- 1Bobby R. Alford Department of Otolaryngology- Head and Neck Surgery, Baylor College of Medicine, Houston, TX, United States
- 2Ear Nose and Throat Section (ENT), Operative Care Line, Michael E. DeBakey Veterans Affairs Medical Center, Houston, TX, United States
- 3Center for Translational Research on Inflammatory Diseases, Michael E. DeBakey Veterans Affairs Medical Center, Houston, TX, United States
- 4Department of Head and Neck Surgery, Division of Surgery, University of Texas MD Anderson Cancer Center, Houston, TX, United States
- 5Department of Molecular and Cellular Oncology, Division of Surgery, University of Texas MD Anderson Cancer Center, Houston, TX, United States
- 6Department of Radiation Oncology, Division of Surgery, University of Texas MD Anderson Cancer Center, Houston, TX, United States
Oropharyngeal cancer (OPC) poses a complex therapeutic dilemma for patients and oncologists alike, made worse by the epidemic increase in new cases associated with the oncogenic human papillomavirus (HPV). In a counterintuitive manner, the very thing which gives patients hope, the high response rate of HPV-associated OPC to conventional chemo-radiation strategies, has become one of the biggest challenges for the field as a whole. It has now become clear that for ~30-40% of patients, treatment intensity could be reduced without losing therapeutic efficacy, yet substantially diminishing the acute and lifelong morbidity resulting from conventional chemotherapy and radiation. At the same time, conventional approaches to de-escalation at a population (selected or unselected) level are hampered by a simple fact: we lack patient-specific information from individual tumors that can predict responsiveness. This results in a problematic tradeoff between the deleterious impact of de-escalation on patients with aggressive, treatment-refractory disease and the beneficial reduction in treatment-related morbidity for patients with treatment-responsive disease. True precision oncology approaches require a constant, iterative interrogation of solid tumors prior to and especially during cancer treatment in order to tailor treatment intensity to tumor biology. Whereas this approach can be deployed in hematologic diseases with some success, our ability to extend it to solid cancers with regional metastasis has been extremely limited in the curative intent setting. New developments in metabolic imaging and quantitative interrogation of circulating DNA, tumor exosomes and whole circulating tumor cells, however, provide renewed opportunities to adapt and individualize even conventional chemo-radiation strategies to diseases with highly variable biology such as OPC. In this review, we discuss opportunities to deploy developing technologies in the context of institutional and cooperative group clinical trials over the coming decade.
Introduction
OPC incidence is increasing
With more than 63,000 cases annually in the US, head and neck cancer (HNC) represents a significant health burden (1). The epidemic increase in human papillomavirus (HPV)-associated HNC will dramatically increase this burden over the coming decades. Given the epidemiologic shift toward HPV-mediated diseases and lagging vaccination rates, the rise in HNC incidence is projected to not begin to abate until the 2050s. The US healthcare system will need to accommodate medical conditions related to HNC and HNC treatment for well over 4 million patients projected to be diagnosed between 2000 and 2060 (2). Previously a relatively rare entity, HNC was primarily attributable to tobacco and alcohol exposure and was predominantly a disease of elderly male patients (3, 4). The rise in HPV-associated HNC (3, 5) affects most age groups and crosses gender and racial/ethnic barriers (6). Long known to be a cause of cervical, penile, and anal cancer (7–9), HPV has been shown to be the primary driver of the increase in HNC diagnoses particularly for the oropharynx site (OPC). Preclinical and clinical studies have now conclusively linked HPV to OPC tumorigenesis in a majority of new diagnoses in the United States, with an increasing incidence across much of the world (10–19).
Survival is highly variable
The shift toward HPV-associated disease was accompanied by the first significant improvements in HNC treatment response and survival in the last 50 years of clinical research and medicine. First brought to light by the landmark retrospective analysis of RTOG 0129 by Ang et al. (10), HPV-associated OPC demonstrates a drastically improved survival compared to its HPV-independent counterpart. At a population level, younger OPC patients, without a history of tobacco exposure and early T-stage tumors were shown to have a significantly improved survival in the early 2000s compared to the previous half century (3). Despite these promising shifts in survival, the same analysis showed that a subset of OPC patients continues to demonstrate poor disease free and overall survival, consistent with historical data, despite application of new therapeutic strategies (3, 10).
Following a decade of clinical trial and retrospective data analysis, the AJCC Staging Manual received a significant update in its 8th Edition, with a dichotomization of OPC into HPV-associated and HPV-independent disease, and a concomitant reduction in stage in the context of HPV-associated OPC meant to more accurately reflect the improved survival of patients with what in the past would have been considered Stage II-III and even Stage IV disease (20). The newest large scale clinical trials conducted in OPC, including RTOG1016 and De-ESCALaTE confirmed that the survival parameters for HPV-associated OPC had indeed shifted critically compared to historical data (21, 22).
This improvement in survival, predicated on an excellent response to conventional radiation and chemotherapy strategies in a large subset of HPV-associated OPC has given hope to patients and clinicians alike, given the prevailing failures to improve HNC survival over previous decades. Yet at the same time, the same improvement in survival has drastically complicated the clinical management of the disease, at a time when its increasing incidence is exacerbating potential errors in an exponential fashion. Although as a group, HPV-associated OPC patients do well compared to their HPV-independent OPC counterparts, this effect is not uniform. There remains considerable heterogeneity in HPV-associated OPC response to treatment. Among Veterans with high rates of heavy tobacco exposure, survival for HPV-associated OPC remains lower compared to non-smokers by approximately 20% (4, 23) in line with the Ang et al. intermediate-risk rates (4, 24). These characteristics are conserved in both white and black patients, resulting in similar disease behavior and oncologic outcomes (25). Re-analyzed data from RTOG 0129 and RTOG 0522 demonstrated that the overall (OS) and progression free survival (PFS) rates for low-, intermediate- and high-risk OPC patients persisted with a difference in PFS between low- and intermediate- risk groups of over 15% (26). Our recent analysis of over 600 OPC patients treated in the modern era showed that heavy tobacco exposure reduced survival by the same amount as a shift in disease stage of 1 (e.g., stage I migrated to stage II) (27), in line with data published earlier by Vawda et al. (28).
Whereas some risk factors (e.g., tobacco) portend inferior survival in a subset of HPV-associated OPC patients, there is increasing evidence that a subset of HPV-associated OPC patients demonstrates excellent response to chemo-radiation. A recent analysis of over 1000 HPV-associated OPC patients showed that low levels of multinucleation identified on analysis of pre-treatment biopsy specimens were associated with dramatic improvements in overall, disease free and distant metastasis free survival, with hazard ratios ranging from 1.78 to 1.94 (29). In parallel, even when the analysis is restricted by stage, as was done by our collaborative group in a cohort of 439 stage I patients, infiltrative lymphocyte levels can drive further stratification of survival with hazard ratios >2.0 (30).
Together these data indicate that new HPV-associated OPC patients cannot be expected to demonstrate uniform response to chemo-radiation and thus equivalent survival. Furthermore, there is no evidence that this divergent survival is likely to change over the coming decades due to significant shifts in treatment paradigms. Surgery has not replaced radiation for most patients and there is no evidence that post-treatment function will be better with surgery (31–33). Targeted agents are inferior to conventional chemotherapy and no less toxic (21, 22). Immunotherapy has not yet demonstrated utility in the definitive, frontline setting for HNC and thus will be unlikely to replace conventional chemotherapy as a radiosensitizer in the near future (34). The only viable option to achieve a precision oncology approach that appropriately balances treatment effectiveness and toxicity is to maximize separation of patients into high-risk and low-risk groups.
Limitations to conventional risk stratification
Although largely self-evident, it remains important to understand why it is critical that we accurately risk-stratify OPC patients. Standard NCCN guidelines for OPC treatment include definitive external beam radiation (EBRT) regimens (66-70Gy) or surgical resection followed by adjuvant EBRT with a slight reduction in dose based on pathologic features of the disease and conventional chemotherapy with cisplatin being the current standard of care (21, 22). As indicated above, these conventional approaches are extremely effective in a majority of HPV-associated OPC patients but they carry significant acute toxicity and the potential for life-long debilitating morbidity (e.g., chronic renal insufficiency, peripheral neurotoxicity, chronic aspiration, lower cranial nerve neuropathies) (35–43). There is currently no definitive evidence that we can safely shift away from current NCCN guidelines for HPV-associated OPC disease as a whole. Omission of cisplatin has not been shown to be safe at a population level prospectively (HN002) (44) and direct replacement of cisplatin with cetuximab has failed in 2 prospective clinical trials (21, 22). Replacement of cisplatin with immune checkpoint inhibitors does not appear to be on the horizon for at least another decade based on the most recent negative clinical trial data (34). Altered fractionation regimens designed to reduce EBRT toxicity have been investigated for over 3 decades without a significant impact, although IMRT has indeed greatly reduced toxicity over previous EBRT delivery approaches (35). Dose de-escalation appears promising in very select patients, but has not yet been shown to be safe across the broader HPV-associated OPC population in large randomized clinical trials. Incorporation of surgery into treatment paradigms for OPC has shown promise as it relates to risk stratification and tailoring adjuvant treatment to disease burden. In EA3311, investigators were able to show that patients deemed intermediate-risk based on surgical pathologic parameters could receive a reduced dose of adjuvant radiation of 50Gy without a clear decrease in treatment efficacy as measured using progression free survival (PFS) (45).
Recurrence from HPV-associated OPC is deadly; no less so compared to that from HPV-independent disease. Salvage with surgery, re-irradiation or systemic treatment fails in >60% of recurrent disease patients (46–49). Taken together, the severe toxicity from current treatment regimens and the nearly uniform fatality of recurrent disease create a Hobson’s choice for patients and a difficult balancing act for oncologists. Reducing treatment intensity at a population level will undoubtedly result in more recurrences yet failure to reduce treatment intensity will result in overtreatment and unnecessary toxicity in a large fraction of the OPC population. Importantly, some of this toxicity will translate into treatment related mortality (e.g., aspiration), making the need for accurate risk-stratification of OPC patients critical.
