- 1Department of Microbiology, Immunology & Tropical Medicine, and Research Center for Neglected Diseases of Poverty, School of Medicine and Health Sciences, George Washington University, Washington, DC, United States
- 2Department of Internal Medicine, Washington University of St. Louis School of Medicine, St. Louis, MO, United States
- 3Charles River Analytics, Inc., Cambridge, MA, United States
- 4Centre for Molecular Therapeutics, Australian Institute of Tropical Health and Medicine, James Cook University, Cairns, QLD, Australia
- 5Parasitology Reference and Research Laboratory, Centro Nacional de Microbiología, Instituto de Salud Carlos III, Madrid, Spain
Intricate molecular communication between schistosome flatworms and their mammalian host, as well as between paired male and female schistosomes has shaped the secreted proteome of these flatworms. Whereas the schistosome egg is responsible for the disease manifestations of chronic schistosomiasis, the long lived, adult female and male stages also release different mediators including glycans, lipids, proteins and small molecules, known as excretory/secretory products (ESPs), that facilitate their survival. Given their importance, deeper analysis focused on analyzing the ESPs from adult schistosomes would likely be informative, beyond current understanding of the complement of ESP proteins. Here, taking advantage of highly accurate and sensitive mass spectrometers, the excretory/secretory proteome from cultured Schistosoma mansoni male or female adult worms was identified, quantified, compared and contrasted using a label-free proteomic approach. Approximately 1,000 proteins were identified, from which almost 800 could be quantified. Considering the proteins uniquely identified and proteins with a significantly regulated expression pattern in male or female flukes, a total of 370 and 140 proteins were uniquely or more abundantly secreted by males and females, respectively. Using functional analysis networks showing the gene ontology terms and KEGG pathways with the highest significance, we observed that male schistosomes secrete proteins related to carbohydrate metabolism and cytoskeletal organization more abundantly than females, while female worms secreted more hydrolases and proteins involved in cellular homeostasis than males. This analysis doubles the number of reported excreted/secreted proteins from S. mansoni, contributing to deeper understanding of the host-parasite interaction and parasitism. Furthermore, these findings expand potential vaccine and diagnostic candidates for this neglected tropical disease pathogen, and thereby also provide leads for novel intervention to control this disease and its transmission.
Introduction
Schistosomiasis is a major neglected tropical disease and is considered the most important helminthiasis in terms of morbidity and mortality. More than 200 million people are infected worldwide with 700 million at risk of infection. This remains a major public health problem, particularly in sub-Saharan Africa (Colley et al., 2014; Mcmanus et al., 2018). Human schistosomiasis is caused by six species of blood flukes: Schistosoma guineensis, S. haematobium, S. intercalatum, S. japonicum, S. mansoni, and S. mekongi. Nowadays, the predominant human species are S. haematobium and S. mansoni, given the reduction of infection in recent decades caused by S. japonicum in the Yangtze River basin provinces of China (Wang et al., 2021). Urogenital schistosomiasis caused by hybrids of S. haematobium and S. bovis and relatives is spreading in West Africa (Webster et al., 2006; Huyse et al., 2009) and in Corsica (Boissier et al., 2016; Rothe et al., 2021).
Male and female schistosomes dwell in copula within the mesenteric veins (S. mansoni, S. japonicum) or the vesical venous plexus (S. haematobium) of the human, laying hundreds to thousands (depending on the species) of fertilized eggs each day. The eggs traverse the intestinal wall (e.g., S. mansoni) or the bladder wall (S. haematobium) and exit the host to the external environment in feces or urine, respectively. However, many eggs fail to exit the infected person and are retained in host tissues where they induce inflammation, granuloma, and fibrosis (Mcmanus et al., 2018). In the external environment, the eggs hatch when they reach freshwater, each releasing a miracidium, which undergoes cycles of asexual reproduction within the intermediate host (a gastropod snail) shedding thousands of cercariae into the water. After penetrating the skin of the definitive host, the cercaria sheds its tail and the juvenile larva, the schistosomulum, migrates within the circulatory system, reaching the lungs, the liver, and eventually the portal venous system or the venous system that drains the pelvic organs where the fully mature flukes pair and the female produces eggs, completing the developmental cycle.
Whereas the schistosome egg is responsible for the disease manifestations of chronic schistosomiasis, as well as orchestrating the hallmark immunological transition from a Th1 to Th2 response (Pearce and Macdonald, 2002; Schwartz and Fallon, 2018; Acharya et al., 2021), the long lived, adult female and male stages also release mediators that facilitate their intra-vascular existence in a hostile niche where they are bathed in immune cells and effector molecules. These mediators, also known as excretory/secretory products (ESPs), are secreted (or released) from the esophageal gland, the gut epithelium and from the tegument of schistosomes, making it a highly diverse mixture of molecules. The protein composition of the ESPs from several developmental stages and species of schistosomes has been described in depth (Liu et al., 2009; Mathieson and Wilson, 2010; Hall et al., 2011; Dvorak et al., 2016; Sotillo et al., 2016, 2019; Floudas et al., 2017; De Marco Verissimo et al., 2019; Kifle et al., 2020; Neves et al., 2020; Chen et al., 2022), although the diversity, role, and packaging of these secreted and excreted antigens, including as cargo within extracellular vesicles, may not yet be fully characterized or deciphered (Acharya et al., 2021). An early study of adult stage S. japonicum ESPs showed the presence of conserved proteins such as metabolic enzymes, heat shock proteins (HSPs), detoxification proteins, and peptidases (Liu et al., 2009). Other studies focusing on the schistosome “vomitus” and other secreted proteins highlighted the presence of different saposins, ferritins and cathepsins, among other molecules (Hall et al., 2011). Furthermore, tetraspanins, annexins, calpain, transporters, and cytoskeletal proteins have been identified on the tegument of S. mansoni adult worms (Braschi and Wilson, 2006; Braschi et al., 2006a,b).
Here, the ESPs in cultured supernatants from adult male and adult female S. mansoni were isolated and compared using a label-free proteomic approach for the first time, increasing the coverage of the published secretome. About 1,000 proteins were identified, from which ~ 800 could be finally quantified. In sum, this new analysis at least doubles the number of proteins known in these extracts (Hall et al., 2011; Wilson, 2012), substantially expanding the catalog of the protein complement of the ESPs from S. mansoni, which provides new insights of the host-parasite interplay. In turn, we augment the number of potential vaccine and diagnostic candidates listed previously for this neglected tropical disease agent.
Materials and Methods
Ethics
Mice experimentally infected with S. mansoni, obtained from the Schistosomiasis Resource Center (SRC) at the Biomedical Research Institute (BRI), MD were housed at the Animal Research Facility of the George Washington University (GWU), which is accredited by the American Association for Accreditation of Laboratory Animal Care (AAALAC no. 000347) and has an Animal Welfare Assurance on file with the National Institutes of Health, Office of Laboratory Animal Welfare, OLAW assurance number A3205-01. All procedures employed were consistent with the Guide for the Care and Use of Laboratory Animals. The Institutional Animal Care and Use Committee (IACUC) at GWU approved the protocol used for maintenance of mice and recovery of schistosomes.
Schistosomes
Swiss-Webster albino mice were euthanized seven weeks after infection with S. mansoni, livers were removed at necropsy, schistosome eggs recovered from the livers, and adult worms from the portal circulation as described (Dalton et al., 1997).
Isolation of Adult Excretory/Secretory Products
For the collection of excretory-secretory products (ESPs), adult S. mansoni were provided by Schistosomiasis Resource Center (SRC) of the Biomedical Research Institute (BRI), Rockville, MD. The worms were sorted with forceps to separate males (group 1) and females (group 2) or left unpaired (group 3), rinsed briefly in 1 × phosphate buffered saline (PBS) (Corning), each group was then separated into three different batches, and subsequently transferred to tissue culture plates (Sarstedt) and incubated at a density of 13 worms/ml in serum-free Dulbecco's Modification of Eagle's Medium (DMEM) (Corning) supplemented with 2% Antibiotic-Antimycotic (Gibco). Secretion of ESP into the serum-free medium was facilitated by continuous incubation at 37°C, 5% CO2 in air (Neves et al., 2020). A total of 20 mL of culture supernatant was removed with minimal disturbance to the schistosomes at 4, 24, 48, 72 h, 5 and 7 days of culture, centrifuged at 400 g and 2,000 g and stored at −80°C. The drawn medium was retained for storage and was replaced with fresh medium to the culture at each time point. At the conclusion of the collection period, the ESP-containing media were thawed gradually on wet ice, after which ESP was concentrated using Centricon Plus-70 Centrifugal Filter Units (Millipore) featuring a 3 kDa nominal molecular size limit. Concentration by centrifugation on the 3 kDa membrane was undertaken at 3,220 g at 4°C using an Eppendorf 5810R centrifuge fitted with an A-4-62 swinging bucket rotor. Concentrated ESP was resuspended and reconcentrated twice using volumes of chilled PBS equivalent to the starting volume of the sample. Protein concentration was ascertained by the Pierce BCA Protein Assay Kit (Thermo Fisher) method, and concentrated ESP was aliquoted and stored at −80°C.
