- 1Department of Radiology, Zhongnan Hospital of Wuhan University, Wuhan University, Wuhan, China
- 2Department of Systems Biology, The University of Texas, MD Anderson Cancer Center, Houston, TX, United States
- 3Department of Hematology, Zhongnan Hospital of Wuhan University, Wuhan University, Wuhan, China
- 4Department of Laboratory Medicine, Zhongnan Hospital of Wuhan University, Wuhan University, Wuhan, China
- 5Breast Tumor Center, Sun Yat-Sen Memorial Hospital, Sun Yat-Sen University, Guangzhou, Guangdong, China
C-Jun activation domain-binding protein-1 (Jab1) involves in controlling cellular proliferation, cell cycle, apoptosis, affecting a series of pathways, as well as regulating genomic instability and DNA damage response (DDR). Jab1/COPS5 dysregulation contributes to oncogenesis by deactivating several tumor suppressors and activating oncogenes. Jab1 overexpression was found in many tumor types, illuminating its important role in cancer initiation, progression, and prognosis. Jab1/COPS5 has spurred a strong research interest in developing inhibitors of oncogenes/oncoproteins for cancer therapy. In this paper, we present evidences demonstrating the importance of Jab1/COPS5 overexpression in several cancer types and recent advances in dissecting the Jab1/COPS5 upstream and downstream signaling pathways. By conducting ingenuity pathway analysis (IPA) based on the Ingenuity Knowledge Base, we investigated signaling network that interacts with Jab1/COPS5. The data confirmed the important role of Jab1/COPS5 in tumorigenesis, demonstrating the potential of Jab1/COPS5 to be used as a biomarker for cancer patients, and further support that Jab1/COPS5 may serve as a potential therapeutic target in different cancers.
Introduction
Jab1/COPS5 was initially identified as c-Jun activation domain-binding protein-1 (Jab1) (Claret et al., 1996), a c-Jun coactivator, and subsequently discovered to be the fifth component of the constitutive photomorphogenic-9 signalosome (COPS5, CSN5), a multifunctional protein implicated in regulating a wide variety of cellular and developmental processes, including signal transduction, cells proliferation, cell cycle, apoptosis, DNA damage response (DDR), and tumorigenesis (Lee M. H. et al., 2011). Jab1/COPS5 gene is localized at chromosome 8Q13.2 and encodes Jab1 protein with a molecular mass of 38 kDa. Jab1/COPS5 contains a c-Jun binding domain (JBD), an Mpr1-Pad1-N-terminal (MPN) domain containing the Zn2+-metalloprotease motif (JAMM) that provides the catalytic center to the complex for the CSN isopeptidase activity (Ambroggio et al., 2003; Wei and Deng, 2003; Lingaraju et al., 2014), a nuclear export signal (NES) domain, and a p27 binding domain (PBD) at the C-terminal end. Increasing evidence indicates that dysregulation of Jab1/COPS5, which functionally interacts with several tumor-related genes, such as the cyclin-dependent kinase (CDK) inhibitor 1B p27 (Tomoda et al., 1999; Sui et al., 2001; Esteva et al., 2003), transformation-related phosphoprotein p53 (Zhang et al., 2008), cell signaling protein mothers against decapentaplegic homolog 4/7 (SMAD4/7) (Li et al., 2015), transcriptional coregulatory protein nuclear receptor co-repressor (NcoR) (Lu et al., 2016), Cyclin-dependent kinase inhibitor 1C (p57) (Guo et al., 2016), transmembrane protein Programmed death-ligand 1 (PD-L1) (Lim et al., 2016), and so on, contributes to tumorigenesis. Jab1/COPS5 as an oncogene, is aberrantly overexpressed in various human cancers. However, the mechanism by which Jab1/COPS5 facilitates cancer progression remains largely elusive. Herein, we review advances in understanding the oncogenic role of Jab1/COPS5 in tumorigenesis as well as its therapeutic implications in combating cancer.
Basic Function of Jab1/COPS5
Structure-Based Role of Jab1/COPS5
Binding Protein
Jab1/COPS5 is a key COP9 signalosome (CSN) subunit, which is able to integrate multiple functions of the CSN complex. The CSN was originally characterized as a retardant of plant photomorphogenesis (Wei and Deng, 1992). It contains eight subunits, CSN1 to CSN8 (Gusmaroli et al., 2007). Among them, 6 subunits contain a PCI (proteasome-COP9 signalosome-initiation factor 3) domain that serves as a structure scaffold of the assembly of CSN, while only 2 subunits contain an MPN (MPR1-PAD1-N-terminal) domain (Wei et al., 2008). Jab1/COPS5 contains a nuclear export signal (NES) domain and a p27 binding domain (PBD) at the end of the C-terminal (Shackleford and Claret, 2010). Through the binding of P27 to PBD, Jab1/COPS5 carries p27 protein out of cell nucleus in a Exportin 1 (XPO1)-dependent manner through the NES sequence between amino acids 233 and 242 at its C-terminal end (Shackleford and Claret, 2010; Wang L. et al., 2016).
Isopeptidase Activity
While both Csn5 and Csn6 contain an MPN domain, only Csn5 has an embedded JAMM (JAB1 MPN domain metalloenzyme) motif, which takes effect as the catalytic center for CSN isopeptidase activity (Tran et al., 2003; Wei et al., 2008; Echalier et al., 2013). The MPN domain of Jab1/COPS5 is responsible for regulating the cullin deneddylation process by activating the CRL (Cullin-RING family of ubiquitin ligases) activity (Schwechheimer and Deng, 2001; Wolf et al., 2003; Duda et al., 2008). The MPN domain stands for a pseudo enzyme domain that has been converted into a protein-protein interaction platform which has been confirmed by experimental results (Rosel and Kimmel, 2006; Busch et al., 2007). Adler et al. showed evidence that the isopeptidase activity of Jab1/COPS5 was critical for human and mouse mammary epithelial transformation and progression (Adler et al., 2008).
Jab1/COPS5 Inside or Independently Outside the CSN
Jab1/COPS5 is a pivotal CSN subunit, which is able to integrate various functions of the CSN complex (Chamovitz and Segal, 2001). As a unique subunit of the CSN, COPS5 harbors the catalytic center of the CSN isopeptidase activity and also steadily exists independent of CSN complex in vivo (Wei and Deng, 2003). Jab1/COPS5 energetically takes part in crucial biological activities, both as a part of the CSN complex and independent of the CSN complex. A large portion of Jab1/COPS5 is discovered in free form, which seem to be located in both cytoplasm and nucleus (Wei and Deng, 2003; Wei et al., 2008), while the CSN-associated Jab1/COPS5 is mainly in nuclear. The multi-functionality of Jab1/COPS5 originates from the fact that it exists as a morphon or a sub-complex out of the integral CSN complex, and it was suggested as an individual factor or the central active component of the CSN complex (Kwok et al., 1998; Oron et al., 2002; Sharon et al., 2009). The CSN modulation of the CRL family of ubiquitin E3 complexes depends on its deneddylation function, which is fulfilled by removing NEDD8/Rub1 (an ubiquitin-like molecule) from the cullin subunit of cullin-containing E3 ligases (Cope and Deshaies, 2003; Dubiel, 2009). The JAMM of Jab1/COPS5 seems critical to the deneddylation activity of CSN and is essential for Jab1/COPS5's coactivation of MYC as well as Jab1/COPS5's transformative effects in breast epithelial model which is also proved to be dependent on the whole CSN (Sharon et al., 2009). The CSN is a multi-subunit complex that regulates protein stability by modulating the CRL family and acts as a regulator in several cellular processes, such as gene transcription, cell cycle, and DDR (Cope and Deshaies, 2003; Adler et al., 2006; Wei et al., 2008; Chamovitz, 2009; Kato and Yoneda-Kato, 2009). Other than being a CSN's catalytic center that is required for the deneddylase activity, Jab1/COPS5 alone has no metalloproteinase activity, and other CSN components, or maybe the whole complex, are needed for this deneddylase activity (Cope et al., 2002; Cope and Deshaies, 2003). The nuclear accumulation of Jab1/COPS5 dependent on other CSN components has been clearly demonstrated in the CSN-like complex of budding yeast (Maytal-Kivity et al., 2002). Although Jab1/COPS5 locates in both nuclear and cytoplasmic, whether Jab1/COPS5 acts independently or as part of the CSN complex in cancers needs further investigation (Pan and Claret, 2012).
Jab1/COPS5 Overexpression in Human Cancer
Jab1/COPS5 Overexpressed in Cancer
Cancer is a progressive disease which usually results from genomic instability that could be caused by chromosomal translocations, which leads to aberrant expression of oncogenes, such as Jab1/COPS5 or MYC. Here we searched the GEPIA database (http://gepia.cancer-pku.cn/index.html) to systematically assess the differential expression of Jab1 in a variety of carcinomas. As demonstrated in Figure 1, while Jab1/COPS5 is systemically expressed in both tumor tissues and normal tissues, Jab1/COPS5 expression levels in cancers are significantly higher than those in normal tissues. Actually, Jab1/COPS5 overexpression was reported in many kinds of cancers, including hepatocellular carcinoma (HCC) (Hsu et al., 2008b), pancreatic cancer (Kouvaraki et al., 2006), breast cancer (Kouvaraki et al., 2003), non-small cell lung cancer (NSCLC) (Osoegawa et al., 2006), nasopharyngeal carcinoma (NPC) (Pan et al., 2012), and many others (Pan and Claret, 2012; Pan et al., 2014).
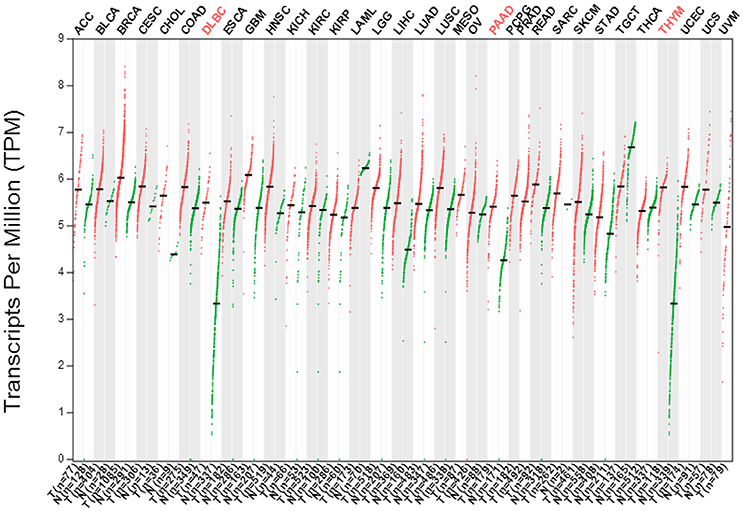
Figure 1. The gene expression profile across all tumor samples (T) and paired normal tissues (N) (Dot plot). Each dot represent expression level of samples. Data was downloaded from GEPIA database (http://gepia.cancer-pku.cn/index.html).
