- 1Department of Pharmaceutics and Pharmaceutical Technology, L.M. College of Pharmacy, Ahmedabad, India
- 2Pharmacy Section, L.M. College of Pharmacy, Ahmedabad, India
- 3Department of Pharmaceutical Science, College of Pharmacy and Health Sciences, St. John’s University, New York, NY, United States
- 4Department of Preventive Medicine,Shantou University Medical College, Shantou, China
- 5Department of Endocrine and Metabolic Diseases, Longhu Hospital, The First Afliated Hospital of Shantou University Medical College, Shantou, China
Cancer is a prominent cause of mortality globally, and it becomes fatal and incurable if it is delayed in diagnosis. Chemotherapy is a type of treatment that is used to eliminate, diminish, or restrict tumor progression. Chemotherapeutic medicines are available in various formulations. Some tumors require just one type of chemotherapy medication, while others may require a combination of surgery and/or radiotherapy. Treatments might last from a few minutes to many hours to several days. Each medication has potential adverse effects associated with it. Researchers have recently become interested in the use of natural bioactive compounds in anticancer therapy. Some phytochemicals have effects on cellular processes and signaling pathways with potential antitumor properties. Beneficial anticancer effects of phytochemicals were observed in both in vivo and in vitro investigations. Encapsulating natural bioactive compounds in different drug delivery methods may improve their anticancer efficacy. Greater in vivo stability and bioavailability, as well as a reduction in undesirable effects and an enhancement in target-specific activity, will increase the effectiveness of bioactive compounds. This review work focuses on a novel drug delivery system that entraps natural bioactive substances. It also provides an idea of the bioavailability of phytochemicals, challenges and limitations of standard cancer therapy. It also encompasses recent patents on nanoparticle formulations containing a natural anti-cancer molecule.
Highlights
● Traditional medicines hold a significant role in many healthcare systems around the world.
● According to a World Health Organization (WHO) assessment, conventional medicine meets and/or supplements the fundamental health needs of around 80% of the community in underdeveloped nations.
● Plant-derived anticancer medicines are highly sought after because they are effective inhibitors of cancer cells.
● Although the development of nano drugs is fraught with uncertainty, and the development of potent bioactive chemicals from natural origin is not a popular alternative today, improving the effectiveness of known natural bioactive substances using nanotechnology has become a typical aspect.
● Different drug delivery systems entrapping natural bioactive compounds for cancer treatment are summarized.
● Application of Nano drug-delivery systems for natural anticancer agents can improve bioavailability, biodistribution, therapeutic activity, drug targetting, and stability.
Introduction
Cancer is an abnormal and autonomous cell proliferation caused by a lack of replication control (1, 2). Delayed assessment and non-responsive treatments are the leading reasons for elevated death rates in cancer patients. Knowledge of the principles underlying tumor biology has resulted in substantial advances in cancer control, diagnosis, and therapy in recent years. Surgery, immunotherapy, radiation therapy, chemotherapy, and targeted hormone medications are all traditional ways of cancer management (3). These strategies are often restricted due to their inadequate specificity, since they might also influence healthy cells and the host immune system, resulting in undesirable adverse effects. Furthermore, except for surgery, all medicines employed in cancer management can generate drug resistance in tumor cells. Therefore, anticancer medication research continues for new and better ways to treat tumors. Medication tolerance remains a major issue in cancer care (3). When there is a poor or no reaction to anticancer medication at the start or throughout therapy, resistance might be innate or inherited. Multidrug resistance (MDR) occurs when a patient develops resistance to one treatment and becomes tolerant to additional unrelated drugs (4). Tumors are made of two fundamental components, replicating neoplastic cells and supporting stroma of connective tissues with blood vessels. Mixed tumors, tumors of parenchyma cells, mesenchymal tumors, and tumors of more than one germ cell layer are all different forms of cancer (5, 6). Exogenous carcinogens might be physical, chemical, or biological (7). Biological carcinogens comprise viruses, bacteria, and parasites. Endogenous chemicals of anabolic and catabolic processes, and cells carrying a dormant virus, are the foundation of carcinogenesis (8). Prevention of carcinogen production, reduction of their activation, and enhancement of their elimination can aid in the prevention of cancers (9). Cancer risk factors in humans involve alcohol use, cigarette use, nutritional inadequacy, insufficient physical activity, pollution, and noncommunicable diseases. Chronic diseases including H. pylori, hepatitis B, hepatitis C, human papillomavirus, and Epstein-Barr virus are also considered risk factors for cancer (10). Diagnosis of cancer is carried out by tissue sample analysis, blood test, Computed Tomography (CT) scan, endoscopy, and many more such methods. Radiotherapy, chemotherapy, and surgery are the most common cancer treatments; however, novel technologies are also underway (11). Most radiation therapy is used for the treatment of glioblastoma, breast cancer, cervical cancer, laryngeal cancer, and others. Radiation includes high-energy ionizing radiation like X-ray and gamma rays which target the tumor cells and damage DNA directly or through the generation of free radicals (12).
Chemotherapy is a term that refers to a medication that involves the use of synthetic chemicals. Anticancer drugs can be used alone or in combination to damage cancer cells or prevent the growth of a tumor. The main issue with chemotherapy is the side effects associated with anticancer drugs. Side effects result from a lack of specificity towards tumor-causing cells. Anticancer drugs can also affect our normal cells, which may lead to side effects (13, 14). Vinca alkaloids, taxanes, epipodophyllotoxins, camptothecins, genistein, and quercetin or rutin are some plant products associated with cancer treatment. Such herbal medications have a lower index of side effects than allopathic medications that are effective in cancer treatment. Some hormonal treatments are also available for the treatment of tumors. Hormones such as glucocorticoids, estrogen, progesterone, and gonadotropin-releasing hormone (GRH) analogs are effective against some cancers. Radioactive isotopes also have a place in chemotherapy, for example, radioactive iodine 131I is used to treat thyroid cancer (15). Recent developments of plant-based medicines and research associated with them describe that herbal medications for cancer treatment may replace chemotherapy and can be more effective against tumors (16).
The Role of Ayurveda in Cancer Therapy
Ayurveda is one of the ancient medicinal plant systems (6000 years old) utilized in the current time for curing or suppressing numerous cancers using natural drugs and their extracts (17, 18). The objective of Ayurveda therapy in cancer treatment is to supplement the mind’s self-healing powers. It aids in the diagnosis of cancers and provides information on herbs and alternative treatments (19). Cancer-like diseases not only affect physical health but also affect mental health. Discordances between the mind and the body cause a variety of symptoms such as lethargy, anxiety, restlessness, and depression (20). When cancer strikes, it causes disorders in the body and affects the Tamas (it is the energy that holds all things together over time) and the Kapha. Ayurveda offers a significant psychotherapy technique that has been utilized and is currently being implemented in various nations. Plants are an important resource for medication. Plants like vinca, shattering, guduchi, triphala, and tulsi have been utilized as anticancer agents (21). Ayurveda’s goal in treating cancer is to provide preventive, curative, therapeutic, and prophylactic care. Cachexia is a tumor-induced metabolic alteration that triggers an immune response, for which several ayurvedic herbs are used. Ayurvedic medications may not only promote full recovery but also minimize adverse effects in cancer therapy (20). The first goal of Ayurvedic cancer therapy is to regulate and manage Tridosha [Vata (wind), Pitta (bile), and Kapha (phlegm), corresponding to the three elements of the universe: air, fire, and water] and Triguna (Sattva, Rajas, and Tamas - integral components of the mind). As per Charaka and Sushruta Samhitas, cancer can be classified as Arbuda (major neoplasm), and Granthi cancers (minor neoplasm) which fall under group 1 cancer as per Ayurveda that includes Raktarbuda (leukemia), Mamsarbuda (melanoma), and Mukharbuda (oral tumor) (22, 23). Group 2 cancer comprises tumors that are Tridosaj Gulmas (tumor of stomach and liver). Group 3 diseases include Asadhya Kamala (jaundice) and Nadi Varna (sinusitis) (22, 24).
Triguna has an important role in health research fields which contains guna (energy), sattva (quality of reality and purity), rajas (enthusiasm), and tamas (quality of dullness) (25). Triguna is available to everyone and variations in that indicate the health status and nature of a person (26). Ayurvedic treatment of cancer (Figure 1) involves i) cleaning and removal of doshas (Sodhna Chikitsa using Panchkarma), ii) serenity of doshas (Somana Chikitsa), iii) rectification of defects (Dhatwangi Chikitsa), iv) immunotherapy (Rasayana Proyaga), v) antineoplastic drugs (Vyadhipratyanika Chikitsa), vi) symptomatic therapy (Lakshanika Chikitsa), vii) surgery (Sastra Chikitsa), viii) maintenance of patient health (Prakritisthapani Chikitsa), ix) restoring the body from disease condition (Rognashini Chikitsa), and x) spiritual treatment (Naishthiki Chikitsa) (26, 27). The general ayurvedic treatment includes maintenance of healthy lifestyle, maintenance of fit digestive power, removal of toxins from the body by Panchkarma (method of cleansing the body of all the unwanted waste after lubricating it), revivification by use of Rasayana. Ayurvedic treatment can also be used along with chemotherapy or radiotherapy (28).
Herbal Plants Exhibiting Anticancer Activities
Human bodies are made of millions of cells, each of which is a self-contained living organism in and of itself. Ordinary body cells proliferate and replicate for a short amount of time before ceasing to do so. Afterward, they only proliferate when they need to rebuild damaged or dead cells. When this cellular replication mechanism becomes uncontrollable, a tumor develops. Cancer cells’ aberrant expansion and division are triggered by DNA destruction in these cells (20).
Traditional medicines hold a significant role in many healthcare systems around the world. Plants’ medical and economic advantages are being more widely recognized and developed in both developing and developed countries. A plant or plant component utilized for its aroma, flavor, and/or medicinal characteristics is known as the herb. Herbal medications and phytomedicines are all terms for products manufactured from herbs that are used to preserve or promote wellbeing (29, 30). Plant-based medications make up around a quarter of the current Indian pharmacopeia, according to estimates. Conventional medicinal herbs are organically existing plant-derived drugs that have been utilized to alleviate disease in local or regional healing traditions with little or no chemical modification (31). Preventive care, disease control, serious adverse effects associated with synthetic medicines, and inadequate therapeutic choices for critical diseases are some of the factors for using herbal pharmaceuticals (22, 30). Tibetan traditional medicine is still somewhat localized in their nation of origin, while others, like Ayurvedic and Chinese traditional medicines, are becoming more widely utilized across the world. Plants filled with chemicals that may have chemoprotective properties are undergoing clinical studies (32). Table 1 summarized and describes several plant species based on their common mechanism of action and anticancer therapeutic activities.
Bioavailability of Phytochemicals
Food-derived chemicals reach the circulatory system and are transported to certain tissues where they exert physiological properties. Nutritional phytochemicals are metabolized and carried by the gastrointestinal epithelium before entering the circulatory system (111). The complexity of the biological system include:
● Food material variety and human subject (digestion difference in infants, adults, and the elderly, even there is difference between female and male digestion)
● Complex interactions occur between food/chemicals throughout storage, processing, digestion, and absorption that potentially alter health benefits.
● The process pathways such as compound solubility, gut pH, gut microbiota metabolism, penetration through the intestinal wall, efficient efflux mechanism, and metabolic activities in the first pass may alter the bioavailability of phytochemicals (112, 113).
As per Meyskens and Szabo, the majority of studies recognize one nutrient as the potential cause while ignoring the impact of other phytonutrients or biological factors. As a consequence, in clinical investigations for a certain molecule, the entire diet must be considered (114). According to Lipinski’s rule of five, a substance will be more effective if it contains not more than (NMT) 5 hydrogen-bond donors, NMT 10 hydrogen-bond acceptors, a molecular mass of NMT 500 daltons, a partition coefficient (log P-value) of NMT 5, and NMT 10 rotatable bonds (115). The exceptions include polyphenols like curcumin and green tea. The lymphatic system, instead of the circulatory system, provides phenols with better bioavailability. Molecular forms of phenolics, including glycone or aglycone, can affect absorption that results in variations in bioavailability.
Through uptake and efflux channels on the epithelial cell surface, the intestinal epithelium tissue provides a significant impact on bioavailability. P-glycoprotein, breast cancer resistance protein, and multidrug resistance protein 2 are the primary chemopreventive drug transporters (116). The ATP-binding cassette transporter family has the transport proteins that help nutrients, medications, and metabolites get back into the intestinal lumen. Only nanomolar amounts were available in the blood because of migration (117).
Many medicines that were transferred from in vitro to in vivo studies were rejected because their findings were insufficient. The chemicals were discovered to be unstable in the stomach and to have low bioavailability, which might have contributed to the clinical failure. Higher dosages were used to overcome the low absorption, which resulted in toxicity to numerous organs despite effectiveness (118). Adverse responses were also seen in some trials, including the SELECT (Selenium and Vitamin E Cancer Prevention Trial) in prostate cancer, which was terminated owing to a negligible rise in prostate cancer incidences. A follow-up analysis of the individuals revealed a substantial increase in prostate cancer incidence in the high vitamin E group years later, even though they had stopped using the supplements (119).
As a result, assessing the bioavailability of drugs purely based on their physicochemical characteristics becomes challenging. Molecular metabolism changes the bioavailability of the compound. Chemicals that prevent the metabolism of other chemicals can enhance their bioavailability. Piperine (derived from black pepper) inhibits the glucuronidation (metabolism) of some chemicals such as tea polyphenol, epigallocatechin-3-gallate, and curcumin increasing their bioavailability (120). Piperine has also been expected to enhance the length of the intestinal microvilli and the fluidity of the intestinal brush border membrane with an enlarged absorptive surface in the small intestine (121). Quercetin and myricetin can prevent resveratrol sulfation and glucuronidation, resulting in increased resveratrol bioavailability (122, 123).
Drug Delivery Systems Entrapping Natural Bioactive Compounds for Cancer
Multiple novel drug delivery systems (NDDS) have been established during the last two decades, with the primary goal of improving medication bioavailability, preventing adverse impacts, and preventing drug degradation (124). A drug delivery system is based on the idea that pharmaceuticals should be given directly to the region of activity by the demand of the body, and another delivery system is routed via drug delivery into the site of action (125). Additional benefits of NDDS include greater solubility, improved bioavailability, less undesirable side effects, boosted therapeutic action, better stability, and better drug distribution. It also involves the regulation of pharmacokinetics, pharmacodynamics, and immunogenicity (126).
The benefit of NDDS is for more patient convenience in drug administration (126–128). Drugs are released from the drug delivery systems via two mechanisms, passive and active targeting. One instance of passive targeting is the preservation of chemotherapeutic drugs in solid tumors, which results in enhanced tumor vascular permeability. Active targeting which includes specific receptors on the surface of the cell and ligand-receptor interactions, is extremely specific for interaction (20, 129).
Through this review article, we tried to thoroughly evaluate recent statistics on the effectiveness of herbal medicines in cancer with nano-formulations. We have started searching through major databases such as Web of Science, PubMed, Scopus, Elsevier, Springer, and Google Scholar with keywords like “herbs for cancer’ “Nanoherbal formulations for cancer”, “Anticancer Phytochemicals” and then we started with a specific search. From the identified components, first, we have omitted the components for which no strong literature support is available. From the remaining components, we have considered the compounds based on the in vivo data evaluation and searched for specific research around anticancer phytochemicals. Later on, from the highly researched components against anticancer phytochemicals, a clinical trial database is evaluated. We have incorporated clinical trial outcomes for the majority of the components if not then in certain cases a cogent discussion on in vivo studies is incorporated. Very recent published data is considered for the review of anticancer herbal nanoformulations. NDDS of various sorts are made up of various ingredients, and these drug delivery systems are used for drug administration in the body as well as with certain additional pharmacokinetics and pharmacodynamics properties. Table 2 illustrates the various drug delivery systems for herbal anti-cancer substances while Figure 2 summarizes the various nanotechnology-based drug delivery platforms for the delivery of anticancer phytochemicals.
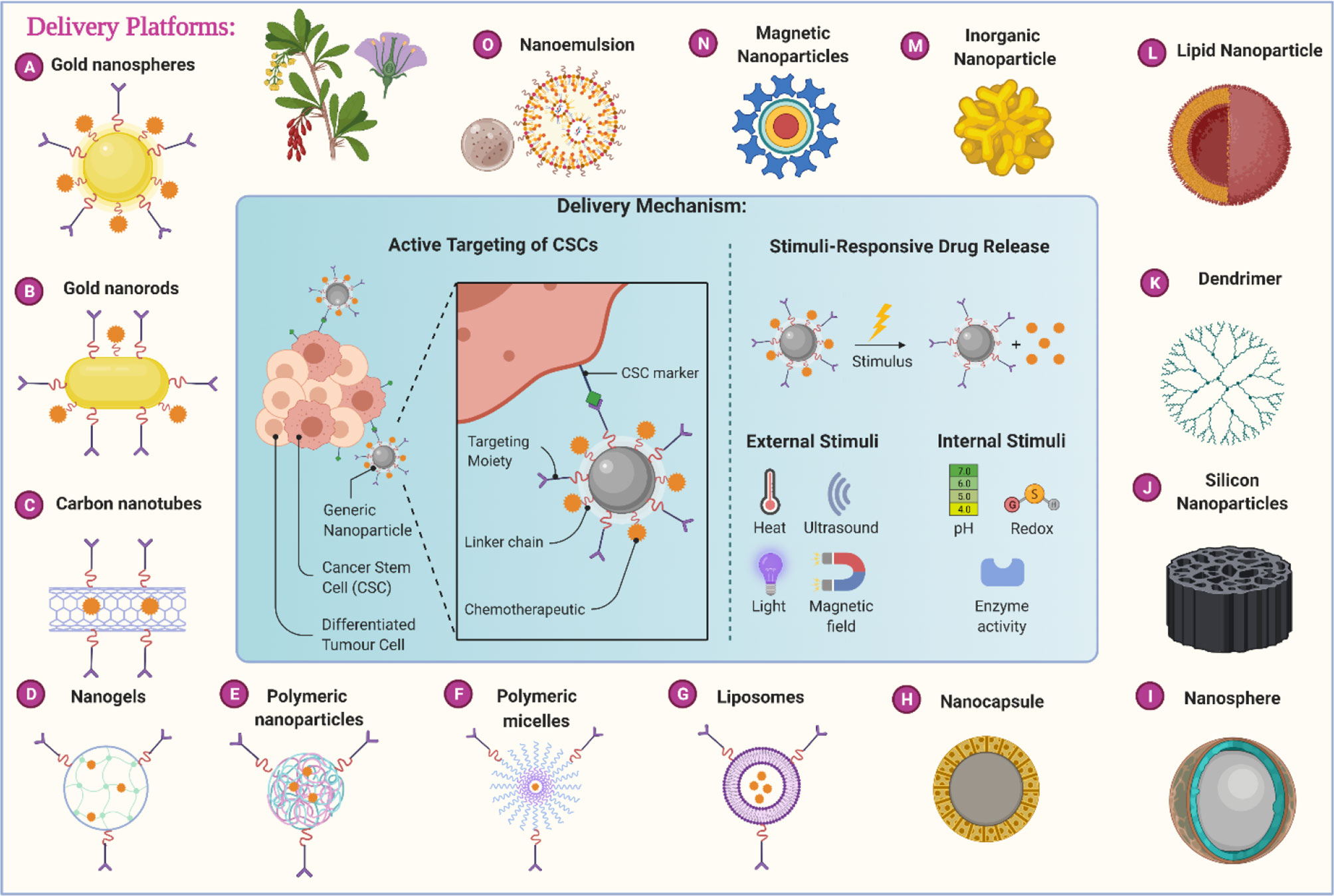
Figure 2 Different nanotechnology-based carrier platforms for the efficient delivery of anticancer phytochemicals and different delivery mechanisms.