Mass Spectrometry
For the mass spectrometry analysis, the ESPs from all time points were combined for each sample (male, female or mixed sexes) and analyzed as follows. Three biological replicates of ES from males, females and mixed samples were individually processed as follows. Samples were freeze-dried and dissolved with 22 mL of 50 mM ammonium bicarbonate (ABC). Two (2) mL was used to quantify the protein concentration with Qubit (Invitrogen) reagent according to the manufacturer's instructions. Ten (10) mg of protein was taken and volumes set to 22.5 mL of 50 mM ABC. Reduction and alkylation were performed by incubating samples at 60°C for 20 min with 2 mM dithiothreitol followed by a 30 min incubation at RT in the dark with 5.5 mM 2-iodoacetamide. Thereafter, samples were in-solution digested with 400 ng trypsin overnight at 37°C and acidified with 10% TFA to a final concentration of 1%. Last, digested peptides were concentrated by speed vacuum to 15 μL.
Five (5) μl of peptide mixtures were loaded onto a trap column (3 μm C18-CL, 350 μm ×0.5 mm; Eksigent Technologies, Redwood City, CA) and desalted with 0.1% TFA at 5 μl/min during 5 min. The peptides were then loaded onto an analytical column (3 μm C18-CL 120 Ă, 0.075 ×150 mm; Eksigent) equilibrated in 5% acetonitrile 0.1% FA. Elution was accomplished with a linear gradient of 15–40% B in A for 60 min (A: 0.1% FA; B: ACN, 0.1% FA) at a flow rate of 300 nL/min. Peptides were analyzed in a mass spectrometer nanoESI qQTOF (6,600+ TripleTOF, ABSCIEX). Sample was ionized in a Source Type: Optiflow <1 μL Nano applying 3.0 kV to the spray emitter at 175°C. Analysis was carried out in a data-dependent mode. Survey MS1 scans were acquired from 350–1,400 m/z for 250 ms. The quadrupole resolution was set to “LOW” for MS2 experiments, which were acquired 100–1,500 m/z for 25 ms in “high sensitivity” mode. The following switch criteria were used: charge: 2+ to 4+; minimum intensity; 250 counts per second (cps). Up to 100 ions were selected for fragmentation after each survey scan. Dynamic exclusion was set to 15 s.
Database Search and Protein Quantification
Database searches were performed using FragPipe (v16.0) with MSFragger (v3.3) (Kong et al., 2017) and Philosopher (v4.0) (Da Veiga Leprevost et al., 2020) against a concatenated target/decoy database consisting of the S. mansoni proteome (UP000008854) and common contaminants from Uniprot (downloaded 30 June 2021; 14,615 proteins). For the MSFragger analysis, precursor and fragment mass tolerance were both set to 20 ppm. Mass calibration and parameter optimization were enabled, and isotope error was set to 0/1/2 with two missed trypsin cleavages allowed. The peptide length was set from 7 to 50, and the peptide mass was set to 500–5,000 Da. Carbamidomethylation of C (+57.021464 Da) was set as fixed modification and Oxidation of M (+15.994915 Da) and acetylation of protein N-term (+42.010565 Da) as variable modifications. Philosopher (Da Veiga Leprevost et al., 2020) with PeptideProphet (Keller et al., 2002) and ProteinProphet (Nesvizhskii et al., 2003) was used to estimate the identification FDR. The PSMs were filtered at 1% PSM and 1% protein identification FDR. Quantification and match between runs (MBR) was performed with IonQuant using default values (Yu et al., 2021).
Mass spectrometry data along with the identification results have been deposited in the ProteomeXchange Consortium via the PRIDE partner repository (Vizcaino et al., 2013) with the dataset identifier PXD030699.
Bioinformatic Analysis of Proteomic Sequence Data
Label-free quantitative (LFQ) analysis of identified proteins was performed with the MSstats R package (Choi et al., 2014) using default parameters (equalizeMedians as normalization method; log transformation: 2; Tukey's median polish as the summary method; censored values in intensity column: null and MBimpute: false). Using a power calculation of 0.9 and FDR of 0.05, fold-changes were considered as significant when ≥2.450 and adjusted p-value ≤ 0.05. STRINGDB https://string-db.org was used to perform a PPI analysis based on confidence in the interaction (minimum required interaction score ≥0.7) and the network was visualized using Cytoscape. The Cytoscape plugin ClueGO was used to integrate the Kyoto Encyclopedia of Genes and Genomes (KEGG), and Gene Ontology information (including biological processes, immune processes and molecular functions) (Bindea et al., 2009). The enrichment tests for terms and groups were two-sided tests based on the hyper-geometric distribution with a Kappa Score Threshold of 0.4. All GO terms that were significant with P <0.05 (after correcting for multiple testing using the Bonferroni step down FDR correction), ranged between 3 and 8 tree intervals and contained a minimum of three genes (representing at least 4% from the total number of associated genes) were selected for further analysis. For each group/cluster, only the node with the smallest adjusted P-value was annotated.
The 4,143 S. mansoni genes associated with 68 molecularly distinct and annotated clusters identified by single cell RNA-seq profiling (scRNAseq) from an earlier report (Wendt et al., 2020) were retrieved and intersected with the 793 detected ESP proteins. Binomial distribution tests (MS Excel) were used to identify significant enrichment of ESP proteins in each cluster using the total number of ESPs across the clusters as the number of “trials,” the number of ESPs in the specific cluster as the number of “successes” and the proportion of the 4,413 total cluster-enriched genes in the specific cluster as the “expected probability.” FDR correction was applied to the resulting P-values for multiple test corrections. A threshold FDR value of 0.01 was considered significant.
Results
S. mansoni Adult Males Secrete More Proteins in Maintenance Culture Than Adult Female Worms
S. mansoni adult worms were obtained from experimentally infected mice and separated into three different groups: males (M), females (F) or left coupled as male and female pairs (MF). The worms were maintained for seven days and the secreted proteins analyzed by LC-MS/MS. A label-free quantitative (LFQ) analysis was performed to identify the proteins with significantly up- or down-regulated abundance in the secretomes of all three sample groups. An input file containing unique and razor peptides for 934 validated proteins was generated by MSFragger (Supplementary Table S1). Data were normalized using medians of summed intensities (Supplementary Figure S1). MSstats was used to estimate the power of the analysis performed. For our analysis, and to have a power calculation = 0.9 and FDR = 0.05, fold-changes were considered as significant when ≥2.450 (Log2 ≥ 1.3) and the adjusted P-value ≤ 0.05 (Supplementary Figure S2).
The quantitative proteomic analysis revealed a clear sex-dependent protein profile. For the M vs. F comparison, a total of 793 proteins were quantified, from which 237 and 75 were uniquely detected in males and females, respectively (Supplementary Table S2). Furthermore, the relative abundance of 133 proteins was significantly higher in the secretome of males (Table 1; Figure 1; Supplementary Table S2), while the abundance level of 65 proteins was significantly higher in the secretome of females (Table 2; Figure 1; Supplementary Table S2).
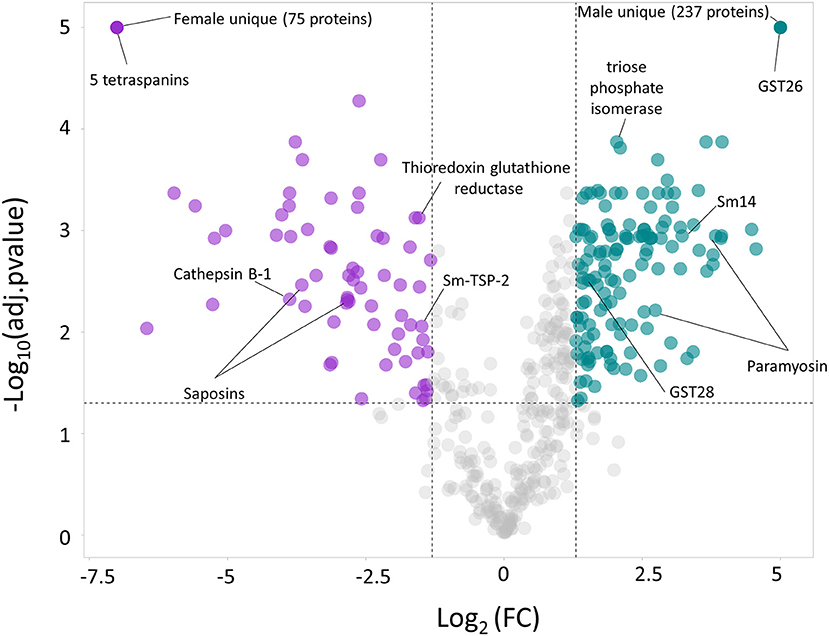
Figure 1. Volcano plot highlighting the proteins with significantly divergent relative protein abundance in the secretome of the male (M) and female (F) Schistosoma mansoni flukes. Since uniquely expressed proteins cannot be plotted in a volcano plot, they were assigned arbitrary values of –Log10(adj.pvalue) = 5 and Log2FC = 5 (males) and Log2FC = −7.5 (females).