Breast cancer patients without Jab1/COPS5 expression did not recur or progression (Kouvaraki et al., 2003). Besides, Jab1/COPS5 was overexpressed in ovarian tumors and correlated with poor overall survival (Sui et al., 2001). Interestingly, overexpression of Jab1/COPS5 was positively associated with HCV infection and negatively correlated with HBV infection in HCC, indicating a possible mechanism that promotes hepatocarcinogenesis (Hsu et al., 2008b). However, there was no statistically significant relationship between Jab1/COPS5 and clinicopathological parameters or patient survival in intrahepatic cholangiocarcinomas and HCC (Berg et al., 2007; Hashimoto et al., 2009). Among NSCLC, patients with high Jab1/COPS5 expression levels have poor outcomes with a 5-year overall survival rate of 43.9%, compared with 63.1% for patients who have low level of Jab1 expression (Osoegawa et al., 2006). Researchers also showed that Jab1/COPS5 expression was closely linked to histological differentiation, lymph node metastasis, and clinical stage in oral squamous cell carcinoma (Gao et al., 2012). Patients with Jab1/COPS5 overexpression tended to have larger tumor size in thyroid carcinoma and poor overall survival in oral squamous cell carcinoma, NPC, and laryngeal squamous cell carcinomas, indicating its critical role in head and neck cancer (Dong et al., 2005; Ahn et al., 2009; Gao et al., 2012; Xu et al., 2015). Furthermore, Jab1/COPS5 was involved in chemotherapy and radiotherapy resistance (Pan et al., 2013b). Suppression of Jab1/COPS5 made NPC cells more sensitive to cisplatin and ionizing radiation (Pan et al., 2013b). Impressive progress has been made in deciphering the critical role of Jab1/COPS5 in diverse cellular and developmental processes.
Why Jab1/COPS5 Overexpressed in Cancer?
Three regulatory mechanisms may contribute to the dysregulated expression of Jab1/COPS5 in cancer.
First, overexpression of Jab1/COPS5 may originate from gene amplification. The Jab1/COPS5 locus which locates on chromosome 8q13.1, is found to be frequently amplified in human cancers (Dimova et al., 2009; Lu et al., 2016). We did a statistics of DNA alteration frequency of Jab1 in various cancer types on cBioportal database, which is shown in Figure 2. Data downloaded from cBioportal shows that 41.4, 22.9, and 20.7% genetic alterations of Jab1/COPS5 have been recorded in neuroendocrine prostate cancer (NEPC), colorectal adenocarcinoma triplets (MSKCC) and breast cancer, respectively.
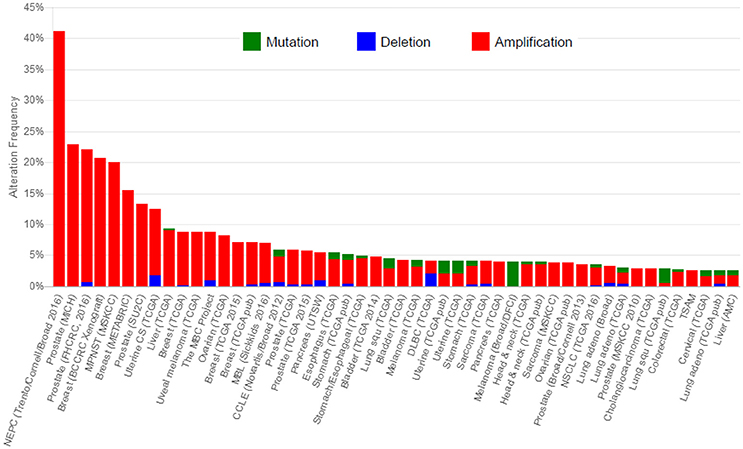
Figure 2. Cross-cancer alteration summary for COPS5. Data was downloaded from cBioportal (http://www.cbioportal.org/).
Second, miRNAs may regulate Jab1 expression. Our group recently, discovered that miR-24 interacted with both the 3′UTR and 5′UTR of Jab1, resulting in Jab1 mRNA degradation and translational suppression. Although miRNAs always target the 3′UTR of the target gene (Lal et al., 2009), miR-24 could target both the 3′UTR and 5′UTR of Jab1 (Wang S. et al., 2016). Furthermore, miR-24 levels inversely associated with Jab1 mRNA and protein levels in both NPC cells and patients (Wang S. et al., 2016). Additionally, Jab1/COPS5 expressed at a higher level in recurrent NPC tissue than the matched primary tissue from the same patients. Jab1/COPS5 overexpression is correlated with a short duration from initial treatment to NPC recurrence (Wang S. et al., 2016). miR-24-Jab1/COPS5 axis represents a novel biomarker for NPC.
Third, other signaling pathways may contribute to Jab1/COPS5 overexpression, such as IL6-Stat3 signaling, HER2-AKT signaling, and Bcr-Abl signaling, which is described below. Moreover, Psoriasin (S100A7), a small calcium-binding protein, enhances Jab1/COPS5 activity as well as AP-1 activity, and promotes tumorigenesis (Emberley et al., 2003).
The mechanism of Jab1/COPS5 dysregulation in cancer patients still needs further exploration, but at least we can conceive that Jab1/COPS5 is a promising biomarker for cancer.
Jab1/COPS5 Associated Signaling Pathways and Targets in Cancer
Jab1/COPS5 lies at the intersection of many signaling pathways that are believed to play important roles in tumorgenesis. Finding out the role of Jab1/COPS5 in these signaling pathways may help us to understand tumorigenesis. Specifically, we performed ingenuity pathway analysis (IPA) based on the Ingenuity Knowledge Base to understand the Jab1/COPS5 related network (Figure 3), we will also discuss Jab1/COPS5 related targets in the following section.
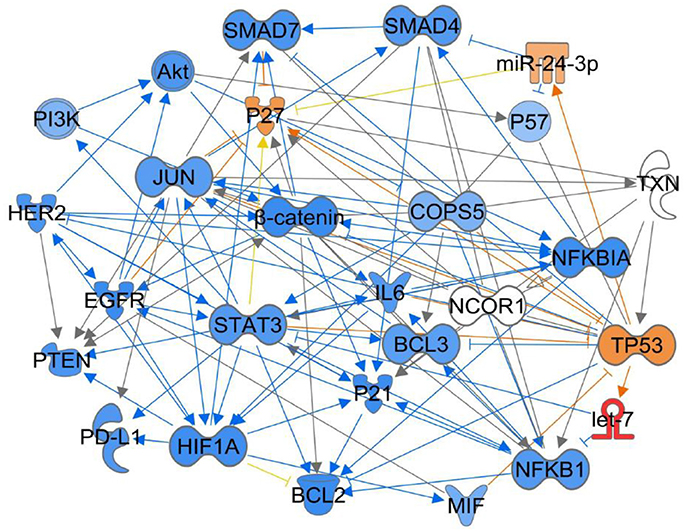
Figure 3. Ingenuity Pathway Analysis (IPA) for Jab1/COPS5 regulation network in cancer. Jab1/COPS5 is a multiple protein and plays an essential role in tumorigenesis. In this case we showed the predicted expression level of other genes in the regulation network in response to increased measured level of let-7 based on the IPA database. The color of each gene represents the corresponding expression level. Light-red means activation and light-blue means inhibition, while red means increased measurement and green means decreased measurement.
Upstream Signaling Pathways
MiR-24-Jab1 Signaling
miR-24 functions as tumor suppressor and radiosensitizer in NPC cells and xenografts by inhibiting Jab1 translation through targeting both the 3′ untranslated region (3′UTR) and 5′UTR of Jab1, leading to tumor growth inhibition, and sensitizes NPC tumors to radiation in vivo (Wang S. et al., 2016). For other predicting miRNAs shown in Figure 4, their associations with cancer are waiting to be investigated.
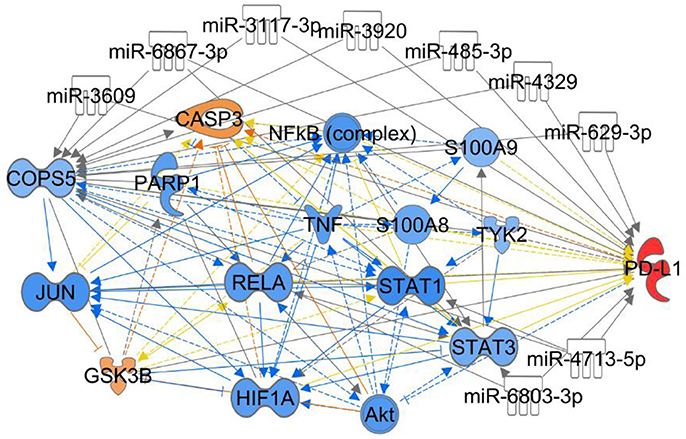
Figure 4. Ingenuity Pathway Analysis for the interaction between Jab1/COPS5 and PD-L1. In this case we showed the predicted expression level of other genes in the regulation network in response to increased measured level of PD-L1 based on the IPA database. The miRNAs in the figure are predicted ones that can target both Jab1/COPS5 and PD-L1, but no demonstration was reported yet. The color of each gene represents the corresponding expression level. Light-red means activation and light-blue means inhibition, while red means increased measurement and green means decreased measurement.
IL6-Stat3 Signaling
Jab1/COPS5 regulates DNA-binding activity of unphosphorylated Stat3 through protein–protein interaction. Knockdown of Jab1/COPS5 significantly induced reduction of unphosphorylated Stat3 DNA-binding activity and Stat3 target genes, but nuclear Stat3 level was found increased in human colon cancer cell (Nishimoto et al., 2013). This non-intuitive phenomenon needs further investigation to discover how Jab1/COPS5 determines the fate of the transcription factors.
Our studies also revealed that Stat3 functions by binding to Jab1/COPS5 promoter, promotes its activity and increases Jab1/COPS5 transcription. Knockdown of Stat3 greatly impairs Jab1/COPS5 promoter activity and decreases Jab1/COPS5 RNA and protein levels (Shackleford et al., 2011; Pan et al., 2017b). Besides, upstream activators of Stat3, such as IL-6 and Src, also promote the activation of Jab1/COPS5 transcription and translation through interacting with Stat3.
HER-2 and EGFR Signaling
It is reported that overexpression of Jab1/COPS5 is associated with human epidermal growth factor receptor-2 (HER-2) levels in breast cancer (Hsu et al., 2008a). HER-2 enhances Jab1/COPS5 promoter activity and increases Jab1/COPS5 expression through AKT/β-catenin pathway (Hsu et al., 2007). Through Inhibition of HER-2 by herceptin, Jab1/COPS5 expression was attenuated in various breast cancer cell lines (Le et al., 2005). In addition, Jab1/COPS5 is also a target in epidermal growth factor receptor (EGFR) pathway. Jab1/COPS5 expression level associates with EGFR level in breast cancer. Activation of EGFR promotes the translocation of Jab1/COPS5 from the cytoplasm to the nucleus and regulates p27 which is a representative gene downstream of Jab1/COPS5 (Wang et al., 2008).
TGF-β Signaling
Jab1/COPS5 affects TGF-β signaling activity by specifically controlling the subcellular localization and degrading two key down-stream targets, Smad4 and Smad7 (Wan et al., 2002; Kim et al., 2004). By binding to Smad4, the receptor-regulated Smad protein (R-Smads) translocates into the nucleus and activates transcription of various target genes. Smad7 inhibits responses mediated by TGF-β and acts in a negative feedback loop to regulate the intensity or duration of the TGF-β signal. Jab1/COPS5 directly binds to Smad4 and degrades it through the proteasome pathway and thus attenuate TGF-β mediated gene transcription, whereas its degradation of Smad7 results in enhanced TGF-β signaling effects.