Conventional risk stratification has been standard for HNC ever since the first introduction of TNM classification and has continued throughout the 8 editions of the AJCC Staging Manual. That conventional risk stratification is clinically useful is evidenced by the significant divergence of survival by disease stage across tens of thousands of treated patients; that incorporation of HPV status into OPC staging has been impactful is similarly made plain by both prospective and retrospective datasets (10, 11, 21, 27, 46, 50). Yet at the same time, conventional risk stratification has had a modest impact on our ability to develop treatment regimens better tailored to disease biology. Whereas positive margins and extra-nodal extension (ENE) were shown to be useful in assigning patients to treatment escalation with the addition of conventional chemotherapy in the adjuvant setting, their utility in the setting of HPV-associated disease may be more limited (47). For aggressive, advanced-stage disease, attempted escalation with induction chemotherapy failed to improve survival in the PARADIGM and DECIDE trials (51, 52), and in a recent in-depth retrospective analysis appeared to be associated with reduced survival in OPC patients (53). As mentioned above, changing from cisplatin to cetuximab, a drug assumed to be more tolerable and thus better suited for the lower risk HPV-associated OPC population failed to maintain adequate survival in both RTOG1016 (which included intermediate-risk OPC) and De-ESCALaTE (which included exclusively low-risk OPC) trials (21). HN002 concluded that although a modest reduction in EBRT dose was safe, the omission of cisplatin could not be deemed safe even in non-smokers with HPV-associated OPC (low-risk OPC) (44).
One limitation of conventional risk stratification is that it requires very large signals (difference in survival), very large cohorts or both. An excellent example of this is the initial Ang et al. study in which HPV-associated OPC demonstrated ~75% survival at 2 years compared to HPV-independent OPC patients which demonstrated ~30% survival at 2 years, with HPV-associated smokers essentially in the middle (10). These very large differences have persisted in retrospective analysis across multiple cohorts and are reproduced in the aggregate when data from RTOG1016 and De-ESCALaTE are analyzed head to head. Despite decades of investigation, no other biological variable in HNC has demonstrated such dramatic stratifying effects (e.g., TP53) across multiple prospective and retrospective cohorts and thus, no other biological variables are included in the AJCC staging or considered in NCCN guidelines for HNC treatment generally. Effect sizes from shifts in treatment are similarly small. When averaged over tens of thousands of patients, the effect size for adding conventional chemotherapy to radiation in the definitive setting results in merely a 7-8% improvement in survival in the latest MACH-NC analysis, yet its elimination in the setting of low risk disease has not been shown to be safe (54). For decades, nodal metastasis was considered one of the most compelling predictors of survival in HNC, and indeed for HPV-independent disease it remains so as was recently show in oral cavity disease (47). In contrast, HPV-associated OPC demonstrates excellent survival even when nodal metastasis is present, which resulted in the substantial down-staging of tumors with significant nodal disease in the 8th edition of the AJCC Staging Manual (20).
A second limitation of conventional risk stratification is a fundamental lack of knowledge - we simply don’t know what we don’t know. Decades were required to properly observe the presence of, measure the impact for, and develop risk stratification based on, HPV status alone in OPC. More recent work from us and others has now identified other potential stratifiers for treatment response and survival, including multinucleation, infiltration of tumors by cytotoxic immunocytes, the presence of complex immune frameworks in a subset of OPC tumors and differential tumor mutational burden (30, 46, 55, 56). Yet none of these potential risk-stratification markers are fully proven, and it is unlikely that they would be incorporated into staging and used for treatment de-intensification without extensive prospective testing.
One critical limitation to incorporating biologically specific biomarkers into risk stratification algorithms stems from the potential for false negative findings. Many individual genomic events (e.g., TP53 mutation, KEAP1 mutation) can be quite rare depending on the subtype of OPC and thus most retrospective and prospective institutional datasets and even cooperative group trial cohorts will be underpowered to truly examine their risk stratification potential. The need to develop large cohorts, with comprehensive clinical data and appropriate matching tissue has now been recognized by investigators and funding agencies alike (e.g., National Institute of Dental and Craniofacial Research).
Risk-stratification and therapeutic response drivers
Many aspects of tumor biology can confer “risk” as manifested by reduced survival. However, only those biological events which drive treatment response can really inform our ability to modulate existing therapeutic strategies in a meaningful way to reduce toxicity or improve overall response. In breast cancer and prostate cancer, hormonal receptor status is utilized to characterize the disease because it fundamentally influences response to hormonal blockade (57, 58). In melanoma and to a lesser degree in thyroid carcinoma, BRAF mutational status is a critical biomarker because it predicts response to a specific treatment, namely BRAF +/- MEK inhibition. Unlike in these diseases, and multiple other examples in adjacent solid tumors (e.g., lung cancer) (59, 60), HNC broadly and OPC in particular manifests few, if any, examples of biologically consistent drivers of response to chemotherapy and radiation which can be used to mechanistically inform modulation of therapy, especially de-escalation strategies.
Even within the context of HPV-driven disease, the superior response of disease to conventional chemotherapy and radiation remains unclear. Some speculate that maintenance of a wild-type TP53 status allows for activation of the tumor suppressor under oxidative stress conditions (e.g., during treatment) and may explain the improved response rate (61). Others, including us, believe that an improved tumor immune micro-environment (i.e., enriched for functional immunocytes) may somehow result in an improved response, although this is somewhat mechanistically unclear since HPV-associated tumors do not demonstrate a substantially better response to immune checkpoint inhibitors compared to their HPV-independent counterparts (46, 62, 63). Another subset of investigators suggest that higher levels of oncogene-driven replication stress in HPV-associated tumors allows them to more easily activate programmed cell death pathways (61) or that non-canonical p16 signaling may be key to enhanced radiation response in this disease subset (64). The fact that we cannot consistently explain WHY HPV-associated OPC responds better to radiation (with or without chemotherapy) provides a clear impediment to a logical escalation or de-escalation strategy for this patient population. Whereas HPV oncogenic infections and their downstream impact on intra-cellular tumor suppressors and signaling cascades have been studied for years, some of the more recent pathomic and radiomic features correlated with improved survival in HPV-associated disease have never been mechanistically explored and thus are highly unlikely to really impact treatment intensity decisions for the near future without extensive preclinical and clinical investigation.
This limitation also applies to what many consider the treatment of the future, namely immunotherapy in the form of immune checkpoint inhibitors (ICIs). Starting with CheckMate141 and followed by Keynote048, ICIs have now demonstrated meaningful activity in HNC broadly and OPC specifically in the recurrent metastatic disease setting (63, 65). However, their use has encountered some of the same difficulties experienced when trying to improve upon the radiation vs surgery +/- conventional chemotherapy approach with targeted agents (e.g., cetuximab) or conventional induction chemotherapy in previous decades: treatment optimization. Combinatorial therapy studies have failed in the definitive upfront setting to date (e.g., JAVELIN Head and Neck 100) (34). In part, this is likely driven by the same limitation we face with conventional treatment. We have no predictive biomarker of ICI response in HNC or OPC specifically. PDL1 status although utilized, is far from being informative enough to further optimize utilization beyond the dichotomous chemotherapy versus no chemotherapy decision point. More sophisticated transcriptomic approaches published in recent years (e.g., TGEP) or our pathomic approaches (MuNI, OP-TIL) remain far from being prospectively validated and even with validation they remain poorly linked mechanistically to ICI effects (29, 30, 66, 67). It is also important to note, that immunotherapy in the form of existing ICIs, is not quite as benign as was initially hoped. Significant levels of immunotherapy-related adverse events (irAEs) have been reported in non-small cell lung cancer (NSCLC) (68, 69), melanoma (70) and HNSCC (71) especially when multiple ICIs are combined. Particularly problematic is the consistent observation that ICI toxicity and effectiveness are extremely correlated suggesting a substantial hurdle to ICI deployment for HNSCC particularly when combined with other toxic regimens/treatments.
Adaptive risk stratification
In the second half of the last century, John Boyd introduced the OODA (observe, orient, decide, act) loop concept, first in the context of military conflict and then more generally in the context of human behavior and interaction. Conventional risk stratification for cancers has optimized the utilization of the OODA concept, even more so with the revolution in genomic, transcriptomic and proteomic characterization of tumors. However, a key component of Boyd’s approach to action was the loop itself, the iterative and ever informative nature of a repetitive cycle. Modern risk stratification runs the loop once; after the decision to act is made, no further information is easily available to the oncologist until the complete course of chemo-radiation runs its course. This approach violates basic principles of biology, which is adaptive in the setting of exogenous stress (particularly in highly flexible cancer cells) in addition to reducing the proven benefits of the loop. Oncologists are not to blame for this failure. The failure stems from the difficulties of obtaining new information from solid tumors that are meaningful, actionable, and timely. Yet new techniques are being increasingly deployed which may make this a reality in the not-too-distant future.
In addition to the difficulties associated with conventional risk stratification outlined above, conventional risk-stratification suffers from a fatal flaw. It is static; it ignores the effect of the treatment itself which can manifest in many ways. Radiation can impact ICI response through local destruction of immunocytes. Chemotherapy can impact ICI effectiveness through systemic myelosuppression. Both can generate significant shifts in tumor biology which may be anti-immunogenic (72). Conversely, these interactions can occur in a positive feedback loop through damage-associated molecular patterns (DAMPs) or generation of mutational or more commonly expression-based neoantigens (73, 74). Unlike other solid cancers, truly ingrained events such as BRAF, ALK and EGFR mutations simply do not exist in OPC or even HNC with sufficient frequency to drive treatment selection on the basis of predicted response. As a result, all biological shifts during treatment, small and subtle as they may be, can greatly impact the effectiveness of the chosen treatment and affect the predictive potential of any risk stratification schema (Figure 1). This limitation applies to ICIs as well which still lack a robustly informative biomarker of response in OPC and to some degree in many other solid tumors.
