, apparent diffusion coefficient; AMPK, adenosine monophosphate-activated protein kinase; CPA, cerebellopontine angle; FA, fractional anisotropy; MD, mean diffusivity; MMP-2, metalloproteinase-2; NLRP3, nucleotide-binding domain (NOD)-like receptor protein 3; lncRNA, Long non-coding RNA; RD, radial diffusivity; REZ, root entry/exit zone; WM, white matter.
Another hemodynamic parameter affecting HFS patients is higher pressure at the REZ. Zhang et al. found strong positive correlations between measured intraoperative pressure readings in NVC and (a) preoperative spasm severity according to Cohen scores and (b) the time to complete recovery after MVD (Zhang et al., 2019).
Other Hemodynamical Factors Predisposing to NVC
Interestingly, TN can be caused by arterialization of the superior petrosal vein in the context of a dural or cerebral arteriovenous shunt (Robert et al., 2015). Robert et al. observed this arterialization in 10 (100%) patients with TN, while superior petrosal vein ectasia explained the compression of the CNV in two (20%). This could suggest that venous reflux rather than nerve compression is the primary mechanism of TN in this group of patients (Robert et al., 2015).
Factors Associated With the Changes in the Nerve Structure
Selected factors associated with the affected nerve (e.g., length and volume of its central myelin portion) correlated strongly with the incidence of symptomatic NVC (Guclu et al., 2011). In this section, we collected NVC-related changes in the affected nerve: vasculature pathology, demyelination, REZ modeling, and alerted nerve structure revealed by MRI/diffusion tensor imaging (see Figure 2).
Vasculature Pathology
Marinkovic et al. considered possible pathological vasculature among TN patients (n = 8; two with confirmed vascular compression). They examined biopsy specimens obtained during partial rhizotomy by electron microscopy and immunohistochemistry and found vascular pathological alterations in three of the patients, including the two with confirmed vascular compression. Electron microscopy revealed signs of apoptosis and/or degeneration of endothelial and smooth muscle cells in the trigeminal arteriole wall. Immunohistochemical tests gave stronger than normal reactions against factor VIII, laminin, fibronectin, and collagen IV, but weaker against CD31, CD34, and α-smooth muscle actin, among TN patients (Marinković et al., 2007).
Demyelination
A study of a rat model of HFS by Kuroki and Møller showed that both CNVII demyelination and vascular compression are needed to induce facial hyperactivity. In other words, they confirmed that close contact between a peripheral branch of the facial nerve and an artery facilitates the development of abnormal muscle response, but only if the facial nerve had previously been slightly injured at the point of the arterial contact. They also revealed that blocking neural conduction in the facial nerve proximal to the artificial vascular compression point abolishes the abnormal muscle contraction. This observation could suggest that the anatomical location of cross-transmission is central to vascular compression (Kuroki and Møller, 1994).
The demyelination component of CNV can be estimated using diffusion tensor MRI radial diffusivity (RD). This approach was employed by Willsey et al. to predict the recurrence of TN following MVD. They noted that normalized RD correlates negatively with the duration of symptoms, possibly a useful predictor of pain-free remission (Willsey et al., 2021). However, this should be juxtaposed with histopathological examination. Such evidence was presented by Marinković et al., in the form of detailed ultrastructural and immunohistochemical examinations of trigeminal axons surrounded by the peripheral type of myelin. They confirmed not only central zone myelin changes (e.g., deformation, thickening, demyelination, and remyelination), but also peripheral myelin alterations visible by electron microscopy (e.g., atrophy or hypertrophy, increased neurofilaments, loss of the myelin and occasional sprouting) (Marinković et al., 2009). Similar findings were described by Devor et al., who examined trigeminal root specimens obtained during MVD (n = 12) ultrastructurally. They observed the following pathological changes: demyelination (n = 6), a range of less severe myelin abnormalities (dysmyelination: n = 11), and the presence of excess collagen (n = 8). They also noted axonopathy and axonal loss and residual myelin debris (Devor et al., 2002).
REZ Modeling
Luo et al. investigated glial plasticity in the REZ of a rat TN model induced by compression injury. Their results revealed that mechanical compression injury leads to the activation of various cells (especially oligodendrocytes, astrocytes, Schwann cells, and macrophages) in the TZ (Luo et al., 2019). These alterations in glial cell plasticity could result in TN.
Similar observations were made by Lin et al., who also examined rat models: a TN group with CNV root compression, a sham operation group, a group with TN plus selective histone deacetylase inhibitor injection into the REZ, and a group with TN plus vehicle injection into the REZ. Their results also suggested that chronic nerve root compression could lead to TN via alerted glial plasticity and the level of histone acetylation in the REZ (Lin et al., 2019).
Alerted Nerve Structure
NVC-linked structural alterations are not always apparent on conventional imaging (DeSouza et al., 2014). Therefore, we focus here on the description of NVC-induced lesions, which can easily be observed in magnetic resonance (MRI) and diffusion tensor (DTI) imaging.
Magnetic Resonance Imaging Findings
High-resolution MRI can be used to explore nerve microstructure among patients with NVC. Using this approach, Wang et al. observed a lower CNV volume among TN patients than controls (Wang et al., 2019b). This was confirmed by Cheng et al. in a prospective case-control study of 60 consecutive patients diagnosed with TN compared with 30 sex- and age-matched healthy controls. They observed a significantly lower mean volume of the affected CNV than in either the non-affected side or the controls (Cheng et al., 2017).