When comparing MF vs. M, 27 and 15 proteins were exclusively identified in MF and M, respectively, and thus could not be quantified. However, the relative abundance of the remaining 700 proteins was not significantly different (Supplementary Figure S3), suggesting that males contribute more to the total MF secretome than females. It is worth noting that for some well-characterized Schistosoma spp. vaccine candidates (26) were upregulated in the secretome of females [i.e., A0A5K4F8N6_SCHMA (Sm-TSP-2), Q8MNY2_SCHMA (cathepsin B-1), A0A5K4EE66_SCHMA (thioredoxin glutathione reductase)] or males [i.e., A0A3Q0KIP4_SCHMA, A0A3Q0KD88_SCHMA (paramyosin), FABP_SCHMA (Sm14), G4V6B9_SCHMA (triose phosphate isomerase)]. Furthermore, GST26_SCHMA (glutathione S-transferase 26) was uniquely identified in the secretome from males and two saposins (G4VBU4_SCHMA and A0A3Q0KK40_SCHMA) were also upregulated in the secretome of females. It is also worth highlighting that five different tetraspanins (A0A3Q0KTH5_SCHMA, G4LUN6_SCHMA, A0A5K4FD80_SCHMA, Q86D97_SCHMA and G4LWW2_SCHMA) were uniquely found in the secretome of females, while Sm-TSP-2 was found upregulated in the secretome of females.
S. mansoni Male Adult Worms Secrete Proteins Implicated in Carbohydrate Metabolism, Redox Function, and Cytoskeletal Organization
A protein-protein association (PPA) analysis of proteins uniquely secreted or upregulated in the secretome of S. mansoni adult males revealed a strong network of interacting proteins. Approximately 70% of the proteins interacted in one or multiple clusters with other proteins. Whereas, most unique and upregulated proteins interacted together, one cluster of male-unique proteins could be observed (Supplementary Figure S4, circle), which included splicing factors, RNA binding proteins and ribonucleoproteins. Furthermore, a clear cluster of proteins with upregulated abundance in males was associated with glycolysis and gluconeogenesis pathways (Supplementary Figure S4, rectangle).
The functional analysis showed at least 7 groups (adjusted P-value <0.0005) containing 67 non-redundant biological terms (adjusted P-value <0.05), including “glucose metabolic process” (GO:0006006), “cell redox homeostasis” (GO:0045454), “pentose phosphate pathway” (KEGG:00030), “starch and sucrose metabolism” (KEGG:00500, “glycolysis/gluconeogenesis” (KEGG:00010), “actin filament depolymerization” (GO:0030042) and “carbohydrate metabolic process” (GO:0005975) (Figure 2; Supplementary Table S3).
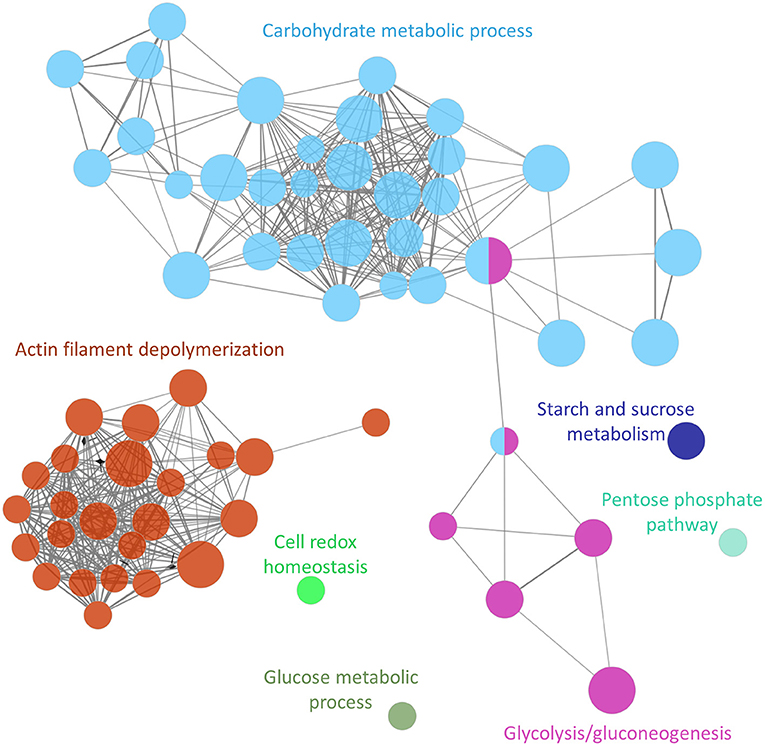
Figure 2. Functional analysis network showing the gene ontology terms and KEGG pathways with the highest significance of proteins uniquely secreted or upregulated in the secretome of the adult Schistosoma mansoni male.
The cluster containing the highest number of terms (31 nodes) and proteins (total of 54 proteins) was associated with pathways involved in carbohydrate metabolism (a gene ontology term also associated with the KEGG pathway glycolysis/gluconeogenesis) (Figure 2; Supplementary Table S3). Proteins involved in this pathway included phosphoenolpyruvate carboxykinase, glucose-6-phosphate dehydrogenase and malate dehydrogenase among others. The cluster with the next highest number of terms (24 nodes) and proteins (22 proteins) was associated with a cytoskeleton organization function, and included calponin, paramyosin, filamin, and tubulin.
S. mansoni Female Adult Worms Secrete Proteins With Hydrolase Activity and Cellular Homeostasis
The PPI analysis of proteins uniquely secreted or upregulated in the secretome of the adult female showed that only around 50% of the proteins interacted in one or multiple clusters with other proteins (Supplementary Figure S5). Contrary to what was observed for male schistosomes, uniquely female-secreted proteins or proteins with an upregulated expression in the secretome of females did not cluster together (Supplementary Figure S5).
The functional analysis showed at least 6 groups (adjusted P-value <0.005) containing 54 non-redundant biological terms (adjusted P-value <0.05), including “lysosome” (KEGG:04142), “calcium-dependent phospholipid binding” (GO:0005544), “transmembrane transporter activity” (GO:0022857), “hydrolase activity” (GO:0016798), “peptidase activity” (GO:0070011) and “transition metal ion transport” (GO:0000041) (Figure 3; Supplementary Table S4). The cluster with the highest number of terms and proteins was associated with the cellular homeostasis process (28 nodes and 20 proteins) and included ferritin and thioredoxin glutathione reductase (Figure 3; Supplementary Table S4). Additionally, two clusters associated with peptidase activity (9 nodes and 15 proteins) and hydrolase activity (7 nodes and 11 proteins) were also highlighted to be of importance in this analysis (Figure 3; Supplementary Table S4). These two clusters included galactosidase, glucosidase, cathepsin B, cathepsin L, carboxypeptidase, other cysteine- and serine-peptidases, and others (Supplementary Table S4).
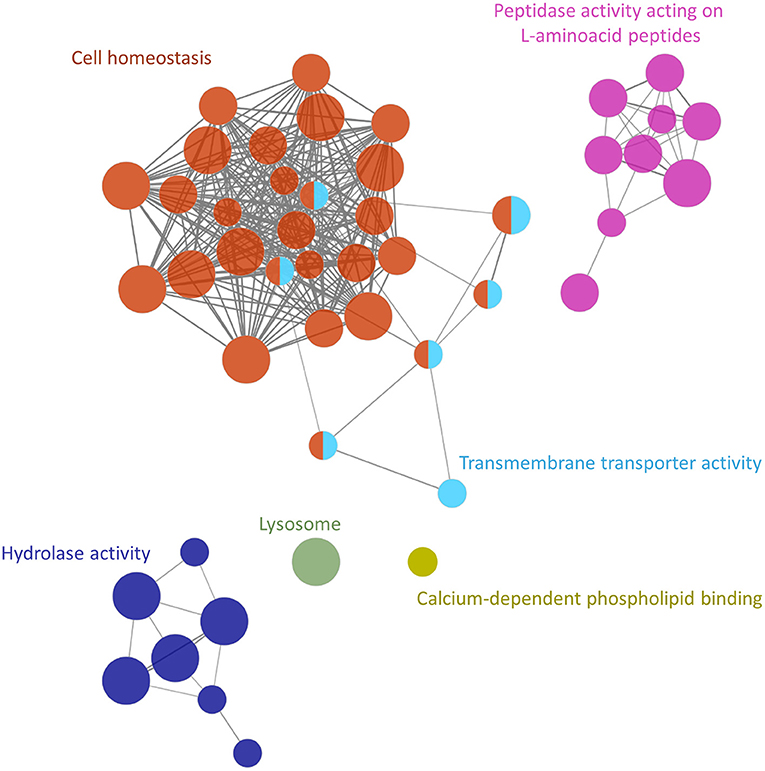
Figure 3. Functional analysis network showing the gene ontology terms and KEGG pathways with the highest significance of proteins uniquely secreted or upregulated in the secretome of the Schistosoma mansoni adult female.