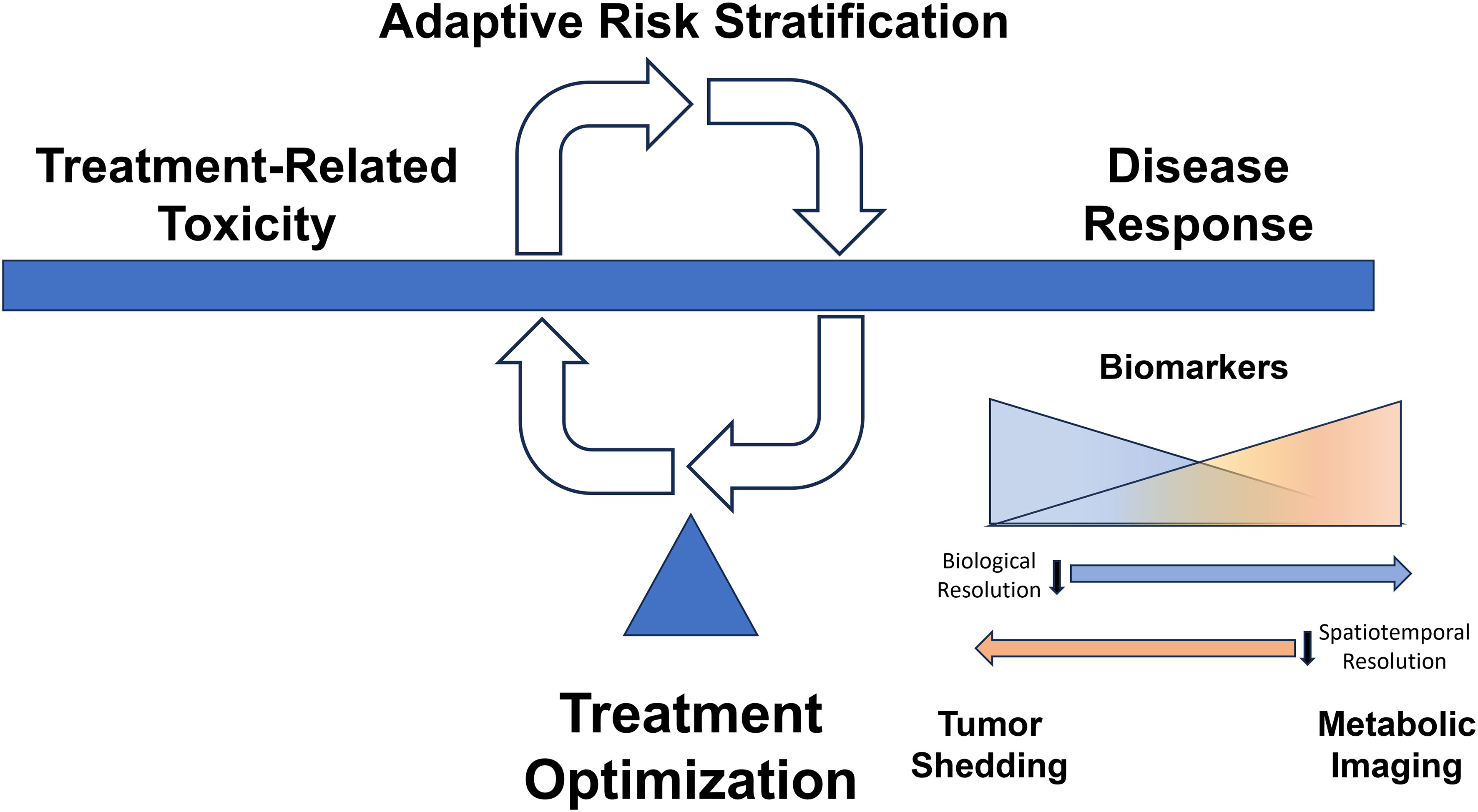
Figure 1 Dynamic Adaptive Risk Stratification. Treatment decisions for our patients balance maximizing disease response and minimizing treatment-related toxicity. There is currently a scarcity of clear biologically consistent drivers of response to therapy which can be used to mechanistically inform modulation of therapy, especially de-escalation strategies. Dynamic assessment of treatment response may allow therapeutic modification to balance disease control with toxicity. Tumor shedding creates a multitude of circulating biomarkers (e.g., viral DNA, tumor exosomes, viable circulating tumor cells) that provide high biological resolution regarding response to therapy, while imaging-based parameters may afford high spatiotemporal resolution reflective of tumor heterogeneity in response to treatment.
Leveraging tumor shedding for adaptive risk stratification
While hematopoietic malignancies have an intrinsic circulating component, solid tumors are highly anatomically restricted and defined (even in the metastatic setting). However, the presence of solid tumors can be detected at a systemic level through a multitude of circulating markers, including viral DNA (for oncogenic viruses such as EBV and HPV), tumor exosomes, cell free DNA (cfDNA), and even fully viable circulating tumor cells. These markers provide a compelling avenue to indirectly interrogate events in solid tumors to inform treatment selection and make clinical decisions in an iterative fashion for an individual patient.
Plasma EBV DNA levels have been capable of detecting a prior infection and associated malignancies for over 2 decades (75, 76). Nearly 80% of patients with active nasopharyngeal cancer mediated by EBV shed detectable EBV DNA prior to treatment and EBV remains systemically detectable in the post-treatment setting when patients presented with initially higher stage disease (77). In contrast to serology, circulating DNA levels can be at least partially correlated to relative tumor burden generating a more useful biomarker of relative tumor burden in the post treatment setting (78). Recent studies have extended this approach to the HPV counterpart of EBV leveraging the fact that both are oncogenic viruses with a direct link to the biological genesis of the underlying disease. Oncogenic HPV infection can be detected at a single-cell level in basal keratinocytes suggestive of potential for a mechanistic biomarker with a high sensitivity albeit likely a low specificity for development of cancer in the short term (79). HPV viral loads have been correlated with survival in patients with OPC (80) in both retrospective and prospective series. The ability to detect measurable changes in circulating tumor tissue modified viral DNA (TTMV) during treatment holds some potential to inform de-escalation strategies for patients with HPV-associated OPC. Although the accuracy of such a biomarker would need to be extremely high, a more proximate application of this approach is as early biomarker of recurrence. TTMV has been utilized in large series (81) of patients (>1000) to track recurrence post-treatment with an overall positive predictive value for recurrent disease of 95% and a point-in-time negative predictive value is 95% (with the caveat that some patients with a one-time negative test did go on to develop recurrence). Detection of EBV and HPV can thus be useful but is not currently actionable as it does not reflect events downstream from the individual viral oncogenes and thus cannot inform how chemotherapy, radiation or ICIs might interact with an individual tumor’s biological features.
Whereas viral DNA can be useful in the setting of virally mediated HNC, circulating tumor DNA (ctDNA) can be broadly utilized regardless of underlying tumor pathogenesis. We and others have previously deployed ctDNA to detect actionable oncogenic events in solid tumors including melanoma and anaplastic thyroid carcinoma such as the V600E BRAF mutation (82). Other investigators (83) have used ctDNA and phylogenetic analysis to track the evolution of lung cancer and development of chemotherapy resistance. In contrast to HNC and NSCLC, in SCLC high rates of hematogenous spread are commonly encountered resulting in rapid and widespread distant metastasis. In this setting ctDNA is thought to be particularly informative and representative of the intrinsic tumor biology (84) as was shown via paired analysis of primary tumors and ctDNA of variant allele frequency of clonal mutations. Simply put, shifts in ctDNA during and post-treatment can reflect, albeit with caveats, similar shifts within the primary and metastatic tumor sites which may be indicative of cure or recurrence as a function of clonal expansion and/extinction. Recent work by Cao et al. highlighted the utility of a combined ctDNA/imaging-based approach to early detection of treatment response in AJCC (8th edition) stage III OPC patients and demonstrate significant correlation with freedom from disease progression (85). Similarly, Chera et al. showed that rapid clearance of HPV ctDNA (defined as a favorable clearance profile) achieved cure with conventional chemo-radiation in contrast to patients with an unfavorable clearance profile (86).
A broader biological approach is to assess exosomes (87), sub-micrometer tumor cell vesicles, which can be stable in body fluids and contain not just DNA, but also RNA, tumor proteins, lipids, and metabolites. In some cases, proteins can be particularly informative as in the case of PDL1 (88) which has been correlated to HNC disease progression as compared to non-exosomal plasma PDL1 levels. Exosomes and their counterpart microvesicles (89) can be used in a largely agnostic fashion to characterize data from both tumor and viral DNA as well as associated proteins and metabolites, forming a biologically rich dataset and providing increased stability for macromolecules in inhospitable fluid environments such as saliva which can be of critical importance to HNC. At the extreme end of the spectrum, the entire biological landscape of a subset of tumor clones can be captured in the form of whole, viable circulating tumor cells (CTCs) (90). In HNC, a pooled survival analysis of 22 studies eligible for systematic review found that presence of CTCs was associated with shorter disease-free survival (DFS, HR 4.62, 95% CI 2.51-8.52) with a very high overall specificity but low sensitivity. An important limitation to circulating biomarkers is that their actionability remains in question at this time in the context of OPSCC. All existing systemic treatments inclusive of ICIs incur significant toxicity for limited survival benefit and almost none for lasting cure. As such, treatment in the recurrent/metastatic setting is reserved for either imaging identifiable lesions (e.g. radiation based treatment of oligometastasis, surgical resection of isolated regional recurrence) or for symptomatic disease (e.g. palliative intent chemotherapy and/or chemo-ICIs). Since there is limited evidence that earlier initiation of treatment is either feasible, in the setting of imaging invisible disease, or beneficial, in the setting of disseminated disease, the utility of early detection of recurrence/metastasis for this particular disease site remains unclear, particularly since it often precedes conventionally detectable disease by only several weeks to months. As such, utility may be initially limited to early detection of response to primary treatment that could assist escalation/de-escalation decision making.
Leveraging metabolic imaging for adaptive risk stratification
Whereas ctDNA, CTCs and exosomes can provide high biologic resolution and identify a multitude of genomic, transcriptomic, and proteomic events related to tumorigenesis and evolution prior to and during treatment delivery, spatial resolution is absent. Although a signal may be detected, we have no idea where that signal is coming from (i.e., primary tumor, regional or distant metastases, etc). In contrast, imaging can provide outstanding spatial resolution, but significantly lower biological resolution. It is not the goal of this review to summarize the massive literature on the subject of biologic imaging of solid tumors, but rather to highlight some recent advances in imaging which may be applicable to dynamic or adaptive risk stratification strategies for OPC.
Starting with extensive work using F-labeled fluoromisonidazole (F-FMISO) (91), pre-treatment measurements of tumor hypoxia have long been utilized to ascertain potential radio-sensitivity/radio-resistance of whole tumors or individual tumor voxels given the known correlation between tumor hypoxia and radiation responsiveness. The counterpart of hypoxia, namely vascularity can be ascertained with fairly high sensitivity and specificity using dynamic contrast-enhanced MRI (DCE-MRI). DCE-MRI can be deployed in translationally relevant settings particularly when utilizing anti-angiogenic agents where imaging parameters may be altered prior to clinical effect (92). By capturing vascular parameters throughout the entire treatment field (tumor and adjacent normal tissue) DCE-MRI has the additional potential to be a real-time biomarker of normal tissue toxicity driven by shifts in vascularity. One such application pioneered by our group is the use of DCE-MRI for early detection of subclinical osteoradionecrosis (ORN) and identification of patients at high risk for severe ORN (93–95).
Extension of this work using multi-parametric (MRI) (96) has been used to predict complete response (CR) in patients with OPC prior to treatment completion in a manner suitable for potential treatment de-escalation in responders. Although additional work will be required to optimize multi-parametric and even DCE-MRI to fully capture biological data from the primary tumor and associated cervical lymphadenopathy common to OPC, preliminary findings are promising (97). This is particularly true since the approach appears to be scalable across institutions as shown in a comprehensive analysis (98) of the accuracy of diffusion-weighted imaging (DWI) for predicting locoregional failure of chemo-radiation in HNC across 9 studies and 421 patients, with a sensitivity of 82%, specificity of 70% and an area under the sROC curve of 84%.
