- 1Department of Animal Experimentation, Noguchi Memorial Institute for Medical Research (NMIMR), College of Health Sciences, University of Ghana, Accra, Ghana
- 2Department of Anatomy, University of Ghana Medical School, College of Health Sciences, University of Ghana, Korle-Bu Campus, Accra, Ghana
- 3Department of Electron Microscopy and Histopathology, Noguchi Memorial Institute for Medical Research (NMIMR), College of Health Sciences, University of Ghana, Accra, Ghana
- 4Department of Clinical Pathology, Noguchi Memorial Institute for Medical Research (NMIMR), College of Health Sciences, University of Ghana, Accra, Ghana
- 5Department of Epidemiology, Noguchi Memorial Institute for Medical Research (NMIMR), College of Health Sciences, University of Ghana, Accra, Ghana
- 6Department of Immunology, Noguchi Memorial Institute for Medical Research (NMIMR), College of Health Sciences, University of Ghana, Accra, Ghana
- 7Department of Pathology, Korle-Bu Teaching Hospital, Accra, Ghana
Introduction: Increasing levels of anti-malarial drug resistance makes the development of novel drugs against malaria a necessity. Honeybee products, such as propolis, venom and honey, have been employed in traditional medicine to prevent and treat many diseases since ancient times. In theory, extracting the biologically active components of such natural products could result in the development of non-toxic and highly potent antimicrobial agents. The objectives of this study were to evaluate the pre-clinical anti-plasmodial activity and safety of BEEMAR, a patented formulation extracted from specific parts of the honeybee colony frames and suspended in-enhanced marine plasma.
Methods: Different concentrations of the BEEMAR were tested against Plasmodium falciparum (3D7 strain) in vitro using Sybr green growth inhibitory assay and Plasmodium berghei (NK65 strain) in vivo using Rane’s test. Acute and sub-acute oral toxicity tests, based on Organization for Economic Cooperation and Development (OECD) guidelines, were used to assess the safety profile of the product.
Results: The product demonstrated significant (p<0.05) dose-dependent inhibition of the growth of 3D7 P. falciparum strain, in vitro, with IC50 of 0.55 mg/ml. In Rane’s in vivo test, a maximum suppression of 69% was obtained at the highest dose of 1% (w/v) of the product compared to that of the standard drug, Artemether-Lumefantrine (64% at a concentration of 4mg/kg body weight) in ICR mice. Administration of the product did not result in any clinical signs of haematological or biochemical toxidromes in treated SD rats compared to the control SD rats.
Discussion: This study suggests that the BEEMAR product displays promising anti-plasmodial activity that is dose dependent. BEEMAR also exhibits an appreciable safety profile that requires further investigation.
Introduction
Malaria is one of the most significant infectious diseases globally, resulting in an estimated 247 million cases and approximately 619,000 deaths in 2021 (World malaria report, 2022). According to the World Health Organization, nearly half of the world’s population still lives in areas where the disease is transmitted. Plasmodium falciparum is responsible for most of these cases and deaths, particularly in sub-Saharan Africa, where the disease burden is highest (Liu et al., 2021). Current treatments for malaria are combination therapies that include artemisinin products as one major component but there is increasing evidence of the development of parasite resistance to these drugs, especially in South-East Asia (Lyu et al., 2021). The emergence of drug-resistant strains of P. falciparum has hampered efforts to control malaria, necessitating the development of alternative therapies. Natural products, especially those derived from plants and fungi have shown promise as anti-malarial agents (Seabrooks and Hu, 2017; Adnani et al., 2017). There is a growing interest in expanding the sources of antimicrobial and antimalarial drugs by using unprecedented multi-species resources to discover new therapies. In this context, the exploitation of honeybee products by humans for medicine and therapy has been going on for centuries.
Honeybees are known to produce honey, propolis, royal jelly, and bee venom, which have been reported to possess antimicrobial, anti-inflammatory, antioxidant, and immunomodulatory properties (Kocot et al., 2018; Alvarez-Suarez et al., 2014; Pharmaceutical Prospects of bee products, 2025). Bees forage over large distances to provide food for the hive, and in the process encounter a myriad and ever-changing array of bacteria, spores, parasites and viruses on the plants, and foliage they interact with (Santorelli et al., 2023). Despite their social interactions in the populated hive environment and the potential for any pathogens they carry to spread, bees are quite resilient and may benefit from some type of “herd” immunity (Chan et al., 2009). The hive has evolved highly effective nanomaterials to deal with the daily multitude of returning pathogens. Central to the honeybee immunological strategy is lysozyme, which is known to have direct antimicrobial properties but also modulates the host immune response to infection. Lysozymes generally are highly active antimicrobial agents of the innate immune system of animals and plants and are active against bacteria, viruses and fungi (Ferraboschi et al., 2021). In parallel to honeybee bioactive compounds, marine organisms’ resources are limitless and consistently prove efficacious at combatting viruses, bacteria, cancers, and other pathogens. Their unique chemical structures and diversity introduce novel modes of action, making them especially valuable against drug-resistant pathogens (Geahchan et al., 2021).
BEEMAR is a proprietary natural formulation that contains extracts from specific parts of the colony frames that are rich in lysozymes and other immune derivatives, suspended in Enhanced Marine Plasma, a modified ion and vitamin-rich seawater obtained from the depths of the ocean vortex where phytoplankton and zooplankton interact (Plasma Marino, 2025). The formulation is owned by the Irish biotech company CellNUA It is believed that BEEMAR could be effective against a wide range of pathogens, including viruses, bacteria, protozoan parasites, and fungi. The anti-Plasmodium efficacy and toxicological effects of BEEMAR have not been investigated. Vertebrate animals are used as models in medical testing because their genetic, biological and behaviour characteristics closely resemble those of humans, and many symptoms of human conditions can be replicated in several of these organisms. Rats and mice are mammals that share many processes with humans and are appropriate for use to answer many research questions. Mice and rat models of many human diseases have also been developed to advance the studies of disease pathogenesis, and to evaluate the effectiveness and toxicities of various candidate drugs. Their result can easily be extrapolated in humans. In this study, we aimed to assess the anti-plasmodial activities and toxicological effects of BEEMAR using both in vitro and in vivo approaches to generate data on BEEMAR’s potential as an alternative anti-malarial product. We demonstrate significant anti-malarial activity against both human and murine malaria parasites and show a generally safe profile at the acute and sub-acute oral toxicity levels. There were however some few exceptions such as increased ALT levels and moderate interstitial inflammation in the lungs, as well as decreased monocyte and platelet counts in treated animals compared to control animals.
Materials and methods
Ethics statement
This study protocol was approved by the NMIMR Scientific and technical committee with reference number 8(4) 2021-22 and the animal study was reviewed and approved by the University of Ghana Institutional Animal Care and Use Committee (Certified Protocol Number: UG-IACUC 009/21-22). All procedures and techniques used on animals in this study were in accordance with the ARRIVE guidelines (Supplementary Material). These animals were originally obtained from Harlan and were bred and maintained in the specific pathogen-free (SPF) barrier suite of the Animal Facility at the Noguchi Memorial Institute for Medical Research, University of Ghana. All animals used in this experiment were transferred from the breeding suit and allowed to acclimatize for seven (Alvarez-Suarez et al., 2014) days before commencement of experiment. Grouping of animals was based on weight-matching. Animals were humanely euthanised prior to necrotic assessment and, tissue and organ harvesting, by injecting sodium pentobarbital at a dose of 100 mg/kg intraperitoneally. Quality assurance was maintained in accordance with good laboratory practice.
