- National Research Institute for Family Planning, Chinese Academy of Medical Sciences & Peking Union Medical College, Beijing, China
The discovery and use of exosomes ushered in a new era of cell-free therapy. Exosomes are a subgroup of extracellular vesicles that show great potential in disease treatment. Engineered exosomes. with their improved functions have attracted intense interests of their application in translational medicine research. However, the technology of engineering exosomes still faces many challenges which have been the great limitation for their clinical application. This review summarizes the current status of research on engineered exosomes and the difficulties encountered in recent years, with a view to providing new approaches and ideas for future exosome modification and new drug development.
1 Background
Following the studies of Chargaff and West in 1946, which opened the field of extracellular vesicle (EV) biology, several studies in 1990 showed that exosome expression levels were altered in disease states. Since then, research on exosomes in the field of disease treatment has grown rapidly (1–5). For example, exosomes of immune cell origin have been shown to affect the function of the immune system (6). In addition, with the development of exosome research technology, researchers have the ability to detect individual exosomes, announcing that exosome research has entered the era of individual exosomes (7, 8).
Exosomes, with an average diameter of ~100 nanometers, are a subset of EVs (9). Almost all types of cells release exosomes, which can be seen as a regular physiological activity of cells (10). Cells are the most basic building blocks of the human body, and their abnormal state often leads to disease. With the development of research methods and techniques, researchers have discovered that in addition to cells, exosomes also play a crucial role in the onset and progression of disease (9, 11, 12). Exosomes are usually characterized by low immunogenicity, high safety, high tissue penetration, and can circulate to almost all body cavities (13). In addition, exosomes secreted by different cells have different tissue selectivity (14).
With the deepening of exosome research, the great potential of engineered exosomes in the treatment of diseases, especially cancer, has been gradually recognized. Currently, engineered exosomes are mainly used to enhance the therapeutic effect of diseases by enhancing targeting, regulating gene expression, acting as drug carriers, altering the tumor microenvironment, and regulating inclusion bodies, etc. However, there is no one technique for all situations. Researchers must endow exosomes with a variety of characteristics depending on the actual need. For example, melanoma-derived, loaded doxorubicin (DOX) and peptide-targeted exosomes preferentially target disease sites while minimizing systemic off-target problems (15, 16).
This paper reviews the development process of exosomes in recent years, the application of immune cell-derived exosomes in tumor therapy and the difficulties encountered in the clinical translation of engineered exosomes. In order to provide a new method and idea for the clinical translation of clinically applied exosomes.
2 The biogenesis and isolation of exosomes
Exosome production is divided into two main steps, plasma membrane double invagination and intracellular multivesicular body (MVB) formation (10). Specifically, the plasma membrane invaginates to form a cup-shaped structure, leading to the re-formation of early sorting endosomes (ESEs). During synthesis, the trans-Golgi network and endoplasmic reticulum play a facilitating role (17–22). After a period of time, the ESE matures into late sorting endosomes (LSEs), which eventually form MVBs. MVBs are formed by double invagination of the plasma membrane, resulting in the formation of MVBs containing multiple intraluminal vesicles (ILVs). During release, MVBs are degraded by fusion mainly with lysosomes or autophagosomes or by direct fusion with plasma membranes to release exosomes (18, 23). At the same time, exosome uptake is an important step in intercellular cargo transportation. The uptake of exosomes by recipient cells occurs mainly through endocytosis, phagocytosis, or direct fusion with the plasma membrane (24–26). Exocytosis can be categorized into lattice protein-mediated exocytosis, lipid raft-mediated exocytosis, and heparan sulfate proteoglycan-dependent exocytosis. Ultimately, successful uptake of exosomes accomplishes the exchange of intercellular substances and transfers cellular information from the donor cell to the recipient cell (Figure 1).
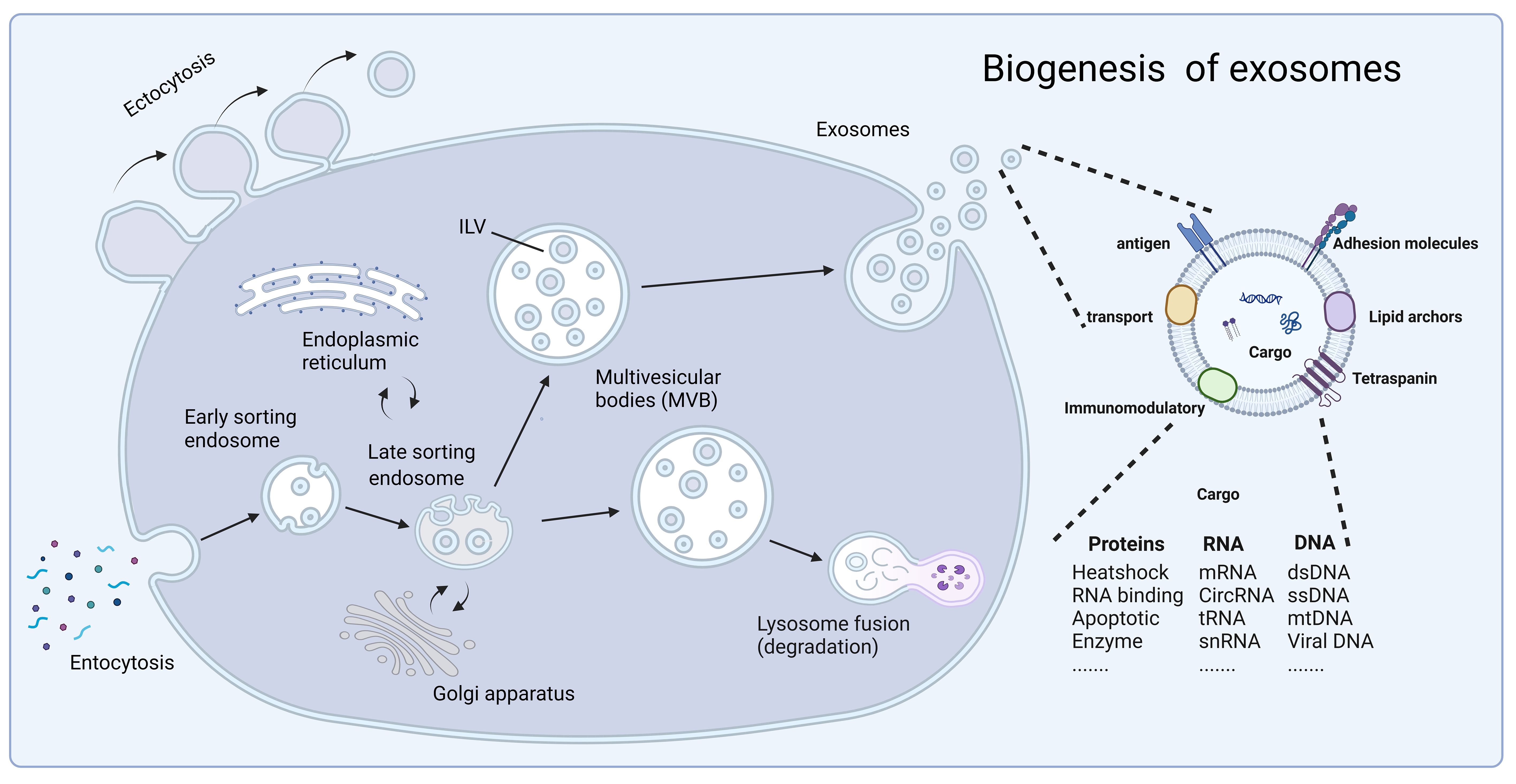
Figure 1 The biogenesis of exosomes. Fluid and extracellular components enter the cell by endocytosis, and exosome production is divided into two steps: plasma membrane double invagination and intracellular multivesicular body formation. In this, the trans-Golgi network and endoplasmic reticulum play a facilitating role. Subsequently, exosomes are released through cytolysis with lipid bilayers oriented similarly to the plasma membrane. Exosomes contain different types of cell surface proteins, intracellular proteins, RNA, DNA, amino acids, and other metabolites, and serve as mediators of proximal and distal intercellular communication in health and disease. At the same time, secretion and uptake of exosomes accomplishes the exchange of substances between cells and the transfer of cellular information from donor cells to recipient cells. Created with BioRender.com.
The isolation of exosomes is important for the study of their mechanisms and clinical applications, and the production of large-scale, high-purity and low-cost exosomes is a major challenge that limits the use of exosomes for clinical translation. Currently, the main methods for exosome isolation include ultracentrifugation, ultrafiltration, chromatography and precipitation (27). In recent years, researchers are continuously improving exosome extraction methods in order to obtain higher quantity and quality of exosomes. For example, the immunoaffinity method originally utilized protein interactions to extract exosomes. To improve this method, researchers have used submicron-sized magnetic particles for immunoaffinity capture-magnetic immunocapture, which increases the amount of exosomes captured by 10-15 times (28). Another example is the application of microfluidics to the isolation, detection and analysis of exosomes. Microfluidics-based separation techniques not only utilize common separation elements such as size and density, but also incorporate some innovative sorting devices such as electrophoresis and electromagnetism (29). The researchers utilized a micro- and nanofluidic device to separate and capture exosomes of liposarcoma origin, increasing the throughput per unit of time by a factor of five (30). While this improvement has led to improved yields of exosomes and is achievable in the laboratory, they are often limited in clinical-grade applications by various constraints, such as high costs, low yields, and complex procedures.
In conclusion, isolating high-quality exosomes is a crucial step in studying their effects in tumor therapy, and the usual methods often fail to meet the multiple requirements for isolation, such as high purity, high throughput, low cost, and few volume constraints. Therefore, integration of multiple methods is essential for isolating exosomes with high purity and yield, which is also an important direction for the future development of engineered exosomes.
3 Roles and functions of exosomes in vivo
When exosomes were discovered in the early days, researchers usually thought they were just metabolic waste products of cells (31). However, with the improvement of research techniques, researchers are coming to realize that exosomes may play an important role in the biological process of disease (10). This review summarize the role of exosomes in disease from four perspectives: material transport, information exchange, disease diagnosis and disease treatment (Figure 2).