While tumor vascularity, cellularity and hypoxia are transient on a slow scale (days-weeks), tumor metabolism is a continuously changing biological variable that has extremely high temporal resolution (minutes-hours), and when interrogated via metabolic imaging can be analyzed with an equally high spatial resolution. Over the last 2 decades, both FDG-PET and hyperpolarized magnetic resonance imaging (HP-MRI) techniques have been used to assess the aggressiveness of solid tumors including HNC and have been explored as tools to predict treatment response in preclinical models and patients (99–109). Although FDG-PET is available in clinical settings, prospective clinical trial data suggest that measurement of mid-therapy glucose uptake does not allow for adaptive reduction in tumor volumes (110–115). Furthermore, glucose uptake does not correlate with radiation response and provides no information on intracellular metabolic fluxes (116–118). In contrast HP-MRI of labeled pyruvate and lactate provides a unique opportunity to obtain real-time metabolic information from within solid tumors. Its ability to detect differential metabolic activity in tumor tissue has been established (99, 109). Substantial work from other groups has advanced the development of HP-MRI into a clinically viable tool for characterization of intrinsic tumor aggressiveness (prostate) and towards deployment of HP-MRI as a tool to measure treatment response (e.g., breast cancer) (101, 103, 119–121). HNC sensitivity to genotoxic agents is a function of multiple discrete biological events, such as activation of pathways associated with the human papillomavirus (HPV) or mutation of tumor suppressors such as TP53. Unfortunately, we and others have shown that individual patient responses are not completely uniform across patient groups (e.g., HPV-associated vs. HPV-independent, wildtype vs. mutant TP53), and this may be due in large part to the heterogenous activation of acquired resistance pathways once treatment starts (3, 4, 10, 122–127). Therefore, even if genomic biomarkers such as TP53 and HPV start to be used in treatment-selection decisions at baseline, tailoring treatment intensity to individual patients in the face of acquired resistance potentially based upon changes in metabolic response will still be required for true precision oncology approaches and personalized cancer treatment.
In 2014, we were the first to show that kPL measured with noninvasive HP [1- (13)C]-pyruvate MRI is decreased under conditions of depleted REDOX following genotoxic stress in animal models of HNC and other tumors (128). We have developed a multi-compartment model of intracellular kPL which increases the fidelity of our measurements (129). In 2020, for the first time, we measured these metabolic changes in a patient during treatment. This first-in-human assessment of metabolic response to treatment serves as a critical proof-of-principle and demonstrates our technical capability to execute the proposed studies. On the basis of these robust preliminary data, we propose to test the potential of metabolic interrogation as a clinical tool that can (1) predict treatment response and (2) be used to develop treatment strategies tailored to individual tumor biology. Our innovative approach is supported by (1) studies that link reducing potential to genotoxic stress (127, 130–136); (2) clinical and preclinical data that link lactate to tumor progression and treatment response (137–139); and (3) studies that confirm the excellent spatiotemporal resolution of HP [1- (13)C]-pyruvate MRI (128, 140–143).
The biologically rich data from anatomic and metabolic studies can be enhanced by nearly an order of magnitude when combined with artificial intelligence (AI)/machine learning (ML) approaches. Utilization of machine learning approaches (144) can generate meaningful data even from relatively data poor CECT studies to identify radiomic features which when combined can distinguish invasive cancer from more benign solid tumors. This approach has also been deployed (145) to generate combined radiomic risk scores which can predict disease free and overall survival in the context of either conventional treatment or in the presence of immunomodulatory combinatorial strategies.
A path toward clinical translation
Conventional and adaptive risk stratification are not mutually exclusive. They represent 2 aspects of a combined approach designed to deliver maximal anti-tumor activity, using the most appropriate agents, at the lowest possible dose that will achieve a durable cure. In order to maximize the therapeutic index of both conventional and targeted strategies the most effective future algorithms will start with conventional risk stratification that combines biological data with clinical risk factors. Upon this baseline approach, treatment algorithms will then incorporate a complex adaptive risk stratification strategy that combines feasible aspects of biological interrogation using circulating and imaging tumor markers (Figure 1). Critically, this second layer of data will be truly personalized, specific not only to the individual tumor, but also to the interaction between the individual tumor and the chosen treatment regimen. Successful implementation of such an approach will require a rigorous process, outlined by Pepe et al. nearly 2 decades ago (146), whose key ingredients include carefully defining the target population (carefully selected based on clinically relevant criteria and relevant disease biology) and the expected outcome for each individual biomarker (e.g. impact on local recurrence vs distant metastasis rates), testing in populations large enough to reduce the number of false negative studies, and a priori definitions of expected effect size and clinical impact. For solid tumors, which present challenges to repetitive interrogation with high biological and spatial resolution (see above), an “n of 1” precision oncology algorithm is somewhat unlikely using existing approaches and technologies, however, careful integrated of layered biomarkers can provide a significant advantage over current clinical paradigms for OPC. For widespread clinical translation it is critical to identify circulating markers (high biological resolution) and imaging modalities (high temporal and spatial resolution) which can be rapidly deployed and relatively cost-effective. Finally, the entire platform and associated algorithms must be readily replicated across institutions and healthcare delivery systems. Our patients deserve no less.
Author contributions
SL: Conceptualization, Formal analysis, Funding acquisition, Project administration, Supervision, Writing – original draft, Writing – review & editing. VS: Conceptualization, Formal analysis, Funding acquisition, Project administration, Supervision, Writing – original draft, Writing – review & editing. RK: Writing – original draft, Writing – review & editing.
Funding
The author(s) declare financial support was received for the research, authorship, and/or publication of this article. This work was supported by a Career Development Award to VS from the Veterans Administration Clinical Science Research and Development division (1IK2CX001953). VS is supported by an American Cancer Society Research Scholar Grant RSG-21-182-01-CDP. SL and VS receive research support from R01DE025248, R01CA280980, U01DE032168, and U54CA274321. SL has additional research funding from R01DE032521, R01DE028290, R21CA259839, and T32CA261856.
Conflict of interest
The authors declare that the research was conducted in the absence of any commercial or financial relationships that could be construed as a potential conflict of interest.
Publisher’s note
All claims expressed in this article are solely those of the authors and do not necessarily represent those of their affiliated organizations, or those of the publisher, the editors and the reviewers. Any product that may be evaluated in this article, or claim that may be made by its manufacturer, is not guaranteed or endorsed by the publisher.
References
1. Siegel RL, Miller KD, Fuchs HE, Jemal A. Cancer Statistics, 2021. CA: A Cancer J Clin. (2021) 71:7–33. doi: 10.3322/caac.21654
2. Zhang Y, Fakhry C, D'Souza G. Projected Association of Human Papillomavirus Vaccination With Oropharynx Cancer Incidence in the US, 2020-2045. JAMA Oncol. (2021) 7:e212907. doi: 10.1001/jamaoncol.2021.2907
3. Dahlstrom KR, Calzada G, Hanby JD, Garden AS, Glisson BS, Li G, et al. An evolution in demographics, treatment, and outcomes of oropharyngeal cancer at a major cancer center: a staging system in need of repair. Cancer. (2013) 119:81–9. doi: 10.1002/cncr.27727
4. Sandulache VC, Hamblin J, Lai S, Pezzi T, Skinner HD, Khan NA, et al. Oropharyngeal squamous cell carcinoma in the veteran population: Association with traditional carcinogen exposure and poor clinical outcomes. Head Neck. (2015) 37:1246–53. doi: 10.1002/hed.23740
5. Chaturvedi AK, Engels EA, Pfeiffer RM, Hernandez BY, Xiao W, Kim E, et al. Human Papillomavirus and Rising Oropharyngeal Cancer Incidence in the United States. J Clin Oncol. (2011) 29:4294–301. doi: 10.1200/jco.2011.36.4596
6. Lu DJ, Luu M, Mita A, Scher K, Shiao SL, Yoshida EP, et al. Human papillomavirus–associated oropharyngeal cancer among patients aged 70 and older: Dramatically increased prevalence and clinical implications. Eur J Cancer. (2018) 103:195–204. doi: 10.1016/j.ejca.2018.08.015
7. Gupta SM, Mania-Pramanik J. Molecular mechanisms in progression of HPV-associated cervical carcinogenesis. J BioMed Sci. (2019) 26:28. doi: 10.1186/s12929-019-0520-2
8. Castellsague X. Natural history and epidemiology of HPV infection and cervical cancer. Gynecol Oncol. (2008) 110:S4–7. doi: 10.1016/j.ygyno.2008.07.045
9. Shrestha AD, Neupane D, Vedsted P, Kallestrup P. cervical cancer prevalence, incidence and mortality in low and middle income countries: A systematic review. Asian Pac J Cancer Prev. (2018) 19:319–24. doi: 10.22034/APJCP.2018.19.2.319
10. Ang KK, Harris J, Wheeler R, Weber R, Rosenthal DI, Nguyen-Tan PF, et al. Human papillomavirus and survival of patients with oropharyngeal cancer. N Engl J Med. (2010) 363:24–35. doi: 10.1056/NEJMoa0912217
11. Gillison ML, D'Souza G, Westra W, Sugar E, Xiao W, Begum S, et al. Distinct risk factor profiles for human papillomavirus type 16-positive and human papillomavirus type 16-negative head and neck cancers. J Natl Cancer Inst. (2008) 100:407–20. doi: 10.1093/jnci/djn025
12. Gleber-Netto FO, Rao X, Guo T, Xi Y, Gao M, Shen L, et al. Variations in HPV function are associated with survival in squamous cell carcinoma. JCI Insight. (2019) 4(1):e124762. doi: 10.1172/jci.insight.124762
13. Muller S, Khuri FR, Kono SA, Beitler JJ, Shin DM, Saba NF. HPV positive squamous cell carcinoma of the oropharynx. Are we observing an unusual Pattern metastases? Head Neck Pathol. (2012) 6:336–44. doi: 10.1007/s12105-012-0355-6
14. Shay SG, Chang E, Lewis MS, Wang MB. Characteristics of Human Papillomavirus- Associated Head and Neck Cancers in a Veteran Population. JAMA Otolaryngol Head Neck Surg. (2015) 141:790–6. doi: 10.1001/jamaoto.2015.1447
15. Garnaes E, Kiss K, Andersen L, Therkildsen MH, Franzmann MB, Filtenborg-Barnkob B, et al. A high and increasing HPV prevalence in tonsillar cancers in Eastern Denmark, 2000–2010: The largest registry-based study to date. Int J Cancer. (2015) 136:2196–203. doi: 10.1002/ijc.29254
16. Garnaes E, Kiss K, Andersen L, Therkildsen MH, Franzmann MB, Filtenborg-Barnkob B, et al. Increasing incidence of base of tongue cancers from 2000 to 2010 due to HPV: the largest demographic study of 210 Danish patients. Br J Of Cancer. (2015) 113:131. doi: 10.1038/bjc.2015.198. https://www.nature.com/articles/bjc2015198#supplementary-information.