Preparation of BEEMAR doses
An admixture of lysozyme and other bioactive molecules were extracted from specific parts of the colony frames by weighing 20 g of the caps or crushed honeycombs and mixing with 330 mL of distilled water. This was then homogenized into a suspension using a mixer at 35,000 rpm for 5 min. The suspension formed is centrifuged at 11,650 × g for 10 min. The supernatant was collected, and the sediment further homogenized in 160 mL of demineralised water and centrifuged at 11,650 × g for 5 min. The supernatant from the second centrifugation was added to the first. The supernatant was collected and filtered using the membrane filter (pore size of 0.2–0.45 µm). The resulting solution was collected and dried using a vacuum evaporator at 180–200 rpm in a water bath set to between 38°C and 41°C and a vacuum pressure of 0.15–0.20 Bar. The extract was subsequently resuspended in enhanced marine plasma and a 5% solution prepared as stock for in vitro and in vivo testing.
In vitro anti-plasmodial assessment of BEEMAR
The in vitro sybr green growth inhibitory assay was used to determine the activity of BEEMAR against the 3D7 laboratory strain of P. falciparum. Briefly, a 1% solution of BEEMAR was serially diluted from 0.25% to 0.00195% (2-fold serial dilutions) in complete parasite culture medium (CPM) which consists of Incomplete Parasite Medium (IPM) (RPMI-1640 media containing 50 μg/mL Hypoxanthine, 25 mM HEPES, 2 mg/mL NaHCO3, 5 μg/mL gentamicin and 0.3 mg/ml L-glutamine) supplemented with 0.5% Albumax II and 2% O-positive normal human serum. An aliquot of 50 µL of every test concentration was added in triplicates to a 96-well plate. Chloroquine and CPM were also added to the plates as positive and negative controls respectively for activity. This was followed by the addition of 50 µL of synchronized 3D7 test parasite culture at 4% haematocrit and 1% parasitaemia. The plates were then placed in a modular incubation chamber, gassed with a gas mixture containing 5% CO2, 5% O2, and 90% N2, and the chamber incubated at 37°C for 72 h. The plates were then frozen at −20°C overnight, after which they were thawed and each well incubated with 100 µL of lysis buffer containing 1X sybr green 1 at room temperature for 1.5 h. Afterwards, fluorescence in the plates were read in a Varioskan Lux fluorometer at an excitation of 497 nm and emission of 530 nm wavelength. The IC50 for each compound was determined using GraphPad Prism software and the data used to fit a dose-response curve. Three independent experiments were carried out to determine the IC50 of BEEMAR.
In vivo anti-plasmodial evaluation of BEEMAR
Animals
A total of 40 female Institute of Cancer Research (ICR) mice (Hsd:ICR) aged 6–8 weeks old were used in this study. They were housed in normal rodent cages with dimensions 425 mm × 276 mm × 153 mm (Techniplast S.p.A, Italy) with sterilized soft wood shavings as bedding and were provided with rodent feed pellets from AGRIFEEDS (AGRICARE LTD., Kumasi, Ghana), as well as clean water ad libitum. The animals were kept under controlled laboratory conditions, which included a temperature of 23°C ± 2°C, relative humidity of 60%–70%, and a 12-h light-dark cycle.
Preparation of inoculum
The study used chloroquine-sensitive Plasmodium berghei (NK65 strain) that had been frozen at 5% parasitized red blood cells. The frozen cells were thawed and injected into donor ICR mice via the intraperitoneal route using a 200 μL volume per mouse. The mice were then observed daily to detect the development of parasitaemia, which was determined by counting the number of infected red blood cells relative to the total number of red blood cells. When parasitaemia levels reached 5%, the donor mice were humanely euthanatized by injecting sodium pentobarbital at a dose of 100 mg/kg intraperitoneally, and blood was collected through cardiac puncture using a sterile syringe and placed in a heparinized tube. The blood was diluted with 0.9% saline to achieve a concentration of 1 × 107 P. berghei-infected red blood cells. Finally, 0.2 mL of the infected blood was administered to each of the test mice via intraperitoneal injection.
Curative test (Rane’s test)
Evaluation of the curative potential of the BEEMAR product was carried out according to a previously described method (Ryley and Peters, 1970; Uzor et al., 2021). On day 0, the standard inocula of 1 × 107 P. berghei-infected red blood cells (0.2 mL) were injected intraperitoneally (n = 40) and the mice were observed for the establishment of infection. Seventy-two hours later, the mice were randomly grouped into 8 (n = 5/cage) and were dosed orally from day 3 to day 7, once daily. Test groups (groups 2–7) orally received varying doses (100–10−5) (w/v) of BEEMAR diluted with distilled water. The highest dose of 1% (w/v) was equivalent to 98.7 mg/kg, and this was serially diluted 10-fold to obtain the other five doses. The positive control group (group 1) was given 4 mg/kg of artemether/lumefantrine (AL) as a control drug, and the negative control group (group 8) was given 10 mL/kg of distilled water. A summary of the various animal treatments is provided in Table 1.
Parasitaemia measurement
Parasitaemia was monitored daily, beginning on day 3, by preparing a thin blood film from the tail of each mouse. The smears were prepared on microscope slides (76 × 26 mm) (Menzel-Glaser, Germany), air-dried, fixed with absolute methanol for 1–3 min, air dried and stained with 10% Giemsa at pH 7.2 for 15 min. The stained slides were then washed gently using distilled water and air-dried at room temperature.
Two stained slides for each mouse were examined under a light microscope (Olympus with an oil immersion nosepiece of ×100 magnification). Three different fields on each slide were examined to calculate the average parasitaemia as previously described (Bantie et al., 2014) and shown below;
where “para” means parasitaemia.
Additionally, the percent parasitaemia suppression of the product was compared to the controls, and parasitaemia suppression was calculated as described previously (Kalra et al., 2006).
Toxicological assessment of BEEMAR
Acute toxicity study
The single-dose acute oral toxicity study following the recommendations by OECD Guidelines (425) (OECD, 2022) was used to evaluate the acute oral toxicity of BEEMAR in young, nulliparous, and nonpregnant female Sprague Dawley (SD) rats (Hsd:SD). The rats were kept in polycarbonate cages dimensions 480 mm × 265 mm × 210 mm (Techniplast S.p.A, Italy) with sterilized soft wood shavings as bedding and were provided with rodent feed pellets from AGRIFEEDS (AGRICARE LTD., Kumasi, Ghana), as well as clean water ad libitum. The animals were kept under controlled laboratory conditions, which included a temperature of 23°C ± 2°C, relative humidity of 60%–70%, and a 12-h light-dark cycle. A one-time oral administration of treatments and control was given to the experimental animals. 10 female SD rats were randomly divided into two (Liu et al., 2021) groups of five (Adnani et al., 2017) per cage, with one group receiving an undiluted dose (5%) of BEEMAR and the other group serving as a control and receiving distilled water. The rats were observed for clinical signs of toxic effects such as piloerection, draping, tremors, excitability, miosis, mydriasis, twitching, salivation, morbidity and mortality. This observation was conducted on the day of administration and daily for the next 14 days. Body weight was measured before administration, on the day of administration, and weekly thereafter. On day 15, the animals were euthanized by injecting sodium pentobarbital at a dose of 100 mg/kg intraperitoneally, blood was collected via cardiac puncture for haematological and serum biochemical analysis and a postmortem examination was performed on the major organs.