Intersection and Significant Enrichment of Detected ESPs Among Tissue Cluster-Enriched Gene Sets
Wendt et al. recently identified transcriptomic signatures associated with specific tissues in S. mansoni using single cell RNA-seq profiling (scRNAseq) (Wendt et al., 2020), with 4,143 genes significantly upregulated in one or more of the 68 molecularly-distinct annotated clusters. Of the 793 ESPs from adult female and male stages of S. mansoni, 449 were identified among the cluster-enriched genes from this scRNAseq study. Significant enrichment (after FDR correction) was identified for 24 tissue clusters among all 793 ESPs, nine clusters among the 302 genes either unique or higher in adult female ESPs, and 12 clusters among the 370 genes either unique or higher in adult male ESPs (Table 3; Supplementary Table S7). The 793 total ESPs and the 302 female-specific or overexpressed ESPs were most significantly enriched among the “gut” cluster, with 54.3% and 43.2% of the gut cluster-associated genes being identified in these ESP sets (respectively; P <10−15 for both comparisons; Supplementary Table S7). This indicated that ESPs from the females are likely secreted from the intestine, whereas the male ESPs contribute to the enrichment of the muscle-associated clusters identified in the combined ESP set (Table 3; Supplementary Table S7).
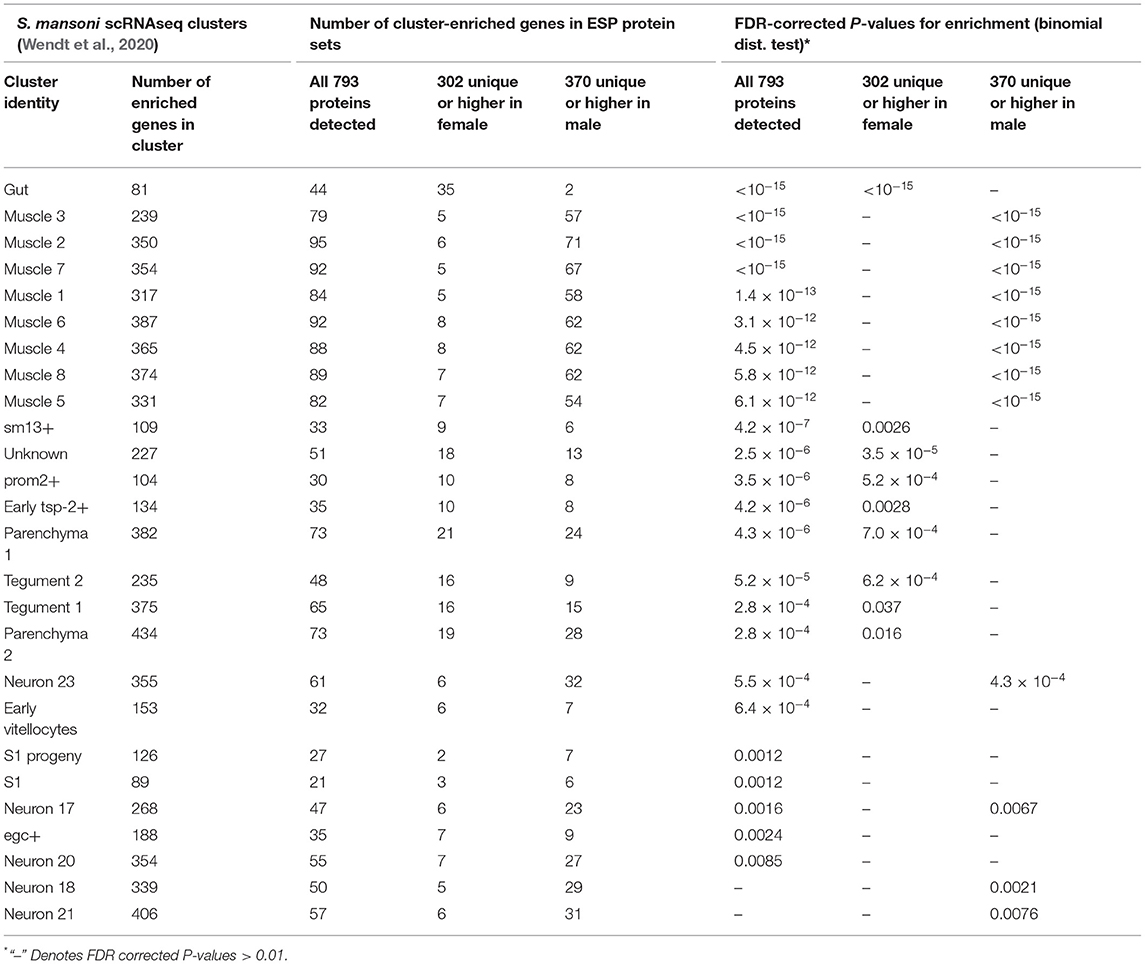
Table 3. Intersection and significant enrichment of ESPs among tissue cluster-enriched gene sets identified from the scRNAseq study of Wendt et al. (2020).
Discussion
Schistosomes are among the most important human helminths in terms of public health, morbidity and mortality. Unlike other trematodes, they are usually found as paired couples, with the female occupying the gynaecophoric canal of the male (Mcmanus et al., 2018). This dioecy distinguishes schistosomes from other human flatworms. Moreover, the sexual dimorphism has biological implications, including the need for pairing to achieve sexual maturity in females (reviewed in Moore et al., 1954), and favoring a division of labor between the muscular male migrating toward oviposition sites and the more delicate, filiform female reaching small vessels to discharge the eggs (Loker and Brant, 2006). Yet despite their importance in male-female communication, only a handful of studies have analyzed in depth the molecules, mediators and receptors involved in these interactions (Armstrong, 1965; Basch and Basch, 1984; Gupta and Basch, 1987; Hall et al., 2011; Chen et al., 2022). In this study, we set out to comprehensively characterize the protein complement of the ESPs from adult stage female and male S. mansoni to increase knowledge into the biology of these worms and to augment the repertoire of potential diagnostic and vaccine candidates for schistosomiasis. We cultured adult worms separately for seven days in vitro and analyzed the ESPs by mass spectrometry. Although extended incubation times (as well as pairing of adult males and females) have been shown to influence the worms [e.g., loss of mature vitellocytes after 6 days of culture (Wang et al., 2019)], other studies have also cultured worms for 4 days or longer (Hall et al., 2011). A time-series proteomic analysis as well as differences in ESPs in adult worms from single-sex infections would likely be informative with respect to culture conditions and pairing in schistosome development.
Proteins released and secreted by schistosomes, including tegumental proteins and digestive enzymes (in the vomitus Hall et al., 2011) play a key role in host-parasite and male-female interactions, and their expression and secretion is driven by the divergent requirements and functions of both schistosome sexes. For instance, in S. japonicum and S. bovis, the male schistosome exhibits significantly more proteins in its tegument than the female, both in total number and in unique proteins (Perez-Sanchez et al., 2008; Zhang et al., 2013). Our present findings agree with this assessment and, indeed, were not unexpected given the larger size of the male schistosome. Furthermore, the protein and small RNA content of S. japonicum-secreted extracellular vesicles and the expression of phosphoproteins from S. mekongi are also sex-dependant (Du et al., 2020). The present results also revealed a gender-specific divergence in the secretome landscape in S. mansoni, which, as shown at the transcriptomic level, could be related to the sexual dimorphism of the schistosome (Fitzpatrick et al., 2005; Anderson et al., 2015; Picard et al., 2016). S. japonicum female-tegumental proteins are involved in protein glycosylation and lysosome function, while male-tegumental proteins play a role in intracellular signal transduction, regulation of actin filament polymerization, and proteasome core complex (Zhang et al., 2013). Our findings also showed functions related to actin filament depolymerisation and lysosome to be important in male and female-secreted proteins, respectively. While proteins belonging to the lysosome KEGG ontology are a markedly heterogeneous group including cathepsin B peptidases, tetraspanins and glycosidases, proteins related to actin filament depolymerisation play a specific role in cytoskeletal regulation. These results confirm previous transcriptomic studies and support the hypothesis of the role of the male schistosome in physical support of the female to facilitate migration against the flow of the portal circulation toward the mesenteric venules where the schistosome eggs are deposited (Fitzpatrick et al., 2005; Cai et al., 2016; Phuphisut et al., 2018). Indeed, when comparing male-specific and significantly expressed male ESPs with recently published scRNA data, we observed and enrichment of the muscle-associated clusters.