The drug delivery systems listed in Table 2 are extensively and separately discussed in the following section of this review.
Biopolymer-Based Nanocarrier (BBN)
Biopolymers are macromolecules that are divided into three classes: polysaccharides (chitosan, dextran, cyclodextrins, pectin, hyaluronan, guar gum, sodium alginate, cellulose, and starch), proteins (gelatin, albumin, and milk proteins), and nucleic acids (161). To use BBN as a drug delivery vehicle, it is critical to control particle size, the charge on the particle, and specific surface area (161, 162). BBN can provide the high possibility of transporting bioactive compounds to the site of action, which has attracted more attention from chemists, biologists, and pharmaceutical scientists (163). The primary goal of BBN is to elevate the drug’s water solubility, stability, reduce destruction, improve bioavailability, bioactivity, biodegradability, lower toxicity, and flexibility of gel formation (164). Spray drying, electrospray, high–pressure homogenization, supercritical fluid, electrospinning, emulsion–diffusion, reverse micelle, and emulsion – droplet coalescence are just a few of the fabrication methods to engineer BBN (165).
Biopolymer-based biomaterials were studied for use in accelerated diabetic wound healing. Cashew gum (CG) based bio nanocomposite was used as a platform for trials. Crude samples of cashew gum exudate were collected from Anacardium occidentale L. for the trial, cashew gum-coated prussian blue nanoparticles (PBNPs) were prepared in situ and characterized. CG coating decreased the size of the spherical PBNPs from 50 to 5 nm and the crystallinity of PBNP’s core was increased. The use of CG polysaccharides was found to be an excellent and effective strategy for covering and controlling the size, shape, and crystallinity of prussian blue via an in-situ synthesis (166). Sebak et al. created noscapine-loaded nanoparticles (NPs) with human serum albumin (HSA) for targeted administration and tested them on SK-BR-3 breast cancer cells using the pH coacervation technique. The results showed that noscapine NPs were substantially more effective than free noscapine in lowering SK-BR-3 cell viability (167). Cationic chitosan (CS)- and anionic sodium alginate (Alg)-coated PLGA NPs loaded with revasterol have been developed by the nanoprecipitation technique (168). For six months, the nanoparticles can inhibit trans isoform breakdown and revasterol escape from the carrier.
Liposomes
Liposomes are formed from combining cholesterol with phospholipids (128). They shield the enclosed medication from enzyme and other inhibitor activity. They can transport any medication via endocytosis and are hence commonly employed for compounds that cannot pass biological membranes. Their disadvantages involve instability, identification by the immune system, and phagocytosis. Conjugation with polyethylene glycol (PEGylation) can shield them from phagocytosis, making them particularly efficient in carrying anticancer medicines (169). Using a thin-film hydration and extrusion method, Lu et al. prepared folic acid conjugated PEGylated liposomes of vincristine for multidrug-resistant cancer therapy and evaluated their cytotoxicity on KBv200 carcinoma cell line and also performed in vivo antitumor efficacy studies (tumor growth inhibition and apoptosis assessment studies by TUNEL) (170). The IC50 of the PEGylated folic acid-linked vincristine liposomes was 23.99 nM, compared to 1.10 nM for free vincristine and 363.08 nM for PEG-LS/vincristine. In vivo tests revealed that folic acid conjugation greatly improved the antitumor activity of the PEGylated liposomes of vincristine, as well as a higher apoptotic index was captured in the TUNEL assay (24.1 vs. 14.4). In another research study, Liposomal ursolic acid showed improved anticancer activity for breast cancer and prostate cancer cell lines than free ursolic acid (171). Recently, a cationic liposome encapsulating hydroxycamptothecin and 5-aminolevulinic acid was administered along with chemo-sonodynamic therapy against metastatic lung cancer that has demonstrated a better apoptotic behavior (172). Moreover, a phase-1 study with 96 non-randomized participants was carried out to assess the effect of liposomal topotecan intravenous injection for the treatment of advanced solid lung tumors. Topotecan is the semi-synthetic derivative of the naturally obtained topoisomerase inhibitor, camptothecin, and the results are yet to be disclosed but the trial is reported to enter phase-2 study by the beginning of the year 2023 (NCT04047251). Inhalable liposomes are being developed and their preparation is studied for use in pulmonary diseases (173). Daunorubicin and cytarabine (vyxeos®) were encapsulated in liposomes (Vyxeos®, CPX‐351) for the treatment of adults with newly diagnosed, therapy‐related acute myeloid leukemia or acute myeloid leukemia with myelodysplasia related changes (NCT01696084). Vyxeos® is an orphan drug and its clinical trial proved to be remarkable. A clinical study CLTR0310‐301 (also referred to as Study 301) was a phase III, multicenter, open‐label, randomized, trial of vyxeos® (daunorubicin‐cytarabine) liposomal injection versus standard 3 + 7 daunorubicin and cytarabine in patients aged 60–75 years with untreated high‐risk (secondary) (173, 174). A recent clinical trial is investigating the efficacy and safety of paclitaxel liposome as first-line therapy in patients with advanced pancreatic cancer. The patients were given paclitaxel liposome 175 mg/m2 intravenously every 3 weeks for 3 weeks until the disease recurrence. All five parameters, including overall response rate, overall survival, disease control rate, quality of life, and adverse events are examined (NCT04217096). Moreover, an open-label, phase-1 study on the use of liposomal irinotecan injection was carried out on 136 participants to study its effects on advanced breast cancer and evaluate its efficacy and safety (NCT04728035). Transfersomes are phosphatidylcholine-containing liposomes with an edge activator (175). Because of greater penetration, celecoxib loaded into transfersomes containing soy phosphatidylcholine combined with sodium deoxycholate was found to be a therapeutically efficient drug-delivery approach for the treatment of rheumatoid arthritis (176).
Niosomes
Niosomes are obtained through combining an alkyl or dialkyl polyglycerol-based nonionic surfactant and subsequent hydration in an aqueous environment. Because of their comparable construction, they are frequently employed as a replacement for liposomes (132). They are not toxic and restrict the action of drugs to specific locations. Niosomes have also been shown to be manipulable as a part of advanced drug delivery strategies and can deliver drugs via intravenous, transdermal, ocular, and pulmonary routes. Cancer therapy, gene therapy, and targeting medicine via the nasal route are some of the other uses for niosome based drug delivery. It is also used in immunological applications, such as a hemoglobin transporter, and for cosmetic reasons (177). Morusin-loaded niosomes have been produced for cancer therapy (178). Nanomorusin, unlike free morusin, was shown to be easily dispersible in aqueous environments. Morusin was shown to have an extraordinarily high drug entrapment efficiency (97%), regulated and prolonged release, and increased therapeutic effectiveness in cancer cell lines from four distinct lineages. A different research study evaluated the anticancer activity of niosomes containing both curcumin and methotrexate against colorectal cancer cell lines (179). The niosomal formulation showed more in vitro cell toxicity than their combination in free form. The anti-arthritic efficiency of luteolin (LT)-loaded niosomes made with different nonionic surfactants was tested in vitro and in vivo, with improved entrapment efficacy and higher transdermal flow across the rat skinned discharge. The cytotoxicity study showed a lesser IC50 value from LT-NVs than the pure LT (180). Thus, it can be concluded that LT-NVs are a natural alternative to synthetic drug in the treatment of lung cancer. The therapeutic purpose of niosomes is to deliver anticancer drugs, protein, peptide, and natural product delivery with improved bioavailability. Numerous experts are now intrigued by the delivery of natural compounds such as curcumin, which has low solubility and bioavailability (177). As a result, clinical trials with the combination of Curcuma longa L. and doxorubicin are underway (NCT04996004). Aside from curcumin, there are natural compounds called morusin that have powerful antibacterial, anti-inflammatory, and anticancer properties. Rahman et al. developed a formulation in which morusin is loaded with niosomes as a carrier, removing the barrier of low solubility and stability to target the specific cell (126). A study to evaluate the efficacy and safety of niosomal docetaxel lipid suspension compared to taxotere® in triple-negative breast cancer patients (after failure to previous chemotherapy) was conducted (NCT03671044). In this randomized, open-label clinical trial, females 18-65 years of age were eligible to apply. The niosomal docetaxel lipid suspension was administered intravenously at three-week intervals at a dose of 75 mg/m2. Patients received drug doses until disease progression and unacceptable toxicity occurred. This procedure was repeated with a 100 mg/m2 dose of niosomal docetaxel lipid suspension. The trial’s primary goal is to compare the proportion of patients with the best overall response rate in niosomal docetaxel lipid suspension and taxotere® (NCT03671044).
Polymeric Micelles
Micelles are lipid molecules that organize themselves in a spherical shape in aqueous solutions. They fluctuate in size from 10 to 100 nm and are generally quite narrow in terms of the size distribution (181). They can improve drug absorption and retention by shielding the drug from inactivation by its micellar environment. This system is made up of a core-shell structure with a lipophilic core and a shell made up of hydrophilic polymer blocks (182). The physicochemical properties of the drug and their position in the micelle influence their release. Certain physical stimuli, such as pH, temperature, ultrasound, and light can also promote drug release from the micelles in the targeted location (183). Micelles also provide an increased drug accumulation at the tumor site. Recently, doxorubicin delivery through covalent attachment with the hydrophobic portions of an amphiphilic block copolymer resulted in pH-regulated drug release (184). Paclitaxel, doxorubicin, 5-fluorouracil, 9-nitrocamptothecin, cisplatin, triptorelin, dexamethasone, and xanthone were all microencapsulated in poly lactic-co-glycolic acid (PLGA), polymerized lactic acid (PLA), and polycaprolactone (PCL) nanostructures with a micellar backbone (185). The medications are released to the target. Paclitaxel, for example, is encapsulated in polymeric micelles, which improves the systemic pyruvate kinase (PK) profile and interferes with drug-producing adverse effects such as neurotoxicity. It is USFDA approved and the formulation is marketed as Genexol® PM (183). As a solution to breast cancer and better intracellular delivery of docetaxel, novel pH-triggered biocompatible polymeric micelles have been developed based on heparin–α-tocopherol conjugate. For this, the amphiphilic copolymer was synthesized by grafting α-tocopherol onto the heparin backbone by a pH-cleavable bond (186). The paclitaxel and cisplatin-loaded polymeric micelles in advance non-small cell lung cancer were evaluated as a comparative phase 2 study with 276 participants. The primary endpoint of this study is a response rate of up to 6 cycles to ensure that the overall anticancer response is achieved. The secondary outcome measure is overall survival over three years (NCT01023347). The safety results are satisfactory (data not available in the public domain) and they have started phase 3 studies (NCT02667743).
Magnetic Microspheres
Magnetic microspheres are made up of microscopic supramolecular particles that are surrounded by capillaries. The primary goal of magnetic microspheres is to deliver drugs to the specific site of action that ultimately reduces the adverse effects. Magnetic microspheres are composed of tiny particles such as protein, silica, hydroxylapatite, magnetite (Fe3O4), maghemite (gamma Fe2O3), and synthetic polymers (143). The drug delivery is divided into two parts: spatial placement and temporal delivery of the drug (143). Magnetic microspheres cause pharmaceuticals to be released in a sustained manner rather than having a high bioavailability. Examples of magnetic microspheres-containing products are lupron depot® and nutropin depot® (187). Solvent evaporation, multiple emulsion techniques, phase separation emulsion polymerization, emulsion solvent extraction method, hot melt microencapsulation, and dispersion copolymerization are some methods for producing magnetic microspheres (183). It is used for the treatment of alveolar echinococcosis, a human helminthic disease caused by the larvae of Echinococcus multilocularis tapeworms. Treatment of this disease uses iron oxide magnetic particles. Spray-drying was used to create magnetic microspheres, which were then studied for their physicochemical features and dissolution profile. They were also tested for therapeutic effectiveness in both in vitro and in vivo systems. In vitro experiments in B16 melanoma cells found that using 30 M or 50 M sulforaphane with iron oxide in the polymeric carrier inhibited cell survival by around 13% -16%. In vivo data from C57BL/6 mice demonstrated that magnetic microspheres (located to the tumor site with the assistance of a powerful magnet) prevented 18% more tumor development than sulforaphane in solution (188). The magnetic microsphere of curcumin and doxorubicin demonstrated potential in vitro anticancer activity in cell viability and MTT cytotoxicity studies (189).
Nanoemulsion
Nanoemulsions (NE) is a colloidal dispersion system, and one of their most essential functions is to act as a tool for increasing the bioavailability of poorly water-soluble medications (147, 148). They are composed of oil, water, amphiphile, phospholipid, alkyl polyglycosides, PEGylated fatty acid ester, and fatty alcohol. They are having mean droplet sizes less than 100 nm. The NE system has prolonged drug release, high solubility, higher skin permeability, more drug absorption, extremely low viscosity, less allergic response, and less drug degradation (190). Oral administration of the medication enhances bioavailability. The hydrophilic substances have higher permeability when administered subcutaneously or intramuscularly, and they are condensed into the lymphatic system (145). Over the last few years, NE has created novel systems such as Transcuto® P, alkyl polyglycosides, etc. Natural herbal medications like rutin, genistein, brucea javanica oil, and coixenolide have been grated into NE for some applications. The antioxidant properties of Syagrus romanzoffiana (Cham.)Glassman, fruit O/W (oil in water) NE are assessed using the phase inversion technique (190). Paclitaxel nanoemulsion containing tocopherol as oil phase has been developed and evaluated for targeted cancer therapy that reached phase 3 clinical trials (NCT01620190) (191). The effects of eugenol nanoemulsion on pain were studied via a randomized double-blinded controlled cross-over trial (158). For the phonophoretic application of glucosamine and chondroitin in the treatment of knee chondropathies, nanoemulsion (nano CG) has been made and evaluated. Nano CG was previously applied in randomized and controlled clinical trials (192). In this experiment, patients in arm 1 receive nanoemulsion curcumin orally twice daily for up to 3 months. In arm 2, a placebo was administered orally twice daily for three months. This trial’s result was measured using the functional evaluation of cancer therapy (endocrine system inhibitor) to detect changes in aromatase inhibitor. This experiment is currently ongoing, however, it is not currently recruiting participants (NCT03865992). Plumbagin improves the efficacy of androgen deprivation therapy (ADT) in prostate cancer and it is now being evaluated in phase I clinical trial (193). A recent study found that plumbagin decreased PTEN-P2 driven tumor development only in castrated mice but not in intact animals, indicating that dihydrotestosterone (DHT) was generated in the testes and was primarily responsible for inhibiting prostate cell death (194). An oleic-acid-based nanoemulsion of plumbagin increases its antitumor efficacy (193). Nanoemulsion formulation of piplartine resulted in enhanced solubility, oral bioavailability, and anti-tumor efficacy of piplartine (195).
Nanoparticles (NPs)
NPs are constructed of a range of materials, some of which are poisonous. Biodegradable materials are less harmful. NP systems are being investigated for a wide range of biological applications. NP protects the drug from enzymatic breakdown and allows for regulated drug release (196). Through adhesion to the capillary wall, NPs may improve the oral bioavailability of poorly soluble medicines and tissue absorption following parenteral administration. They may also improve drug transport across membranes. They are vulnerable to phagocytosis and endocytosis because of their hydrophobic surface, which is quickly covered with plasma proteins and taken up through the mononuclear phagocytic system (MPS) present in organs like the liver, spleen, and bone marrow (169).
Applying the ionic gelation process, berberine-loaded NPs were synthesized for anticancer potential (197). The rotary evaporated film ultrasonication process was used to create glycyrrhizic acid-loaded NPs (198). For testing therapeutic synergy in the ulcerative colitis (UC) model, pH-sensitive NPs of the curcumin-celecoxib combination were optimized. Its effectiveness was later validated in a UC model in rats, as per the tests (199). Gold NPs (AuNP) were created using a greener method that included digested Acorus calamus L. rhizome as a reductant and chloroauric acid as a starting material. The existence of a surface plasmon resonance (SPR) peak in the ultraviolet (UV)–visible spectral analysis revealed the formation of AuNP (200). Hexagonal antibacterial Zn0.95Ag0.05O (ZnAgO) NPs have been made using rosemary leaf extracts as a green chemistry method whose formation was confirmed by X-Ray Diffraction (XRD), Fourier transform infrared spectroscopy (FTIR), and UV–visible spectra (201). Clinical trials on magnetic fluid hyperthermia based on magnetic NPs were conducted and studied (NCT01411904). Nanoparticle role in photodynamic therapy for solid tumors has been studied via a clinical trial. The aim of the use of nanotechnology-based approaches as delivery tools for photosensitizer (PS) is to study the improvement in their cancer cellular uptake and their toxic properties, as well as the photodynamic therapy’s therapeutic impact (202). Potential anticancer activity is reported for apigenin-loaded PLGA nanoparticles against skin tumor in mice models (203). These nanoparticles showed ameliorative potentials in combating skin cancer and therefore have a greater prospect of use in the therapeutic management of skin cancer. Similarly, significant anticancer activity was reported against osteosarcoma cancer cells (MG63 and Saos-2 osteosarcoma cell lines) by formulating PLGA nanoparticles of etoposide and paclitaxel (204). The results of quercetin-loaded PLGA nanoparticle administration showed potential action against C6 glioma cells (205). It has demonstrated antioxidant properties and cellular oxidative resistance. The results showed that the improved batch (Qu1NPs) exhibited better cellular absorption at a lower IC50 value (29.9 gmL-1) after 48 hours of incubation. The NPs may lessen resistance in brain cancers by lowering oxidative stress. A nano-phytocomposite consisting of phytochemical extract (BRM270) has cytotoxic potential against HepG2 human hepatoma cancer cells (206). A randomized, double-blind, placebo-controlled phase 2/3 study is now underway to assess the effectiveness of berberine hydrochloride against the development of new colorectal adenomas in 1,000 people who have a history of colorectal cancer (NCT03281096). A phase-2 trial is investigating the adverse effects of paclitaxel albumin-stabilized nanoparticle formulation for treating diseases such as fallopian tube cancer, primary peritoneal carcinoma, and recurrent ovarian carcinoma. The major goal of the experiment is to assess tumor response after each cycle for the first six months, as well as the frequency and severity of side effects seen. This research is open to people of all ages, however, only women are permitted (NCT00499252).