28-day repeated dose oral toxicity study
A repeated 28-day oral toxicity study was conducted using the OECD Guideline 407 (OECD, 2008). A total of 20 SD rats were randomly divided into four groups (n = 5/cage) and were housed as described previously. The control group was given distilled water (10 mL/kg), while the other three groups were orally administered BEEMAR daily through gavage at low (1%), medium (2.5%), and high (5%) doses for 28 days. Throughout the 28-day treatments, the animals were observed daily for general health and clinical signs of toxicity, whereas body weight changes were recorded on days 0, 7, 14, 21, and 28 of the experiment, with the euthanasia criteria being 15% weight loss. On day 29, the animals were euthanized using isoflurane. Following euthanasia, blood was collected via cardiac puncture for haematological and serum biochemical analysis. Organs were then harvested and processed for histopathological analyses.
Haematological and biochemical analyses
Blood samples were collected into two different types of tubes for both the acute (day 15) and subacute (day 29): EDTA tubes and serum separator tubes. The blood in EDTA tubes was immediately analysed using the SYSMEX haematology autoanalyzer (Kobe, Japan). This analysis included determining the leukocyte count, erythrocyte count, haemoglobin concentration, haematocrit, mean corpuscular volume (MCV), mean corpuscular haemoglobin (MCH), mean corpuscular haemoglobin concentration (MCHC), reticulocyte ratio, platelet count, and differential leukocyte counts. The clotted blood in serum separator tubes was centrifuged at 4,000 g for 15 min to separate the serum. The resulting serum samples were then transferred into cryovials for immediate analysis. The biochemical assays were performed using the SELECTRA JUNIOR Version 04 autoanalyzer (Vital Scientific, Spankeren, Netherlands) and included determining the levels of total bilirubin (TBIL), direct bilirubin (DBIL), aspartate aminotransferase (AST), alanine aminotransferase (ALT), total protein (TP), albumin (ALB), globulin (GB), alkaline phosphatase (ALP), ɣ-glutamyl transpeptidase (ɣ-GT), urea (URE), and creatinine (CR).
Histopathological tissue processing and examination
For each of the test groups of rats used in the toxicological assessment of the product, four (Seabrooks and Hu, 2017) out of the five rats were randomly selected for histological processing of the six (Kocot et al., 2018) major organs. The kidneys, liver, lung, spleen, brain and heart of treated and control animals that were randomly selected, were harvested, and wet organ weights were measured following gross necropsy. The organs were then fixed using 10% neutral buffered formalin. Histological processing and examinations were done as previously described (Mahama et al., 2022). Micrographs were obtained from images taken from an average of three (Lyu et al., 2021) different fields.
Data and statistical analyses
GraphPad v8.0 was used to conduct all statistical analyses. All animals used in the experiments were included in the data analysis. The Student’s t-test and one-way ANOVA (analysis of Variance) were used to compare the mean variations in data from animal/organ weights, haematological and biochemical tests. A post hoc Tukey test was performed at a 95% confidence interval for multiple comparisons where appropriate. The results were expressed as mean ± S.D. Histology scores were compared between treated animals and controls using the Mann-Whitney U test. Statistical significance in all cases was considered at p < 0.05.
Results
In vitro anti-plasmodial activity of BEEMAR against Plasmodium falciparum
To assess the potency of BEEMAR against the human malaria parasite, the product was tested at - 1% (w/v) against the 3D7 laboratory strain of P. falciparum in vitro. There was a dose-dependent inhibition of the growth of the parasite (Table 2; Supplementary Material) with an average IC50 of 0.055% (0.55 mg/mL) from three repeat assays.
In vivo anti-plasmodial activity against Plasmodium berghei
The BEEMAR product was further tested in vivo using ICR mice and the mouse malaria parasite P. berghei. The results of the antimalarial test, based on Rane’s curative model, showed a dose-dependent inhibition of parasitaemia by BEEMAR, compared to the control group. The lowest dose of BEEMAR (10−5) inhibited parasite multiplication by 34.63% while the highest dose (100) produced an inhibition of 69.04%. The highest dose of BEEMAR gave a slightly higher inhibition than the standard drug, AL (64.76% inhibition at a concentration of 4 mg/kg body weight). While the longest survival time of the untreated mice (control group) was about 22 days, the higher concentrations of the BEEMAR product prolonged the survival time of mice up to 29 days (Figure 1).
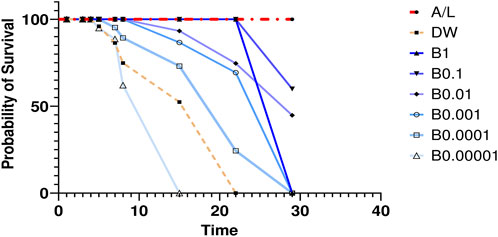
Figure 1. Survival times of mice treated with different concentrations of BEEMAR Artemether/lumefantrine (AL) was used as a positive control, and the untreated control group were administered distilled water (DW).
Toxicological profile of BEEMAR in SD rats
Effect of BEEMAR® at the acute level
The acute toxicity study indicated that the BEEMAR product caused no mortality at a 5% (w/v) concentration within the first 24 h and for the subsequent 14 days. Administration of the product did not result in any clinical signs of toxidromes such as abnormality in movements, salivation, difficulty in breathing, frequency, and consistency of stool, and mortality as compared to the control group. There was, however, a significant increase (p = 0.0033, Student’s t-test) in the body weight of the BEEMAR product-treated rat (25.20 ± 2.332) compared to the control (13.2 ± 1.744) as shown in Table 3. Though the relative weights of the kidneys, spleen, heart and lungs were similar, a significant variation in the liver weight between the test and control groups was observed (p = 0.025, Student’s t-test, Table 4). Post-mortem examination of the viscera, kidney, liver, brain, spleen and heart revealed no visible abnormal changes in the treatment groups in comparison with the control. Haematology and serum biochemistry analyses at the end of the 14-day study did not reveal statistically significant differences in most parameters except for a reduction in the % monocytes and plate counts (Table 5) as well as an increase in ALP (Table 6) in treated mice compared to controls.
Effect of BEEMAR on weight of rats at the sub-acute level
There was no significant difference in the body weight between animals who were treated with different concentrations of BEEMAR and control mice over the 28 days (Figure 2). The relative organ weights of the animals were also not significantly different between the treated groups and the control group (Table 7).