Wnt Signaling
Jab1/COPS5 controls the Wnt/β-catenin signaling by assisting the formation of a β-catenin-degrading super-complex through deneddylation and simultaneous stabilization of adenomatous polyposis coli (APC) by CSN-associated deubiquitinylase, ubiquitin specific peptidase 15 (USP15) (Huang et al., 2009). Disturbance of the balance between β-catenin and APC can cause cancer by driving cell transformation, tumor angiogenesis and metastasis. Additionally, a clear-cut reduction in β-catenin levels as well as its phosphorylated form has been observed in Jab1/COPS5 knock-down colorectal cancer cells, indicating that Jab1/COPS5 plays an important role in regulating β-catenin and the associated Wnt pathway (Schutz et al., 2012).
NF-κB Signaling
Jab1/COPS5 has also emerged as a regulator of NF-κB signaling by interacting with the proto-oncogene Bcl-3, a member of the Iκ B family that is present predominantly in the nucleus. While most members of the Iκ B family (IκBα, IκBβ, and IκBκ) act to inhibit NF-κB, Bcl-3 on the other hand, can activate NF-κB transcription. Jab1/COPS5 enhances NF-κB p50-Bcl-3-DNA complex formation and coactivates Bcl-3 stimulated NF-κB transcription through interacting with Bcl-3 (Dechend et al., 1999). A distinct cross-talk between NF-κB pathway and CSN-signalosome was then confirmed by the fact that Jab1/COPS5 controls NF-kB activity through CSN-associated deubiquitinase USP15, which causes deubiquitination of IκBα, a negative feedback between degradation of IκBα and activation of NF-κB (Schweitzer et al., 2007). Additionally, Jab1/COPS5 may be regulated by CSN-interacting kinases such as IκB kinase (IKK) (Orel et al., 2010).
MIF-PI3K-AKT Signaling
An indirect mechanism between Jab1/COPS5 and PI3K/AKT pathway has been identified that Jab1/COPS5 inhibits MIF (autocrine macrophage migration inhibitory factor) secretion and its autocrine pro-survival activities and subsequently controls MIF mediated activation of PI3K/AKT signaling (Lue et al., 2007). In contrast, MIF also negatively regulates Jab1/COPS5 by specifically interacting with Jab1/COPS5 and inhibiting the activity of activator protein 1 (AP-1) and c-Jun amino terminal kinase (JNK) (Kleemann et al., 2000).
Bcr-Abl Signaling
Bcr-Abl regulates Jab1/COPS5 by the cooperative interaction with β-catenin and Stat1 in leukemia cells. Suppression of Bcr-Abl by imatinib decreases Jab1/COPS5 expression in Bcr-Abl-positive leukemia cells (Yang et al., 2011). Besides, PI3K/Akt may participate in the regulation of Jab1/COPS5 by Bcr-Abl since PI3K/Akt inhibitor LY294002 decreases the promoter activity and mRNA level of Jab1/COPS5 (Tomoda et al., 2005).
Jab1/COPS5 Related Targets
Jab1/COPS5-p27 Axis
A growing body of evidence has interpreted that overexpression of Jab1/COPS5 is negatively correlated with p27 level and associated with worse prognosis in a variety of human cancers (Shackleford and Claret, 2010). P27 is deemed to be a primary modulator of cell cycle progression (Shackleford and Claret, 2010), and leads to cell cycle arrest in G1 phase and eventually suppress cell proliferation. Jab1/COPS5 directly combines with p27 and shuttles nuclear p27 to cytoplasm through NES (Zhang et al., 2008). Furthermore, p27's cytoplasmic translocalization has been noticed in human cancers and is tightly correlated with poor survival (Guo et al., 1997; Loda et al., 1997; Masciullo et al., 1999). Many studies demonstrated that Jab1/COPS5 increased the p27 nuclear translocation, and subsequent accelerated p27 degradation through the ubiquitin-proteasome pathway (Pan et al., 2012).
Jab1/COPS5- PD-L1 Axis
The association between Jab1/COPS5 and PD-L1 was first reported by Mien-Chie Huang's group. They found that inflammation increases PD-L1 expression in tumors through TNF-α-mediated activation of NF-kB, resulting in transactivation of Jab1/COPS5. Jab1/COPS5 reduces PD-L1 ubiquitination and stabilizes it. Jab1/COPS5 enzyme activity controls T cell suppression via PD-L1. Further, inhibition of Jab1/COPS5 cooperates with anti-CTLA4 to enhance anti-tumor T cell function and decrease tumor growth (Lim et al., 2016). Although, IPA indicates multiple targets involves in Jab1/COPS5 and PD-L1 network (Figure 4), further studies need to be performed to elicit the regulatory mechanism.
Jab1/COPS5- NcoR Axis
Jab1/COPS5 involves in breast cancer progression by regulating a variety of targets. NCoR is a molecular target of Jab1/COPS5 which mediates endocrine-resistance in breast cancer (Lu et al., 2016). Lin Guo et al. observed a remarkable reduction of NcoR protein expression in the tamoxifen-resistant MCF7 clones while Jab1/COPS5 is overexpressed, indicating a functional interaction between Jab1/COPS5 and NcoR complex. It was also confirmed that Jab1/COPS5 binds to NcoR. Intriguingly, while Jab1/COPS5 is significantly over-expressed in refractory tumors, NCoR is under-expressed in tamoxifen-resistant breast tumors. It is also confirmed that overexpression of Jab1/COPS5 degrades NCoR protein through the ubiquitination-proteasome pathway in breast cancer (Lu et al., 2016).
Jab1/COPS5-p57 Axis
P57 is a member of CKIs family that also includes p21 and p27 (Matsuoka et al., 1995). P57 plays a role in cell proliferation, apoptosis, differentiation, and cell migration (Zhang et al., 1997; Matsumoto et al., 2014). Depletion of Jab1 stimulated Smad 2/3 phosphorylation and decreased CDK6 and cyclin D1 (Lee Y. H. et al., 2011). It has also been reported that cyclin D1, Smad, and TGF-β have cross-talk with p57 in cell functions (Zou et al., 2011). Our group recently evaluated Jab1 and p57 levels in HCC and found that Jab1 is inversely correlated with p57 in HCC tissues (Guo et al., 2016). Further studies revealed that Jab1 promotes p57's degradation through 26S proteasome pathway (Guo et al., 2016). We also observed that Jab1 physically interacts with p57 and p57 proteolysis occurred independently of Skp2 and Akt pathways in HCC cells (Guo et al., 2016). Importantly, downregulation of Jab1 sensitized HCC cells to cisplatin (Guo et al., 2016), indicating that Jab1/p57 pathway render chemotherapy resistance and may represent a potential target for treatment of HCC.
Jab1/COPS5-MDM2-p53 Axis
Jab1/COPS5 is a critical modifier for both MDM2 and p53 (Zhang et al., 2008). It was demonstrated that p53's function is lost in almost half of human cancers (Nigro et al., 1989) and that maintaining the stability of p53 is fateful for its tumor suppressor function. Murine double minute 2 (MDM2), a ring domain-containing E3 ubiquitin ligase for anti-oncogene p53, can debase the half-life and stability of p53 through the ubiquitin-proteasome pathway (Iwakuma and Lozano, 2003). It is found that Jab1/COPS5 has a dual effect in decreasing the stability of p53 and facilitating MDM2-mediated p53 degradation. Jab1/COPS5 can also hinder MDM2 mediated self-ubiquitination (Fang et al., 2000), as a consequence, stabilizing MDM2 protein, which in reverse promoted p53 ubiquitination (Zhang et al., 2008). Jab1/COPS5 can implement its biological function by insulating its target proteins, and the most iconic and persuasive example is that Jab1/COPS5 triggers p27 protein translocating from cell nucleus to cytoplasm and subsequently prompts p27 degradation (Tomoda et al., 1999). Jab1/COPS5 is efficient in accelerating p53 nuclear export (Zhang et al., 2008). However, it is not explicit how Jab1/COPS5 can stabilize MDM2.
The above studies have revealed the crosstalk between Jab1/COPS5 and well-known signaling pathways and oncogenes and suggested that Jab1/COPS5 participated in cancer progression. Figure 3 generalizes some of Jab1/COPS5 related signaling pathways in human cancer.
Jab1/COPS5- Bcl2 Axis
The Bcl-2 family of proteins is the key regulator of cell apoptosis at the mitochondria level and predominantly acts as an upstream of irreversible cellular damage in the intrinsic apoptotic pathway (Adams and Cory, 1998; Reed, 1998; Gross et al., 1999). Bcl-2 family contains both proapoptotic and anti-apoptotic members, all of which contain at least one of four conserved Bcl-2 homology (BH) domains, designated BH1–BH4 (Adams and Cory, 1998; Gross et al., 1999). BclGs (Bcl-Gonad short form), originally identified as an alternative mRNA splicing short form of BCL-G gene (Guo et al., 2001), is specifically highly expressed in human testis. Apoptosis induced by BclGs is dependent of the BH3 domain and suppressed by co-expression of anti-apoptotic Bcl-XL/Bcl-2 protein (Guo et al., 2001). Bcl-XL/Bcl-2 was also co-immunoprecipitated with BclGs but not with mutants of BclGs (Guo et al., 2001). Liu X, et al. have screened proteins that interact with BclGs in a human testis cDNA library (Liu et al., 2008) and found that Jab1 can regulate mitochondria apoptosis pathway via its interaction with BclGs. Jab1 binds to the N-terminal region of BclGs (aa 1–67) but not the BH3 domain and competes with Bcl-XL/Bcl-2 to bind to BclGs; thus, promotes apoptosis (Liu et al., 2008).
Bax is a pro-apoptotic member of the Bcl-2 family proteins which serve as critical regulators of pathways involved in apoptosis, acting to either inhibit or promote cell death (Reed, 1998). Bax can be regulated by p53 and show increased expression in certain tissues after apoptotic stimuli (Pena-Blanco and Garcia-Saez, 2017). In contrast with Bax, Bcl-2 is an anti-apoptotic protein. It was demonstrated that knockdown of Jab1 can mediate regulation of Bax and Bcl-2 (Sang et al., 2015). Upregulated of Bax and downregulated of Bcl-2 were observed when Jab1 was knocked down in AGS and MKN45 cells (Sang et al., 2015), which may explain the decreased cell proliferation and the increased apoptosis. Whether the regulation of Bax and Bcl-2 is controlled by the ubiquitin ligase activity or other ways remains to be determined.
Biological Significances of Jab1/COPS5
Jab1/COPS5 in Cell Proliferation and Cell Cycle Progression
Jab1/COPS5 is a major driving force for cell cycle by promoting degradation of several cyclins and CKIs (Shackleford and Claret, 2010; Yoshida et al., 2010). P27 is a universal CKI that directly inhibits the enzymatic activity of cyclin-Cdk complexes, resulting in cell-cycle arrest at G1 phase. Indeed, accumulating evidence has shown the inverse association between Jab1/COPS5 and p27 expression in various human malignancies, including HCC (Berg et al., 2007), NPC (Pan et al., 2012), oral squamous cell carcinomas (Gao et al., 2012) and papillary thyroid carcinoma (Ahn et al., 2009). Jab1/COPS5 negatively regulates p27 expression by exporting p27 from the nucleus to the cytoplasm, mediating p27 degradation via the proteasome pathway and promoting cell-cycle progression (Tomoda et al., 1999). Knockdown of Jab1/COPS5 results in the accumulation of p27 in cell nucleus, induces cell-cycle arrest and inhibits cell proliferation (Pan et al., 2012). It was also reported in a small number of cases that high Jab1/COPS5 expression had no correlation with low p27 expression (Schutz et al., 2012). One possible reason for these discrepancies could be tissue or cell type-specific differences. In addition, other factors, such as the ubiquitin ligase, Skp2, also contributes to the regulation of p27 (Carrano et al., 1999). In fact, regulation of p27 levels is complex, and more detailed functional studies may be warranted to explore this issue further. Expression of many other cell-cycle regulators, such as cyclin D, p16, p21, c-myc, and Bcl-XL (a member of the Bcl-2 family proteins), may also be regulated by Jab1/COPS5 (Shackleford and Claret, 2010). For example, Doronkin et al. demonstrated that depletion of Jab1/COPS5 induced cyclin E stability whereas Jab1/COPS5 overexpression rapidly degraded cyclin E (Doronkin et al., 2003). It is undoubted that Jab1/COPS5 plays critical role in the regulating cell cycle.