Glucose metabolism is essential in the female schistosome due to the energy requirement to support the production and release of the large number of eggs—around 300 eggs per female per day in S. mansoni (Cheever et al., 1994). Glycogen consumption, however, is paramount for other functions such as muscle contraction and tegumental membrane repair, both being significantly enriched among proteins abundant in the adult male vs. the female (Gobert et al., 2003). Earlier transcriptomic investigation revealed that the expression of the glucose transporters gtp1 and gtp4 was uninfluenced by schistosome gender (Cai et al., 2016). Here, by contrast, significantly elevated abundance of GTP1 (Smp_012440, Q26579_SCHMA) and other glucose transporters (Smp_105410, G4VC44_SCHMA) was evident in the female S. mansoni, likely the result of an increased expression in the tegument. These discrepancies may in part be explained since transcriptomic analysis was performed on whole worms whereas the present study focused on the ESPs. Other glycolytic enzymes such as aldolase (Smp_042160.1, ALF_SCHMA) and glycerol-3-phosphate dehydrogenase (G3PDH, Smp_030500.1, C4Q5J8_SCHMA) were more abundantly secreted by the male. Our findings conformed with previous reports of higher consumption of glucose and importance of glycogen storage in the male worm, which could reflect the muscular effort involved in transporting the female through the portal vasculature, and the need by the female to transport these metabolites from the male (Skelly et al., 2014). Notably from the viewpoint of infection control, aldolase and G3PDH have been a focus of vaccines and other intervention targets (Dessein et al., 1988; Goudot-Crozel et al., 1989; Tallima et al., 2017).
In addition, the findings indicate that hydrolases and peptidases play an important role in the biology of female schistosomes. The hydrolase grouping includes lipases, phosphatases, and glycosidases among others. In the case of the female schistosome, several glycosidases were found more abundantly secreted, including beta-glucosidase (Smp_043390, A0A3Q0KF32_SCHMA), alpha-galactosidase (Smp_089290, G4VLE3_SCHMA), and several alpha-amylases. Furthermore, it has been suggested that hydrolases released by the schistosome egg contribute to the transit of the egg and the circumoval granuloma across the intestinal wall and also with the nutritional requirements of the embryo (Cesari et al., 2000). Despite our findings, we cannot rule out that hydrolases detected here could have been secreted by eggs when released by females in vitro and may not originate from the adult female. Other peptidases including cathepsins participate in invasion of the skin by the cercaria (Dvorak et al., 2008), hemoglobin degradation by the adult stage (Gotz and Klinkert, 1993; Dalton et al., 1995), and egg hatching (Rinaldi et al., 2009), and have profound immunogenic properties (Soloviova et al., 2019). Females secreted several cathepsins more abundantly than males, including SmCB1 (Q8MNY2_SCHMA), which is known to be secreted from the gut of schistosomes and to contribute to Th2 polarization responses (De Oliveira Fraga et al., 2010). This enzyme has been validated as a potent anti-schistosome chemotherapeutic target (Abdulla et al., 2007; Jilkova et al., 2021).
A number of other well-characterized vaccine candidates were upregulated in the secretome of the male S. mansoni adult, i.e., Sm14 (Smp_095360.1, FABP_SCHMA), GST26 (Smp_163610.1, GST26_SCHMA), GST28 (Smp_054160.1, GST28_SCHMA), three paramyosin isoforms (Smp_046060.1, A0A3Q0KFC2_SCHMA; Smp_085540.6, A0A3Q0KIP4_SCHMA; Smp_021920.3, A0A3Q0KD88_SCHMA), and in the secretome of the female, i.e., Sm-TSP-2 (Smp_335630.1, A0A5K4F8N6_SCHMA), thioredoxin glutathione reductase (A0A5K4EE66_SCHMA), cathepsin B1 (Smp_103610.1, Q8MNY2_SCHMA) and several saposins (Smp_194910.1, G4VHH1_SCHMA; Smp_130100.1, G4VBU4_SCHMA, Smp_105450.1, A0A3Q0KK40_SCHMA). Most tetraspanins identified were significantly upregulated in the secretome of female worms. Sm-TSP-2 formulated with glucopyranosyl lipid adjuvant has proven safe in a phase I trial (Keitel et al., 2019), and a homolog in S. haematobium (Sh-TSP-2) shows efficacy in a heterologous mouse model of schistosomiasis (Mekonnen et al., 2020). Furthermore, tetraspanins represent confirmed diagnostic markers of urogenital schistosomiasis (Pearson et al., 2021; Mekonnen et al., 2022). Notably, we observed five additional tetraspanins uniquely present in the female ESP. Given the expanding reputation of tetraspanins as diagnostic antigens for detection of infection with S. haematobium (Pearson et al., 2021; Mekonnen et al., 2022), these homologs from S. mansoni might be worthy of consideration for serodiagnosis. It is also noteworthy that both GST26 and GST28 were upregulated in the secretome of the male. Although GSTs have been extensively investigated as vaccine candidates (Riveau et al., 1998), recent reports reveal a lack of efficacy against urogenital schistosomiasis (Riveau et al., 2018). Intriguingly, tetraspanins (including Sm-TSP-2) and GSTs have been identified in the extracellular vesicles secreted by S. mansoni adult worms (Sotillo et al., 2016). Although beyond the scope of this report, it would be valuable to analyse the contribution of each sex to the secretion of extracellular vesicles and to determine their composition and biochemical profiles.
We have additionally identified 75 and 237 proteins uniquely secreted by female and male worms, respectively. Whereas, these proteins might reflect the gender-specific biology, they also likely contribute to male-female communication. Recent reports have highlighted the secretion by male schistosomes of a specific small molecule (ß-alanyl-tryptamine) that is key for the development and laying of eggs by females (Chen et al., 2022) (ß -alanyl-tryptamine is a diminutive peptide of ~300 Daltons in mass which would not have been retained in our samples during centrifugation which employed a 3 kDa cutoff membrane). Furthermore, in S. japonicum, biogenic amine neurotransmitters are implicated in male-female sexual communication (Wang et al., 2017). Based on the present findings, we posit that the development of drugs interrupting male-female communication could lead to novel and effective control measures.
To conclude, a broader coverage of the S. mansoni ESP profile, and deeper knowledge of the secretome contributes to our understanding of schistosome biology, and thus these new findings provide new information including leads for the development of novel vaccine strategies. Moreover, identification of the most abundantly secreted proteins of the schistosome sexes should enhance analysis of the regulatory elements and motifs that control the expression of the corresponding genes. This will be of assistance in the development of transgenic schistosomes that over-express endogenous proteins, or even secrete foreign proteins. Access by the field to transgenic schistosomes that (conditionally) secrete reporters, model antigens, and other informative gene products, along with advances in human challenge models (Langenberg et al., 2020) can be expected to hasten progress in the immunobiology and pharmacology of these flukes (Hoffmann et al., 2014; Zamanian and Andersen, 2016; Mcveigh and Maule, 2019; Douglas et al., 2021; Quinzo et al., 2022).
Data Availability Statement
Mass spectrometry data along with the identification results have been deposited in the ProteomeXchange Consortium via the PRIDE partner repository with the dataset identifier PXD030699.
Ethics Statement
The animal study was reviewed and approved by Institutional Animal Care and Use Committee (IACUC) at GWU.
Author Contributions
VM, EK, WI, PB, and JS designed the experiments. EK, MM, BR, BB, AL, PB, and JS analyzed the data. JS, EK, VM, and PB drafted the manuscript with input from all the co-authors. WI, EK, and VM contributed the helminth materials. JS performed mass spectrometry focused analysis. JS, AL, and PB supervised the project. JS, AL, MM, PB, and BB arranged the funding. All authors read and approved the final draft.
Funding
The findings were obtained from work partially supported by the Defense Advanced Research Projects Agency (DARPA) and Naval Information Warfare Center Pacific (NIWC Pacific), under Contract No. N66001-21-C-4013 (Approved for Public Release, Distribution Unlimited).
Author Disclaimer
The views, opinions, and/or findings expressed are those of the authors and should not be interpreted as representing the official views or policies of the Department of Defense or the U.S. Government.
Conflict of Interest
BB was employed by Charles River Analytics, Inc.
The remaining authors declare that the research was conducted in the absence of any commercial or financial relationships that could be construed as a potential conflict of interest.
Publisher's Note
All claims expressed in this article are solely those of the authors and do not necessarily represent those of their affiliated organizations, or those of the publisher, the editors and the reviewers. Any product that may be evaluated in this article, or claim that may be made by its manufacturer, is not guaranteed or endorsed by the publisher.