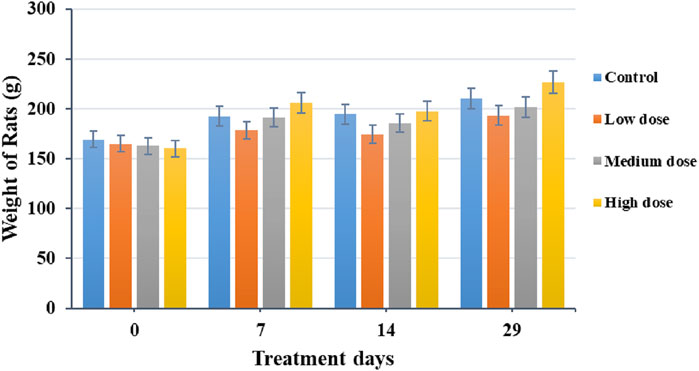
Figure 2. Effect of BEEMAR on body weight (g) during the 28-day sub-acute acute toxicity study. The bars represent the average weight of animals in each treatment group and error bars represent the standard deviation of weights per treatment group. Low dose = 1% (w/v) of BEEMAR in enhanced marine plasma, medium dose = 2.5%(w/v), and high dose = 5%(w/v).
Effect of BEEMAR on haematological indices of rats at the sub-acute level
Results of the study indicate that the administration of the product generally caused no variation in the haematological parameters of the rats. However, there was a significant decrease in the red cell distribution width (RDW) and RDW_CV for the high dose, when compared to the control (p = 0.02, Tukey post hoc test, Table 8). With respect to biochemical parameters, the medium (p = 0.013, Tukey Post-hoc test) and high (p = 0.019, Tukey Post-hoc test) doses of the product resulted in statistically significant increase in ALT of treated animals compared to controls (Table 9). Also, the high dose of the product resulted in a significantly higher ALT/AST ratio compared to the control animals.
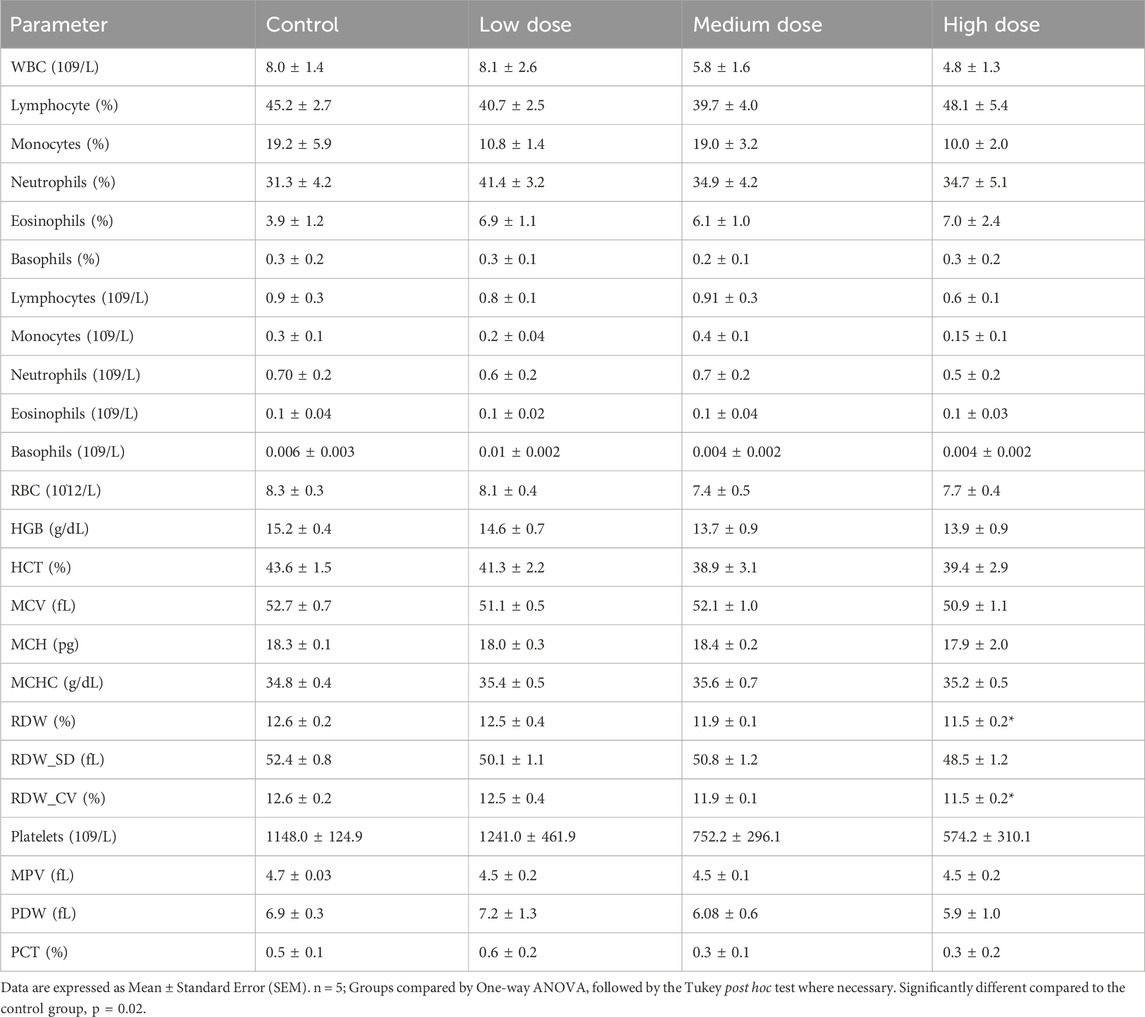
Table 8. Effect of BEEMAR on the haematological indices of the rats in the 28-day sub-acute toxicity study.
Effect of BEEMAR on tissue damage
Histological evaluations of all six main organs were performed to verify the product’s overall toxicological profile at both acute and sub-acute levels. No necropsy or other histological damages were observed in the brain, heart, kidney, liver, or spleen at the acute stage (Figure 3). Although none of the treated rats (n = 5) exhibited any congestion, haemorrhage or interstitial fibrosis in the lungs, there was mild interstitial inflammation (pockets of interstitial space infiltration, n = 5) with significant (p < 0.0001) histological quantification score compared to the control (Figure 4).
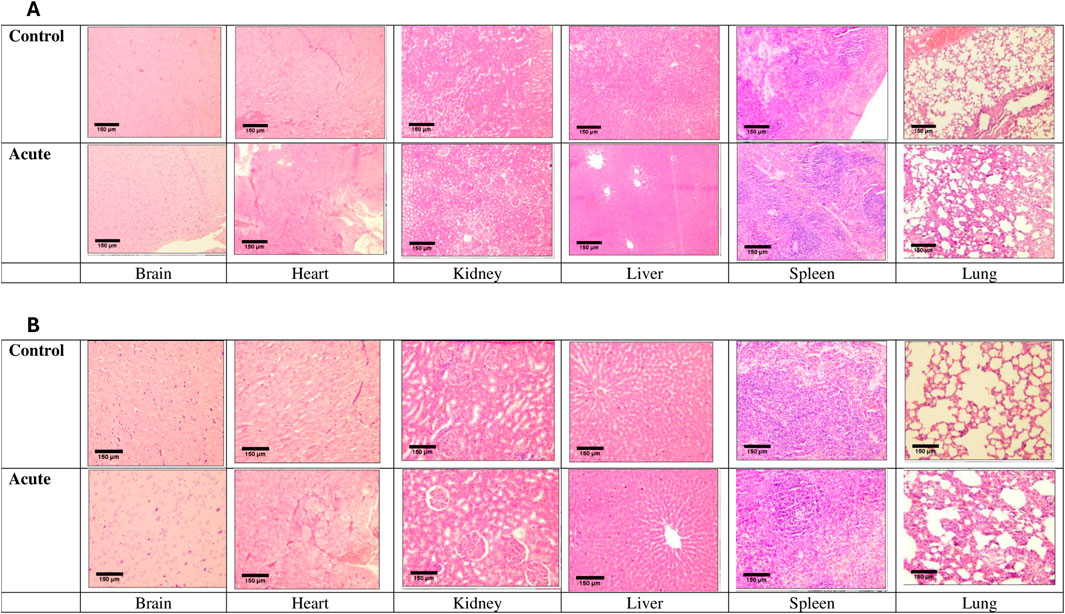
Figure 3. Micrographs of organs of rats under acute treatment compared with control. Images were captured at both x40 (A) and ×100 (B) objective magnifications and a ×10 eyepiece magnification using an Olympus light microscope.