Jab1/COPS5 in Apoptosis
Evidence has shown that embryos of Jab1/COPS5-null mice have an elevated p53 levels, indicating this protein is a crucial regulator of p53 stability (Tomoda et al., 2004). In addition, targeted disruption of Jab1/COPS5 in mice leads to early embryonic lethality owing to accelerated apoptosis (Tian et al., 2010). Moreover, Jab1/COPS5 depletion results in more cisplatin-, ionizing radiation (IR)-, or ultraviolet (UV)-induced apoptosis, which was correlated with accumulated p53 in NPC (Pan et al., 2013b). Likewise, Sang et al. have reported that inhibition of Jab1/COPS5 promoted the apoptosis through p53-related apoptotic pathways in gastric cancer cells (Sang et al., 2015).
Jab1/COPS5 is also involved in many other apoptotic related pathways. For example, depletion of Jab1/COPS5 resulted in aberrant expression of the apoptosis-triggering protein Fas ligand in pro-B cells (Sitte et al., 2012). By specifically interacting with Jab1/COPS5, fibronectin type III and ankyrin repeat domains 1 (Fank1) inhibits cell apoptosis by activating the AP-1 induced anti-apoptotic pathway (Wang et al., 2011). In contrast, coexpression of BclGs (Bcl-Gonad short form) and Jab1/COPS5 synergistically promotes apoptosis since Jab1/COPS5 could compete with Bcl-XL/Bcl-2 to interact with BclGs (Liu et al., 2008). Jab1/COPS5 promotes E2F1-dependent induction of apoptosis as an E2F1-specific binding protein. Cells depleted of Jab1/COPS5 are deficient in both E2F1-induced apoptosis and p53 accumulation (Lu et al., 2011).
Jab1/COPS5 in DNA Damage Response
Instability of genome caused by DNA damage can issue in cell cycle arrest and apoptosis. Loss of genomic integrity due to inactivation of DDR genes may induce accumulating gene mutation, which may greatly contributes to cancer development. Increasing evidence suggests that Jab1/COPS5 affects both the activity and stability of proteins involved in DDR (Pan et al., 2014). Jab1/COPS5 is crucial for tumor survival and enhances the resistance of tumor cells to chemotherapy and radiotherapy (Pan et al., 2013b). Importantly, Jab1/COPS5 promotes several DDR-related proteins' nuclear export and degradation. CSN can regulate the ubiquitinligase activity of the damaged DNA binding protein 1 (DDB1) or DDB2 and cockayne syndrome group A complexes (CSA) in response to damaging reagents. Loss of Jab1/COPS5 impaired the repair vitality of DDB2 (Groisman et al., 2003). Besides, Jab1/COPS5 also regulates Cdc10-dependent transcript 1 (CDT1) which is degraded after DNA damage. Jab1/COPS5 deficiency may block the degradation and lead to accumulation of CDT1 (Higa et al., 2003).
The Rad9-Rad1-Hus1 (9-1-1) complex is a DNA-damage sensor and plays an important role in initiating cellular responses to DNA damage. The 9-1-1 complex promotes ATR-mediated phosphorylation and activation of the Chk1, a protein kinase that regulates S-phase progression, G2/M arrest, and replication fork stabilization (Parrilla-Castellar et al., 2004). Jab1/COPS5 is involved in mediating the translocation and regulating the stability of the 9-1-1 complex in cells, which provides novel information about Jab1/COPS5's role in cell cycle checkpoint and DDR.
Rad51, a key DNA-repairing protein, plays an important role in DDR by facilitating production of nucleoprotein filaments and mediating strand conversion between DNA duplexes (Shinohara et al., 1992). Jab1/COPS5 has been shown to interact with Rad51 in homologous recombination (HR) repair pathway (Shinohara et al., 1992). Inhibition of Jab1/COPS5 not only decreases Rad51 level but also impaired its activity, resulting in an increase in cell apoptosis after DNA stimulus. Further studies demonstrated that Jab1/COPS5 regulates Rad51 through p53 pathway (Pan et al., 2013b).
Jab1/COPS5 in Reactive Oxygen Species (ROS) Regulation
Cancer cells generate ROS as a result of stimulation of oncogenes, mitochondrial dysfunction, abnormal metabolism, and the microenvironment. Oncogenic mutations promote aberrant metabolism and protein translation, resulting in increased ROS production. Recent studies have begun to shed light on the ROS involved in modulating biological cell functions, cell signaling, and homeostasis in tumor cells (Shinohara et al., 2014). For example, acute myelocytic leukemia (AML) patients with FMS-like tyrosine kinase 3 (FLT3) mutations have a very high relapse rate (Gaballa et al., 2017), and FLT3 induced elevated levels of ROS (Sallmyr et al., 2008). Increased production of ROS or an inefficient antioxidant system leads to oxidative stress, which influences tumorgenesis. We recently investigated gene expression under oxidative stress in leukemia cell lines and blood samples obtained from AML patients at primary diagnosis and compared those with AML cells from the same patients obtained at relapse. High expression levels of Jab1 and thioredoxin (Trx) were associated with disease progression and poor prognosis in relapsed AML-M5. Mechanistically, Jab1 interacts with and positively regulates Trx expression. Increased ROS level stimulates aberrant gene expression and promotes the proliferation of leukemic blasts. We identified Jab1 as a new target of the ROS pathway that is implicated to have a role in the relapse and progression of malignant AML-M5. Specifically, we demonstrated that Jab1 interacts with and positively regulates Trx expression under oxidative stress (Zhou et al., 2017).
Jab1/COPS5 in Hypoxia
When cells are exposed to hypoxia, they either die or adapt to the hypoxic conditions. Both processes involve upregulation of the transcription factor hypoxia inducible factor-1 (HIF-1). One interesting function of Jab1 is its ability to promote oncogenesis by stabilizing HIF-1α. Importantly, Jab1 has been shown to interact directly with the CODD (C-terminal oxygen-dependent degradation domain) of HIF-1α as well as the pVHL E3 ligase (Bae et al., 2002; Bemis et al., 2004). Overexpression of Jab1 is associated with cancer progression and could reflect the deregulation of aerobic HIF stability, which is thought to be an important transition in tumor progression.
As another possible explanation for HIF-1 stability regulated by Jab1, the association between CSN and HIF-1 could be suggested. Jab1 may serve as a docking site for CSN complex-mediated phosphorylation and subsequently result in stabilizing HIF-1 protein. Additionally, CSN complex phosphorylates p53 at Thr-155 residue, and the phosphorylated p53 is subsequently degraded through ubiquitin-proteasome pathway rapidly (Bech-Otschir et al., 2001), suggesting that p53 degradation by CSN may lead to up-regulation of HIF-1 protein.
While Jab1 promotes HIF-1α stability and transactivation, p53 degrades HIF-1a and decreases its transactivation (Ravi et al., 2000; Hansson et al., 2002). Correspondingly, HIF-1a stabilizes p53, promoting apoptosis in this way (An et al., 1998; Chen et al., 2003). The binding of either Jab1 or p53 may also control the dephosphorylation of HIF-1α during an extended period of hypoxia. Thus we concluded that the interaction of Jab1 with HIF-1a promotes adaptation to hypoxia, in contrast to the interaction of p53 and HIF-1a at the same domain, which promotes apoptosis.
Jab1/COPS5 in Senescence
Cellular senescence is implemented in response to severe cellular insults such as oncogenic activation or chemotherapy and is a failsafe program that protects organismic integrity by excluding potentially harmful cells from further expansion (Narita and Narita, 2017). Senescence has been shown to cancel the pro-tumorigenic potential of Ras-/Raf-driven cancerous lesions (Braig et al., 2005; Michaloglou et al., 2005), and to contribute to the outcome of anticancer chemotherapy in vivo (Michaloglou et al., 2005).
It was found that a significant population of Jab1-depleted cells exhibited a senescence-associated heterochromatin foci (SAHF)-like structure regardless of the absence or presence of p53, indicating that Jab1-depletion initiated a senescence program independently on p53 (Yoshida et al., 2010). The function in the absence of p53 occurred with no significant change in the level of Skp2, which was implicated in the regulation of senescence (Lin et al., 2010). Further studies revealed that depletion of Jab1 inhibited cell proliferation, and induced premature senescence characterized by upregulation of senescence-associated- β-galactosidase activity and increased expression of CDK inhibitors (Tsujimoto et al., 2012). Jab1 was found to interact with CDK2 in vivo and in vitro (Yoshida et al., 2013). Depletion of Jab1 enhanced the phosphorylation of CDK2 by Akt, resulting in cytoplasmic accumulation of CDK2 together with cyclin E. Additional knockdown of CDK2, which reduced the expression of cyclin E to normal level, did not restore cell proliferation, but significantly inhibited senescence in Jab1- deficient cells. Enforced expression of cytoplasmic cyclin E induced premature senescence in immortalized cell lines (Yoshida et al., 2013). These results demonstrate that Jab1 functions through CDK2 to control premature senescence in a novel way (Yoshida et al., 2013).
It will be promising to explore small molecules which inhibit interaction between Jab1 and CDK2 but don't affect the deneddylase activity of Jab1, which may induce senescence without affecting global proliferation and the cell cycle, resulting in the development of anti-cancer drugs with minimum side effects.
Clinical Implication
Efficiency of Jab1/COPS5 in Combination with Immunotherapies
Tumor cells can evade immune surveillance through expression of the inhibitory programmed cell death-ligand 1 (PD-L1) on the cell surface. However, the mechanisms by which PD-L1 is regulated have not been fully elucidated. Recently, researchers have investigated antitumor immunity in mouse model, demonstrating that inflammation promoted tumor growth and enhanced the number of tumor-infiltrating lymphocytes and macrophages, but decreased the cytotoxic activity of T cells. Inflammatory cytokines released by macrophages induced upregulation of PD-L1 protein in breast cancer. Mechanistically, inflammation induced macrophages to secrete TNFα that activated tumor cell NF-κB signaling, allowing the p65 subunit to bind to the promoter and promote transcription of Jab1/COPS5. Jab1/COPS5 encodes a deubiquitinating enzyme that was able to deubiquitinate and to stabilize PD-L1, leading to increased PD-L1 expression in response to inflammatory TNFα signaling. Further, the Jab1/COPS5 inhibitor curcumin blocked TNFα-induced PD-L1 stabilization in various cancer cells. Jab1/COPS5 expression was correlated with expression of PD-L1 in breast cancer. Moreover, increased Jab1/COPS5 expression was correlated with worse survival in breast cancer patients. Curcumin enhanced the therapeutic efficacy of anti-CTLA4 in mice, leading to decreased tumor growth and enhanced survival. Furthermore, curcumin plus anti-CTLA4 was effective in inhibiting tumor growth (Lim et al., 2016). These results suggest that Jab1/COPS5 stabilizes PD-L1 to promote tumor immune evasion and that suppression of Jab1/COPS5 may be effective in combination with immunotherapy.