Diffusion Tensor Imaging Findings
DeSouza et al. used DTI to measure fractional anisotropy (FA) as well as identify abnormalities of mean (MD), radial (RD), and axial (AD) diffusivity from the REZ of CNV. They observed the following nerve alternations among TN patients: lower FA and higher RD, AD, and MD than in the control group. The MD and RD alterations could indicate that neuroinflammation and/or edema is an important element in TN pathophysiology (DeSouza et al., 2014).
Even more interesting observations were made by Leal et al., who employed DTI for a pre- and post-operative comparative study 4 years after MVD. The preoperative observations were similar to those of DeSouza et al.: significantly lower FA on the affected side than on the unaffected side or in controls. Affected nerves had smaller cross-sectional areas and volumes than unaffected nerves or the controls. Moreover, the apparent diffusion coefficient (ADC) on the affected side was significantly lower than that on the unaffected side or in the control group (Leal et al., 2019). These observations accord with the study by Pang et al. (2019). The postoperative findings showed that MVD did not change the differences in FA, but the ADC normalized in the affected nerves after MVD (Leal et al., 2019).
Other Findings
Another parameter related to the affected nerve structure is an indentation defined as a furrowed hole on the facial nerve. Ko et al. estimated that 47% of HFS patients had an indented CNVII. Moreover, those patients required a longer recovery time after MVD than HFS patients without CNVII indentation (Ko et al., 2021).
Nucleus Hyperexcitability
Several recent studies suggest that nucleus hyperexcitability is an important component in the pathogenesis of NVC-related symptoms. The relative involvements of the vascular component and hyperexcitability differed among patients, indicating that something other than a vascular component is involved in HSF pathology (Hirono et al., 2014). HFS was associated with ectopic excitation and ephaptic transmission of the facial nerve (Nielsen, 1984; Møller and Jannetta, 1986). By measuring facial motor evoked potentials during the MVD procedure, Wilkinson et al. showed that the suppressive effects of desflurane were less pronounced on the spasm side than on the non-spasm side, supporting a mechanism of central pathophysiology for HFS (Wilkinson et al., 2017). Ishikawa et al. measured F waves to investigate antidromically-activated neurons of the facial motor nucleus before and after the MVD procedure. They showed that in some patients, despite a successful MVD procedure, the increased F waves and abnormal muscle response persisted for several months and the muscle response normalized only after the F waves decreased (Ishikawa et al., 1996, 1997). A study by Teresaka et al. corroborated this observation. They indicated a correlation between preoperative anticonvulsant therapy and delayed cure after MVD, indicating an important role for hyperexcitation of the facial nucleus in the pathogenesis of HFS (Terasaka et al., 2016). This observation suggested that patients who did not respond to the MVD procedure should not be re-operated until the hyperexcitability of the facial motor nucleus has disappeared. The aforementioned neurophysiological observations are consistent with the known clinical observation (HFS patients after MVD present minor facial spasms for a few days after surgery with slow alleviation), which they explain perfectly (Illingworth et al., 1996).
A few pathomechanisms have been proposed to explain the role of disturbed neural activation in NVC-related symptoms. Cai et al. described a mouse model in which silencing of lncRNA Gm14461 led to activation of the AMPK and Akt/mTOR signaling pathways, enhancing autophagy and decreasing astrocyte activation (Cai et al., 2020). In another study, non-invasive CNX stimulation caused by prostaglandin infusion led to a rise in glutamate and decreased trigeminal nociceptive stimulation in allodynic rats (Oshinsky et al., 2014). Interestingly, in patients with obsessive-compulsive spectrum symptoms, where there are abnormalities in the stratio-thalamocortical circuits, focal dystonia and HFS were highly represented (Mula et al., 2012).
This phenomenon is somehow reminiscent of a restricted epileptic activity which might explain why, in the majority of cases, TN as well as the other NVC neuralgias well respond to an antiepileptic drug, namely to carbamazepine or gabapentin (see Figure 3). However, one must be aware that these medications are not helpful in HFS and other non-neuralgic NVCs.
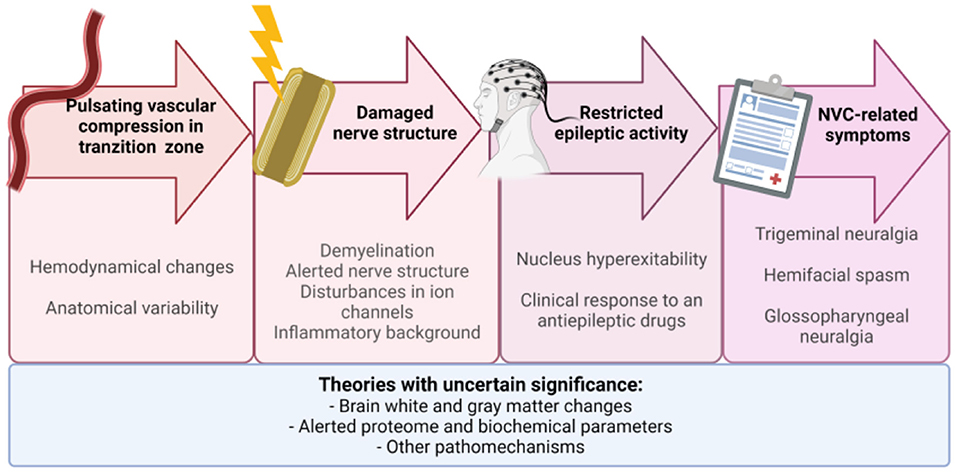
Figure 3. The proposed chain of events leading to neurovascular compression-related symptoms: vascular compression in transition zone → demyelination → nucleus hyperexcitability → symptoms. Not all factors mentioned in Figure 2 are presented here. Future research is needed to understand their role. NVC, neurovascular compression.