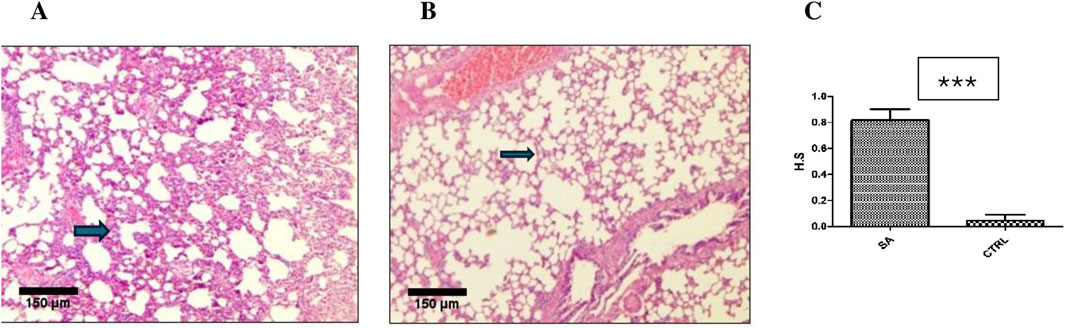
Figure 4. Effect of BEEMAR on rat lung at the acute level. (A) Photomicrograph of lung sections (H&E, x 4, scale bar = 150 um) of BEEMAR treated rats (acute) with mild infiltration of the interstitial space. Note the corresponding thicker septum (arrow). (B) Normal lung tissue of a control (CTRL). Note the relatively thinner septum (arrow). (C) Histological scoring (H.S) of acute treated rats compared with controls. The difference in scores was significant (p < 0.0001) upon analysis with the Man-Whitney U test.
This was further demonstrated at the sub-acute level, where there was mild to moderate interstitial lymphocytic infiltration in the lungs: low dose (n = 3), medium dose (n = 1), and high dose (n = 4). Two low dose and three high dose rats showed moderate interstitial lymphocytic infiltration of the interstitium, which thickened the interalveolar septa without causing fibrosis (Figure 5).
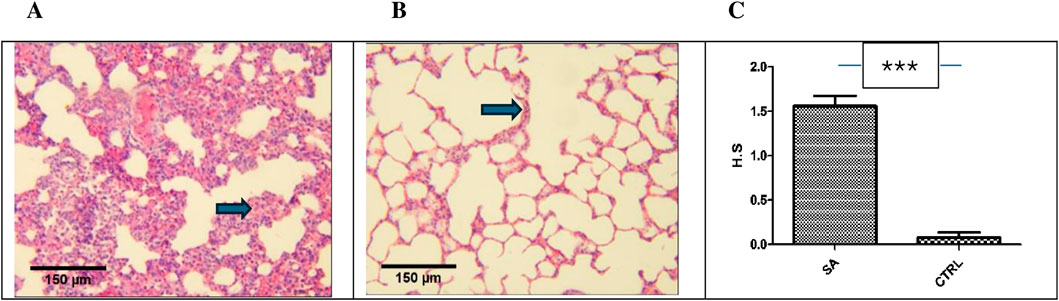
Figure 5. Photomicrograph of lung sections from sub-acute study (H&E, x 10, scale bar = 150 um). (A) BEEMAR treated rats (sub-acute) showing moderate lymphocytic interstitial infiltration. Note the thickened septa (arrow). (B) Control mice with fairly normal lung tissue. Note the relatively normal thickness of the septum (arrow) (C) The median histological scores (H.S) for sub-acute (S.A) treated rats compared with control mice (CTRL) were statistically significant (p < 0.0001) upon analysis with the Mann-Whitney’s U test.
Kidneys of BEEMAR treated rats (sub-acute) showed mild interstitial lymphocytic infiltration but with normal architecture of glomeruli, tubules and vessels as compared to the control group (Figure 6). The median histological scores compared to control, however, was not statistically significant (p > 0.05). In the case of the liver, without sinusoidal congestion, haemorrhage, hydropic alteration, steatosis, bridging necrosis, or inflammation, two high dose rats developed isolated moderate periportal inflammation (Figure 7). The lobular architecture was normal, and the median histological scores showed no significant difference statistically (p > 0.05).
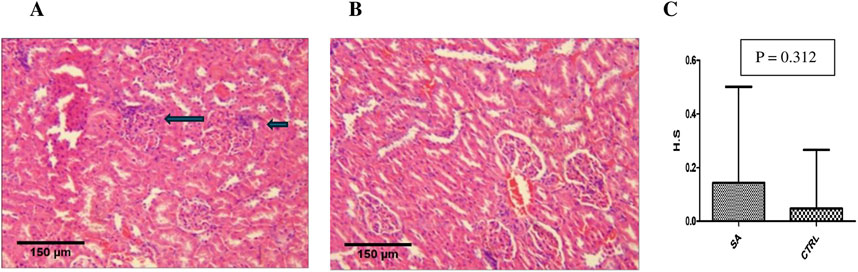
Figure 6. Photomicrograph of kidney sections from sub-acute study (H&E, x 10, scale bar = 150 um). (A) BEEMAR treated rats (sub-acute) showing mild interstitial lymphocytic infiltration (arrows). Note the glomeruli, tubules and vessels are all normal in architecture. (B) Control rats with fairly normal histological architecture. (C) The median histological scores (H.S) for sub-acute (S.A) treated rats as compared to control (CTRL) rats. Note that the difference between the two animal groups is not statistically significant (p > 0.05) upon analysis with the Mann-Whitney’s U test.
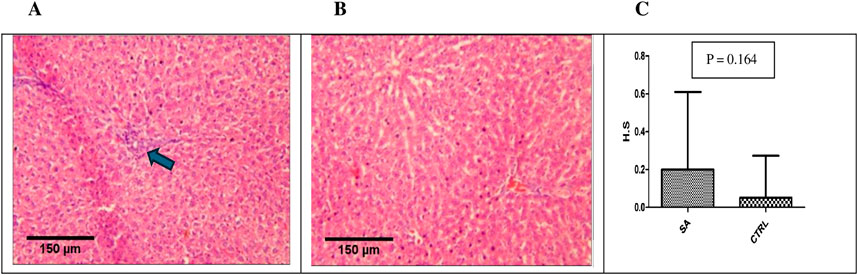
Figure 7. Photomicrograph of liver sections from sub-acute study (H&E, x 10, scale bar = 150 um). (A) BEEMAR treated rats (sub-acute) showing mild periportal lymphocytic infiltration (arrow). (B) control rats with relativley normal liver histological architecture. (C) Median histological scores (H.S) for sub-acute (S.A) treated rats compared with controls (CTRL). Note the difference is not statisticaly significant (p > 0.05).