Therapeutic Role of Jab1 in Cancer
Increasing studies have suggested that Jab1/COPS5 is a novel therapeutic target given its prominent functions in different stages of tumorigenesis. Since Jab1/COPS5 overexpression is usually correlated with poor prognosis, development of Jab1/COPS5-specific inhibitors is likely to have a significant effect on cancer therapy (Table 1).
Curcumin is a yellow plant pigment that has been shown to directly inhibit the activity of CSN associated kinases, making tumor cells arrest at the mitotic phase and prone to apoptosis through the inactivation of CSN (Fullbeck et al., 2005). It has been reported that polyethylene glycosylated curcumin (PEGylated curcumin), a water-soluble compound, inhibited pancreatic cancer cell growth by inhibiting Jab1/COPS5. Importantly, this compound induced sensitivity of pancreatic cancer cells to gemcitabine (Li et al., 2009). A novel curcumin analog T83 also significantly induced G2/M arrest and apoptosis in NPC cells in a dose- and time-dependent manner. In addition, T83 was effective in inhibiting Jab1/COPS5 expression and sensitizing NPC cells to radiotherapy (Pan et al., 2013a).
Another potential target drug is troglitazone which suppress Jab1/COPS5 promoter activity by inhibiting Sp1- and Tcf4-mediated Jab1 transcription (Hsu et al., 2008b). Both in vitro and in vivo studies have shown that troglitazone attenuates tumor growth and up-regulates p27 level effectively in a time- and dose-dependent manner (Motomura et al., 2000; Yu et al., 2006). Animal studies confirmed that troglitazone inhibited hepatocellular carcinima cell growth and decreased Jab1/COPS5 expression in tumor tissues (Hsu et al., 2008b).
As we know, the isopeptidase activity of Jab1/COPS5 resides in the JAMM motif, a zinc metalloproteinase-like domain which can be blocked by Zn2+ chelators (Echalier et al., 2013). Noteworthy, the JAMM motif of Jab1/COPS5 is an attractive therapeutic target because there is a plenty of experience in pharmacologic inhibition of metalloproteinases using small-molecule drugs, including popular antihypertensive drugs such as captopril and benazepril (Nalepa and Wade Harper, 2003). For example, Axel Diernfellner group recently demonstrated that Thiolutin, a disulfide-containing antibiotic and anti-angiogenic compound produced by Streptomyces, inhibits the JAMM metalloproteases of Jab1/COPS5 (Lauinger et al., 2017).
Others have provided insights into peptidomimetic antagonists for Jab1/COPS5 specific inhibitors since the C-terminal tail of one molecule docks in the active site of another one (Echalier et al., 2013). Anita Schlierf and colleague conducted a high-throughput screening campaign using a biochemical assay with recombinant CSN and a fluorescence-labeled and NEDD8-modified CRL substrate. They discovered a potent, selective and orally available Jab1/COPS5 inhibitor CSN5i-3. The compound traps CRLs in the neddylated state, which leads to inactivation of a subset of CRLs by inducing degradation of their substrate recognition module. CSN5i-3 was shown to differentially affect the viability of tumor cell lines and suppress the growth of a human xenograft in mice (Schlierf et al., 2016). These results provide insights into how CSN regulates CRLs and suggest that Jab1/COPS5 inhibition has potential for anti-tumor therapy.
Increasing evidence has supported Jab1/COPS5 as a therapeutic target and a network may exist between chemotherapy and Jab1/COPS5 (Figure 5). However, efforts are still needed to develop effective Jab1/COPS5 specific inhibitors suitable for clinical therapy. The degree, duration, and tissue specificity required for Jab1/COPS5 blockade to inhibit cancer growth need to be deeply explored.
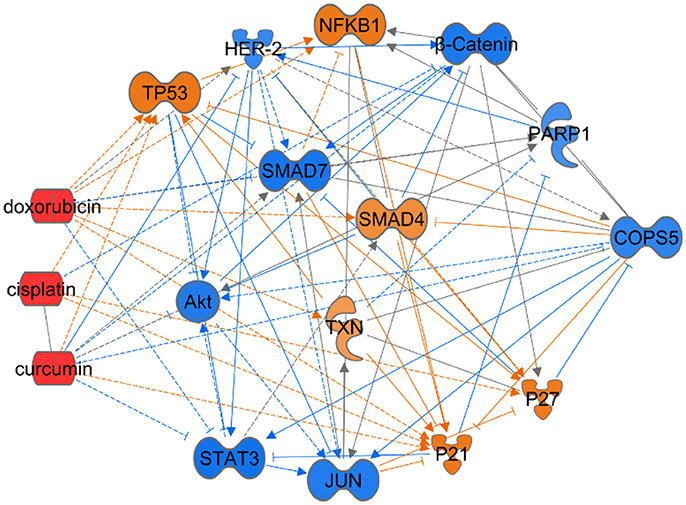
Figure 5. Ingenuity Pathway Analysis to predict the effect of chemical drugs on level of Jab1/COPS5. In this case we showed the predicted expression level of genes in the regulation network in response to increased level of doxorubicin, cisplatin, or curcumin based on the IPA database. The color of each gene represents the corresponding expression level. Light-red means activation and light-blue means inhibition, while red means increased measurement and green means decreased measurement.
Jab1/COPS5 as Biomarker for Diagnostic and Prognosis
Jab1/COPS5 has been observed to overexpressed in many cancers including colon, ovarian, lung and breast cancer, and so on (Pan et al., 2014). Jab1/COPS5 expression is associated with tumor progression and outcomes in many cancer patients. Recently, we retrospectively collected and analyzed data of 88 lung cancer and 76 breast cancer patients, and found that lung cancer patients with higher Jab1 level was less responsive to chemotherapy. Recurrent breast cancer patients had much higher Jab1 level (Hou et al., 2017). Both breast cancer patients and lung cancer patients with higher Jab1 level had significantly shorter disease-free survival and overall survival. In a multivariate survival analysis, Jab1 was correlated with disease-free survival and overall survival in breast cancer (Hou et al., 2017). The Jab1 level was found to be a possible biomarker for clinical response to chemotherapy in lung cancer patients and for postoperative relapse in breast cancer patients who received adjuvant chemotherapy (Hou et al., 2017), indicating that Jab1 predicts treatment response in lung cancer and relapse in breast cancer patients.
Conclusion and Overall Perspectives
In this review, we briefly illustrate the structure and functions of Jab1/COPS5. With its multiple prominent functions, Jab1/COPS5 affects various targets and signaling pathways, which are normally carcinogenic. Jab1/COPS5 has been proved to be associated with therapeutic response and adverse outcome in cancer patients. Although the exact underlying mechanism is inexplicit, it provides a new research direction of anticancer treatment. As a target of anticancer therapy, more further studies of Jab1/COPS5 will promote the development of novel therapeutic strategies against cancer. Considering the pivotal cellular role of Jab1/COPS5, it is reasonable to assume that Jab1/COPS5 is a promising diagnostic and prognostic biomarker for cancer in the future.
Furthermore, we summarized several compounds that could target Jab1 and may act as anti-cancer drugs. Due to non-toxic features, inhibition of Jab1 by natural agents would be a novel and safe approach for cancer therapy. However, further pre-clinical research is urgent to find the right combinations with chemotherapy or radiotherapy toward better treatment of human cancers. We hope this article could promote further studies for the development of specific inhibitors for Jab1 in cancer treatment by either single agent or by a combinational approach. Collectively, targeted inhibition of Jab1 would become a novel strategy for the prevention of tumor progression and successful treatment of human cancers in the future.
Author Contributions
YP: conceived the study; GL and YP: analyzed data and wrote original draft; FZ and FXC: reviewed and edited the manuscript.
Funding
This work was supported by the National Natural Science Foundation of China (81372816), Zhongnan Hospital of Wuhan University Science, Technology and Innovation Seed Fund (ZNPY2017029), the Fundamental Research Funds for the Central Universities, the Natural Science Foundation of Hubei Province.
Conflict of Interest Statement
The authors declare that the research was conducted in the absence of any commercial or financial relationships that could be construed as a potential conflict of interest.
References
Adams, J. M., and Cory, S. (1998). The Bcl-2 protein family: arbiters of cell survival. Science 281, 1322–1326. doi: 10.1126/science.281.5381.1322
Adler, A. S., Lin, M., Horlings, H., Nuyten, D. S., van de Vijver, M. J., and Chang, H. Y. (2006). Genetic regulators of large-scale transcriptional signatures in cancer. Nat. Genet. 38, 421–430. doi: 10.1038/ng1752
Adler, A. S., Littlepage, L. E., Lin, M., Kawahara, T. L., Wong, D. J., Werb, Z., et al. (2008). CSN5 isopeptidase activity links COP9 signalosome activation to breast cancer progression. Cancer Res. 68, 506–515. doi: 10.1158/0008-5472.CAN-07-3060
Ahn, J., Hong, S. A., Lee, S. E., Kim, J., Oh, Y. S., Park, S. J., et al. (2009). Cytoplasmic localization of Jab1 and p27 Kip1 might be associated with invasiveness of papillary thyroid carcinoma. Endocr. J. 56, 707–713. doi: 10.1507/endocrj.K08E-372
Altmann, E., Erbel, P., Renatus, M., Schaefer, M., Schlierf, A., Druet, A., et al. (2017). Azaindoles as zinc-binding small-molecule inhibitors of the JAMM protease CSN5. Angew. Chem. Int. Ed Engl. 56, 1294–1297. doi: 10.1002/anie.201608672
Ambroggio, X. I., Rees, D. C., and Deshaies, R. J. (2003). JAMM: a metalloprotease-like zinc site in the proteasome and signalosome. PLoS Biol. 2:e2. doi: 10.1371/journal.pbio.0020002
An, W. G., Kanekal, M., Simon, M. C., Maltepe, E., Blagosklonny, M. V., and Neckers, L. M. (1998). Stabilization of wild-type p53 by hypoxia-inducible factor 1alpha. Nature 392, 405–408. doi: 10.1038/32925
Bae, M. K., Ahn, M. Y., Jeong, J. W., Bae, M. H., Lee, Y. M., Bae, S. K., et al. (2002). Jab1 interacts directly with HIF-1alpha and regulates its stability. J. Biol. Chem. 277, 9–12. doi: 10.1074/jbc.C100442200
Bech-Otschir, D., Kraft, R., Huang, X., Henklein, P., Kapelari, B., Pollmann, C., et al. (2001). COP9 signalosome-specific phosphorylation targets p53 to degradation by the ubiquitin system. EMBO J. 20, 1630–1639. doi: 10.1093/emboj/20.7.1630
Bemis, L., Chan, D. A., Finkielstein, C. V., Qi, L., Sutphin, P. D., Chen, X., et al. (2004). Distinct aerobic and hypoxic mechanisms of HIF-alpha regulation by CSN5. Genes Dev. 18, 739–744. doi: 10.1101/gad.1180104
Berg, J. P., Zhou, Q., Breuhahn, K., Schirmacher, P., Patil, M. A., Chen, X., et al. (2007). Inverse expression of Jun activation domain binding protein 1 and cell cycle inhibitor p27Kip1: influence on proliferation in hepatocellular carcinoma. Hum. Pathol. 38, 1621–1627. doi: 10.1016/j.humpath.2007.03.007
Braig, M., Lee, S., Loddenkemper, C., Rudolph, C., Peters, A. H., Schlegelberger, B., et al. (2005). Oncogene-induced senescence as an initial barrier in lymphoma development. Nature 436, 660–665. doi: 10.1038/nature03841
Busch, S., Schwier, E. U., Nahlik, K., Bayram, O., Helmstaedt, K., Draht, O. W., et al. (2007). An eight-subunit COP9 signalosome with an intact JAMM motif is required for fungal fruit body formation. Proc. Natl. Acad. Sci. U.S.A. 104, 8089–8094. doi: 10.1073/pnas.0702108104
Carrano, A. C., Eytan, E., Hershko, A., and Pagano, M. (1999). SKP2 is required for ubiquitin-mediated degradation of the CDK inhibitor p27. Nat. Cell Biol. 1, 193–199. doi: 10.1038/12013
Chamovitz, D. A. (2009). Revisiting the COP9 signalosome as a transcriptional regulator. EMBO Rep. 10, 352–358. doi: 10.1038/embor.2009.33
Chamovitz, D. A., and Segal, D. (2001). JAB1/CSN5 and the COP9 signalosome. A complex situation. EMBO Rep. 2, 96–101. doi: 10.1093/embo-reports/kve028
Chen, D., Li, M., Luo, J., and Gu, W. (2003). Direct interactions between HIF-1 alpha and Mdm2 modulate p53 function. J. Biol. Chem. 278, 13595–13598. doi: 10.1074/jbc.C200694200
Claret, F. X., Hibi, M., Dhut, S., Toda, T., and Karin, M. (1996). A new group of conserved coactivators that increase the specificity of AP-1 transcription factors. Nature 383, 453–457. doi: 10.1038/383453a0
Cope, G. A., and Deshaies, R. J. (2003). COP9 signalosome: a multifunctional regulator of SCF and other cullin-based ubiquitin ligases. Cell 114, 663–671. doi: 10.1016/S0092-8674(03)00722-0
Cope, G. A., Suh, G. S., Aravind, L., Schwarz, S. E., Zipursky, S. L., Koonin, E. V., et al. (2002). Role of predicted metalloprotease motif of Jab1/Csn5 in cleavage of Nedd8 from Cul1. Science 298, 608–611. doi: 10.1126/science.1075901
Dechend, R., Hirano, F., Lehmann, K., Heissmeyer, V., Ansieau, S., Wulczyn, F. G., et al. (1999). The Bcl-3 oncoprotein acts as a bridging factor between NF-kappaB/Rel and nuclear co-regulators. Oncogene 18, 3316–3323. doi: 10.1038/sj.onc.1202717
Dimova, I., Orsetti, B., Negre, V., Rouge, C., Ursule, L., Lasorsa, L., et al. (2009). Genomic markers for ovarian cancer at chromosomes 1, 8 and 17 revealed by array CGH analysis. Tumori 95, 357–366.