Acknowledgments
The proteomic analysis was performed in the proteomics facility of SCSIE University of Valencia. For the purposes of Open Access, the authors have applied a CC BY public copyright license to any Author Accepted Manuscript version arising from this submission. Schistosome-infected mice and adult schistosomes were provided by the NIAID Schistosomiasis Resource Center of the Biomedical Research Institute, Rockville, Maryland through NIH-NIAID Contract HHSN272201000005I for distribution through BEI Resources.
Supplementary Material
The Supplementary Material for this article can be found online at: https://www.frontiersin.org/articles/10.3389/fpara.2022.950744/full#supplementary-material
Supplementary Figure S1. Data normalization using medians of summed intensities after label-fere quantitative analysis.
Supplementary Figure S2. Power calculation and false discovery rate of the label-free quantitative analysis performed using MSstats.
Supplementary Figure S3. Volcano plot of Schistosoma mansoni secreted proteins from male vs male-female (mix). Statistically significant differences were not apparent.
Supplementary Figure S4. Protein-protein association network of all proteins uniquely present or with significantly higher abundance in the secretome of the adult S. mansoni male.
Supplementary Figure S5. Protein-protein association network of all proteins uniquely present or with a significantly upregulated expression in the secretome of the adult S. mansoni female.
Supplementary Table S1. MSstats input file containing unique and razor peptides generated by MSFragger.
Supplementary Table S2. Label-free quantitative analysis of male vs. Female secreted proteins.
Supplementary Table S3. Functional analysis of differentially abundant male secreted proteins using ClueGO.
Supplementary Table S4. Functional analysis of differentially abundant female secreted proteins using ClueGO.
Supplementary Table S5. Corresponding Uniprot and Schistosoma mansoni genome codes and annotations for the GO/KEGG terms of proteins uniquely secreted or upregulated in the secretome of S. mansoni adult males.
Supplementary Table S6. Corresponding Uniprot and Schistosoma mansoni genome codes and annotations for the GO/KEGG terms of proteins uniquely secreted or upregulated in the secretome of S. mansoni adult females.
Supplementary Table S7. Proteins secreted by male or female Schistosoma mansoni adult worms intersecting and significantly enriched among gut-enriched gene sets identified from a previous scRNAseq study [1].
References
Abdulla, M. H., Lim, K. C., Sajid, M., Mckerrow, J. H., Caffrey, C. R. (2007). Schistosomiasis mansoni: novel chemotherapy using a cysteine protease inhibitor. PLoS Med. 4, e14. doi: 10.1371/journal.pmed.0040014
Acharya, S., Da'dara, A. A., Skelly, P. J. (2021). Schistosome immunomodulators. PLoS Pathog. 17, e1010064. doi: 10.1371/journal.ppat.1010064
Anderson, L., Amaral, M. S., Beckedorff, F., Silva, L. F., Dazzani, B., Oliveira, K. C., et al. (2015). Schistosoma mansoni egg, adult male and female comparative gene expression analysis and identification of novel genes by RNA-Seq. PLoS Negl. Trop. Dis. 9, e0004334. doi: 10.1371/journal.pntd.0004334
Armstrong, J. C.. (1965). Mating behavior and development of schistosomes in the mouse. J. Parasitol. 51, 605–616. doi: 10.2307/3276242
Basch, P. F., Basch, N. (1984). Intergeneric reproductive stimulation and parthenogenesis in Schistosoma mansoni. Parasitology 89(Pt 2), 369–376. doi: 10.1017/S0031182000001372
Bindea, G., Mlecnik, B., Hackl, H., Charoentong, P., Tosolini, M., Kirilovsky, A., et al. (2009). ClueGO: a Cytoscape plug-in to decipher functionally grouped gene ontology and pathway annotation networks. Bioinformatics 25, 1091–1093. doi: 10.1093/bioinformatics/btp101
Boissier, J., Grech-Angelini, S., Webster, B. L., Allienne, J. F., Huyse, T., Mas-Coma, S., et al. (2016). Outbreak of urogenital schistosomiasis in Corsica (France): an epidemiological case study. Lancet Infect. Dis. 16, 971–979. doi: 10.1016/S1473-3099(16)00175-4
Braschi, S., Borges, W. C., Wilson, R. A. (2006a). Proteomic analysis of the schistosome tegument and its surface membranes. Mem. Inst. Oswaldo Cruz. 101(Suppl. 1), 205–212. doi: 10.1590/S0074-02762006000900032
Braschi, S., Curwen, R. S., Ashton, P. D., Verjovski-Almeida, S., Wilson, A. (2006b). The tegument surface membranes of the human blood parasite Schistosoma mansoni: a proteomic analysis after differential extraction. Proteomics 6, 1471–1482. doi: 10.1002/pmic.200500368
Braschi, S., Wilson, R. A. (2006). Proteins exposed at the adult schistosome surface revealed by biotinylation. Mol. Cell Proteomics 5, 347–356. doi: 10.1074/mcp.M500287-MCP200
Cai, P., Liu, S., Piao, X., Hou, N., Gobert, G. N., Mcmanus, D. P., et al. (2016). Comprehensive transcriptome analysis of sex-biased expressed genes reveals discrete biological and physiological features of male and female Schistosoma japonicum. PLoS Negl Trop Dis 10, e0004684. doi: 10.1371/journal.pntd.0004684
Cesari, I. M., Ballen, D. E., Perrone, T., Oriol, O., Hoebeke, J., Bout, D. (2000). Enzyme activities in Schistosoma mansoni soluble egg antigen. J. Parasitol. 86, 1137–1140. doi: 10.1645/0022-3395(2000)086[1137:EAISMS]2.0.CO;2
Cheever, A. W., Macedonia, J. G., Mosimann, J. E., Cheever, E. A. (1994). Kinetics of egg production and egg excretion by Schistosoma mansoni and S. japonicum in mice infected with a single pair of worms. Am. J. Trop. Med. Hyg. 50, 281–295. doi: 10.4269/ajtmh.1994.50.281
Chen, R., Wang, J., Gradinaru, I., Vu, H. S., Geboers, S., Naidoo, J., Ready, J. M., Williams, N. S., Deberardinis, R. J., Ross, E. M., Collins, J. J. III. (2022). A male-derived nonribosomal peptide pheromone controls female schistosome development. Cell. 1506–1520. doi: 10.1016/j.cell.2022.03.017
Choi, M., Chang, C. Y., Clough, T., Broudy, D., Killeen, T., Maclean, B., et al. (2014). MSstats: an R package for statistical analysis of quantitative mass spectrometry-based proteomic experiments. Bioinformatics 30, 2524–2526. doi: 10.1093/bioinformatics/btu305
Colley, D. G., Bustinduy, A. L., Secor, W. E., King, C. H. (2014). Human schistosomiasis. Lancet 383, 2253–2264. doi: 10.1016/S0140-6736(13)61949-2
Da Veiga Leprevost, F., Haynes, S. E., Avtonomov, D. M., Chang, H. Y., Shanmugam, A. K., Mellacheruvu, D., et al. (2020). Philosopher: a versatile toolkit for shotgun proteomics data analysis. Nat. Methods 17, 869–870. doi: 10.1038/s41592-020-0912-y
Dalton, J. P., Clough, K. A., Jones, M. K., Brindley, P. J. (1997). The cysteine proteinases of Schistosoma mansoni cercariae. Parasitology 114(Pt 2), 105–112. doi: 10.1017/S003118209600830X
Dalton, J. P., Smith, A. M., Clough, K. A., Brindley, P. J. (1995). Digestion of haemoglobin by schistosomes: 35 years on. Parasitol. Today 11, 299–303. doi: 10.1016/0169-4758(95)80045-X
De Marco Verissimo, C., Potriquet, J., You, H., Mcmanus, D. P., Mulvenna, J., Jones, M. K. (2019). Qualitative and quantitative proteomic analyses of Schistosoma japonicum eggs and egg-derived secretory-excretory proteins. Parasit. Vect. 12, 173. doi: 10.1186/s13071-019-3403-1
De Oliveira Fraga, L. A., Lamb, E. W., Moreno, E. C., Chatterjee, M., Dvorak, J., Delcroix, M., et al. (2010). Rapid induction of IgE responses to a worm cysteine protease during murine pre-patent schistosome infection. BMC Immunol. 11, 56. doi: 10.1186/1471-2172-11-56
Dessein, A. J., Begley, M., Demeure, C., Caillol, D., Fueri, J., Dos Reis, M. G., et al. (1988). Human resistance to Schistosoma mansoni is associated with IgG reactivity to a 37-kDa larval surface antigen. J. Immunol. 140, 2727–2736.