Lipid-Based Nanoparticles (Solid Lipid Nanoparticles - SLN)
PEGylated surfactants are used in the production of lipid-based NPs. Lipids act as penetration enhancers of drugs. It also enhances drug solubility and diffusion from the lymphatic to the circulatory system. One of its applications is to improve central nervous system (CNS) bioavailability. They may be manufactured via quick, solvent-free, and scalable procedures. Some methods of preparation include nanoprecipitation, emulsification–solvent evaporation (ESE), or high-pressure homogenization (207). The microemulsion technology was used to create curcuminoid solid lipid NPs with the active component being curcuminoids. It has antioxidant and anticancer effects (199). Exocytosis of NPs is the step that details the cytosolic administration of therapeutic agents via NP carriers (208). Silymarin-loaded nanostructured lipid carrier (NLC) is the best example that has been used clinically to overcome low solubility, permeability, and bioavailability issues in hepatic diseases (126). Cardamom essential oil (CEO)-loaded NLCs have successfully been synthesized using food-grade lipids which include cocoa butter and olive oil. This modification led to a small size (90%), improved loading capacity (>25%), and provided good physical and chemical stability (126). Paclitaxel-loaded solid lipid nanoparticles showed better anticancer action in the murine breast cancer mice model (209). Several solid lipid nanoparticles have been created for the administration of cytotoxic medications such as doxorubicin, idarubicin, paclitaxel, camptothecan, 7-ethyl-10-hydroxy-20(S)-CPT, etoposide, flurodooxyuridine, and retinoic acid, as well as cholesterylbutyrate (210). The Ferula assafoetida seed oil (FSEO) -SLN nanoparticles inhibited the development of the human NT-2 cancer stem cell line significantly (211). They caused apoptosis by increasing the expression of TNF-, P21, and Cas3 genes. The FSEO-SLN inhibited angiogenesis in CAM tissue by reducing the length and quantity of blood vessels. As a result, it has the potential to be investigated as an effective anti-cancer agent. Similarly, Epigallocatechin-3-gallate (EGCG) SLN can improve EGCG bioavailability and stability and can be employed as an alternate approach for EGCG oral delivery (212). In the rat model, SLN-EGCG had neither acute or sub-chronic toxicity when compared to free EGCG.
Carbon-Based Nanoparticles
Fullerenes, carbon nanotubes (CNTs), single-walled CNTs (SWCNTs), and graphene oxide (GO) are used in the production of carbon-based NPs. They have great antibacterial action and are utilized to limit energy metabolism, reduce or enhance O2 absorption, and inhibit bacterial growth (152). They are 3–10 nm in diameter. Their interior structure is made up of graphite sheets that have been glued together to form a quasi-spherical nanoparticle. They are also utilized to make biosensors.
CNTs have been extensively investigated for their potential to deliver anticancer herbal compounds with better therapeutic effectiveness and safety (213). CNTs have been used in both therapeutic and environmental settings. Ocimum tenuiflorum L. (tulsi extract) containing photosynthesized silver nanoparticles (AgNP) loaded into emulsified multiwalled carbon nanotube (MWCNT) was developed for fertility analysis targeted to the intracellular part of the sperm cell. It was characterized by a spherical shape, 5–40 nm in size, and surface plasmon resonance imaging (SPR) at 430 nm (126). The total polyphenols content and antioxidant activity of Echinacea purpurea (L.)Moench. extracts were determined using glassy carbon electrodes modified with CNTs and chitosan (214). In a recent research study, carbon quantum dots of Echinops persicus extract demonstrated efficient antiradical activity (215). The biosynthesized silver nanoparticles of the leaf extract of Teucrium polium exhibited significant anticancer activity against the MNK45 human gastric cancer cell line (216).
Polymeric Nanoparticle and Dendrimers
Polymeric nanoparticles (PNs) are colloidal solid particles that are made up of various polymers such as chitosan, collagen, polylactic acid (PLA), PLGA, or other biodegradable polymers (146). PN enhances the bioavailability of the poorly soluble drugs by allowing the oral administration of the drug and increasing accumulation of the drugs at the site of action since the smaller size of the particle allows penetration into the capillaries and the cells (155). Methods of preparation of polymeric NPs include complex coacervation method, coprecipitation, salting out method, solvent displacement method, and solvent emulsification-diffusion method (185). Solvent evaporation, emulsification/solvent diffusion, nanoprecipitation, emulsification/reverse, and salting-out methods are all used to create nanospheres while nanoprecipitation is used to create nanocapsules. An organic phase is initially generated in the solvent evaporation process, which comprises a polar organic solvent in which the polymer is dissolved and the active component has incorporated either by dissolving or dispersion. Now PNs are approved by FDA for clinical use in the treatment of breast and pancreatic cancer and also some clinical trials are performed in many of the compounds such as ABI-008, ABI-009, and ABI-011(NCT04229004). The free radical process was used to create A. absinthium extract-loaded polymeric NPs (NVA-AA), which were efficient against breast cancer cell lines; MCF-7 and MDA MB-231 (217). Uncaria tomentosa (Willd. ex Schult.) DC. (UT) extracts have been shown to have promising antitumor activity for the enhancement and delivery, in which PCL and PLGA were employed for the generation of NPs loaded with UT extract by a single emulsion solvent evaporation method (218).
Dendritic packaging of bioactive agents allows for the separation of the active site, which has a structure similar to active sites in biomaterials (137). In addition, unlike most polymers, water-soluble dendrimers may be created by functionalizing their outer shell with charged species or other hydrophilic groups. Capsaicin-loaded dendrimers showed significant cytotoxicity on VERO cell line with an IC50 of 1.25 μg/mL and MCF-7 and HEp2 cell lines with an IC50 of 0.62 μg/mL (219). Polyamidoamine (PAMAM) dendrimers as oral drug delivery carriers for quercetin were evaluated. It was discovered to be in the nanometer range (100 nm) with a low polydispersity index. An in vitro investigation indicated a biphasic release pattern of quercetin, with an initial rapid release phase followed by a sustained release phase, and a pharmacodynamic analysis offered early proof of concept for the potential of quercetin-PAMAM complexes (220). Poly (propylene imine) dendrimer is also evaluated for different anticancer phytochemicals (221).
Nanosphere (NS)
NSs are amorphous colloidal aqueous solutions with sizes ranging from 10 to 200 nm. Because they are tiny in size, they may be easily delivered orally, locally, and systemically, resulting in better bioavailability. They are made up of synthetic polymers such as PLA, polyglycolic acid (PGA), PLGA, and others (222). The main functions of the system include extending drug action due to delayed drug release, boosting bioavailability, enhancing drug entrapment, and boosting drug stability. It can also act against chemical degradation and minimize drug toxicity. Albumin NS, modified NS, starch NS, gelatin NS, polypropylene dextran NS, and polylactic acid NS are all examples of biodegradable NSs (223). Presently, two novel forms of NS have emerged: immune NS and magnetic NS. Both of these NSs are joined to form the immunomagnetic NS, and they considerably enhance drug targeting (222, 224). The targeting ability of the NS is currently being investigated in HeLa cells. Researchers have discovered that the C-phycocyanin (C-pc) NS is employed as a fluorescent producer. The intensity of fluorescence is higher in C-pc, indicating that it was directed at HeLa cells. Studies have revealed that the CD55 specific ligand peptide (CD55sp) is a potent anti-cancer factor. CD55sp is injected into the body of a mouse to assess the distribution of NSs, which are collected in the spleen, liver, and tumor. The liver and spleen metabolize the medication and start phagocytosis against malignancies (225). The pre-clinical trial such as oridonin (ORI)-loaded poly (D, L – lactic acid) (PLA) and polymer (RGD – Gly – Asp peptides) for the better antitumor actions (126). Another study created a near-infrared-responsive pharmacological system based on Au nanocages with Biotin–PEG–SH modification for the combination of doxorubicin and quercetin. The resulting nanocomplex have substantially more effective effects on MCF-7/ADR cell growth suppression under near-infrared irradiation. Furthermore, co-administration of doxorubicin and quercetin might significantly boost doxorubicin intracellular accumulation and dispersion in nuclei (226).
Nanocapsule
Nanocapsule (NC) is a colloidal dispersion system with a conventional core-shell structure, and the medicine is captured into the cavity of the central regions of the shell, which is surrounded by various polymer components such as polystyrene, titanium oxide (TIO2), and silver (Ag). The NC platform has high drug encapsulation efficiency, low polymer content, and a core-shell that protects the medication from degradation elements such as pH and light (227). Nanoprecipitation, arc discharge method, emulsion–diffusion, double emulsification, interfacial polymerization, emulsion–coacervation, polymer coating, and layer–by–layer coating are some of the methods used to create nanocapsules. The layer–by–layer approach is used to improve the hydrophilicity of crystals, such as artemisinin (ART, Artemisia annua L) crystals, having good anticancer action. Herbal compounds have been encapsulated with polyelectrolytes for pharmacological self-release (150). There is one other method of preparation known as interfacial polymerization that yields a dispersion of aqueous core nanocapsules (228). Biogenic synthesis of PEG-enhanced Moringa oleifera Lam Ag nanocapsules was performed and its antibacterial activity was tested. The Ag nanocapsule (AgNs) formed had a single-phase cubic structure as seen under X-ray spectroscopy (229). Chitosan nanocapsules of tarragon essential oil were made, and had low cytotoxicity and long-lasting activity as a green nano-larvicide. Its cytotoxicity was checked on human skin lines (230). This system is already a clinical trial is completed such as an anti – HER2 mAb with paclitaxel and a mAb with doxorubicin (Doxo®) (231). Mardani et al. discovered that curcumin nanomicelles might suppress lung metastasis and melanoma cell growth (B16 F10). The time and concentration of incubation are critical elements in imparting the potential activity of nanomicelle. Curcumin nanomicelles cause apoptosis even at low concentrations of 20 M. Curcumin also reduces angiogenesis and controls T Cell activation (232).
Metallic Nanoparticle
Metallic NPs (MNPs) are comprised of a metal core made of inorganic metal or metal oxide, which is generally surrounded by a shell also made of organic or inorganic material or metal oxide. Gold, silver, iron oxide, copper, zinc oxide, TiO2, platinum, selenium, gadolinium, palladium, and cerium dioxide are some of the metals and metal oxides that are employed. Because NPs are poisonous to live cells, their manufacturing and usage in powder form are deemed risky. To address this issue, highly reactive MNPs can be encapsulated (233). Aqueous plant extracts were mixed with metal salt solutions to create MNPs. Secondary plant metabolites are continually involved in the redox process that produces environmentally favorable NPs. The initial indication for MNP production is detected as a color shift. UV visible spectroscopy can be used to track the development of the reaction. Because of the drawbacks of the other approaches, the biological synthesis of NPs is the ideal way for producing these MNPs (234). It is a one-step bio-reduction approach that requires less energy to synthesize eco-friendly NPs. The biochemical reaction of AgNO3 reacting with plant broth results in the synthesis of AgNPs, according to the bio-reduction technique. Tribulus terrestris L extract was combined with various molar concentrations of silver nitrate solution to create eco-friendly AgNPs with distinct morphological properties. The methanolic extract of the Callicarpa maingayi Kjng and Gamble stem is employed in the manufacture of AgNPs and the formation of [Ag (Callicarpa maingayi)] + complex. The aldehyde group contained in the extract is mainly involved in the reduction of silver ions into metallic AgNPs (235). Piper nigrum L., leaves were stated to contain an important bioactive compound, involved in the NP synthesis by an eco-friendly method. Artemisia nilagirica(C.B.Clarke) Pamp., leaves were also used for the synthesis of NPs (224). These NPs are used because of their various properties. AgNPs are used as an antibacterial agent, wound dressing material, bone and tooth cement, and for water purification. MNPs are being used in a variety of fields, not simply in medicine and agriculture. MNPs are also employed in the manufacture of several bacteria, including Klebsiella pneumonia and Pseudomonas aeruginosa. It is also employed in the production of bioactive compounds and phytochemical constituents (236). In now a day the clinical trial is performed in some inorganic nanomaterials such as gold nanoparticles and silica nanoparticles (237). Folic acid surface modification improves the targetability of curcumin magnetic nanoparticles. Curcumin-loaded folate-grafted magnetic nanoparticles inhibit KB nasopharyngeal cancer cells and MCF-7 breast cancer cells significantly. The nanoparticles demonstrated targeted thermo-chemotherapy leading to apoptosis by a selective interaction with folate receptors, which are abundantly expressed in cancer cells, based on magnetic effect (238). The eupatorium-containing mPEG-b-PLGA-coated iron oxide nanoparticles were investigated towards prostate cancer cell lines (DU-145 and LNcaP). Furthermore, it exhibits increased apoptosis and reduced necrosis in the sub G1 phase, as well as enhanced cell populations, rendering it a suitable option for drug-resistant cancer treatment (239). The MTT experiment revealed that eupatorin-loaded Fe3O4@mPEG-b-PLGA nanoparticles significantly slowed the development of DU-145 and LNcaP cells, with IC50 values of 100 M and 75 M, respectively.
Inorganic Nanoparticle
Inorganic nanoparticles are composed of silver, gold, iron oxide, silica, platinum, and gadolinium hydroxide (Gd(OH)3). Magnetic (superparamagnetic iron oxide particles), nanoshell (dielectric silica core in thin gold metal shell), metallic, and ceramic (porous biocompatible substance) nanoparticles are its additional subclassifications based on the material used (240). They have good biocompatibility, can reduce cytotoxicity of medicines, increase the formation of reactive oxygen species (ROS), and have antibacterial action. In comparison to organic materials, these NPs are nontoxic, hydrophilic, biocompatible, and very stable. Inorganic nano-drug delivery methods such as mesoporous silica NPs, CNTs, layered double hydroxides, superparamagnetic iron oxide NPs, and calcium phosphate NPs have been developed as therapeutic uses in a variety of disorders, including neurodegenerative diseases. Magnetic NPs (MN) are employed in magnetic resonance imaging (MRI). Using zirconium oxide NPs, the antifungal activity of neem and aloe vera formulations was sustained and increased (241). In recent years, inorganic NPs have been synthesized and conjugated with tiny drugs for a variety of therapies. Inorganic NPs, which are mostly based on selenium, metallic chalcogenide, silicon, and carbon, are employed in oncotherapy (242). A pre-clinical trial of Anti-EGFR antibody cetuximab (C255) approved by FDA for the treatment of EGFR – positive colorectal cancer and palladium compound (TOOKAD) is a clinical trial by photo-dynamic therapy for the treatment of prostate cancer (65).
Recent Patents
Experts are currently working to modify particles by combining many newer sorts of compounds. The plant bioactive molecule has been found to provide considerable benefits in cancer therapy; however, administering this component is not easy (243). Consequently, a recent patent provides evidence of a unique herbal ingredient-delivery technique. Although there are still challenges to be resolved, this will become a significant delivery method soon. It has already achieved notable progress in the fields of NP separation, purification, and creation (145). Table 3 shows several recently issued patents for nanoparticle formulations comprising natural anti-cancer molecules.
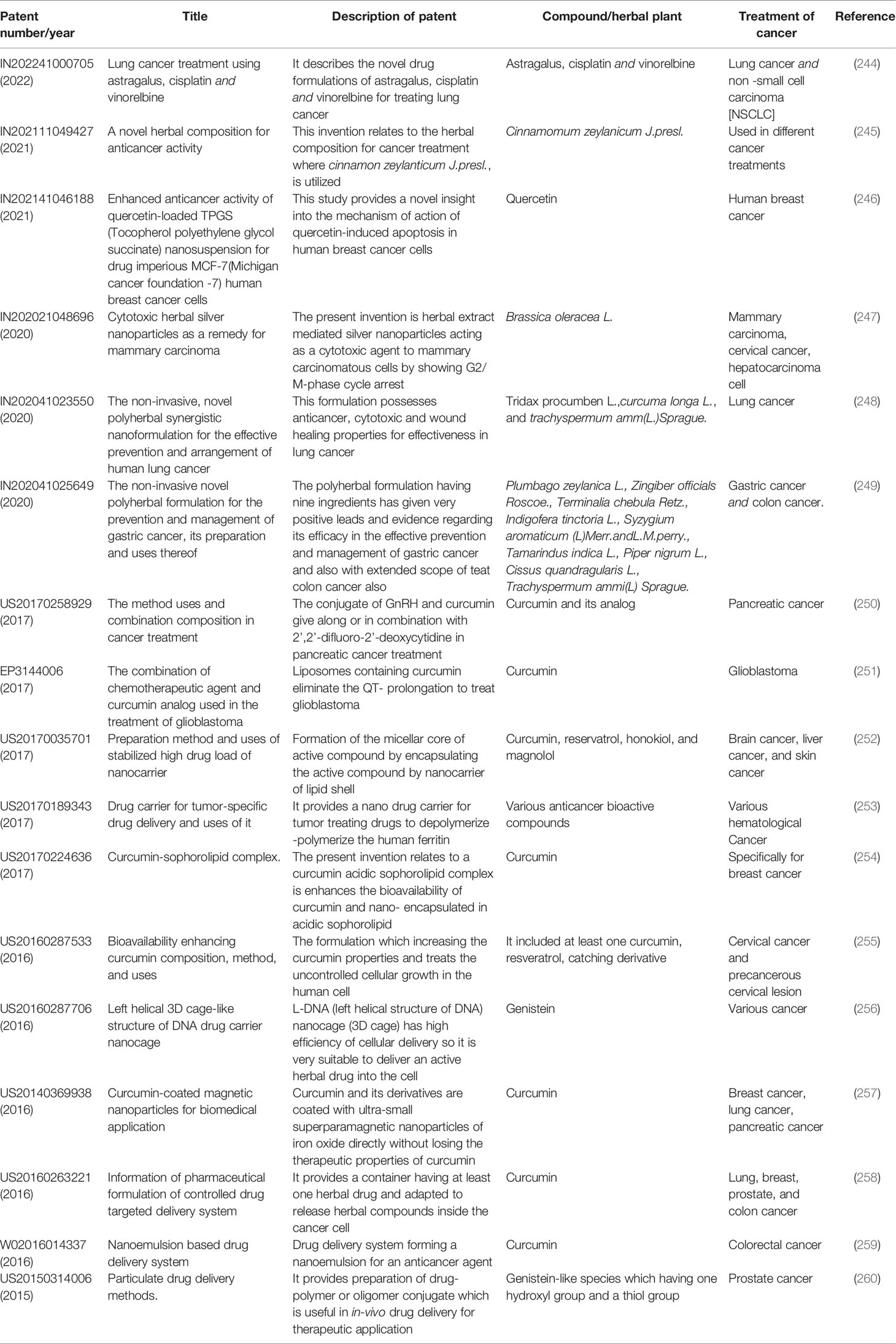
Table 3 Summary of recently published patents related to nanoparticle formulation with the natural anti-cancer molecule.