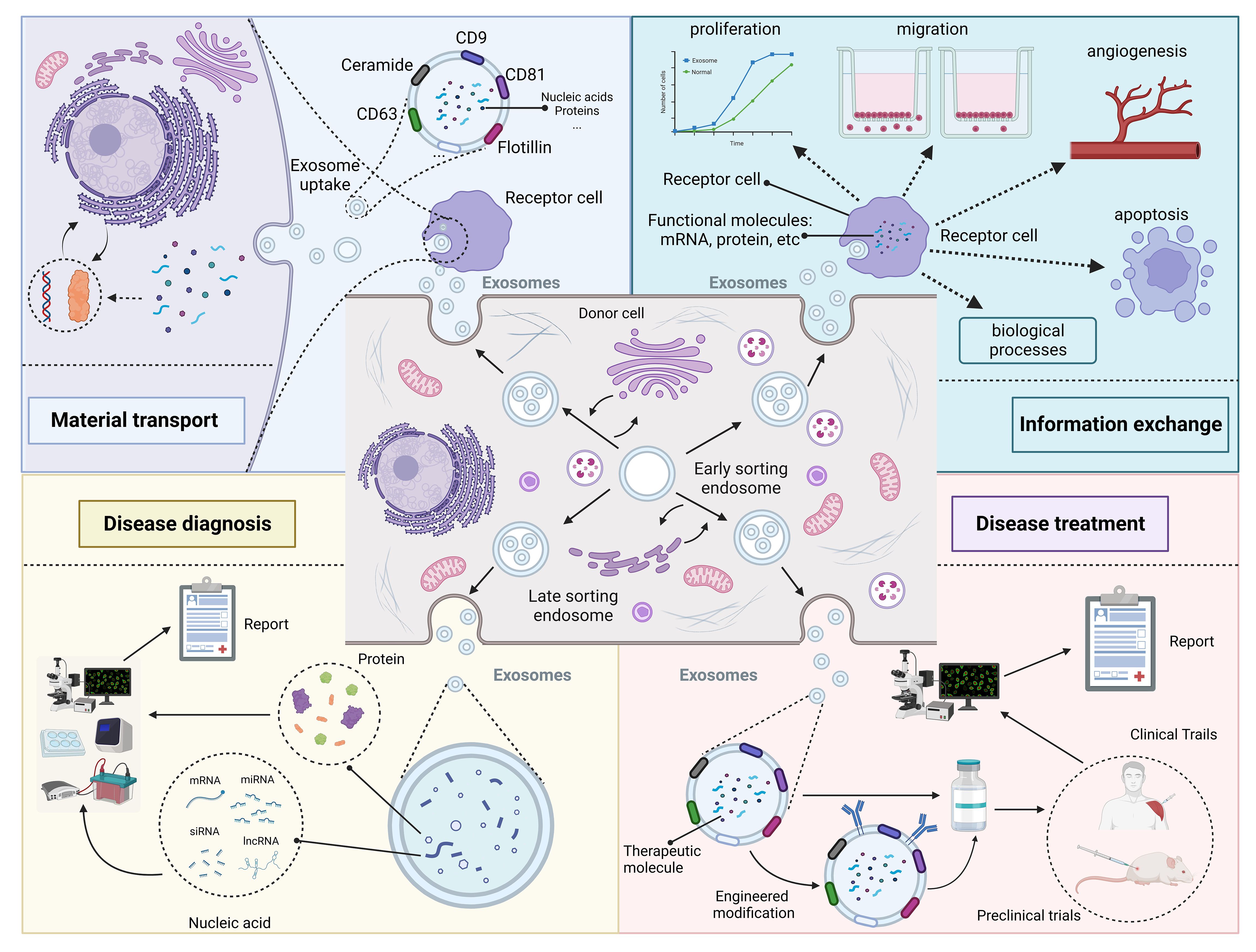
Figure 2 Roles and functions of exosomes in vivo. Exosomes from different cellular sources contain different nucleic acid and protein components and perform different functions. Some exosomes secreted by donor cells contain substances essential for the survival of recipient cells, which play a role in substance transportation. In addition, exosomes carry genetic information that can enter the recipient cell and cause changes in its phenotype. Changes in exosome composition often reflect the health status of the organism and can be used for disease diagnosis. Exosomes contain specific therapeutic molecules, such as miRNA, which can be used in disease treatment. Created with BioRender.com.
3.1 Material transport
In general, when an exosome secreted by one cell enters another cell, it brings a variety of active substances into the recipient cell, realizing the transportation of substances between different cells. Many studies have demonstrated that intercellular substance exchange is crucial for intracellular substance homeostasis. For example, mRNAs are essential for protein production in cells, and it has been found that there is a class of mRNAs specifically present in exosomes, which can accompany the exosomes into the recipient cells, translate and alter their protein expression (32, 33). In addition, some proteins undergo post-translational lipid modification, which prevents them from diffusing freely in the hydrophilic extracellular environment, making it difficult to transport substances between cells, as in the case of WNT. Exosomes, on the other hand, can transport proteins directly into recipient cells for substance replenishment (34). Indeed, exosomes have emerged as effective vehicles for the diffusion of lipophilic ligands in the extracellular environment (35–38). At the same time, uptake and efflux of exosomes are essential for the renewal of cell membrane components.
3.2 Information exchange
Recent studies have confirmed that exosomes can be transported over long distances in vivo, mediate intercellular information transfer, and affect various physiological functions of recipient cells, especially the nucleic acids and proteins contained in exosomes (39, 40). The message-exchange function of exosomes has been demonstrated in a variety of diseases, particularly cancer. For example, genetic experiments conducted by researchers in mice have shown that small amounts of functional mRNA can follow exosomes into receptor cells to act, and that the probability of this occurring is increased in mouse models with peritonitis or subcutaneous tumors (41–43). In addition to nucleic acids, exosomal proteins reflect the protein composition of donor cells, and exosomal proteins also cause phenotypic changes in recipient cells after uptake by recipient cells. In addition, exosomes secreted by glioblastoma cells that highly express epidermal growth factor receptor variant III (EGFRvIII) are specifically enriched for migration-promoting protein molecules and enhance their migratory ability after being taken up by recipient cells. Second, neural stem cells affected by inflammatory factors produce exosomes containing interferon gamma. When ingested by receptor cells, it induces the production of interferon γ by receptor cells (44). The above results suggest that cell-generated signaling molecules can be loaded into exosomes and selectively induce specific signals in recipient cells to regulate various biological processes.
3.3 Disease diagnosis
Exosomes contain membrane proteins, cytoplasmic and nuclear proteins, extracellular matrix proteins, metabolites and nucleic acids (45–48). Meanwhile, exosomes are heterogeneous; exosomes obtained from different cell sources, isolation methods and isolation stages have different sizes, components and functions (20, 49, 50). Exosomes from different sources may have different effects on the same cell, which may be due to differences in the inclusions of the exosomes. Also, exosomes from the same cellular source may have different effects on different receptor cells, and this heterogeneity may be due to the role and function of the receptor cells (51). Based on these reasons, the membrane proteins of exosomes, or the nucleic acids and proteins contained in exosomes, can be used as biomarkers of disease, especially in the diagnosis of cancer. In cancer, in addition to the ease of obtaining samples compared to other assays, exosomes have the significant advantage that only living cells can release exosomes, and the contents of tumor cells reveal information about the living cells of the tumor, which is more conducive to the patient’s diagnosis of the disease (52–55). In addition to their potential as diagnostic markers, exosomes can improve the sensitivity of other methods such as liquid biopsy (52). The combination of exosomal RNA and ctDNA produced a significant increase in the number of mutant copies compared to ctDNA alone, significantly improving the chances of detecting mutations from blood samples (52). Thus, the combined analysis significantly improved the correlation of biomarkers with treatment outcomes compared with ctDNA alone, and the method significantly improved the success rate of liquid biopsy trials (53, 54).
3.4 Disease treatment
Exosomes have shown great potential in the treatment of a wide range of diseases, and cell-free therapies represented by exosomes have greatly expanded the therapeutic approaches for a wide range of diseases, including cancer (56–59). First, compared with other drug delivery vehicles, exosomes as cell products have extremely low immunogenicity (60–62). For example, in triple-negative breast cancer, exosomes with effective lung-targeting ability were identified from autologous breast cancer cells, and exosomes were used to deliver siRNAs to improve drug delivery to pre-metastatic niche (PMN) in the lung. Demonstrating favorable biocompatibility, higher lung affinity and gene silencing effects, it is a promising strategy for suppressing postoperative breast cancer metastasis (63). In addition, exosomes have the ability to cross the blood-brain barrier and can circulate in the body for longer periods of time to maintain therapeutic effects (64). The ability to deliver drugs efficiently is one of the most important factors affecting the efficacy of glioblastoma (GBM), a major obstacle when the blood-brain barrier exists. Wang et al. prepared a biomimetic nanodrug delivery platform using exosomes to efficiently target the brain without target modification, and delivered drug and immune adjuvants at the same time for safe and efficient chemo- and immuno-therapy of GBM (65). Currently, cancer is one of the most important diseases facing mankind and one of the major causes of human deaths worldwide (66). Tumors are treated in a variety of ways, including surgery, radiotherapy, chemotherapy, immunotherapy, and targeted therapy. Radiotherapy and chemotherapy are very common treatments, but many patients have lower treatment effects and poorer prognosis (67, 68). Drug resistance in tumor cells is one of the underlying causes (69). It has been reported that Mesenchymal stem cell-derived (MSC-derived) exosomes can directly deliver functional proteins and RNAs, such as miRNAs, which in turn modulate apoptosis-associated proteins and reduce cellular chemotherapy resistance (70). Exosomes from immune cells can also directly kill tumor cells. For example, the study by Li et al. proved that NK cells are known to exert cytotoxicity by cleaving cytotoxic substances in granules, and the transmembrane protein Fasl on their surface determines the fate of target cells, which provides a new approach to the treatment of secondary hepatocellular carcinoma. Compared to NK cells, exosomes from NK cells are enriched to more Fasl and perforin proteins, and have a greater ability to kill tumor cells (71). Exosomes also play an important role in the remodeling of the tumor microenvironment, where tumor cells live. For example, macrophages are abundant in the TME and have two distinct phenotypes, including m1-polarized macrophages and m2-polarized macrophages. m1-polarized macrophages and their exosomes kill tumor cells by promoting immune responses in the tumor microenvironment (72). In addition to the above pathways, cellular exosomes are rich in proteins and nucleic acids (e.g., circRNAs, miRNAs, and lncRNAs), which play an important role in the malignant phenotype of tumors. In conclusion, these studies demonstrate the important function of exosomes in tumor therapy (73–75).
However, although exosomes have shown great potential in the treatment of a wide range of diseases, there are still some difficulties that need to be addressed. For example, the heterogeneity of cell growth and proliferation can lead to a decrease in exosome function, thus affecting the therapeutic effect after drug preparation. Second, exosomes are secreted by different cells in different microenvironments as a means of exchanging material and information between cells and between cells and the environment. Thus, the composition and production of cellular exosomes are to some extent related to the cellular state and cell culture conditions. Different cell culture medium compositions and oxygen levels in cell culture led to differences in the production and composition of exosomes, which in turn lead to differences in their function. Third, the composition and function of exosomes produced by different cells vary widely. The packaging mechanisms of effector molecules in exosomes of different cell types are not clear, and the existence of packaging signals that influence the entry of molecules into exosomes remains to be explored. Fourthly, the development of quality standards and the selection of quality control methods in the production process of exosomes still need to be explored, and the efficient and stable long-term storage of exosomes is also an important research element in the study of exosome chemogenesis. Engineering strategies for exosomes, such as targeted modifications, provide a new approach and idea to address these issues. In addition, engineered exosomes in clinical translation is also faced with the source, regulation and cost of many aspects of the problem, how to solve these problems will be an important direction for the future development of engineered exosomes.
4 Outstanding properties of engineered exosomes
Above, we have discussed the role of exosomes in the treatment of diseases. At the same time, exosomes can be engineered, and engineered exosomes can enhance or even give some new properties to exosomes (76). There are many studies using engineered exosomes to treat diseases (77–79). Currently, the engineering of exosomes is divided into four main routes: biological modification, immune modification, physical modification and chemical modification. The most common method for extracting exosomes is ultrafast centrifugation. In this paper, we will introduce the advantages of engineered exosomes over natural exosomes and their applications in various disease models (Figure 3).