17. Rietbergen MM, Leemans CR, Bloemena E, Heideman DAM, Braakhuis BJM, Hesselink AT, et al. Increasing prevalence rates of HPV attributable oropharyngeal squamous cell carcinomas in the Netherlands as assessed by a validated test algorithm. Int J Cancer. (2013) 132:1565–71. doi: 10.1002/ijc.27821
18. Shin A, Jung Y-S, Jung K-W, Kim K, Ryu J, Won Y-J. Trends of human papillomavirus-related head and neck cancers in Korea: National cancer registry data. Laryngoscope. (2013) 123:E30–E37. doi: 10.1002/lary.24243
19. Hwang T-Z, Hsiao J-R, Tsai C-R, Chang JS. Incidence trends of human papillomavirus-related head and neck cancer in Taiwan, 1995–2009. Int J Cancer. (2015) 137:395–408. doi: 10.1002/ijc.29330
20. O'Sullivan B, Huang SH, Su J, Garden AS, Sturgis EM, Dahlstrom K, et al. Development and validation of a staging system for HPV-related oropharyngeal cancer by the International Collaboration on Oropharyngeal cancer Network for Staging (ICON-S): a multicentre cohort study. Lancet Oncol. (2016) 17:440–51. doi: 10.1016/S1470-2045(15)00560-4
21. Gillison ML, Trotti AM, Harris J, Eisbruch A, Harari PM, Adelstein DJ, et al. Radiotherapy plus cetuximab or cisplatin in human papillomavirus-positive oropharyngeal cancer (NRG Oncology RTOG 1016): a randomised, multicentre, non-inferiority trial. Lancet. (2019) 393:40–50. doi: 10.1016/S0140-6736(18)32779-X
22. Mehanna H, Robinson M, Hartley A, Kong A, Foran B, Fulton-Lieuw T, et al. Radiotherapy plus cisplatin or cetuximab in low-risk human papillomavirus-positive oropharyngeal cancer (De- ESCALaTE HPV): an open-label randomised controlled phase 3 trial. Lancet. (2019) 393:51–60. doi: 10.1016/S0140-6736(18)32752-1
23. Park J, McPike V, Kambhampati S, Huang C, Fields-Meehan J, Verkruyse L, et al. Positivity Rates in Oropharyngeal and Nonoropharyngeal Head and Neck Cancer in the VA. Fed Pract. (2018) 35:S44–S47.
24. Feinstein AJ, Shay SG, Chang E, Lewis MS, Wang MB. Treatment outcomes in veterans with HPV-positive head and neck cancer. Am J Otolaryngol. (2017) 38:188–92. doi: 10.1016/j.amjoto.2017.01.005
25. Zevallos JP, Sandulache VC, Hamblin J, Skinner HD, Kramer J, Hartman CM, et al. Impact of race on oropharyngeal squamous cell carcinoma presentation and outcomes among veterans. Head Neck. (2016) 38:44–50. doi: 10.1002/hed.23836
26. Fakhry C, Zhang Q, Gillison ML, Nguyen-Tan PF, Rosenthal DI, Weber RS, et al. Validation of NRG oncology/RTOG-0129 risk groups for HPV-positive and HPV-negative oropharyngeal squamous cell cancer: Implications for risk-based therapeutic intensity trials. Cancer. (2019) 125:2027–38. doi: 10.1002/cncr.32025
27. Elhalawani H, Mohamed ASR, Elgohari B, Lin TA, Sikora AG, Lai SY, et al. Tobacco exposure as a major modifier of oncologic outcomes in human papillomavirus (HPV) associated oropharyngeal squamous cell carcinoma. BMC Cancer. (2020) 20:912. doi: 10.1186/s12885-020-07427-7
28. Vawda N, Banerjee RN, Debenham BJ. Impact of Smoking on Outcomes of HPV-related Oropharyngeal Cancer Treated with Primary Radiation or Surgery. Int J Radiat Oncol Biol Phys. (2019) 103:1125–31. doi: 10.1016/j.ijrobp.2018.11.046
29. Koyuncu CF, Lu C, Bera K, Zhang Z, Xu J, Andrea Toro Castano P, et al. Computerized tumor multinucleation index (MuNI) is prognostic in p16+ oropharyngeal carcinoma: A multi-site validation study. J Clin Invest. (2021) 131(8):e145488. doi: 10.1172/JCI145488
30. Corredor G, Toro P, Koyuncu C, Lu C, Buzzy C, Bera K, et al. An Imaging Biomarker of Tumor- Infiltrating Lymphocytes to Risk-Stratify Patients with HPV-Associated Oropharyngeal Cancer. J Natl Cancer Inst. (2021) 114(4):609–17. doi: 10.1093/jnci/djab215
31. Nichols AC, Theurer J, Prisman E, Read N, Berthelet E, Tran E, et al. Radiotherapy versus transoral robotic surgery and neck dissection for oropharyngeal squamous cell carcinoma (ORATOR): an open-label, phase 2, randomised trial. Lancet Oncol. (2019) 20:1349–59. doi: 10.1016/S1470-2045(19)30410-3
32. Nichols AC, Theurer J, Prisman E, Read N, Berthelet E, Tran E, et al. Randomized trial of radiotherapy versus transoral robotic surgery for oropharyngeal squamous cell carcinoma: long- term results of the ORATOR Trial. J Clin Oncol. (2022) 40:866–75. doi: 10.1200/JCO.21.01961
33. Nichols AC, Yoo J, Hammond JA, Fung K, Winquist E, Read N, et al. Early-stage squamous cell carcinoma of the oropharynx: radiotherapy vs. trans-oral robotic surgery (ORATOR)–study protocol for a randomized phase II trial. BMC Cancer. (2013) 13:133. doi: 10.1186/1471-2407-13-133
34. Lee NY, Ferris RL, Psyrri A, Haddad RI, Tahara M, Bourhis J, et al. Avelumab plus standard-of- care chemoradiotherapy versus chemoradiotherapy alone in patients with locally advanced squamous cell carcinoma of the head and neck: a randomised, double-blind, placebo-controlled, multicentre, phase 3 trial. Lancet Oncol. (2021) 22:450–62. doi: 10.1016/S1470-2045(20)30737-3
35. Beadle BM, Liao KP, Giordano SH, Garden AS, Hutcheson KA, Lai SY, et al. Reduced feeding tube duration with intensity-modulated radiation therapy for head and neck cancer: A Surveillance, Epidemiology, and End Results-Medicare Analysis. Cancer. (2017) 123:283–93. doi: 10.1002/cncr.30350
36. Bhayani MK, Hutcheson KA, Barringer DA, Lisec A, Alvarez CP, Roberts DB, et al. Gastrostomy tube placement in patients with oropharyngeal carcinoma treated with radiotherapy or chemoradiotherapy: factors affecting placement and dependence. Head Neck. (2013) 35:1634–40. doi: 10.1002/hed.23200
37. Goepfert RP, Lewin JS, Barrow MP, Fuller CD, Lai SY, Song J, et al. Predicting two-year longitudinal MD Anderson Dysphagia Inventory outcomes after intensity modulated radiotherapy for locoregionally advanced oropharyngeal carcinoma. Laryngoscope. (2017) 127:842–8. doi: 10.1002/lary.26153
38. Goepfert RP, Lewin JS, Barrow MP, Gunn GB, Fuller CD, Beadle BM, et al. Long-term, prospective performance of the md anderson dysphagia inventory in "Low-Intermediate Risk" oropharyngeal carcinoma after intensity modulated radiation therapy. Int J Radiat Oncol Biol Phys. (2017) 97:700–8. doi: 10.1016/j.ijrobp.2016.06.010
39. Hutcheson KA, Barrow MP, Lisec A, Barringer DA, Gries K, Lewin JS. What is a clinically relevant difference in MDADI scores between groups of head and neck cancer patients? Laryngoscope. (2016) 126:1108–13. doi: 10.1002/lary.25778
40. Hutcheson KA, Nurgalieva Z, Zhao H, Gunn GB, Giordano SH, Bhayani MK, et al. Two-year prevalence of dysphagia and related outcomes in head and neck cancer survivors: An updated SEER- Medicare analysis. Head Neck. (2019) 41:479–87. doi: 10.1002/hed.25412
41. Wong ATT, Lai SY, Gunn GB, Beadle BM, Fuller CD, Barrow MP, et al. Symptom burden and dysphagia associated with osteoradionecrosis in long-term oropharynx cancer survivors: A cohort analysis. Oral Oncol. (2017) 66:75–80. doi: 10.1016/j.oraloncology.2017.01.006
42. Chhabria K, Kansara S, Badr H, Stach C, Vernese M, Lerner A, et al. Gastrostomy utilization by oropharyngeal cancer patients is partially driven by swallowing function. Laryngoscope. (2019) 130(9):2153–9. doi: 10.1002/lary.28312
43. Harms A, Kansara S, Stach C, Richardson PA, Chen G, Lai S, et al. Swallowing function in survivors of oropharyngeal cancer is associated with advanced t classification. Ann Otol Rhinol Laryngol. (2019) 128:696–703. doi: 10.1177/0003489419839091