Challenges
Medicinal plants may have a large number of active ingredients, some of which may be beneficial in therapy while others may be harmful (261). There is a lack of standard protocols and meta-analytic evidence for the use of herbal medications. The predominant role of herbal medication in cancer is to treat symptoms of neoplasm and strengthen the immune system (262). Herbal medicines are used as adjuvant therapy to treat side effects associated with chemotherapy but they have not been used alone to cure or prevent cancer. In vitro studies have been successfully performed for the treatment of cancer but in clinical trials, herbal medicine did not succeed much (32). Herbal medicines can be useful in the case of primary cancer but they cannot be useful to treat severe cases of cancer until yet (32). Satisfactory evidence regarding the administration of herbal medication through the parenteral route and proper guidance for the route of administration is not available. A target-based specific treatment for the cancerous cell is not commercially available in herbal therapy. The database of specific interactions of these medications along with other prescription drugs is deficient (263). Several compounds show structural similarity but verification of their structural relationship and function are not well defined (264). In a patient taking cancer treatment, herbal medicine may interfere and alter pharmacokinetic properties. Along with the above challenges, one more challenge is the clarity of the mechanism behind its pharmacological action. While conventional medicine has a proper monitoring system, Ayurvedic treatments do not have a sufficient monitoring system, data analysis, objective level, measuring of the drug, and tools of standardization (265). When herbal medication is used along with other chemotherapeutic agents, it can reduce the bioavailability of chemotherapeutic agents (169).
Some of the herbal medications do not just act on target cells, but also a wide range of normal cells. The limitation of herbal medication is that it can have numerous targets. In some instances, herbal extracts may produce adverse effects. Resistance is common in herbal treatments such as camptothecin (266). Vincristine, a component of the vinca alkaloid, may cause swelling because of fluid accumulation in tissue. If a person consumes vinca, it may cause heart and blood vessel problems. Coma, drowsiness, nerve discomfort, motor dysfunction, and other neurological problems might occur because of vinca ingestion. Vinca alkaloids cause skin responses, as well as baldness and rapid hair loss. Indigestion, vomiting, and cramping are some of the gastrointestinal complications associated with this therapy (267). Decreased bone marrow activity and pain due to nerve damage are consequences associated with taxols (e.g., paclitaxel) (268). Chaga mushroom powder, which is used to treat cancer, has been linked to calcium oxalate buildup, which can lead to tubular damage in the lumin of tubules, and oxalate crystals may be observed (269). Treatment with glycyrrhiza may result in tumor-bearing pressure, lack of appetite, and a reduction in potassium levels in the blood. It also causes hypokalemic conditions when other hypokalemic drugs are co-administered with it (270, 271). Patients who choose herbal medication as a cancer treatment may have various safety problems, such as a reduction of cancer drug concentration in the blood. Mephitic symptoms have been observed in cancer patients who are receiving herbal medicines. Herbal medications, in addition to their benefits, have certain disadvantages, such as changes in metabolism caused by drug interactions and changes in absorption. The maidenhair tree increases the harmful effects of paclitaxel by blocking its metabolism (272). Menorrhagia and dementia can occur when herbal medications are taken in conjunction with pregnancy-preventive medications (273).
Future Prospects
Currently, people are focusing on herbal medication, which is cheaper and is commonly available so, advancements in their formulation and proper drug delivery system should be introduced. Drug regulatory standards related to safety, quality, and efficacy should be developed and managed. Interaction of other anticancer drugs with herbal medications should be studied and data should be collected for proper buildup and usage of herbal drugs. A wide variety of compounds will be available because many types of research are ongoing for herbal medications. Advancement can be made by modification of constituents to produce semisynthetic medicines. Many genetically modified natural products can be established by the use of the latest biotechnological methods. Complementary and alternative medicine (CAM) formulations play an important role and have achieved significant results in anticancer treatment. Production, sale, marketing, and use of these medicines should be properly controlled by the government and specific norms should be introduced for their use. It has a high potential for treating cancer patients in the future. Some herbal drugs are administered via NPs-based formulations, which can cause toxicity, and some of their interactions should not be overlooked. As a result, a herbal bioactive chemical is loaded with a naturally produced NP, such as exosomes, which can create a high reaction while being low in toxicity. Many drugs are difficult to isolate at times, and the amount of substance available is sometimes limited. To solve this challenge, one must first obtain that specific gene implanted in a host and then provide the right environmental conditions for proper development and culture.
Theranostics is a concept that combines the phrases therapeutics and diagnostics, and it refers to the use of medications for cancer diagnosis and therapy using the concept of theranostic and for overcoming chemoresistance in breast cancer, the co-delivery system for doxorubicin (DOX) and curcumin (CUR) was designed, in a sustained pH-dependent manner, which acted as a chemosensitizer. The surface of magnetic NC was decorated by hydroxyapatite that was cross-linked with β-cyclodextrin leading to the improvement in curcumin bioavailability. The therapeutic efficiency was established by the reduction in tumor size (274, 275). In another research, nano curcumin was combined with rare-earth-doped upconversion nanoparticles (UCNPs). Poly (lactic-co-glycolic acid) (PLGA) was used to promote sustained drug delivery and drug release, targeting, minimizing the non-specific consumption by undesirable tissues, and enhancing its aqueous solubility (276). Curcumin-incorporated PLGA nanoparticles have exhibited increased cellular uptake, induction of apoptosis, suppression of tumor cell proliferation, and improvement in bioavailability. The advantage of combining UCNP and nano curcumin lies in the upconversion of visible light leading to the excitement of photosensitizer and favoring the fluorescence resonance energy transfer (FRET) which can be used in the examination of in vivo interaction between bimolecular entities (276).
Berberine with etoposide via albumin nanoparticle was used in the Nano-in Nano approach. Co-encapsulation of BER was hypothesized to reduce the therapeutic dose of ETP, which would minimize its toxicity and overcome the problem of developing resistance and enhance its antitumor efficacy (275). When given in lung cancer-bearing mice, it leads to a decrease in vascular endothelial growth factor (VEGF) expression level, triggered caspase activation with tumor cell apoptosis, and enhanced antiangiogenic effect that might refer to synergistic topoisomerase II inhibition and reduction of multidrug resistance (MDR) effect in A549 cells (232, 275, 277). Thus, various plants possessing anticancer properties can be formulated with modifications to make them suitable for use as a theranostic leading to a substantial increase in the potency of anticancer drugs in the future.
Conclusion
Conventional cancer therapies are sometimes restricted due to their low specificity, which can result in significant side effects and toxicity, as well as the likely creation of MDR phenotype. As a corollary, the necessity to precisely destroy cancer cells, overcome MDR, and boost the specificity of a medication using spatial, temporal, and dosage regulation of its release is driving the hunt for more effective anti-cancer therapy. Natural products are a key source for the development of innovative anti-cancer medicines that may be used both preventively and therapeutically. Phytochemicals at lower doses initiate adaptive response but as one increases the dose it produces acute autophagic response and apoptosis. There are several types of drug carriers available today, each with distinct and varied qualities that make them suitable for the treatment of cancers. After appropriate studies, it is now possible to create multipurpose nanocarriers that permit the slow and selective release of various drugs at specific tumor locations. In vitro and preclinical research have shown that NPs transporting and delivering both chemotherapeutic drugs and natural ingredients are extremely efficient for both curative and chemosensitizing reasons. Allopathic drugs have some challenges with them, thus diverse research is ongoing for herbal medication to improve their effectiveness and for acknowledgment of their side effects. Plant extracts appear to be less effective and efficient than refined extracted herbal components. The inclusion of mixed elements may lead to antagonistic activity or undesirable side effects, as well as a reduction in the therapeutic potency of the main substance. The herbal component’s anticancer activity should be recognized based on IC50 for cancer cell survival. Herbal medications with high IC50 values have little anti-cancer potency and so are not good options. Drug-drug interactions among herbal ingredients and other traditional FDA-approved drugs should be extensively explored to find the most effective additive mixtures. The importance of herbal medicines may increase in the future via such a nano-drug delivery system. Further research is requisite to address the safety issues related to these systems.
Author Contributions
VC has prepared the backbone of the manuscript. VC and AP wrote the original draft of the manuscript with KM and SS. Z-SC, ZW, KH, AP, and VC refined the first draft. Z-SC and VC critically revise the manuscript for intellectually correct content. KH supports the project. All authors contributed to the article and approved the submitted version.
Conflict of Interest
The authors declare that the research was conducted in the absence of any commercial or financial relationships that could be construed as a potential conflict of interest.
Publisher’s Note
All claims expressed in this article are solely those of the authors and do not necessarily represent those of their affiliated organizations, or those of the publisher, the editors and the reviewers. Any product that may be evaluated in this article, or claim that may be made by its manufacturer, is not guaranteed or endorsed by the publisher.
Acknowledgments
VC wants to dedicate this article to L M College of Pharmacy, Ahmedabad (India) on the 75th Year celebration of the college. VC would like to thank Vishal Vaghasiya, Vishwa Modi, and Shivangi D. Bagadia for their support in this manuscript preparation. The figures are created with BioRender.com.
References
1. Kooti W, Servatyari K, Behzadifar M, Asadi-Samani M, Sadeghi F, Nouri B, et al. Effective Medicinal Plant in Cancer Treatment, Part 2: Review Study. J Evid Based Complement Altern Med (2017) 22(4):982–95. doi: 10.1177/2156587217696927
2. Chavda VP, Ertas YN, Walhekar V, Modh D, Doshi A, Shah N, et al. Advanced Computational Methodologies Used in the Discovery of New Natural Anticancer Compounds. Front Pharmacol (2021) 12:702611. doi: 10.3389/fphar.2021.702611
3. Arruebo M, Vilaboa N, Sáez-Gutierrez B, Lambea J, Tres A, Valladares M, et al. Assessment of the Evolution of Cancer Treatment Therapies. Cancers (Basel) (2011) 3(3):3279–330. doi: 10.3390/cancers3033279
4. Mansoori B, Mohammadi A, Davudian S, Shirjang S, Baradaran B. The Different Mechanisms of Cancer Drug Resistance: A Brief Review. Adv Pharm Bull (2017) 7(3):339–48. doi: 10.15171/apb.2017.041
5. Jardim DL, Goodman A, de Melo Gagliato D, Kurzrock R. The Challenges of Tumor Mutational Burden as an Immunotherapy Biomarker. Cancer Cell (2021) 39(2):154–73. doi: 10.1016/j.ccell.2020.10.001
6. Ulbright TM. Germ Cell Tumors of the Gonads: A Selective Review Emphasizing Problems in Differential Diagnosis, Newly Appreciated, and Controversial Issues. Mod Pathol (2005) 18(2):S61–79. doi: 10.1038/modpathol.3800310
7. Zeeshan R, Mutahir Z. Cancer Metastasis - Tricks of the Trade. Bosn J Basic Med Sci (2017) 17(3):172–82. doi: 10.17305/bjbms.2017.1908
8. Hecker E. Definitions and Terminology in Cancer (Tumour) Etiology. Bull World Health Organ (1976) 54(1):1–10.
9. Weisburger JH. Antimutagens, Anticarcinogens, and Effective Worldwide Cancer Prevention. J Environ Pathol (1999) 18(2):85–93.
10. de Martel C, Georges D, Bray F, Ferlay J, Clifford GM. Global Burden of Cancer Attributable to Infections in 2018: A Worldwide Incidence Analysis. Lancet Glob Heal (2020) 8(2):e180–90. doi: 10.1016/S2214-109X(19)30488-7
11. Ferlay J, Colombet M, Soerjomataram I, Mathers C, Parkin DM, Piñeros M, et al. Estimating the Global Cancer Incidence and Mortality in 2018: GLOBOCAN Sources and Methods. Int J Cancer (2019) 144(8):1941–53. doi: 10.1002/ijc.31937
12. Martin MC. Radiation Oncology—External-Beam Radiation Therapy. Health Phys (2019) 116(2):184–8. doi: 10.1097/HP.0000000000001034
13. Dawood S, Leyland-Jones B. Pharmacology and Pharmacogenetics of Chemotherapeutic Agents. Cancer Invest (2009) 27(5):482–8. doi: 10.1080/07357900802574660
15. Wyszomirska A. Iodine-131 for Therapy of Thyroid Diseases. Physical and Biological Basis. Nucl Med Rev Cent East Eur (2012) 15(2):120–3.
16. Cragg GM, Pezzuto JM. Natural Products as a Vital Source for the Discovery of Cancer Chemotherapeutic and Chemopreventive Agents. Med Princ Pract (2016) 25(2):41–59. doi: 10.1159/000443404
17. Aggarwal BB, Prasad S, Reuter S, Kannappan R, Yadev VR, Park B, et al. Identification of Novel Anti-Inflammatory Agents From Ayurvedic Medicine for Prevention of Chronic Diseases: “Reverse Pharmacology” and “Bedside to Bench” Approach. Curr Drug Targets (2011) 12(11):1595–653. doi: 10.2174/138945011798109464
18. Baliga MS, Meera S, Vaishnav LK, Rao S, Palatty PL. Rasayana Drugs From the Ayurvedic System of Medicine as Possible Radioprotective Agents in Cancer Treatment. Integr Cancer Ther (2013) 12(6):455–63. doi: 10.1177/1534735413490233
19. Pilmeijer A. Cancer & Ayurveda as a Complementary Treatment. Int J Complement Altern Med (2017) 6(5):00202. doi: 10.15406/ijcam.2017.06.00202
20. Smit HF, Woerdenbag HJ, Singh RH, Meulenbeld GJ, Labadie RP, Zwaving JH. Ayurvedic Herbal Drugs With Possible Cytostatic Activity. J Ethnopharmacol (1995) 47(2):75–84. doi: 10.1016/0378-8741(95)01255-C
21. Chauhan A, Semwal DK, Mishra SP, Semwal RB. Ayurvedic Research and Methodology: Present Status and Future Strategies. Ayu (2015) 36(4):364–9. doi: 10.4103/0974-8520.190699
22. Balachandran P, Govindarajan R. Cancer–An Ayurvedic Perspective. Pharmacol Res (2005) 51(1):19–30. doi: 10.1016/j.phrs.2004.04.010
23. Singh RH. An Assessment of the Ayurvedic Concept of Cancer and a New Paradigm of Anticancer Treatment in Ayurveda. J Altern Complement Med (2002) 8(5):609–14. doi: 10.1089/107555302320825129
24. Trawick M. An Ayurvedic Theory of Cancer. Med Anthropol (1991) 13(1–2):121–36. doi: 10.1080/01459740.1991.9966044
25. Cragg GM, Newman DJ. Plants as a Source of Anti-Cancer Agents. J Ethnopharmacol (2005) 100(1–2):72–9. doi: 10.1016/j.jep.2005.05.011
26. Jain R, Kosta S, Tiwari A. Ayurveda and Cancer. Pharmacognosy Res (2010) 2(6):393–4. doi: 10.4103/0974-8490.75463
27. Sumantran VN, Tillu G. Cancer, Inflammation, and Insights From Ayurveda. Evid-Based Complement Altern Med (2012) 2012:306346. doi: 10.1155/2012/306346
29. Agarwal N, Majee C, Chakraborthy GS. Natural Herbs as Anticancer Drugs. Int J PharmTech Res (2012) 4(3):1142–53. doi: 10.1201/9781315366753-15
30. Bisht D, Kumar D, Kumar D, Dua K, Chellappan DK. Phytochemistry and Pharmacological Activity of the Genus Artemisia. Arch Pharm Res (2021) 44(5):439–74. doi: 10.1007/s12272-021-01328-4
31. Pan S-Y, Litscher G, Gao S-H, Zhou S-F, Yu Z-L, Chen H-Q, et al. Historical Perspective of Traditional Indigenous Medical Practices: The Current Renaissance and Conservation of Herbal Resources. Evid-Based Complement Altern Med (2014) 2014:525340. doi: 10.1155/2014/525340
32. Choudhari AS, Mandave PC, Deshpande M, Ranjekar P, Prakash O. Phytochemicals in Cancer Treatment: From Preclinical Studies to Clinical Practice. Front Pharmacol (2020) 10:1614. doi: 10.3389/fphar.2019.01614
33. Akter R, Uddin SJ, Grice ID, Tiralongo E. Cytotoxic Activity Screening of Bangladeshi Medicinal Plant Extracts. J Nat Med (2014) 68(1):246–52. doi: 10.1007/s11418-013-0789-5
34. Du J, Lu X, Long Z, Zhang Z, Zhu X, Yang Y, et al. In Vitro and In Vivo Anticancer Activity of Aconitine on Melanoma Cell Line B16. Molecules (2013) 18(1):757–67. doi: 10.3390/molecules18010757
35. Svoboda GH, Poore GA, Simpson PJ, Boder GB. Alkaloids of Acronychia Baueri Schott I: Isolation of the Alkaloids and a Study of the Antitumor and Other Biological Properties of Acronycine. J Pharm Sci (1966) 55(8):758–68. doi: 10.1002/jps.2600550803
36. Martha Thomson MA. Garlic [Allium Sativum]: A Review of its Potential Use as an Anti-Cancer Agent. Curr Cancer Drug Targets (2003) 3(1):67–81. doi: 10.2174/1568009033333736
37. Milner JA. A Historical Perspective on Garlic and Cancer. J Nutr (2001) 131(3):1027S–31S. doi: 10.1093/jn/131.3.1027S
38. Bianchini F, Vainio H. Allium Vegetables and Organosulfur Compounds: Do They Help Prevent Cancer? Environ Health Perspect (2001) 109(9):893–902. doi: 10.1289/ehp.01109893
39. Nakagawa H, Tsuta K, Kiuchi K, Senzaki H, Tanaka K, Hioki K, et al. Growth Inhibitory Effects of Diallyl Disulfide on Human Breast Cancer Cell Lines. Carcinogenesis (2001) 22(6):891–7. doi: 10.1093/carcin/22.6.891
40. Khalil N, Bishr M, Desouky S, Salama O. Ammi Visnaga L., a Potential Medicinal Plant: A Review. Molecules (2020) 25(2):301. doi: 10.3390/molecules25020301
41. Asadi-Samani M, Kooti W, Aslani E, Shirzad H. A Systematic Review of Iran’s Medicinal Plants With Anticancer Effects. J Evid-Based Complement Altern Med (2016) 21(2):143–53. doi: 10.1177/2156587215600873
42. Yang Y, Yue Y, Runwei Y, Guolin Z. Cytotoxic, Apoptotic and Antioxidant Activity of the Essential Oil of Amomum Tsao-Ko. Bioresour Technol (2010) 101(11):4205–11. doi: 10.1016/j.biortech.2009.12.131
43. Chen C, You F, Wu F, Luo Y, Zheng G, Xu H, et al. Antiangiogenesis Efficacy of Ethanol Extract From Amomum Tsaoko in Ovarian Cancer Through Inducing ER Stress to Suppress P-STAT3/NF-Kb/IL-6 and VEGF Loop. Evid-Based Complement Altern Med (2020) 2020:2390125. doi: 10.1155/2020/2390125
44. Sœur J, Marrot L, Perez P, Iraqui I, Kienda G, Dardalhon M, et al. Selective Cytotoxicity of Aniba Rosaeodora Essential Oil Towards Epidermoid Cancer Cells Through Induction of Apoptosis. Mutat Res - Genet Toxicol Environ Mutagen (2011) 718(1–2):24–32. doi: 10.1016/j.mrgentox.2010.10.009
45. Zhou HJ, Wang WQ, Wu GD, Lee J, Li A. Artesunate Inhibits Angiogenesis and Downregulates Vascular Endothelial Growth Factor Expression in Chronic Myeloid Leukemia K562 Cells. Vascul Pharmacol (2007) 47(2–3):131–8. doi: 10.1016/j.vph.2007.05.002
46. Kim J, Jung KH, Yan HH, Cheon MJ, Kang S, Jin X, et al. Artemisia Capillaris Leaves Inhibit Cell Proliferation and Induce Apoptosis in Hepatocellular Carcinoma. BMC Complement Altern Med (2018) 18(1):1–10. doi: 10.1186/s12906-018-2217-6
47. Jung KH, Rumman M, Yan H, Cheon MJ, Choi JG, Jin X, et al. An Ethyl Acetate Fraction of Artemisia Capillaris (ACE-63) Induced Apoptosis and Anti-Angiogenesis via Inhibition of PI3K/AKT Signaling in Hepatocellular Carcinoma. Phyther Res (2018) 32(10):2034–46. doi: 10.1002/ptr.6135
48. Cho WCS, Leung KN. In Vitro and In Vivo Anti-Tumor Effects of Astragalus Membranaceus. Cancer Lett (2007) 252(1):43–54. doi: 10.1016/j.canlet.2006.12.001
49. Li S, Sun Y, Huang J, Wang B, Gong Y, Fang Y, et al. Anti-Tumor Effects and Mechanisms of Astragalus Membranaceus (AM) and Its Specific Immunopotentiation: Status and Prospect. J Ethnopharmacol (2020) 258:112797. doi: 10.1016/j.jep.2020.112797
50. Abdolmohammadi MH, Fouladdel S, Shafiee A, Amin G, Ghaffari SM, Azizi E. Antiproliferative and Apoptotic Effect of Astrodaucus Orientalis (L.) Drude on T47D Human Breast Cancer Cell Line: Potential Mechanisms of Action. Afr J Biotechnol (2009) 8(17):4265–76. doi: 10.5897/AJB09.323
51. Nazemiyeh H, Sciences TU of M, Razavi SM, Ardabili U of M, Delazar A, Sciences TU of M, et al. Distribution Profile of Volatile Constituents in Different Parts of Astrodaucus Orientalis (L.) Drude. Rec Nat Prod (2009) 3(3):126–30. Available at: https://acgpubs.org/article/records-of-natural-products/2009/3-july-september/distribution-profile-of-volatile-constituents-in-different-parts-of-astrodaucus-orientalis-l-drude.