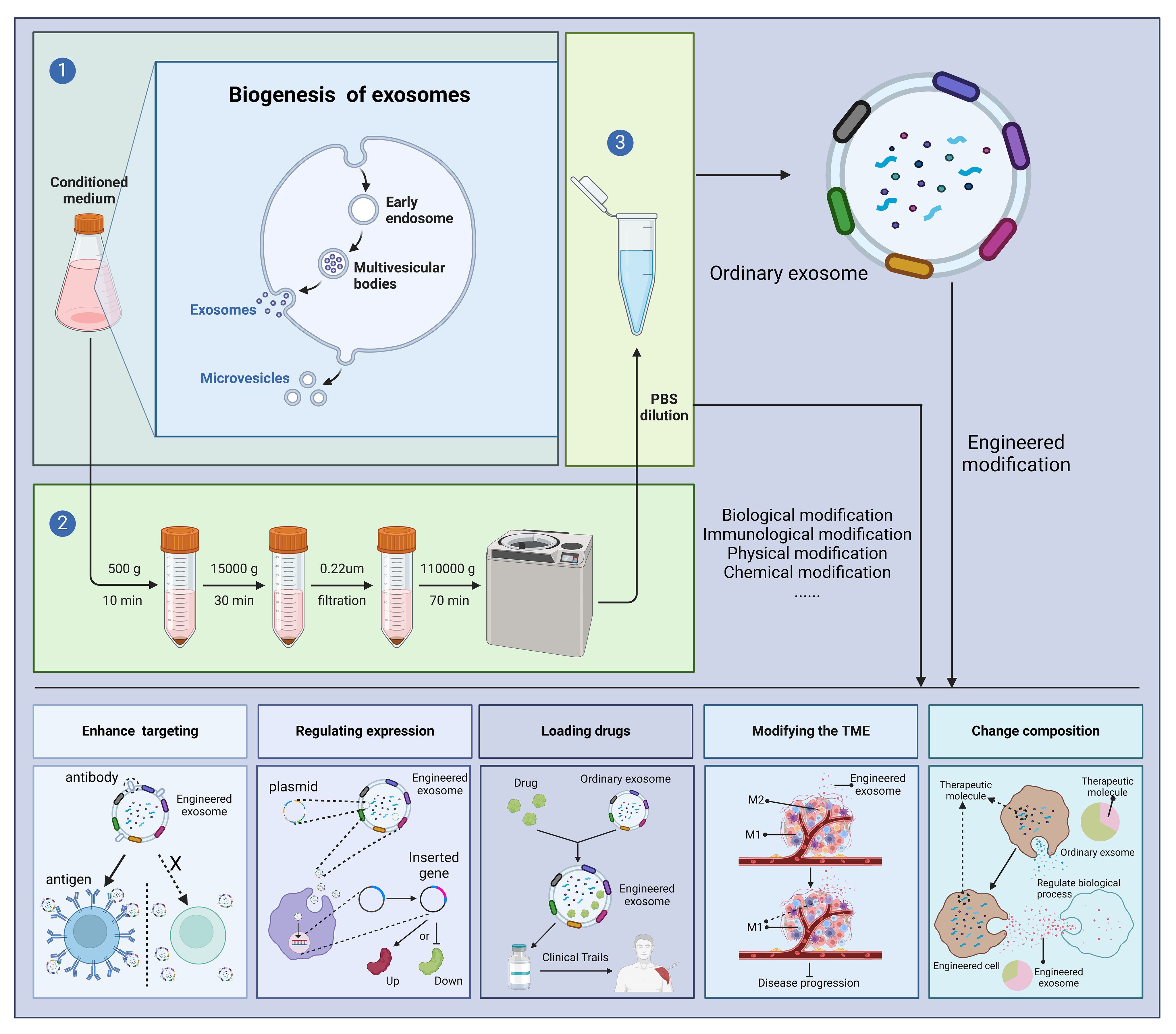
Figure 3 Outstanding properties of engineered exosomes. Ultracentrifugation is a very common method for exosome isolation. Depending on the purpose of the experiment, we can modify the donor cells or exosomes, including biological modification, immunological modification, physical modification and chemical modification, to enhance the various potentials of exosomes and to treat diseases. "X" means that in the absence of a targeting peptide, there is no targeting of the exosome into the receptor cell. Created with BioRender.com.
4.1 Enhance exosome targeting
It has been found that exosomes can enter receptor cells through a variety of pathways. It can either fuse directly with the plasma membrane or be taken up by the recipient cell through phagocytosis and endocytosis mediated by vesicular and lattice proteins (20, 80). From the current research, it is clear that exosomes can enter almost any cell. One of the fundamental reasons that exosomes are internalized by cells is the ability of these receptor cells to recognize exosome membrane surface molecules. For example, the CXCR4/SDF-1α interaction has been shown to mediate the selective transfer of endothelial colony-forming cell-derived exosomes to renal (81). These receptor-ligand interactions enhance exosome-targeted delivery (82). This mechanism of exocytosis suggests that we can design exosome membrane surface proteins to facilitate their targeted transport capacity (76). In recent years, researchers have identified a number of specific proteins on the membrane surface of exosomes, such as lysosome-associated membrane protein 2b (Lamp-2b), tetramin (CD63, CD81, CD9), lactomucin (LA), and glycosylphosphatidylinositol (GPI). In addition, surface modification strategies of exosomes, such as genetic engineering and covalent and noncovalent modification of exosomes, have been used for exosome-targeted therapy (83–87). For example, Lam2b, a commonly used membrane surface protein, is significantly enriched in dendritic cell-derived exosomes (88, 89). Lamp2b is highly expressed on the cell surface and is frequently fused to target peptides to obtain peptide-modified exosomes for disease therapy (90). Meanwhile, the use of plasmids encoding target ligands genetically engineered to fuse with transmembrane proteins in exosome donor cells has been widely used to create engineered exosomes (83). For example, Kim et al. co-transfected HEK293 cells with pcDNA - cardiac-targeting peptide (CTP)-Lamp2b to generate cardiac-targeting exosomes (91). Regardless of the modification, the ultimate goal is to allow the exosome to enter as many receptor cells as possible, rather than other cells, to improve therapeutic efficacy and minimize side effects.
4.2 Regulating gene expression
Many human diseases have a genetic basis, and the timely detection and testing of these genes is important for the diagnosis and treatment of diseases (92). Studies on exosomes have shown that their enriched nucleic acids play a crucial role in regulating gene expression in recipient cells (74, 75, 93). In addition, exosomes can serve as vectors for the regulation of gene expression. A common approach to treating hereditary diseases is gene therapy, in which genetic material is introduced to treat the disease (94, 95). The choice of vector significantly affects the efficacy of gene therapy. Adeno-associated virus (AAV) is widely used in gene therapy. However, AAV vectors often elicit an immune response in the host, leading to their rapid degradation, which seriously affects the effectiveness of gene therapy (96). Cell-secreted exosomes are highly biocompatible, low clearance, and targeted delivery, making them well suited for gene delivery (97). Encapsulation of exosomes protects AAV vectors from host cells and delivers them through the cytoplasmic membrane (98). Engineered exosomes can also be used directly as gene expression regulatory vectors (99). For example, researchers have designed an exosome-based chondrocyte-targeted miRNA delivery system for cartilage defect repair (100). Specialized exosomes loaded with the CRISPR-Cas9 system can be accurately delivered to target cells (101, 102). The lipid bilayer of the exosome membrane fuses with liposomes to form exosome-liposome heterodimers that can encapsulate and deliver large molecules of DNA (103, 104). Therefore, exosomes have great value and translational potential as gene expression regulatory vectors.
4.3 As a tool of loading drugs
In addition to serving as gene expression regulatory vectors, exosomes can also be used to deliver drug (105). Prior to the discovery of exosomes, lipid nanoparticles (LNPs) have been recognized as advantageous carriers for the protection, transport and delivery of various drugs and vaccines to cells (106). However, the low bioavailability, toxicity and immune response of LNPs complexes still constrain their clinical application (105). After the discovery of exosomes, researchers realized that exosomes have many of the properties of liposomes that give them an advantage in drug delivery, such as the ability of exosomes to cross biological barriers, low immunogenicity, and the presence of unique targeting proteins on the membrane (107, 108). Take macrophage-derived exosomes as an example. Macrophage-derived exosomes have surface membrane properties similar to those of macrophages, and thus M1 - macrophage-derived exosomes (M1-exos) can be used to deliver a variety of anticancer drugs for tumor therapy (109). Harney et al. developed M1-exo/PTX and M1-exo/DOX for solid tumor mice (110). Kim et al. use ultrasound to load PTX into M1-exos to treat drug-resistant tumors (111). Nie et al. demonstrated that M1-exo blocked CD47 and SIRPα and converted M2 macrophages to M1 macrophages, thereby enhancing macrophage phagocytosis of tumor cells (112). In addition to macrophages, exosomes from a variety of cells have been explored for the treatment of diseases, including cancer, potentially offering a new cell-free therapy.
4.4 Modifying the tumor microenvironment
The inhibitory tumor microenvironment severely affects the effectiveness of immunotherapy, and researchers have found that engineered exosomes can remodel the tumor microenvironment to improve the effectiveness of immunotherapy. For example, M1-type macrophages are usually considered as tumor-killing macrophages, which mainly play anti-tumor and immune-promoting roles. M2-type tumor-associated macrophages are a major subpopulation of suppressor immune cells. How to reprogram macrophages from M2-type to M1-type is an important way to reverse the immunosuppressive microenvironment of tumors (113, 114). M1 macrophage-derived exosomes loaded with antisense oligonucleotides (ASOs) targeting STAT6 induce the expression of nitric oxide synthase 2 (NOS2), an M1 macrophage marker that leads to the remodeling of the tumor-immunosuppressive microenvironment and the activation of CD8 t-cell-mediated adaptive immune responses (115). In addition to macrophages, fibroblast activating protein alpha (FAP) cancer-associated fibroblasts (CAF) are important targets for remodeling the tumor microenvironment. targeting of FAP genes to tumor-derived exosome-like nanovesicles (eNVs-FAP) triggers a potent and specific cytotoxic T-lymphocyte (CTL) immune response against both tumor cells and FAP CAFs in multiple models of Remodeling of immunosuppressive TME (116).
4.5 Increase therapeutic component
The role of natural exosomes in tumor therapy relies on the substances they contain, such as nucleic acids and proteins (117, 118). For these reasons, researchers have attempted to enhance the therapeutic capabilities of exosomes by altering their composition. Exosomes contain a variety of non-coding rna, especially miRNAs, which are very important exosomal cargoes that can influence the expression of various oncogenes and tumor suppressors, thus affecting the course of the disease (32, 39, 119, 120). The researchers found that insertion or deletion of these specific cellular motifs or exonic motifs in the miRNA increased or decreased the amount of the corresponding mirna in the intracellular production of exosomes. In addition, increased miRNA delivery mediated by EXOmotifs resulted in enhanced repression of target genes in distal cells (121). Therefore, improving the efficacy of exosome therapy by altering exosome composition may be a new direction.
5 Engineered exosomes can be used as drug delivery platforms for disease therapy
5.1 Exosomes as nanodelivery systems
The characterization of exosomes and the emergence of exosome engineering strategies have greatly expanded their capabilities as nanodelivery systems. First, a variety of modification strategies can enhance or confer certain properties to exosomes, and common modification strategies include biological, chemical, physical, and immunological modifications. One example regarding biomodification is the enhancement of exosome targeting by the addition of targeting peptides. For example, in a study by Shao et al. on osteosarcoma, researchers coupled Exo-MEG3 with a tumor-targeting cRGD peptide, which demonstrated precise tumor-targeting ability and enhanced anti-tumor effects in an osteosarcoma model (122). Exosomes can also be chemically modified, an example of which is the development of a dual stimulus responsive acoustic sensitizer using exosomes by Cao et al. That is, indocyanine green (ICG), which functions as both an acoustic sensitizer and a photoacoustic (PA) visualizer, was loaded into EVs along with paclitaxel (PTX) and sodium bicarbonate (SBC) to achieve combined chemoacoustic-dynamic therapy (123). Exosomes also allow for more precise tumor targeting under conditions of external physical interference. For example, Zhang et al. modified exosomes derived from neutrophils with superparamagnetic iron oxide nanoparticles (SPIONs), allowing these nanoparticles to selectively accumulate at tumor sites under the interference of external magnetic fields (124). In addition, immunomodified exosomes can elicit strong immune responses. One example is the use of exosomes with fibroblast activating protein-alpha (FAP) as a tumor vaccine, which can elicit a strong immune response leading to tumor therapy (116). In summary, engineered exosomes have been shown to transport specific substances to specific sites at specific spaces and times, which is expected to open up new areas for future drug delivery platforms.
5.2 Application of engineered exosomes in disease therapy
Disease treatment methods include general treatment, drug treatment, surgical treatment, radiation therapy and so on. With the deepening of exosome research, cell-free therapy has gradually entered people’s vision as a new disease treatment method (125). Cell-derived exosomes exhibit multiple biological activities and therapeutic potential in a wide range of diseases (125). To further enhance the efficacy of cell-free therapy, researchers have begun to modify natural exosomes, and research on engineered exosomes has addressed a wide range of diseases, including tumors (100, 122, 126). For example, in cell therapy for chronic wound healing, its effectiveness is greatly limited by immune rejection and difficulties in maintaining cellular activity (127). Exosomes can provide a therapeutic effect similar to that of promoting cell regeneration and are thought to be a way to overcome these obstacles (128). Mei et al. encapsulated humscs-derived exosomes in a bioactive scaffold composed of polyvinyl alcohol (PVA)/sodium alginate (Alg) nanohydrogel (exo@H) for wound healing in diabetic rats (127). In the treatment of osteoarthritis, drug delivery to chondrocytes through the dense, non-vascularized extracellular matrix of chondrocytes is still a challenge, and there is currently no effective treatment method (129). Due to the high permeability of exosomes and their potential as vectors, He et al. have designed an exosome-based vector to achieve specific targeted delivery of miR-140 to chondrocytes in a rat model for the treatment of osteoarthritis (85, 130, 131).