Brain White and Gray Matter Changes
Using high-resolution MRI, Wang et al. observed reduced gray matter volume in selected pain-related brain regions (e.g., insula, secondary somatosensory cortex, hippocampus, dorsal anterior cingulate cortex, precuneus, and several areas of the temporal lobe) (Wang et al., 2019b). Wu et al. compared morphological images of TN patients' brains with and without NVC to those of healthy controls. They employed voxel-based and surface-based morphometry to analyze whole-brain gray matter quantitatively. They observed significantly lower gray matter volume and cortical thickness in TN with NVC than in TN without NVC (Wu et al., 2020).
Moreover, they observed extensive alterations in white matter integrity (Wu et al., 2020). DeSouza et al., using diffusion tensor imaging data, also found abnormalities in brain white matter: lower FA, and higher RD, AD, and MD. These changes were especially visible in the corpus callosum, cingulum, posterior corona radiata, and superior longitudinal fasciculus (DeSouza et al., 2014).
Disturbances in ION Channels
Many studies have highlighted the effect of disturbances in ion channels on neuropathic pain. This resembles the disturbances observed among NVC patients (Charlesworth et al., 2012; Wei et al., 2013; Choi et al., 2016; Li et al., 2021; Romero et al., 2021). Interestingly, the relationships between disturbances in ion channels and NVC have been explored in a few papers. Xia et al. observed overexpression of Nav1.8 in injured peripheral nerves. These molecular abnormalities were associated with the generation of HFS (Xia et al., 2014). Liu et al. used a rat infraorbital nerve chronic constriction injury model to test the character of TN as an ectopic impulse induced by a sodium channel modulated by cytokines. Nav1.3 was upregulated in the compressed CNV (Liu et al., 2020). Finally, functional testing of a mutation observed among NVC patients, TRPM8 (NM_024080.5):c.89G>A (p.Arg30Gln), revealed that it enhances channel activation and increases the basal current amplitude and intracellular calcium concentration, which could lead to TN (Gualdani et al., 2021). In the context of the hypothesis made in chapter 5, the disturbances in ion channels may be considered a functional variant of “damaged nerve” in the proposed chain of events leading to NVC symptoms.
Inflammatory Background of Symptomatic NVC
Inflammatory cytokines induced by demyelination are known to cause neuropathic pain (Liu et al., 2019). Moreover, sticky inflammatory arachnoid membranes in the area of the NVC were a common finding during MVD (Chen et al., 2019a; Mazzucchi et al., 2019). Those observations suggested that an inflammatory response could be relevant to NVC-related syndromes.
An animal model revealed that the expression of a nucleotide-binding oligomerization domain containing a leucine-rich repeat and pyrin domain (NLRP), a type of NOD-like receptor belonging to innate immunity proteins, effectively modulates TN (Ren et al., 2021). Moreover, Chen et al. showed that injection of complete Freund's adjuvant to induce trigeminal pain leads to increased expression of the mRNAs for NLRP3, interleukin (IL)-1b, and IL-18. Further, NLRP3, IL-1b, and IL-18 expression was significantly inhibited by microRNA-186, which alleviated symptoms of neuralgia in CFA-treated mice (Chen et al., 2018). Accordingly, Liu et al. showed that upregulation of Nav 1.3 in TN seems to occur via an increase of IL-6 following constriction injury. Zhao et al. showed that expression of Toll-like receptor 8 (TLR8) is increased in trigeminal neurons in a mouse model of trigeminal neuropathic pain. In addition, deletion or knockdown of Tlr8 reduced the activation of ERK and p38-MAPK, decreased the expression of proinflammatory cytokines, and alleviated the neuralgia (Zhao et al., 2021). Liu et al. indicated that the purinoceptor P2X4, an essential receptor for preventing allodynia, could be involved in migraine chronicity via brain-derived neurotrophic factor-tyrosine receptor kinase B signaling following repeated inflammatory stimulation. Interestingly, resveratrol, a natural component of red wine, inhibited chronic constriction injury-induced allodynia via activation of matrix metalloproteases-9/2 and reduction of MAP kinase phosphorylation (Yang et al., 2016; Yin et al., 2019).
These observations were corroborated by studies on patients. Serum levels of IL-1β, IL-6, IL-8, TNF-α, white blood cell count, and neutrophil count in patients with HFS or TN were significantly higher than controls (Liu et al., 2018; Chen et al., 2019a). Ericson et al. used a proximity extension assay to analyze the levels of 92 inflammation-related protein biomarkers in cerebrospinal fluid from patients before and after microvascular decompression. They showed that surgery leads to a significant decrease of the immunological proteins to a level comparable with healthy controls (Ericson et al., 2019). Moreover, a study by Goebel et al. revealed differences in concentration of interferon-gamma between sample locations on the trigeminal nerve, highest in the distal zone (Goebel et al., 2018).
Alerted Proteome and Biochemical Parameters
Recent studies have shown that the concentrations of several proteins in serum and cerebrospinal fluid of patients with NVC-related symptoms are distinctively different from healthy controls. Expressions of transthyretin, retinol-binding protein 4, and alpha-1-acid glycoprotein 2 among TN patients were higher than in the control group (Farajzadeh et al., 2018). Federico et al. showed by single-voxel proton magnetic resonance spectroscopy that patients with idiopathic spasmodic torticollis have a greater reduction of N-acetyl-aspartate in the basal ganglia, suggesting neuronal impairment in this region (Federico et al., 2001). The concentrations of high-density lipoproteins such as apolipoproteins A4, M, and A1 were increased in the cerebrospinal fluid of TN patients (Hamdeh et al., 2020). Moreover, Chen et al. showed that treatment with botulinum toxin type A inhibited the synthesis of vasoactive intestinal peptide and increased the synthesis of beta-endorphin, indicating a role for these proteins in the pathogenesis of TN (Chen et al., 2019b).