Dong, Y., Sui, L., Watanabe, Y., Yamaguchi, F., Hatano, N., and Tokuda, M. (2005). Prognostic significance of Jab1 expression in laryngeal squamous cell carcinomas. Clin. Cancer Res. 11, 259–266.
Doronkin, S., Djagaeva, I., and Beckendorf, S. K. (2003). The COP9 signalosome promotes degradation of Cyclin E during early Drosophila oogenesis. Dev. Cell 4, 699–710. doi: 10.1016/S1534-5807(03)00121-7
Dubiel, W. (2009). Resolving the CSN and CAND1 paradoxes. Mol. Cell 35, 547–549. doi: 10.1016/j.molcel.2009.08.011
Duda, D. M., Borg, L. A., Scott, D. C., Hunt, H. W., Hammel, M., and Schulman, B. A. (2008). Structural insights into NEDD8 activation of cullin-RING ligases: conformational control of conjugation. Cell 134, 995–1006. doi: 10.1016/j.cell.2008.07.022
Echalier, A., Pan, Y., Birol, M., Tavernier, N., Pintard, L., Hoh, F., et al. (2013). Insights into the regulation of the human COP9 signalosome catalytic subunit, CSN5/Jab1. Proc. Natl. Acad. Sci. U.S.A. 110, 1273–1278. doi: 10.1073/pnas.1209345110
Emberley, E. D., Niu, Y., Leygue, E., Tomes, L., Gietz, R. D., Murphy, L. C., et al. (2003). Psoriasin interacts with Jab1 and influences breast cancer progression. Cancer Res. 63, 1954–1961.
Esteva, F. J., Sahin, A. A., Rassidakis, G. Z., Yuan, L. X., Smith, T. L., Yang, Y., et al. (2003). Jun activation domain binding protein 1 expression is associated with low p27(Kip1)levels in node-negative breast cancer. Clin. Cancer Res. 9, 5652–5659.
Fang, S., Jensen, J. P., Ludwig, R. L., Vousden, K. H., and Weissman, A. M. (2000). Mdm2 is a RING finger-dependent ubiquitin protein ligase for itself and p53. J. Biol. Chem. 275, 8945–8951. doi: 10.1074/jbc.275.12.8945
Fullbeck, M., Huang, X., Dumdey, R., Frommel, C., Dubiel, W., and Preissner, R. (2005). Novel curcumin- and emodin-related compounds identified by in silico 2D/3D conformer screening induce apoptosis in tumor cells. BMC Cancer 5:97. doi: 10.1186/1471-2407-5-97
Gaballa, S., Saliba, R., Oran, B., Brammer, J. E., Chen, J., Rondon, G., et al. (2017). Relapse risk and survival in patients with FLT3 mutated acute myeloid leukemia undergoing stem cell transplantation. Am. J. Hematol. 92, 331–337. doi: 10.1002/ajh.24632
Gao, L., Huang, S., Ren, W., Zhao, L., Li, J., Zhi, K., et al. (2012). Jun activation domain-binding protein 1 expression in oral squamous cell carcinomas inversely correlates with the cell cycle inhibitor p27. Med. Oncol. 29, 2499–2504. doi: 10.1007/s12032-012-0177-0
Groisman, R., Polanowska, J., Kuraoka, I., Sawada, J., Saijo, M., Drapkin, R., et al. (2003). The ubiquitin ligase activity in the DDB2 and CSA complexes is differentially regulated by the COP9 signalosome in response to DNA damage. Cell 113, 357–367. doi: 10.1016/S0092-8674(03)00316-7
Gross, A., McDonnell, J. M., and Korsmeyer, S. J. (1999). BCL-2 family members and the mitochondria in apoptosis. Genes Dev. 13, 1899–1911. doi: 10.1101/gad.13.15.1899
Guo, B., Godzik, A., and Reed, J. C. (2001). Bcl-G, a novel pro-apoptotic member of the Bcl-2 family. J. Biol. Chem. 276, 2780–2785. doi: 10.1074/jbc.M005889200
Guo, H., Jing, L., Cheng, Y., Atsaves, V., Lv, Y., and Wu, T. (2016). Down-regulation of the cyclin-dependent kinase inhibitor p57 is mediated by Jab1/Csn5 in hepatocarcinogenesis. Hepatology 63, 898–913. doi: 10.1002/hep.28372
Guo, Y., Sklar, G. N., Borkowski, A., and Kyprianou, N. (1997). Loss of the cyclin-dependent kinase inhibitor p27(Kip1) protein in human prostate cancer correlates with tumor grade. Clin. Cancer Res. 3(12 Pt 1), 2269–2274.
Gusmaroli, G., Figueroa, P., Serino, G., and Deng, X. W. (2007). Role of the MPN subunits in COP9 signalosome assembly and activity, and their regulatory interaction with Arabidopsis Cullin3-based E3 ligases. Plant Cell 19, 564–581. doi: 10.1105/tpc.106.047571
Hansson, L. O., Friedler, A., Freund, S., Rudiger, S., and Fersht, A. R. (2002). Two sequence motifs from HIF-1alpha bind to the DNA-binding site of p53. Proc. Natl. Acad. Sci. U.S.A. 99, 10305–10309. doi: 10.1073/pnas.122347199
Hashimoto, N., Yachida, S., Okano, K., Wakabayashi, H., Imaida, K., Kurokohchi, K., et al. (2009). Immunohistochemically detected expression of p27(Kip1) and Skp2 predicts survival in patients with intrahepatic cholangiocarcinomas. Ann. Surg. Oncol. 16, 395–403. doi: 10.1245/s10434-008-0236-0
Higa, L. A., Mihaylov, I. S., Banks, D. P., Zheng, J., and Zhang, H. (2003). Radiation-mediated proteolysis of CDT1 by CUL4-ROC1 and CSN complexes constitutes a new checkpoint. Nat. Cell Biol. 5, 1008–1015. doi: 10.1038/ncb1061
Hou, J., Liu, G., Yuan, Y., Wang, D., Jiao, P., Xing, L., et al. (2017). Increased Jab1/COPS5 is associated with therapeutic response and adverse outcome in lung cancer and breast cancer patients. Oncotarget 8:97504. doi: 10.18632/oncotarget.22146
Hsu, M. C., Chai, C. Y., Hou, M. F., Chang, H. C., Chen, W. T., and Hung, W. C. (2008a). Jab1 is overexpressed in human breast cancer and is a downstream target for HER-2/neu. Mod. Pathol. 21, 609–616. doi: 10.1038/modpathol.2008.23
Hsu, M. C., Chang, H. C., and Hung, W. C. (2007). HER-2/neu transcriptionally activates Jab1 expression via the AKT/beta-catenin pathway in breast cancer cells. Endocr. Relat. Cancer 14, 655–667. doi: 10.1677/ERC-07-0077
Hsu, M. C., Huang, C. C., Chang, H. C., Hu, T. H., and Hung, W. C. (2008b). Overexpression of Jab1 in hepatocellular carcinoma and its inhibition by peroxisome proliferator-activated receptor{gamma} ligands in vitro and in vivo. Clin. Cancer Res. 14, 4045–4052. doi: 10.1158/1078-0432.CCR-07-5040
Huang, X., Langelotz, C., Hetfeld-Pechoc, B. K., Schwenk, W., and Dubiel, W. (2009). The COP9 signalosome mediates beta-catenin degradation by deneddylation and blocks adenomatous polyposis coli destruction via USP15. J. Mol. Biol. 391, 691–702. doi: 10.1016/j.jmb.2009.06.066
Kato, J. Y., and Yoneda-Kato, N. (2009). Mammalian COP9 signalosome. Genes Cells 14, 1209–1225. doi: 10.1111/j.1365-2443.2009.01349.x
Kim, B. C., Lee, H. J., Park, S. H., Lee, S. R., Karpova, T. S., McNally, J. G., et al. (2004). Jab1/CSN5, a component of the COP9 signalosome, regulates transforming growth factor beta signaling by binding to Smad7 and promoting its degradation. Mol. Cell. Biol. 24, 2251–2262.
Kleemann, R., Hausser, A., Geiger, G., Mischke, R., Burger-Kentischer, A., Flieger, O., et al. (2000). Intracellular action of the cytokine MIF to modulate AP-1 activity and the cell cycle through Jab1. Nature 408, 211–216. doi: 10.1038/35041591
Kouvaraki, M. A., Korapati, A. L., Rassidakis, G. Z., Tian, L., Zhang, Q., Chiao, P., et al. (2006). Potential role of Jun activation domain-binding protein 1 as a negative regulator of p27kip1 in pancreatic adenocarcinoma. Cancer Res. 66, 8581–8589. doi: 10.1158/0008-5472.CAN-06-0975
Kouvaraki, M. A., Rassidakis, G. Z., Tian, L., Kumar, R., Kittas, C., and Claret, F. X. (2003). Jun activation domain-binding protein 1 expression in breast cancer inversely correlates with the cell cycle inhibitor p27(Kip1). Cancer Res. 63, 2977–2981.