5.3 Combination of engineered exosomes and tumor therapies
Engineered exosomes can be used in combination with other therapies in addition to modifying themselves to fight tumors. First, engineered exosomes are used in conjunction with chemotherapy drugs. For example, in the treatment of ovarian cancer, researchers used HEK-293T cells and tumor-derived exosomes loaded with PTX, which not only inhibited tumor growth but also prevented breast cancer recurrence and metastasis (123). An exosome obtained from macrophages, in combination with aminoethyl anisidine-polyethylene glycol (AA-PEG) and PTX, showed significant anticancer effects (132). Among them, PEG reduced the recognition and internalization of MPS and significantly increased the residence time of exosomes in vivo (133). Second, engineered exosomes can be used in combination with radiotherapy. One example is the use of M1 macrophage-derived engineered exosomes in combination with radiotherapy to treat lung cancer by Ma et al. The mechanism is that the expression of catalase in the membrane of M1 macrophage-derived exosomes not only improves hypoxia in the tumor microenvironment, but also enhances DNA damage in tumor cells, and DNA damage repair inhibitors encapsulated in M1Exos can significantly limit DNA damage repair (134). In addition, engineered exosomes can be used in combination with gene therapy. Gene therapy involves the delivery of genetic material to a patient to treat a disease through the expression of therapeutic genes. One example is that Bose et al. encapsulated miRNA into uPA-engineered exosomes, which not only enhanced tumor-targeting ability, but also greatly improved progression-free survival of patients (135). Another example is the loading of CRISPR/Cas9 into engineered exosomes. Liu et al. loaded Cas9 RNPs into purified exosomes isolated from hepatic stellate cells by electroporation, which showed strong therapeutic potential in mouse models of acute liver injury, chronic liver fibrosis and hepatocellular carcinoma (136). In addition to the above therapies, engineered exosomes can be used in combination with photothermal and photodynamic therapies to treat tumors and have demonstrated even more powerful results, and we summarize the applications of engineered exosomes in the treatment of various diseases in recent years (Table 1).
6 Immune cell-derived engineered exosomes and tumor therapy
A variety of cells in the body can secrete exosomes, which tend to have similar properties to their secreting cells, such as anti-cancer and anti-aging (169). In recent years, numerous immunotherapies have shown great potential in cancer treatment and are gradually gaining recognition among researchers (170). Immunotherapy refers to the use of the self-protection ability of the body’s immune system to achieve the effect of killing tumors. This therapy targets human immune cells, not tumor cells, and does not cause great harm to the patient’s body as radiotherapy does, which is highly expected in terms of accuracy, effectiveness and safety (171). However, problems such as short survival time and duration of immune cells and difficulty in breaking through the solid tumor microenvironment constrain the effectiveness of immunotherapy (172). Exosomes based on immune cell sources are emerging as a potential therapeutic approach to address these limitations of cell-based therapies. Exosomes derived from immune cells have some or all of the anti-tumor properties of immune cells, as well as properties such as high penetration capacity (173). Therefore, cell-free therapy is an important complement to current anti-tumor therapies. The engineering technology of immune cell-derived exosomes confers better anti-tumor properties.
6.1 T cell
T cell-derived exosomes are produced only after T cells are activated. Interactions between tetraspanins, myelin, lymphocyte proteins, and ceramides were found to be critical for the biogenesis of T cell-derived exosomes (174). The study by Wang et al. proved that T-cell-derived exosomes may reflect the immune properties of T cells, for example, killing target cells directly, acting in association with B cells, producing cytokines, and creating optimal conditions for immune cells to function in a paracrine or autocrine form (175). Currently, engineering for T-lymphocytes is mainly focused on acting as gene expression vectors, which means constructing himeric antigen receptor T (CAR-T) cells for tumor therapy (176). Zhu et al. proved that CAR-T cell-derived exosomes can reduce the cytotoxicity of CAR-T therapies and have the ability to cross the blood-brain barrier and carry many cytotoxic molecules (FasL, Apo2L, perforin, etc.), which have demonstrated great efficacy as a gene-expression-regulating vector in tumor therapy (177). Hong et al.demonstrated that CAR-T cell-derived exosomes inhibit solid tumors, including triple negative breast cancer and lung cancer, and can affect the tumor microenvironment with relative safety (178, 179). Meanwhile, Haque et al. found that CAR-T cell-derived exosomes can be used to enhance the effects of cancer immunochemotherapy as well as to induce cell-contact toxicity (177, 180). These results demonstrate the great potential of T cell-derived exosomes for tumor therapy.
6.2 Natural killer cell
Natural killer cells (NK cells) are important immune cells in the body, derived from bone marrow, belong to the lymphocytes, the third type of lymphocytes except T cells and B cells, and can kill tumor cells non-specifically without prior sensitization (181). NK cells are classified into four main anti-tumor modalities: perforin/granzyme pathway, mediation of tumor apoptosis, ADCC pathway, and secretion of cytokines. NK cell-derived exosomes possess anti-tumor functions similar to those of their secreting cells (182). However, NK cells are a somewhat heterogeneous group of cells with differences in the function of their derived exosomes, potentially affecting future therapeutic outcomes. NK92 cells are human-derived NK cell lines that have a stable source, less difficulty in genetic modification manipulation relative to primary NK cells, and greater cytotoxicity and cytokine-producing capacity relative to primary NK cells (183). NK92 cells are human-derived NK cell lines with stable source, lower difficulty of gene modification operation relative to NK cells, and stronger cytotoxicity and cytokine-producing ability relative to NK cells, which is a hotspot for anti-tumor research at present (184). In view of the therapeutic properties of NK92 cell exosomes, researchers have modified the exosome surface proteins, combined them with single-chain antibodies recognizing tumor-associated antigens, and established the exosome surface display technology of single-chain antibodies to tumor-associated antigens, which enhances the broad-spectrum tumor targeting of the exosomes or the targeting of specific tumor cells or improves the therapeutic efficacy of antitumor drugs through the endogenous overexpression of effector molecules in the cells (185).
6.3 Other innate immune cells
Neutrophils are the most abundant innate immune cells circulating in the body, and their derived exosomes induce apoptosis in tumor cells by delivering cytotoxic proteins and activating the caspase signaling pathway (124). Based on these properties, the researchers modified N-Ex with superparamagnetic iron oxide nanoparticles (SPIONs) for higher tumor-targeting therapeutic effects. Meanwhile, exosome-loaded DOX was used to enhance the inhibition of tumor cells (124). Dendritic cells (DCs) are sentinel antigen-presenting cells of the immune system. DC-derived exosomes, which also contain functional MHC peptide complexes, co-stimulatory molecules, and other components that interact with immune cells, have the potential to promote immune cell-dependent tumor rejection and offer significant advantages over cell-based immunotherapies involving DC (186). Macrophages are immune cells that are widely distributed in the blood and tissues and are classified into M1 and M2 types (187, 188). M1-Exos)and M2-Exos have different functions, and the direction of macrophage polarization also affects the therapeutic efficacy, reprogramming of macrophages induces macrophage polarization in the M1-type direction and improves the therapeutic efficacy (189).
7 Conclusion
There is growing evidence that exosomes can be used as a vehicle for disease treatment with encouraging results. However, natural exosomes have some drawbacks, for example, heterogeneity, which can greatly reduce the effectiveness of disease treatment. Unlike natural exosomes, engineered exosomes can be modified in a variety of ways according to specific human wishes, thus enhancing or even conferring some new properties, and exosomes of immune cell origin have shown great potential in tumor therapy. However, the clinical translation of engineered exosomes also faces some difficulties, such as exosome isolation, quantification and analysis of exosomes in the clinical stage of engineered exosomes standardized methods still lack consensus, and how to accurately quantify the components of exosomes is also a difficult problem. Therefore, if we want to develop exosome-based drug delivery systems on a large scale in the clinic, we need to solve the above problems first.
Author contributions
CS: Writing – original draft. JG: Writing – review & editing. XM: Writing – review & editing.
Funding
The author(s) declare that no financial support was received for the research, authorship, and/or publication of this article.
Conflict of interest
The authors declare that the research was conducted in the absence of any commercial or financial relationships that could be construed as a potential conflict of interest.
Publisher’s note
All claims expressed in this article are solely those of the authors and do not necessarily represent those of their affiliated organizations, or those of the publisher, the editors and the reviewers. Any product that may be evaluated in this article, or claim that may be made by its manufacturer, is not guaranteed or endorsed by the publisher.