Other Pathomechanisms
Besides the abovementioned studies related to the pathomechanism of NVC, a few studies have indicated that this pathology could have a different nature. According to the transaxonal short circuits hypothesis, squeezing nerve fibers together leads to the destruction of myelin sheaths and results in a transaxonal spread of impulses between neighboring neurons (Hankinson and Wilson, 1976; Kim and Fukushima, 1984; Kameyama et al., 2016). Accordingly, Truini et al. observed an association between demyelinating plaque, neurovascular compression, and TN in patients with multiple sclerosis (Truini et al., 2016). Nevertheless, it should be highlighted that MVD is considered as ineffective among MS patients presenting symptoms from NVC spectrum. Another mechanism was proposed in a study by Chen et al., who suggested that trigeminal nerve compression has an arterial rather than a venous origin (Chen et al., 2014). Another study indicated that c-Abl-p38alpha signaling mediates dopamine neuron loss and further TN (Fu et al., 2020). On the other hand, Zhou et al. proposed a role for disturbed signaling of the autonomic nervous system in the HFS. They showed that neurotransmitters released from autonomic nerves can induce action potentials in demyelinated nerves and could trigger HFS (Zhou et al., 2012). These insights should be reconsidered in the context of further research to avoid bias in their interpretation.
Conclusions
Neurovascular compression syndromes remain challenging disorders for neurosurgeons, clinical neurologists, and scientists. Their underlying pathogenesis is not clear. There are many partial explanations. Specific anatomical and/or hemodynamical changes alone seem insufficient to account for NVC-related symptoms. Such anatomical variability must coexist with additional changes such as factors associated with affected nerve (e.g., demyelination, REZ modeling), nucleus hyperexcitability, brain white and/or gray matter changes, or disturbances in ion channels. Moreover, the effects of inflammatory background alerted proteome, and biochemical parameters on symptomatic NVC cannot be ignored. Further studies are needed to gain better insight into NVC pathophysiology.
Practice Points
• Clinically, we can distinguish the following neurovascular compression (NVC) conditions: trigeminal neuralgia, hemifacial spasm, and glossopharyngeal neuralgia. Also, rare cases of geniculate neuralgia and superior laryngeal neuralgia are reported. Other syndromes, e.g., disabling positional vertigo, hypertension in the course of NVC at the CN IX-X root entry/exit zone, and torticollis, have insufficient clinical evidence for microvascular decompression.
• NVC syndromes remain challenging disorders. The exact pathomechanism leading to characteristic NVC-related symptoms remains unclear. The most likely chain of events leading to NVC symptoms is vascular compression in transition zone → demyelination → nucleus hyperexcitability → symptoms.
• The root entry/exit zone should not be equated with the transition zone (TZ) between the central and peripheral myelin. This observation has a significant clinical consequence. During the surgery, it is important to visualize the compression of an appropriate vessel on the nerve in the TZ. When the TZ is retracted toward the pons, the neurosurgeon should look for conflict between vessel and pons rather than nerve.
Data Availability Statement
The original contributions presented in the study are included in the article/Supplementary Material, further inquiries can be directed to the corresponding author/s.
Author Contributions
Conceptualization: BS, MR, and DJJ. Literature search and manuscript preparation: BS and JS. Revision of the manuscript: MB, JJ, PPL, DJJ, GW, FKK, AG, MS, RST, and MR. All authors read and approved the final manuscript.
Conflict of Interest
The authors declare that the research was conducted in the absence of any commercial or financial relationships that could be construed as a potential conflict of interest.
Publisher's Note
All claims expressed in this article are solely those of the authors and do not necessarily represent those of their affiliated organizations, or those of the publisher, the editors and the reviewers. Any product that may be evaluated in this article, or claim that may be made by its manufacturer, is not guaranteed or endorsed by the publisher.
Acknowledgments
Graphical Abstract, Figure 2, and Figure 3 were created with BioRender.com.
Supplementary Material
The Supplementary Material for this article can be found online at: https://www.frontiersin.org/articles/10.3389/fnmol.2022.923089/full#supplementary-material
Abbreviations
AD, axial diffusivity; ADC, apparent diffusion coefficient; CPA, cerebellopontine angle; DBS, deep brain stimulation; FA, fractional anisotropy; HFS, hemifacial spasm; IL, interleukin; MD, mean diffusivity; MVD, microvascular decompression; NLRP, Nucleotide-binding oligomerization domain, Leucine-rich Repeat and Pyrin domain-containing; NVC, Neurovascular compression syndromes; RD, radial diffusivity; REZ, root entry/exit zone (also known as Obersteiner-Redlich zone); TLR8, Toll-like receptor8; TN, trigeminal neuralgia; TZ, transition zone; WM, white matter.