52. Kapadia GJ, Azuine MA, Rao GS, Arai T, Iida A, Tokuda H. Cytotoxic Effect of the Red Beetroot (Beta Vulgaris L.) Extract Compared to Doxorubicin (Adriamycin) in the Human Prostate (PC-3) and Breast (MCF-7) Cancer Cell Lines. Anticancer Agents Med Chem (2011) 11(3):280–4. doi: 10.2174/187152011795347504
53. Suhail MM, Wu W, Cao A, Mondalek FG, Fung K-M, Shih P-T, et al. Boswellia Sacra Essential Oil Induces Tumor Cell-Specific Apoptosis and Suppresses Tumor Aggressiveness in Cultured Human Breast Cancer Cells. BMC Complement Altern Med (2011) 11:129. doi: 10.1186/1472-6882-11-129
54. Becer E, Kabadayı H, Başer KHC, Vatansever HS. Boswellia Sacra Essential Oil Manages Colon Cancer Stem Cells Proliferation and Apoptosis: A New Perspective for Cure. J Essent Oil Res (2021) 33(1):53–62. doi: 10.1080/10412905.2020.1839586
55. Filippini T, Malavolti M, Borrelli F, Izzo AA, Fairweather-Tait SJ, Horneber M, et al. Green Tea (Camellia Sinensis) for the Prevention of Cancer. Cochrane Database Syst Rev (2020) (3):CD005004. doi: 10.1002/14651858.CD005004.pub3
56. Rafieian-Kopaei M, Movahedi M. Breast Cancer Chemopreventive and Chemotherapeutic Effects of Camellia Sinensis (Green Tea): An Updated Review. Electron Phys (2017) 9(2):3838–44. doi: 10.19082/3838
57. Lorence A, Medina-Bolivar F, Nessler CL. Camptothecin and 10-Hydroxycamptothecin From Camptotheca Acuminata Hairy Roots. Plant Cell Rep (2004) 22(6):437–41. doi: 10.1007/s00299-003-0708-4
58. Felipe KB, Kviecinski MR, da Silva FO, Bücker NF, Farias MS, Castro LSEPW, et al. Inhibition of Tumor Proliferation Associated With Cell Cycle Arrest Caused by Extract and Fraction From Casearia Sylvestris (Salicaceae). J Ethnopharmacol (2014) 155(3):1492–9. doi: 10.1016/j.jep.2014.07.040
59. Ahmad NH, Rahim RA, Mat I. Catharanthus Roseus Aqueous Extract Is Cytotoxic to Jurkat Leukaemic T-Cells But Induces the Proliferation of Normal Peripheral Blood Mononuclear Cells. Trop Life Sci Res (2010) 21(2):101–13.
60. Nayab D, Ali D, Arshad N, Malik A, Choudhary MI, Ahmed Z. Cucurbitacin Glucosides From Citrullus Colocynthis. Nat Prod Res (2006) 20(5):409–13. doi: 10.1080/14786410500044997
61. Wasfi IA. Some Pharmacological Studies on Citrullus Colocynthis. J Herbs Spices Med Plants (2010) 2(2):49–74. doi: 10.1300/J044v02n02_08
62. Wineman E, Douglas I, Wineman V, Sharova K, Jaspars M, Meshner S, et al. Commiphora Gileadensis Sap Extract Induces Cell Cycle-Dependent Death in Immortalized Keratinocytes and Human Dermoid Carcinoma Cells. J Herb Med (2015) 5(4):199–206. doi: 10.1016/j.hermed.2015.08.001
63. Zhang Z, Wang CZ, Wen XD, Shoyama Y, Yuan CS. Role of Saffron and Its Constituents on Cancer Chemoprevention. Pharm Biol (2013) 51(7):920–4. doi: 10.3109/13880209.2013.771190
64. Chryssanthi DG, Lamari FN, Iatrou G, Pylara A, Karamanos NK, Cordopatis P. Inhibition of Breast Cancer Cell Proliferation by Style Constituents of Different Crocus Species. Anticancer Res (2007) 27(1 A):357–62.
65. Tomeh MA, Hadianamrei R, Zhao X. A Review of Curcumin and Its Derivatives as Anticancer Agents. Int J Mol Sci (2019) 20(5):1033. doi: 10.3390/ijms20051033
66. Lakshmi S, Padmaja G, Remani P. Antitumour Effects of Isocurcumenol Isolated From Curcuma Zedoaria Rhizomes on Human and Murine Cancer Cells. Int J Med Chem (2011) 2011:1–13. doi: 10.1155/2011/253962
67. Shin Y, Lee Y. Cytotoxic Activity From Curcuma Zedoaria Through Mitochondrial Activation on Ovarian Cancer Cells. Toxicol Res (2013) 29(4):257–61. doi: 10.5487/TR.2013.29.4.257
68. Shebaby WN, Mroueh M, Bodman-Smith K, Mansour A, Taleb RI, Daher CF, et al. Daucus Carota Pentane-Based Fractions Arrest the Cell Cycle and Increase Apoptosis in MDA-MB-231 Breast Cancer Cells. BMC Complement Altern Med (2014) 14:387. doi: 10.1186/1472-6882-14-387
69. Bagheri SM, Abdian-Asl A, Moghadam MT, Yadegari M, Mirjalili A, Zare-Mohazabieh F, et al. Antitumor Effect of Ferula Assa Foetida Oleo Gum Resin Against Breast Cancer Induced by 4T1 Cells in BALB/c Mice. J Ayurveda Integr Med (2017) 8(3):152–8. doi: 10.1016/j.jaim.2017.02.013
70. Gioti K, Papachristodoulou A, Benaki D, Beloukas A, Vontzalidou A, Aligiannis N, et al. Glycyrrhiza Glabra-Enhanced Extract and Adriamycin Antiproliferative Effect on PC-3 Prostate Cancer Cells. Nutr Cancer (2020) 72(2):320–32. doi: 10.1080/01635581.2019.1632357
71. Karmakar SR, Biswas SJ. ARK-B. Anti-Carcinogenic Potentials of a Plant Extract (Hydrastis Canadensis): I. Evidence From In Vivo Studies in Mice (Mus Musculus). Asian Pac J Cancer Prev (2010) 11(2):545–51.
72. Guo B, Li X, Song S, Chen M, Cheng M, Zhao D, et al. Hydrastine Suppresses the Proliferation and Invasion of Human Lung Adenocarcinoma Cells by Inhibiting PAK4 Kinase Activity. Oncol Rep (2016) 35(4):2246–56. doi: 10.3892/or.2016.4594
73. Arata S, Watanabe J, Maeda M, Yamamoto M, Matsuhashi H, Mochizuki M, et al. Continuous Intake of the Chaga Mushroom (Inonotus Obliquus) Aqueous Extract Suppresses Cancer Progression and Maintains Body Temperature in Mice. Heliyon (2016) 2(5):e00111–1. doi: 10.1016/j.heliyon.2016.e00111
74. Prajapati RP, Kalariya M, Parmar SK, Sheth NR. Phytochemical and Pharmacological Review of Lagenaria Sicereria. J Ayurveda Integr Med (2010) 1(4):266–72. doi: 10.4103/0975-9476.74431
75. Bongiovanni G, Cantero J, Eynard A, Goleniowski M. Organic Extracts of Larrea Divaricata Cav. Induced Apoptosis on Tumoral MCF7 Cells With an Higher Cytotoxicity Than Nordihydroguaiaretic Acid or Paclitaxel. J Exp Ther Oncol (2008) 7(1):1–7.
76. Al-Fatimi M, Friedrich U, Jenett-Siems K. Cytotoxicity of Plants Used in Traditional Medicine in Yemen. Fitoterapia (2005) 76(3–4):355–8. doi: 10.1016/j.fitote.2005.02.009
77. Montero-Villegas S, Crespo R, Rodenak-Kladniew B, Castro MA, Galle M, Cicció JF, et al. Cytotoxic Effects of Essential Oils From Four Lippia Alba Chemotypes in Human Liver and Lung Cancer Cell Lines. J Essent Oil Res (2018) 30(3):167–81. doi: 10.1080/10412905.2018.1431966
78. Mekuria AN, Tura AK, Hagos B, Sisay M, Abdela J, Mishore KM, et al. Anti-Cancer Effects of Lycopene in Animal Models of Hepatocellular Carcinoma: A Systematic Review and Meta-Analysis. Front Pharmacol (2020) 11:1306. doi: 10.3389/fphar.2020.01306
79. Gatouillat G, Magid AA, Bertin E, Okiemy-Akeli M-G, Morjani H, Lavaud C, et al. Cytotoxicity and Apoptosis Induced by Alfalfa (Medicago Sativa) Leaf Extracts in Sensitive and Multidrug-Resistant Tumor Cells. Nutr Cancer (2014) 66(3):483–91. doi: 10.1080/01635581.2014.884228
80. Rosenthal GA, Nkomo P. The Natural Abundance of L-Canavanine, an Active Anticancer Agent, in Alfalfa, Medicago Sativa (L.). Pharm Biol (2000) 38(1):1–6. doi: 10.1076/1388-0209(200001)3811-BFT001
81. Eo HJ, Park JH, Park GH, Lee MH, Lee JR, Koo JS, et al. Anti-Inflammatory and Anti-Cancer Activity of Mulberry (Morus Alba L.) Root Bark. BMC Complement Altern Med (2014) 14:1–9. doi: 10.1186/1472-6882-14-200
82. Alipour G, Dashti S, Hosseinzadeh H. Review of Pharmacological Effects of Myrtus Communis L. And its Active Constituents. Phyther Res (2014) 28(8):1125–36. doi: 10.1002/ptr.5122
83. Majdalawieh AF, Fayyad MW. Recent Advances on the Anti-Cancer Properties of Nigella Sativa, a Widely Used Food Additive. J Ayurveda Integr Med (2016) 7(3):173–80. doi: 10.1016/j.jaim.2016.07.004
84. O’Hanlon FJ, Fragkos KC, Fini L, Patel PS, Mehta SJ, Rahman F, et al. Home Parenteral Nutrition in Patients With Advanced Cancer: A Systematic Review and Meta-Analysis. Nutr Cancer (2021) 73(6):943–55. doi: 10.1080/01635581.2020.1784441
85. Hong H, Baatar D, Hwang SG. Anticancer Activities of Ginsenosides, the Main Active Components of Ginseng. Evid Based Complement Alternat Med (2021) 2021:8858006. doi: 10.1155/2021/8858006
86. AL Shabanah OA, Alotaibi M, Al Rejaie SS, Alhoshani AR, Almutairi MM, Alshammari MA, et al. Inhibitory Effect of Ginseng on Breast Cancer Cell Line Growth Via Up-Regulation of Cyclin Dependent Kinase Inhibitor, P21 and P53. Asian Pac J Cancer Prev (2016) 17(11):4965–71. doi: 10.22034/APJCP.2016.17.11.4965
87. Deng Y, Ye X, Chen Y, Ren H, Xia L, Liu Y, et al. Chemical Characteristics of Platycodon Grandiflorum and Its Mechanism in Lung Cancer Treatment. Front Pharmacol (2021) 11:609825. doi: 10.3389/fphar.2020.609825
88. Shultz B, University W, Wilkes-Barre P. Medical Attributes of Podophyllum Peltatum - Mayapple. Molecules (2021) 26(17):5179.
89. Wu X, Li Q, Feng Y, Ji Q. Antitumor Research of the Active Ingredients From Traditional Chinese Medical Plant Polygonum Cuspidatum. Evid Based Complement Alternat Med (2018) 2018:2313021. doi: 10.1155/2018/2313021
90. Mahmoudi E, Abolfathi M, Hassanzadeh N, Milasi YE, Dehghani-Samani M, Khaledi M, et al. Prunus Armeniaca Effects on Expression of Genes Related to Apoptosis in Human Breast Cancer Cells. Transl Med Commun (2019) 4(1):4–9. doi: 10.1186/s41231-019-0036-5
91. Jeong SJ, Koh W, Kim B, Kim SH. Are There New Therapeutic Options for Treating Lung Cancer Based on Herbal Medicines and Their Metabolites? J Ethnopharmacol (2011) 138(3):652–61. doi: 10.1016/j.jep.2011.10.018
92. Majumder M, Debnath S, Gajbhiye RL, Saikia R, Gogoi B, Samanta SK, et al. Ricinus Communis L. Fruit Extract Inhibits Migration/Invasion, Induces Apoptosis in Breast Cancer Cells and Arrests Tumor Progression In Vivo. Sci Rep (2019) 9(1):14493. doi: 10.1038/s41598-019-50769-x
93. Choi W, Jung H, Kim K, Lee S, Yoon S, Park J, et al. Rhus Verniciflua Stokes Against Advanced Cancer: A Perspective From the Korean Integrative Cancer Center. J BioMed Biotechnol (2012) 2012:874276. doi: 10.1155/2012/874276
94. Lee JO, Moon JW, Lee SK, Kim SM, Kim N, Ko SG, et al. Rhus Verniciflua Extract Modulates Survival of MCF-7 Breast Cancer Cells Through the Modulation of AMPK-Pathway. Biol Pharm Bull (2014) 37(5):794–801. doi: 10.1248/bpb.b13-00893
95. Köhne CH, Bruce C, Folprecht G, Audisio R. Role of New Agents in the Treatment of Colorectal Cancer. Surg Oncol (2004) 13(2-3):75–81. doi: 10.1016/j.suronc.2004.09.004
96. Leaver CA, Yuan H, Wallen GR. Apoptotic Activities of Sanguinaria Canadensis: Primary Human Keratinocytes, C-33A, and Human Papillomavirus HeLa Cervical Cancer Lines. Integr Med (Encinitas) (2018) 17(1):32–7.