Glossary
References
1. Chargaff E, West R. The biological significance of the thromboplastic protein of blood. J Biol Chem. (1946) 166:189–97. doi: 10.1016/S0021-9258(17)34997-9
2. Nunez EA, Wallis J, Gershon MD. Secretory processes in follicular cells of the bat thyroid. 3. The occurrence of extracellular vesicles and colloid droplets during arousal from hibernation. Am J Anat. (1974) 141:179–201. doi: 10.1002/aja.1001410203
3. Harding C, Heuser J, Stahl P. Receptor-mediated endocytosis of transferrin and recycling of the transferrin receptor in rat reticulocytes. J Cell Biol. (1983) 97:329–39. doi: 10.1083/jcb.97.2.329
4. Pan BT, Johnstone RM. Fate of the transferrin receptor during maturation of sheep reticulocytes in vitro: selective externalization of the receptor. Cell. (1983) 33:967–78. doi: 10.1016/0092-8674(83)90040-5
5. Powell JJ, Harvey RS, Thompson RP. Microparticles in Crohn’s disease–has the dust settled? Gut. (1996) 39:340–1. doi: 10.1136/gut.39.2.340
6. Skokos D, Botros HG, Demeure C, Morin J, Peronet R, Birkenmeier G, et al. Mast cell-derived exosomes induce phenotypic and functional maturation of dendritic cells and elicit specific immune responses in vivo. J Immunol. (2003) 170:3037–45. doi: 10.4049/jimmunol.170.6.3037
7. Verweij FJ, Revenu C, Arras G, Dingli F, Loew D, Pegtel DM, et al. Live tracking of inter-organ communication by endogenous exosomes in vivo. Dev Cell. (2019) 48:573–589 e4. doi: 10.1016/j.devcel.2019.01.004
8. Hyenne V, Ghoroghi S, Collot M, Bons J, Follain G, Harlepp S, et al. Studying the fate of tumor extracellular vesicles at high spatiotemporal resolution using the zebrafish embryo. Dev Cell. (2019) 48:554–572 e7. doi: 10.1016/j.devcel.2019.01.014
9. Dai J, Su Y, Zhong S, Cong L, Liu B, Yang J, et al. Exosomes: key players in cancer and potential therapeutic strategy. Signal Transduct Target Ther. (2020) 5:145. doi: 10.1038/s41392-020-00261-0
10. Kalluri R, LeBleu VS. The biology, function, and biomedical applications of exosomes. Science. (2020) 367(6478):eaau6977. doi: 10.1126/science.aau6977
11. Pegtel DM, Gould SJ. Exosomes. Annu Rev Biochem. (2019) 88:487–514. doi: 10.1146/annurev-biochem-013118-111902
12. Li W, Li C, Zhou T, Liu X, Liu X, Li X, et al. Role of exosomal proteins in cancer diagnosis. Mol Cancer. (2017) 16:145. doi: 10.1186/s12943-017-0706-8
13. Cecchin R, Troyer Z, Witwer K, Morris KV. Extracellular vesicles: The next generation in gene therapy delivery. Mol Ther. (2023) 31:1225–30. doi: 10.1016/j.ymthe.2023.01.021
14. Weng Z, Zhang B, Wu C, Yu F, Han B, Li B, et al. Therapeutic roles of mesenchymal stem cell-derived extracellular vesicles in cancer. J Hematol Oncol. (2021) 14:136. doi: 10.1186/s13045-021-01141-y
15. Wang J, Chen P, Dong Y, Xie H, Wang Y, Soto F, et al. Designer exosomes enabling tumor targeted efficient chemo/gene/photothermal therapy. Biomaterials. (2021) 276:121056. doi: 10.1016/j.biomaterials.2021.121056
16. Harishkumar M, Radha M, Yuichi N, Muthukalianan GK, Kaoru O, Shiomori K, et al. Designer exosomes: smart nano-communication tools for translational medicine. Bioengineering (Basel). (2021) 8(11):158. doi: 10.3390/bioengineering8110158
17. Kalluri R. The biology and function of exosomes in cancer. J Clin Invest. (2016) 126:1208–15. doi: 10.1172/JCI81135
18. van Niel G, D’Angelo G, Raposo G. Shedding light on the cell biology of extracellular vesicles. Nat Rev Mol Cell Biol. (2018) 19:213–28. doi: 10.1038/nrm.2017.125
19. McAndrews KM, Kalluri R. Mechanisms associated with biogenesis of exosomes in cancer. Mol Cancer. (2019) 18:52. doi: 10.1186/s12943-019-0963-9
20. Mathieu M, Martin-Jaular L, Lavieu G, Thery C. Specificities of secretion and uptake of exosomes and other extracellular vesicles for cell-to-cell communication. Nat Cell Biol. (2019) 21:9–17. doi: 10.1038/s41556-018-0250-9
21. Willms E, Cabanas C, Mager I, Wood MJA, Vader P. Extracellular vesicle heterogeneity: subpopulations, isolation techniques, and diverse functions in cancer progression. Front Immunol. (2018) 9:738. doi: 10.3389/fimmu.2018.00738
22. Hessvik NP, Llorente A. Current knowledge on exosome biogenesis and release. Cell Mol Life Sci. (2018) 75:193–208. doi: 10.1007/s00018-017-2595-9
23. Kahlert C, Kalluri R. Exosomes in tumor microenvironment influence cancer progression and metastasis. J Mol Med (Berl). (2013) 91:431–7. doi: 10.1007/s00109-013-1020-6
24. Wu B, Wang Q, Shi X, Jiang M. Targeting endocytosis and cell communications in the tumor immune microenvironment. Cell Commun Signal. (2022) 20:161. doi: 10.1186/s12964-022-00968-3
25. Parada N, Romero-Trujillo A, Georges N, Alcayaga-Miranda F. Camouflage strategies for therapeutic exosomes evasion from phagocytosis. J Adv Res. (2021) 31:61–74. doi: 10.1016/j.jare.2021.01.001
26. Xue Y, Riva N, Zhao L, Shieh JS, Chin YT, Gatt A, et al. Recent advances of exosomes in soft tissue injuries in sports medicine: A critical review on biological and biomaterial applications. J Control Release. (2023) 364:90–108. doi: 10.1016/j.jconrel.2023.10.031
27. Peterson MF, Otoc N, Sethi JK, Gupta A, Antes TJ. Integrated systems for exosome investigation. Methods. (2015) 87:31–45. doi: 10.1016/j.ymeth.2015.04.015
28. Zarovni N, Corrado A, Guazzi P, Zocco D, Lari E, Radano G, et al. Integrated isolation and quantitative analysis of exosome shuttled proteins and nucleic acids using immunocapture approaches. Methods. (2015) 87:46–58. doi: 10.1016/j.ymeth.2015.05.028
29. Davies RT, Kim J, Jang SC, Choi EJ, Gho YS, Park J. Microfluidic filtration system to isolate extracellular vesicles from blood. Lab Chip. (2012) 12:5202–10. doi: 10.1039/c2lc41006k
30. Casadei L, Choudhury A, Sarchet P, Mohana Sundaram P, Lopez G, Braggio D, et al. Cross-flow microfiltration for isolation, selective capture and release of liposarcoma extracellular vesicles. J Extracell Vesicles. (2021) 10:e12062. doi: 10.1002/jev2.12062
31. Johnstone RM, Adam M, Hammond JR, Orr L, Turbide C. Vesicle formation during reticulocyte maturation. Association of plasma membrane activities with released vesicles (exosomes). J Biol Chem. (1987) 262:9412–20. doi: 10.1016/S0021-9258(18)48095-7
32. Skog J, Wurdinger T, van Rijn S, Meijer DH, Gainche L, Sena-Esteves M, et al. Glioblastoma microvesicles transport RNA and proteins that promote tumour growth and provide diagnostic biomarkers. Nat Cell Biol. (2008) 10:1470–6. doi: 10.1038/ncb1800
33. Valadi H, Ekstrom K, Bossios A, Sjostrand M, Lee JJ, Lotvall JO. Exosome-mediated transfer of mRNAs and microRNAs is a novel mechanism of genetic exchange between cells. Nat Cell Biol. (2007) 9:654–9. doi: 10.1038/ncb1596
34. Maggio S, Ceccaroli P, Polidori E, Cioccoloni A, Stocchi V, Guescini M. Signal exchange through extracellular vesicles in neuromuscular junction establishment and maintenance: from physiology to pathology. Int J Mol Sci. (2019) 20(11):2804. doi: 10.3390/ijms20112804
35. Luga V, Zhang L, Viloria-Petit AM, Ogunjimi AA, Inanlou MR, Chiu E, et al. Exosomes mediate stromal mobilization of autocrine Wnt-PCP signaling in breast cancer cell migration. Cell. (2012) 151:1542–56. doi: 10.1016/j.cell.2012.11.024
36. Korkut C, Ataman B, Ramachandran P, Ashley J, Barria R, Gherbesi N, et al. Trans-synaptic transmission of vesicular Wnt signals through Evi/Wntless. Cell. (2009) 139:393–404. doi: 10.1016/j.cell.2009.07.051
37. Gross JC, Chaudhary V, Bartscherer K, Boutros M. Active Wnt proteins are secreted on exosomes. Nat Cell Biol. (2012) 14:1036–45. doi: 10.1038/ncb2574
38. Beckett K, Monier S, Palmer L, Alexandre C, Green H, Bonneil E, et al. Drosophila S2 cells secrete wingless on exosome-like vesicles but the wingless gradient forms independently of exosomes. Traffic. (2013) 14:82–96. doi: 10.1111/tra.12016
39. Xu Z, Chen Y, Ma L, Chen Y, Liu J, Guo Y, et al. Role of exosomal non-coding RNAs from tumor cells and tumor-associated macrophages in the tumor microenvironment. Mol Ther. (2022) 30:3133–54. doi: 10.1016/j.ymthe.2022.01.046
40. Lakshmi S, Hughes TA, Priya S. Exosomes and exosomal RNAs in breast cancer: A status update. Eur J Cancer. (2021) 144:252–68. doi: 10.1016/j.ejca.2020.11.033
41. Ridder K, Keller S, Dams M, Rupp AK, Schlaudraff J, Del Turco D, et al. Extracellular vesicle-mediated transfer of genetic information between the hematopoietic system and the brain in response to inflammation. PloS Biol. (2014) 12:e1001874. doi: 10.1371/journal.pbio.1001874
42. Zomer A, Maynard C, Verweij FJ, Kamermans A, Schafer R, Beerling E, et al. In Vivo imaging reveals extracellular vesicle-mediated phenocopying of metastatic behavior. Cell. (2015) 161:1046–57. doi: 10.1016/j.cell.2015.04.042
43. Tkach M, Thery C. Communication by extracellular vesicles: where we are and where we need to go. Cell. (2016) 164:1226–32. doi: 10.1016/j.cell.2016.01.043
44. Cossetti C, Iraci N, Mercer TR, Leonardi T, Alpi E, Drago D, et al. Extracellular vesicles from neural stem cells transfer IFN-gamma via Ifngr1 to activate Stat1 signaling in target cells. Mol Cell. (2014) 56:193–204. doi: 10.1016/j.molcel.2014.08.020
45. Keerthikumar S, Chisanga D, Ariyaratne D, Al Saffar H, Anand S, Zhao K, et al. ExoCarta: A web-based compendium of exosomal cargo. J Mol Biol. (2016) 428:688–92. doi: 10.1016/j.jmb.2015.09.019
46. Pathan M, Fonseka P, Chitti SV, Kang T, Sanwlani R, Van Deun J, et al. Vesiclepedia 2019: a compendium of RNA, proteins, lipids and metabolites in extracellular vesicles. Nucleic Acids Res. (2019) 47:D516–9. doi: 10.1093/nar/gky1029
47. van Balkom BW, Eisele AS, Pegtel DM, Bervoets S, Verhaar MC. Quantitative and qualitative analysis of small RNAs in human endothelial cells and exosomes provides insights into localized RNA processing, degradation and sorting. J Extracell Vesicles. (2015) 4:26760. doi: 10.3402/jev.v4.26760
48. Lasda E, Parker R. Circular RNAs co-precipitate with extracellular vesicles: A possible mechanism for circRNA clearance. PloS One. (2016) 11:e0148407. doi: 10.1371/journal.pone.0148407
49. Bebelman MP, Smit MJ, Pegtel DM, Baglio SR. Biogenesis and function of extracellular vesicles in cancer. Pharmacol Ther. (2018) 188:1–11. doi: 10.1016/j.pharmthera.2018.02.013
50. Ciardiello C, Cavallini L, Spinelli C, Yang J, Reis-Sobreiro M, de Candia P, et al. Focus on extracellular vesicles: new frontiers of cell-to-cell communication in cancer. Int J Mol Sci. (2016) 17:175. doi: 10.3390/ijms17020175