References
Cai, J., Yan, Y., Zhang, D., Zhu, M., Wang, Z., and Zhang, X. (2020). Silencing of lncRNA Gm14461 alleviates pain in trigeminal neuralgia through inhibiting astrocyte activation. IUBMB Life. 72, 2663–2671. doi: 10.1002/iub.2395
Charlesworth, G., Plagnol, V., Holmström, K. M., Bras, J., Sheerin, U-. M., and Preza, E. (2012). Mutations in ANO3 cause dominant craniocervical dystonia: ion channel implicated in pathogenesis. Am. J. Hum. Genet. 91, 1041–1050. doi: 10.1016/j.ajhg.2012.10.024
Chen, G., Wang, X., Wang, L., and Zheng, J. (2014). Arterial compression of nerve is the primary cause of trigeminal neuralgia. Neurol. Sci. 35, 61–66. doi: 10.1007/s10072-013-1518-2
Chen, M., Lin, K., and Lin, S. (2018). NLRP3 inflammasome signaling as an early molecular response is negatively controlled by miR-186 in CFA-induced prosopalgia mice. Braz. J. Med. Biol. Res. 51, 1–10. doi: 10.1590/1414-431x20187602
Chen, M., Yang, M., Zhou, W., and Li, S. (2019a). Preliminary study on the relationship between inflammation and hemifacial spasm. World Neurosurg. 125:e214–e220. doi: 10.1016/j.wneu.2019.01.044
Chen, Y., Wang, Y., Lian, Y., Zhang, H., and Xie, N. (2019b). Plasma concentration change of VIP, β-ep, ENK, and PGE2 in classical trigeminal neuralgia patients after BTX-a treatment. Acta Medica Mediterr. 305, 803–812. doi: 10.19193/0393-6384_2019_2_121
Cheng, J., Meng, J., Liu, W., Zhang, H., Lei, D., and Hui, X. (2017). Nerve atrophy and a small trigeminal pontine angle in primary trigeminal neuralgia: a morphometric magnetic resonance imaging study. World Neurosurg. 104, 575–580. doi: 10.1016/j.wneu.2017.05.057
Choi, S., Yu, E., Hwang, E., and Llinás, R. R. (2016). Pathophysiological implication of Ca V 3, 1. T-type Ca 2+ channels in trigeminal neuropathic pain. Proc. Natl. Acad. Sci. U. S. A. 113:2270–2275. doi: 10.1073/pnas.1600418113
DeSouza, D. D., Hodaie, M., and Davis, K. D. (2014). Abnormal trigeminal nerve microstructure and brain white matter in idiopathic trigeminal neuralgia. Pain. 155, 37–44. doi: 10.1016/j.pain.2013.08.029
Devor, M., Govrin-Lippmann, R., and Rappaport, Z. H. (2002). Mechanism of trigeminal neuralgia: an ultrastructural analysis of trigeminal root specimens obtained during microvascular decompression surgery. J. Neurosurg. 96, 532–543. doi: 10.3171/jns.2002.96.3.0532
Dou, N-. N., Zhong, J., Liu, M-. X., Xia, L., Sun, H., and Li, B. (2016). Management of bilateral hemifacial spasm with microvascular decompression. World Neurosurg. 87, 640–645. doi: 10.1016/j.wneu.2015.10.091
Ericson, H., Abu Hamdeh, S., Freyhult, E., Stiger, F., Bäckryd, E., and Svenningsson, A. (2019). Cerebrospinal fluid biomarkers of inflammation in trigeminal neuralgia patients operated with microvascular decompression. Pain. 160, 2603–2611. doi: 10.1097/j.pain.0000000000001649
Farajzadeh, A., Bathaie, S. Z., Arabkheradmand, J., Ghodsi, S. M., and Faghihzadeh, S. (2018). Different pain states of trigeminal neuralgia make significant changes in the plasma proteome and some biochemical parameters: a preliminary cohort study. J. Mol. Neurosci. 66, 524–534. doi: 10.1007/s12031-018-1183-2
Federico, F., Lucivero, V., Simone, I. L., Defazio, G., De Salvia, R, Mezzapesa, D. M., et al. (2001). Proton MR spectroscopy in idiopathic spasmodic torticollis. Neuroradiology. 43, 532–536. doi: 10.1007/s002340000532
Fu, J., Mu, G., Qiu, L., Zhao, J., and Ou, C. (2020). c-Abl-p38 α signaling pathway mediates dopamine neuron loss in trigeminal neuralgia. Mol. Pain. 16, 174480692093085. doi: 10.1177/1744806920930855
Goebel, A., Lee, M. K., Cacciola, F., Cross, A., and Eldridge, P. (2018). A technique to assess perineuronal mediators. Br. J. Neurosurg. 32, 697–699. doi: 10.1080/02688697.2017.1416058
Greenberg, M. (2020). “Functional Neurosurgery and Stereotactic Neurosurgery,” in Handbook of Neurosurgery (New York, NY; Stuttgart; New Delhi; Rio de Janeiro: Thieme), 1623–1660.
Gualdani, R., Yuan, J-. H., Effraim, P. R., Stefano, Di Truini, G., Cruccu, A. G., et al. (2021). Trigeminal Neuralgia TRPM8 Mutation. Neurol. Genet. 7, e550. doi: 10.1212/NXG.0000000000000550
Guan, H-. X., Zhu, J., and Zhong, J. (2011). Correlation between idiopathic hemifacial spasm and the MRI characteristics of the vertebral artery. J. Clin. Neurosci. 18, 528–530. doi: 10.1016/j.jocn.2010.08.015
Guclu, B., Sindou, M., Meyronet, D., Streichenberger, N., Simon, E., and Mertens, P. (2011). Cranial nerve vascular compression syndromes of the trigeminal, facial and vago-glossopharyngeal nerves: comparative anatomical study of the central myelin portion and transitional zone; correlations with incidences of corresponding hyperactive dysfunctio. Acta Neurochir. (Wien). 153, 2365–2375. doi: 10.1007/s00701-011-1168-1
Hamdeh, S. A., Khoonsari, P. E., Shevchenko, G., Gordh, T., Ericson, H., and Kultima, K. (2020). Increased CSF levels of apolipoproteins and complement factors in trigeminal neuralgia patients–in depth proteomic analysis using mass spectrometry. J. Pain. 21, 1075–1084. doi: 10.1016/j.jpain.2020.03.002
Hankinson, H. L., and Wilson, C. B. (1976). Microsurgical treatment of hermifacial spasm. West. J. Med. 124, 191–3.