97. Gazak R, Walterova D, Kren V. Silybin and Silymarin - New and Emerging Applications in Medicine. Curr Med Chem (2007) 14(3):315–38. doi: 10.2174/092986707779941159
98. Jin BJ, Hyung JJ, Jae HP, Sun HL, Jeong RL, Hee KL, et al. Cancer-Preventive Peptide Lunasin From Solanum Nigrum L. Inhibits Acetylation of Core Histones H3 and H4 and Phosphorylation of Retinoblastoma Protein (Rb). J Agric Food Chem (2007) 55(26):10707–13. doi: 10.1021/jf072363p
99. Son YO, Kim J, Lim JC, Chung Y, Chung GH, Lee JC. Ripe Fruits of Solanum Nigrum L. Inhibits Cell Growth and Induces Apoptosis in MCF-7 Cells. Food Chem Toxicol (2003) 41(10):1421–8. doi: 10.1016/S0278-6915(03)00161-3
100. Bhagya N, Chandrashekar KR. Tetrandrine and Cancer – An Overview on the Molecular Approach. BioMed Pharmacother (2018) 97:624–32. doi: 10.1016/j.biopha.2017.10.116
101. Kingston DG. Taxol, an Exciting Anticancer Drug From Taxus Brevifolia. Hum Med Agents Plants (1993) 534(10):138–48. doi: 10.1021/bk-1993-0534.ch010
102. Gautam N, Mantha AK, Mittal S. Essential Oils and Their Constituents as Anticancer Agents: A Mechanistic View. BioMed Res Int (2014) 2014:154106. doi: 10.1155/2014/154106
103. Keefover-Ring K, Thompson JD. YBL. Beyond Six Scents: Defining a Seventh Thymus Vulgaris Chemotype New to Southern France by Ethanol Extraction. Flavours Fragr J (2009) 24(3):117–22. doi: 10.1002/ffj.1921
104. Abaza MSI, Orabi KY, Al-Quattan E, Al-Attiyah RJ. Growth Inhibitory and Chemo-Sensitization Effects of Naringenin, a Natural Flavanone Purified From Thymus Vulgaris, on Human Breast and Colorectal Cancer. Cancer Cell Int (2015) 15(1):1–19. doi: 10.1186/s12935-015-0194-0
105. Lee YJ, Song K, Cha SH, Cho S, Kim YS, Park Y. Sesquiterpenoids From Tussilago Farfara Flower Bud Extract for the Eco-Friendly Synthesis of Silver and Gold Nanoparticles Possessing Antibacterial and Anticancer Activities. Nanomaterials (2019) 9(6):819. doi: 10.3390/nano9060819
106. Esposito S, Bianco A, Russo R, Di Maro A, Isernia C, Pedone PV. Therapeutic Perspectives of Molecules From Urtica Dioica Extracts for Cancer Treatment. Molecules (2019) 24(15):2753. doi: 10.3390/molecules24152753
107. Yousefnia S, Naseri D, Seyed Forootan F, Tabatabaeian M, Moattar F, Ghafghazi T, et al. Suppressive Role of Viola Odorata Extract on Malignant Characters of Mammosphere-Derived Breast Cancer Stem Cells. Clin Transl Oncol (2020) 22(9):1619–34. doi: 10.1007/s12094-020-02307-9
108. Kienle GS, Glockmann A, Schink M, Kiene H. Viscum Album L. Extracts in Breast and Gynaecological Cancers: A Systematic Review of Clinical and Preclinical Research. J Exp Clin Cancer Res (2009) 28(1):79. doi: 10.1186/1756-9966-28-79
109. Balea ŞS, Pârvu AE, Pârvu M, Vlase L, Dehelean CA, Pop TI. Antioxidant, Anti-Inflammatory and Antiproliferative Effects of the Vitis Vinifera L. Var. Fetească Neagră and Pinot Noir Pomace Extracts. Front Pharmacol (2020) 11:990. doi: 10.3389/fphar.2020.00990
110. Habib SHM, Makpol S, Hamid NAA, Das S, Ngah WZW, Yusof YAM. Ginger Extract (Zingiber Officinale) has Anti-Cancer and Anti-Inflammatory Effects on Ethionine-Induced Hepatoma Rats. Clinics (2008) 63(6):807–13. doi: 10.1590/S1807-59322008000600017
111. Rowland I, Gibson G, Heinken A, Scott K, Swann J, Thiele I, et al. Gut Microbiota Functions: Metabolism of Nutrients and Other Food Components. Eur J Nutr (2018) 57(1):1–24. doi: 10.1007/s00394-017-1445-8
112. Zhang X, Han Y, Huang W, Jin M, Gao Z. The Influence of the Gut Microbiota on the Bioavailability of Oral Drugs. Acta Pharm Sin B (2021) 11(7):1789–812. doi: 10.1016/j.apsb.2020.09.013
113. Martínez-Ballesta Mc, Gil-Izquierdo Á, García-Viguera C, Domínguez-Perles R. Nanoparticles and Controlled Delivery for Bioactive Compounds: Outlining Challenges for New “Smart-Foods” for Health. Foods (Basel Switzerland) (2018) 7(5):72. doi: 10.3390/foods7050072
114. Weaver CM, Miller JW. Challenges in Conducting Clinical Nutrition Research. Nutr Rev (2017) 75(7):491–9. doi: 10.1093/nutrit/nux026
115. Benet LZ, Hosey CM, Ursu O, Oprea TI. BDDCS, the Rule of 5 and Drugability. Adv Drug Deliv Rev (2016) 101:89–98. doi: 10.1016/j.addr.2016.05.007
116. Karlsson JE, Heddle C, Rozkov A, Rotticci-Mulder J, Tuvesson O, Hilgendorf C, et al. High-Activity P-Glycoprotein, Multidrug Resistance Protein 2, and Breast Cancer Resistance Protein Membrane Vesicles Prepared From Transiently Transfected Human Embryonic Kidney 293-Epstein-Barr Virus Nuclear Antigen Cells. Drug Metab Dispos (2010) 38(4):705–14. doi: 10.1124/dmd.109.028886
117. Vasiliou V, Vasiliou K, Nebert DW. Human ATP-Binding Cassette (ABC) Transporter Family. Hum Genomics (2009) 3(3):281–90. doi: 10.1186/1479-7364-3-3-281
118. Wen H, Jung H, Li X. Drug Delivery Approaches in Addressing Clinical Pharmacology-Related Issues: Opportunities and Challenges. AAPS J (2015) 17(6):1327–40. doi: 10.1208/s12248-015-9814-9
119. Lippman SM, Klein EA, Goodman PJ, Lucia MS, Thompson IM, Ford LG, et al. Effect of Selenium and Vitamin E on Risk of Prostate Cancer and Other Cancers: The Selenium and Vitamin E Cancer Prevention Trial (SELECT). JAMA (2009) 301(1):39–51. doi: 10.1001/jama.2008.864
120. Kesarwani K, Gupta R, Mukerjee A. Bioavailability Enhancers of Herbal Origin: An Overview. Asian Pac J Trop Biomed (2013) 3(4):253–66. doi: 10.1016/S2221-1691(13)60060-X
121. De Santi C, Pietrabissa A, Spisni R, Mosca F, Pacifici GM. Sulphation of Resveratrol, a Natural Product Present in Grapes and Wine, in the Human Liver and Duodenum. Xenobiotica (2000) 30(6):609–17. doi: 10.1080/004982500406435
122. Lee J-A, Ha SK, Cho E, Choi I. Resveratrol as a Bioenhancer to Improve Anti-Inflammatory Activities of Apigenin. Nutrients (2015) 7(11):9650–61. doi: 10.3390/nu7115485
123. Li Y, Yao J, Han C, Yang J, Chaudhry MT, Wang S, et al. Quercetin, Inflammation and Immunity. Nutrients (2016) 8(3):167. doi: 10.3390/nu8030167
124. Rajalakshmi S, Vyawahare N, Pawar A, Mahaparale P, Chellampillai B. Current Development in Novel Drug Delivery Systems of Bioactive Molecule Plumbagin. Artif Cells Nanomed Biotechnol (2018) 46(sup1):209–18. doi: 10.1080/21691401.2017.1417865
125. Yun YH, Lee BK, Park K. Controlled Drug Delivery: Historical Perspective for the Next Generation. J Control Release (2015) 219:2–7. doi: 10.1016/j.jconrel.2015.10.005
126. Rahman HS, Othman HH, Hammadi NI, Yeap SK, Amin KM, Abdul Samad N, et al. Novel Drug Delivery Systems for Loading of Natural Plant Extracts and Their Biomedical Applications. Int J Nanomed (2020) 15:2439–83. doi: 10.2147/IJN.S227805
127. Chavda VP. Nanotherapeutics and Nanobiotechnology. In: Applications of Targeted Nano Drugs and Delivery Systems. Cambridge, MA: Elsevier (2019). p. 1–13. doi: 10.1016/B978-0-12-814029-1.00001-6
128. Chavda VP, Thomas SBT-A of TND and DS. Chapter 4 - Nanobased Nano Drug Delivery: A Comprehensive Review. In: Mohapatra SS, Ranjan S, Dasgupta N, Mishra RK, editors. Micro and Nano Technologies. Cambridge, MA: Elsevier (2019). p. 69–92. doi: 10.1016/B978-0-12-814029-1.00004-1
129. Yoo J, Park C, Yi G, Lee D, Koo H. Active Targeting Strategies Using Biological Ligands for Nanoparticle Drug Delivery Systems. Cancers (Basel) (2019) 11(5):640. doi: 10.3390/cancers11050640
130. Goktas Z, Zu Y, Abbasi M, Galyean S, Wu D, Fan Z, et al. Recent Advances in Nanoencapsulation of Phytochemicals to Combat Obesity and Its Comorbidities. J Agric Food Chem (2020) 68(31):8119–31. doi: 10.1021/acs.jafc.0c00131
131. Bozzuto G, Molinari A. Liposomes as Nanomedical Devices. Int J Nanomed (2015) 10:975–99. doi: 10.2147/IJN.S68861
132. Akbarzadeh A, Rezaei-Sadabady R, Davaran S, Joo SW, Zarghami N, Hanifehpour Y, et al. Liposome: Classification, Preparation, and Applications. Nanoscale Res Lett (2013) 8(1):102. doi: 10.1186/1556-276X-8-102
133. Bardania H, Shojaosadati SA, Kobarfard F, Morshedi D, Aliakbari F, Tahoori MT, et al. RGD-Modified Nano-Liposomes Encapsulated Eptifibatide With Proper Hemocompatibility and Cytotoxicity Effect. Iran J Biotechnol (2019) 17(2):e2008–8. doi: 10.21859/ijb.2008
134. Panahi Y, Farshbaf M, Mohammadhosseini M, Mirahadi M, Khalilov R, Saghfi S, et al. Recent Advances on Liposomal Nanoparticles: Synthesis, Characterization and Biomedical Applications. Artif Cells Nanomed Biotechnol (2017) 45(4):788–99. doi: 10.1080/21691401.2017.1282496
135. Noriega-Luna B, Godínez LA, Rodríguez FJ, Rodríguez A, Zaldívar-Lelo De Larrea G, Sosa-Ferreyra CF, et al. Applications of Dendrimers in Drug Delivery Agents, Diagnosis, Therapy, and Detection. J Nanomater (2014) 2014:1–35. doi: 10.1155/2014/507273
136. Kaur A, Jain K, Mehra NK, Jain NK. Development and Characterization of Surface Engineered PPI Dendrimers for Targeted Drug Delivery. Artif Cells Nanomed Biotechnol (2017) 45(3):414–25. doi: 10.3109/21691401.2016.1160912
137. Yousefi M, Narmani A, Jafari SM. Dendrimers as Efficient Nanocarriers for the Protection and Delivery of Bioactive Phytochemicals. Adv Colloid Interf Sci (2020) 278:102125. doi: 10.1016/j.cis.2020.102125
138. Chavda VP. Niosome: A Vesicular Weapon for Targeted and Controlled Drug Delivery. Indian J Nov Drug Deliv (2016) 8(3):133–56. doi: 10.22270/jddt.v11i1.4479
139. Barani M, Mirzaei M, Torkzadeh-Mahani M, Adeli-sardou M. Evaluation of Carum-Loaded Niosomes on Breast Cancer Cells: Physicochemical Properties, In Vitro Cytotoxicity, Flow Cytometric, DNA Fragmentation and Cell Migration Assay. Sci Rep (2019) 9(1):7139. doi: 10.1038/s41598-019-43755-w
140. Jhaveri AM, Torchilin VP. Multifunctional Polymeric Micelles for Delivery of Drugs and siRNA. Front Pharmacol (2014) 5:77. doi: 10.3389/fphar.2014.00077
141. Majumder N, Das N G, Das SK. Polymeric Micelles for Anticancer Drug Delivery. Ther Deliv (2020) 11(10):613–35. doi: 10.4155/tde-2020-0008
142. Abedanzadeh M, Salmanpour M, Farjadian F, Mohammadi S, Tamaddon AM. Curcumin Loaded Polymeric Micelles of Variable Hydrophobic Lengths by RAFT Polymerization: Preparation and in-Vitro Characterization. J Drug Deliv Sci Technol (2020) 58:101793. doi: 10.1016/j.jddst.2020.101793
143. Hamad Farah F. Magnetic Microspheres: A Novel Drug Delivery System. J Anal Pharm Res (2016) 3(4):14–12. doi: 10.15406/japlr.2016.03.00067
144. Taherkhani A, Fazli H, Taherkhani F. Application of Janus Magnetic Nanoparticle Fe3O4@SiN Functionalized With Beta-Cyclodextrin in Thymol Drug Delivery Procedure: An In Vitro Study. Appl Organomet Chem (2021) 35(11):e6399. doi: 10.1002/aoc.6399
145. Kotta S, Khan AW, Pramod K, Ansari SH, Sharma RK, Ali J. Exploring Oral Nanoemulsions for Bioavailability Enhancement of Poorly Water-Soluble Drugs. Expert Opin Drug Deliv (2012) 9(5):585–98. doi: 10.1517/17425247.2012.668523
146. Wang J, Hu X, Xiang D. Nanoparticle Drug Delivery Systems: An Excellent Carrier for Tumor Peptide Vaccines. Drug Deliv (2018) 25(1):1319–27. doi: 10.1080/10717544.2018.1477857
147. Jamali SN, Assadpour E, Jafari SM. Formulation and Application of Nanoemulsions for Nutraceuticals and Phytochemicals. Curr Med Chem (2020) 27(18):3079–95. doi: 10.2174/0929867326666190620102820
148. Chavda VP, Shah DB. A Review on Novel Emulsification Technique: A Nanoemulsion. Res Rev J Pharmacol Toxicol Stud (2017) 5(1):29–38.