Douglas, B., Wei, Y., Li, X., Ferguson, A., Hung, L. Y., Pastore, C., et al. (2021). Transgenic expression of a T cell epitope in Strongyloides ratti reveals that helminth-specific CD4+ T cells constitute both Th2 and Treg populations. PLoS Pathog. 17, e1009709. doi: 10.1371/journal.ppat.1009709
Du, P., Giri, B. R., Liu, J., Xia, T., Grevelding, C. G., Cheng, G. (2020). Proteomic and deep sequencing analysis of extracellular vesicles isolated from adult male and female Schistosoma japonicum. PLoS Negl. Trop. Dis. 14, e0008618. doi: 10.1371/journal.pntd.0008618
Dvorak, J., Fajtova, P., Ulrychova, L., Leontovyc, A., Rojo-Arreola, L., Suzuki, B. M., et al. (2016). Excretion/secretion products from Schistosoma mansoni adults, eggs and schistosomula have unique peptidase specificity profiles. Biochimie 122, 99–109. doi: 10.1016/j.biochi.2015.09.025
Dvorak, J., Mashiyama, S. T., Braschi, S., Sajid, M., Knudsen, G. M., Hansell, E., et al. (2008). Differential use of protease families for invasion by schistosome cercariae. Biochimie 90, 345–358. doi: 10.1016/j.biochi.2007.08.013
Fitzpatrick, J. M., Johnston, D. A., Williams, G. W., Williams, D. J., Freeman, T. C., Dunne, D. W., et al. (2005). An oligonucleotide microarray for transcriptome analysis of Schistosoma mansoni and its application/use to investigate gender-associated gene expression. Mol. Biochem. Parasitol. 141, 1–13. doi: 10.1016/j.molbiopara.2005.01.007
Floudas, A., Cluxton, C. D., Fahel, J., Khan, A. R., Saunders, S. P., Amu, S., et al. (2017). Composition of the Schistosoma mansoni worm secretome: identification of immune modulatory Cyclophilin A. PLoS Negl. Trop. Dis. 11, e0006012. doi: 10.1371/journal.pntd.0006012
Gobert, G. N., Stenzel, D. J., Mcmanus, D. P., Jones, M. K. (2003). The ultrastructural architecture of the adult Schistosoma japonicum tegument. Int. J. Parasitol. 33, 1561–1575. doi: 10.1016/S0020-7519(03)00255-8
Gotz, B., Klinkert, M. Q. (1993). Expression and partial characterization of a cathepsin B-like enzyme (Sm31) and a proposed 'haemoglobinase' (Sm32) from Schistosoma mansoni. Biochem. J. 290(Pt 3), 801–806. doi: 10.1042/bj2900801
Goudot-Crozel, V., Caillol, D., Djabali, M., Dessein, A. J. (1989). The major parasite surface antigen associated with human resistance to schistosomiasis is a 37-kD glyceraldehyde-3P-dehydrogenase. J. Exp. Med. 170, 2065–2080. doi: 10.1084/jem.170.6.2065
Gupta, B. C., Basch, P. F. (1987). Evidence for transfer of a glycoprotein from male to female Schistosoma mansoni during Pairing. J. Parasitol. 73, 674–675. doi: 10.2307/3282159
Hall, S. L., Braschi, S., Truscott, M., Mathieson, W., Cesari, I. M., Wilson, R. A. (2011). Insights into blood feeding by schistosomes from a proteomic analysis of worm vomitus. Mol. Biochem. Parasitol. 179, 18–29. doi: 10.1016/j.molbiopara.2011.05.002
Hoffmann, K. F., Brindley, P. J., Berriman, M. (2014). Medicine. Halting harmful helminths. Science 346, 168–169. doi: 10.1126/science.1261139
Huyse, T., Webster, B. L., Geldof, S., Stothard, J. R., Diaw, O. T., Polman, K., et al. (2009). Bidirectional introgressive hybridization between a cattle and human schistosome species. PLoS Pathog. 5, e1000571. doi: 10.1371/journal.ppat.1000571
Jilkova, A., Rubesova, P., Fanfrlik, J., Fajtova, P., Rezacova, P., Brynda, J., et al. (2021). Druggable Hot spots in the schistosomiasis cathepsin B1 target identified by functional and binding mode analysis of potent vinyl sulfone inhibitors. ACS Infect. Dis. 7, 1077–1088. doi: 10.1021/acsinfecdis.0c00501
Keitel, W. A., Potter, G. E., Diemert, D., Bethony, J., El Sahly, H. M., Kennedy, J. K., et al. (2019). A phase 1 study of the safety, reactogenicity, and immunogenicity of a Schistosoma mansoni vaccine with or without glucopyranosyl lipid A aqueous formulation (GLA-AF) in healthy adults from a non-endemic area. Vaccine 37, 6500–6509. doi: 10.1016/j.vaccine.2019.08.075
Keller, A., Nesvizhskii, A. I., Kolker, E., Aebersold, R. (2002). Empirical statistical model to estimate the accuracy of peptide identifications made by MS/MS and database search. Anal. Chem. 74, 5383–5392. doi: 10.1021/ac025747h
Kifle, D. W., Pearson, M. S., Becker, L., Pickering, D., Loukas, A., Sotillo, J. (2020). Proteomic analysis of two populations of Schistosoma mansoni-derived extracellular vesicles: 15k pellet and 120k pellet vesicles. Mol. Biochem. Parasitol. 236, 111264. doi: 10.1016/j.molbiopara.2020.111264
Kong, A. T., Leprevost, F. V., Avtonomov, D. M., Mellacheruvu, D., Nesvizhskii, A. I. (2017). MSFragger: ultrafast and comprehensive peptide identification in mass spectrometry-based proteomics. Nat. Methods 14, 513–520. doi: 10.1038/nmeth.4256
Langenberg, M. C. C., Hoogerwerf, M. A., Koopman, J. P. R., Janse, J. J., Kos-Van Oosterhoud, J., Feijt, C., et al. (2020). A controlled human Schistosoma mansoni infection model to advance novel drugs, vaccines and diagnostics. Nat. Med. 26, 326–332. doi: 10.1038/s41591-020-0759-x
Liu, F., Cui, S. J., Hu, W., Feng, Z., Wang, Z. Q., Han, Z. G. (2009). Excretory/secretory proteome of the adult developmental stage of human blood fluke, Schistosoma japonicum. Mol. Cell Proteomics 8, 1236–1251. doi: 10.1074/mcp.M800538-MCP200
Loker, E. S., Brant, S. V. (2006). Diversification, dioecy and dimorphism in schistosomes. Trends Parasitol. 22, 521–528. doi: 10.1016/j.pt.2006.09.001
Mathieson, W., Wilson, R. A. (2010). A comparative proteomic study of the undeveloped and developed Schistosoma mansoni egg and its contents: the miracidium, hatch fluid and secretions. Int. J. Parasitol. 40, 617–628. doi: 10.1016/j.ijpara.2009.10.014
Mcmanus, D. P., Dunne, D. W., Sacko, M., Utzinger, J., Vennervald, B. J., Zhou, X. N. (2018). Schistosomiasis. Nat. Rev. Dis. Primers 4, 13. doi: 10.1038/s41572-018-0013-8
Mcveigh, P., Maule, A. G. (2019). Can CRISPR help in the fight against parasitic worms? Elife 8, e44382. doi: 10.7554/eLife.44382
Mekonnen, G. G., Tedla, B. A., Pearson, M. S., Becker, L., Field, M., Amoah, A. S., et al. (2022). Characterisation of tetraspanins from Schistosoma haematobium and evaluation of their potential as novel diagnostic markers. PLoS Negl. Trop. Dis. 16, e0010151. doi: 10.1371/journal.pntd.0010151
Mekonnen, G. G., Tedla, B. A., Pickering, D., Becker, L., Wang, L., Zhan, B., et al. (2020). Schistosoma haematobium extracellular vesicle proteins confer protection in a heterologous model of schistosomiasis. Vaccines 8, 416. doi: 10.3390/vaccines8030416
Moore, D. V., Yolles, T. K., Meleney, H. E. (1954). The relationship of male worms to the sexual development of female Schistosoma mansoni. J. Parasitol. 40, 166–185. doi: 10.2307/3274297
Nesvizhskii, A. I., Keller, A., Kolker, E., Aebersold, R. (2003). A statistical model for identifying proteins by tandem mass spectrometry. Anal. Chem. 75, 4646–4658. doi: 10.1021/ac0341261
Neves, L. X., Wilson, R. A., Brownridge, P., Harman, V. M., Holman, S. W., Beynon, R. J., et al. (2020). Quantitative proteomics of enriched esophageal and gut tissues from the human blood fluke Schistosoma mansoni pinpoints secreted proteins for vaccine development. J. Proteome Res. 19, 314–326. doi: 10.1021/acs.jproteome.9b00531
Pearce, E. J., Macdonald, A. S. (2002). The immunobiology of schistosomiasis. Nat. Rev. Immunol. 2, 499–511. doi: 10.1038/nri843