All other organs were normal. There was neither fibrosis nor necrosis, nor was there any intramuscular bleeding in the heart. The brains of all test animals were free of congestion, haemorrhage, inflammatory infiltration, oedema, and gliosis while the spleens, on the other hand, displayed typical red and white pulp and no macroscopic abnormalities.
Discussion
Despite potentially encountering several microbes as they forage for nectar, and the possibility of easy spread of such microbes through their social interactions in populated hives, honeybees seem quite resilient to infection and disease-causing microbes. Humans have historically used honeybee products for medical and therapeutic purposes. However, their utility in modern medicine is limited due to a lack of scientific support. The potential side effects and adverse reactions associated with these remedies, like the cytotoxicity of honeybee venom, raise concerns about their widespread use in medical treatments. Few investigations on bioactive molecules and compounds derived from honeybee products have sought to understand their modes of action and targets, and more specifically their antigenicity, immunogenicity, and allergenicity. Honeybee products including propolis, honey, royal jelly, honeybee venom and wax have all been demonstrated to have antimicrobial properties (Loukas and Maria, 2023; Obeidat et al., 2024). Research on the pharmacological activity of honeybee products has increased in recent decades, disclosing numerous biological properties. The medicinal properties of honeybee products may in part be due to their strong innate immune defences, and lysozymes secreted by honeybees have been identified as one key component of this efficient innate defence. This forms the basis for the formulation of BEEMAR, which is an extract of bioactive compounds from specific parts of honeybee colony frames that are rich in lysozymes and other immune derivatives, and suspended in Enhanced Marine Plasma, a modified ion rich seawater obtained from highly specific locations in ocean vortices. This study therefore evaluated the anti-plasmodial and toxicological effects of BEEMAR using both in vitro and in vivo approaches.
The tested product showed in vitro activity against the 3D7 laboratory strain of P. falciparum tested although with a high IC50 of 0.55 mg/mL. The BEEMAR production protocol is expected to yield a high concentration of proteins, mainly lysozymes, which have been reported to have activity against microbes (Kunat-Budzyńska et al., 2023). Confirmation of the antimalarial activity of honeybee lysozyme alone, however, will require purification of the honeybee lysozyme from the crude product for subsequent testing, which was not done in this study. Although no study has reported on any type of lysozyme as having antimalarial activity in vitro, in vivo studies have shown that silencing lysozyme activity in mosquitoes decreased their infectivity with mouse malaria parasites (Kajla et al., 2011), suggesting a protective role of mosquito lysozyme for the malaria parasite. There is the possibility that the antimalarial activity of BEEMAR observed in this study is due to other components of BEEMAR that may be playing an indispensable role together with the lysozyme to produce the observed antimalarial activity. Also, as a crude extract, BEEMAR is expected to have some other honeycomb-extract bioactive compounds such as polyphenols and free fatty acids as reported from hydro-ethanolic extracts from honeycomb (Zhao et al., 2020). Polyphenols from different sources have also been reported to have anti-plasmodial activity (Dumitru et al., 2022; Mamede et al., 2020).
The in vivo anti-plasmodial assessment was done using a mouse model since the model considers the possible involvement of the immune system and prodrug effect in the activity of the test substance against the parasite (Kifle et al., 2020). ICR mice were chosen because they provide an excellent model that allows for rapid parasitaemia establishment with P. berghei and this presents a similar model of P. falciparum malaria in man suitable for in vivo drug testing. Several conventional antimalarial agents such as chloroquine, halofantrine, mefloquine and more recently artemisinin derivatives have been identified using the rodent model (Tarkang et al., 2014). Rane’s test was used to study curative ability during established infection by P. berghei (which is sensitive to chloroquine). In vivo anti-plasmodial activity can be classified as moderate, good, and very good if an extract displayed a percentage parasitaemia suppression equal to or greater than 50% (Deharo et al., 2001). Based on this classification, BEEMAR has shown a very good anti-plasmodial activity, suppressing up to 69% of parasites in vivo, making the product qualified for further evaluation of its antimalarial property. Additionally, the prolongation of the survival time of the infected mice for up to 29 days by the product was comparable to that of the standard drug and is further proof of the strong antimalarial potential of the product.
Toxicity may be described as the degree to which a chemical substance causes damage to exposed tissue and includes its effect on the entire organism as well as cells and organs (Mensah M et al., 2019). In this study, acute and 28-day subacute oral toxicity was done to ascertain the safety of the product. At a high dose of 5% (see dosage preparation), the product did not result in any death of the experimental animals, and no clinical signs of local or systemic toxic effects were observed. These results were positive signs of general safety and denoted that the product does not affect general behaviour.
At the acute level, there was a significant elevation in ALP and reduced relative weight of the liver. Increased hepatic enzyme activity demonstrably parallels the rise in serum ALP activity and may remain elevated for up to 1 week after the resolution of biliary obstruction (Green and Sambrook, 2020) but moderate levels are nonspecific as it can occur in a variety of conditions affecting the liver (Lowe et al., 2023). Additionally, relative organ weight indicates whether the organ has been exposed to injury or otherwise (Kharchoufa et al., 2020). In effect, elevated ALP coupled with the reduced relative weight of the liver suggests some damage to the liver, but this was not the case in the histopathological examination (Figure 3). Acute toxicity data are usually of limited clinical application. Therefore, sub-acute toxicity study was carried out. The normal levels of ALP and relative organ weight of the liver during the 28–day subacute study (Tables 8, 9) suggest the damage during the acute study was reversible.
The repeated dose toxicity tests provide information on toxic effects, identification of target organs, effects on animal physiology, haematology, biochemical profile, and histopathology. In the present study, the body weight and the relative organ weights of all treated rats did not differ significantly (P > 0.05) from those of the control groups. It indicates that the product did not affect appetite or adverse effects on the growth of the animals.
Histologically, given that interstitial inflammation (in the lungs and kidney) and periportal inflammation (in the liver) was evident in treated rats, compared to controls, and was more evident in those that received the high dose (Figures 4–7), it is possible that this was caused by exposure to the product (drug reaction). The effects were particularly significant on the lungs at both acute and sub-acute levels, but the test animals’ ability to perform cardiorespiratory functions was unaffected, nevertheless. Nonetheless, this calls for further investigation into the effect of BEEMAR on lung health.
Rats that received the high dose treatment had minor, focal periportal inflammation in their liver, which did not harm the hepatocytes. This was consistent with the animals’ normal liver biochemistry tests performed. Honey products are widely recognized for their abundant vitamin content and bioactive components, which enhance their potential therapeutic benefits, with demonstrated hepato-protection (Mohd et al., 2024). Overall, we can conclude that the rats either acutely or sub-acutely tolerated the product at the dosage tested.
In summary, the results from this study show that BEEMAR has both in vitro and in vivo anti-plasmodial activity as it inhibits the growth of the 3D7 strain of P. falciparum and suppresses the growth of the NK65 strain of the P. berghei, respectively. The product also exhibited a generally safe profile at the acute and sub-acute oral toxicity levels, with few exceptions such as increased ALT levels, the significantly moderate interstitial inflammation in the lungs, and decreased monocyte and platelet counts compared to control animals. These results highlight the application of insect immune factors as potential treatment for human diseases and warrants further investigations into the effectiveness of the products against other pathogens.