149. Chavda VP, Shah DB, Domadiya K. Microemulsion: Novel Carrier for Drug Delivery. Trends Drug Deliv (2016) 3(1):1–18.
150. Moura RP, Pacheco C, Pêgo AP, des Rieux A, Sarmento B. Lipid Nanocapsules to Enhance Drug Bioavailability to the Central Nervous System. J Control Rel (2020) 322:390–400. doi: 10.1016/j.jconrel.2020.03.042
151. Chavda VP. Chapter 4 Nanobased Nano Drug Delivery A Comprehensive Review. In: Micro and Nano Technologies. United States: Elsevier (2021). p. 69–92. doi: 10.1016/B978-0-12-814029-1.00004-1
152. Maleki Dizaj S, Mennati A, Jafari S, Khezri K, Adibkia K. Antimicrobial Activity of Carbon-Based Nanoparticles. Adv Pharm Bull (2015) 5(1):19–23. doi: 10.5681/apb.2015.003"10.5681/apb.2015.003
153. Maiti D, Tong X, Mou X, Yang K. Carbon-Based Nanomaterials for Biomedical Applications: A Recent Study. Front Pharmacol (2019) 9. doi: 10.3389/fphar.2018.01401
154. Begines B, Ortiz T, Pérez-Aranda M, Martínez G, Merinero M, Argüelles-Arias F, et al. Polymeric Nanoparticles for Drug Delivery: Recent Developments and Future Prospects. Nanomater (Basel Switzerland) (2020) 10(7):1403. doi: 10.3390/nano10071403
155. Pan J, Rostamizadeh K, Filipczak N, Torchilin VP. Polymeric Co-Delivery Systems in Cancer Treatment: An Overview on Component Drugs’ Dosage Ratio Effect. Molecules (2019) 24(6):1035. doi: 10.3390/molecules24061035
156. Gigliobianco MR, Casadidio C, Censi R, Di Martino P. Nanocrystals of Poorly Soluble Drugs: Drug Bioavailability and Physicochemical Stability. Pharmaceutics (2018) 10(3):134. doi: 10.3390/pharmaceutics10030134
157. Sivadasan D, Sultan MH, Madkhali O, Almoshari Y, Thangavel N. Polymeric Lipid Hybrid Nanoparticles (PLNs) as Emerging Drug Delivery Platform—A Comprehensive Review of Their Properties, Preparation Methods, and Therapeutic Applications. Pharmaceutics (2021) 13(8):1291. doi: 10.3390/pharmaceutics13081291
158. de Souza A, Marins DSS, Mathias SL, Monteiro LM, Yukuyama MN, Scarim CB, et al. Promising Nanotherapy in Treating Leishmaniasis. Int J Pharm (2018) 547(1–2):421–31. doi: 10.1016/j.ijpharm.2018.06.018
159. Bhattacharyya S, Kudgus RA, Bhattacharya R, Mukherjee P. Inorganic Nanoparticles in Cancer Therapy. Pharm Res (2011) 28(2):237–59. doi: 10.1007/s11095-010-0318-0
160. Yaqoob AA, Ahmad H, Parveen T, Ahmad A, Oves M, Ismail IMI, et al. Recent Advances in Metal Decorated Nanomaterials and Their Various Biological Applications: A Review. Front Chem (2020) 8:341. doi: 10.3389/fchem.2020.00341
161. Vasile C, Pamfil D, Stoleru E, Baican M. New Developments in Medical Applications of Hybrid Hydrogels Containing Natural Polymers. Molecules (2020) 25(7):1539. doi: 10.3390/molecules25071539
162. Ulldemolins A, Seras-Franzoso J, Andrade F, Rafael D, Abasolo I, Gener P, et al. Perspectives of Nano-Carrier Drug Delivery Systems to Overcome Cancer Drug Resistance in the Clinics. Cancer Drug Resist (2021) 4(1):44–68. doi: 10.20517/cdr.2020.59
163. Duong TT, Isomäki A, Paaver U, Laidmäe I, Tõnisoo A, Yen TTH, et al. Nanoformulation and Evaluation of Oral Berberine-Loaded Liposomes. Molecules (2021) 26(9):2591. doi: 10.3390/molecules26092591
164. Macchione MA, Aristizabal Bedoya D, Figueroa FN, Muñoz-Fernández MÁ, Strumia MC. Nanosystems Applied to HIV Infection: Prevention and Treatments. Int J Mol Sci (2020) 21(22):8647. doi: 10.3390/ijms21228647
165. Nitta SK, Numata K. Biopolymer-Based Nanoparticles for Drug/Gene Delivery and Tissue Engineering. Int J Mol Sci (2013) 14(1):1629–54. doi: 10.3390/ijms14011629
166. Shah SA, Sohail M, Khan S, Minhas MU, de Matas M, Sikstone V, et al. Biopolymer-Based Biomaterials for Accelerated Diabetic Wound Healing: A Critical Review. Int J Biol Macromol (2019) 139:975–93. doi: 10.1016/j.ijbiomac.2019.08.007
167. Sebak S, Mirzaei M, Malhotra M, Kulamarva A, Prakash S. Human Serum Albumin Nanoparticles as an Efficient Noscapine Drug Delivery System for Potential Use in Breast Cancer: Preparation and In Vitro Analysis. Int J Nanomed (2010) :5:525–32. doi: 10.2147/IJN.S10443
168. Sanna V, Roggio AM, Siliani S, Piccinini M, Marceddu S, Mariani A, et al. Development of Novel Cationic Chitosan-and Anionic Alginate-Coated Poly(D,L-Lactide-Co-Glycolide) Nanoparticles for Controlled Release and Light Protection of Resveratrol. Int J Nanomed (2012) 7:5501–16. doi: 10.2147/IJN.S36684
169. Aqil F, Munagala R, Jeyabalan J, Vadhanam MV. Bioavailability of Phytochemicals and its Enhancement by Drug Delivery Systems. Cancer Lett (2013) 334(1):133–41. doi: 10.1016/j.canlet.2013.02.032
170. Wang C, Feng L, Yang X, Wang F, Lu W. Folic Acid-Conjugated Liposomal Vincristine for Multidrug Resistant Cancer Therapy. Asian J Pharm Sci (2013) 8(2):118–27. doi: 10.1016/j.ajps.2013.07.015
171. Caldeira de Araújo Lopes S, Vinícius Melo Novais M, Salviano Teixeira C, Honorato-Sampaio K, Tadeu Pereira M, Ferreira LAM, et al. Preparation, Physicochemical Characterization, and Cell Viability Evaluation of Long-Circulating and pH-Sensitive Liposomes Containing Ursolic Acid. Surguchov A, Editor. BioMed Res Int (2013) 2013:467147. doi: 10.1155/2013/467147
172. Xiao Z, Zhuang B, Zhang G, Li M, Jin Y. Pulmonary Delivery of Cationic Liposomal Hydroxycamptothecin and 5-Aminolevulinic Acid for Chemo-Sonodynamic Therapy of Metastatic Lung Cancer. Int J Pharm (2021) 601:120572. doi: 10.1016/j.ijpharm.2021.120572
173. Mehta PP, Ghoshal D, Pawar AP, Kadam SS, Dhapte-Pawar VS. Recent Advances in Inhalable Liposomes for Treatment of Pulmonary Diseases: Concept to Clinical Stance. J Drug Deliv Sci Technol (2020) 56:101509. doi: 10.1016/j.jddst.2020.101509
174. Tzogani K, Penttilä K, Lapveteläinen T, Hemmings R, Koenig J, Freire J, et al. EMA Review of Daunorubicin and Cytarabine Encapsulated in Liposomes (Vyxeos, CPX-351) for the Treatment of Adults With Newly Diagnosed, Therapy-Related Acute Myeloid Leukemia or Acute Myeloid Leukemia With Myelodysplasia-Related Changes. Oncologist (2020) 25(9):e1414–20. doi: 10.1634/theoncologist.2019-0785
175. Chen R-P, Chavda VP, Patel AB, Chen Z-S. Phytochemical Delivery Through Transferosome (Phytosome): An Advanced Transdermal Drug Delivery for Complementary Medicines. Front Pharmacol (2022) 13:850862. doi: 10.3389/fphar.2022.850862
176. Opatha SAT, Titapiwatanakun V, Chutoprapat R. Transfersomes: A Promising Nanoencapsulation Technique for Transdermal Drug Delivery. Pharmaceutics (2020) 12(9):855. doi: 10.3390/pharmaceutics12090855
177. Raafat KM, El-Zahaby SA. Niosomes of Active Fumaria Officinalis Phytochemicals: Antidiabetic, Antineuropathic, Anti-Inflammatory, and Possible Mechanisms of Action. Chin Med (United Kingdom) (2020) 15(1):1–22. doi: 10.1186/s13020-020-00321-1
178. Agarwal S, Mohamed MS, Raveendran S, Rochani AK, Maekawa T, Kumar DS. Formulation, Characterization and Evaluation of Morusin Loaded Niosomes for Potentiation of Anticancer Therapy. RSC Adv (2018) 8(57):32621–36. doi: 10.1039/C8RA06362A
179. Mousazadeh N, Gharbavi M, Rashidzadeh H, Nosrati H, Danafar H, Johari B. Anticancer Evaluation of Methotrexate and Curcumin-Coencapsulated Niosomes Against Colorectal Cancer Cell Lines. Nanomedicine (2022) 17(4):201–17. doi: 10.2217/nnm-2021-0334
180. Imam SS, Alshehri S, Altamimi MA, Hussain A, Alyahya KH, Mahdi WA, et al. Formulation and Evaluation of Luteolin-Loaded Nanovesicles: In Vitro Physicochemical Characterization and Viability Assessment. ACS Omega (2022) 7(1):1048–56. doi: 10.1021/acsomega.1c05628
181. Hanafy NAN, El-Kemary M, Leporatti S. Micelles Structure Development as a Strategy to Improve Smart Cancer Therapy. Cancers (Basel) (2018) 10(7):238. doi: 10.3390/cancers10070238
182. Modi G, Pillay V, Choonara YE, Ndesendo VMK, du Toit LC, Naidoo D. Nanotechnological Applications for the Treatment of Neurodegenerative Disorders. Prog Neurobiol (2009) 88(4):272–85. doi: 10.1016/j.pneurobio.2009.05.002
183. Li Z, Zhang G, Luo Y, Gao Q, Wang J, Chen C, et al. In Vivo Effect of Magnetic Microspheres Loaded With E2-A in the Treatment of Alveolar Echinococcosis. Sci Rep (2020) 10(1):12589. doi: 10.1038/s41598-020-69484-z
184. Xiao Y, Hong H, Javadi A, Jonathan W Engle WX, Yang Y, Zhang Y, et al. Multifunctional Unimolecular Micelles for Cancer-Targeted Drug Delivery and Positron Emission Tomography Imaging. Biomaterials (2012) 33(11):3071–82. doi: 10.1016/j.biomaterials.2011.12.030
185. Kumari A, Yadav SK, Yadav SC. Biodegradable Polymeric Nanoparticles Based Drug Delivery Systems. Colloids Surf B Biointerf (2010) 75(1):1–18. doi: 10.1016/j.colsurfb.2009.09.001
186. Emami J, Kazemi M, Hasanzadeh F, Minaiyan M, Mirian M, Lavasanifar A. Novel pH-Triggered Biocompatible Polymeric Micelles Based on Heparin–α-Tocopherol Conjugate for Intracellular Delivery of Docetaxel in Breast Cancer. Pharm Dev Technol (2020) 25(4):492–509. doi: 10.1080/10837450.2019.1711395
187. Anselmo AC, Mitragotri S. An Overview of Clinical and Commercial Impact of Drug Delivery Systems. J Control Rel (2014) :190:15–28. doi: 10.1016/j.jconrel.2014.03.053
188. Enriquez GG, Rizvi SAA, D’Souza MJ, Do DP. Formulation and Evaluation of Drug-Loaded Targeted Magnetic Microspheres for Cancer Therapy. Int J Nanomed (2013) 8:1393–402. doi: 10.2147/IJN.S43479
189. Wang X, Jing X, Zhang X, Liu Q, Liu J, Song D, et al. A Versatile Platform of Magnetic Microspheres Loaded With Dual-Anticancer Drugs for Drug Release. Mater Chem Phys (2016) 177:213–9. doi: 10.1016/j.matchemphys.2016.04.021
190. Gugleva V, Ivanova N, Sotirova Y, Andonova V. Dermal Drug Delivery of Phytochemicals With Phenolic Structure via Lipid-Based Nanotechnologies. Pharmaceuticals (2021) 14(9):837. doi: 10.3390/ph14090837
191. Shakhwar S, Darwish R, Kamal MM, Nazzal S, Pallerla S, Abu Fayyad A. Development and Evaluation of Paclitaxel Nanoemulsion for Cancer Therapy. Pharm Dev Technol (2020) 25(4):510–6. doi: 10.1080/10837450.2019.1706564
192. Leite CBS, Coelho JM, Ferreira-Nunes R, Gelfuso GM, Durigan JLQ, Azevedo RB, et al. Phonophoretic Application of a Glucosamine and Chondroitin Nanoemulsion for Treatment of Knee Chondropathies. Nanomedicine (2020) 15(7):647–59. doi: 10.2217/nnm-2019-0317
193. Chrastina A, Baron VT, Abedinpour P, Rondeau G, Welsh J, Borgström P. Plumbagin-Loaded Nanoemulsion Drug Delivery Formulation and Evaluation of Antiproliferative Effect on Prostate Cancer Cells. BioMed Res Int (2018) 2018:9035452. doi: 10.1155/2018/9035452
194. Rondeau G, Abedinpour P, Chrastina A, Pelayo J, Borgstrom P, Welsh J. Differential Gene Expression Induced by Anti-Cancer Agent Plumbagin Is Mediated by Androgen Receptor in Prostate Cancer Cells. Sci Rep (2018) 8(1):2694. doi: 10.1038/s41598-018-20451-9
195. Fofaria NM, Qhattal HSS, Liu X, Srivastava SK. Nanoemulsion Formulations for Anti-Cancer Agent Piplartine–Characterization, Toxicological, Pharmacokinetics and Efficacy Studies. Int J Pharm 498(1–2):12–22. doi: 10.1016/j.ijpharm.2015.11.045
196. Kamaly N, Yameen B, Wu J, Farokhzad OC. Degradable Controlled-Release Polymers and Polymeric Nanoparticles: Mechanisms of Controlling Drug Release. Chem Rev (2016) 116(4):2602–63. doi: 10.1021/acs.chemrev.5b00346
197. Javed Iqbal M, Quispe C, Javed Z, Sadia H, Qadri QR, Raza S, et al. Nanotechnology-Based Strategies for Berberine Delivery System in Cancer Treatment: Pulling Strings to Keep Berberine in Power. Front Mol Biosci (2021) 7:624494. doi: 10.3389/fmolb.2020.624494
198. Ansari SH, Islam F, Sameem M. Influence of Nanotechnology on Herbal Drugs: A Review. J Adv Pharm Technol Res (2012) 3(3):142–6. doi: 10.4103/2231-4040.101006
199. Gugulothu D, Kulkarni A, Patravale V, Dandekar P. PH-Sensitive Nanoparticles of Curcumin-Celecoxib Combination: Evaluating Drug Synergy in Ulcerative Colitis Model. J Pharm Sci (2014) 103(2):687–96. doi: 10.1002/jps.23828
200. Ganesan RM, Gurumallesh Prabu H. Synthesis of Gold Nanoparticles Using Herbal Acorus Calamus Rhizome Extract and Coating on Cotton Fabric for Antibacterial and UV Blocking Applications. Arab J Chem (2019) 12(8):2166–74. doi: 10.1016/j.arabjc.2014.12.017
201. Hojjati-Najafabadi A, Davar F, Enteshari Z, Hosseini-Koupaei M. Antibacterial and Photocatalytic Behaviour of Green Synthesis of Zn0.95Ag0.05O Nanoparticles Using Herbal Medicine Extract. Ceram Int (2021) 47(22):31617–24. doi: 10.1016/j.ceramint.2021.08.042
202. Alsaab HO, Alghamdi MS, Alotaibi AS, Alzhrani R, Alwuthaynani F, Althobaiti YS, et al. Progress in Clinical Trials of Photodynamic Therapy for Solid Tumors and the Role of Nanomedicine. Cancers (Basel) (2020) 12(10):1–26. doi: 10.3390/cancers12102793
203. Das S, Das J, Samadder A, Paul A, Khuda-Bukhsh AR. Efficacy of PLGA-Loaded Apigenin Nanoparticles in Benzo[a]pyrene and Ultraviolet-B Induced Skin Cancer of Mice: Mitochondria Mediated Apoptotic Signalling Cascades. Food Chem Toxicol (2013) 62:670–80. doi: 10.1016/j.fct.2013.09.037
204. Chen Y, Zheng X-L, Fang D-L, Yang Y, Zhang J-K, Li H-L, et al. Dual Agent Loaded PLGA Nanoparticles Enhanced Antitumor Activity in a Multidrug-Resistant Breast Tumor Eenograft Model. Int J Mol Sci (2014) 15(2):2761–72. doi: 10.3390/ijms15022761
205. Ersoz M, Erdemir A, Derman S, Arasoglu T, Mansuroglu B. Quercetin-Loaded Nanoparticles Enhance Cytotoxicity and Antioxidant Activity on C6 Glioma Cells. Pharm Dev Technol (2020) 25(6):757–66. doi: 10.1080/10837450.2020.1740933
206. Gera M, Kim N, Ghosh M, Sharma N, Huynh DL, Chandimali N, et al. Synthesis and Evaluation of the Antiproliferative Efficacy of BRM270 Phytocomposite Nanoparticles Against Human Hepatoma Cancer Cell Lines. Mater Sci Eng C Mater Biol Appl (2019) 97:166–76. doi: 10.1016/j.msec.2018.11.055
207. Mukherjee A, Waters AK, Kalyan P, Achrol AS, Kesari S, Yenugonda VM. Lipid-Polymer Hybrid Nanoparticles as a Nextgeneration Drug Delivery Platform: State of the Art, Emerging Technologies, and Perspectives. Int J Nanomed (2019) 14:1937–52. doi: 10.2147/IJN.S198353
208. Kumar Sarwa K, Rudrapal M, Mazumder B. Topical Ethosomal Capsaicin Attenuates Edema and Nociception in Arthritic Rats. Drug Deliv (2015) 22(8):1043–52. doi: 10.3109/10717544.2013.861041
209. Ma P, Mumper RJ. Paclitaxel Nano-Delivery Systems: A Comprehensive Review. J Nanomed Nanotechnol (2013) 4(2):1000164. doi: 10.4172/2157-7439.1000164
210. Wei T, Chen C, Liu J, Liu C, Posocco P, Liu X, et al. Anticancer Drug Nanomicelles Formed by Self-Assembling Amphiphilic Dendrimer to Combat Cancer Drug Resistance. Proc Natl Acad Sci (2015) 112(10):2978 LP–2983. doi: 10.1073/pnas.1418494112
211. Sadat Khadem F, Es-Haghi A, Homayouni Tabrizi M, Shabestarian H. The Loaded Ferula Assa-Foetida Seed Essential Oil in Solid Lipid Nanoparticles (FSEO-SLN) as the Strong Apoptosis Inducer Agents in Human NTERA-2 Embryocarcinoma Cells. Mater Technol (2021) 36(7):1–9. doi: 10.1080/10667857.2021.1924436
212. Ramesh N, Mandal AKA. Pharmacokinetic, Toxicokinetic, and Bioavailability Studies of Epigallocatechin-3-Gallate Loaded Solid Lipid Nanoparticle in Rat Model. Drug Dev Ind Pharm (2019) 45(9):1506–14. doi: 10.1080/03639045.2019.1634091
213. Jogi H, Maheshwari R, Raval N, Kuche K, Tambe V, Mak KK, et al. Carbon Nanotubes in the Delivery of Anticancer Herbal Drugs. Nanomedicine (2018) 13(10):1187–220. doi: 10.2217/nnm-2017-0397
214. Banica F, Bungau S, Tit DM, Behl T, Otrisal P, Nechifor AC, et al. Determination of the Total Polyphenols Content and Antioxidant Activity of Echinacea Purpurea Extracts Using Newly Manufactured Glassy Carbon Electrodes Modified With Carbon Nanotubes. Processes (2020) 8(7):833. doi: 10.3390/pr8070833
215. Nasseri MA, Keshtkar H, Kazemnejadi M, Allahresani A. Phytochemical Properties and Antioxidant Activity of Echinops Persicus Plant Extract: Green Synthesis of Carbon Quantum Dots From the Plant Extract. SN Appl Sci (2020) 2(4):670. doi: 10.1007/s42452-020-2466-0
216. Hashemi SF, Tasharrofi N, Saber MM. Green Synthesis of Silver Nanoparticles Using Teucrium Polium Leaf Extract and Assessment of Their Antitumor Effects Against MNK45 Human Gastric Cancer Cell Line. J Mol Struct (2020) 1208:127889. doi: 10.1016/j.molstruc.2020.127889
217. Mughees M, Wajid S, Samim M. Cytotoxic Potential of Artemisia Absinthium Extract Loaded Polymeric Nanoparticles Against Breast Cancer Cells: Insight Into the Protein Targets. Int J Pharm (2020) 586(June):119583. doi: 10.1016/j.ijpharm.2020.119583
218. Ribeiro AF, Santos JF, Mattos RR, Barros EGO, Nasciutti LE, Cabral LM, et al. Characterization and In Vitro Antitumor Activity of Polymeric Nanoparticles Loaded With Uncaria Tomentosa Extract. Acad Bras Cienc (2020) 92(1):e20190336. doi: 10.1590/0001-3765202020190336
219. Khan T, Gurav P. PhytoNanotechnology: Enhancing Delivery of Plant Based Anti-Cancer Drugs. Front Pharmacol (2018) 8:1002. doi: 10.3389/fphar.2017.01002
220. Madaan K, Lather V, Pandita D. Evaluation of Polyamidoamine Dendrimers as Potential Carriers for Quercetin, a Versatile Flavonoid. Drug Deliv (2016) 23(1):254–62. doi: 10.3109/10717544.2014.910564
221. Singh V, Sahebkar A, Kesharwani P. Poly (Propylene Imine) Dendrimer as an Emerging Polymeric Nanocarrier for Anticancer Drug and Gene Delivery. Eur Polym J (2021) 158:110683. doi: 10.1016/j.eurpolymj.2021.110683
222. Singh A, Garg G, Sharma PK. Nanospheres: A Novel Approach for Targeted Drug Delivery System. Int J Pharm Sci Rev Res (2010) 5(3):84–8.