Hirono, S., Yamakami, I., Sato, M., Kado, K., Fukuda, K., and Nakamura, T. (2014). Continuous intraoperative monitoring of abnormal muscle response in microvascular decompression for hemifacial spasm; a real-time navigator for complete relief. Neurosurg. Rev. 37, 311–320. doi: 10.1007/s10143-013-0507-5
Illingworth, R. D., Porter, D. G., and Jakubowski, J. (1996). Hemifacial spasm: a prospective long-term follow up of 83 cases treated by microvascular decompression at two neurosurgical centres in the United Kingdom. J. Neurol. Neurosurg. Psychiatry. 60, 72–77. doi: 10.1136/jnnp.60.1.72
Ishikawa, M., Ohira, T., Namiki, J., Gotoh, K., Takase, M., and Toya, S. (1996). Electrophysiological investigation of hemifacial spasm: F-waves of the facial muscles. Acta Neurochir. (Wien). 138, 24–32. doi: 10.1007/BF01411719
Ishikawa, M., Ohira, T., Namiki, J., Kobayashi, M., Takase, M., and Kawase, T. (1997). Electrophysiological investigation of hemifacial spasm after microvascular decompression: F waves of the facial muscles, blink reflexes, and abnormal muscle responses. J. Neurosurg. 86, 654–661. doi: 10.3171/jns.1997.86.4.0654
Kameyama, S., Masuda, H., Shirozu, H., Ito, Y., Sonoda, M., and Kimura, J. (2016). Ephaptic transmission is the origin of the abnormal muscle response seen in hemifacial spasm. Clin. Neurophysiol. 127, 2240–2245. doi: 10.1016/j.clinph.2016.02.004
Kamiguchi, H., Ohira, T., Ochiai, M., and Kawase, T. (1997). Computed tomographic analysis of hemifacial spasm: narrowing of the posterior fossa as a possible facilitating factor for neurovascular compression. J. Neurol. Neurosurg. Psychiatry. 62, 532–534. doi: 10.1136/jnnp.62.5.532
Karki, P., Yamagami, M., Takasaki, K., Bohara, M., Hosoyama, H., and Hanada, T. (2019). Microvascular decompression in patients aged 30 years or younger. Asian J. Neurosurg. 14, 111. doi: 10.4103/ajns.AJNS_266_17
Kim, J. P., Chung, J. C., Chang, W. S., Chung, S. S., and Chang, J. W. (2012). Outcomes of surgical treatment for hemifacial spasm associated with the vertebral artery: severity of compression, indentation, and color change. Acta Neurochir. (Wien). 154, 501–508. doi: 10.1007/s00701-011-1247-3
Kim, P., and Fukushima, T. (1984). Observations on synkinesis in patients with hemifacial spasm. J. Neurosurg. 60, 821–827. doi: 10.3171/jns.1984.60.4.0821
Ko, H. C., Lee, S. H., and Shin, H. S. (2021). Facial nerve indentation in hemifacial spasm: an analysis of factors contributing to the formation of and consequent effects associated with indentation. World Neurosurg. 146, e1083–e1091. doi: 10.1016/j.wneu.2020.11.086
Kuroki, A., and Møller, A. R. (1994). Facial nerve demyelination and vascular compression are both needed to induce facial hyperactivity: a study in rats. Acta Neurochir. (Wien). 126, 149–157. doi: 10.1007/BF01476426
Leal, P. R. L., Roch, J., Hermier, M., Berthezene, Y., and Sindou, M. (2019). Diffusion tensor imaging abnormalities of the trigeminal nerve root in patients with classical trigeminal neuralgia: a pre- and postoperative comparative study 4 years after microvascular decompression. Acta Neurochir. (Wien). 161, 1415–1425. doi: 10.1007/s00701-019-03913-5
Li, C-. L., Yang, R., Sun, Y., Feng, Y., and Song, Y-. B. (2021). N58A exerts analgesic effect on trigeminal neuralgia by regulating the MAPK pathway and tetrodotoxin-resistant sodium channel. Toxins (Basel). 13, 357. doi: 10.3390/toxins13050357
Lin, R., Luo, L., Gong, Y., Zheng, J., Wang, S., and Du, J. (2019). Immunohistochemical analysis of histone H3 acetylation in the trigeminal root entry zone in an animal model of trigeminal neuralgia. J. Neurosurg. 131, 828–838. doi: 10.3171/2018.5.JNS172948
Liu, C., Zhang, Y., Liu, Q., Jiang, L., Li, M., and Wang, S. (2018). P2X4-receptor participates in EAAT3 regulation via BDNF-TrkB signaling in a model of trigeminal allodynia. Mol. Pain. 14, 174480691879593. doi: 10.1177/1744806918795930
Liu, M-. X., Zhong, J., Xia, L., Dou, N-. N., and Li, S-. T. (2019). A correlative analysis between inflammatory cytokines and trigeminal neuralgia or hemifacial spasm. Neurol. Res. 41, 335–340. doi: 10.1080/01616412.2018.1564188
Liu, M. X., Zhong, J., Xia, L., Dou, N. N., and Li, S. T. (2020). IL-6 contributes to Nav1.3 up-regulation in trigeminal nerve following chronic constriction injury. Neurol Res. 42, 504–514. doi: 10.1080/01616412.2020.1747719