44. Yom SS, Torres-Saavedra P, Caudell JJ, Waldron JN, Gillison ML, Xia P, et al. Reduced-Dose Radiation Therapy for HPV-Associated Oropharyngeal Carcinoma (NRG Oncology HN002). J Clin Oncol. (2021) 39:956–65. doi: 10.1200/JCO.20.03128
45. Ferris RL, Flamand Y, Weinstein GS, Li S, Quon H, Mehra R, et al. Phase II randomized trial of transoral surgery and low-dose intensity modulated radiation therapy in resectable p16+ locally advanced oropharynx cancer: An ECOG-ACRIN cancer research group trial (E3311). J Clin Oncol. (2022) 40:138–49. doi: 10.1200/JCO.21.01752
46. Wilde DC, Castro PD, Bera K, Lai S, Madabhushi A, Corredor G, et al. Oropharyngeal cancer outcomes correlate with p16 status, multinucleation and immune infiltration. Mod Pathol. (2022) 35(8):1045–54. doi: 10.1038/s41379-022-01024-8
47. Sandulache VC, Michikawa C, Kataria P, Gleber-Netto FO, Bell D, Trivedi S, et al. High-risk TP53 mutations are associated with extranodal extension in oral cavity squamous cell carcinoma. Clin Cancer Res. (2018) 24:1727–33. doi: 10.1158/1078-0432.CCR-17-0721
48. Sandulache VC, Vandelaar LJ, Skinner HD, Cata J, Hutcheson K, Fuller CD, et al. Salvage total laryngectomy after external-beam radiotherapy: A 20-year experience. Head Neck. (2016) 38 Suppl 1:E1962–1968. doi: 10.1002/hed.24355
49. Sandulache VC, Kubik MW, Skinner HD, Malsky JA, Gelbard AH, Zevallos JP. Impact of race/ethnicity on laryngeal cancer in patients treated at a Veterans Affairs Medical Center. Laryngoscope. (2013) 123:2170–5. doi: 10.1002/lary.24058
50. Gillison ML, Chaturvedi AK, Anderson WF, Fakhry C. Epidemiology of Human Papillomavirus- Positive Head and Neck Squamous Cell Carcinoma. J Clin Oncol. (2015) 33:3235–42. doi: 10.1200/JCO.2015.61.6995
51. Haddad R, O'Neill A, Rabinowits G, Tishler R, Khuri F, Adkins D, et al. Induction chemotherapy followed by concurrent chemoradiotherapy (sequential chemoradiotherapy) versus concurrent chemoradiotherapy alone in locally advanced head and neck cancer (PARADIGM): a randomised phase 3 trial. Lancet Oncol. (2013) 14:257–64. doi: 10.1016/S1470-2045(13)70011-1
52. Cohen EE, Karrison TG, Kocherginsky M, Mueller J, Egan R, Huang CH, et al. Phase III randomized trial of induction chemotherapy in patients with N2 or N3 locally advanced head and neck cancer. J Clin Oncol. (2014) 32:2735–43. doi: 10.1200/JCO.2013.54.6309
53. Guo TW, Saiyed F, Yao C, Kiong KL, Martinez J, Sacks R, et al. Outcomes of patients with oropharyngeal squamous cell carcinoma treated with induction chemotherapy followed by concurrent chemoradiation compared with those treated with concurrent chemoradiation. Cancer. (2021) 127:2916–25. doi: 10.1002/cncr.33491
54. Lacas B, Carmel A, Landais C, Wong SJ, Licitra L, Tobias JS, et al. Meta-analysis of chemotherapy in head and neck cancer (MACH-NC): An update on 107 randomized trials and 19,805 patients, on behalf of MACH-NC Group. Radiother Oncol. (2021) 156:281–93. doi: 10.1016/j.radonc.2021.01.013
55. Koyuncu CF, Nag R, Lu C, Corredor G, Viswanathan VS, Sandulache VC, et al. Image analysis reveals differences in tumor multinucleations in Black and White patients with human papillomavirus-associated oropharyngeal squamous cell carcinoma. Cancer. (2022) 128:3831–42. doi: 10.1002/cncr.34446
56. Wahle BM, Zolkind P, Ramirez RJ, Skidmore ZL, Anderson SR, Mazul A, et al. Integrative genomic analysis reveals low T-cell infiltration as the primary feature of tobacco use in HPV-positive oropharyngeal cancer. iScience. (2022) 25:104216. doi: 10.1016/j.isci.2022.104216
57. Falato C, Schettini F, Pascual T, Braso-Maristany F, Prat A. Clinical implications of the intrinsic molecular subtypes in hormone receptor-positive and HER2-negative metastatic breast cancer. Cancer Treat Rev. (2022) 112:102496. doi: 10.1016/j.ctrv.2022.102496
58. Pagliuca M, Donato M, D'Amato AL, Rosanova M, Russo AOM, Scafetta R, et al. New steps on an old path: Novel estrogen receptor inhibitors in breast cancer. Crit Rev Oncol Hematol. (2022) 180:103861. doi: 10.1016/j.critrevonc.2022.103861
59. Giugliano F, Crimini E, Tarantino P, Zagami P, Uliano J, Corti C, et al. First line treatment of BRAF mutated advanced melanoma: Does one size fit all? Cancer Treat Rev. (2021) 99:102253. doi: 10.1016/j.ctrv.2021.102253
60. Bonomi PD, Gandara D, Hirsch FR, Kerr KM, Obasaju C, Paz-Ares L, et al. Predictive biomarkers for response to EGFR-directed monoclonal antibodies for advanced squamous cell lung cancer. Ann Oncol. (2018) 29:1701–9. doi: 10.1093/annonc/mdy196
61. Spiotto MT, Taniguchi CM, Klopp AH, Colbert LE, Lin SH, Wang L, et al. Biology of the Radio- and Chemo-Responsiveness in HPV Malignancies. Semin Radiat Oncol. (2021) 31:274–85. doi: 10.1016/j.semradonc.2021.02.009
62. Kemnade JO, Elhalawani H, Castro P, Yu J, Lai S, Ittmann M, et al. CD8 infiltration is associated with disease control and tobacco exposure in intermediate-risk oropharyngeal cancer. Sci Rep. (2020) 10:243. doi: 10.1038/s41598-019-57111-5
63. Ferris RL, Blumenschein G Jr., Fayette J, Guigay J, Colevas AD, Licitra L, et al. Nivolumab for Recurrent Squamous-Cell Carcinoma of the Head and Neck. N Engl J Med. (2016) 375:1856–67. doi: 10.1056/NEJMoa1602252
64. Molkentine DP, Molkentine JM, Bridges KA, Valdecanas DR, Dhawan A, Bahri R, et al. p16 Represses DNA Damage Repair via a Novel Ubiquitin-Dependent Signaling Cascade. Cancer Res. (2022) 82:916–28. doi: 10.1158/0008-5472.CAN-21-2101
65. Ferris RL, Blumenschein G Jr., Fayette J, Guigay J, Colevas AD, Licitra L, et al. Nivolumab vs investigator's choice in recurrent or metastatic squamous cell carcinoma of the head and neck: 2- year long-term survival update of CheckMate 141 with analyses by tumor PD-L1 expression. Oral Oncol. (2018) 81:45–51. doi: 10.1016/j.oraloncology.2018.04.008
66. Damotte D, Warren S, Arrondeau J, Boudou-Rouquette P, Mansuet-Lupo A, Biton J, et al. The tumor inflammation signature (TIS) is associated with anti-PD-1 treatment benefit in the CERTIM pan- cancer cohort. J Transl Med. (2019) 17:357. doi: 10.1186/s12967-019-2100-3
67. Danaher P, Warren S, Lu R, Samayoa J, Sullivan A, Pekker I, et al. Pan-cancer adaptive immune resistance as defined by the Tumor Inflammation Signature (TIS): results from The Cancer Genome Atlas (TCGA). J Immunother Cancer. (2018) 6:63. doi: 10.1186/s40425-018-0367-1
68. Cook S, Samuel V, Meyers DE, Stukalin I, Litt I, Sangha R, et al. Immune-Related Adverse Events and Survival Among Patients With Metastatic NSCLC Treated With Immune Checkpoint Inhibitors. JAMA Netw Open. (2024) 7:e2352302. doi: 10.1001/jamanetworkopen.2023.52302
69. Ghanbar MI, Suresh K. Pulmonary toxicity of immune checkpoint immunotherapy. J Clin Invest. (2024) 134(2):e170503. doi: 10.1172/JCI170503
70. Benhima N, Belbaraka R, Fontsa ML. Single agent vs combination immunotherapy in advanced melanoma: a review of the evidence. Curr Opin Oncol. (2024). doi: 10.1097/CCO.0000000000001014
71. Reyes-Gibby CC, Qdaisat A, Ferrarotto R, Fadol A, Bischof JJ, Coyne CJ, et al. Cardiovascular events after cancer immunotherapy as oncologic emergencies: Analyses of 610 head and neck cancer patients treated with immune checkpoint inhibitors. Head Neck. (2023) 46(3):627–35. doi: 10.1002/hed.27604
72. Kazi MA, Veeramachaneni R, Deng D, Putluri N, Cardinas M, Sikora A, et al. Glutathione peroxidase 2 is a metabolic driver of the tumor immune microenvironment and immune checkpoint inhibitor response. J ImmunoTherapy Cancer. (2022) 10(8):e004752.