223. Hubenthal F. 1.13 - Noble Metal Nanoparticles: Synthesis and Optical Properties. In: Andrews DL, Scholes GD, Wiederrecht GPBT-CN and T, editors. Comprehensive Nanoscience and Nanotechnology. Amsterdam: Academic Press (2011). p. 375–435. doi: 10.1016/B978-0-12-374396-1.00034-9
224. Kuppusamy P, Yusoff MM, Maniam GP, Govindan N. Biosynthesis of Metallic Nanoparticles Using Plant Derivatives and Their New Avenues in Pharmacological Applications – An Updated Report. Saudi Pharm J (2016) 24(4):473–84. doi: 10.1016/j.jsps.2014.11.013
225. Liu G, Xu X, Jiang L, Ji H, Zhu F, Jin B, et al. Targeted Antitumor Mechanism of C-PC/CMC-CD55sp Nanospheres in HeLa Cervical Cancer Cells. Front Pharmacol (2020) 11(June):1–13. doi: 10.3389/fphar.2020.00906
226. Wei Q-Y, He K-M, Chen J-L, Xu Y-M, Lau ATY. Phytofabrication of Nanoparticles as Novel Drugs for Anticancer Applications. Molecules (2019) 24(23):4246. doi: 10.3390/molecules24234246
227. Burger KNJ, Staffhorst RWHM, de Vijlder HC, Velinova MJ, Bomans PH, Frederik PM, et al. Nanocapsules: Lipid-Coated Aggregates of Cisplatin With High Cytotoxicity. Nat Med (2002) 8(1):81–4. doi: 10.1038/nm0102-81
228. Kothamasu P, Kanumur H, Ravur N, Maddu C, Parasuramrajam R, Thangavel S. Nanocapsules: The Weapons for Novel Drug Delivery Systems. BioImpacts (2012) 2(2):71–81. doi: 10.5681/bi.2012.011
229. Aisida SO, Ugwoke E, Uwais A, Iroegbu C, Botha S, Ahmad I, et al. Incubation Period Induced Biogenic Synthesis of PEG Enhanced Moringa Oleifera Silver Nanocapsules and its Antibacterial Activity. J Polym Res (2019) 26(9):225. doi: 10.1007/s10965-019-1897-z
230. Osanloo M, Sedaghat MM, Sereshti H, Rahmani M, Landi FS, Amani A. Chitosan Nanocapsules of Tarragon Essential Oil With Low Cytotoxicity and Long-Lasting Activity as a Green Nano-Larvicide. J Nanostructures (2019) 9(4):723–35. doi: 10.22052/JNS.2019.04.014
231. Thi TTH, Suys EJA, Lee JS, Nguyen DH, Park KD, Truong NP. Lipid-Based Nanoparticles in the Clinic and Clinical Trials: From Cancer Nanomedicine to COVID-19 Vaccines. Vaccines (2021) 9(4):359. doi: 10.3390/vaccines9040359
232. Mardani R, Hamblin MR, Taghizadeh M, Banafshe HR, Nejati M, Mokhtari M, et al. Nanomicellar-Curcumin Exerts its Therapeutic Effects via Affecting Angiogenesis, Apoptosis, and T Cells in a Mouse Model of Melanoma Lung Metastasis. Pathol Res Pract (2020) 216(9):153082. doi: 10.1016/j.prp.2020.153082
233. Jeevanandam J, Barhoum A, Chan YS, Dufresne A, Danquah MK. Review on Nanoparticles and Nanostructured Materials: History, Sources, Toxicity and Regulations. Beilstein J Nanotechnol (2018) 9:1050–74. doi: 10.3762/bjnano.9.98
234. Gopinath V, MubarakAli D, Priyadarshini S, Priyadharsshini NM, Thajuddin N, Velusamy P. Biosynthesis of Silver Nanoparticles From Tribulus Terrestris and its Antimicrobial Activity: A Novel Biological Approach. Colloids Surf B Biointerf (2012) 96:69–74. doi: 10.1016/j.colsurfb.2012.03.023
235. Shameli K, Ahmad MB, Jaffar Al-Mulla EA, Ibrahim NA, Shabanzadeh P, Rustaiyan A, et al. Green Biosynthesis of Silver Nanoparticles Using Callicarpa Maingayi Stem Bark Extraction. Molecules (2012) 17(7):8506–17. doi: 10.3390/molecules17078506
236. Aboyewa JA, Sibuyi NRS, Meyer M, Oguntibeju OO. Green Synthesis of Metallic Nanoparticles Using Some Selected Medicinal Plants From Southern Africa and Their Biological Applications. Plants (2021) 10(9):1929. doi: 10.3390/plants10091929
237. Tremi I, Spyratou E, Souli M, Efstathopoulos EP, Makropoulou M, Georgakilas AG, et al. Requirements for Designing an Effective Metallic Nanoparticle (NP)-Boosted Radiation Therapy (RT). Cancers (Basel) (2021) 13(13):3185. doi: 10.3390/cancers13133185
238. Montazerabadi A, Beik J, Irajirad R, Attaran N, Khaledi S, Ghaznavi H, et al. Folate-Modified and Curcumin-Loaded Dendritic Magnetite Nanocarriers for the Targeted Thermo-Chemotherapy of Cancer Cells. Artif Cells nanomedicine Biotechnol (2019) 47(1):330–40. doi: 10.1080/21691401.2018.1557670
239. Tousi MS, Sepehri H, Khoee S, Farimani MM, Delphi L, Mansourizadeh F. Evaluation of Apoptotic Effects of mPEG-B-PLGA Coated Iron Oxide Nanoparticles as a Eupatorin Carrier on DU-145 and LNCaP Human Prostate Cancer Cell Lines. J Pharm Anal (2021) 11(1):108–21. doi: 10.1016/j.jpha.2020.04.002
240. Mamillapalli V, Atmakuri AM, Khantamneni P. Nanoparticles for Herbal Extracts. Asian J Pharm (2016) 10(2):S54–60. doi: 10.22377/ajp.v10i2.623
241. Muthuvinothini A, Stella S. Green Synthesis of Metal Oxide Nanoparticles and Their Catalytic Activity for the Reduction of Aldehydes. Process Biochem (2019) 77:48–56. doi: 10.1016/j.procbio.2018.12.001
242. Sun M, Wang T, Li L, Li X, Zhai Y, Zhang J, et al. The Application of Inorganic Nanoparticles in Molecular Targeted Cancer Therapy: EGFR Targeting. Front Pharmacol (2021) 12(July):1–15. doi: 10.3389/fphar.2021.702445
243. Chauhan P, Tyagi BK. Herbal Novel Drug Delivery Systems and Transfersomes. J Drug Deliv Ther (2018) 8(3):162–8. doi: 10.22270/jddt.v8i3.1772
244. Karthigeyan P, Biswa BK, Sanjaya KP, Das D, Tapas KP, et al. Lung Cancer Treatment Using Astragalus, Cisplatin and Vinorelbine-IN202241000705. (2022) (15-02-02022). Available at: https://patentscope.wipo.int/search/en/detail.jsf?docId=IN348662046&_cid=P10-L0JOBD-96201-1.
245. Khan MA. - A Novel Herbal Composition for Anticancer Activity-IN202111049427. (2021) (18-02-2022). Available at: https://patentscope.wipo.int/search/en/detail.jsf?docId=IN342290833&_cid=P10-L0JOJ9-00214-1.
246. Sumathi DR, Tamizharasi DS, Punitha S, Sivakumar DT. Enhanced Anticancer Activity of Quercetin-Loaded Tags Nanosuspension for Drug Impervious Mcf-7 Human Breast Cancer Cells-IN202141046188. (2021) (11-02-2022). Available at: https://patentscope.wipo.int/search/en/detail.jsf?docId=IN346038271&_cid=P10-L0JPH5-16373-1.
247. Sonali BD, Ziyaurrahman A, Kiran SB. Cytotoxic Herbal Silver Nanoparticles as a Remedy for Mammary Carcinoma-IN202021048696. (2020) (19-02-2022). Available at: https://patentscope.wipo.int/search/en/detail.jsf?docId=IN312323714&_cid=P10-L0JPNY-19493-1.
248. Walter MT. Non Invasive, Novel Poly Herbal Synergistic Formulation for the Effective Prevention and Management of Human Lung Cancer-IN202041023550. (2020) (19-02-2022). Available at: https://patentscope.wipo.int/search/en/detail.jsf?docId=IN296580467&_cid=P10-L0JPT2-21531-1.
249. Walter MT. Non Invasive, Novel Poly Herbal Synergistic Formulation for the Prevention and Management of Gastric Cancer-IN202041025649. (2020) (09-02-2022). Available at: https://patentscope.wipo.int/search/en/detail.jsf?docId=IN298658813&_cid=P11-L0JQ0O-79635-1.
250. Cardelli J, Ana-Maria D. Cancer Treatment Combination Composition Methods and Uses-US20170258929. (2017) (19-02-2022). Available at: https://patentscope.wipo.int/search/en/detail.jsf?docId=US203767547&_cid=P11-L0JQ5J-81563-1.
251. Laura AS, Peter PS, Lawrence H. Use of a Combination of a Curcuminoid and a Chemotherapeutic Agent for Use in Treatment of Glioblastoma-EP3144006. (2017) (17-02-2022). Available at: https://patentscope.wipo.int/search/en/detail.jsf?docId=EP193434896&_cid=P11-L0JQ9K-83371-1
252. Ming TS, Ho HO, Shing CH, Yuan SH, Jun-jen L. Stabilized High Drug Load Nanocarriers Methods for their Preparation and Use there of-US20170035701. (2017) (14-02-2022). https://patentscope.wipo.int/search/en/detail.jsf?docId=US192184121&_cid=P11-L0JQEY-85764-1
253. Xiyun Y, Kelong F, Minmin L, Wang F, Demin D, Zhang D, et al. Drug Carrier for Tumor-Specific Targeted Drug Delivery and Use Thereof-US20170189343. (2017) (21-02-2022). Available at: https://patentscope.wipo.int/search/en/detail.jsf?docId=US200469162&_cid=P11-L0JQKR-87893-1.
254. Pradeep KS, Armitage AP, Satishchandra BO. Curcumin Sophorolipid Complex-US20170224636. (2017) (24-02-2022). Available at: https://patentscope.wipo.int/search/en/detail.jsf?docId=US202131929&_cid=P11-L0JQOG-89324-1
255. Banerjee P, Mario RC, Priya RD, Szerszen A, Jimmie EF, et al. Activity Enhancing Curcumin Composition and Method of Use-US20160287533. (2016) (15-02-2022). Available at: https://patentscope.wipo.int/search/en/detail.jsf?docId=US178074760&_cid=P11-L0JQS0-90576-1.
256. Dae RA. Drug Carrier Having L-DNA Nanocage Structure-US20160287706. (2016) (17-02-2022). Available at: https://patentscope.wipo.int/search/en/detail.jsf?docId=US178074933&_cid=P11-L0JQV4-91752-1.
257. Alias JP, Kunmoth NJ. Curcumin Coated Magnetize Nanoparticles for Biomedical Application-US20140369938. (2014) (20-02-2022). Available at: https://patentscope.wipo.int/search/en/detail.jsf?docId=US128464340&_cid=P11-L0JQXJ-92835-1.
258. George K, Elena E. Multi-Responsive Targeting Drug Delivery Systems for Controlled-Release Pharmaceutical Formulation-US20160263221. (2016) (25-02-2022). Available at: https://patentscope.wipo.int/search/en/detail.jsf?docId=US177607376&_cid=P11-L0JR0S-93959-1.
259. Ganta S, Coleman T. Drug Delivery Nanoemulsion Systems-WO2016014337. (2016) (27-02-2022). Available at: https://patentscope.wipo.int/search/en/detail.jsf?docId=WO2016014337&_cid=P10-KSU8AB-96626-1
260. Cheng J, Tong R. Particulate Drug Delivery Method-US20150314006. (2015) (17-02-2022). Available at: https://patentscope.wipo.int/search/en/detail.jsf?docId=US152963728&_cid=P21-L0JR7Q-96854-1.
261. Ekor M. The Growing Use of Herbal Medicines: Issues Relating to Adverse Reactions and Challenges in Monitoring Safety. Front Pharmacol (2014) 4:177. doi: 10.3389/fphar.2013.00177
262. Yin S-Y, Wei W-C, Jian F-Y, Yang N-S. Therapeutic Applications of Herbal Medicines for Cancer Patients. Motoo Y, Editor. Evid-Based Complement Altern Med (2013) 2013:302426. doi: 10.1155/2013/302426
263. Yang N-S, Shyur L-F, Chen C-H, Wang S-Y, Tzeng C-M. Medicinal Herb Extract and a Single-Compound Drug Confer Similar Complex Pharmacogenomic Activities in Mcf-7 Cells. J BioMed Sci (2004) 11(3):418–22. doi: 10.1007/BF02254447
264. You L, An R, Liang K, Wang X. Anti-Breast Cancer Agents From Chinese Herbal Medicines. Mini Rev Med Chem (2013) 13(1):101–5. doi: 10.2174/138955713804484785
265. Yang S-YY, N-S, Agricultural Biotechnology Research Center, Academia Sinica T, Taiwan RO. Immuno-Modulatory Effects of Phytomedicines Evaluated Using Omics Approaches. USA: IntechOpen (2011). p. 28.
266. Martino E, Della Volpe S, Terribile E, Benetti E, Sakaj M, Centamore A, et al. The Long Story of Camptothecin: From Traditional Medicine to Drugs. Bioorg Med Chem Lett (2017) 27(4):701–7. doi: 10.1016/j.bmcl.2016.12.085
267. Seneca. CHAPTER 2 - Alkaloid Chemistry. In: Aniszewski TBT-A-S of L, Editor. Alkaloids - Secrets of Life. Amsterdam: Elsevier (2007) p. 61–139. doi: 10.1016/B978-044452736-3/50004-0
268. Marupudi NI, Han JE, Li KW, Renard VM, Tyler BM, Brem H. Paclitaxel: A Review of Adverse Toxicities and Novel Delivery Strategies. Expert Opin Drug Saf (2007) 6(5):609–21. doi: 10.1517/14740338.6.5.609
269. Lee S, Lee HY, Park Y, Ko EJ, Ban TH, Chung BH, et al. Development of End Stage Renal Disease After Long-Term Ingestion of Chaga Mushroom: Case Report and Review of Literature. J Korean Med Sci (2020) 35(19):e122–2. doi: 10.3346/jkms.2020.35.e122
270. Nazari S, Maryam Rameshrad HH. Toxicological Effects of Glycyrrhiza Glabra (Licorice). Phytother Res (2017) 31(11):1635–50. doi: 10.1002/ptr.5893
271. Isbrucker RA, Burdock GA. Risk and Safety Assessment on the Consumption of Licorice Root (Glycyrrhiza Sp.), its Extract and Powder as a Food Ingredient, With Emphasis on the Pharmacology and Toxicology of Glycyrrhizin. Regul Toxicol Pharmacol (2006) 46(3):167–92. doi: 10.1016/j.yrtph.2006.06.002
272. Ben-Arye E, Samuels N, Goldstein LH, Mutafoglu K, Omran S, Schiff E, et al. Potential Risks Associated With Traditional Herbal Medicine Use in Cancer Care: A Study of Middle Eastern Oncology Health Care Professionals. Cancer (2016) 122(4):598–610. doi: 10.1002/cncr.29796
273. Izzo AA, Ernst E. Interactions Between Herbal Medicines and Prescribed Drugs. Drugs (2001) 61(15):2163–75. doi: 10.2165/00003495-200161150-00002
274. Rastegar R, Akbari Javar H, Khoobi M, Dehghan Kelishadi P, Hossein Yousefi G, Doosti M, et al. Evaluation of a Novel Biocompatible Magnetic Nanomedicine Based on Beta-Cyclodextrin, Loaded Doxorubicin-Curcumin for Overcoming Chemoresistance in Breast Cancer. Artif Cells Nanomedicine Biotechnol (2018) 46(sup2):207–16. doi: 10.1080/21691401.2018.1453829
275. Anwar DM, El-Sayed M, Reda A, Fang JY, Khattab SN, Elzoghby AO. Recent Advances in Herbal Combination Nanomedicine for Cancer: Delivery Technology and Therapeutic Outcomes. Expert Opin Drug Deliv (2021) 18(11):1609–25. doi: 10.1080/17425247.2021.1955853
276. Lakshmanan A, Akasov RA, Sholina NV, Demina PA, Generalova AN, Gangadharan A, et al. Nanocurcumin-Loaded UCNPs for Cancer Theranostics: Physicochemical Properties, In Vitro Toxicity, and In Vivo Imaging Studies. Nanomaterials (2021) 11(9):2234. doi: 10.3390/nano11092234
Keywords: cancer, chemotherapy, drug delivery system, natural bioactive compound, phytochemical, nanomedicine
Citation: Chavda VP, Patel AB, Mistry KJ, Suthar SF, Wu Z-X, Chen Z-S and Hou K (2022) Nano-Drug Delivery Systems Entrapping Natural Bioactive Compounds for Cancer: Recent Progress and Future Challenges. Front. Oncol. 12:867655. doi: 10.3389/fonc.2022.867655
Received: 01 February 2022; Accepted: 24 February 2022;
Published: 29 March 2022.
Edited by:
Peixin Dong, Hokkaido University, JapanReviewed by:
Jianxiang Li, Soochow University Medical College, ChinaShanzhi Wang, University of Arkansas at Little Rock, United States
Zhaoliang Li, University of Utah, United States
Copyright © 2022 Chavda, Patel, Mistry, Suthar, Wu, Chen and Hou. This is an open-access article distributed under the terms of the Creative Commons Attribution License (CC BY). The use, distribution or reproduction in other forums is permitted, provided the original author(s) and the copyright owner(s) are credited and that the original publication in this journal is cited, in accordance with accepted academic practice. No use, distribution or reproduction is permitted which does not comply with these terms.
*Correspondence: Vivek P. Chavda, Vivek7chavda@gmail.com; Zhe-Sheng Chen, Chenz@stjohns.edu; Kaijian Hou, kaijianhou@126.com
†ORCID: Vivek P. Chavda, orcid.org/0000-0002-7701-8597
Zhe-Sheng Chen, orcid.org/0000-0002-8289-097X
Kaijian Hou, orcid.org/0000-0003-1733-0068
Aayushi B. Patel, orcid.org/0000-0002-1086-4369
Kavya J. Mistry, orcid.org/0000-0003-0710-3144
Suresh F. Suthar, orcid.org/0000-0002-3588-880X
‡These authors have contributed equally to this work and share first authorship