Kwok, S. F., Solano, R., Tsuge, T., Chamovitz, D. A., Ecker, J. R., Matsui, M., et al. (1998). Arabidopsis homologs of a c-Jun coactivator are present both in monomeric form and in the COP9 complex, and their abundance is differentially affected by the pleiotropic cop/det/fus mutations. Plant Cell 10, 1779–1790. doi: 10.1105/tpc.10.11.1779
Lal, A., Navarro, F., Maher, C. A., Maliszewski, L. E., Yan, N., O'Day, E., et al. (2009). miR-24 Inhibits cell proliferation by targeting E2F2, MYC, and other cell-cycle genes via binding to “seedless” 3'UTR microRNA recognition elements. Mol. Cell 35, 610–625. doi: 10.1016/j.molcel.2009.08.020
Lauinger, L., Li, J., Shostak, A., Cemel, I. A., Ha, N., Zhang, Y., et al. (2017). Thiolutin is a zinc chelator that inhibits the Rpn11 and other JAMM metalloproteases. Nat. Chem. Biol. 13, 709–714. doi: 10.1038/nchembio.2370
Le, X. F., Pruefer, F., and Bast, R. C., and Jr. (2005). HER2-targeting antibodies modulate the cyclin-dependent kinase inhibitor p27Kip1 via multiple signaling pathways. Cell Cycle 4, 87–95. doi: 10.4161/cc.4.1.1360
Lee, M. H., Zhao, R., Phan, L., and Yeung, S. C. (2011). Roles of COP9 signalosome in cancer. Cell Cycle 10, 3057–3066. doi: 10.4161/cc.10.18.17320
Lee, Y. H., Judge, A. D., Seo, D., Kitade, M., Gomez-Quiroz, L. E., Ishikawa, T., et al. (2011). Molecular targeting of CSN5 in human hepatocellular carcinoma a mechanism of therapeutic response. Oncogene 30, 4175–4184. doi: 10.1038/onc.2011.126
Li, J., Gu, Z., Li, S., Xiao, Z., and Sun, K. (2015). Reverse correlation of Jab1 and Smad4 in PANC-1 cells involved in the pathogenesis of pancreatic cancer. Int. J. Clin. Exp. Pathol. 8, 9279–9285.
Li, J., Wang, Y., Yang, C., Wang, P., Oelschlager, D. K., Zheng, Y., et al. (2009). Polyethylene glycosylated curcumin conjugate inhibits pancreatic cancer cell growth through inactivation of Jab1. Mol. Pharmacol. 76, 81–90. doi: 10.1124/mol.109.054551
Lim, S. O., Li, C. W., Xia, W., Cha, J. H., Chan, L. C., Wu, Y., et al. (2016). Deubiquitination and Stabilization of PD-L1 by CSN5. Cancer Cell 30, 925–939. doi: 10.1016/j.ccell.2016.10.010
Lin, H. K., Chen, Z., Wang, G., Nardella, C., Lee, S. W., Chan, C. H., et al. (2010). Skp2 targeting suppresses tumorigenesis by Arf-p53-independent cellular senescence. Nature 464, 374–379. doi: 10.1038/nature08815
Lingaraju, G. M., Bunker, R. D., Cavadini, S., Hess, D., Hassiepen, U., Renatus, M., et al. (2014). Crystal structure of the human COP9 signalosome. Nature 512, 161–165. doi: 10.1038/nature13566
Liu, X., Pan, Z., Zhang, L., Sun, Q., Wan, J., Tian, C., et al. (2008). JAB1 accelerates mitochondrial apoptosis by interaction with proapoptotic BclGs. Cell. Signal. 20, 230–240. doi: 10.1016/j.cellsig.2007.10.012
Loda, M., Cukor, B., Tam, S. W., Lavin, P., Fiorentino, M., Draetta, G. F., et al. (1997). Increased proteasome-dependent degradation of the cyclin-dependent kinase inhibitor p27 in aggressive colorectal carcinomas. Nat. Med. 3, 231–234. doi: 10.1038/nm0297-231
Lu, H., Liang, X., Issaenko, O. A., and Hallstrom, T. C. (2011). Jab1/CSN5 mediates E2F dependent expression of mitotic and apoptotic but not DNA replication targets. Cell Cycle 10, 3317–3326. doi: 10.4161/cc.10.19.17618
Lu, R., Hu, X., Zhou, J., Sun, J., Zhu, A. Z., Xu, X., et al. (2016). COPS5 amplification and overexpression confers tamoxifen-resistance in ERalpha-positive breast cancer by degradation of NCoR. Nat. Commun. 7:12044. doi: 10.1038/ncomms12044
Lue, H., Thiele, M., Franz, J., Dahl, E., Speckgens, S., Leng, L., et al. (2007). Macrophage migration inhibitory factor (MIF) promotes cell survival by activation of the Akt pathway and role for CSN5/JAB1 in the control of autocrine MIF activity. Oncogene 26, 5046–5059. doi: 10.1038/sj.onc.1210318
Masciullo, V., Sgambato, A., Pacilio, C., Pucci, B., Ferrandina, G., Palazzo, J., et al. (1999). Frequent loss of expression of the cyclin-dependent kinase inhibitor p27 in epithelial ovarian cancer. Cancer Res. 59, 3790–3794.
Matsumoto, A., Takeishi, S., and Nakayama, K. I. (2014). p57 regulates T-cell development and prevents lymphomagenesis by balancing p53 activity and pre-TCR signaling. Blood 123, 3429–3439. doi: 10.1182/blood-2013-10-532390
Matsuoka, S., Edwards, M. C., Bai, C., Parker, S., Zhang, P., Baldini, A., et al. (1995). p57KIP2, a structurally distinct member of the p21CIP1 Cdk inhibitor family, is a candidate tumor suppressor gene. Genes Dev. 9, 650–662. doi: 10.1101/gad.9.6.650
Maytal-Kivity, V., Piran, R., Pick, E., Hofmann, K., and Glickman, M. H. (2002). COP9 signalosome components play a role in the mating pheromone response of S. cerevisiae. EMBO Rep. 3, 1215–1221. doi: 10.1093/embo-reports/kvf235
Michaloglou, C., Vredeveld, L. C., Soengas, M. S., Denoyelle, C., Kuilman, T., van der Horst, C. M., et al. (2005). BRAFE600-associated senescence-like cell cycle arrest of human naevi. Nature 436, 720–724. doi: 10.1038/nature03890
Motomura, W., Okumura, T., Takahashi, N., Obara, T., and Kohgo, Y. (2000). Activation of peroxisome proliferator-activated receptor gamma by troglitazone inhibits cell growth through the increase of p27KiP1 in human. Pancreatic carcinoma cells. Cancer Res. 60, 5558–5564.
Nalepa, G., and Wade Harper, J. (2003). Therapeutic anti-cancer targets upstream of the proteasome. Cancer Treat. Rev. 29 (Suppl.1), 49–57. doi: 10.1016/S0305-7372(03)00083-5
Narita, M., and Narita, M. (2017). Autophagy detection during oncogene-induced senescence using fluorescence microscopy. Methods Mol. Biol. 1534, 89–98. doi: 10.1007/978-1-4939-6670-7_8
Nigro, J. M., Baker, S. J., Preisinger, A. C., Jessup, J. M., Hostetter, R., Cleary, K., et al. (1989). Mutations in the p53 gene occur in diverse human tumour types. Nature 342, 705–708. doi: 10.1038/342705a0
Nishimoto, A., Kugimiya, N., Hosoyama, T., Enoki, T., Li, T. S., and Hamano, K. (2013). JAB1 regulates unphosphorylated STAT3 DNA-binding activity through protein-protein interaction in human colon cancer cells. Biochem. Biophys. Res. Commun. 438, 513–518. doi: 10.1016/j.bbrc.2013.07.105
Orel, L., Neumeier, H., Hochrainer, K., Binder, B. R., and Schmid, J. A. (2010). Crosstalk between the NF-κB activating IKK-complex and the CSN signalosome. J. Cell. Mol. Med. 14, 1555–1568. doi: 10.1111/j.1582-4934.2009.00866.x
Oron, E., Mannervik, M., Rencus, S., Harari-Steinberg, O., Neuman-Silberberg, S., Segal, D., et al. (2002). COP9 signalosome subunits 4 and 5 regulate multiple pleiotropic pathways in Drosophila melanogaster. Development 129, 4399–4409.
Osoegawa, A., Yoshino, I., Kometani, T., Yamaguchi, M., Kameyama, T., Yohena, T., et al. (2006). Overexpression of Jun activation domain-binding protein 1 in nonsmall cell lung cancer and its significance in p27 expression and clinical features. Cancer 107, 154–161. doi: 10.1002/cncr.21961
Pan, Y., and Claret, F. X. (2012). Targeting Jab1/CSN5 in nasopharyngeal carcinoma. Cancer Lett. 326, 155–160. doi: 10.1016/j.canlet.2012.07.033
Pan, Y., Liu, D., Wei, Y., Su, D., Lu, C., Hu, Y., et al. (2017a). Azelaic acid exerts antileukemic activity in acute myeloid leukemia. Front. Pharmacol. 8:359. doi: 10.3389/fphar.2017.00359
Pan, Y., Wang, M., Bu, X., Zuo, Y., Wang, S., Wang, D., et al. (2013a). Curcumin analogue T83 exhibits potent antitumor activity and induces radiosensitivity through inactivation of Jab1 in nasopharyngeal carcinoma. BMC Cancer 13:323. doi: 10.1186/1471-2407-13-323
Pan, Y., Wang, S., Su, B., Zhou, F., Zhang, R., Xu, T., et al. (2017b). Stat3 contributes to cancer progression by regulating Jab1/Csn5 expression. Oncogene 36, 1069–1079. doi: 10.1038/onc.2016.271
Pan, Y., Yang, H., and Claret, F. X. (2014). Emerging roles of Jab1/CSN5 in DNA damage response, DNA repair, and cancer. Cancer Biol. Ther. 15, 256–262. doi: 10.4161/cbt.27823
Pan, Y., Zhang, Q., Atsaves, V., Yang, H., and Claret, F. X. (2013b). Suppression of Jab1/CSN5 induces radio- and chemo-sensitivity in nasopharyngeal carcinoma through changes to the DNA damage and repair pathways. Oncogene 32, 2756–2766. doi: 10.1038/onc.2012.294
Pan, Y., Zhang, Q., Tian, L., Wang, X., Fan, X., Zhang, H., et al. (2012). Jab1/CSN5 negatively regulates p27 and plays a role in the pathogenesis of nasopharyngeal carcinoma. Cancer Res. 72, 1890–1900. doi: 10.1158/0008-5472.CAN-11-3472
Parrilla-Castellar, E. R., Arlander, S. J., and Karnitz, L. (2004). Dial 9-1-1 for DNA damage the Rad9-Hus1-Rad1 (9-1-1) clamp complex. DNA Repair. 3, 1009–1014. doi: 10.1016/j.dnarep.2004.03.032
Pena-Blanco, A., and Garcia-Saez, A. J. (2017). Bax, Bak and beyond - mitochondrial performance in apoptosis. FEBS J. 285, 416–431. doi: 10.1111/febs.14186
Ravi, R., Mookerjee, B., Bhujwalla, Z. M., Sutter, C. H., Artemov, D., Zeng, Q., et al. (2000). Regulation of tumor angiogenesis by p53-induced degradation of hypoxia-inducible factor 1alpha. Genes Dev. 14, 34–44.