73. Chen WY, Chen YL, Lin HW, Chang CF, Huang BS, Sun WZ, et al. Stereotactic body radiation combined with oncolytic vaccinia virus induces potent anti-tumor effect by triggering tumor cell necroptosis and DAMPs. Cancer Lett. (2021) 523:149–61. doi: 10.1016/j.canlet.2021.09.040
74. Zhu M, Yang M, Zhang J, Yin Y, Fan X, Zhang Y, et al. Immunogenic Cell Death Induction by Ionizing Radiation. Front Immunol. (2021) 12:705361. doi: 10.3389/fimmu.2021.705361
75. Niesters HG, van Esser J, Fries E, Wolthers KC, Cornelissen J, Osterhaus AD. Development of a real-time quantitative assay for detection of Epstein-Barr virus. J Clin Microbiol. (2000) 38:712–5. doi: 10.1128/JCM.38.2.712-715.2000
76. Bortolin MT, Pratesi C, Dolcetti R, Bidoli E, Vaccher E, Zanussi S, et al. Clinical value of Epstein- Barr virus DNA levels in peripheral blood samples of Italian patients with undifferentiated carcinoma of nasopharyngeal type. Cancer Lett. (2006) 233:247–54. doi: 10.1016/j.canlet.2005.03.015
77. Pow EH, Law MY, Tsang PC, Perera RA, Kwong DL. Salivary Epstein-Barr virus DNA level in patients with nasopharyngeal carcinoma following radiotherapy. Oral Oncol. (2011) 47:879–82. doi: 10.1016/j.oraloncology.2011.06.507
78. Fan H, Nicholls J, Chua D, Chan KH, Sham J, Lee S, et al. Laboratory markers of tumor burden in nasopharyngeal carcinoma: a comparison of viral load and serologic tests for Epstein-Barr virus. Int J Cancer. (2004) 112:1036–41. doi: 10.1002/ijc.20520
79. Lukowski SW, Tuong ZK, Noske K, Senabouth A, Nguyen QH, Andersen SB, et al. Detection of HPV E7 Transcription at Single-Cell Resolution in Epidermis. J Invest Dermatol. (2018) 138:2558–67. doi: 10.1016/j.jid.2018.06.169
80. Cohen EEW, Soulieres D, Le Tourneau C, Dinis J, Licitra L, Ahn MJ, et al. Pembrolizumab versus methotrexate, docetaxel, or cetuximab for recurrent or metastatic head-and-neck squamous cell carcinoma (KEYNOTE-040): a randomised, open-label, phase 3 study. Lancet. (2019) 393:156–67. doi: 10.1016/S0140-6736(18)31999-8
81. Berger BM, Hanna GJ, Posner MR, Genden EM, Lautersztain J, Naber SP, et al. Detection of Occult Recurrence Using Circulating Tumor Tissue Modified Viral HPV DNA among Patients Treated for HPV-Driven Oropharyngeal Carcinoma. Clin Cancer Res. (2022) 28:4292–301. doi: 10.1158/1078-0432.CCR-22-0562
82. Sandulache VC, Williams MD, Lai SY, Lu C, William WN, Busaidy NL, et al. Real-Time Genomic Characterization Utilizing Circulating Cell-Free DNA in Patients with Anaplastic Thyroid Carcinoma. Thyroid. (2017) 27:81–7. doi: 10.1089/thy.2016.0076
83. Abbosh C, Birkbak NJ, Wilson GA, Jamal-Hanjani M, Constantin T, Salari R, et al. Phylogenetic ctDNA analysis depicts early-stage lung cancer evolution. Nature. (2017) 545:446–51. doi: 10.1038/nature22364
84. Nong J, Gong Y, Guan Y, Yi X, Yi Y, Chang L, et al. Circulating tumor DNA analysis depicts subclonal architecture and genomic evolution of small cell lung cancer. Nat Commun. (2018) 9:3114. doi: 10.1038/s41467-018-05327-w
85. Cao Y, Haring CT, Brummel C, Bhambhani C, Aryal M, Lee C, et al. Early HPV ctDNA kinetics and imaging biomarkers predict therapeutic response in p16+ oropharyngeal squamous cell carcinoma. Clin Cancer Res. (2022) 28:350–9. doi: 10.1158/1078-0432.CCR-21-2338
86. Chera BS, Kumar S, Beaty BT, Marron D, Jefferys S, Green R, et al. Rapid clearance profile of plasma circulating tumor HPV Type 16 DNA during chemoradiotherapy correlates with disease control in HPV-associated oropharyngeal cancer. Clin Cancer Res. (2019) 25:4682–90. doi: 10.1158/1078-0432.CCR-19-0211
87. Cao J, Zhang M, Xie F, Lou J, Zhou X, Zhang L, et al. Exosomes in head and neck cancer: Roles, mechanisms and applications. Cancer Lett. (2020) 494:7–16. doi: 10.1016/j.canlet.2020.07.005
88. Theodoraki MN, Yerneni SS, Hoffmann TK, Gooding WE, Whiteside TL. Clinical significance of PD-L1(+) exosomes in plasma of head and neck cancer patients. Clin Cancer Res. (2018) 24:896–905. doi: 10.1158/1078-0432.CCR-17-2664
89. Principe S, Hui AB, Bruce J, Sinha A, Liu FF, Kislinger T. Tumor-derived exosomes and microvesicles in head and neck cancer: implications for tumor biology and biomarker discovery. Proteomics. (2013) 13:1608–23. doi: 10.1002/pmic.201200533
90. Wu XL, Tu Q, Faure G, Gallet P, Kohler C, Bittencourt Mde C. Diagnostic and prognostic value of circulating tumor cells in head and neck squamous cell carcinoma: a systematic review and meta-analysis. Sci Rep. (2016) 6:20210. doi: 10.1038/srep20210
91. Halmos GB, Bruine de Bruin L, Langendijk JA, van der Laan BF, Pruim J, Steenbakkers RJ. Head and neck tumor hypoxia imaging by 18F-fluoroazomycin-arabinoside (18F-FAZA)-PET: a review. Clin Nucl Med. (2014) 39:44–8. doi: 10.1097/RLU.0000000000000286
92. Gule MK, Chen Y, Sano D, Frederick MJ, Zhou G, Zhao M, et al. Targeted therapy of VEGFR2 and EGFR significantly inhibits growth of anaplastic thyroid cancer in an orthotopic murine model. Clin Cancer Res. (2011) 17:2281–91. doi: 10.1158/1078-0432.CCR-10-2762
93. Joint H, Neck Radiation Therapy MRIDC, Mohamed ASR, He R, Ding Y, Wang J, et al. Quantitative dynamic contrast-enhanced mri identifies radiation-induced vascular damage in patients with advanced osteoradionecrosis: Results of a prospective study. Int J Radiat Oncol Biol Phys. (2020) 108:1319–28. doi: 10.1016/j.ijrobp.2020.07.029
94. Joint H, Neck Radiotherapy MRIDC. Dynamic contrast-enhanced MRI detects acute radiotherapy-induced alterations in mandibular microvasculature: prospective assessment of imaging biomarkers of normal tissue injury. Sci Rep. (2016) 6:29864. doi: 10.1038/srep29864
95. Head MDA, Neck Cancer Symptom Working G. Dose-volume correlates of mandibular osteoradionecrosis in Oropharynx cancer patients receiving intensity-modulated radiotherapy: Results from a case-matched comparison. Radiother Oncol. (2017) 124:232–9. doi: 10.1016/j.radonc.2017.06.026
96. Ding Y, Hazle JD, Mohamed AS, Frank SJ, Hobbs BP, Colen RR, et al. Intravoxel incoherent motion imaging kinetics during chemoradiotherapy for human papillomavirus-associated squamous cell carcinoma of the oropharynx: preliminary results from a prospective pilot study. NMR BioMed. (2015) 28:1645–54. doi: 10.1002/nbm.3412
97. Ng SP, Cardenas CE, Bahig H, Elgohari B, Wang J, Johnson JM, et al. Changes in apparent diffusion coefficient (ADC) in serial weekly mri during radiotherapy in patients with head and neck cancer: results from the PREDICT-HN study. Curr Oncol. (2022) 29:6303–13. doi: 10.3390/curroncol29090495
98. Zhou Q, Zeng F, Ding Y, Fuller CD, Wang J. Meta-analysis of diffusion-weighted imaging for predicting locoregional failure of chemoradiotherapy in patients with head and neck squamous cell carcinoma. Mol Clin Oncol. (2018) 8:197–203. doi: 10.3892/mco.2017.1504
99. Aggarwal R, Vigneron DB, Kurhanewicz J. Hyperpolarized 1-[13C]-Pyruvate Magnetic Resonance Imaging Detects an Early Metabolic Response to Androgen Ablation Therapy in Prostate Cancer. Eur Urol. (2017) 72:1028–9. doi: 10.1016/j.eururo.2017.07.022
100. Autry AW, Park I, Kline C, Chen HY, Gordon JW, Raber S, et al. Pilot study of hyperpolarized (13)C metabolic imaging in pediatric patients with diffuse intrinsic pontine glioma and other cns cancers. AJNR Am J Neuroradiol. (2020) 42(1):178–84. doi: 10.3174/ajnr.A6937
101. Chen HY, Aggarwal R, Bok RA, Ohliger MA, Zhu Z, Lee P, et al. Hyperpolarized (13)C-pyruvate MRI detects real-time metabolic flux in prostate cancer metastases to bone and liver: a clinical feasibility study. Prostate Cancer Prostatic Dis. (2020) 23:269–76. doi: 10.1038/s41391-019-0180-z
102. Cunningham CH, Lau JY, Chen AP, Geraghty BJ, Perks WJ, Roifman I, et al. Hyperpolarized 13C Metabolic MRI of the Human Heart: Initial Experience. Circ Res. (2016) 119:1177–82. doi: 10.1161/CIRCRESAHA.116.309769
103. Gallagher FA, Woitek R, McLean MA, Gill AB, Manzano Garcia R, Provenzano E, et al. Imaging breast cancer using hyperpolarized carbon-13 MRI. Proc Natl Acad Sci United States America. (2020) 117:2092–8. doi: 10.1073/pnas.1913841117
104. Grist JT, McLean MA, Riemer F, Schulte RF, Deen SS, Zaccagna F, et al. Quantifying normal human brain metabolism using hyperpolarized [1-(13)C]pyruvate and magnetic resonance imaging. Neuroimage. (2019) 189:171–9. doi: 10.1016/j.neuroimage.2019.01.027
105. Miloushev VZ, Granlund KL, Boltyanskiy R, Lyashchenko SK, DeAngelis LM, Mellinghoff IK, et al. Metabolic imaging of the human brain with hyperpolarized (13)C pyruvate demonstrates (13)c lactate production in brain tumor patients. Cancer Res. (2018) 78:3755–60. doi: 10.1158/0008-5472.CAN-18-0221
106. Park I, Larson PEZ, Gordon JW, Carvajal L, Chen HY, Bok R, et al. Development of methods and feasibility of using hyperpolarized carbon-13 imaging data for evaluating brain metabolism in patient studies. Magn Reson Med. (2018) 80:864–73. doi: 10.1002/mrm.27077
107. Stodkilde-Jorgensen H, Laustsen C, Hansen ESS, Schulte R, Ardenkjaer-Larsen JH, Comment A, et al. Pilot study experiences with hyperpolarized [1-(13) C]pyruvate mri in pancreatic cancer patients. J Magn Reson Imaging. (2020) 51:961–3. doi: 10.1002/jmri.26888
108. Tran M, Latifoltojar A, Neves JB, Papoutsaki MV, Gong F, Comment A, et al. First-in-human in vivo non-invasive assessment of intra-tumoral metabolic heterogeneity in renal cell carcinoma. BJR Case Rep. (2019) 5(3):20190003. doi: 10.1259/bjrcr.20190003
109. Woitek R, McLean MA, Gill AB, Grist JT, Provenzano E, Patterson AJ, et al. Hyperpolarized (13)C mri of tumor metabolism demonstrates early metabolic response to neoadjuvant chemotherapy in breast cancer. Radiol Imaging Cancer. (2020) 2:e200017. doi: 10.1148/rycan.2020200017
110. Moses WW. Fundamental Limits of Spatial Resolution in PET. Nucl Instrum Methods Phys Res A 2011;. (648) Supplement1:S236–40. doi: 10.1016/j.nima.2010.11.092
111. Levin CS, Hoffman EJ. Calculation of positron range and its effect on the fundamental limit of positron emission tomography system spatial resolution. Phys Med Biol. (1999) 44:781–99.