Lorenzoni, J., David, P., and Levivier, M. (2012). Patterns of neurovascular compression in patients with classic trigeminal neuralgia: a high-resolution MRI-based study. Eur. J. Radiol. 81, 1851–1857. doi: 10.1016/j.ejrad.2009.09.017
Luo, D. S., Lin, R., Luo, L. L., Li, Q. H., Chen, T., and Qiu, R. H. (2019). Glial Plasticity in the Trigeminal Root Entry Zone of a Rat Trigeminal Neuralgia Animal Model. Neurochem. Res. 44, 1893–1902. doi: 10.1007/s11064-019-02824-2
Marinković, S., Gibo, H., Todorović, V., Antić, B., Kovačević, D., and Milisavljević, M. (2009). Ultrastructure and immunohistochemistry of the trigeminal peripheral myelinated axons in patients with neuralgia. Clin. Neurol. Neurosurg. 111, 795–800. doi: 10.1016/j.clineuro.2009.07.020
Marinković, S., Todorović, V., Gibo, H., Budeč, M., Drndarević, N., and Pešić, D. (2007). The trigeminal vasculature pathology in patients with neuralgia. Headache J. Head Face Pain 47, 1334–1339. doi: 10.1111/j.1526-4610.2007.00933.x
Mazzucchi, E., Brinzeu, A., and Sindou, M. (2019). Arachnoiditis as an outcome factor for microvascular decompression in classical trigeminal neuralgia. Acta Neurochir. (Wien). 161, 1589–1598. doi: 10.1007/s00701-019-03981-7
Møller, A. R., and Jannetta, P. J. (1986). Physiological abnormalities in hemifacial spasm studied during microvascular decompression operations. Exp. Neurol. 93, 584–600. doi: 10.1016/0014-4886(86)90178-0
Mula, M., Strigaro, G., Marotta, A. E., Ruggerone, S., Tribolo, A., and Monaco, R. (2012). Obsessive-Compulsive-Spectrum Symptoms in Patients With Focal Dystonia, Hemifacial Spasm, and Healthy Subjects. J. Neuropsychiatry Clin. Neurosci. 24, 81–86. doi: 10.1176/appi.neuropsych.11020048
Nielsen, V. K. (1984). Pathophysiology of hemifacial spasm: I. Ephaptic transmission and ectopic excitation. Neurology. 34, 418–418. doi: 10.1212/WNL.34.4.418
Oshinsky, M. L., Murphy, A. L., Hekierski, H., Cooper, M., and Simon, B. J. (2014). Noninvasive vagus nerve stimulation as treatment for trigeminal allodynia. Pain. 155, 1037–1042. doi: 10.1016/j.pain.2014.02.009
Pang, H., Sun, H., and Fan, G. (2019). Correlations between the trigeminal nerve microstructural changes and the trigeminal-pontine angle features. Acta Neurochir. (Wien). 161, 2505–2511. doi: 10.1007/s00701-019-04099-6
Park, J. S., Koh, E. J., Choi, H. Y., and Lee, J. M. (2015). Characteristic anatomical conformation of the vertebral artery causing vascular compression against the root exit zone of the facial nerve in patients with hemifacial spasm. Acta Neurochir. (Wien). 157, 449–454. doi: 10.1007/s00701-014-2338-8
Peker, S., Kurtkaya, Ö., Üzün, I. I., and Pamir, M. N. (2006). Microanatomy of the Central Myelin-Peripheral Myelin Transition Zone of the Trigeminal Nerve. Neurosurgery. 59, 354–359. doi: 10.1227/01.NEU.0000223501.27220.69
Ren, C., Chen, M., Mu, G., Peng, S., Liu, X., and Ou, C. (2021). NLRP3 inflammasome mediates neurodegeneration in rats with chronic neuropathic pain. Shock. 56, 840–849. doi: 10.1097/SHK.0000000000001832
Robert, T., Blanc, R., Ciccio, G., Smajda, S., Redjem, H., and Fahed, R. (2015). Trigeminal neuralgia due to arterialization of the superior petrosal vein in the context of dural or cerebral arteriovenous shunt. Clin. Neurol. Neurosurg. 138, 83–88. doi: 10.1016/j.clineuro.2015.08.004
Romero, J., Costa, G. M. F., Rocha, L. P. C., Siqueira, S., Moreira, P. R., and Almeida-Leite, C. M. (2021). Polymorphisms of Nav1.6 sodium channel, Brain-derived Neurotrophic Factor, Catechol-O-methyltransferase and Guanosine Triphosphate Cyclohydrolase 1 genes in trigeminal neuralgia. Clin. Neurol. Neurosurg. 208, 106880. doi: 10.1016/j.clineuro.2021.106880
Terasaka, S., Asaoka, K., Yamaguchi, S., Kobayashi, H., Motegi, H., and Houkin, K. (2016). A significant correlation between delayed cure after microvascular decompression and positive response to preoperative anticonvulsant therapy in patients with hemifacial spasm. Neurosurg. Rev. 39, 607–613. doi: 10.1007/s10143-016-0729-4
Truini, A., Prosperini, L., Calistri, V., Fiorelli, M., Pozzilli, C., and Millefiorini, E. (2016). A dual concurrent mechanism explains trigeminal neuralgia in patients with multiple sclerosis. Neurology. 86, 2094–2099. doi: 10.1212/WNL.0000000000002720
Wang, Q., Yuan, Y., Xiong, N., Fu, P., Huang, T., and Yang, B. (2019a). Anatomic Variation and Hemodynamic Evolution of Vertebrobasilar Arterial System May Contribute to the Development of Vascular Compression in Hemifacial Spasm. World Neurosurg. 124, e233–e240. doi: 10.1016/j.wneu.2018.12.074