51. Wen SW, Lima LG, Lobb RJ, Norris EL, Hastie ML, Krumeich S, et al. Breast cancer-derived exosomes reflect the cell-of-origin phenotype. Proteomics. (2019) 19:e1800180. doi: 10.1002/pmic.201800180
52. Krug AK, Enderle D, Karlovich C, Priewasser T, Bentink S, Spiel A, et al. Improved EGFR mutation detection using combined exosomal RNA and circulating tumor DNA in NSCLC patient plasma. Ann Oncol. (2018) 29:2143. doi: 10.1093/annonc/mdy261
53. Castellanos-Rizaldos E, Grimm DG, Tadigotla V, Hurley J, Healy J, Neal PL, et al. Exosome-based detection of EGFR T790M in plasma from non-small cell lung cancer patients. Clin Cancer Res. (2018) 24:2944–50. doi: 10.1158/1078-0432.CCR-17-3369
54. Mohrmann L, Huang HJ, Hong DS, Tsimberidou AM, Fu S, Piha-Paul SA, et al. Liquid biopsies using plasma exosomal nucleic acids and plasma cell-free DNA compared with clinical outcomes of patients with advanced cancers. Clin Cancer Res. (2018) 24:181–8. doi: 10.1158/1078-0432.CCR-17-2007
55. Yu W, Hurley J, Roberts D, Chakrabortty SK, Enderle D, Noerholm M, et al. Exosome-based liquid biopsies in cancer: opportunities and challenges. Ann Oncol. (2021) 32:466–77. doi: 10.1016/j.annonc.2021.01.074
56. Lotfy A, AboQuella NM, Wang H. Mesenchymal stromal/stem cell (MSC)-derived exosomes in clinical trials. Stem Cell Res Ther. (2023) 14:66. doi: 10.1186/s13287-023-03287-7
57. Su L, Li R, Zhang Z, Liu J, Du J, Wei H. Identification of altered exosomal microRNAs and mRNAs in Alzheimer’s disease. Ageing Res Rev. (2022) 73:101497. doi: 10.1016/j.arr.2021.101497
58. He X, Kuang G, Wu Y, Ou C. Emerging roles of exosomal miRNAs in diabetes mellitus. Clin Transl Med. (2021) 11:e468. doi: 10.1002/ctm2.468
59. Zhang L, Yu D. Exosomes in cancer development, metastasis, and immunity. Biochim Biophys Acta Rev Cancer. (2019) 1871:455–68. doi: 10.1016/j.bbcan.2019.04.004
60. Fitts CA, Ji N, Li Y, Tan C. Exploiting exosomes in cancer liquid biopsies and drug delivery. Adv Healthc Mater. (2019) 8:e1801268. doi: 10.1002/adhm.201801268
61. Barile L, Vassalli G. Exosomes: Therapy delivery tools and biomarkers of diseases. Pharmacol Ther. (2017) 174:63–78. doi: 10.1016/j.pharmthera.2017.02.020
62. Ferguson SW, Nguyen J. Exosomes as therapeutics: The implications of molecular composition and exosomal heterogeneity. J Control Release. (2016) 228:179–90. doi: 10.1016/j.jconrel.2016.02.037
63. Zhao L, Gu C, Gan Y, Shao L, Chen H, Zhu H. Exosome-mediated siRNA delivery to suppress postoperative breast cancer metastasis. J Control Release. (2020) 318:1–15. doi: 10.1016/j.jconrel.2019.12.005
64. Morad G, Carman CV, Hagedorn EJ, Perlin JR, Zon LI, Mustafaoglu N, et al. Tumor-derived extracellular vesicles breach the intact blood-brain barrier via transcytosis. ACS Nano. (2019) 13:13853–65. doi: 10.1021/acsnano.9b04397
65. Cui J, Wang X, Li J, Zhu A, Du Y, Zeng W, et al. Immune exosomes loading self-assembled nanomicelles traverse the blood-brain barrier for chemo-immunotherapy against glioblastoma. ACS Nano. (2023). doi: 10.1021/acsnano.2c10219
66. Siegel RL, Miller KD, Jemal A. Cancer statistics, 2020. CA Cancer J Clin. (2020) 70:7–30. doi: 10.3322/caac.21590
67. Gatenby RA, Brown JS. Integrating evolutionary dynamics into cancer therapy. Nat Rev Clin Oncol. (2020) 17:675–86. doi: 10.1038/s41571-020-0411-1
68. Shahar N, Larisch S. Inhibiting the inhibitors: Targeting anti-apoptotic proteins in cancer and therapy resistance. Drug Resist Update. (2020) 52:100712. doi: 10.1016/j.drup.2020.100712
69. Lin Z, Wu Y, Xu Y, Li G, Li Z, Liu T. Mesenchymal stem cell-derived exosomes in cancer therapy resistance: recent advances and therapeutic potential. Mol Cancer. (2022) 21:179. doi: 10.1186/s12943-022-01650-5
70. Luo T, Liu Q, Tan A, Duan L, Jia Y, Nong L, et al. Mesenchymal Stem Cell-Secreted Exosome Promotes Chemoresistance in Breast Cancer via Enhancing miR-21-5p-Mediated S100A6 Expression. Mol Ther Oncolytics. (2020) 19:283–93. doi: 10.1016/j.omto.2020.10.008
71. Zhu L, Kalimuthu S, Gangadaran P, Oh JM, Lee HW, Baek SH, et al. Exosomes derived from natural killer cells exert therapeutic effect in melanoma. Theranostics. (2017) 7:2732–45. doi: 10.7150/thno.18752
72. Wang J, Long R, Han Y. The role of exosomes in the tumour microenvironment on macrophage polarisation. Biochim Biophys Acta Rev Cancer. (2022) 1877:188811. doi: 10.1016/j.bbcan.2022.188811
73. Hu Z, Chen G, Zhao Y, Gao H, Li L, Yin Y, et al. Exosome-derived circCCAR1 promotes CD8 + T-cell dysfunction and anti-PD1 resistance in hepatocellular carcinoma. Mol Cancer. (2023) 22:55. doi: 10.1186/s12943-023-01759-1
74. Zhao S, Mi Y, Guan B, Zheng B, Wei P, Gu Y, et al. Tumor-derived exosomal miR-934 induces macrophage M2 polarization to promote liver metastasis of colorectal cancer. J Hematol Oncol. (2020) 13:156. doi: 10.1186/s13045-020-00991-2
75. Liang Y, Song X, Li Y, Chen B, Zhao W, Wang L, et al. LncRNA BCRT1 promotes breast cancer progression by targeting miR-1303/PTBP3 axis. Mol Cancer. (2020) 19:85. doi: 10.1186/s12943-020-01206-5
76. Liang Y, Duan L, Lu J, Xia J. Engineering exosomes for targeted drug delivery. Theranostics. (2021) 11:3183–95. doi: 10.7150/thno.52570
77. Batrakova EV, Kim MS. Using exosomes, naturally-equipped nanocarriers, for drug delivery. J Control Release. (2015) 219:396–405. doi: 10.1016/j.jconrel.2015.07.030
78. Quah BJ, O’Neill HC. The immunogenicity of dendritic cell-derived exosomes. Blood Cells Mol Dis. (2005) 35:94–110. doi: 10.1016/j.bcmd.2005.05.002
79. Zheng M, Huang M, Ma X, Chen H, Gao X. Harnessing exosomes for the development of brain drug delivery systems. Bioconjug Chem. (2019) 30:994–1005. doi: 10.1021/acs.bioconjchem.9b00085
80. Tian T, Zhu YL, Zhou YY, Liang GF, Wang YY, Hu FH, et al. Exosome uptake through clathrin-mediated endocytosis and macropinocytosis and mediating miR-21 delivery. J Biol Chem. (2014) 289:22258–67. doi: 10.1074/jbc.M114.588046
81. Vinas JL, Spence M, Gutsol A, Knoll W, Burger D, Zimpelmann J, et al. Receptor-ligand interaction mediates targeting of endothelial colony forming cell-derived exosomes to the kidney after ischemic injury. Sci Rep. (2018) 8:16320. doi: 10.1038/s41598-018-34557-7
82. Mulcahy LA, Pink RC, Carter DR. Routes and mechanisms of extracellular vesicle uptake. J Extracell Vesicles 3. (2014). doi: 10.3402/jev.v3.24641
83. Mentkowski KI, Snitzer JD, Rusnak S, Lang JK. Therapeutic potential of engineered extracellular vesicles. AAPS J. (2018) 20:50. doi: 10.1208/s12248-018-0211-z
84. Smyth T, Petrova K, Payton NM, Persaud I, Redzic JS, Graner MW, et al. Surface functionalization of exosomes using click chemistry. Bioconjug Chem. (2014) 25:1777–84. doi: 10.1021/bc500291r
85. Armstrong JP, Holme MN, Stevens MM. Re-engineering extracellular vesicles as smart nanoscale therapeutics. ACS Nano. (2017) 11:69–83. doi: 10.1021/acsnano.6b07607
86. Tamura R, Uemoto S, Tabata Y. Augmented liver targeting of exosomes by surface modification with cationized pullulan. Acta Biomater. (2017) 57:274–84. doi: 10.1016/j.actbio.2017.05.013
87. Nakase I, Futaki S. Combined treatment with a pH-sensitive fusogenic peptide and cationic lipids achieves enhanced cytosolic delivery of exosomes. Sci Rep. (2015) 5:10112. doi: 10.1038/srep10112
88. Thery C, Amigorena S, Raposo G, Clayton A. Isolation and characterization of exosomes from cell culture supernatants and biological fluids. Curr Protoc Cell Biol Chapter. (2006) 3:3 22. doi: 10.1002/0471143030.cb0322s30
89. Fujiwara Y, Kikuchi H, Aizawa S, Furuta A, Hatanaka Y, Konya C, et al. Direct uptake and degradation of DNA by lysosomes. Autophagy. (2013) 9:1167–71. doi: 10.4161/auto.24880
90. Akasaki K, Fukuzawa M, Kinoshita H, Furuno K, Tsuji H. Cycling of two endogenous lysosomal membrane proteins, lamp-2 and acid phosphatase, between the cell surface and lysosomes in cultured rat hepatocytes. J Biochem. (1993) 114:598–604. doi: 10.1093/oxfordjournals.jbchem.a124223
91. Kim H, Yun N, Mun D, Kang JY, Lee SH, Park H, et al. Cardiac-specific delivery by cardiac tissue-targeting peptide-expressing exosomes. Biochem Biophys Res Commun. (2018) 499:803–8. doi: 10.1016/j.bbrc.2018.03.227
92. Fagiuoli S, Daina E, D’Antiga L, Colledan M, Remuzzi G. Monogenic diseases that can be cured by liver transplantation. J Hepatol. (2013) 59:595–612. doi: 10.1016/j.jhep.2013.04.004
93. Wang X, Chen T, Li C, Li W, Zhou X, Li Y, et al. CircRNA-CREIT inhibits stress granule assembly and overcomes doxorubicin resistance in TNBC by destabilizing PKR. J Hematol Oncol. (2022) 15:122. doi: 10.1186/s13045-022-01345-w
94. High KA, Roncarolo MG. Gene therapy. N Engl J Med. (2019) 381:455–64. doi: 10.1056/NEJMra1706910
95. Anguela XM, High KA. Entering the modern era of gene therapy. Annu Rev Med. (2019) 70:273–88. doi: 10.1146/annurev-med-012017-043332
96. Mendell JR, Al-Zaidy SA, Rodino-Klapac LR, Goodspeed K, Gray SJ, Kay CN, et al. Current clinical applications of in vivo gene therapy with AAVs. Mol Ther. (2021) 29:464–88. doi: 10.1016/j.ymthe.2020.12.007
97. O’Brien K, Breyne K, Ughetto S, Laurent LC, Breakefield XO. RNA delivery by extracellular vesicles in mammalian cells and its applications. Nat Rev Mol Cell Biol. (2020) 21:585–606. doi: 10.1038/s41580-020-0251-y
98. Liu B, Li Z, Huang S, Yan B, He S, Chen F, et al. AAV-containing exosomes as a novel vector for improved gene delivery to lung cancer cells. Front Cell Dev Biol. (2021) 9:707607. doi: 10.3389/fcell.2021.707607
99. Joorabloo A, Liu T. Engineering exosome-based biomimetic nanovehicles for wound healing. J Control Release. (2023) 356:463–80. doi: 10.1016/j.jconrel.2023.03.013
100. Liang Y, Xu X, Li X, Xiong J, Li B, Duan L, et al. Chondrocyte-targeted microRNA delivery by engineered exosomes toward a cell-free osteoarthritis therapy. ACS Appl Mater Interfaces. (2020) 12:36938–47. doi: 10.1021/acsami.0c10458
101. Yao X, Lyu P, Yoo K, Yadav MK, Singh R, Atala A, et al. Engineered extracellular vesicles as versatile ribonucleoprotein delivery vehicles for efficient and safe CRISPR genome editing. J Extracell Vesicles. (2021) 10:e12076. doi: 10.1002/jev2.12076
102. Osteikoetxea X, Silva A, Lazaro-Ibanez E, Salmond N, Shatnyeva O, Stein J, et al. Engineered Cas9 extracellular vesicles as a novel gene editing tool. J Extracell Vesicles. (2022) 11:e12225. doi: 10.1002/jev2.12225