Pearson, M. S., Tedla, B. A., Mekonnen, G. G., Proietti, C., Becker, L., Nakajima, R., et al. (2021). Immunomics-guided discovery of serum and urine antibodies for diagnosing urogenital schistosomiasis: a biomarker identification study. Lancet Microbe 2, e617–e626. doi: 10.1016/S2666-5247(21)00150-6
Perez-Sanchez, R., Valero, M. L., Ramajo-Hernandez, A., Siles-Lucas, M., Ramajo-Martin, V., Oleaga, A. (2008). A proteomic approach to the identification of tegumental proteins of male and female Schistosoma bovis worms. Mol. Biochem. Parasitol. 161, 112–123. doi: 10.1016/j.molbiopara.2008.06.011
Phuphisut, O., Ajawatanawong, P., Limpanont, Y., Reamtong, O., Nuamtanong, S., Ampawong, S., et al. (2018). Transcriptomic analysis of male and female Schistosoma mekongi adult worms. Parasit. Vect. 11, 504. doi: 10.1186/s13071-018-3086-z
Picard, M. A., Boissier, J., Roquis, D., Grunau, C., Allienne, J. F., Duval, D., et al. (2016). Sex-biased transcriptome of Schistosoma mansoni: host-parasite interaction, genetic determinants and epigenetic regulators are associated with sexual differentiation. PLoS Negl. Trop. Dis. 10, e0004930. doi: 10.1371/journal.pntd.0004930
Quinzo, M. J., Perteguer, M. J., Brindley, P. J., Loukas, A., Sotillo, J. (2022). Transgenesis in parasitic helminths: a brief history and prospects for the future. Parasit. Vec. 15, 110. doi: 10.1186/s13071-022-05211-z
Rinaldi, G., Morales, M. E., Alrefaei, Y. N., Cancela, M., Castillo, E., Dalton, J. P., et al. (2009). RNA interference targeting leucine aminopeptidase blocks hatching of Schistosoma mansoni eggs. Mol. Biochem. Parasitol. 167, 118–126. doi: 10.1016/j.molbiopara.2009.05.002
Riveau, G., Poulain-Godefroy, O. P., Dupre, L., Remoue, F., Mielcarek, N., Locht, C., et al. (1998). Glutathione S-transferases of 28kDa as major vaccine candidates against schistosomiasis. Mem. Inst. Oswaldo Cruz 93(Suppl. 1), 87–94. doi: 10.1590/S0074-02761998000700012
Riveau, G., Schacht, A. M., Dompnier, J. P., Deplanque, D., Seck, M., Waucquier, N., et al. (2018). Safety and efficacy of the rSh28GST urinary schistosomiasis vaccine: a phase 3 randomized, controlled trial in Senegalese children. PLoS Negl. Trop. Dis. 12, e0006968. doi: 10.1371/journal.pntd.0006968
Rothe, C., Zimmer, T., Schunk, M., Wallrauch, C., Helfrich, K., Gultekin, F., et al. (2021). Developing endemicity of schistosomiasis, Corsica, France. Emerg. Infect. Dis. 27, 319–321. doi: 10.3201/eid2701.204391
Schwartz, C., Fallon, P. G. (2018). Schistosoma “Eggs-Iting” the host: granuloma formation and egg excretion. Front. Immunol. 9, 2492. doi: 10.3389/fimmu.2018.02492
Skelly, P. J., Da'dara, A. A., Li, X. H., Castro-Borges, W., Wilson, R. A. (2014). Schistosome feeding and regurgitation. PLoS Pathog. 10, e1004246. doi: 10.1371/journal.ppat.1004246
Soloviova, K., Fox, E. C., Dalton, J. P., Caffrey, C. R., Davies, S. J. (2019). A secreted schistosome cathepsin B1 cysteine protease and acute schistosome infection induce a transient T helper 17 response. PLoS Negl. Trop. Dis. 13, e0007070. doi: 10.1371/journal.pntd.0007070
Sotillo, J., Pearson, M., Potriquet, J., Becker, L., Pickering, D., Mulvenna, J., et al. (2016). Extracellular vesicles secreted by Schistosoma mansoni contain protein vaccine candidates. Int J Parasitol 46, 1–5. doi: 10.1016/j.ijpara.2015.09.002
Sotillo, J., Pearson, M. S., Becker, L., Mekonnen, G. G., Amoah, A. S., Van Dam, G., et al. (2019). In-depth proteomic characterization of Schistosoma haematobium: towards the development of new tools for elimination. PLoS Negl. Trop. Dis. 13, e0007362. doi: 10.1371/journal.pntd.0007362
Tallima, H., Dvorak, J., Kareem, S., Abou El Dahab, M., Abdel Aziz, N., Dalton, J. P., et al. (2017). Protective immune responses against Schistosoma mansoni infection by immunization with functionally active gut-derived cysteine peptidases alone and in combination with glyceraldehyde 3-phosphate dehydrogenase. PLoS Negl. Trop. Dis. 11, e0005443. doi: 10.1371/journal.pntd.0005443
Vizcaino, J. A., Cote, R. G., Csordas, A., Dianes, J. A., Fabregat, A., Foster, J. M., et al. (2013). The PRoteomics IDEntifications (PRIDE) database and associated tools: status in 2013. Nucleic Acids Res. 41, D1063–1069. doi: 10.1093/nar/gks1262
Wang, J., Chen, R., Collins, J. J. III. (2019). Systematically improved in vitro culture conditions reveal new insights into the reproductive biology of the human parasite Schistosoma mansoni. PLoS Biol. 17, e3000254. doi: 10.1371/journal.pbio.3000254
Wang, J., Yu, Y., Shen, H., Qing, T., Zheng, Y., Li, Q., et al. (2017). Dynamic transcriptomes identify biogenic amines and insect-like hormonal regulation for mediating reproduction in Schistosoma japonicum. Nat. Commun. 8, 14693. doi: 10.1038/ncomms14693
Wang, W., Bergquist, R., King, C. H., Yang, K. (2021). Elimination of schistosomiasis in China: current status and future prospects. PLoS Negl. Trop. Dis. 15, e0009578. doi: 10.1371/journal.pntd.0009578
Webster, B. L., Southgate, V. R., Littlewood, D. T. (2006). A revision of the interrelationships of Schistosoma including the recently described Schistosoma guineensis. Int. J. Parasitol. 36, 947–955. doi: 10.1016/j.ijpara.2006.03.005
Wendt, G., Zhao, L., Chen, R., Liu, C., O'donoghue, A. J., Caffrey, C. R., Reese, M. L., Collins, J. J. III. (2020). A single-cell RNA-seq atlas of Schistosoma mansoni identifies a key regulator of blood feeding. Science 369, 1644–1649. doi: 10.1126/science.abb7709
Wilson, R. A.. (2012). Proteomics at the schistosome-mammalian host interface: any prospects for diagnostics or vaccines? Parasitology 139, 1178–1194. doi: 10.1017/S0031182012000339
Yu, F., Haynes, S. E., Nesvizhskii, A. I. (2021). IonQuant enables accurate and sensitive label-free quantification with FDR-controlled match-between-runs. Mol. Cell Proteomics 20, 100077. doi: 10.1016/j.mcpro.2021.100077
Zamanian, M., Andersen, E. C. (2016). Prospects and challenges of CRISPR/Cas genome editing for the study and control of neglected vector-borne nematode diseases. FEBS J. 283, 3204–3221. doi: 10.1111/febs.13781
Keywords: Schistosoma, proteomics, secretome, label-free quantification, adults
Citation: Kenney ET, Mann VH, Ittiprasert W, Rosa BA, Mitreva M, Bracken BK, Loukas A, Brindley PJ and Sotillo J (2022) Differential Excretory/Secretory Proteome of the Adult Female and Male Stages of the Human Blood Fluke, Schistosoma mansoni. Front. Parasitol. 1:950744. doi: 10.3389/fpara.2022.950744
Received: 23 May 2022; Accepted: 22 June 2022;
Published: 18 July 2022.
Edited by:
James Anthony Cotton, University of Glasgow, United KingdomReviewed by:
Maude Dagenais, McGill University, CanadaNina Germitsch, University of Prince Edward Island, Canada
Teresa Attenborough, Wellcome Sanger Institute (WT), United Kingdom
Russell M. Morphew, Aberystwyth University, United Kingdom
Copyright © 2022 Kenney, Mann, Ittiprasert, Rosa, Mitreva, Bracken, Loukas, Brindley and Sotillo. This is an open-access article distributed under the terms of the Creative Commons Attribution License (CC BY). The use, distribution or reproduction in other forums is permitted, provided the original author(s) and the copyright owner(s) are credited and that the original publication in this journal is cited, in accordance with accepted academic practice. No use, distribution or reproduction is permitted which does not comply with these terms.
*Correspondence: Javier Sotillo, amF2aWVyLnNvdGlsbG9AaXNjaWlpLmVz; Paul J. Brindley, cGJyaW5kbGV5QGd3dS5lZHU=; Victoria H. Mann, dm1hbm5AZ3d1LmVkdQ==
†These authors have contributed equally to this work