Wang, Y., Yang, Q., Cao, D., Seminowicz, D., Remeniuk, B., and Gao, L. (2019b). Correlation between nerve atrophy, brain grey matter volume and pain severity in patients with primary trigeminal neuralgia. Cephalalgia. 39, 515–525. doi: 10.1177/0333102418793643
Wei, B., Kumada, T., Furukawa, T., Inoue, K., Watanabe, M., and Sato, K. (2013). Pre- and post-synaptic switches of GABA actions associated with Cl- homeostatic changes are induced in the spinal nucleus of the trigeminal nerve in a rat model of trigeminal neuropathic pain. Neuroscience. 228, 334–348. doi: 10.1016/j.neuroscience.2012.10.043
Wilkinson, M. F., Chowdhury, T., Mutch, W. A., and Kaufmann, A. M. (2017). Analysis of facial motor evoked potentials for assessing a central mechanism in hemifacial spasm. J. Neurosurg. 126, 379–385. doi: 10.3171/2016.2.JNS151384
Willsey, M. S., Mossner, J. M., Chestek, C. A., Sagher, O., and Patil, P. G. (2021). Classifier using pontine radial diffusivity and symptom duration accurately predicts recurrence of trigeminal neuralgia after microvascular decompression: a pilot study and algorithm description. Neurosurgery. 89, 777–783. doi: 10.1093/neuros/nyab292
Wu, M., Jiang, X., Qiu, J., Fu, X., and Niu, C. (2020). Gray and white matter abnormalities in primary trigeminal neuralgia with and without neurovascular compression. J. Headache Pain. 21, 136. doi: 10.1186/s10194-020-01205-3
Xia, L., Dou, N-. N., Zhong, J., Zhu, J., Wang, Y-. N., and Liu, M-. X. (2014). Upregulation of Nav1.8 in demyelinated facial nerves might be relevant to the generation of hemifacial spasm. J. Craniofac. Surg. 25, 1334–1336. doi: 10.1097/SCS.0000000000000802
Yang, Y., Hu, L., Xia, Y., Jiang, C., Miao, C., and Yang, C. (2016). Resveratrol suppresses glial activation and alleviates trigeminal neuralgia via activation of AMPK. J. Neuroinflammation. 13, 84. doi: 10.1186/s12974-016-0550-6
Yin, Y., Guo, R., Shao, Y., Ge, M., Miao, C., and Cao, L. (2019). Pretreatment with resveratrol ameliorate trigeminal neuralgia by suppressing matrix metalloproteinase-9/2 in trigeminal ganglion. Int. Immunopharmacol. 72, 339–347. doi: 10.1016/j.intimp.2019.04.014
Zhang, P., Selim, M. H., Wang, H., Kuang, W., Wu, M., and Ji, C. (2019). Intraoperative measuring of the offending vessel's pressure on the facial nerve at root exit zone in patients with hemifacial spasm during microvascular decompression: a prospective study. World Neurosurg. 122, e89–e95. doi: 10.1016/j.wneu.2018.09.080
Zhao, L., Jiang, M., and Cao, X. B. D. (2021). TLR8 in the trigeminal ganglion contributes to the maintenance of trigeminal neuropathic pain in mice. Neurosci. Bull. 37, 550–562. doi: 10.1007/s12264-020-00621-4
Zhou, Q., Zhong, J., Jiao, W., Zhu, J., Yang, X., and Ying, T. (2012). The role of autonomic nervous system in the pathophysiology of hemifacial spasm. Neurol. Res. 34, 643–648. doi: 10.1179/1743132812Y.0000000057
Keywords: neurovascular compression syndromes, neurovascular conflicts, trigeminal neuralgia, glossopharyngeal neuralgia, hemifacial spasm, pathogenesis
Citation: Szmyd B, Sołek J, Błaszczyk M, Jankowski J, Liberski PP, Jaskólski DJ, Wysiadecki G, Karuga FF, Gabryelska A, Sochal M, Tubbs RS and Radek M (2022) The Underlying Pathogenesis of Neurovascular Compression Syndromes: A Systematic Review. Front. Mol. Neurosci. 15:923089. doi: 10.3389/fnmol.2022.923089
Received: 18 April 2022; Accepted: 09 June 2022;
Published: 04 July 2022.
Edited by:
Robert John Vandenberg, The University of Sydney, AustraliaReviewed by:
Yan JianHao, Guangdong Second Provincial General Hospital, ChinaTomasz Trojanowski, Medical University of Lublin, Poland
Copyright © 2022 Szmyd, Sołek, Błaszczyk, Jankowski, Liberski, Jaskólski, Wysiadecki, Karuga, Gabryelska, Sochal, Tubbs and Radek. This is an open-access article distributed under the terms of the Creative Commons Attribution License (CC BY). The use, distribution or reproduction in other forums is permitted, provided the original author(s) and the copyright owner(s) are credited and that the original publication in this journal is cited, in accordance with accepted academic practice. No use, distribution or reproduction is permitted which does not comply with these terms.
*Correspondence: Maciej Radek, bWFjaWVqLnJhZGVrQHVtZWQubG9kei5wbA==