112. Fogarty GB, Peters LJ, Stewart J, Scott C, Rischin D, Hicks RJ. The usefulness of fluorine 18-labelled deoxyglucose positron emission tomography in the investigation of patients with cervical lymphadenopathy from an unknown primary tumor. Head Neck. (2003) 25:138–45. doi: 10.1002/hed.10191
113. Hentschel M, Appold S, Schreiber A, Abramyuk A, Abolmaali N, Kotzerke J, et al. Serial FDG-PET on patients with head and neck cancer: implications for radiation therapy. Int J Radiat Biol. (2009) 85:796–804. doi: 10.1080/09553000903039180
114. Porceddu SV, Pryor DI, Burmeister E, Burmeister BH, Poulsen MG, Foote MC, et al. Results of a prospective study of positron emission tomography-directed management of residual nodal abnormalities in node-positive head and neck cancer after definitive radiotherapy with or without systemic therapy. Head Neck. (2011) 33:1675–82. doi: 10.1002/hed.21655
115. Yao M, Graham MM, Smith RB, Dornfeld KJ, Skwarchuk M, Hoffman HT, et al. Value of FDG PET in assessment of treatment response and surveillance in head-and-neck cancer patients after intensity modulated radiation treatment: a preliminary report. Int J Radiat Oncol Biol Phys. (2004) 60:1410–8. doi: 10.1016/j.ijrobp.2004.05.058
116. Lin MY, Wu M, Brennan S, Campeau MP, Binns DS, Macmanus M, et al. Absence of a Relationship between Tumor 18F-fluorodeoxyglucose Standardized Uptake Value and Survival in Patients Treated with Definitive Radiotherapy for Non-Small-Cell Lung Cancer. J Thorac Oncol. (2014) 9:377–82. doi: 10.1097/JTO.0000000000000096
117. Schinagl DA, Span PN, Oyen WJ, Kaanders JH. Can FDG PET predict radiation treatment outcome in head and neck cancer? Results of a prospective study. Eur J Nucl Med Mol Imaging. (2011) 38:1449–58. doi: 10.1007/s00259-011-1789-x
118. Jacobson O, Chen X. Interrogating tumor metabolism and tumor microenvironments using molecular positron emission tomography imaging. Theranostic approaches to improve Ther Pharmacol Rev. (2013) 65:1214–56. doi: 10.1124/pr.113.007625
119. Albers MJ, Bok R, Chen AP, Cunningham CH, Zierhut ML, Zhang VY, et al. Hyperpolarized 13C lactate, pyruvate, and alanine: noninvasive biomarkers for prostate cancer detection and grading. Cancer Res. (2008) 68:8607–15. doi: 10.1158/0008-5472.CAN-08-0749
120. Chen AP, Chu W, Gu YP, Cunnhingham CH. Probing Early Tumor Response to Radiation Therapy Using Hyperpolarized [1-(13)C]pyruvate in MDA-MB-231 Xenografts. PloS One. (2013) 8:e56551. doi: 10.1371/journal.pone.0056551PONE-D-12-14990
121. Granlund KL, Tee SS, Vargas HA, Lyashchenko SK, Reznik E, Fine S, et al. Hyperpolarized MRI of human prostate cancer reveals increased lactate with tumor grade driven by monocarboxylate transporter 1. Cell Metab. (2020) 31:105–114 e103. doi: 10.1016/j.cmet.2019.08.024
122. Garden AS, Kies MS, Morrison WH, Weber RS, Frank SJ, Glisson BS, et al. Outcomes and patterns of care of patients with locally advanced oropharyngeal carcinoma treated in the early 21st century. Radiat Oncol. (2013) 8:21. doi: 10.1186/1748-717X-8-21
123. Lai S, Wenaas AE, Sandulache VC, Hartman C, Chiao E, Kramer J, et al. Prognostic Significance of p16 Cellular Localization in Oropharyngeal Squamous Cell Carcinoma. Ann Clin Lab Sci. (2016) 46:132–9.
124. Poeta ML, Manola J, Goldwasser MA, Forastiere A, Benoit N, Califano JA, et al. TP53 mutations and survival in squamous-cell carcinoma of the head and neck. N Engl J Med. (2007) 357:2552–61. doi: 10.1056/NEJMoa073770
125. Neskey DM, Osman AA, Ow TJ, Katsonis P, McDonald T, Hicks SC, et al. Evolutionary action score of TP53 identifies high-risk mutations associated with decreased survival and increased distant metastases in head and neck cancer. Cancer Res. (2015) 75:1527–36. doi: 10.1158/0008-5472.CAN-14-2735
126. Osman AA, Neskey DM, Katsonis P, Patel AA, Ward AM, Hsu TK, et al. Evolutionary action score of TP53 coding variants is predictive of platinum response in head and neck cancer patients. Cancer Res. (2015) 75:1205–15. doi: 10.1158/0008-5472.CAN-14-2729
127. Skinner HD, Sandulache VC, Ow TJ, Meyn RE, Yordy JS, Beadle BM, et al. TP53 disruptive mutations lead to head and neck cancer treatment failure through inhibition of radiation-induced senescence. Clin Cancer Res. (2012) 18:290–300. doi: 10.1158/1078-0432.CCR-11-2260
128. Sandulache VC, Skinner HD, Wang Y, Chen Y, Dodge CT, Ow TJ, et al. Glycolytic inhibition alters anaplastic thyroid carcinoma tumor metabolism and improves response to conventional chemotherapy and radiation. Mol Cancer Ther. (2012) 11:1373–80. doi: 10.1158/1535-7163.MCT-12-0041
129. Bankson JA, Walker CM, Ramirez MS, Stefan W, Fuentes D, Merritt ME, et al. Kinetic modeling and constrained reconstruction of hyperpolarized [1-13C]-pyruvate offers improved metabolic imaging of tumors. Cancer Res. (2015) 75:4708–17. doi: 10.1158/0008-5472.CAN-15-0171
130. Rosi A, Grande S, Luciani AM, Palma A, Giovannini C, Guidoni L, et al. Role of glutathione in apoptosis induced by radiation as determined by 1H MR spectra of cultured tumor cells. Radiat Res. (2007) 167:268–82. doi: 10.1667/RR0578.1
131. Sandulache VC, Ow TJ, Pickering CR, Frederick MJ, Zhou G, Fokt I, et al. Glucose, not glutamine, is the dominant energy source required for proliferation and survival of head and neck squamous carcinoma cells. Cancer. (2011) 117:2926–38. doi: 10.1002/cncr.25868
132. Sandulache VC, Skinner HD, Ow TJ, Zhang A, Xia X, Luchak JM, et al. Individualizing antimetabolic treatment strategies for head and neck squamous cell carcinoma based on TP53 mutational status. Cancer. (2012) 118:711–21. doi: 10.1002/cncr.26321
133. Sharma PK, Dwarakanath BS, Varshney R. Radiosensitization by 2-deoxy-D-glucose and 6-aminonicotinamide involves activation of redox sensitive ASK1-JNK/p38MAPK signaling in head and neck cancer cells. Free Radic Biol Med. (2012) 53:1500–13. doi: 10.1016/j.freeradbiomed.2012.07.001S0891-5849(12)00387-5
134. Simons AL, Ahmad IM, Mattson DM, Dornfeld KJ, Spitz DR. 2-Deoxy-D-glucose combined with cisplatin enhances cytotoxicity via metabolic oxidative stress in human head and neck cancer cells. Cancer Res. (2007) 67:3364–70. doi: 10.1158/0008-5472.CAN-06-3717
135. Simons AL, Fath MA, Mattson DM, Smith BJ, Walsh SA, Graham MM, et al. Enhanced response of human head and neck cancer xenograft tumors to cisplatin combined with 2-deoxy-D-glucose correlates with increased 18F-FDG uptake as determined by PET imaging. Int J Radiat Oncol Biol Phys. (2007) 69:1222–30. doi: 10.1016/j.ijrobp.2007.07.2343
136. Simons AL, Parsons AD, Foster KA, Orcutt KP, Fath MA, Spitz DR. Inhibition of glutathione and thioredoxin metabolism enhances sensitivity to perifosine in head and neck cancer cells. J Oncol. (2009) 2009:519563. doi: 10.1155/2009/519563
137. Brizel DM, Schroeder T, Scher RL, Walenta S, Clough RW, Dewhirst MW, et al. Elevated tumor lactate concentrations predict for an increased risk of metastases in head-and-neck cancer. Int J Radiat Oncol Biol Phys. (2001) 51:349–53.
138. Quennet V, Yaromina A, Zips D, Rosner A, Walenta S, Baumann M, et al. Tumor lactate content predicts for response to fractionated irradiation of human squamous cell carcinomas in nude mice. Radiother Oncol. (2006) 81:130–5. doi: 10.1016/j.radonc.2006.08.012
139. Sandulache VC, Chen Y, Lee J, Rubinstein A, Ramirez MS, Skinner HD, et al. Evaluation of hyperpolarized [1-(1)(3)C]-pyruvate by magnetic resonance to detect ionizing radiation effects in real time. PloS One. (2014) 9:e87031. doi: 10.1371/journal.pone.0087031
140. Ramirez MS, Lee J, Walker CM, Chen Y, Kingsley CV, de la Cerda J, et al. Feasibility of multianimal hyperpolarized (13) C MRS. Magn Reson Med. (2015) 73:1726–32. doi: 10.1002/mrm.25307
141. Ramirez MS, Lee J, Walker CM, Sandulache VC, Hennel F, Lai SY, et al. Radial spectroscopic MRI of hyperpolarized [1-C] pyruvate at 7 tesla. Magn Reson Med. (2014) 72(4):986–95. doi: 10.1002/mrm.25004
143. Michel KA, Ragavan M, Walker CM, Merritt ME, Lai SY, Bankson JA. Comparison of Selective Excitation and Multi-Echo Chemical Shift Encoding for Imaging of Hyperpolarized [1-13C]Pyruvate. J Magn Reson. (2021) 325:106927.1016/j.jmr.2021.106927
144. Vaidya P, Bera K, Linden PA, Gupta A, Rajiah PS, Jones DR, et al. Combined Radiomic and Visual Assessment for Improved Detection of Lung Adenocarcinoma Invasiveness on Computed Tomography Scans: A Multi-Institutional Study. Front Oncol. (2022) 12:902056. doi: 10.3389/fonc.2022.902056
145. Jazieh K, Khorrami M, Saad A, Gad M, Gupta A, Patil P, et al. Novel imaging biomarkers predict outcomes in stage III unresectable non-small cell lung cancer treated with chemoradiation and durvalumab. J Immunother Cancer. (2022) 10(3):e003778. doi: 10.1136/jitc-2021-003778
Keywords: oropharynx, hyperpolarized MRI, circulating tumor cells, cell free DNA, smoking, radiation
Citation: Sandulache VC, Kirby RP and Lai SY (2024) Moving from conventional to adaptive risk stratification for oropharyngeal cancer. Front. Oncol. 14:1287010. doi: 10.3389/fonc.2024.1287010
Received: 01 September 2023; Accepted: 20 February 2024;
Published: 14 March 2024.
Edited by:
Martin Böttcher, Otto-von-Guericke University Magdeburg, GermanyReviewed by:
Musaddiq Javvad Awan, Medical College of Wisconsin, United StatesChad Brenner, University of Michigan, United States
Copyright © 2024 Sandulache, Kirby and Lai. This is an open-access article distributed under the terms of the Creative Commons Attribution License (CC BY). The use, distribution or reproduction in other forums is permitted, provided the original author(s) and the copyright owner(s) are credited and that the original publication in this journal is cited, in accordance with accepted academic practice. No use, distribution or reproduction is permitted which does not comply with these terms.
*Correspondence: Stephen Y. Lai, sylai@mdanderson.org; Vlad C. Sandulache, Vlad.Sandulache@bcm.edu