Data availability statement
The original contributions presented in the study are included in the article/Supplementary Material, further inquiries can be directed to the corresponding author.
Ethics statement
The animal study was approved by University of Ghana Institutional Animal Care and Use Committee (Certified Protocol Number: UG-IACUC 009/21-22). The study was conducted in accordance with the local legislation and institutional requirements.
Author contributions
SA: Conceptualization, Investigation, Methodology, Supervision, Validation, Writing – original draft. RO-K: Data curation, Formal Analysis, Investigation, Writing – review and editing. IA: Data curation, Formal Analysis, Investigation, Visualization, Writing – review and editing. CA-T: Data curation, Investigation, Visualization, Writing – review and editing. DA: Investigation, Visualization, Writing – review and editing. SO: Formal Analysis, Investigation, Visualization, Writing – original draft. FA: Formal Analysis, Methodology, Visualization, Writing – review and editing. SD: Data curation, Formal Analysis, Investigation, Supervision, Visualization, Writing – review and editing. KD: Data curation, Investigation, Validation, Visualization, Writing – review and editing. MN: Investigation, Methodology, Validation, Writing – review and editing. MH: Data curation, Formal Analysis, Investigation, Visualization, Writing – review and editing. IE: Data curation, Formal Analysis, Investigation, Visualization, Writing – review and editing. FK: Data curation, Formal Analysis, Investigation, Methodology, Writing – original draft, Writing – review and editing. LA: Investigation, Methodology, Validation, Visualization, Writing – original draft. KK: Conceptualization, Data curation, Funding acquisition, Investigation, Methodology, Project administration, Resources, Supervision, Validation, Visualization, Writing – original draft, Writing – review and editing.
Funding
The author(s) declare that financial support was received for the research and/or publication of this article. This project was made possible with financial support from CellNUA UK Ltd, who also own the BEEMAR product formula. Funders however had no role in the study performance, manuscript preparation or the decision to publish this report.
Acknowledgments
Authors are grateful to the animal care and technical staff of the Animal Experimentation, Electron Microscopy and Histopathology as well as Immunology Departments at NMIMR. The following reagents were obtained through BEI Resources, NIAID, NIH: Plasmodium berghei, Strain NK65, MRA-268, contributed by Victor Nussenzweig and Plasmodium falciparum, Strain 3D7, MRA-102, contributed by Daniel J. Carucci.
Conflict of interest
The authors declare that the research was conducted in the absence of any commercial or financial relationships that could be construed as a potential conflict of interest.
Generative AI statement
The author(s) declare that no Generative AI was used in the creation of this manuscript.
Publisher’s note
All claims expressed in this article are solely those of the authors and do not necessarily represent those of their affiliated organizations, or those of the publisher, the editors and the reviewers. Any product that may be evaluated in this article, or claim that may be made by its manufacturer, is not guaranteed or endorsed by the publisher.
Supplementary material
The Supplementary Material for this article can be found online at: https://www.frontiersin.org/articles/10.3389/fntpr.2025.1602899/full#supplementary-material
References
Adnani, N., Rajski, S. R., and Bugni, T. S. (2017). Symbiosis-inspired approaches to antibiotic discovery. Nat. Prod. Rep. 34 (7), 784–814. doi:10.1039/c7np00009j
Alvarez-Suarez, J. M., Gasparrini, M., Forbes-Hernández, T. Y., Mazzoni, L., and Giampieri, F. (2014). The composition and biological activity of honey: a focus on manuka honey. Foods 3 (3), 420–432. doi:10.3390/foods3030420
Bantie, L., Assefa, S., Teklehaimanot, T., and Engidawork, E. (2014). In vivo antimalarial activity of the crude leaf extract and solvent fractions of Croton macrostachyus Hocsht. (Euphorbiaceae) against Plasmodium berghei in mice. BMC Complement. Altern. Med. 14, 79. doi:10.1186/1472-6882-14-79
Chan, Q. W., Melathopoulos, A. P., Pernal, S. F., and Foster, L. J. (2009). The innate immune and systemic response in honey bees to a bacterial pathogen, Paenibacillus larvae. BMC Genomics 10, 387. doi:10.1186/1471-2164-10-387
Deharo, E., Bourdy, G., Quenevo, C., Muñoz, V., Ruiz, G., and Sauvain, M. (2001). A search for natural bioactive compounds in Bolivia through a multidisciplinary approach. Part V. Evaluation of the antimalarial activity of plants used by the Tacana Indians. J. Ethnopharmacol. 77 (1), 91–98. doi:10.1016/s0378-8741(01)00270-7
Dumitru, C. D., Neacsu, I. A., Grumezescu, A. M., and Andronescu, E. (2022). Bee-derived products: chemical composition and applications in skin tissue engineering. Pharmaceutics 14 (4), 750. doi:10.3390/pharmaceutics14040750
Ferraboschi, P., Ciceri, S., and Grisenti, P. (2021). Applications of lysozyme, an innate immune defense factor, as an alternative antibiotic. Antibiot. (Basel) 10 (12), 1534. doi:10.3390/antibiotics10121534
Geahchan, S., Ehrlich, H., and Rahman, M. A. (2021). The anti-viral applications of marine resources for COVID-19 treatment: an overview. Mar. Drugs 19 (8), 409. doi:10.3390/md19080409
Green, M. R., and Sambrook, J. (2020). Alkaline phosphatase. Cold Spring Harb. Protoc. 2020 (8), 100768. doi:10.1101/pdb.top100768
Kajla, M. K., Shi, L., Li, B., Luckhart, S., Li, J., and Paskewitz, S. M. (2011). A new role for an old antimicrobial: lysozyme c-1 can function to protect malaria parasites in Anopheles mosquitoes. PLoS One 6 (5), e19649. doi:10.1371/journal.pone.0019649
Kalra, B. S., Chawla, S., Gupta, P., and Valecha, N. (2006). Screening of antimalarial drugs: an overview. Indian J. Pharmacol. 38 (1), 5. doi:10.4103/0253-7613.19846
Kharchoufa, L., Bouhrim, M., Bencheikh, N., El Assri, S., Amirou, A., Yamani, A., et al. (2020). Acute and subacute toxicity studies of the aqueous extract from Haloxylon scoparium pomel (Hammada scoparia (pomel)) by oral administration in rodents. BioMed Res. Int. 2020, e4020647. doi:10.1155/2020/4020647
Kifle, Z. D., Adinew, G. M., Mengistie, M. G., Gurmu, A. E., Enyew, E. F., Goshu, B. T., et al. (2020). Evaluation of antimalarial activity of methanolic root extract of myrica salicifolia A rich (myricaceae) against Plasmodium berghei-infected mice. J. Evid. Based Integr. Med. 25, 2515690X2092053. doi:10.1177/2515690x20920539
Kocot, J., Kiełczykowska, M., Luchowska-Kocot, D., Kurzepa, J., and Musik, I. (2018). Antioxidant potential of propolis, bee pollen, and royal jelly: possible medical application. Oxid. Med. Cell Longev. 2018, 7074209. doi:10.1155/2018/7074209
Kunat-Budzyńska, M., Rysiak, A., Wiater, A., Grąz, M., Andrejko, M., Budzyński, M., et al. (2023). Chemical composition and antimicrobial activity of new honey varietals. Int. J. Environ. Res. Public Health 20 (3), 2458. doi:10.3390/ijerph20032458
Liu, Q., Jing, W., Kang, L., Liu, J., and Liu, M. (2021). Trends of the global, regional and national incidence of malaria in 204 countries from 1990 to 2019 and implications for malaria prevention. J. Travel Med. 28 (5), taab046. doi:10.1093/jtm/taab046
Loukas, P., and Maria, T. (2023). The Application of Honeybee Products in the Health Sector. The Application of Honeybee Products in the Health Sector 13. doi:10.4236/abc.2023.131001
Lowe, D., Sanvictores, T., Zubair, M., and John, S. (2023). “Alkaline phosphatase,” in StatPearls (Treasure Island (FL): StatPearls Publishing). Available online at: http://www.ncbi.nlm.nih.gov/books/NBK459201/.