103. Lin Y, Wu J, Gu W, Huang Y, Tong Z, Huang L, et al. Exosome-liposome hybrid nanoparticles deliver CRISPR/cas9 system in MSCs. Adv Sci (Weinh). (2018) 5:1700611. doi: 10.1002/advs.201700611
104. Evers MJW, van de Wakker SI, de Groot EM, de Jong OG, Gitz-Francois JJJ, Seinen CS, et al. Functional siRNA delivery by extracellular vesicle-liposome hybrid nanoparticles. Adv Healthc Mater. (2022) 11:e2101202. doi: 10.1002/adhm.202101202
105. Tenchov R, Sasso JM, Wang X, Liaw WS, Chen CA, Zhou QA. Exosomes horizontal line Nature’s Lipid Nanoparticles, a Rising Star in Drug Delivery and Diagnostics. ACS Nano. (2022) 16:17802–46. doi: 10.1021/acsnano.2c08774
106. Tenchov R, Bird R, Curtze AE, Zhou Q. Lipid Nanoparticles horizontal line From Liposomes to mRNA Vaccine Delivery, a Landscape of Research Diversity and Advancement. ACS Nano. (2021) 15:16982–7015. doi: 10.1021/acsnano.1c04996
107. Koynova R, Tenchov B, MacDonald RC. Nonlamellar phases in cationic phospholipids, relevance to drug and gene delivery. ACS Biomater Sci Eng. (2015) 1:130–8. doi: 10.1021/ab500142w
108. Dougherty JA, Kumar N, Noor M, Angelos MG, Khan M, Chen CA, et al. Extracellular vesicles released by human induced-pluripotent stem cell-derived cardiomyocytes promote angiogenesis. Front Physiol. (2018) 9:1794. doi: 10.3389/fphys.2018.01794
109. Bunggulawa EJ, Wang W, Yin T, Wang N, Durkan C, Wang Y, et al. Recent advancements in the use of exosomes as drug delivery systems. J Nanobiotechnol. (2018) 16:81. doi: 10.1186/s12951-018-0403-9
110. Haney MJ, Zhao Y, Jin YS, Li SM, Bago JR, Klyachko NL, et al. Macrophage-derived extracellular vesicles as drug delivery systems for triple negative breast cancer (TNBC) therapy. J Neuroimmune Pharmacol. (2020) 15:487–500. doi: 10.1007/s11481-019-09884-9
111. Kim MS, Haney MJ, Zhao Y, Mahajan V, Deygen I, Klyachko NL, et al. Development of exosome-encapsulated paclitaxel to overcome MDR in cancer cells. Nanomedicine. (2016) 12:655–64. doi: 10.1016/j.nano.2015.10.012
112. Nie W, Wu G, Zhang J, Huang LL, Ding J, Jiang A, et al. Responsive exosome nano-bioconjugates for synergistic cancer therapy. Angew Chem Int Ed Engl. (2020) 59:2018–22. doi: 10.1002/anie.201912524
113. Wang X, Ding H, Li Z, Peng Y, Tan H, Wang C, et al. Exploration and functionalization of M1-macrophage extracellular vesicles for effective accumulation in glioblastoma and strong synergistic therapeutic effects. Signal Transduct Target Ther. (2022) 7:74. doi: 10.1038/s41392-022-00894-3
114. Wang D, Wan Z, Yang Q, Chen J, Liu Y, Lu F, et al. Sonodynamical reversion of immunosuppressive microenvironment in prostate cancer via engineered exosomes. Drug Delivery. (2022) 29:702–13. doi: 10.1080/10717544.2022.2044937
115. Kamerkar S, Leng C, Burenkova O, Jang SC, McCoy C, Zhang K, et al. Exosome-mediated genetic reprogramming of tumor-associated macrophages by exoASO-STAT6 leads to potent monotherapy antitumor activity. Sci Adv. (2022) 8:eabj7002. doi: 10.1126/sciadv.abj7002
116. Hu S, Ma J, Su C, Chen Y, Shu Y, Qi Z, et al. Engineered exosome-like nanovesicles suppress tumor growth by reprogramming tumor microenvironment and promoting tumor ferroptosis. Acta Biomater. (2021) 135:567–81. doi: 10.1016/j.actbio.2021.09.003
117. Zhu L, Sun HT, Wang S, Huang SL, Zheng Y, Wang CQ, et al. Isolation and characterization of exosomes for cancer research. J Hematol Oncol. (2020) 13:152. doi: 10.1186/s13045-020-00987-y
118. Li C, Teixeira AF, Zhu HJ, Ten Dijke P. Cancer associated-fibroblast-derived exosomes in cancer progression. Mol Cancer. (2021) 20:154. doi: 10.1186/s12943-021-01463-y
119. Li C, Ni YQ, Xu H, Xiang QY, Zhao Y, Zhan JK, et al. Roles and mechanisms of exosomal non-coding RNAs in human health and diseases. Signal Transduct Target Ther. (2021) 6:383. doi: 10.1038/s41392-021-00779-x
120. Thomou T, Mori MA, Dreyfuss JM, Konishi M, Sakaguchi M, Wolfrum C, et al. Adipose-derived circulating miRNAs regulate gene expression in other tissues. Nature. (2017) 542:450–5. doi: 10.1038/nature21365
121. Garcia-Martin R, Wang G, Brandao BB, Zanotto TM, Shah S, Kumar Patel S, et al. MicroRNA sequence codes for small extracellular vesicle release and cellular retention. Nature. (2022) 601:446–51. doi: 10.1038/s41586-021-04234-3
122. Huang X, Wu W, Jing D, Yang L, Guo H, Wang L, et al. Engineered exosome as targeted lncRNA MEG3 delivery vehicles for osteosarcoma therapy. J Control Release. (2022) 343:107–17. doi: 10.1016/j.jconrel.2022.01.026
123. Nguyen Cao TG, Kang JH, Kim W, Lim J, Kang SJ, You JY, et al. Engineered extracellular vesicle-based sonotheranostics for dual stimuli-sensitive drug release and photoacoustic imaging-guided chemo-sonodynamic cancer therapy. Theranostics. (2022) 12:1247–66. doi: 10.7150/thno.65516
124. Zhang J, Ji C, Zhang H, Shi H, Mao F, Qian H, et al. Engineered neutrophil-derived exosome-like vesicles for targeted cancer therapy. Sci Adv. (2022) 8:eabj8207. doi: 10.1126/sciadv.abj8207
125. Watanabe Y, Tsuchiya A, Terai S. The development of mesenchymal stem cell therapy in the present, and the perspective of cell-free therapy in the future. Clin Mol Hepatol. (2021) 27:70–80. doi: 10.3350/cmh.2020.0194
126. Bei HP, Hung PM, Yeung HL, Wang S, Zhao X. Bone-a-petite: engineering exosomes towards bone, osteochondral, and cartilage repair. Small. (2021) 17:e2101741. doi: 10.1002/smll.202101741
127. Zhang Y, Zhang P, Gao X, Chang L, Chen Z, Mei X. Preparation of exosomes encapsulated nanohydrogel for accelerating wound healing of diabetic rats by promoting angiogenesis. Mater Sci Eng C Mater Biol Appl. (2021) 120:111671. doi: 10.1016/j.msec.2020.111671
128. He N, Zhang Y, Zhang S, Wang D, Ye H. Exosomes: cell-free therapy for cardiovascular diseases. J Cardiovasc Transl Res. (2020) 13:713–21. doi: 10.1007/s12265-020-09966-7
129. Loeser RF, Collins JA, Diekman BO. Ageing and the pathogenesis of osteoarthritis. Nat Rev Rheumatol. (2016) 12:412–20. doi: 10.1038/nrrheum.2016.65
130. Tian T, Zhang HX, He CP, Fan S, Zhu YL, Qi C, et al. Surface functionalized exosomes as targeted drug delivery vehicles for cerebral ischemia therapy. Biomaterials. (2018) 150:137–49. doi: 10.1016/j.biomaterials.2017.10.012
131. Alvarez-Erviti L, Seow Y, Yin H, Betts C, Lakhal S, Wood MJ. Delivery of siRNA to the mouse brain by systemic injection of targeted exosomes. Nat Biotechnol. (2011) 29:341–5. doi: 10.1038/nbt.1807
132. Kim MS, Haney MJ, Zhao Y, Yuan D, Deygen I, Klyachko NL, et al. Engineering macrophage-derived exosomes for targeted paclitaxel delivery to pulmonary metastases: in vitro and in vivo evaluations. Nanomedicine. (2018) 14:195–204. doi: 10.1016/j.nano.2017.09.011
133. Kooijmans SAA, Fliervoet LAL, van der Meel R, Fens M, Heijnen HFG, van Bergen En Henegouwen PMP, et al. PEGylated and targeted extracellular vesicles display enhanced cell specificity and circulation time. J Control Release. (2016) 224:77–85. doi: 10.1016/j.jconrel.2016.01.009
134. Ma X, Yao M, Gao Y, Yue Y, Li Y, Zhang T, et al. Functional immune cell-derived exosomes engineered for the trilogy of radiotherapy sensitization. Adv Sci (Weinh). (2022) 9:e2106031. doi: 10.1002/advs.202106031
135. Bose RJ, Kumar US, Garcia-Marques F, Zeng Y, Habte F, McCarthy JR, et al. Engineered cell-derived vesicles displaying targeting peptide and functionalized with nanocarriers for therapeutic microRNA delivery to triple-negative breast cancer in mice. Adv Healthc Mater. (2022) 11:e2101387. doi: 10.1002/adhm.202101387
136. Wan T, Zhong J, Pan Q, Zhou T, Ping Y, Liu X. Exosome-mediated delivery of Cas9 ribonucleoprotein complexes for tissue-specific gene therapy of liver diseases. Sci Adv. (2022) 8:eabp9435. doi: 10.1126/sciadv.abp9435
137. Huang H, Shao L, Chen Y, Tang L, Liu T, Li J, et al. Synergistic strategy with hyperthermia therapy based immunotherapy and engineered exosomes-liposomes targeted chemotherapy prevents tumor recurrence and metastasis in advanced breast cancer. Bioeng Transl Med. (2022) 7:e10284. doi: 10.1002/btm2.10284
138. Bose RJC, Uday Kumar S, Zeng Y, Afjei R, Robinson E, Lau K, et al. Tumor cell-derived extracellular vesicle-coated nanocarriers: an efficient theranostic platform for the cancer-specific delivery of anti-miR-21 and imaging agents. ACS Nano. (2018) 12:10817–32. doi: 10.1021/acsnano.8b02587
139. Tian R, Wang Z, Niu R, Wang H, Guan W, Chang J. Tumor exosome mimicking nanoparticles for tumor combinatorial chemo-photothermal therapy. Front Bioeng Biotechnol. (2020) 8:1010. doi: 10.3389/fbioe.2020.01010
140. Nguyen Cao TG, Kang JH, You JY, Kang HC, Rhee WJ, Ko YT, et al. Safe and targeted sonodynamic cancer therapy using biocompatible exosome-based nanosonosensitizers. ACS Appl Mater Interfaces. (2021) 13:25575–88. doi: 10.1021/acsami.0c22883
141. Limoni SK, Moghadam MF, Moazzeni SM, Gomari H, Salimi F. Engineered exosomes for targeted transfer of siRNA to HER2 positive breast cancer cells. Appl Biochem Biotechnol. (2019) 187:352–64. doi: 10.1007/s12010-018-2813-4
142. O’Brien KP, Khan S, Gilligan KE, Zafar H, Lalor P, Glynn C, et al. Employing mesenchymal stem cells to support tumor-targeted delivery of extracellular vesicle (EV)-encapsulated microRNA-379. Oncogene. (2018) 37:2137–49. doi: 10.1038/s41388-017-0116-9
143. Wang L, Zhou X, Zou W, Wu Y, Zhao J, Chen X, et al. Exosomes containing miRNAs targeting HER2 synthesis and engineered to adhere to HER2 on tumor cells surface exhibit enhanced antitumor activity. J Nanobiotechnol. (2020) 18:153. doi: 10.1186/s12951-020-00711-5
144. Cheng Q, Dai Z, Smbatyan G, Epstein AL, Lenz HJ, Zhang Y. Eliciting anti-cancer immunity by genetically engineered multifunctional exosomes. Mol Ther. (2022) 30:3066–77. doi: 10.1016/j.ymthe.2022.06.013
145. Di Bonito P, Ridolfi B, Columba-Cabezas S, Giovannelli A, Chiozzini C, Manfredi F, et al. HPV-E7 delivered by engineered exosomes elicits a protective CD8(+) T cell-mediated immune response. Viruses. (2015) 7:1079–99. doi: 10.3390/v7031079
146. Huang L, Rong Y, Tang X, Yi K, Qi P, Hou J, et al. Engineered exosomes as an in situ DC-primed vaccine to boost antitumor immunity in breast cancer. Mol Cancer. (2022) 21:45. doi: 10.1186/s12943-022-01515-x