Rosel, D., and Kimmel, A. R. (2006). The COP9 signalosome regulates cell proliferation of Dictyostelium discoideum. Eur. J. Cell Biol. 85, 1023–1034. doi: 10.1016/j.ejcb.2006.04.006
Sallmyr, A., Fan, J., Datta, K., Kim, K. T., Grosu, D., Shapiro, P., et al. (2008). Internal tandem duplication of FLT3 (FLT3/ITD) induces increased ROS production, DNA damage, and misrepair implications for poor prognosis in AML. Blood 111, 3173–3182. doi: 10.1182/blood-2007-05-092510
Sang, M. M., Du, W. Q., Zhang, R. Y., Zheng, J. N., and Pei, D. S. (2015). Suppression of CSN5 promotes the apoptosis of gastric cancer cells through regulating p53-related apoptotic pathways. Bioorg. Med. Chem. Lett. 25, 2897–2901. doi: 10.1016/j.bmcl.2015.05.057
Schlierf, A., Altmann, E., Quancard, J., Jefferson, A. B., Assenberg, R., Renatus, M., et al. (2016). Targeted inhibition of the COP9 signalosome for treatment of cancer. Nat. Commun. 7:13166. doi: 10.1038/ncomms13166
Schutz, A. K., Hennes, T., Jumpertz, S., Fuchs, S., and Bernhagen, J. (2012). Role of CSN5/JAB1 in Wnt/beta-catenin activation in colorectal cancer cells. FEBS Lett. 586, 1645–1651. doi: 10.1016/j.febslet.2012.04.037
Schwechheimer, C., and Deng, X. W. (2001). COP9 signalosome revisited: a novel mediator of protein degradation. Trends Cell Biol. 11, 420–426. doi: 10.1016/S0962-8924(01)02091-8
Schweitzer, K., Bozko, P. M., Dubiel, W., and Naumann, M. (2007). CSN controls NF-kappaB by deubiquitinylation of IkappaBalpha. EMBO J. 26, 1532–1541. doi: 10.1038/sj.emboj.7601600
Shackleford, T. J., and Claret, F. X. (2010). JAB1/CSN5: a new player in cell cycle control and cancer. Cell Div. 5:26. doi: 10.1186/1747-1028-5-26
Shackleford, T. J., Zhang, Q., Tian, L., Vu, T. T., Korapati, A. L., Baumgartner, A. M., et al. (2011). Stat3 and CCAAT/enhancer binding protein beta (C/EBP-beta) regulate Jab1/CSN5 expression in mammary carcinoma cells. Breast Cancer Res. 13:R65. doi: 10.1186/bcr2902
Sharon, M., Mao, H., Boeri Erba, E., Stephens, E., Zheng, N., and Robinson, C. V. (2009). Symmetrical modularity of the COP9 signalosome complex suggests its multifunctionality. Structure 17, 31–40. doi: 10.1016/j.str.2008.10.012
Shinohara, A., Imai, Y., Nakagawa, M., Takahashi, T., Ichikawa, M., and Kurokawa, M. (2014). Intracellular reactive oxygen species mark and influence the megakaryocyte-erythrocyte progenitor fate of common myeloid progenitors. Stem Cells 32, 548–557. doi: 10.1002/stem.1588
Shinohara, A., Ogawa, H., and Ogawa, T. (1992). Rad51 protein involved in repair and recombination in S. cerevisiae is a RecA-like protein. Cell 69, 457–470. doi: 10.1016/0092-8674(92)90447-K
Sitte, S., Glasner, J., Jellusova, J., Weisel, F., Panattoni, M., Pardi, R., et al. (2012). JAB1 is essential for B cell development and germinal center formation and inversely regulates Fas ligand and Bcl6 expression. J. Immunol. 188, 2677–2686. doi: 10.4049/jimmunol.1101455
Sui, L., Dong, Y., Ohno, M., Watanabe, Y., Sugimoto, K., Tai, Y., et al. (2001). Jab1 expression is associated with inverse expression of p27(kip1) and poor prognosis in epithelial ovarian tumors. Clin. Cancer Res. 7, 4130–4135.
Tian, L., Peng, G., Parant, J. M., Leventaki, V., Drakos, E., Zhang, Q., et al. (2010). Essential roles of Jab1 in cell survival, spontaneous DNA damage and DNA repair. Oncogene 29, 6125–6137. doi: 10.1038/onc.2010.345
Tomoda, K., Kato, J. Y., Tatsumi, E., Takahashi, T., Matsuo, Y., and Yoneda-Kato, N. (2005). The Jab1/COP9 signalosome subcomplex is a downstream mediator of Bcr-Abl kinase activity and facilitates cell-cycle progression. Blood 105, 775–783. doi: 10.1182/blood-2004-04-1242
Tomoda, K., Kubota, Y., and Kato, J. (1999). Degradation of the cyclin-dependent-kinase inhibitor p27Kip1 is instigated by Jab1. Nature 398, 160–165. doi: 10.1038/18230
Tomoda, K., Yoneda-Kato, N., Fukumoto, A., Yamanaka, S., and Kato, J. Y. (2004). Multiple functions of Jab1 are required for early embryonic development and growth potential in mice. J. Biol. Chem. 279, 43013–43018. doi: 10.1074/jbc.M406559200
Tran, H. J., Allen, M. D., Lowe, J., and Bycroft, M. (2003). Structure of the Jab1/MPN domain and its implications for proteasome function. Biochemistry 42, 11460–11465. doi: 10.1021/bi035033g
Tsujimoto, I., Yoshida, A., Yoneda-Kato, N., and Kato, J. Y. (2012). Depletion of CSN5 inhibits Ras-mediated tumorigenesis by inducing premature senescence in p53-null cells. FEBS Lett. 586 4326–4331. doi: 10.1016/j.febslet.2012.10.042
Wan, M., Cao, X., Wu, Y., Bai, S., Wu, L., Shi, X., et al. (2002). Jab1 antagonizes TGF-beta signaling by inducing Smad4 degradation. EMBO reports 3, 171–176. doi: 10.1093/embo-reports/kvf024
Wang, H., Song, W., Hu, T., Zhang, N., Miao, S., Zong, S., et al. (2011). Fank1 interacts with Jab1 and regulates cell apoptosis via the AP-1 pathway. Cell. Mol. Life Sci. 68, 2129–2139. doi: 10.1007/s00018-010-0559-4
Wang, J., Barnes, R. O., West, N. R., Olson, M., Chu, J. E., and Watson, P. H. (2008). Jab1 is a target of EGFR signaling in ERalpha-negative breast cancer. Breast Cancer Research 10:R51. doi: 10.1186/bcr2105
Wang, L., Zheng, J.-N., and Pei, D.-S. (2016). The emerging roles of Jab1/CSN5 in cancer. Med. Oncol. 33, 1–11. doi: 10.1007/s12032-016-0805-1
Wang, S., Pan, Y., Zhang, R., Xu, T., Wu, W., Wang, C., et al. (2016). Hsa-miR-24-3p increases nasopharyngeal carcinoma radiosensitivity by targeting both the 3′ UTR and 5′ UTR of Jab1/CSN5. Oncogene 35, 6096–6108. doi: 10.1038/onc.2016.147
Wei, N., and Deng, X. W. (1992). COP9: a new genetic locus involved in light-regulated development and gene expression in Arabidopsis. Plant cell 4, 1507–1518. doi: 10.1105/tpc.4.12.1507
Wei, N., and Deng, X. W. (2003). The COP9 signalosome. Annu. Rev. Cell Dev. Biol. 19, 261–286. doi: 10.1146/annurev.cellbio.19.111301.112449
Wei, N., Serino, G., and Deng, X. W. (2008). The COP9 signalosome: more than a protease. Trends Biochem. Sci. 33, 592–600. doi: 10.1016/j.tibs.2008.09.004
Wolf, D. A., Zhou, C., and Wee, S. (2003). The COP9 signalosome: an assembly and maintenance platform for cullin ubiquitin ligases? Nat. Cell Biol. 5, 1029–1033. doi: 10.1038/ncb1203-1029
Xu, T., Su, B., Wang, C., Wang, S., Huang, H., Pan, Y., et al. (2015). Molecular markers to assess short-term disease local recurrence in nasopharyngeal carcinoma. Oncol. Rep. 33, 1418–1426. doi: 10.3892/or.2015.3739
Yang, K. T., Wang, M. C., Chen, J. Y., Hsu, M. C., and Hung, W. C. (2011). Bcr-Abl oncogene stimulates Jab1 expression via cooperative interaction of beta-catenin and STAT1 in chronic myeloid leukemia cells. J. Cell. Physiol. 226, 2849–2856. doi: 10.1002/jcp.22633
Yoshida, A., Yoneda-Kato, N., and Kato, J. Y. (2013). CSN5 specifically interacts with CDK2 and controls senescence in a cytoplasmic cyclin E-mediated manner. Sci. Rep. 3:1054. doi: 10.1038/srep01054
Yoshida, A., Yoneda-Kato, N., Panattoni, M., Pardi, R., and Kato, J. Y. (2010). CSN5/Jab1 controls multiple events in the mammalian cell cycle. FEBS Lett. 584, 4545–4552. doi: 10.1016/j.febslet.2010.10.039
Yu, J., Qiao, L., Zimmermann, L., Ebert, M. P., Zhang, H., Lin, W., et al. (2006). Troglitazone inhibits tumor growth in hepatocellular carcinoma in vitro and in vivo. Hepatology 43, 134–143. doi: 10.1002/hep.20994
Zhang, P., Liegeois, N. J., Wong, C., Finegold, M., Hou, H., Thompson, J. C., et al. (1997). Altered cell differentiation and proliferation in mice lacking p57KIP2 indicates a role in Beckwith-Wiedemann syndrome. Nature 387, 151–158. doi: 10.1038/387151a0
Zhang, X. C., Chen, J., Su, C. H., Yang, H. Y., and Lee, M. H. (2008). Roles for CSN5 in control of p53/MDM2 activities. J. Cell. Biochem. 103, 1219–1230. doi: 10.1002/jcb.21504
Zhou, F., Pan, Y., Wei, Y., Zhang, R., Bai, G., Shen, Q., et al. (2017). Jab1/Csn5-thioredoxin signaling in relapsed acute monocytic leukemia under oxidative stress. Clin. Cancer Res. 23, 4450–4461. doi: 10.1158/1078-0432.CCR-16-2426
Keywords: Jab1, biomarker, prognostic marker, therapeutic target, COPS5
Citation: Liu G, Claret FX, Zhou F and Pan Y (2018) Jab1/COPS5 as a Novel Biomarker for Diagnosis, Prognosis, Therapy Prediction and Therapeutic Tools for Human Cancer. Front. Pharmacol. 9:135. doi: 10.3389/fphar.2018.00135
Received: 17 November 2017; Accepted: 07 February 2018;
Published: 27 February 2018.
Edited by:
Zhi Sheng, Virginia Tech, United StatesReviewed by:
Paul B. Fisher, Virginia Commonwealth University, United StatesHarikumar K. B., Rajiv Gandhi Centre for Biotechnology, India
Copyright © 2018 Liu, Claret, Zhou and Pan. This is an open-access article distributed under the terms of the Creative Commons Attribution License (CC BY). The use, distribution or reproduction in other forums is permitted, provided the original author(s) and the copyright owner are credited and that the original publication in this journal is cited, in accordance with accepted academic practice. No use, distribution or reproduction is permitted which does not comply with these terms.
*Correspondence: Yunbao Pan, panyunbao@outlook.com