Lyu, H. N., Ma, N., Meng, Y., Zhang, X., Wong, Y. K., Xu, C., et al. (2021). Study towards improving artemisinin-based combination therapies. Nat. Prod. Rep. 38 (7), 1243–1250. doi:10.1039/d0np00079e
Mahama, A., Chama, M. A., Oppong Bekoe, E., Asare, G. A., Obeng-Kyeremeh, R., Amoah, D., et al. (2022). Assessment of toxicity and anti-plasmodial activities of chloroform fractions of Carapa procera and Alchornea cordifolia in murine models. Front. Pharmacol. 13, 1077380. doi:10.3389/fphar.2022.1077380Available online at: https://www.frontiersin.org/journals/pharmacology/articles/10.3389/fphar.2022.1077380/full.
Mamede, L., Ledoux, A., Jansen, O., and Frédérich, M. (2020). Natural phenolic compounds and derivatives as potential antimalarial agents. Planta Med. 86 (9), 585–618. doi:10.1055/a-1148-9000
Mensah M, L. K., Komlaga, G., Forkuo A, D., Firempong, C., Anning A, K., Dickson, A., et al. (2019). in Safety implications of herbal medicines used in Africa. Editor P. F. Builders Available online at: https://www.intechopen.com/books/herbal-medicine/toxicity-and-safety-implications-of-herbal-medicines-used-in-africa.
Mohd, N. S., Ismail, A. F., Tuan Ismail, T. S., Wan Abdul Rahman, W. F., Wan, A. W. A. N., Tengku Din, TADAA, et al. (2024). Hepatic and renal effects of oral stingless bee honey in a streptozotocin-induced diabetic rat model. World J. Exp. Med. 14 (1), 91271. doi:10.5493/wjem.v14.i1.91271
Obeidat, M., Haddad, M. A., and Ghnamat, S. A. (2024). Antimicrobial activities of seasonally collected bee products: honey, propolis, royal jelly, venom, and mellitin. Braz. J. Biol. (84), e286731. doi:10.1590/1519-6984.286731
OECD (2008). Test No. 407: repeated dose 28-day oral toxicity study in rodents. Paris: Organisation for Economic Co-operation and Development. Available online at: https://www.oecd-ilibrary.org/environment/test-no-407-repeated-dose-28-day-oral-toxicity-study-in-rodents_9789264070684-en.
OECD (2022). Test No. 425: acute oral toxicity: up-and-down procedure. Paris: Organisation for Economic Co-operation and Development. Available online at: https://www.oecd-ilibrary.org/environment/test-no-425-acute-oral-toxicity-up-and-down-procedure_9789264071049-en.
Pharmaceutical Prospects of bee products (2025). special focus on anticancer, antibacterial, antiviral, and antiparasitic properties - ProQuest. Available online at: https://www.proquest.com/openview/66a3ab815171db8c994e9814f3018ed2/1?pq-origsite=gscholar&cbl=2032437
Plasma Marino (2025). Available online at: https://www.ibizaformenteramarineplasma.com/
Ryley, J. F., and Peters, W. (1970). The antimalarial activity of some quinolone esters. Ann. Trop. Med. Parasitol. 64 (2), 209–222. doi:10.1080/00034983.1970.11686683
Santorelli, L. A., Wilkinson, T., Abdulmalik, R., Rai, Y., Creevey, C. J., Huws, S., et al. (2023). Beehives possess their own distinct microbiomes. Environ. Microbiome 18 (1), 1. doi:10.1186/s40793-023-00460-6
Seabrooks, L., and Hu, L. (2017). Insects: an underrepresented resource for the discovery of biologically active natural products. Acta Pharm. Sin. B 7 (4), 409–426. doi:10.1016/j.apsb.2017.05.001
Tarkang, P. A., Okalebo, F. A., Ayong, L. S., Agbor, G. A., and Guantai, A. N. (2014). Anti-malarial activity of a polyherbal product (Nefang) during early and established Plasmodium infection in rodent models. Malar. J. 13, 456. doi:10.1186/1475-2875-13-456
Uzor, P. F., Onyishi, C. K., Omaliko, A. P., Nworgu, S. A., Ugwu, O. H., and Nwodo, N. J. (2021). Study of the antimalarial activity of the leaf extracts and fractions of Persea americana and Dacryodes edulis and their HPLC analysis. Evidence-Based Complementary Altern. Med. 2021, 1–11. doi:10.1155/2021/5218294
World malaria report (2022). Available online at: https://www.who.int/teams/global-malaria-programme/reports/world-malaria-report-2022
Keywords: anti-plasmodial, Plasmodium falciparum, Plasmodium berghei, BEEMAR, malaria, acute toxicity, subacute toxicity
Citation: Adjei S, Obeng-Kyeremeh R, Ackah I, Agbemelo-Tsomafo C, Amoah D, Obeng SA, Asamoah F, Damanka S, Danquah KO, Ntiri MP, Honyo ME, Erskine IJ, Kojo Acquah F, Amoah LE and Kusi KA (2025) Anti-plasmodial and toxicological effects of BEEMAR - a natural formulation of harnessed honeycomb bioactive compounds suspended in enhanced marine plasma. Front. Nat. Prod. 4:1602899. doi: 10.3389/fntpr.2025.1602899
Received: 30 March 2025; Accepted: 24 April 2025;
Published: 27 May 2025.
Edited by:
Abdel Nasser B. Singab, Ain Shams University, EgyptReviewed by:
Mariana Guadalupe Vallejo, Universidad Nacional de Córdoba, ArgentinaHimanshi Tanwar, University of Maryland, United States
Copyright © 2025 Adjei, Obeng-Kyeremeh, Ackah, Agbemelo-Tsomafo, Amoah, Obeng, Asamoah, Damanka, Danquah, Ntiri, Honyo, Erskine, Kojo Acquah, Amoah and Kusi. This is an open-access article distributed under the terms of the Creative Commons Attribution License (CC BY). The use, distribution or reproduction in other forums is permitted, provided the original author(s) and the copyright owner(s) are credited and that the original publication in this journal is cited, in accordance with accepted academic practice. No use, distribution or reproduction is permitted which does not comply with these terms.
*Correspondence: Kwadwo Asamoah Kusi, YWt1c2lAbm9ndWNoaS51Zy5lZHUuZ2g=