147. Shi X, Cheng Q, Hou T, Han M, Smbatyan G, Lang JE, et al. Genetically engineered cell-derived nanoparticles for targeted breast cancer immunotherapy. Mol Ther. (2020) 28:536–47. doi: 10.1016/j.ymthe.2019.11.020
148. Liang G, Zhu Y, Ali DJ, Tian T, Xu H, Si K, et al. Engineered exosomes for targeted co-delivery of miR-21 inhibitor and chemotherapeutics to reverse drug resistance in colon cancer. J Nanobiotechnol. (2020) 18:10. doi: 10.1186/s12951-019-0563-2
149. Hui B, Lu C, Wang J, Xu Y, Yang Y, Ji H, et al. Engineered exosomes for co-delivery of PGM5-AS1 and oxaliplatin to reverse drug resistance in colon cancer. J Cell Physiol. (2022) 237:911–33. doi: 10.1002/jcp.30566
150. Kim S, Sohn HJ, Lee HJ, Sohn DH, Hyun SJ, Cho HI, et al. Use of engineered exosomes expressing HLA and costimulatory molecules to generate antigen-specific CD8+ T cells for adoptive cell therapy. J Immunother. (2017) 40:83–93. doi: 10.1097/CJI.0000000000000151
151. Wu T, Liu Y, Cao Y, Liu Z. Engineering macrophage exosome disguised biodegradable nanoplatform for enhanced sonodynamic therapy of glioblastoma. Adv Mater. (2022) 34:e2110364. doi: 10.1002/adma.202110364
152. Tian T, Liang R, Erel-Akbaba G, Saad L, Obeid PJ, Gao J, et al. Immune checkpoint inhibition in GBM primed with radiation by engineered extracellular vesicles. ACS Nano. (2022) 16:1940–53. doi: 10.1021/acsnano.1c05505
153. Zhang Z, Guo X, Guo X, Yu R, Qian M, Wang S, et al. MicroRNA-29a-3p delivery via exosomes derived from engineered human mesenchymal stem cells exerts tumour suppressive effects by inhibiting migration and vasculogenic mimicry in glioma. Aging (Albany NY). (2021) 13:5055–68. doi: 10.18632/aging.v13i4
154. Guo Z, Zhang Y, Xu W, Zhang X, Jiang J. Engineered exosome-mediated delivery of circDIDO1 inhibits gastric cancer progression via regulation of MiR-1307-3p/SOCS2 Axis. J Transl Med. (2022) 20:326. doi: 10.1186/s12967-022-03527-z
155. Lee JH, Jung KH, Mina K, Lee KH. Extracellular vesicles deliver sodium iodide symporter protein and promote cancer cell radioiodine therapy. Sci Rep. (2022) 12:11190. doi: 10.1038/s41598-022-15524-9
156. Liang G, Kan S, Zhu Y, Feng S, Feng W, Gao S. Engineered exosome-mediated delivery of functionally active miR-26a and its enhanced suppression effect in HepG2 cells. Int J Nanomed. (2018) 13:585–99. doi: 10.2147/IJN
157. He C, Jaffar Ali D, Li Y, Zhu Y, Sun B, Xiao Z. Engineering of HN3 increases the tumor targeting specificity of exosomes and upgrade the anti-tumor effect of sorafenib on HuH-7 cells. PeerJ. (2020) 8:e9524. doi: 10.7717/peerj.9524
158. Baldari S, Di Rocco G, Magenta A, Picozza M, Toietta G. Extracellular vesicles-encapsulated microRNA-125b produced in genetically modified mesenchymal stromal cells inhibits hepatocellular carcinoma cell proliferation. Cells. (2019) 8(12):1560. doi: 10.3390/cells8121560
159. Ishiguro K, Yan IK, Lewis-Tuffin L, Patel T. Targeting liver cancer stem cells using engineered biological nanoparticles for the treatment of hepatocellular cancer. Hepatol Commun. (2020) 4:298–313. doi: 10.1002/hep4.1462
160. Ye Y, Zhang X, Xie F, Xu B, Xie P, Yang T, et al. An engineered exosome for delivering sgRNA : Cas9 ribonucleoprotein complex and genome editing in recipient cells. Biomater Sci. (2020) 8:2966–76. doi: 10.1039/D0BM00427H
161. Morishita M, Takahashi Y, Matsumoto A, Nishikawa M, Takakura Y. Exosome-based tumor antigens-adjuvant co-delivery utilizing genetically engineered tumor cell-derived exosomes with immunostimulatory CpG DNA. Biomaterials. (2016) 111:55–65. doi: 10.1016/j.biomaterials.2016.09.031
162. Fan M, Liu H, Yan H, Che R, Jin Y, Yang X, et al. A CAR T-inspiring platform based on antibody-engineered exosomes from antigen-feeding dendritic cells for precise solid tumor therapy. Biomaterials. (2022) 282:121424. doi: 10.1016/j.biomaterials.2022.121424
163. Xie Y, Bai O, Zhang H, Yuan J, Zong S, Chibbar R, et al. Membrane-bound HSP70-engineered myeloma cell-derived exosomes stimulate more efficient CD8(+) CTL- and NK-mediated antitumour immunity than exosomes released from heat-shocked tumour cells expressing cytoplasmic HSP70. J Cell Mol Med. (2010) 14:2655–66. doi: 10.1111/j.1582-4934.2009.00851.x
164. Wang Y, Guo M, Lin D, Liang D, Zhao L, Zhao R, et al. Docetaxel-loaded exosomes for targeting non-small cell lung cancer: preparation and evaluation in vitro and in vivo. Drug Delivery. (2021) 28:1510–23. doi: 10.1080/10717544.2021.1951894
165. Xue Q, Yang Y, Yang L, Yan X, Shen Z, Liu J, et al. miR-371b-5p-engineered exosomes enhances tumor inhibitory effect. Front Cell Dev Biol. (2021) 9:750171. doi: 10.3389/fcell.2021.750171
166. Zhang K, Dong C, Chen M, Yang T, Wang X, Gao Y, et al. Extracellular vesicle-mediated delivery of miR-101 inhibits lung metastasis in osteosarcoma. Theranostics. (2020) 10:411–25. doi: 10.7150/thno.33482
167. Cheng L, Zhang X, Tang J, Lv Q, Liu J. Gene-engineered exosomes-thermosensitive liposomes hybrid nanovesicles by the blockade of CD47 signal for combined photothermal therapy and cancer immunotherapy. Biomaterials. (2021) 275:120964. doi: 10.1016/j.biomaterials.2021.120964
168. Severic M, Ma G, Pereira SGT, Ruiz A, Cheung CCL, Al-Jamal WT. Genetically-engineered anti-PSMA exosome mimetics targeting advanced prostate cancer in vitro and in vivo. J Control Release. (2021) 330:101–10. doi: 10.1016/j.jconrel.2020.12.017
169. Zhang M, Hu S, Liu L, Dang P, Liu Y, Sun Z, et al. Engineered exosomes from different sources for cancer-targeted therapy. Signal Transduct Target Ther. (2023) 8:124. doi: 10.1038/s41392-023-01382-y
170. Finck AV, Blanchard T, Roselle CP, Golinelli G, June CH. Engineered cellular immunotherapies in cancer and beyond. Nat Med. (2022) 28:678–89. doi: 10.1038/s41591-022-01765-8
171. Cannac M, Nikolic J, Benaroch P. Cancer immunotherapies based on genetically engineered macrophages. Cancer Immunol Res. (2022) 10:1156–66. doi: 10.1158/2326-6066.CIR-22-0030
172. Chen Y, Yu Z, Tan X, Jiang H, Xu Z, Fang Y, et al. CAR-macrophage: A new immunotherapy candidate against solid tumors. BioMed Pharmacother. (2021) 139:111605. doi: 10.1016/j.biopha.2021.111605
173. Xu Z, Zeng S, Gong Z, Yan Y. Exosome-based immunotherapy: a promising approach for cancer treatment. Mol Cancer. (2020) 19:160. doi: 10.1186/s12943-020-01278-3
174. Zhou Q, Wei S, Wang H, Li Y, Fan S, Cao Y, et al. T cell-derived exosomes in tumor immune modulation and immunotherapy. Front Immunol. (2023) 14:1130033. doi: 10.3389/fimmu.2023.1130033
175. van der Leun AM, Thommen DS, Schumacher TN. CD8(+) T cell states in human cancer: insights from single-cell analysis. Nat Rev Cancer. (2020) 20:218–32. doi: 10.1038/s41568-019-0235-4
176. Maalej KM, Merhi M, Inchakalody VP, Mestiri S, Alam M, Maccalli C, et al. CAR-cell therapy in the era of solid tumor treatment: current challenges and emerging therapeutic advances. Mol Cancer. (2023) 22:20. doi: 10.1186/s12943-023-01723-z
177. Zhu T, Chen Z, Jiang G, Huang X. Sequential targeting hybrid nanovesicles composed of chimeric antigen receptor T-cell-derived exosomes and liposomes for enhanced cancer immunochemotherapy. ACS Nano. (2023) 17:16770–86. doi: 10.1021/acsnano.3c03456
178. Skurikhin E, Pershina O, Zhukova M, Widera D, Ermakova N, Pan E, et al. Potential of stem cells and CART as a potential polytherapy for small cell lung cancer. Front Cell Dev Biol. (2021) 9:778020. doi: 10.3389/fcell.2021.778020
179. Hong M, Clubb JD, Chen YY. Engineering CAR-T cells for next-generation cancer therapy. Cancer Cell. (2020) 38:473–88. doi: 10.1016/j.ccell.2020.07.005
180. Haque S, Vaiselbuh SR. CD19 chimeric antigen receptor-exosome targets CD19 positive B-lineage acute lymphocytic leukemia and induces cytotoxicity. Cancers (Basel). (2021) 13(6):1401. doi: 10.3390/cancers13061401
181. Shimasaki N, Jain A, Campana D. NK cells for cancer immunotherapy. Nat Rev Drug Discovery. (2020) 19:200–18. doi: 10.1038/s41573-019-0052-1
182. Myers JA, Miller JS. Exploring the NK cell platform for cancer immunotherapy. Nat Rev Clin Oncol. (2021) 18:85–100. doi: 10.1038/s41571-020-0426-7
183. Liu J, Ren L, Li S, Li W, Zheng X, Yang Y, et al. The biology, function, and applications of exosomes in cancer. Acta Pharm Sin B. (2021) 11:2783–97. doi: 10.1016/j.apsb.2021.01.001
184. Cao B, Liu M, Huang J, Zhou J, Li J, Lian H, et al. Development of mesothelin-specific CAR NK-92 cells for the treatment of gastric cancer. Int J Biol Sci. (2021) 17:3850–61. doi: 10.7150/ijbs.64630
185. Motais B, Charvatova S, Walek Z, Hajek R, Bago JR. NK92 expressing anti-BCMA CAR and secreted TRAIL for the treatment of multiple myeloma: preliminary in vitro assessment. Cells. (2023) 12(23):2748. doi: 10.3390/cells12232748
186. Pitt JM, Andre F, Amigorena S, Soria JC, Eggermont A, Kroemer G, et al. Dendritic cell-derived exosomes for cancer therapy. J Clin Invest. (2016) 126:1224–32. doi: 10.1172/JCI81137
187. Wynn TA, Chawla A, Pollard JW. Macrophage biology in development, homeostasis and disease. Nature. (2013) 496:445–55. doi: 10.1038/nature12034
188. Locati M, Curtale G, Mantovani A. Diversity, mechanisms, and significance of macrophage plasticity. Annu Rev Pathol. (2020) 15:123–47. doi: 10.1146/annurev-pathmechdis-012418-012718
Keywords: exosome, cell-free therapy, engineered exosomes, modification, drug development
Citation: Si C, Gao J and Ma X (2024) Engineered exosomes in emerging cell-free therapy. Front. Oncol. 14:1382398. doi: 10.3389/fonc.2024.1382398
Received: 05 February 2024; Accepted: 14 March 2024;
Published: 26 March 2024.
Edited by:
Rabeea Siddique, Second Affiliated Hospital of Zhengzhou University, ChinaReviewed by:
Celio Geraldo Freire-de-Lima, Federal University of Rio de Janeiro, BrazilDilpreet Singh, Chandigarh University, India
Copyright © 2024 Si, Gao and Ma. This is an open-access article distributed under the terms of the Creative Commons Attribution License (CC BY). The use, distribution or reproduction in other forums is permitted, provided the original author(s) and the copyright owner(s) are credited and that the original publication in this journal is cited, in accordance with accepted academic practice. No use, distribution or reproduction is permitted which does not comply with these terms.
*Correspondence: Xu Ma, maxu@nrifp.org.cn; Jianen Gao, gaojianen@nrifp.org.cn